- 1BENFRA Botanical Dietary Supplements Research Center, Oregon Health & Science University, Portland, OR, United States
- 2Department of Neurology, Oregon Health & Science University, Portland, OR, United States
- 3Department of Chemistry, Oregon State University, Corvallis, OR, United States
- 4Oregon’s Wild Harvest, Redmond, OR, United States
- 5School of Public Health, Oregon Health & Science University-Portland State University, Portland, OR, United States
- 6Department of Behavioral Neuroscience, Oregon Health & Science University, Portland, OR, United States
- 7Parkinson’s Disease Research Education and Clinical Care Center, Veterans’ Administration Portland Healthcare System, Portland, OR, United States
- 8Linus Pauling Institute, Oregon State University, Corvallis, OR, United States
Background: A water extract (CAW) of the Ayurvedic plant Centella asiatica administered in drinking water has been shown to improve cognitive deficits in mouse models of aging and neurodegenerative diseases. Here the effects of CAW administered in drinking water or the diet on cognition, measures of anxiety and depression-like behavior in healthy aged mice are compared.
Methods: Three- and eighteen-month-old male and female C57BL6 mice were administered rodent AIN-93M diet containing CAW (0, 0.2, 0.5 or 1% w/w) to provide 0, 200 mg/kg/d, 500 mg/kg/d or 1,000 mg/kg/d CAW for a total of 5 weeks. An additional group of eighteen-month-old mice were treated with CAW (10 mg/mL) in their drinking water CAW for a total of 5 weeks to deliver the same exposure of CAW as the highest dietary dose (1,000 mg/kg/d). CAW doses delivered were calculated based on food and water consumption measured in previous experiments. In the fourth and fifth weeks, mice underwent behavioral testing of cognition, anxiety and depression (n = 12 of each sex per treatment group in each test).
Results: Aged mice of both sexes showed cognitive deficits relative to young mice while only female aged mice showed increased anxiety compared to the young female mice and no differences in depression were observed between the different ages. CAW (1,000 mg/kg/d) in the drinking water improved deficits in aged mice in learning, executive function and recognition memory in both sexes and attenuated the increased measures of anxiety observed in the aged female mice. However, CAW in the diet only improved executive function in aged mice at the highest dose (1,000 mg/kg/d) in both sexes and did so less robustly than when given in the water. There were no effects of CAW on depression-like behavior in aged animals regardless of whether it was administered in the diet or the water.
Conclusions: These results suggest that CAW can ameliorate age-related changes in measures of anxiety and cognition and that the mode of administration is important for the effects of CAW on resilience to these age-related changes.
Introduction
The proportion of people aged 65 and older in the United States (US) was estimated at 16.8% in 2020 and that number is predicted to be over 20%, or about 65 million people, by the year 2040 (Institute, 2020; Aging Ao, 2021). By 2030, older people are projected to outnumber children in the US for the first time in history (Vespa et al., 2020). Aging is associated with a variety of multi-system changes that significantly affect quality of life. Alterations in cognitive function and mood are two such changes that can have a significant impact on the lives of elderly people. A majority of elderly individuals experience some form of memory loss that affects their activities of daily life (Murman, 2015). These include episodic and source memory deficits (Cansino, 2009; Johnson et al., 2016; Park et al., 2017), decreased sensitivity to novelty (Fandakova et al., 2014) and impairment in executive function tasks such as attention, planning, inhibitory control and cognitive flexibility (Park et al., 2002; Buckner, 2004; Methqal et al., 2017). Changes in mood are also widespread in the aging population. It is estimated that 29% of people over the age of 55 experience some type of mental health concern, among which anxiety and depression are most common (CfDCaPaNAoCD, 2008). The proportion of older adults with depressive symptoms increases with age (Services UDoHaH, 1999) and the prevalence of anxiety disorders is estimated to be as high as 17% in the elderly population. Despite the prevalence of these age-related changes, therapeutic options remain limited, which has led many in the elderly population to seek out alternative therapies. It is estimated that about 60% of older individuals in the US have used at least one complementary and alternative medicine in the last year (Golden et al., 2023). In particular, botanical interventions are popular as they have been purported to promote resilience to age-related changes (Nascimento et al., 2016; Ward and Pasinetti, 2016).
Centella asiatica (CA) is an edible plant used in traditional Chinese and Ayurvedic medicine to improve brain function and boost memory (Kapoor, 1990; Shinomol and Muralidhara, 2011) and is marketed in the United States as the dietary supplement “gotu kola” (Newall et al., 1996). CA has many reported neurobehavioral effects, including anxiolytic, anti-depressant and cognitive enhancing properties. Anxiolytic and anti-depressant-like effects of the plant have been observed in healthy rodents as well as multiple models of stress including olfactory bulbectomy, chronic immobilization stress and sleep deprivation-induced stress (Wijeweera et al., 2006; Kalshetty et al., 2012; Selvi et al., 2012; Wanasuntronwong et al., 2012; Chanana and Kumar, 2016; Jagadeesan et al., 2019). Similar effects have been noted in a handful of human trials where CA treatment reduced anxiety in elderly adults with mild cognitive impairment (Tiwari et al., 2008) and decreased symptoms of anxiety and depression in middle-aged adults with generalized anxiety disorder (Jana et al., 2010). A substantial literature also supports the cognitive enhancing effects of CA. In human tests, CA extract or dried herb improved cognitive function of healthy middle-aged and elderly (Wattanathorn et al., 2008; Dev et al., 2009) individuals as well as elderly subjects with mild cognitive impairment (Tiwari et al., 2008). These effects have been recapitulated in rodent models as well, where CA was shown to improve learning and memory in healthy rats (Veerendra Kumar and Gupta, 2002) and in mouse models of neurotoxicity (Gupta et al., 2003; Veerendra Kumar and Gupta, 2003). Our own group has also shown that a low dose of the water extract of CA (CAW) in the drinking water can improve cognitive function in mouse models of aging and Alzheimer’s disease (Soumyanath et al., 2012; Gray et al., 2016; Gray et al., 2018a; Gray et al., 2018b).
This study builds on previous work to further investigate the effects of CAW on cognition, measures of anxiety and depressive-like behavior in healthy aged mice. For the first time the effects of increasing concentrations of CAW on cognitive performance in aged mice will be evaluated. Additionally, this is the first study investigating the effects of CAW on anxiety and depression.
The first part of the study evaluated the effects of 5 weeks of treatment with increasing concentrations of CAW integrated in the diet on learning, memory, executive function, measures of anxiety and depressive-like behavior in 3- and 18-month-old C57BL6 mice. The study then went on to compare the behavioral effects in 18-month-old C57BL/6J mice of the highest concentration of CAW administered in the diet versus in the drinking water.
Materials and methods
CAW
CAW was prepared as previously described by our group (Yang et al., 2023). Briefly, CA (dried aerial parts; 4 kg) purchased through Oregon’s Wild Harvest (Redmond, OR, United States) was boiled with deionized water (50 L) for 90 min. The mixture was allowed to cool until safe to handle, allowing plant material to settle to the bottom of the boiling kettle. The upper liquid was filtered to remove insoluble materials including finer plant debris (McMaster-Carr #5162K112 filter bag). The filtrate was frozen in aluminum baking trays and lyophilized in 3 separate batches to yield extracts BEN–CAW-7, 8 and 9 (total weight of 820 g) all of which were used during the course of the present study. Voucher samples of the starting plant material are stored at the Oregon State University Herbarium (Voucher number OSC-V265416) and in our laboratory at Oregon Health & Science University (Voucher number BEN-CA-6), along with voucher samples of the dried CAW extract batches (Voucher numbers BEN-CAW 7, 8 and 9).
Animals
C57BL6 mice were obtained from the National Institute of Aging Aged Rodent Colony. Mice were kept in a climate-controlled environment with a 12-h light/12-h dark cycle. Water and diet (AIN-93M; Dyets Inc., Bethlehem, PA, US) were supplied ad libitum, except during the Odor Discrimination Reversal Learning testing when food was restricted at night and resupplied in the afternoon following testing. All methods were performed in correspondence with the NIH guidelines for the Care and Use of Laboratory Animals and were approved by the Institutional Animal Care and Use Committee of the Portland VA Medical Center.
CAW in diet and drinking water
Diets containing CAW were prepared by Dyets Inc. by mixing CAW (at 0.2%, 0.5% and 1.0% w/w) with the AIN-93M diet components until a homogenous distribution was achieved. After adding cold water (10%), the diet was run through a California Pellet Mill CL-3 lab pellet mill to create pellets, which were air dried at 27°C for 24 h. Finally, the diet was sterilized by gamma irradiation (5.0–20.0 kGy; Sterigenics, Oak Brook, IL, United States).
For administration in drinking water, CAW was dissolved in deionized water at a concentration of 10 mg/mL. Drinking water containing CAW was replaced twice weekly, i.e., every 3 or 4 days.
Composition and content of CAW and CAW-containing diets
The chemical composition of the CAW used was previously reported (Yang et al., 2023). Inclusion of CAW in the rodent diets at the required levels was verified using liquid chromatography multiple reaction monitoring mass spectrometry (LC-MRM-MS) of triterpene and caffeoylquinic acid components as previously reported by our group (Yang et al., 2023). Absence of microbial contamination was verified and the stability of those compounds in the diet over several months storage was confirmed to ensure that degradation was not occurring (Supplementary Tables S1 and S2). Water treatments with CAW freshly dissolved in the drinking water were replaced for the mice every 3–4 days and so the stability of those key compounds in the drinking water was similarly assessed after 4 days (Supplementary Table S3).
