- 1Department of Pediatrics, University of Cincinnati College of Medicine, Cincinnati, OH, United States
- 2Division of Immunobiology Cincinnati Children’s Hospital Medical Center, Cincinnati, OH, United States
- 3Immunology Graduate Program and Medical Scientist Training Program, Cincinnati Children's Hospital Medical Center and The University of Cincinnati College of Medicine, Cincinnati, OH, United States
- 4Medical Scientist Training Program, Cincinnati Children’s Hospital Medical Center and The University of Cincinnati College of Medicine, Cincinnati, OH, United States
- 5Center for Inflammation and Tolerance, Cincinnati Children’s Hospital Medical Center, Cincinnati, OH, United States
- 6Center for Transplant Immunology, Cincinnati Children’s Hospital Medical Center, Cincinnati, OH, United States
Aging and obesity are two conditions characterized by chronic, low-grade inflammation. While both conditions are also associated with dysfunctional immune responses, the shared and distinct underlying mechanisms are just starting to be uncovered. In fact, recent findings have suggested that the effects of obesity on the immune system can be thought of as a state of accelerated aging. Here we propose that chronic, low-grade inflammation seen in obesity and aging is complex, affects multiple cell types, and results in an altered basal immune state. In aging, part of this altered state is the emergence of regulatory immune populations that lead to further immune dysfunction in an attempt to reduce chronic inflammation. While in obesity, part of the altered state is the effect of expanding adipose tissue on immune cell function. Thus, in this review, we compare, and contrast altered immune states in aging and obesity and discuss their potential contribution to a shared clinical problem- decreased vaccine responsiveness.
Introduction
First described in 2000, both chronic, low-grade inflammation, known as “inflammaging,” and age-related changes in the immune system, known as immunosenescence, are now recognized as hallmarks of the defective aging immune system (Franceschi et al., 2000a; Franceschi et al., 2000b). Inflammaging and immunosenescence create a balancing act that the aging immune system must contend with. These states are associated with increased rates of frailty, cardiovascular disease, Alzheimer’s disease, and susceptibility to infection (Giunta et al., 2008; North and Sinclair, 2012; Monti et al., 2017; Leonardi et al., 2018). Likely as a counter-response to low-grade chronic inflammation, we and others have recently demonstrated that aging also promotes the accumulation of anti-inflammatory cells and molecules, which in turn shape the landscape around age-related immune suppression (Sharma et al., 2006; Almanan et al., 2020).
Chronic inflammation is also a hallmark of obesity. Obesity-associated chronic inflammation is pathophysiologically linked to a variety of adverse sequelae, including metabolic syndrome, type II diabetes (T2D), dyslipidemia, non-alcoholic fatty liver disease (NAFLD), cardiovascular disease, Alzheimer’s disease, and diverse cancers (Calle et al., 2003; Weisberg et al., 2003; Strissel et al., 2007; Schenk et al., 2008). Like in aging, extensive characterization of the immune system in obesity has also revealed alteration of anti-inflammatory mechanisms (Pirola and Ferraz, 2017; Liu and Nikolajczyk, 2019), likely in response to obesity-driven low-grade chronic inflammation.
The immune system in obesity has been posited to display an “immunosenescence” phenotype, similar to that seen in aging (Shirakawa et al., 2016; Salvestrini et al., 2019), where cells become more broadly inflammatory even though their cell-specific functionality is altered. Nonetheless, parallels between the elevated basal rate of inflammation in aged and obesity-related inflammation remain insufficiently explored. It can be posited that the immune cells in aging and obesity become more inflammatory as a manner of trying to compensate for their loss of more specific and targeted functionality. Although immune systems of aged and obese individuals are similar in their inflammatory state, immunomodulatory responses of the immune system are different, suggesting aging and obesity use differing programs to quell the onslaught of inflammatory mediators. Though the pro- and anti-inflammatory responses differ somewhat in aging and obesity, they lead to similar outcomes inclusive of immune system dysfunction.
Notably, the mechanistic similarities and differences between the two chronic inflammatory states remain poorly understood, especially when the two conditions co-exist. In this review, we evaluate and compare inflammatory states of cells and mediators that compromise the aging and obese immune system. For simplicity, we describe their role in obesity and aging as either pro- or anti-inflammatory (Figure 1), although in reality inflammation exists on a sliding scale where each cell or mediator can have either pro- or anti-inflammatory roles given the right context. Finally, we compare the impact of altered immune states in both aging and obesity, utilizing decreased vaccine responsiveness as an exemplar. Furthermore, the underlying immune mechanisms linked to immune dysfunction in aging and obesity are likely to impact many other diseases associated with aging and/or obesity.
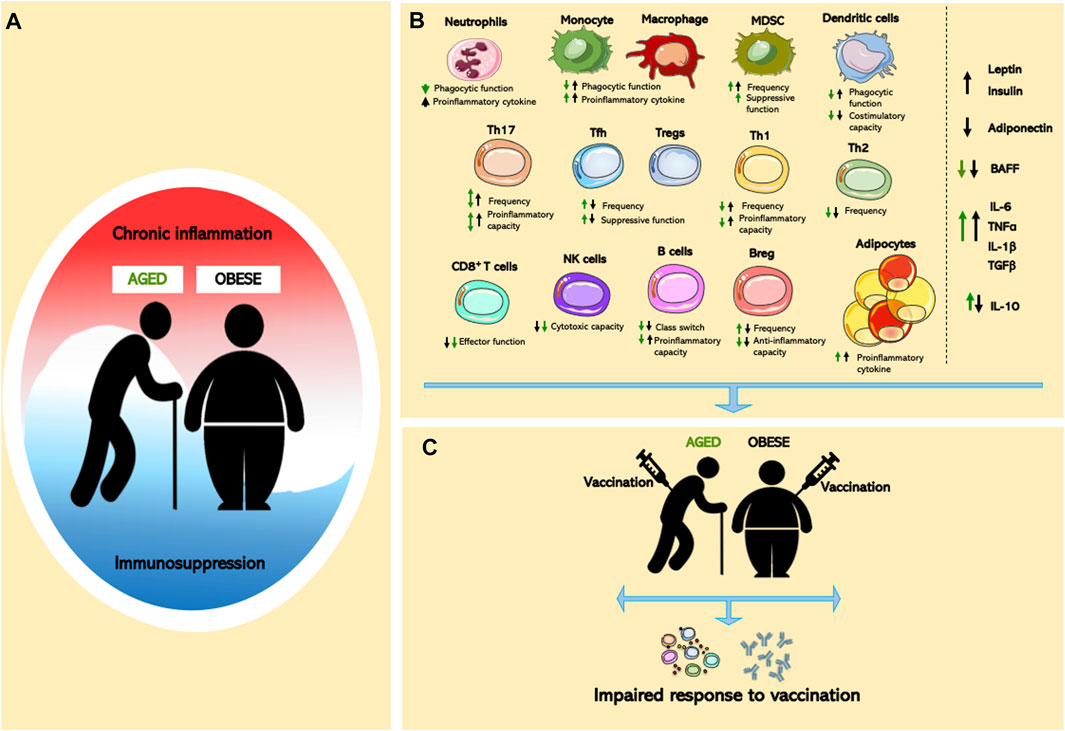
FIGURE 1. Effects of aging and obesity on immune system. (A) Chronic inflammation and immunosuppression are a dynamic process in aging and obesity. (B) Cellular dysregulated adipocytes, innate/adaptive immune cells, and pro- and anti-inflammatory mediators in aged or obese individuals lead to global immune cell dysfunction. (C) Immunological dysfunction contributes to impaired vaccine responses in age and obesity.
Aging-Associated Chronic Inflammation
Inflammaging is the chronic low-grade inflammation associated with aging (Franceschi et al., 2000b). Multiple age-associated diseases including cardiovascular diseases, neurodegenerative diseases, and various cancers are largely driven by this chronic inflammation (Giunta et al., 2008; North and Sinclair, 2012; Monti et al., 2017; Leonardi et al., 2018). Key inflammatory markers (e.g., Interleukin-6 [IL-6]) are elevated in aged humans and mice and contribute to inflammaging (Wei et al., 1992; Giuliani et al., 2001; Raynor et al., 2015). There are multiple theories surrounding the underlying mechanism and cellular source of increase inflammaging-associated inflammatory mediators. Senescent cells, obesity, increased gut permeability, changes to microbiota, inflammasome activation, oxidative stress caused, and chronic infections are likely major contributors. For instance, upon continual cellular stress (e.g., persistent DNA damage signaling, accumulation of reactive oxygen species [ROS], failure to remove defective cellular components) cells can exhibit senescence associated secretory phenotype (SASP), characterized by cellular growth arrest and a pro-inflammatory secretory phenotype (van Deursen, 2014). Senescent cells secrete many inflammatory mediators (e.g., IL-6, Interleukin-1 [IL-1] and colony stimulating factor [CSF]) (Garfinkel et al., 1994; Coppé et al., 2008), thus their accumulation might be directly responsible for systemic increases in inflammatory proteins with age. In addition, stressed senescent cells produce damage-associated molecular patterns (DAMPs) further activating immune cells pro-inflammatory programming (Huang et al., 2015). Moreover, persistent infections acquired throughout an individual’s lifespan exert a constant pressure on the immune system (Brunner et al., 2011; Minciullo et al., 2016). However, whether the presence of persistent infections contributes to age-related immune senescence remains under debate (Nikolich-Žugich, 2008).
Overall, immune cell functionality declines with aging while exhibiting a bias towards pro-inflammatory phenotypes, further contributing to inflammaging. Aging is associated with a shift in myeloid cells output by the bone marrow; however, these myeloid cells are largely dysfunctional (Pang et al., 2011). Published reports have shown that aged human monocytes shift to a more pro-inflammatory phenotype that correlates with increased production of IL-6, tumor necrosis factor (TNF), and IL-1β compared to their younger counterparts (Sadeghi et al., 1999). Also, neutrophil (Wenisch et al., 2000) and macrophage (Linehan et al., 2014; Wong et al., 2017) phagocytosis decreases with age, preventing bacterial clearance and timely and efficient removal of cellular debris in the case of injury or even normal tissue homeostasis. This results in increased prevalence of DAMPs that further amplifies pro-inflammatory pathways. Similarly, dendritic cell (DC) populations are altered in aging. Aged DCs are less phagocytic and less efficient in the cross-presentation of cell-associated antigens and subsequently in the cross-priming of CD8+ T cells and subsequent effector responses compared to their younger counterparts (Nikolich-Žugich et al., 2012; Chougnet et al., 2015). The aging T cell compartment produces more inflammatory cytokines compared to their younger counterparts (Fagiolo et al., 1993), and the involuted thymus produces more autoreactive T cells, which are also known to be more inflammatory (Coder et al., 2015). Furthermore, circulating CD4+ T cells from aged individuals display an increased Th17 associated cytokine profile, driven by increased mitochondrial dysfunction and ROS production (Bharath et al., 2020). Together these findings suggest that dysfunction in both the innate and adaptive immune compartments contribute to the inflammaging and immune dysfunction in the elderly.