CAW treatment
The treatment paradigm is outlined in Figure 1. Briefly, three- and eighteen-month-old mice were kept on AIN-93M treated with CAW for a total of 5 weeks. Young (3-month-old) and old (18-month-old) mice were treated with CAW integrated into AIN-93M at concentrations calculated to deliver 0 mg/kg/day, 200 mg/kg/day, 500 mg/kg/day and 1,000 mg/kg day exposure based on average mouse weights and food consumption from previous studies (Matthews et al., 2019; Matthews et al., 2020). An additional treatment group of old mice exposed to CAW in the drinking water (10 mg/mL) for 5 weeks was also included. This concentration of CAW in the water was selected to approximate the 1,000 mg/kg/day exposure again based on calculations from previous studies (Matthews et al., 2019; Matthews et al., 2020). The number of animals in each treatment group can be found in Table 1. The duration of the behavioral tests required splitting the mice into two cohorts so that testing for each mouse did not exceed 2 weeks. To accomplish that each treatment group was divided in half so that 12 of each sex were evaluated for each behavioral endpoint described below.
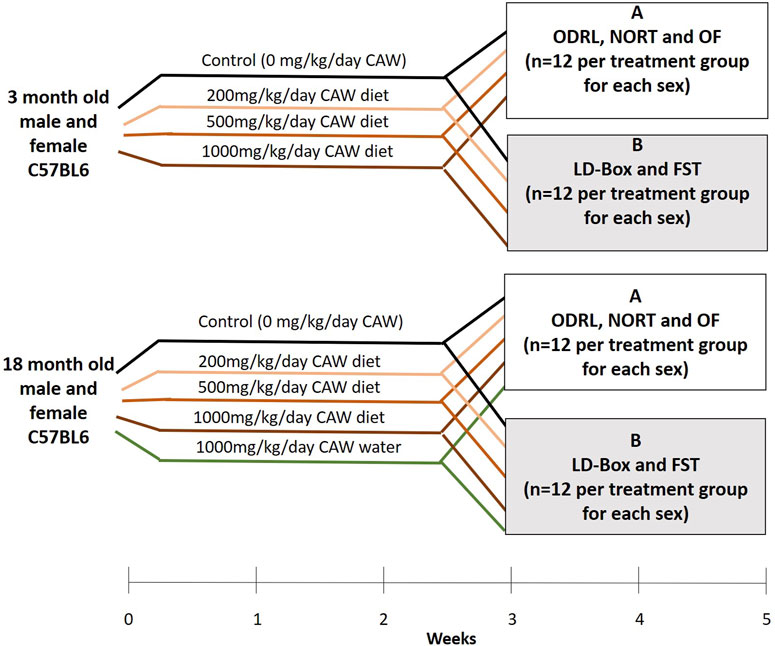
Figure 1. Treatment timeline and tests applied to each of two subgroups groups of mice receiving similar treatments. Mice in group A underwent ODRL: odor discrimination reversal learning, NORT: novel object recognition test and OF: open field. Mice in group B underwent LD-Box: light dark box and FST: forced swim test.
Behavioral testing
In the final 2 weeks of treatment, mice underwent behavioral testing. Mice (n = 12 per treatment) were subjected to one of two groups of behavioral tests (Figure 1), Group A: odor discrimination reversal learning (ODRL), novel object recognition test (NORT) and open field (OF) or Group B: light dark box (LD-BOX) and forced swim test (FST). All testing occurred in the same time window, between 9a.m. and 2p.m., for each animal.
Although 12 male and 12 female receiving each treatment condition were allocated for a given test, data was not collected for that many animals in every behavioral test due to a variety of factors, including technical issues with the software, non-participation of mice in the task, and COVID-related issues with the experimenters. The number of animals that completed each test can be found in Supplementary Table S4.
ODRL: This test assesses learning and cognitive flexibility (Bissonette and Powell, 2012). The test was performed as previously reported (Gray et al., 2018a). Briefly, mice were trained to dig for a food reward after which the acquisition stage followed where mice were presented with two cups, one that contains dried beans and one with string. In every trial, one digging material was scented with a mint odor and the other with vanilla randomly alternative the pairings and balancing the location of the baited cup (right vs. left). Example trials are outlined in Table 2. The criteria for completing the acquisition phase was 8 correct digs in any bout of 10 trials. The number of trials for each mouse to reach criteria was recorded. After a mouse reached the criteria in the acquisition phase, it immediately proceeded to the shift phase. As in the acquisition phase, in the shift phase, mice were presented with two cups containing the same digging materials and odors but in this phase the dried beans were always baited regardless of odor so the mice had learn to associate the food reward with the digging material and ignore the odor. Again, criteria was defined as 8 correct trials in any bout of 10 and trials to criteria was recorded. Mice were food restricted the night before each phase of the ODRL to motivate the animals.
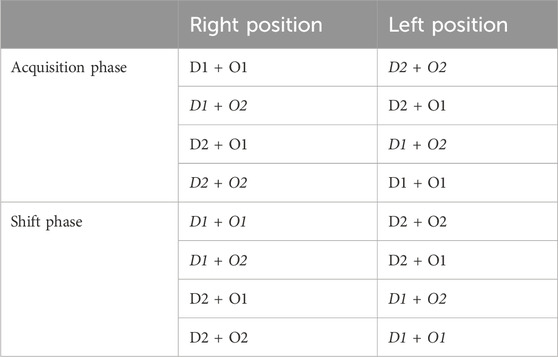
Table 2. Example of test pairings for Odor Discrimination Reversal Learning (ODRL) test: Representative combinations of odor and digging material pairings during each phase of the ODRL. D1: dried beans; D2: pieces of string; O1: vanilla; O2 = mint. Italicized and bold indicates the baited cup.
NORT: This is a test of recognition memory (Lueptow, 2017). It was performed as previously described (Matthews et al., 2019). Briefly, after two 10 min habituation sessions animal are exposed to two identical objects for 10 min, once an hour in a 3 h period. The testing phase occurred 2 h and 24 h after the final training phase where one of the identical objects was replaced with a novel object and the time spent exploring the familiar and novel objects over 5 min was evaluated via a camera placed above the arena, interfaced with ANYmaze video tracking system (Stoelting Co, Wood Dale, IL). At 24 h, a second novel object (distinct from the object used in the 2 h test) is used along with the familiar one from training. Again, the time spent exploring the familiar and novel objects was evaluated.
OF: The OF test can be used to assess anxiety and overall activity (Kraeuter et al., 2019). In the OF test, each mouse was placed into a square arena (38 × 38 × 64 cm high, constructed of white acrylonitrile butadiene styrene) for a 10-min open field session. A camera mounted above the arena, interfaced with a video tracking system (Any-maze, Erie, PA), captured time immobile (s) and time in the center (s). Increased time in the center and reduced time immobile are associated with decreased measures of anxiety.
LD-Box: The testing chamber consists of a square Plexiglas chamber (60 cm x 60 cm x 26 cm) fitted with infrared beams on all sides to measure locomotor activity and a z-axis beam to measure rearing. This test is intended to test for measures of anxiety by placing a dark insert into the chamber (16″x16″x12″). Additional lighting is provided on the open portion to achieve a lux of 3,000 and a cutout door (4″x2″) allows the animal to freely move between zones. Mice are placed in the light portion of the light/dark box and time in each zone, overall activity, and latency to enter the dark were measured with the infrared beams for each zone of the apparatus (light or dark). The test lasted for a total of 10 minutes. Increased time in the light portion of the arena reflects decreased measures of anxiety (Bourin and Hascoët, 2003).
FST: The forced swim test assesses depressive-like behavior (Can et al., 2012). The forced swim test occurred in a plastic tub (22 cm H x 28 cm W x 40 cm L) filled with lukewarm tap water at 25°C (Yankelevitch-Yahav et al., 2015). The test occurs over 2 days with the time spent immobile over a 5- minute period measured each day. Results reported are from the second day of the test when immobility is more pronounced than on the first day. Increased immobility is interpreted as increased depressive-like behavior.
Statistical analyses: Prior to formal analysis, the outcomes were visually examined to check for distribution patterns and possible outliers. No outliers were removed in our analysis. Bivariate plots were created to investigate how the endpoints relate to grouping variables. A linear regression model was then built with a three-way interaction involving treatment, age, and sex. Likelihood ratio tests were conducted to determine the significance of interactions related to sex. If significant, the analysis was stratified by sex and a two-way interaction model for treatment and age was run. Otherwise, sex was included as a covariate. If linear regression was not suitable due to issues like heteroskedasticity and non-normal residuals, a generalized linear model (GLM) was used following the same approach. For endpoints involving percentages or discrimination indices, the data was transformed into proportions and a beta model was applied. Otherwise, a GLM was used with a log-link and Eicker-Huber-White standard errors. A summary of which statistical analyses were conducted for each endpoint can be found in Supplementary Tables S5 and S6.
A priori contrasts were conducted and an MVT adjustment to control family-wise error rates was applied when comparing p-values. All analyses were conducted using the betareg (Cribari-Neto and Zeileis, 2010) and emmeans (Lenth, 2023) packages in R V4.3.0. For endpoints with a significant interaction between age, treatment effect and sex, male and female mice were graphed separately. For endpoints without significant interactions, both sexes were plotted together. All graphs show individual data points and means and error bars reflect the standard error of the mean. Detailed statistical parameters for all results can be found in Supplementary Tables S7 and S8.