Obesity-Induced Chronic Inflammation
Similar to chronic inflammation in aging, the obese chronic inflammatory state is central to the development of obesity-associated sequelae (e.g., T2D, and NAFLD) (Whitlock et al., 2009; Gregor and Hotamisligil, 2011). While obesity impacts circulating immune cells in both pro- and anti-inflammatory manners, nearly all the cellular populations present within adipose tissue develop an inflammatory phenotype (Elgazar-Carmon et al., 2008; Nishimura et al., 2009; Bertola et al., 2012; Talukdar et al., 2012; Shaikh et al., 2015; Wensveen et al., 2015; Lauterbach and Wunderlich, 2017; Russo and Lumeng, 2018; Zhao et al., 2018). Hence, it is not surprising that adipose tissue expansion is considered the key initiator of inflammation in obesity. The adipose tissue-associated inflammatory microenvironment shapes the chronic, low-grade inflammation in obesity with both adipose tissue-resident immune cells and inflammatory-skewed adipocytes contributing to the overall effect (Fontana et al., 2007; Reilly and Saltiel, 2017; Chan et al., 2020; Alarcon et al., 2021). Changes in the adipose tissue microenvironment result in increased volume of inflammatory cells and subsequent secretion of inflammatory mediators (e.g., Leptin, IL-6, IL-1β) (Skurk et al., 2007; Strissel et al., 2007; Grant and Dixit, 2015; Wueest and Konrad, 2018). Increased levels of these inflammatory mediators may also contribute to decreased adaptive immune function as plasma from obese individuals is sufficient to induce senescence in cytotoxic T cells (Parisi et al., 2017). Of note, as adipose tissue distribution varies by race (Stults-Kolehmainen et al., 2013), such changes could contribute to observed differences in immune responses between obese individuals of diverse races and ethnicities.
The impact of obesity on the innate immune compartment has been the most studied thus far. Published reports have shown increased total numbers of circulating monocytes, macrophages, and neutrophils in obesity (Roberts et al., 2018; Friedrich et al., 2019). Further, these innate immune populations are skewed towards a pro-inflammatory state, with macrophages being skewed towards an inflammatory phenotype and neutrophils having elevated cytokine, reactive oxygen species, and extracellular trap formation, contributing to elevated circulating levels of proinflammatory cytokines (e.g., IL-6, TNFα) (Lumeng, 2013; D'Abbondanza et al., 2019; de Heredia et al., 2012). However, obesity doesn’t impact all immune cells equally. Although increased numbers of DCs are observed in obesity, the chronic inflammation leads to impaired responsiveness of DCs to toll-like receptor (TLR) agonists (Pizzolla et al., 2016). The obesity-driven inflammation also negatively impacts effector function of other innate cells (e.g., NK cells), decreasing their total numbers and their cytotoxic potential (O'Shea and Hogan, 2019; Viel et al., 2017). Intriguingly, the impact of obesity on splenic NK cells closely resembles an aging-induced immunosenescent state (Gheorghe et al., 2017), further supporting the concept of obesity induced “accelerated aging” phenotype (Salvestrini et al., 2019).
Recent studies have also begun to explore obesity’s impact on adaptive immune cell function. Obesity-driven inflammation is also associated with decreased proliferation capability of naïve T cells (James et al., 2012). CD4+ helper T cells are skewed towards inflammatory subtypes, with increased Th17 and Th1 (McLaughlin et al., 2017; Moreno-Fernandez et al., 2021a) and decreased Th2 and Treg polarization (Zhao et al., 2018). CD8+ T cells are impacted similarly to NK cells, with decreased numbers and limited cytotoxic potential (Kado et al., 2019). In animal models of obesity, increases in adiposity and leukocyte infiltration that occur in the bone marrow negatively impact B cell bone marrow precursor populations (Adler et al., 2014). This results in B cells being skewed towards an inflammatory phenotype (Frasca et al., 2017a). This inflammatory skewing may lead to an increased population of exhausted memory B cells (Frasca et al., 2016; Frasca et al., 2017b), invoking the possibility that obesity-induced inflammatory states further exacerbate deficiencies in antibody responses and subsequently vaccine unresponsiveness. Importantly, B cells accumulate in the adipose tissue as a result of obesogenic diet (Duffaut et al., 2009; Winer et al., 2011). However, in human subcutaneous and visceral adipose tissue only a small fraction of B cells is observed (McDonnell et al., 2012; García-Rubio et al., 2018). Low frequency of B cells can likely be a consequence of low density of fat-associated lymphoid clusters in omental and subcutaneous human adipose tissues, which are structures associated with B cell accumulation Notably, B cell depletion improved insulin and glucose responses in obesity/T2D, which correlated with decreased inflammation (DeFuria et al., 2013). However, more work is required to mechanistically link chronic adipose tissue inflammation driven by the adaptive immune system to local and systemic immune cell dysfunction/senescence in human disease.
Aging-Associated Immunological Regulation
While aging and obesity are associated with markers of chronic inflammation, both states also lead to altered immunological regulation. Recent work, including findings from our group, have shown that alongside chronic inflammation activation of immune regulatory mechanisms and secretion of anti-inflammatory mediators are exacerbated in aging. Aged mice show an increase in myeloid-derived suppressor cells (MDSCs) in the spleen and peripheral lymph nodes (Heithoff et al., 2008; Enioutina et al., 2011). These aged MDSCs exhibit greater capacity to suppress T cell proliferation and cytotoxic function compared to their younger counterparts (Grizzle et al., 2007; Enioutina et al., 2011). Additionally, macrophages with anti-inflammatory characteristics, including increased secretion of Interleukin-10 (IL-10) and Transforming Growth Factor Beta (TGFβ) are more frequent in aged bone marrow, lymph nodes, and skeletal muscle (Jackaman et al., 2013; Wang et al., 2015).
Regarding the B cell and T cell compartment, regulatory B cells (Bregs) have been shown to increase in aging and conserve their suppressive function by producing similar IL-10 levels compared to young Breg cells (Mori et al., 2016; Freitas et al., 2019). Further, we and others have shown that, in mice and humans, regulatory T cells (Tregs, CD4+FOXP3+) accumulate with age (Nishioka et al., 2006; Sharma et al., 2006; Lages et al., 2008; Raynor et al., 2012). IL-6 drives the accumulation of Tregs suggesting this is a compensatory pathway attempting to dampen the chronic low-grade inflammation associated with aging (Raynor et al., 2015). Aged Tregs can 1) suppress the activation of DCs via decreased CD86 expression, 2) enhance suppression of effector T cell proliferation, and 3) secrete more IL-10 compared to younger counterparts (Garg et al., 2014). However, aged Tregs fail to suppress Th17 cells’ production of IL-17 during autoimmune inflammation (Sun et al., 2012). Together these data suggest that aging Treg have an altered functional profile.
More recently, we show that IL-10 actively suppresses vaccine responses in aged mice as neutralization of IL-10 restored antigen-specific antibody levels to nearly those observed in young mice (Almanan et al., 2020). In this study, the greatest producer of IL-10 in aged mice was a novel population of T follicular helper cells, which we called Tfh10 cells. These Tfh10 cells appear to regulate the systemic IL-6:IL-10 balance which is crucial to healthy aging (Monti et al., 2017; Almanan et al., 2020). Our data also showed that IL-10R blockade resulted in an increase in antigen-specific, germinal center B cells (Almanan et al., 2020), suggesting this accumulation of Tfh10 cells in aging is drastically dampening B cell responses. These B cell responses are vital for producing strong antibodies in both a vaccine and infection setting, both of which significantly decrease with age (Frasca and Blomberg, 2011; Simell et al., 2011; Frasca and Blomberg, 2020). Combined, our data suggest that there is active immune suppression in aging, reversable by neutralization of a single cytokine, IL-10, which is sufficient to restore antibody responses in aged mice. Another IL-10 cellular source is T follicular regulatory (Tfr) cells, a novel CD4+ T cells population that are FoxP3+ and Bcl6+ and express high levels of PD-1 and CXCR5, have been shown to be critical for regulating germinal center B cell reactions such as plasmablast formation, affinity maturation, and class switching (Frasca et al., 2004; Frasca et al., 2011). Tfr cells frequency was reported to be increased in aged mice and humans (Sage et al., 2015; Lefebvre et al., 2016a). Although, at the cellular level Tfr cells were found to display impaired suppressive function due to their age-related decrease in IL-10 production (Lefebvre et al., 2016a; Ito et al., 2019), their suppressive function in aging is associated with expansion. Indeed, we recently reported increased serum IL-10 levels in aged mice (Almanan et al., 2020).
Although inflammaging is well characterized, there is evidence that both pro- and anti-inflammatory immune programs are present in aging, thus a more detailed investigation of how the anti-inflammatory arm of the immune system is regulated in aging and how it interacts with age-associated chronic inflammation is needed. Logically, the overabundance of pro-inflammatory mediators that overwhelm an aged individual is bound to elicit a “brake” response, by upregulating anti-inflammatory cells and mediators. Thus, an improved understanding of the interplay of pro- and anti-inflammatory immune programs in aging may provide important insights into overall immune function and potential revitalization in aging.
Obesity Induced Alteration in Anti-inflammatory Mediators
Obesity is also associated with alterations in multiple types of immunoregulatory cells and mediators, including Tregs, Bregs, MDSC, anti-inflammatory macrophages and IL-10. While such immune changes plausibly contribute to the persistent low-grade immune activation associated with the obesogenic state, how such changes are modified in elderly obese and the contribution of their effects on metabolic disease remains unclear.