Results
CAW administered in the diet improves learning and cognitive flexibility in aged mice in a dose-dependent manner
The ODRL is a test of learning and the cognitive flexibility domain of executive function. An increased number of trials to reach criteria in the acquisition phase suggests deficits in learning, while the same increase in the shift phase indicates impairments in cognitive flexibility. There was no significant interaction between age, sex and treatment in either the acquisition or shift phase of the ODRL and so male and female mice were analyzed together. Eighteen-month-old mice exhibited worse performance, compared to three-month-old mice, in both the acquisition (Figure 2A, p = 5.55E-16) and shift (Figure 2B; p = 1.63E-13) phases of the ODRL. Treatment with CAW integrated into the diet improved performance in both phases for old, 18-month-old, animals and the magnitude of improvement increased with increasing concentrations of CAW (Figures 2A,B; see Supplementary Table S7 for exact p values of each comparison). In contrast, CAW treatment administered in the diet had no effect on the performance of young, 3-month-old, mice in either the acquisition or shift phases of the ODRL regardless of concentration (Figures 2A,B; see Supplementary Table S7 for exact p values of each comparison).
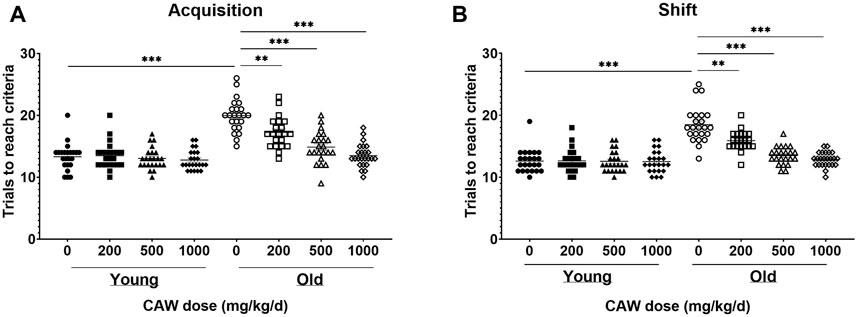
Figure 2. CAW in the diet improves learning and executive function in old mice in a dose-dependent manner. Deficits were seen in the acquisition (A) and shift (B) phases of the ODRL in old mice relative to young animals, which were attenuated with CAW treatment administered in the diet. Increasing concentrations of CAW resulted in greater improvements in ODRL performance. Combined data from male and female mice are shown as sex differences were not observed. n = 22–24 (11-12F and 11-12M) per condition; **p < 0.01, ***p < 0.0001.
CAW in the diet has divergent effects on recognition memory in male and female old mice
The NORT test of recognition records the amount of time the mice spent exploring a novel object versus a familiar one. Decreased time spent with the novel object indicates poorer recognition memory. There was a significant interaction between sex, age and treatment in both the 2- and 24-h retention tests. At the 2-h time point, no significant differences were seen for female mice in the percent time spent with the novel object regardless of age (p = 0.94) or treatment (see Supplementary Table S7 for exact p values of each comparison), although in young female mice there was a non-significant trend towards reduced time with the novel object seen in animals treated with 500 mg/kg/d (Figure 3A; p = 0.051). At the same time point in male mice, there was a significant improvement time spent with the novel object in aged mice treated with 500 mg/kg/d CAW, an effect not observed in young mice (Figure 3B; p = 0.007). In contrast, in aged female mice 500 mg/kg/d CAW improved NORT performance at 24 h (Figure 3C; p = 0.035). In male mice this concentration of CAW had no effect, although 200 mg/kg/d CAW actually decreased time spent with the novel object in aged male mice (Figure 3D; p = 0.049). CAW treatment in the diet did not impact performance in young animals of either sex regardless of concentration.
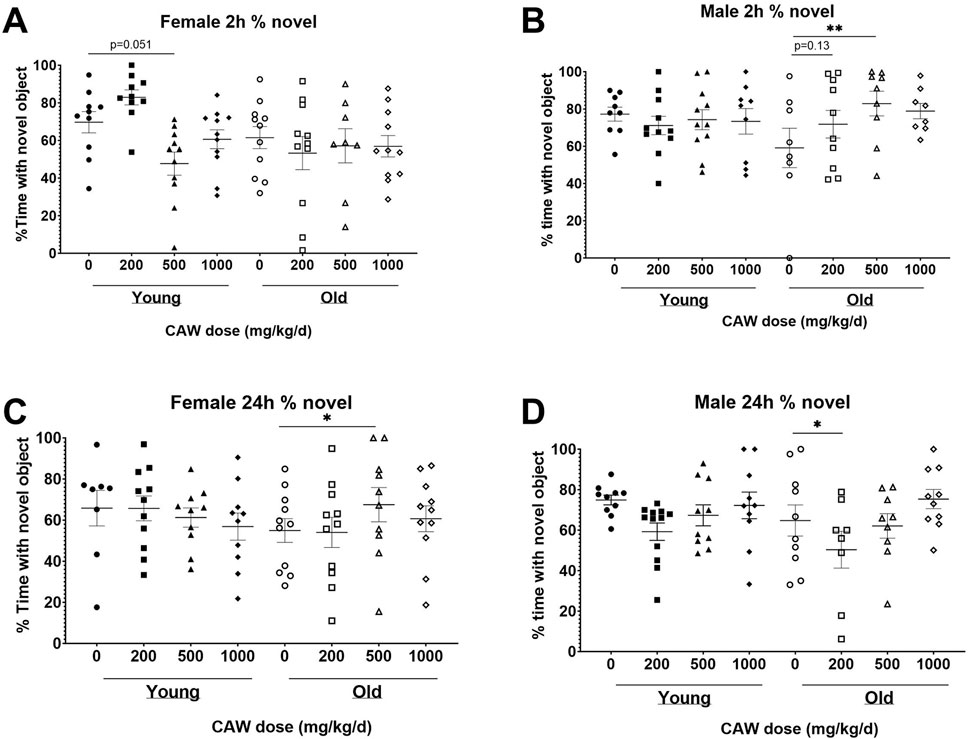
Figure 3. CAW in the diet results in inconsistent modulation of NORT performance. There was a significant interaction between sex, age and treatment in the NORT. (A) No significant effects of age or treatment were observed in female mice during the 2 h test. (B) In aged male mice, 500 mg/kg/d CAW improved performance during the 2 h test. At 24 h, (C) 500 mg/kg/d improved NORT performance in aged female mice while (D) 200 mg/kg/d resulted in impaired performance in aged male mice. n = 13–22 (7-11F and 6-11M) per condition; *p < 0.05, **p < 0.01.
CAW administered in the diet has no effect on behaviors associated with measures of anxiety and depressive-like behavior in old mice and may increase some anxiety-like behavior in young female mice
The OF test of anxiety monitors total time spent in the center of the field as well as time immobile. Decreased time in the center reflects enhanced anxiety. Increased time immobile can also reflect increased anxiety although that effect depends on where in the field (center vs. periphery) the immobility occurs. There was no interaction between age, sex and treatment in time immobile in the OF. No differences in time immobile were observed between young and old control mice nor did CAW treatment affect the time immobile at any concentration at which it was administered in the diet in either the old or the young mice (Supplementary Figure S1A).
There was a significant interaction between age, sex and treatment effect for the time in the center in OF. Aged, control female mice displayed significantly decreased time in the center compared to young, female, control animals (p = 0.0003), although CAW treatment did not affect this endpoint in the old female mice at any concentration (Figure 4A; see Supplementary Table S7 for exact p values of each comparison). Interestingly, all concentrations of CAW significantly reduced time in the center in young female animals (Figure 4A; see Supplementary Table S7 for exact p values of each comparison). In contrast, in male mice no effects of either age or treatment were evident in the time in the center in the OF (Figure 4B; see Supplementary Table S7 for exact p values of each comparison).
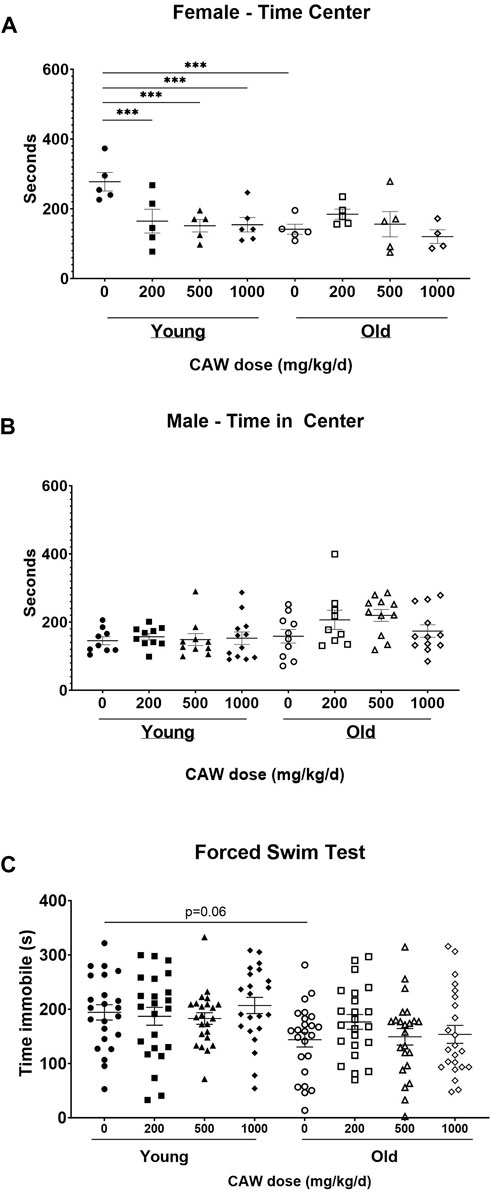
Figure 4. CAW in the diet does not improve measures of anxiety and depression. There was a significant interaction between sex, age and treatment in the time in the center for the Open Field (OF) test. (A) Aged female mice displayed reduced time in the center relative to young mice. CAW did not affect time in the center in old mice at any concentration but higher concentrations of CAW resulted in reduced time in the center of young female mice. n = 4-6 per condition; (B) There were no effects of age or treatment on time in the center for male mice. n = 9–12 per condition; There were similarly no effects of age or treatment on time immobile in the FST (C). n = 21–24 (11-12F and 10-12M) per condition; ***p < 0.001.
LD-Box is another test that evaluates anxiety. More anxious mice will spend more time in the dark than less anxious mice. In this test, however, no differences between groups were observed regardless of age or treatment (Supplementary Figure S1B).
The FST evaluates depressive behavior. Increased time immobile indicates increased depressive behavior. Here again no significant effects of age, sex nor of any concentration of CAW administered in the diet were detected (see Supplementary Table S7 for exact p values of each comparison) although surprisingly there was a non-significant trend towards reduced time immobile in the old control mice relative to the young control animals (Figure 4C; p = 0.17).
CAW given in the drinking water elicits even greater attenuation of age-related impairments in learning and executive function in aged mice than CAW given in the diet
The effects of 5 weeks of treatment with the highest dose of CAW (1,000 mg/kg/d equivalent) administered in the diet was assessed and compared with when CAW was administered in the drinking water. In the acquisition phase of the ODRL, CAW in the drinking water attenuated age-related deficits even more robustly (p = 0.043) than when it was given in the diet (Figure 5A). The same enhanced response in old mice to CAW in the drinking water compared to the diet (p = 0.028) was observed in the shift phase of the ODRL (Figure 5B).
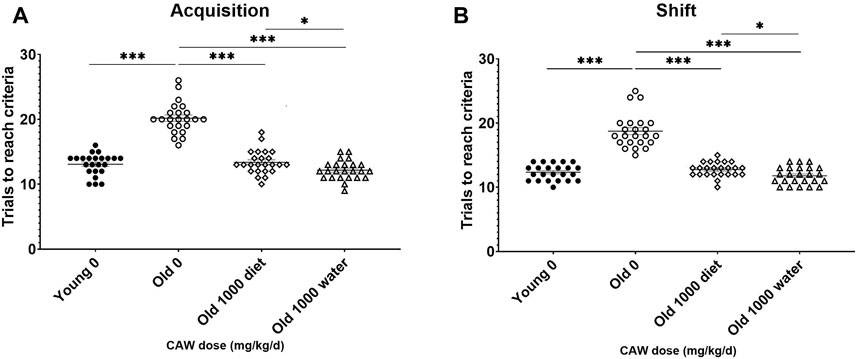
Figure 5. CAW in the drinking water improves learning and executive function in old mice more robustly than when given in the diet. CAW attenuated age-related deficits in ODRL performance in both the acquisition (A) and shift (B) phases of the ODRL. Improvements elicited by CAW in the drinking water were even greater than those seen with CAW treatment in the diet. n = 23–24 (11-12F and 12M) per condition; *p < 0.05, ***p < 0.0001.
CAW in the drinking water improves recognition memory in aged animals
When CAW was administered in the drinking water, there was a significant increase in the percent time spent with the novel object apparent in aged mice during the 2-h retention test (Figure 6A; p = 0.011). A similar but non-significant was observed in aged mice receiving CAW in the drinking water at 24 h (Figure 6B; p = 0.129).
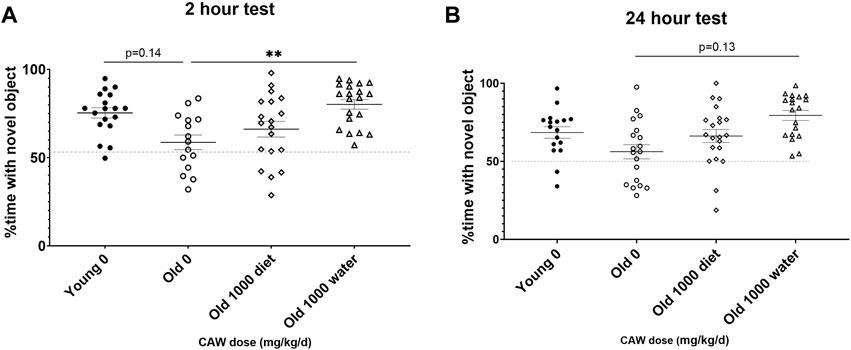
Figure 6. Recognition memory in aged mice is improved by CAW treatment in the drinking water but not in the diet. CAW given in the drinking water (A) significantly increased time spent with the novel object at 2 h and (B) showed a trend to an increase at 24 h n = 15–20 (9-11F and 6-9M) per condition; **p < 0.01.
CAW given in the drinking water has inconsistent effects on different metrics of anxiety but does not affect depressive behavior in aged mice
There was a significant interaction between age, sex and treatment effect when examining time in the center of the OF test. There was a robust increase in time in the center in aged female mice in response to CAW given in the drinking water compared to aged female mice that either received CAW in the diet or did not receive any CAW (Figure 7A; see Supplementary Table S7 for exact p values of each comparison). This suggests a decrease in age-related anxiety in the female mice. However, there was no effect of CAW in the drinking water on time in the center for male mice (Figure 7B; see Supplementary Table S7 for exact p values of each comparison). There was no interaction between age, sex and treatment effect for time immobile in the OF. CAW in the drinking water significantly attenuated the age-related increase in time immobile in aged mice in the OF test (p = 2.57E-5), while CAW in the diet did not affect this endpoint (Figure 7C).
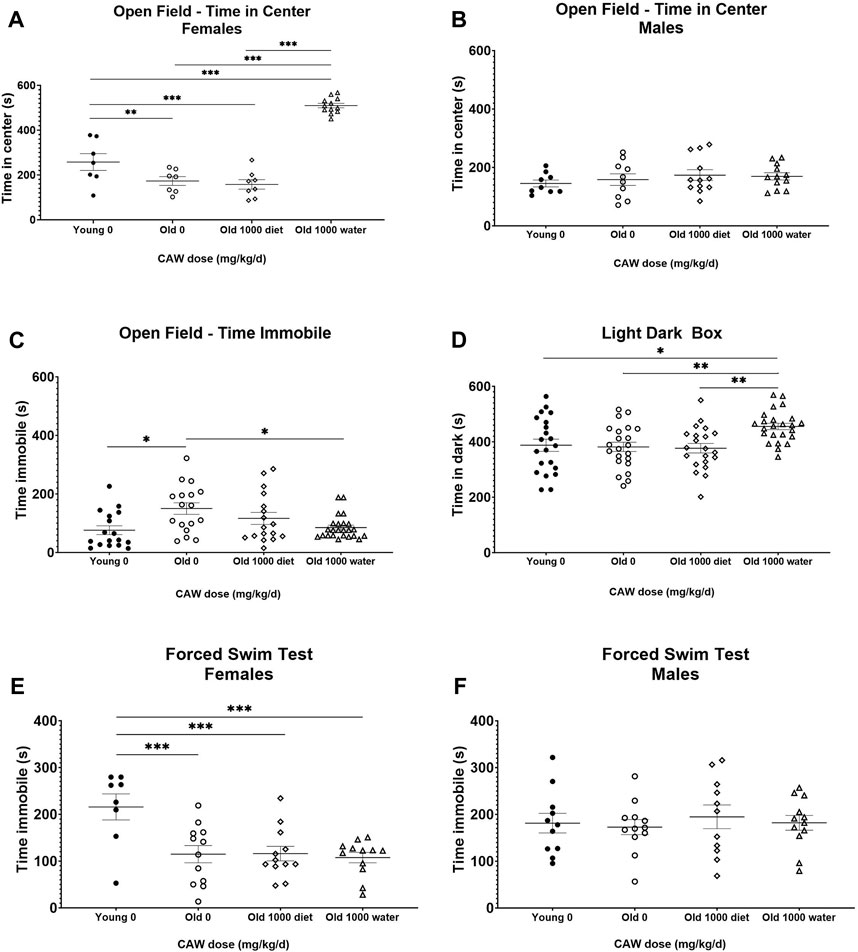
Figure 7. Although CAW in drinking water improves some metrics of anxiety in aged mice, it exacerbates other metrics and has no effect on depression-like behavior. There was a significant interaction between sex, age and treatment in the time in the center for the OF. (A) Aged female mice displayed reduced time in the center relative to young mice. CAW in the diet did not affect time in the center in old mice but CAW in the drinking water resulted in increased time in the center for old female mice. n = 7–12 per condition; (B) There were no effects of age or treatment on time in the center for male mice. n = 9–12 per condition; (C) CAW in the drinking water attenuated increases in time immobile in the open field in old mice while CAW in the diet did not significantly affect this endpoint. n = 16–24 per condition (5-12F and 11-12M); (D) In aged mice CAW the diet did not affect time in the dark in the LD-Box but CAW in the drinking water actually increased time in the dark. n = 21–24 per condition (10-12F and 11-12M); (E) In the FST, aged female mice displayed reduced time immobile relative to young female mice. CAW treatment had no effect on time immobile in the FST whether administered in the drinking water or the diet n = 8–12 per condition; (F) There were no effects of age or treatment on time immobile for male mice in the FST n = 11–12 per condition; *p < 0.05; **p < 0.01; ***p < 0.001.