Treg frequency declines in the peripheral blood in obese humans (Cortez-Espinosa et al., 2015; Yuan et al., 2018) and in the adipose tissue (Wu et al., 2019; Smith et al., 2020). Furthermore, metabolic decline in individuals newly diagnosed with obesity-driven T2D is associated with reduced circulating Treg frequencies (Yuan et al., 2018). In animal models of obesity driven metabolic disease, in homeostatic condition (lean state), Treg cell numbers are expanded in adipose tissue compared to the obesogenic state, where Treg frequency decreased (Feuerer et al., 2009; Bapat et al., 2015). Treg cells play a protective role in insulin sensitivity and energy homeostasis in obesity (Ilan et al., 2010). Increased adipose inflammation was observed in Treg-depleted mice and altered glucose metabolism was ameliorated in obese mice after adoptive transfer of Treg cells (Feuerer et al., 2009; Eller et al., 2011). As Treg frequency differs in a gender dependent manner (Ishikawa et al., 2020; Vasanthakumar et al., 2020), the impact of obesity on Treg homeostasis may differ between genders. Of note, female mice display lower visceral adipose tissue Treg frequency than male mice (Vasanthakumar et al., 2020). This was associated with estrogen levels, as visceral adipose tissue Tregs frequencies increases in estrogen receptor alpha-depleted or testosterone-treated female mice (Vasanthakumar et al., 2020). Treg accrual is also reduced in adipose tissue in obese male mice compared to lean animals (Feuerer et al., 2009; Ishikawa et al., 2020). In contrast, obesogenic diet feeding promoted adipose tissue Treg expansion in female mice (Ishikawa et al., 2020), which was associated with limited induction of metabolic diseases (Moreno-Fernandez et al., 2021b). Thus, change in female sex hormones during aging may be implicated in altered adiposity and Treg frequency in the context of increase adiposity during aging. Further, the above mentioned hormonal effect on the immune system may explain lower propensity of females to age-related metabolic alterations in the elderly.
In addition to altered Treg homeostasis, Treg function is impacted in obesity. Numbers of Tregs expressing CD39, an immunomodulatory ecto-5′- nucleotidases, decline in obese individuals who have T2D (Cortez-Espinosa et al., 2015). Importantly, high levels of expression of PD1 and T cell immunoreceptor with Ig and ITIM domains (TIGIT) is observed on Tregs as well as other CD4+ T cells in the adipose tissue of obese humans and mice (Smith et al., 2020; Porsche et al., 2021). However, the role of PD1 pathway is not clear, since PD1 blockade did not affect T cell function and metabolic alteration in obese mice (Porsche et al., 2021). These data suggest that although T cells PD1 is increased additional mechanisms may contribute to T cell exhaustion in obesity.
Increased insulin levels in obesity, a consequence of insulin resistance, may play an important role in shaping Tregs function. Treg sensing of insulin is important as deletion of the insulin receptor in Tregs improved glucose tolerance and insulin sensitivity and increased numbers of IL-10 producing Tregs in adipose tissue of obese animals (Wu et al., 2020). Additionally, insulin administration decreased IL-10-expressing Tregs and diminished Treg capacity to suppress macrophage function (Han et al., 2014). Similarly, levels of leptin, an adipokine, remain high during obesity as a consequence of leptin resistance. Leptin can skew the T cell balance towards an inflammatory state favoring Th17 cell differentiation (Reis et al., 2015) at the expense of Tregs. Hence, circulating leptin levels inversely correlate with circulating Tregs frequency (Wagner et al., 2013). In contrast, levels of adiponectin, another adipokine, are decreased in the obese state (Musovic and Olofsson, 2019). Adiponectin has anti-inflammatory properties that limit production of reactive oxygen species and Th1 cell polarization (Robinson et al., 2011). Combined, these data suggest that increased insulin and leptin in conjunction with decreased adiponectin in the context of obesity likely shifts the balance towards a more pathogenic/pro-inflammatory environment. These responses may influence Treg differentiation and further promote the accrual of inflammatory T cells.
Bregs are also impacted by obesity. Bregs, a subpopulation of B cells characterized by IL-10 production, play a critical role in the differentiation and maintenance of Tregs and in the suppression of T cell responses (Mizoguchi et al., 2000). Obese individuals have a decreased circulating Breg frequency (García-Hernández et al., 2018). In animal models, Breg numbers are decreased in the adipose tissue of obese animals compared to lean controls. Bregs restrict adipose tissue inflammation and insulin resistance in obese mice in an IL-10 dependent manner (Nishimura et al., 2013). Adoptive transfer of adipose tissue Bregs ameliorated those effects and maintained metabolic homeostasis in the lean adipose tissue (Nishimura et al., 2013). Additionally, CD40 or BCR stimulation of purified B cells from obese/T2D subjects led to decreased IL-10 production (Zhai et al., 2016). These data suggest that the impaired ability to secrete IL-10 and TGFβ by B cells in obese and T2D individuals could be linked to decreased Breg numbers and function in obesity and increased overall inflammation. Of note, reduced serum IL-10 levels are observed in patients with obesity-driven T2D (Yuan et al., 2018). IL-10 is a protective factor against diet-induced insulin resistance in the liver and in skeletal muscle as it attenuates macrophage cytokine secretion (Hong et al., 2009). Thus it would be important to determine whether increased IL-10 levels in aging, may play a beneficial role in the context of obesity-driven metabolic disease by ameliorating disease severity (Moreno-Fernandez et al., 2021b). In addition, B cell function is linked with leptin and BAFF levels (Frasca and Blomberg, 2020). BAFF levels are decreased in aging and in obese mice (Jin et al., 2008; Kim et al., 2009). Of note, increased BAFF levels has been implicated in the modulation of weight gain in mice and humans (Chan et al., 2021). Given that aged and obese individuals exhibit reduced antibody responses, the potentially unifying role of BAFF in aging and obesity should be further explored. Likewise, Breg development in obesity could be affected by the impact of obesity in Tfh cells (Garner-Spitzer et al., 2020). A recent study revealed that bariatric surgery (and subsequent weight loss) resulted in an increase of less inflammatory Tfh cells that had better capability to promote the development of Bregs (Zhan et al., 2017), suggesting that Tfh function during obesity is shifted, which may act as a rheostat that regulates overall inflammation.
Contrary to their other immunoregulatory counterparts, MDSC are poorly studied in obesity. Increased frequency of monocytic CD11b+CD33+CD14+HLADRlow/- MDSC was observed in the peripheral blood of obese individuals (Bao et al., 2015). In mouse models of obesity, a MDSC population expressing Gr-1 and CD11b is highly enriched in the liver and adipose tissue. This particular MDSC population suppressed CD8+ T cell and inflammatory macrophage function in obesity (Xia et al., 2011), suggesting that MDSC may act as another important counter regulatory mechanism of exacerbated inflammatory immune responses in obesity. Overall, though aging and obesity’s effect on immunomodulatory regulation differ, they both are associated with immune cell dysfunctionality, leading to abnormal immune responses.
Inflammation-Induced Immunomodulation: Linking Aging and Obesity
Intriguing unifying parallels between aging- and obesity-dependent impact in the ability of the immune system to properly function and leading to dysfunctional responses warrant further investigation. Examining these trends is critical for understanding the differences and similarities between the associated-pathologies shared by both states. Of significant interest is the intersection of these two states and how immune alterations differ in those who are both elderly and obese in comparison to those that are only elderly or obese. However, many of the studies perform so far have largely focused on either individual state rather than on overlapping conditions. Therefore, which immunomodulatory program obese elderly individuals adopt, and how this helps them compensate for the compounded chronic inflammation are key questions that remain unanswered. Of note, use of thermoneutral housing allows for studying obesity and aging in unison in both male and female mice (Giles et al., 2017; Moreno-Fernandez et al., 2021b). Thus, this new model may allow for future interrogations of obesity and aging in both sexes and may lead to discovery of new underlying mechanisms linking obesity and aging.
One highly clinically relevant similarity between obesity and aging is their impact on vaccine-driven immune responses. Vaccines have saved hundreds of millions of lives by reducing disease mortality and morbidity. However, both elderly and obese individuals have decreased vaccine responsiveness. For example, yearly influenza vaccines provide 65–80% protection for young individuals while only about 30–50% protection to their older counterparts (Nichol et al., 2007). This lack of protection in aged individuals is largely attributed to age-related dysfunction in B and T cells that leads to a decline in antibody responses to the influenza vaccine (Goronzy et al., 2001; Frasca et al., 2010). Aged B cells accumulate intrinsic defects leading to decreased influenza-specific antibody titers, decreased induction of AID (activation-induced cytidine deaminase), a known inducer of Ig class-switch recombination and somatic hypermutation, and decreased memory induction (Frasca et al., 2010). Additionally, intrinsic defects in aged T cells have been implicated in the loss of influenza vaccine protection. Shifts in the aging CD4+ T cell compartment towards Tfh cells, instead of Th1 cells, have been proposed as a mechanism of decreased vaccine efficacy, as Th1 cells are required for effective influenza clearance in the lung (Lefebvre et al., 2016b). Additionally, as mentioned earlier excessive IL-10 production in aging suppresses vaccine responses in mice (Almanan et al., 2020). Other vaccines show similar decreased efficacy with aging. Hepatitis A and hepatitis B vaccines induce poor antibody responses in the elderly leading to a decrease in vaccine efficacy from 92% in young individuals to 63% for hepatitis A and 67–33% for hepatitis B respectively (Wolters et al., 2003). Additionally, the mRNA-1273 COVID-19 vaccine efficacy drops from 95.6% in individuals under 65 years old to 86.4% in those 65 years and older (Baden et al., 2021). Together, these studies suggest that the altered immune state in aged individuals severely limits their ability to produce adequate and appropriate immune responses, thus leaving these highly vulnerable populations without the protection that vaccines normally provide.