In contrast, when using the LD-Box to assess anxiety, an increase in time in the dark was observed in aged mice following CAW administration in the drinking water (Figure 7D; p = 0.002). In this test however age-related changes were not observed (p = 0.995).
In the FST test, there was an interaction between sex, age and treatment for time immobile. Aged female mice had reduced time immobile relative to younger mice on day 2 (Figure 7E; p = 0.002) but the same age effect was not seen in male mice (Figure 7F; p = 0.990). CAW had no effect on FST time immobile in either sex regardless of the route of administration (Figures 7E,F; see Supplementary Table S7 for exact p values of each comparison).
Discussion
Alterations in cognition and mood are highly prevalent in the aging population and negatively affect quality of life. Approximately two out of three Americans experience at least some form of cognitive impairment by age 70 (Hale et al., 2020). Changes in mood are similarly common in the aging population, with a prevalence of depressive symptoms and anxiety of nearly 1 in 5 in elderly people (Services UDoHaH, 1999; CfDCaPaNAoCD, 2008). All of these conditions also often severely curtail daily life activities in older people further underscoring the need for therapies that can increase resilience to these age-related challenges.
The cognitive, antidepressant and anxiolytic effects of C. asiatica have been demonstrated many times in both rodent and human studies (Veerendra Kumar and Gupta, 2003; Wijeweera et al., 2006; Tiwari et al., 2008; Wattanathorn et al., 2008; Dev et al., 2009; Jana et al., 2010; Kalshetty et al., 2012; Selvi et al., 2012; Wanasuntronwong et al., 2012; Chanana and Kumar, 2016). Our group has previously shown that the water extract of C. asiatica (CAW) administered in the drinking water at 2 mg/mL (calculated to deliver 200 mg/kg/d CAW) improves cognitive function in mouse models of aging and neurodegenerative disease (Soumyanath et al., 2012; Gray et al., 2016; Gray et al., 2018a; Gray et al., 2018b; Matthews et al., 2019). This study builds on that previous research to explore the ability of CAW to reverse age-related changes in anxiety and depression. The effects of multiple doses of CAW on cognitive and mood endpoints and assessed sex differences in response to the extract was also evaluated.
Impaired performance in aged mice (compared to young mice) was seen in ODRL, NORT and OF, although for OF the age effect was only observed in female mice. The impaired performance seen in these tasks is in line with previous reports that also demonstrated diminished ODRL, NORT and OF performance in aged mice relative to young ones (Patel and Larson, 2009; Shoji and Miyakawa, 2019; Yang et al., 2019). There was not, however, any observed age-related deficits in LD-Box or FST or for male mice in the OF. This is in contrast to previous reports in the literature where poorer performance was observed in LD-BOX, FST and OF (Stilling et al., 2014; Lim et al., 2018; Shoji and Miyakawa, 2019) and could be related to differences in the ages tested or it might reflect subtle differences in testing protocol and metrics recorded. For instance, in one study using LD-BOX, reduced distance traveled in the dark was seen in 23-month-old mice but not 17-month-old mice as compared to 3-month-old animals (Shoji and Miyakawa, 2019). In the same study time immobile in the FST test was increased in 24-month-old mice relative to 3-month- old animals but no differences were seen between the 3- and 18-month-old groups (Shoji and Miyakawa, 2019). This suggests that perhaps a larger difference in age is required to observe differences in performance in the FST and LD-BOX.
When CAW was administered in the diet, dose-dependent attenuation of age-related deficits in learning and cognitive flexibility were observed. Old mice receiving the highest concentration of CAW in the diet (1,000 mg/kg/d) showed improved performance in the ODRL test compared to what was seen in the young, 3-month-old mice. The effect of CAW in the diet on recognition memory was less robust. Significant improvements were seen in old male mice treated with 500 mg/kg/d in the 2 h NORT test and in old female mice treated with the same concentration in the 24 h test but no significant deficits were detected between old controls and young controls. Surprisingly, there was also a significant impairment in NORT performance in the old male mice treated with 200 mg/kg/d in the 24 h test. The lack of a consistent effect of CAW in the diet on NORT performance is in contrast to the clear benefit observed in the ODRL. It is possible this discrepancy could be explained by a differential effect of CAW in distinct brain regions. The ODRL is mediated by the medial prefrontal cortex (McAlonan and Brown, 2003; Dalley et al., 2004), while NORT performance relies on inputs from perirhinal cortex, hippocampus, medial prefrontal cortex and medial dorsal thalamus (Warburton and Brown, 2015). While further studies are needed to confirm that the levels of CAW constituent compounds are consistent across various brain regions, regional differences seem unlikely given our previous report of similar effects of CAW on antioxidant gene expression in the cortex, hippocampus and cerebellum of aged mice (Gray et al., 2016). It is more probable that the different effects seen in the ODRL and NORT reflect differences in the sensitivity and variability of each test. Another consideration is that anxiety towards exploring novel objects (neophobia) might have contributed to these divergent findings as well. There was a much greater amount of variability observed between animals in NORT performance which likely prevented the detection of more significant and consistent findings.
CAW given in the diet did not appear to affect metrics of anxiety in aged mice. However, there was a statistically significant decrease in time spent in the center of the open field in young female mice at all concentrations of CAW tested, suggesting elevated levels of anxiety. This effect in young mice and the lack of effect in aged mice was surprising given the purported anxiolytic effects of CAW. To the best of our knowledge, there have not been prior reports of anxiogenic effects of C. asiatica in young mice. CAW administered in the diet also had no effect on depressive-like behavior as quantified in the FST.
The limited behavioral effects of CAW in the diet were unexpected. Although our group had not previously investigated the effects of CAW on measures of anxiety or depressive like behavior, based on our many published studies on the cognitive effects of CAW a more robust effect at least on those endpoints was expected. Previous experiments have shown improvements in cognitive function in aged mice following CAW administered in the drinking water at a concentration equivalent to the 200 mg/kg/d dose of CAW used in this study (Gray et al., 2016; Gray et al., 2018a) and in a mouse model of Alzheimer’s disease increasing concentrations of CAW in the drinking water (equivalent to the 500 mg/kg/d and 1,000 mg/kg/d) resulted in even greater improvements in cognitive function (Matthews et al., 2019). Interestingly, NORT performance was also improved in both healthy aged mice treated with 200 mg/kg/d equivalent in the drinking water (Gray et al., 2018a) and with all three concentrations administered in the drinking water in the Alzheimer’s disease mouse model (Matthews et al., 2019) whereas that was not seen in the current study where CAW was administered in the diet. This led us to hypothesize that mode of administration could affect behavioral response. Therefore the next evaluation looked at the effects of the equivalent dose (1000 mg/kg/d) of CAW administered in the drinking water to old mice on the same battery of tests of cognitive function, anxiety and depression.
When old mice were treated with 1,000 mg/kg/d CAW equivalent in the drinking water, there was a significant improvement in both the ODRL and the NORT, and the improvement in ODRL elicited by CAW in the drinking water was even greater than what was seen with CAW (1,000 mg/kg/d) in the diet. There were also significant reductions in anxiety in the open field test but this affect again varied by sex, with aged female mice exhibiting significantly less time in the center than young female mice and aged female mice treated with CAW in the drinking water showing a robust increase in this metric. The apparent sex-specificity of this response is interesting. There are many reports in the literature, in both humans and rodents, of females exhibiting increased anxiety-related behavior relative to males (Bahrami and Yousefi, 2011; McLean et al., 2011; Marchette et al., 2018; Bicakci et al., 2022). It is possible therefore that the increased anxiety evident in the aged female mice made it easier to detect treatment effects than in male mice. Future studies using more sensitive assays of anxiety behavior could help clarify this issue.
The age-related increase in time immobile in the open field was attenuated with CAW in the drinking water in both sexes however, this effect is not necessarily related to changes in anxiety exclusively and might instead reflect a change in overall activity with CAW treatment. Intriguingly, CAW in the drinking water significantly increased time in the dark for aged mice in the LD-BOX test suggesting a potential anxiogenic effect. These apparent contradictory effects of CAW in the drinking water on anxiety-like behavior are puzzling. Previous reports of anxiolytic effects of various extracts of the plant have demonstrated improvements in open field and elevated plus maze (Wijeweera et al., 2006; Chanana and Kumar, 2016; Jagadeesan et al., 2019). LD-BOX was used in a study of a standardized formulation of triterpene compounds (ECa233) from C. asiatica and a reduction in anxiety was observed (Wanasuntronwong et al., 2012). However, the concentration of individual triterpenes in ECa233 was much higher (30%–50%) than in CAW (<5%) and the mice tested had been exposed to chronic immobilization stress; both factors may account for the differences in the effect of the CA extracts. Importantly, none of these reports evaluated anxiety in aged animals. In our study age-related increases in anxiety were only detected in the open field and not the LD-BOX. It would be interesting in future studies to employ the elevated plus maze and determine if deficits are evident in aged mice and if CAW in the drinking water attenuates or exacerbates them.