Similar to aged individuals, the impact of obesity on vaccine efficacy is well-reported (Painter et al., 2015). Obese individuals have decreased antibody levels and an overall faster decline in protective levels of antibodies than their lean counterparts for many vaccines including rabies (Banga et al., 2014), Hepatitis A (Reuman et al., 1997), Hepatitis B (Weber et al., 1985) or tetanus (Eliakim et al., 2006) vaccines. With regards to these normally highly efficient vaccines, obesity-associated chronic inflammation might detrimentally influence the longevity of adaptive immune cells that are known to be impacted by obesity. In addition, obese adults have double the risk of developing influenza-associated pneumonia despite having similar antibody titers to their lean counterparts (Green and Beck, 2017). In this case, worsened disease outcomes (e.g., impaired limitation of viral propagation, lung tissue pathology) have been attributed to the impaired cytotoxicity of NK cells in obese individuals (O'Shea and Hogan, 2019), and/or increased inflammatory propensity of lung macrophages and neutrophils (Narasaraju et al., 2011) in obese individuals. Contribution of obesity-altered adipocytes has also been evoked (Chan et al., 2019; Chan et al., 2020; Chan et al., 2021; Alarcon et al., 2021). This trend might be due to obesity-specific mechanisms, as T2D individuals have similar responsiveness to the influenza vaccine as non T2D individuals (Sheridan et al., 2015; Dos Santos et al., 2018). Nevertheless, it is important to consider these clinically relevant epidemiological trends, as they might have impact on current and future vaccination efforts, such as for SARS-CoV-2, where obese individuals are an “at risk” group (Popkin et al., 2020).
Although there is some evidence to indicate that aging with comorbidities such as obesity leads to worse outcomes in vaccine efficacy, the interplay between both obesity and aging have been largely understudied. Many reports of vaccine efficacy fail to report data of individuals that are both elderly and obese making it difficult to understand the respective and combined impact of these two states. However, recent preliminary studies have begun to include these analyses. For instance, it was suggested that the Johnson and Johnson COVID-19 vaccine efficacy 28 post vaccination could drop to 42.3% in elderly patients with comorbidities including obesity, compared to 72.4% efficacy in healthy elderly patients and 68.0% in healthy, young adults (Administration FaD, 2021). Hence the combinatory effects of both aging and obesity may exacerbate the worsened immune dysfunctional environment than either obesity or aging alone. Thus, further exploration and data analysis in this area are needed as the obese, elderly, and obese-elderly populations continue to increase.
Importantly, these clinical trends might be attributed to a unifying impact of aging- and obesity-inflammation-dependent skewing of the innate and adaptive immune response. However, we also acknowledge that while chronic inflammation appears to drive any similar immune phenotypes, differences do exist, likely driven by mechanism specific to each state. More research using multiomics approaches such as IMM-AGE study (Alpert et al., 2019) will be of great importance to decipher precise unique and share pathways activated in each individual or combine state over time. Further, uncovering of similarities and differences between these two states will also help to uncover whether anti-inflammatory treatment targeted towards amelioration of metabolic dysfunction could be exploitable for aging and/or aging/obesity associated inflammation and improving vaccine responses in these populations. For instance, metformin appears promising for treatment of chronic inflammatory diseases associated with both aging and obesity in pre-clinical models (Bharath et al., 2020). Notably, pioglitazone alone or in combination with metformin has also been shown to have anti-inflammatory properties (Zhang et al., 2008; Shen et al., 2018) and decrease inflammatory mediators in patients with T2D (Forst et al., 2008; Schöndorf et al., 2011). Undeniably, it is attracting to think about repurposing such drugs to improve vaccine efficacy in the elderly and/or obese. For example, preliminary studies of pioglitazone in experimental influenza infection improved survival by favoring protective and limiting exacerbated immune responsiveness in mice (Aldridge et al., 2009; Moseley et al., 2010). A better understanding of these mechanisms is thus imperative to provide better vaccine regimens for obese, elderly, or obese-elderly individuals.
Concluding Remarks
Aging- and obesity-associated chronic inflammation and potential immunosuppression have a profound impact on the functionality of the immune system (Figure 1A). Both conditions exist as a mixed inflammatory state, where pro- and anti-inflammatory cells and mediators co-exist and contribute to the development of aging- and obesity-associated disease (Figure 1B). While similarities between the aged and obese immune systems have been noted, the literature concerning the impact of combined aged and obese state is limited, something that will be critically important to combat major health issues including vaccine efficacy (Figure 1C). Thus, further examination of the parallels between the aged and obese immune system are needed to identify critical inflammatory mechanistic links. Such studies might provide novel therapeutic approaches to ameliorate the clinical burden of disease in an increasingly obese world that continues to age.
Author Contributions
MEM-F, ALT, and PCA contributed equally in the writing of the manuscript. CAC, SD, and DAH contributed equally in editing the manuscript.
Funding
This research was supported by Public Health Service Grants AG033057, AG053498, a Catalyst Award from the Falk Foundation (to DH, CC); DK099222 (to SD); CCRF Endowed Scholar Award (to SD); ADA 1-19-PMF-019 (to MM-F) and T32GM063483-14 (associated with PA).
Conflict of Interest
The authors declare that the research was conducted in the absence of any commercial or financial relationships that could be construed as a potential conflict of interest.
Publisher’s Note
All claims expressed in this article are solely those of the authors and do not necessarily represent those of their affiliated organizations, or those of the publisher, the editors and the reviewers. Any product that may be evaluated in this article, or claim that may be made by its manufacturer, is not guaranteed or endorsed by the publisher.
References
Adler, B. J., Green, D. E., Pagnotti, G. M., Chan, M. E., and Rubin, C. T. (2014). High fat diet rapidly suppresses B lymphopoiesis by disrupting the supportive capacity of the bone marrow niche. PLoS One 9 (3), e90639. doi:10.1371/journal.pone.0090639
Administration FaD (2021). Janssen Ad26.COV2.S Vaccine for the Prevention of COVID-19. Vaccin. Relat. Biol. Prod. Advisory Committee Meet., 1–62.
Alarcon, P. C., Damen, M., Madan, R., Deepe, G. S., Spearman, P., Way, S. S., et al. (2021). Adipocyte inflammation and pathogenesis of viral pneumonias: an overlooked contribution. Mucosal. Immunol. 6, 1–11.
Aldridge, J. R., Moseley, C. E., Boltz, D. A., Negovetich, N. J., Reynolds, C., Franks, J., et al. (2009). TNF/iNOS-producing dendritic cells are the necessary evil of lethal influenza virus infection. Proc. Natl. Acad. Sci. 106 (13), 5306–5311. doi:10.1073/pnas.0900655106
Almanan, M., Raynor, J., Ogunsulire, I., Malyshkina, A., Mukherjee, S., Hummel, S. A., et al. (2020). IL-10-producing Tfh cells accumulate with age and link inflammation with age-related immune suppression. Sci. Adv. 6 (31), eabb0806. doi:10.1126/sciadv.abb0806
Alpert, A., Pickman, Y., Leipold, M., Rosenberg-Hasson, Y., Ji, X., Gaujoux, R., et al. (2019). A clinically meaningful metric of immune age derived from high-dimensional longitudinal monitoring. Nat. Med. 25 (3), 487–495. doi:10.1038/s41591-019-0381-y
Baden, L. R., El Sahly, H. M., Essink, B., Kotloff, K., Frey, S., Novak, R., et al. (2021). Efficacy and Safety of the mRNA-1273 SARS-CoV-2 Vaccine. N. Engl. J. Med. 384 (5), 403–416. doi:10.1056/nejmoa2035389
Banga, N., Guss, P., Banga, A., and Rosenman, K. D. (2014). Incidence and variables associated with inadequate antibody titers after pre-exposure rabies vaccination among veterinary medical students. Vaccine 32 (8), 979–983. doi:10.1016/j.vaccine.2013.12.019
Bao, Y., Mo, J., Ruan, L., and Li, G. (2015). Increased monocytic CD14+HLADRlow/− myeloid-derived suppressor cells in obesity. Mol. Med. Rep. 11 (3), 2322–2328. doi:10.3892/mmr.2014.2927
Bapat, S. P., Myoung Suh, J., Fang, S., Liu, S., Zhang, Y., Cheng, A., et al. (2015). Depletion of fat-resident Treg cells prevents age-associated insulin resistance. Nature 528 (7580), 137–141. doi:10.1038/nature16151
Bertola, A., Ciucci, T., Rousseau, D., Bourlier, V., Duffaut, C., Bonnafous, S., et al. (2012). Identification of adipose tissue dendritic cells correlated with obesity-associated insulin-resistance and inducing Th17 responses in mice and patients. Diabetes 61 (9), 2238–2247. doi:10.2337/db11-1274
Bharath, L. P., Agrawal, M., McCambridge, G., Nicholas, D. A., Hasturk, H., Liu, J., et al. (2020). Metformin Enhances Autophagy and Normalizes Mitochondrial Function to Alleviate Aging-Associated Inflammation. Cel Metab. 32 (1), 44–55. doi:10.1016/j.cmet.2020.04.015
Brunner, S., Herndler-Brandstetter, D., Weinberger, B., and Grubeck-Loebenstein, B. (2011). Persistent viral infections and immune aging. Ageing Res. Rev. 10 (3), 362–369. doi:10.1016/j.arr.2010.08.003
Calle, E. E., Rodriguez, C., Walker-Thurmond, K., and Thun, M. J. (2003). Overweight, obesity, and mortality from cancer in a prospectively studied cohort of U.S. adults. N. Engl. J. Med. 348 (17), 1625–1638. doi:10.1056/nejmoa021423
Chan, C. C., Damen, M. S. M. A., Alarcon, P. C., Sanchez-Gurmaches, J., and Divanovic, S. (2019). Inflammation and Immunity: From an Adipocyte's Perspective. J. Interferon Cytokine Res. 39 (8), 459–471. doi:10.1089/jir.2019.0014
Chan, C. C., Damen, M., Moreno-Fernandez, M. E., Stankiewicz, T. E., Cappelletti, M., Alarcon, P. C., et al. (2020). Type I Interferon Sensing Unlocks Dormant Adipocyte Inflammatory Potential. Nat. Commun. 11 (1), 2745.
Chan, C. C., Harley, I. T. W., Pfluger, P. T., Trompette, A., Stankiewicz, T. E., Allen, J. L., et al. (2021). A BAFF/APRIL axis regulates obesogenic diet-driven weight gain. Nat. Commun. 12 (1), 2911. doi:10.1038/s41467-021-23084-1
Chougnet, C. A., Thacker, R. I., Shehata, H. M., Hennies, C. M., Lehn, M. A., Lages, C. S., et al. (2015). Loss of Phagocytic and Antigen Cross-Presenting Capacity in Aging Dendritic Cells Is Associated with Mitochondrial Dysfunction. J.I. 195 (6), 2624–2632. doi:10.4049/jimmunol.1501006
Coder, B. D., Wang, H., Ruan, L., and Su, D.-M. (2015). Thymic Involution Perturbs Negative Selection Leading to Autoreactive T Cells that Induce Chronic Inflammation. J.I. 194 (12), 5825–5837. doi:10.4049/jimmunol.1500082
Coppé, J.-P., Patil, C. K., Rodier, F., Sun, Y., Muñoz, D. P., Goldstein, J., et al. (2008). Senescence-associated secretory phenotypes reveal cell-nonautonomous functions of oncogenic RAS and the p53 tumor suppressor. Plos Biol. 6 (12), e301. doi:10.1371/journal.pbio.0060301
Cortez-Espinosa, N., Cortés-Garcia, J. D., Martínez-Leija, E., Rodríguez-Rivera, J. G., Barajas-López, C., González-Amaro, R., et al. (2015). CD39 expression on Treg and Th17 cells is associated with metabolic factors in patients with type 2 diabetes. Hum. Immunol. 76 (9), 622–630. doi:10.1016/j.humimm.2015.09.007
D'Abbondanza, M., Martorelli, E. E., Ricci, M. A., De Vuono, S., Migliola, E. N., Godino, C., et al. (2019). Increased plasmatic NETs by-products in patients in severe obesity. Sci. Rep. 9 (1), 14678. doi:10.1038/s41598-019-51220-x
de Heredia, F. P., Gómez-Martínez, S., and Marcos, A. (2012). Obesity, inflammation and the immune system. Proc. Nutr. Soc. 71 (2), 332–338. doi:10.1017/s0029665112000092
DeFuria, J., Belkina, A. C., Jagannathan-Bogdan, M., Snyder-Cappione, J., Carr, J. D., Nersesova, Y. R., et al. (2013). B cells promote inflammation in obesity and type 2 diabetes through regulation of T-cell function and an inflammatory cytokine profile. Proc. Natl. Acad. Sci. 110 (13), 5133–5138. doi:10.1073/pnas.1215840110
Dos Santos, G., Tahrat, H., and Bekkat-Berkani, R. (2018). Immunogenicity, safety, and effectiveness of seasonal influenza vaccination in patients with diabetes mellitus: A systematic review. Hum. Vaccin. Immunother. 14 (8), 1853–1866. doi:10.1080/21645515.2018.1446719
Duffaut, C., Galitzky, J., Lafontan, M., and Bouloumié, A. (2009). Unexpected trafficking of immune cells within the adipose tissue during the onset of obesity. Biochem. Biophysical Res. Commun. 384 (4), 482–485. doi:10.1016/j.bbrc.2009.05.002
Elgazar-Carmon, V., Rudich, A., Hadad, N., and Levy, R. (2008). Neutrophils transiently infiltrate intra-abdominal fat early in the course of high-fat feeding. J. Lipid Res. 49 (9), 1894–1903. doi:10.1194/jlr.m800132-jlr200
Eliakim, A., Swindt, C., Zaldivar, F., Casali, P., and Cooper, D. M. (2006). Reduced tetanus antibody titers in overweight children. Autoimmunity 39 (2), 137–141. doi:10.1080/08916930600597326
Eller, K., Kirsch, A., Wolf, A. M., Sopper, S., Tagwerker, A., Stanzl, U., et al. (2011). Potential role of regulatory T cells in reversing obesity-linked insulin resistance and diabetic nephropathy. Diabetes 60 (11), 2954–2962. doi:10.2337/db11-0358
Enioutina, E. Y., Bareyan, D., and Daynes, R. A. (2011). A Role for Immature Myeloid Cells in Immune Senescence. J.I. 186 (2), 697–707. doi:10.4049/jimmunol.1002987
Fagiolo, U., Cossarizza, A., Scala, E., Fanales-Belasio, E., Ortolani, C., Cozzi, E., et al. (1993). Increased cytokine production in mononuclear cells of healthy elderly people. Eur. J. Immunol. 23 (9), 2375–2378. doi:10.1002/eji.1830230950
Feuerer, M., Herrero, L., Cipolletta, D., Naaz, A., Wong, J., Nayer, A., et al. (2009). Lean, but not obese, fat is enriched for a unique population of regulatory T cells that affect metabolic parameters. Nat. Med. 15 (8), 930–939. doi:10.1038/nm.2002
Fontana, L., Eagon, J. C., Trujillo, M. E., Scherer, P. E., and Klein, S. (2007). Visceral Fat Adipokine Secretion Is Associated with Systemic Inflammation in Obese Humans. Diabetes 56 (4), 1010–1013. doi:10.2337/db06-1656
Forst, T., Karagiannis, E., Lübben, G., Hohberg, C., Schöndorf, T., Dikta, G., et al. (2008). Pleiotrophic and anti-inflammatory effects of pioglitazone precede the metabolic activity in type 2 diabetic patients with coronary artery disease. Atherosclerosis 197 (1), 311–317. doi:10.1016/j.atherosclerosis.2007.05.006
Franceschi, C., Bonafè, M., Valensin, S., Olivieri, F., De Luca, M., Ottaviani, E., et al. (2000). Inflamm-aging. An evolutionary perspective on immunosenescence. Ann. N. Y Acad. Sci. 908 (1), 244–254. doi:10.1111/j.1749-6632.2000.tb06651.x
Franceschi, C., Bonafè, M., and Valensin, S. (2000). Human immunosenescence: the prevailing of innate immunity, the failing of clonotypic immunity, and the filling of immunological space. Vaccine 18 (16), 1717–1720. doi:10.1016/s0264-410x(99)00513-7
Frasca, D., and Blomberg, B. B. (2020). Aging induces B cell defects and decreased antibody responses to influenza infection and vaccination. Immun. Ageing 17 (1), 37–10. doi:10.1186/s12979-020-00210-z
Frasca, D., Blomberg, B. B., and Paganelli, R. (2017). Aging, Obesity, and Inflammatory Age-Related Diseases. Front. Immunol. 8 (1745), 1745. doi:10.3389/fimmu.2017.01745
Frasca, D., and Blomberg, B. B. (2011). Aging affects human B cell responses. J. Clin. Immunol. 31 (3), 430–435. doi:10.1007/s10875-010-9501-7
Frasca, D., Diaz, A., Romero, M., Landin, A. M., and Blomberg, B. B. (2011). Age effects on B cells and humoral immunity in humans. Ageing Res. Rev. 10 (3), 330–335. doi:10.1016/j.arr.2010.08.004
Frasca, D., Diaz, A., Romero, M., Landin, A. M., Phillips, M., Lechner, S. C., et al. (2010). Intrinsic defects in B cell response to seasonal influenza vaccination in elderly humans. Vaccine 28 (51), 8077–8084. doi:10.1016/j.vaccine.2010.10.023
Frasca, D., Diaz, A., Romero, M., Vazquez, T., and Blomberg, B. B. (2017). Obesity induces pro-inflammatory B cells and impairs B cell function in old mice. Mech. Ageing Dev. 162, 91–99. doi:10.1016/j.mad.2017.01.004
Frasca, D., Ferracci, F., Diaz, A., Romero, M., Lechner, S., and Blomberg, B. B. (2016). Obesity decreases B cell responses in young and elderly individuals. Obesity 24 (3), 615–625. doi:10.1002/oby.21383
Frasca, D., Van der Put, E., Riley, R. L., and Blomberg, B. B. (2004). Reduced Ig class switch in aged mice correlates with decreased E47 and activation-induced cytidine deaminase. J. Immunol. 172 (4), 2155–2162. doi:10.4049/jimmunol.172.4.2155
Freitas, G. R. R., da Luz Fernandes, M., Agena, F., Jaluul, O., Silva, S. C., Lemos, F. B. C., et al. (2019). Aging and End Stage Renal Disease Cause A Decrease in Absolute Circulating Lymphocyte Counts with A Shift to A Memory Profile and Diverge in Treg Population. Aging Dis. 10 (1), 49–61. doi:10.14336/ad.2018.0318
Friedrich, K., Sommer, M., Strobel, S., Thrum, S., Blüher, M., Wagner, U., et al. (2019). Perturbation of the Monocyte Compartment in Human Obesity. Front. Immunol. 10, 1874. doi:10.3389/fimmu.2019.01874
García-Hernández, M. H., Rodríguez-Varela, E., García-Jacobo, R. E., Hernández-De la Torre, M., Uresti-Rivera, E. E., González-Amaro, R., et al. (2018). Frequency of regulatory B cells in adipose tissue and peripheral blood from individuals with overweight, obesity and normal-weight. Obes. Res. Clin. Pract. 12 (6), 513–519. doi:10.1016/j.orcp.2018.07.001
García-Rubio, J., León, J., Redruello-Romero, A., Pavón, E., Cozar, A., Tamayo, F., et al. (2018). Cytometric analysis of adipose tissue reveals increments of adipocyte progenitor cells after weight loss induced by bariatric surgery. Sci. Rep. 8 (1), 15203. doi:10.1038/s41598-018-33488-7
Garfinkel, S., Brown, S., Wessendorf, J. H., and Maciag, T. (1994). Post-transcriptional regulation of interleukin 1 alpha in various strains of young and senescent human umbilical vein endothelial cells. Proc. Natl. Acad. Sci. 91 (4), 1559–1563. doi:10.1073/pnas.91.4.1559
Garg, S. K., Delaney, C., Toubai, T., Ghosh, A., Reddy, P., Banerjee, R., et al. (2014). Aging is associated with increased regulatory T ‐cell function. Aging cell 13 (3), 441–448. doi:10.1111/acel.12191
Garner-Spitzer, E., Poellabauer, E.-M., Wagner, A., Guzek, A., Zwazl, I., Seidl-Friedrich, C., et al. (2020). Obesity and Sex Affect the Immune Responses to Tick-Borne Encephalitis Booster Vaccination. Front. Immunol. 11, 860. doi:10.3389/fimmu.2020.00860