In our study, CAW also had no effect on depressive-like behavior quantified in the FST, regardless of whether it was administered in the diet or in the drinking water. These results are not consistent with the existing literature showing anti-depressant effects of C. asiatica extracts on performance in the FST in a mouse model of olfactory bulbectomy and in healthy young rats (Kalshetty et al., 2012; Selvi et al., 2012). Again, however, these studies were not directly investigating the effects in aged rodents and used other types of CA extracts. It is possible the FST is not sensitive enough to detect age-related changes in depressive-like behavior. If that is the case, then it is unsurprising that a treatment effect would also not be seen in the FST. Future studies could employ other tests of depressive behavior such as the tail suspension, sucrose preference test or conditioned place preference (Scheggi et al., 2018), or add an additional stressor to potentially magnify age-related differences, such as restraint stress, cage tilt or noise exposure (Atrooz et al., 2021).
One consistent result observed in the study was that effects of CAW were more often observed in aged animals than young ones. This is in line with our previous findings in younger animals where cognitive improvements were not observed at either 2 or 6 months of age (Gray et al., 2016; Zweig et al., 2021a). One reason that could account for this is that mice at those ages do not display any cognitive deficits. If a ceiling effect of maximal performance is being observed in untreated young mice, then it is not surprising that no improvement could be detected with CAW treatment. Although our group has not previously evaluated the effects of CAW on anxiety and depression in young animals it is possible that the same phenomenon is occurring in those tests as well.
Another of the most consistent findings of the present study was that CAW effects were more pronounced in the animals that received the extract in the drinking water than those that received it in the diet. While individual consumption was not assessed in this study due to the group housing set up, food and water consumption was monitored by cage and no differences were observed between treatment groups (data not shown). This suggests that palatability was not an issue in the CAW treated animals. A possible explanation for the differences in behavioral effect resulting from water or diet administration could be differing bioavailability of the constituent compounds of CAW depending on the mode of administration. In fact, a recent paper published by our group demonstrated exactly that showing that 5xFAD mice treated with CAW in the same manner as in this study (10 mg/mL in the water or the calculated equivalent in the diet for 5 weeks) had elevated plasma concentrations of constituent compounds when CAW was delivered in the drinking water compared to in the diet (Speers et al., 2024). Studies are underway to quantify constituent compounds from CAW in the plasma of treated animals to confirm whether this could explain the behavioral differences observed in this study as well.
Centella asiatica has a number of chemical components (Alcazar et al., 2020) of which triterpenes and caffeoylquinic acids (CQAs), appear to be the most relevant for its neurological effects (Gray et al., 2017). There is evidence in the literature for the ability of these compounds to penetrate the blood-brain barrier. The triterpenes asiatic acid and madecassic acid (found in C. asiatica) were detected in mouse brain following their administration as pure compounds (Yin et al., 2012) and their corresponding glycosides asiaticoside and madecassoside were observed in rat brain following administration of a C. asiatica extract or the pure compounds (Anukunwithaya et al., 2016; Hengjumrut et al., 2018). Mono and di-CQAs (Su et al., 2014), and their potential metabolites caffeic and ferulic acid have been detected in rat brain after administration of the pure compounds or botanical sources (Su et al., 2014; Gasperotti et al., 2015; Zhang et al., 2015). The regional distribution of these compounds within the brain has not been reported. Levels of constituent compounds from CAW have not been measured in the tissue of treated animals before but previous studies have reported changes in antioxidant gene expression and mitochondrial activity in the brains of mice treated with CAW (Gray et al., 2016; Gray et al., 2018b; Zweig et al., 2021b) which might suggest compound penetration although it is also possible that those are secondary effects induced by peripheral changes. Work is ongoing in to determine whether compounds from the CAW extract administered orally do in fact cross the blood brain barrier and whether differences may exist in their abundance depending on route of administration.
In summary, these findings suggest a beneficial effect of CAW on age-related changes in cognition and measures of anxiety, but not on depressive-like behavior. The magnitude of these effects was greater when CAW was administered in the drinking water as compared to when it was given in the diet. Future studies are needed to elucidate the mechanism by which CAW elicits its effects on cognition and measures of anxiety as well as to determine if active compounds from the extract reach greater systemic levels when given in the drinking water than in the diet and if these compound levels vary across different brain regions.
Data availability statement
The raw data supporting the conclusion of this article will be made available by the authors, without undue reservation.
Ethics statement
The animal study was reviewed and approved by Portland VA Institutional Animal Care and Use Committee. The study was conducted in accordance with the local legislation and institutional requirements.
Author contributions
NG: Conceptualization, Data curation, Project administration, Resources, Visualization, Writing–original draft, Writing–review and editing. WH: Investigation, Writing–review and editing. MB: Investigation, Writing–review and editing. JZ: Investigation, Writing–review and editing. LY: Investigation, Methodology, Writing–review and editing. LM: Investigation, Methodology, Writing–review and editing. JC: Investigation, Methodology, Writing–review and editing. AAM: Investigation, Writing–review and editing. NC: Investigation, Writing–review and editing. JM: Investigation, Writing–review and editing. SK: Formal Analysis, Writing–review and editing. TN: Formal Analysis, Writing–review and editing. JR: Data curation, Visualization, Writing–review and editing. JQ: Resources, Writing–review and editing. CM: Funding acquisition, Methodology, Resources, Writing–review and editing. AS: Conceptualization, Funding acquisition, Methodology, Resources, Writing–review and editing.
Funding
The author(s) declare that financial support was received for the research, authorship, and/or publication of this article. This work was funded by NIH-NCCIH grant U19AT010829 and NIH grant S10OD026922. Mice were provided by the NIA Aged Rodent Colony.
Acknowledgments
The authors acknowledge Cody Neff and Noah Gladen-Kolarsky for their assistance with the behavioral assays, Ben Zimmerman and Laura Knittel for collecting the CAW water solutions for stability analysis, and Zachary Wiegand of the Oregon State University Department of Food Science and Technology for assistance with the preparation of CAW.
Conflict of interest
NC and JM are employed by Oregon’s Wild Harvest.
The remaining authors declare that the research was conducted in the absence of any commercial or financial relationships that could be construed as a potential conflict of interest.
The author(s) declared that they were an editorial board member of Frontiers, at the time of submission. This had no impact on the peer review process and the final decision.
Publisher’s note
All claims expressed in this article are solely those of the authors and do not necessarily represent those of their affiliated organizations, or those of the publisher, the editors and the reviewers. Any product that may be evaluated in this article, or claim that may be made by its manufacturer, is not guaranteed or endorsed by the publisher.
Supplementary material
The Supplementary Material for this article can be found online at: https://www.frontiersin.org/articles/10.3389/fragi.2024.1357922/full#supplementary-material
References
Alcazar, M. A., Wright, K., Vaswani, A., Caruso, M., Reed, R. L., Bailey, C. F., et al. (2020). Integration of mass spectral fingerprinting analysis with precursor ion (MS1) quantification for the characterisation of botanical extracts: application to extracts of Centella asiatica (L.) Urban. Phytochem. Anal. 31, 722–738. doi:10.1002/pca.2936
Anukunwithaya, T., Tantisira, M. H., Tantisira, B., and Khemawoot, P. (2016). Pharmacokinetics of a standardized extract of Centella asiatica ECa 233 in rats. Planta Medica 83, 710–717. doi:10.1055/s-0042-122344
Atrooz, F., Alkadhi, K. A., and Salim, S. (2021). Understanding stress: insights from rodent models. Curr. Res. Neurobiol. 2, 100013. doi:10.1016/j.crneur.2021.100013
Bahrami, F., and Yousefi, N. (2011). Females are more anxious than males: a metacognitive perspective. Iran. J. Psychiatry Behav. Sci. Fall 5 (2), 83–90.
Bicakci, A. O., Sarkar, M., Chang, Y. H., Kahl, E., Ragazzi, L., Moldes-Anaya, A., et al. (2022). Anxiolytic-like effects of the positive GABA(B) receptor modulator GS39783 correlate with mice’s individual basal anxiety and stress reactivity. Pharm. (Basel) 15 (2), 233. doi:10.3390/ph15020233
Bissonette, G. B., and Powell, E. M. (2012). Reversal learning and attentional set-shifting in mice. Neuropharmacology 62 (3), 1168–1174. doi:10.1016/j.neuropharm.2011.03.011
Bourin, M., and Hascoët, M. (2003). The mouse light/dark box test. Eur. J. Pharmacol. 463 (1-3), 55–65. doi:10.1016/s0014-2999(03)01274-3
Buckner, R. (2004). Memory and executive function in aging and AD: multiple factors that cause decline and reserve factors that compensate. Neuron 44 (1), 195–208. doi:10.1016/j.neuron.2004.09.006
Can, A., Dao, D. T., Arad, M., Terrillion, C. E., Piantadosi, S. C., and Gould, T. D. (2012). The mouse forced swim test. J. Vis. Exp. (59), e3638. doi:10.3791/3638
Cansino, S. (2009). Episodic memory decay along the adult lifespan: a review of behavioral and neurophysiological evidence. Int. J. Psychophysiol. 71 (1), 64–69. doi:10.1016/j.ijpsycho.2008.07.005
CfDCaPaNAoCD, D. (2008). The state of mental health and aging in America: what do the data tell us? The state of mental health and aging in America.