Gheorghe, A., Pérez de Heredia, F., Hunsche, C., Redondo, N., Díaz, L. E., Hernández, O., et al. (2017). Oxidative stress and immunosenescence in spleen of obese mice can be reversed by 2‐hydroxyoleic acid. Exp. Physiol. 102 (5), 533–544. doi:10.1113/ep086157
Giles, D. A., Moreno-Fernandez, M. E., Stankiewicz, T. E., Graspeuntner, S., Cappelletti, M., Wu, D., et al. (2017). Thermoneutral housing exacerbates nonalcoholic fatty liver disease in mice and allows for sex-independent disease modeling. Nat. Med. 23 (7), 829–838. doi:10.1038/nm.4346
Giuliani, N., Sansoni, P., Girasole, G., Vescovini, R., Passeri, G., Passeri, M., et al. (2001). Serum interleukin-6, soluble interleukin-6 receptor and soluble gp130 exhibit different patterns of age- and menopause-related changes. Exp. Gerontol. 36 (3), 547–557. doi:10.1016/s0531-5565(00)00220-5
Giunta, B., Fernandez, F., Nikolic, W. V., Obregon, D., Rrapo, E., Town, T., et al. (2008). Inflammaging as a prodrome to Alzheimer's disease. J. Neuroinflammation 5 (1), 51–15. doi:10.1186/1742-2094-5-51
Goronzy, J. J., Fulbright, J. W., Crowson, C. S., Poland, G. A., O'Fallon, W. M., and Weyand, C. M. (2001). Value of Immunological Markers in Predicting Responsiveness to Influenza Vaccination in Elderly Individuals. J. Virol. 75 (24), 12182–12187. doi:10.1128/jvi.75.24.12182-12187.2001
Grant, R. W., and Dixit, V. D. (2015). Adipose tissue as an immunological organ. Obesity 23 (3), 512–518. doi:10.1002/oby.21003
Green, W. D., and Beck, M. A. (2017). Obesity Impairs the Adaptive Immune Response to Influenza Virus. Ann. ATS 14 (Suppl. ment_5), S406–S409. doi:10.1513/annalsats.201706-447aw
Gregor, M. F., and Hotamisligil, G. S. (2011). Inflammatory mechanisms in obesity. Annu. Rev. Immunol. 29, 415–445. doi:10.1146/annurev-immunol-031210-101322
Grizzle, W. E., Xu, X., Zhang, S., Stockard, C. R., Liu, C., Yu, S., et al. (2007). Age-related increase of tumor susceptibility is associated with myeloid-derived suppressor cell mediated suppression of T cell cytotoxicity in recombinant inbred BXD12 mice. Mech. Ageing Dev. 128 (11-12), 672–680. doi:10.1016/j.mad.2007.10.003
Han, J. M., Patterson, S. J., Speck, M., Ehses, J. A., and Levings, M. K. (2014). Insulin inhibits IL-10-mediated regulatory T cell function: implications for obesity. J.I. 192 (2), 623–629. doi:10.4049/jimmunol.1302181
Heithoff, D. M., Enioutina, E. Y., Bareyan, D., Daynes, R. A., and Mahan, M. J. (2008). Conditions that Diminish Myeloid-Derived Suppressor Cell Activities Stimulate Cross-Protective Immunity. Infect. Immun. 76 (11), 5191–5199. doi:10.1128/iai.00759-08
Hong, E.-G., Ko, H. J., Cho, Y.-R., Kim, H.-J., Ma, Z., Yu, T. Y., et al. (2009). Interleukin-10 prevents diet-induced insulin resistance by attenuating macrophage and cytokine response in skeletal muscle. Diabetes 58 (11), 2525–2535. doi:10.2337/db08-1261
Huang, J., Xie, Y., Sun, X., Zeh, H. J., Kang, R., Lotze, M. T., et al. (2015). DAMPs, ageing, and cancer: The 'DAMP Hypothesis'. Ageing Res. Rev. 24 (Pt A), 3–16. doi:10.1016/j.arr.2014.10.004
Ilan, Y., Maron, R., Tukpah, A.-M., Maioli, T. U., Murugaiyan, G., Yang, K., et al. (2010). Induction of regulatory T cells decreases adipose inflammation and alleviates insulin resistance in ob/ob mice. Proc. Natl. Acad. Sci. 107 (21), 9765–9770. doi:10.1073/pnas.0908771107
Ishikawa, A., Wada, T., Nishimura, S., Ito, T., Okekawa, A., Onogi, Y., et al. (2020). Estrogen regulates sex-specific localization of regulatory T cells in adipose tissue of obese female mice. PLoS One 15 (4), e0230885. doi:10.1371/journal.pone.0230885
Ito, F., Kamekura, R., Yamamoto, M., Takano, K., Takaki, H., Yabe, H., et al. (2019). IL-10+ T follicular regulatory cells are associated with the pathogenesis of IgG4-related disease. Immunol. Lett. 207, 56–63. doi:10.1016/j.imlet.2019.01.008
Jackaman, C., Radley-Crabb, H. G., Soffe, Z., Shavlakadze, T., Grounds, M. D., and Nelson, D. J. (2013). Targeting macrophages rescues age-related immune deficiencies in C57BL/6J geriatric mice. Aging cell 12 (3), 345–357. doi:10.1111/acel.12062
James, B. R., Tomanek-Chalkley, A., Askeland, E. J., Kucaba, T., Griffith, T. S., and Norian, L. A. (2012). Diet-induced obesity alters dendritic cell function in the presence and absence of tumor growth. J.I. 189 (3), 1311–1321. doi:10.4049/jimmunol.1100587
Jin, R., Kaneko, H., Suzuki, H., Arai, T., Teramoto, T., Fukao, T., et al. (2008). Age-related changes in BAFF and APRIL profiles and upregulation of BAFF and APRIL expression in patients with primary antibody deficiency. Int. J. Mol. Med. 21 (2), 233–238. doi:10.3892/ijmm.21.2.233
Kado, T., Nawaz, A., Takikawa, A., Usui, I., and Tobe, K. (2019). Linkage of CD8+ T cell exhaustion with high-fat diet-induced tumourigenesis. Sci. Rep. 9 (1), 12284. doi:10.1038/s41598-019-48678-0
Kim, Y.-H., Choi, B.-H., Cheon, H.-G., and Do, M.-S. (2009). B cell activation factor (BAFF) is a novel adipokine that links obesity and inflammation. Exp. Mol. Med. 41 (3), 208–216. doi:10.3858/emm.2009.41.3.024
Lages, C. S., Suffia, I., Velilla, P. A., Huang, B., Warshaw, G., Hildeman, D. A., et al. (2008). Functional regulatory T cells accumulate in aged hosts and promote chronic infectious disease reactivation. J. Immunol. 181 (3), 1835–1848. doi:10.4049/jimmunol.181.3.1835
Lauterbach, M. A., and Wunderlich, F. T. (2017). Macrophage function in obesity-induced inflammation and insulin resistance. Pflugers Arch. 469 (3-4), 385–396. doi:10.1007/s00424-017-1955-5
Lefebvre, J. S., Lorenzo, E. C., Masters, A. R., Hopkins, J. W., Eaton, S. M., Smiley, S. T., et al. (2016). Vaccine efficacy and T helper cell differentiation change with aging. Oncotarget 7 (23), 33581–33594. doi:10.18632/oncotarget.9254
Lefebvre, J. S., Masters, A. R., Hopkins, J. W., and Haynes, L. (2016). Age-related impairment of humoral response to influenza is associated with changes in antigen specific T follicular helper cell responses. Sci. Rep. 6 (1), 25051. doi:10.1038/srep25051
Leonardi, G. C., Accardi, G., Monastero, R., Nicoletti, F., and Libra, M. (2018). Ageing: from inflammation to cancer. Immun. Ageing 15 (1), 1–7. doi:10.1186/s12979-017-0112-5
Linehan, E., Dombrowski, Y., Snoddy, R., Fallon, P. G., Kissenpfennig, A., and Fitzgerald, D. C. (2014). Aging impairs peritoneal but not bone marrow-derived macrophage phagocytosis. Aging cell 13 (4), 699–708. doi:10.1111/acel.12223
Liu, R., and Nikolajczyk, B. S. (2019). Tissue Immune Cells Fuel Obesity-Associated Inflammation in Adipose Tissue and beyond. Front. Immunol. 10, 1587. doi:10.3389/fimmu.2019.01587
Lumeng, C. N. (2013). Innate immune activation in obesity. Mol. Aspects Med. 34 (1), 12–29. doi:10.1016/j.mam.2012.10.002
McDonnell, M. E., Ganley-Leal, L. M., Mehta, A., Bigornia, S. J., Mott, M., Rehman, Q., et al. (2012). B lymphocytes in human subcutaneous adipose crown-like structures. Obesity (Silver Spring) 20 (7), 1372–1378. doi:10.1038/oby.2012.54
McLaughlin, T., Ackerman, S. E., Shen, L., and Engleman, E. (2017). Role of innate and adaptive immunity in obesity-associated metabolic disease. J. Clin. Invest. 127 (1), 5–13. doi:10.1172/jci88876
Minciullo, P. L., Catalano, A., Mandraffino, G., Casciaro, M., Crucitti, A., Maltese, G., et al. (2016). Inflammaging and Anti-inflammaging: The Role of Cytokines in Extreme Longevity. Arch. Immunol. Ther. Exp. 64 (2), 111–126. doi:10.1007/s00005-015-0377-3
Mizoguchi, E., Mizoguchi, A., Preffer, F. I., and Bhan, A. K. (2000). Regulatory role of mature B cells in a murine model of inflammatory bowel disease. Int. Immunol. 12 (5), 597–605. doi:10.1093/intimm/12.5.597
Monti, D., Ostan, R., Borelli, V., Castellani, G., and Franceschi, C. (2017). Inflammaging and human longevity in the omics era. Mech. Ageing Dev. 165, 129–138. doi:10.1016/j.mad.2016.12.008
Moreno-Fernandez, M. E., Giles, D. A., Oates, J. R., Chan, C. C., Damen, M. S. M. A., Doll, J. R., et al. (2021). PKM2-dependent metabolic skewing of hepatic Th17 cells regulates pathogenesis of non-alcoholic fatty liver disease. Cell Metab. 33 (6), 1187–e9. doi:10.1016/j.cmet.2021.04.018
Moreno-Fernandez, M. E., Sharma, V., Stankiewicz, T. E., Oates, J. R., Doll, J. R., Damen, M. S. M. A., et al. (2021). Aging mitigates the severity of obesity-associated metabolic sequelae in a gender independent manner. Nutr. Diabetes 11 (1), 15. doi:10.1038/s41387-021-00157-0
Mori, D. N., Shen, H., Galan, A., and Goldstein, D. R. (2016). Aged B cells alter immune regulation of allografts in mice. Eur. J. Immunol. 46 (11), 2650–2658. doi:10.1002/eji.201646353
Moseley, C. E., Webster, R. G., and Aldridge, J. R. (2010). Original Article: Peroxisome proliferator-activated receptor and AMP-activated protein kinase agonists protect against lethal influenza virus challenge in mice. Influenza Other Respir. Viruses 4 (5), 307–311. doi:10.1111/j.1750-2659.2010.00155.x
Musovic, S., and Olofsson, C. S. (2019). Adrenergic stimulation of adiponectin secretion in visceral mouse adipocytes is blunted in high-fat diet induced obesity. Sci. Rep. 9 (1), 10680. doi:10.1038/s41598-019-47113-8
Narasaraju, T., Yang, E., Samy, R. P., Ng, H. H., Poh, W. P., Liew, A.-A., et al. (2011). Excessive neutrophils and neutrophil extracellular traps contribute to acute lung injury of influenza pneumonitis. Am. J. Pathol. 179 (1), 199–210. doi:10.1016/j.ajpath.2011.03.013
Nichol, K. L., Nordin, J. D., Nelson, D. B., Mullooly, J. P., and Hak, E. (2007). Effectiveness of influenza vaccine in the community-dwelling elderly. N. Engl. J. Med. 357 (14), 1373–1381. doi:10.1056/nejmoa070844
Nikolich-Žugich, J. (2008). Ageing and life-long maintenance of T-cell subsets in the face of latent persistent infections. Nat. Rev. Immunol. 8 (7), 512–522. doi:10.1038/nri2318
Nikolich-Žugich, J., Li, G., Uhrlaub, J. L., Renkema, K. R., and Smithey, M. J. (2012). Age-related changes in CD8 T cell homeostasis and immunity to infection. Semin. Immunol. 24 (5), 356–364. doi:10.1016/j.smim.2012.04.009
Nishimura, S., Manabe, I., Nagasaki, M., Eto, K., Yamashita, H., Ohsugi, M., et al. (2009). CD8+ effector T cells contribute to macrophage recruitment and adipose tissue inflammation in obesity. Nat. Med. 15 (8), 914–920. doi:10.1038/nm.1964
Nishimura, S., Manabe, I., Takaki, S., Nagasaki, M., Otsu, M., Yamashita, H., et al. (2013). Adipose Natural Regulatory B Cells Negatively Control Adipose Tissue Inflammation. Cel Metab. 18 (5), 759–766. doi:10.1016/j.cmet.2013.09.017
Nishioka, T., Shimizu, J., Iida, R., Yamazaki, S., and Sakaguchi, S. (2006). CD4+CD25+Foxp3+ T Cells and CD4+CD25−Foxp3+ T Cells in Aged Mice. J. Immunol. 176 (11), 6586–6593. doi:10.4049/jimmunol.176.11.6586
North, B. J., and Sinclair, D. A. (2012). The intersection between aging and cardiovascular disease. Circ. Res. 110 (8), 1097–1108. doi:10.1161/circresaha.111.246876
O'Shea, D., and Hogan, A. E. (2019). Dysregulation of Natural Killer Cells in Obesity. Cancers (Basel) 11 (4), 573. doi:10.3390/cancers11040573
Painter, S. D., Ovsyannikova, I. G., and Poland, G. A. (2015). The weight of obesity on the human immune response to vaccination. Vaccine 33 (36), 4422–4429. doi:10.1016/j.vaccine.2015.06.101
Pang, W. W., Price, E. A., Sahoo, D., Beerman, I., Maloney, W. J., Rossi, D. J., et al. (2011). Human bone marrow hematopoietic stem cells are increased in frequency and myeloid-biased with age. Proc. Natl. Acad. Sci. 108 (50), 20012–20017. doi:10.1073/pnas.1116110108
Parisi, M. M., Grun, L. K., Lavandoski, P., Alves, L. B., Bristot, I. J., Mattiello, R., et al. (2017). Immunosenescence Induced by Plasma from Individuals with Obesity Caused Cell Signaling Dysfunction and Inflammation. Obesity 25 (9), 1523–1531. doi:10.1002/oby.21888
Pirola, L., and Ferraz, J. C. (2017). Role of pro- and anti-inflammatory phenomena in the physiopathology of type 2 diabetes and obesity. Wjbc 8 (2), 120–128. doi:10.4331/wjbc.v8.i2.120
Pizzolla, A., Oh, D. Y., Luong, S., Prickett, S. R., Henstridge, D. C., Febbraio, M. A., et al. (2016). High Fat Diet Inhibits Dendritic Cell and T Cell Response to Allergens but Does Not Impair Inhalational Respiratory Tolerance. PLoS One 11 (8), e0160407. doi:10.1371/journal.pone.0160407
Popkin, B. M., Du, S., Green, W. D., Beck, M. A., Algaith, T., Herbst, C. H., et al. (2020). Individuals with obesity and COVID-19: A global perspective on the epidemiology and biological relationships. Obes. Rev. 21 (11), e13128.
Porsche, C. E., Delproposto, J. B., Geletka, L., O'Rourke, R., and Lumeng, C. N. (2021). Obesity results in adipose tissue T cell exhaustion. JCI Insight 6 (8), e139793. doi:10.1172/jci.insight.139793
Raynor, J., Karns, R., Almanan, M., Li, K.-P., Divanovic, S., Chougnet, C. A., et al. (2015). IL-6 and ICOS Antagonize Bim and Promote Regulatory T Cell Accrual with Age. J.I. 195 (3), 944–952. doi:10.4049/jimmunol.1500443
Raynor, J., Lages, C. S., Shehata, H., Hildeman, D. A., and Chougnet, C. A. (2012). Homeostasis and function of regulatory T cells in aging. Curr. Opin. Immunol. 24 (4), 482–487. doi:10.1016/j.coi.2012.04.005
Reilly, S. M., and Saltiel, A. R. (2017). Adapting to obesity with adipose tissue inflammation. Nat. Rev. Endocrinol. 13 (11), 633–643. doi:10.1038/nrendo.2017.90
Reis, B. S., Lee, K., Fanok, M. H., Mascaraque, C., Amoury, M., Cohn, L. B., et al. (2015). Leptin receptor signaling in T cells is required for Th17 differentiation. J.I. 194 (11), 5253–5260. doi:10.4049/jimmunol.1402996
Reuman, P. D., Kubilis, P., Hurni, W., Brown, L., and Nalin, D. (1997). The effect of age and weight on the response to formalin inactivated, alum-adjuvanted hepatitis A vaccine in healthy adults. Vaccine 15 (10), 1157–1161. doi:10.1016/s0264-410x(96)00310-6
Roberts, H. M., Grant, M. M., Hubber, N., Super, P., Singhal, R., and Chapple, I. L. C. (2018). Impact of Bariatric Surgical Intervention on Peripheral Blood Neutrophil (PBN) Function in Obesity. Obes. Surg. 28 (6), 1611–1621. doi:10.1007/s11695-017-3063-1
Robinson, K., Prins, J., and Venkatesh, B. (2011). Clinical review: Adiponectin biology and its role in inflammation and critical illness. Crit. Care 15 (221), 221. doi:10.1186/cc10021
Russo, L., and Lumeng, C. N. (2018). Properties and functions of adipose tissue macrophages in obesity. Immunology 155 (4), 407–417. doi:10.1111/imm.13002
Sadeghi, H. M., Schnelle, J. F., Thomas, J. K., Nishanian, P., and Fahey, J. L. (1999). Phenotypic and functional characteristics of circulating monocytes of elderly persons☆. Exp. Gerontol. 34 (8), 959–970. doi:10.1016/s0531-5565(99)00065-0
Sage, P. T., Tan, C. L., Freeman, G. J., Haigis, M., and Sharpe, A. H. (2015). Defective TFH Cell Function and Increased TFR Cells Contribute to Defective Antibody Production in Aging. Cel Rep. 12 (2), 163–171. doi:10.1016/j.celrep.2015.06.015
Salvestrini, V., Sell, C., and Lorenzini, A. (2019). Obesity May Accelerate the Aging Process. Front. Endocrinol. 10, 266. doi:10.3389/fendo.2019.00266
Schenk, S., Saberi, M., and Olefsky, J. M. (2008). Insulin sensitivity: modulation by nutrients and inflammation. J. Clin. Invest. 118 (9), 2992–3002. doi:10.1172/jci34260
Schöndorf, T., Musholt, P. B., Hohberg, C., Forst, T., Lehmann, U., Fuchs, W., et al. (2011). The fixed combination of pioglitazone and metformin improves biomarkers of platelet function and chronic inflammation in type 2 diabetes patients: results from the PIOfix study. J. Diabetes Sci. Technol. 5 (2), 426–432. doi:10.1177/193229681100500233
Shaikh, S. R., Haas, K. M., Beck, M. A., and Teague, H. (2015). The effects of diet-induced obesity on B cell function. Clin. Exp. Immunol. 179 (1), 90–99. doi:10.1111/cei.12444
Sharma, S., Dominguez, A. L., and Lustgarten, J. (2006). High accumulation of T regulatory cells prevents the activation of immune responses in aged animals. J. Immunol. 177 (12), 8348–8355. doi:10.4049/jimmunol.177.12.8348
Shen, D., Li, H., Zhou, R., Liu, M.-j., Yu, H., and Wu, D.-F. (2018). Pioglitazone attenuates aging-related disorders in aged apolipoprotein E deficient mice. Exp. Gerontol. 102, 101–108. doi:10.1016/j.exger.2017.12.002
Sheridan, P. A., Paich, H. A., Handy, J., Karlsson, E. A., Schultz-Cherry, S., Hudgens, M., et al. (2015). The antibody response to influenza vaccination is not impaired in type 2 diabetics. Vaccine 33 (29), 3306–3313. doi:10.1016/j.vaccine.2015.05.043
Shirakawa, K., Yan, X., Shinmura, K., Endo, J., Kataoka, M., Katsumata, Y., et al. (2016). Obesity accelerates T cell senescence in murine visceral adipose tissue. J. Clin. Invest. 126 (12), 4626–4639. doi:10.1172/jci88606
Simell, B., Vuorela, A., Ekström, N., Palmu, A., Reunanen, A., Meri, S., et al. (2011). Aging reduces the functionality of anti-pneumococcal antibodies and the killing of Streptococcus pneumoniae by neutrophil phagocytosis. Vaccine 29 (10), 1929–1934. doi:10.1016/j.vaccine.2010.12.121
Skurk, T., Alberti-Huber, C., Herder, C., and Hauner, H. (2007). Relationship between adipocyte size and adipokine expression and secretion. J. Clin. Endocrinol. Metab. 92 (3), 1023–1033. doi:10.1210/jc.2006-1055
Smith, A. J., Liu, J., Yilmaz, A., Wright, V., Bradley, D., and Hsueh, W. A. (2020). Obesity contributes to a dysfunctional regulatory T Cell phenotype within adipose tissue. J. Immunol. 204 (1), 145.