Chanana, P., and Kumar, A. (2016). Possible involvement of nitric oxide modulatory mechanisms in the neuroprotective effect of Centella asiatica against sleep deprivation induced anxiety like behaviour, oxidative damage and neuroinflammation. Phytother. Res. 30 (4), 671–680. doi:10.1002/ptr.5582
Cribari-Neto, F., and Zeileis, A. (2010). Beta regression in R. J. Stat. Softw. 34 (2), 1–24. doi:10.18637/jss.v034.i02
Dalley, J. W., Cardinal, R. N., and Robbins, T. W. (2004). Prefrontal executive and cognitive functions in rodents: neural and neurochemical substrates. Neurosci. Biobehav Rev. 28 (7), 771–784. doi:10.1016/j.neubiorev.2004.09.006
Dev, R. D. O., Mohamed, S., Hambali, Z., and Samah, B. A. (2009). Comparison on cognitive effects of Centella asiatica in healthy middle aged female and male volunteers. Eur. J. Sci. Res. 31 (4), 553–565.
Fandakova, Y., Lindenberger, U., and Shing, Y. L. (2014). Deficits in process-specific prefrontal and hippocampal activations contribute to adult age differences in episodic memory interference. Cereb. Cortex 24 (7), 1832–1844. doi:10.1093/cercor/bht034
Gasperotti, M., Passamonti, S., Tramer, F., Masuero, D., Guella, G., Mattivi, F., et al. (2015). Fate of microbial metabolites of dietary polyphenols in rats: is the brain their target destination? ACS Chem. Neurosci. 6 (8), 1341–1352. doi:10.1021/acschemneuro.5b00051
Golden, J., Kenyon-Pesce, L., Robison, J., Grady, J., and Guerrera, M. P. (2023). Disclosure of complementary and alternative medicine use among older adults: a cross-sectional study. Gerontol. Geriatr. Med. 9, 23337214231179839. doi:10.1177/23337214231179839
Gray, N. E., Harris, C. J., Quinn, J. F., and Soumyanath, A. (2016). Centella asiatica modulates antioxidant and mitochondrial pathways and improves cognitive function in mice. J. Ethnopharmacol. 180, 78–86. doi:10.1016/j.jep.2016.01.013
Gray, N. E., Zweig, J. A., Caruso, M., Martin, M. D., Zhu, J. Y., Quinn, J. F., et al. (2018a). Centella asiatica increases hippocampal synaptic density and improves memory and executive function in aged mice. Brain Behav. 8 (7), e01024. doi:10.1002/brb3.1024
Gray, N. E., Zweig, J. A., Caruso, M., Zhu, J. Y., Wright, K. M., Quinn, J. F., et al. (2018b). Centella asiatica attenuates hippocampal mitochondrial dysfunction and improves memory and executive function in β-amyloid overexpressing mice. Mol. Cell. Neurosci. 93, 1–9. doi:10.1016/j.mcn.2018.09.002
Gray, N. E. A.-M. A., Lak, P., Wright, K. M., Quinn, J., Stevens, J. F., Maier, C. S., et al. (2017). Centella asiatica: phytochemistry and mechanisms of neuroprotection and cognitive enhancement. Phytochem. Rev. 17 (1), 161–194. doi:10.1007/s11101-017-9528-y
Gupta, Y. K., Veerendra Kumar, M. H., and Srivastava, A. K. (2003). Effect of Centella asiatica on pentylenetetrazole-induced kindling, cognition and oxidative stress in rats. Pharmacol. Biochem. Behav. 74 (3), 579–585. doi:10.1016/s0091-3057(02)01044-4
Hale, J. M., Schneider, D. C., Mehta, N. K., and Myrskylä, M. (2020). Cognitive impairment in the U.S.: lifetime risk, age at onset, and years impaired. SSM Popul. Health 11, 100577. doi:10.1016/j.ssmph.2020.100577
Hengjumrut, P., Anukunwithaya, T., Tantisira, M. H., Tantisira, B., and Khemawoot, P. (2018). Comparative pharmacokinetics between madecassoside and asiaticoside presented in a standardised extract of Centella asiatica, ECa 233 and their respective pure compound given separately in rats. Xenobiotica 48 (1), 18–27. doi:10.1080/00498254.2016.1273562
Institute, U. (2020). The US population in aging. Available at: https://www.urban.org/policy-centers/cross-center-initiatives/program-retirement-policy/projects/data-warehouse/what-future-holds/us-population-aging.
Jagadeesan, S., Musa Chiroma, S., Baharuldin, M. T. H., Taib, C. N. M., Amom, Z., Adenan, M. I., et al. (2019). Centella asiatica prevents chronic unpredictable mild stress-induced behavioral changes in rats. Biomed. Res. Ther. 6 (6), 3233–3243. doi:10.15419/bmrat.v6i6.550
Jana, U., Sur, T. K., Maity, L. N., Debnath, P. K., and Bhattacharyya, D. (2010). A clinical study on the management of generalized anxiety disorder with Centella asiatica. Nepal Med. Coll. J. 1 (8-11), 8–11.
Johnson, S., Sacks, P. K., Turner, S. M., Gaynor, L. S., Ormerod, B. K., Maurer, A. P., et al. (2016). Discrimination performance in aging is vulnerable to interference and dissociable from spatial memory. Learn Mem. 23 (7), 339–348. doi:10.1101/lm.042069.116
Kalshetty, P., Aswar, U., Bodhankar, S., Sinnathambi, A., Mohan, V., and Thakurdesai, P. (2012). Antidepressant effects of standardized extract of Centella asiatica L. in olfactory bulbectomy model. Biomed. Aging Pathol. 2 (2), 48–53. doi:10.1016/j.biomag.2012.03.005
Kraeuter, A. K., Guest, P. C., and Sarnyai, Z. (2019). The open field test for measuring locomotor activity and anxiety-like behavior. Methods Mol. Biol. 1916, 99–103. doi:10.1007/978-1-4939-8994-2_9
Lenth, R. (2023). Emmeans: estimated marginal means, aka least-squares means. R. package version 1.8.6.
Lim, J., Bang, Y., Choi, J. H., Han, A., Kwon, M. S., Liu, K. H., et al. (2018). LRRK2 G2019S induces anxiety/depression-like behavior before the onset of motor dysfunction with 5-HT(1A) receptor upregulation in mice. J. Neurosci. 38 (7), 1611–1621. doi:10.1523/jneurosci.4051-15.2017
Lueptow, L. M. (2017). Novel object recognition test for the investigation of learning and memory in mice. J. Vis. Exp., 55718. doi:10.3791/55718
Marchette, R. C. N., Bicca, M. A., Santos, E., and de Lima, T. C. M. (2018). Distinctive stress sensitivity and anxiety-like behavior in female mice: strain differences matter. Neurobiol. Stress 9, 55–63. doi:10.1016/j.ynstr.2018.08.002
Matthews, D. G., Caruso, M., Alcazar, M. A., Wright, K. M., Maier, C. S., Stevens, J. F., et al. (2020). Caffeoylquinic acids in Centella asiatica reverse cognitive deficits in male 5XFAD alzheimer’s disease model mice. Nutrients 12 (11), 3488. doi:10.3390/nu12113488
Matthews, D. G., Caruso, M., Murchison, C. F., Zhu, J. Y., Wright, K. M., Harris, C. J., et al. (2019). Centella asiatica improves memory and promotes antioxidative signaling in 5XFAD mice. Antioxidants 8 (12), 630. doi:10.3390/antiox8120630
McAlonan, K., and Brown, V. J. (2003). Orbital prefrontal cortex mediates reversal learning and not attentional set shifting in the rat. Behav. Brain Res. 146 (1-2), 97–103. doi:10.1016/j.bbr.2003.09.019
McLean, C. P., Asnaani, A., Litz, B. T., and Hofmann, S. G. (2011). Gender differences in anxiety disorders: prevalence, course of illness, comorbidity and burden of illness. J. Psychiatr. Res. 45 (8), 1027–1035. doi:10.1016/j.jpsychires.2011.03.006
Methqal, I., Provost, J. S., Wilson, M. A., Monchi, O., Amiri, M., Pinsard, B., et al. (2017). Age-related shift in neuro-activation during a word-matching task. Front. Aging Neurosci. 9, 265. doi:10.3389/fnagi.2017.00265
Murman, D. L. (2015). The impact of age on cognition. Semin. Hear 36 (3), 111–121. doi:10.1055/s-0035-1555115
Nascimento, A. L., Lozano, A., Melo, J. G., Alves, R. R., and Albuquerque, U. P. (2016). Functional aspects of the use of plants and animals in local medical systems and their implications for resilience. J. Ethnopharmacol. 194, 348–357. doi:10.1016/j.jep.2016.08.017
Newall, C. A., Anderson, L., and Phillipson, J. D. (1996). Herbal medicines. A guide for healthcare professionals. China: Pharmaceutical Press.