Strissel, K. J., Stancheva, Z., Miyoshi, H., Perfield, J. W., DeFuria, J., Jick, Z., et al. (2007). Adipocyte death, adipose tissue remodeling, and obesity complications. Diabetes 56 (12), 2910–2918. doi:10.2337/db07-0767
Stults-Kolehmainen, M. A., Stanforth, P. R., Bartholomew, J. B., Lu, T., Abolt, C. J., and Sinha, R. (2013). DXA estimates of fat in abdominal, trunk and hip regions varies by ethnicity in men. Nutr. Diabetes 3, e64. doi:10.1038/nutd.2013.5
Sun, L., Hurez, V. J., Thibodeaux, S. R., Kious, M. J., Liu, A., Lin, P., et al. (2012). Aged regulatory T cells protect from autoimmune inflammation despite reduced STAT3 activation and decreased constraint of IL-17 producing T cells. Aging cell 11 (3), 509–519. doi:10.1111/j.1474-9726.2012.00812.x
Talukdar, S., Oh, D. Y., Bandyopadhyay, G., Li, D., Xu, J., McNelis, J., et al. (2012). Neutrophils mediate insulin resistance in mice fed a high-fat diet through secreted elastase. Nat. Med. 18 (9), 1407–1412. doi:10.1038/nm.2885
van Deursen, J. M. (2014). The role of senescent cells in ageing. Nature 509 (7501), 439–446. doi:10.1038/nature13193
Vasanthakumar, A., Chisanga, D., Blume, J., Gloury, R., Britt, K., Henstridge, D. C., et al. (2020). Sex-specific adipose tissue imprinting of regulatory T cells. Nature 579 (7800), 581–585. doi:10.1038/s41586-020-2040-3
Viel, S., Besson, L., Charrier, E., Marçais, A., Disse, E., Bienvenu, J., et al. (2017). Alteration of Natural Killer cell phenotype and function in obese individuals. Clin. Immunol. 177, 12–17. doi:10.1016/j.clim.2016.01.007
Wagner, N.-M., Brandhorst, G., Czepluch, F., Lankeit, M., Eberle, C., Herzberg, S., et al. (2013). Circulating regulatory T cells are reduced in obesity and may identify subjects at increased metabolic and cardiovascular risk. Obesity 21 (3), 461–468. doi:10.1002/oby.20087
Wang, Y., Wehling‐Henricks, M., Samengo, G., and Tidball, J. G. (2015). Increases of M2a macrophages and fibrosis in aging muscle are influenced by bone marrow aging and negatively regulated by muscle‐derived nitric oxide. Aging cell 14 (4), 678–688. doi:10.1111/acel.12350
Weber, D. J., Rutala, W. A., Samsa, G. P., Santimaw, J. E., and Lemon, S. M. (1985). Obesity as a predictor of poor antibody response to hepatitis B plasma vaccine. JAMA 254 (22), 3187–3189. doi:10.1001/jama.1985.03360220053027
Wei, J., Xu, H., Davies, J. L., and Hemmings, G. P. (1992). Increase of plasma IL-6 concentration with age in healthy subjects. Life Sci. 51 (25), 1953–1956. doi:10.1016/0024-3205(92)90112-3
Weisberg, S. P., McCann, D., Desai, M., Rosenbaum, M., Leibel, R. L., and Ferrante, A. W. (2003). Obesity is associated with macrophage accumulation in adipose tissue. J. Clin. Invest. 112 (12), 1796–1808. doi:10.1172/jci200319246
Wenisch, C., Patruta, S., Daxböck, F., Krause, R., and Hörl, W. (2000). Effect of age on human neutrophil function. J. Leukoc. Biol. 67 (1), 40–45. doi:10.1002/jlb.67.1.40
Wensveen, F. M., Jelenčić, V., Valentić, S., Šestan, M., Wensveen, T. T., Theurich, S., et al. (2015). NK cells link obesity-induced adipose stress to inflammation and insulin resistance. Nat. Immunol. 16 (4), 376–385. doi:10.1038/ni.3120
Whitlock, G., Whitlock, G., Lewington, S., Sherliker, P., Clarke, R., Emberson, J., et al. (2009). Body-mass index and cause-specific mortality in 900 000 adults: collaborative analyses of 57 prospective studies. Lancet 373 (9669), 1083–1096. doi:10.1016/S0140-6736(09)60318-4
Winer, D. A., Winer, S., Shen, L., Wadia, P. P., Yantha, J., Paltser, G., et al. (2011). B cells promote insulin resistance through modulation of T cells and production of pathogenic IgG antibodies. Nat. Med. 17 (5), 610–617. doi:10.1038/nm.2353
Wolters, B., Junge, U., Dziuba, S., and Roggendorf, M. (2003). Immunogenicity of combined hepatitis A and B vaccine in elderly persons. Vaccine 21 (25-26), 3623–3628. doi:10.1016/s0264-410x(03)00399-2
Wong, C. K., Smith, C. A., Sakamoto, K., Kaminski, N., Koff, J. L., and Goldstein, D. R. (2017). Aging Impairs Alveolar Macrophage Phagocytosis and Increases Influenza-Induced Mortality in Mice. J.I. 199 (3), 1060–1068. doi:10.4049/jimmunol.1700397
Wu, D., Wong, C. K., Han, J. M., Orban, P. C., Huang, Q., Gillies, J., et al. (2020). T reg-specific insulin receptor deletion prevents diet-induced and age-associated metabolic syndrome. J. Exp. Med. 217 (8), e20191542. doi:10.1084/jem.20191542
Wu, D., Han, J. M., Yu, X., Lam, A. J., Hoeppli, R. E., Pesenacker, A. M., et al. (2019). Characterization of regulatory T cells in obese omental adipose tissue in humans. Eur. J. Immunol. 49 (2), 336–347. doi:10.1002/eji.201847570
Wueest, S., and Konrad, D. (2018). The role of adipocyte-specific IL-6-type cytokine signaling in FFA and leptin release. Adipocyte 7 (3), 226–228. doi:10.1080/21623945.2018.1493901
Xia, S., Sha, H., Yang, L., Ji, Y., Ostrand-Rosenberg, S., and Qi, L. (2011). Gr-1+ CD11b+ myeloid-derived suppressor cells suppress inflammation and promote insulin sensitivity in obesity. J. Biol. Chem. 286 (26), 23591–23599. doi:10.1074/jbc.m111.237123
Yuan, N., Zhang, H. F., Wei, Q., Wang, P., and Guo, W. Y. (2018). Expression of CD4+CD25+Foxp3+ Regulatory T Cells, Interleukin 10 and Transforming Growth Factor β in Newly Diagnosed Type 2 Diabetic Patients. Exp. Clin. Endocrinol. Diabetes 126 (2), 96–101. doi:10.1055/s-0043-113454
Zhai, X., Qian, G., Wang, Y., Chen, X., Lu, J., Zhang, Y., et al. (2016). Elevated B Cell Activation is Associated with Type 2 Diabetes Development in Obese Subjects. Cell Physiol. Biochem. 38 (3), 1257–1266. doi:10.1159/000443073
Zhan, J., Huang, L., Ma, H., Chen, H., Yang, Y., Tan, S., et al. (2017). Reduced inflammatory responses of follicular helper T cell promote the development of regulatory B cells after Roux-en-Y gastric bypass. Clin. Exp. Pharmacol. Physiol. 44 (5), 556–565. doi:10.1111/1440-1681.12740
Zhang, W. Y., Schwartz, E. A., Permana, P. A., Reaven, P. D., and Reaven, P. (2008). Pioglitazone inhibits the expression of inflammatory cytokines from both monocytes and lymphocytes in patients with impaired glucose tolerance. Arterioscler Thromb. Vasc. Biol. 28 (6), 2312–2318. doi:10.1161/ATVBAHA.108.175687
Keywords: aging, obesity, inflammaging, anti-inflammation, immune system, immunosenecence, chronic inflammation
Citation: Thomas AL, Alarcon PC, Divanovic S, Chougnet CA, Hildeman DA and Moreno-Fernandez ME (2021) Implications of Inflammatory States on Dysfunctional Immune Responses in Aging and Obesity. Front. Aging 2:732414. doi: 10.3389/fragi.2021.732414
Received: 29 June 2021; Accepted: 25 August 2021;
Published: 22 September 2021.
Edited by:
Daniela Frasca, University of Miami, United StatesReviewed by:
Madhur Agrawal, VIB KU Leuven Center for Cancer Biology, BelgiumJanet E. McElhaney, UConn Center on Aging, United States
Barbara Nikolajczyk, University of Kentucky, United States
Copyright © 2021 Thomas, Alarcon, Divanovic, Chougnet, Hildeman and Moreno-Fernandez. This is an open-access article distributed under the terms of the Creative Commons Attribution License (CC BY). The use, distribution or reproduction in other forums is permitted, provided the original author(s) and the copyright owner(s) are credited and that the original publication in this journal is cited, in accordance with accepted academic practice. No use, distribution or reproduction is permitted which does not comply with these terms.
*Correspondence: Maria E. Moreno-Fernandez, maria.fields@cchmc.org; Alyssa L. Thomas, alyssa.thomas@cchmc.org; Pablo C. Alarcon, pablo.alacron.cabrera@cchmc.org