Park, D., Lautenschlager, G., Hedden, T., Davidson, N. S., Smith, A. D., and Smith, P. K. (2002). Models of visuospatial and verbal memory across the adult life span. Psychol. Aging 17 (2), 299–320. doi:10.1037//0882-7974.17.2.299
Park, I., Ochiai, R., Ogata, H., Kayaba, M., Hari, S., Hibi, M., et al. (2017). Effects of subacute ingestion of chlorogenic acids on sleep architecture and energy metabolism through activity of the autonomic nervous system: a randomised, placebo-controlled, double-blinded cross-over trial. Br. J. Nutr. 117 (7), 979–984. doi:10.1017/S0007114517000587
Patel, R. C., and Larson, J. (2009). Impaired olfactory discrimination learning and decreased olfactory sensitivity in aged C57Bl/6 mice. Neurobiol. Aging 30 (5), 829–837. doi:10.1016/j.neurobiolaging.2007.08.007
Scheggi, S., De Montis, M. G., and Gambarana, C. (2018). Making sense of rodent models of anhedonia. Int. J. Neuropsychopharmacol. 21 (11), 1049–1065. doi:10.1093/ijnp/pyy083
Selvi, P. T. K. M., Rajesh, R., and Kathiravan, T. (2012). Antidepressant activity of ethanolic extract of leaves of Centella asiatica Linn. by in vivo methods. Asian J. Res. Pharm. Sci. 2 (2), 76–79.
Services UDoHaH (1999). “Older adults and mental health,” in Mental health: a report of the surgeon general (USA: National Institute of Mental Health U.S.).
Shinomol, G. K., and Muralidhara, B. M. M. (2011). Exploring the role of "brahmi" (bacopa monnieri and Centella asiatica) in brain function and therapy. Recent Pat. Endocr. Metab. Immune Drug Discov. 5 (1), 33–49. doi:10.2174/187221411794351833
Shoji, H., and Miyakawa, T. (2019). Age-related behavioral changes from young to old age in male mice of a C57BL/6J strain maintained under a genetic stability program. Neuropsychopharmacol. Rep. 39 (2), 100–118. doi:10.1002/npr2.12052
Soumyanath, A., Zhong, Y. P., Henson, E., Wadsworth, T., Bishop, J., Gold, B. G., et al. (2012). Centella asiatica extract improves behavioral deficits in a mouse model of alzheimer’s disease: investigation of a possible mechanism of action. Int. J. Alzheimers Dis. 2012, 381974. doi:10.1155/2012/381974
Speers, A. B., Wright, K., Brandes, M. S., Kedjejian, N., Matthews, D. G., Caruso, M., et al. (2024). Mode of administration influences plasma levels of active Centella asiatica compounds in 5xFAD mice while markers of neuroinflammation remain unaltered. Front. Neurosci. 18, 1277626. ePub ahead of print. doi:10.3389/fnins.2024.1277626
Stilling, R. M., Benito, E., Gertig, M., Barth, J., Capece, V., Burkhardt, S., et al. (2014). De-regulation of gene expression and alternative splicing affects distinct cellular pathways in the aging hippocampus. Front. Cell. Neurosci. 8, 373. doi:10.3389/fncel.2014.00373
Su, D., Huang, J., Song, Y., and Feng, Y. (2014). Comparative pharmacokinetics and tissue distribution study of mono-and di-caffeoylquinic acids isomers of Ainsliaea fragrans Champ by a fast UHPLC-MS/MS method. Fitoterapia 99 (1), 139–152. doi:10.1016/j.fitote.2014.09.011
Tiwari, S., Singh, S., Patwardhan, K., Gehlot, S., and Gambhir, I. S. (2008). Effect of Centella asiatica on mild cognitive impairment (MCI) and other common agerelated clinical problems. Dig. J. Nanomater. Biostructures 3 (4), 215–220.
Veerendra Kumar, M. H., and Gupta, Y. K. (2002). Effect of different extracts of Centella asiatica on cognition and markers of oxidative stress in rats. J. Ethnopharmacol. 79 (2), 253–260. doi:10.1016/s0378-8741(01)00394-4
Veerendra Kumar, M. H., and Gupta, Y. K. (2003). Effect of Centella asiatica on cognition and oxidative stress in an intracerebroventricular streptozotocin model of Alzheimer’s disease in rats. Clin. Exp. Pharmacol. Physiol. 30 (5-6), 336–342. doi:10.1046/j.1440-1681.2003.03842.x
Vespa, J., Armstrong, D. M., and Medina, L. (2020). “Demographic turning points for the United States: population projections for 2020 to 2060,” in Current Population Reports. Washington, DC: U.S. Census Bureau P25-1144, 1–15.
Wanasuntronwong, A., Tantisira, M. H., Tantisira, B., and Watanabe, H. (2012). Anxiolytic effects of standardized extract of Centella asiatica (ECa 233) after chronic immobilization stress in mice. J. Ethnopharmacol. 143 (2), 579–585. doi:10.1016/j.jep.2012.07.010
Warburton, E. C., and Brown, M. W. (2015). Neural circuitry for rat recognition memory. Behav. Brain Res. 285, 131–139. doi:10.1016/j.bbr.2014.09.050
Ward, L., and Pasinetti, G. M. (2016). Recommendations for development of botanical polyphenols as "natural drugs" for promotion of resilience against stress-induced depression and cognitive impairment. Neuromolecular Med. 18 (3), 487–495. doi:10.1007/s12017-016-8418-6
Wattanathorn, J., Mator, L., Muchimapura, S., Tongun, T., Pasuriwong, O., Piyawatkul, N., et al. (2008). Positive modulation of cognition and mood in the healthy elderly volunteer following the administration of Centella asiatica. J. Ethnopharmacol. 116 (2), 325–332. doi:10.1016/j.jep.2007.11.038
Wijeweera, P., Arnason, J. T., Koszycki, D., and Merali, Z. (2006). Evaluation of anxiolytic properties of Gotukola--(Centella asiatica) extracts and asiaticoside in rat behavioral models. Phytomedicine 13 (9-10), 668–676. doi:10.1016/j.phymed.2006.01.011
Yang, L., Marney, L., Magana, A. A., Choi, J., Wright, K., Mcferrin, J., et al. (2023). Quantification of caffeoylquinic acids and triterpenes as targeted bioactive compounds of Centella asiatica in extracts and formulations by liquid chromatography mass spectrometry. J. Chromatogr. Open 4doi, 100091. doi:10.1016/j.jcoa.2023.100091
Yang, W., Zhou, X., and Ma, T. (2019). Memory decline and behavioral inflexibility in aged mice are correlated with dysregulation of protein synthesis capacity. Front. Aging Neurosci. 11, 246. doi:10.3389/fnagi.2019.00246
Yankelevitch-Yahav, R., Franko, M., Huly, A., and Doron, R. (2015). The forced swim test as a model of depressive-like behavior. J. Vis. Exp. 97, 52587. doi:10.3791/52587
Yin, M. C., Lin, M. C., Mong, M. C., and Lin, C. Y. (2012). Bioavailability, distribution, and antioxidative effects of selected triterpenes in mice. J. Agric. Food Chem. 60 (31), 7697–7701. doi:10.1021/jf302529x
Zhang, W., Li, D., Leng, A., Ai, J., Du, Y., Meng, Y., et al. (2015). Tissue distribution and excretion of the five components of Portulaca oleracea L. extract in rat assessed by UHPLC. Braz. J. Pharm. Sci. 51 (3), 643–651. doi:10.1590/S1984-82502015000300016
Zweig, J. A., Brandes, M. S., Brumbach, B. H., Caruso, M., Wright, K. M., Quinn, J. F., et al. (2021a). Loss of NRF2 accelerates cognitive decline, exacerbates mitochondrial dysfunction, and is required for the cognitive enhancing effects of Centella asiatica during aging. Neurobiol. Aging 100, 48–58. doi:10.1016/j.neurobiolaging.2020.11.019
Zweig, J. A., Brandes, M. S., Brumbach, B. H., Caruso, M., Wright, K. M., Quinn, J. F., et al. (2021b). Prolonged treatment with Centella asiatica improves memory, reduces amyloid-β pathology, and activates NRF2-regulated antioxidant response pathway in 5xFAD mice. J. Alzheimers Dis. 81 (4), 1453–1468. doi:10.3233/JAD-210271
Keywords: aging, cognition, anxiety, depression, Centella asiatica
Citation: Gray NE, Hack W, Brandes MS, Zweig JA, Yang L, Marney L, Choi J, Magana AA, Cerruti N, McFerrin J, Koike S, Nguyen T, Raber J, Quinn JF, Maier CS and Soumyanath A (2024) Amelioration of age-related cognitive decline and anxiety in mice by Centella asiatica extract varies by sex, dose and mode of administration. Front. Aging 5:1357922. doi: 10.3389/fragi.2024.1357922
Received: 19 December 2023; Accepted: 10 April 2024;
Published: 06 May 2024.
Edited by:
Anne Marie Minihane, University of East Anglia, United KingdomReviewed by:
Musa Samaila Chiroma, Newcastle University Medicine Malaysia, MalaysiaRoger Gutiérrez-Juárez, National Autonomous University of Mexico, Mexico
Copyright © 2024 Gray, Hack, Brandes, Zweig, Yang, Marney, Choi, Magana, Cerruti, McFerrin, Koike, Nguyen, Raber, Quinn, Maier and Soumyanath. This is an open-access article distributed under the terms of the Creative Commons Attribution License (CC BY). The use, distribution or reproduction in other forums is permitted, provided the original author(s) and the copyright owner(s) are credited and that the original publication in this journal is cited, in accordance with accepted academic practice. No use, distribution or reproduction is permitted which does not comply with these terms.
*Correspondence: Nora E. Gray, Z3JheW5Ab2hzdS5lZHU=
†Present address: Armando Alcazar Magana, Life Sciences Institute, University of British Columbia, Vancouver, BC, Canada