- 1Department of Microbiology and Immunology, University of Miami Miller School of Medicine, Miami, FL, United States
- 2Sylvester Comprehensive Cancer Center, University of Miami Miller School of Medicine, Miami, FL, United States
Our previous work has shown that young and elderly patients with Type-2 Diabetes Mellitus (T2DM) treated with Metformin have optimal B cell function and serum antibodies specific for the seasonal influenza vaccine. In this paper, we have evaluated B cell function and the metabolic requirements of B cell antibody responses in elderly T2DM patients (ET2DM) taking or not Metformin, and compared to those of healthy elderly (EH) and healthy young (YH) individuals. Results show that Metformin significantly increases in vivo B cell function, measured by influenza vaccine-specific serum antibodies, in ET2DM patients to the levels observed in EH and more importantly in YH individuals. Metformin also decreases the frequencies of pro-inflammatory B cell subsets, as well as intrinsic inflammation and metabolic requirements of peripheral B cells from ET2DM. This hyper-metabolic phenotype of B cells from ET2DM is needed to support intrinsic inflammation, measured by the expression of transcripts for markers of the senescence-associated secretory phenotype (SASP), and the secretion of autoimmune antibodies. Importantly, B cell function in ET2DM patients taking Metformin is not only increased as compared to that in ET2DM patients not taking Metformin, but is comparable to B cell function measured in YH individuals. These results altogether strongly support the anti-aging effects of Metformin on humoral immunity.
Introduction
Aging is associated with inflammaging known as the increased chronic low-grade serum inflammatory status (Franceschi et al., 2000), which is a significant risk factor for morbidity and mortality of older adults. Inflammaging is in fact involved in the pathogenesis of several debilitating chronic diseases. These include Type-2 Diabetes Mellitus (T2DM) (Prattichizzo et al., 2018). Inflammaging initiates and supports intrinsic inflammation in immune cells leading to reduced protective responses against infections and decreased humoral immunity to infections and vaccination (Bryl et al., 2001; Parish et al., 2009; Frasca et al., 2014). Inflammaging is also involved in metabolic dysfunction and in the development of insulin resistance (IR) (Hotamisligil, 2017).
T2DM is one of the most prevalent chronic inflammatory disease of older adults (https://www.cdc.gov/diabetes/data/statistics-report/newly-diagnosed-diabetes.html). It is a metabolic disease not associated with autoimmunity, but often characterized by obesity, hypertension, dyslipidemia, accelerated atherosclerosis, and increased mortality (Alberti and Zimmet, 1998). Its development has been related to inflammaging, in particular to the acquisition of the senescence-associated secretory phenotype (SASP) and related oxidative stress and endoplasmic reticulum stress (Prattichizzo et al., 2016), two processes also associated with physiological aging (Muriach et al., 2014; Bonomini et al., 2015). Life expectancy of T2DM patients has been reported to be about 6 years shorter than that of age-matched healthy controls, mainly due to the increased risk for progressive disability due to T2DM-associated chronic diseases and increased age (Huang et al., 2014). In contrast, catastrophic disability has been attributed to illness events associated with infections, including respiratory tract infections with influenza and associated complications (pneumonia, ischemic heart disease, congestive heart failure, and stroke) (Guralnik et al., 2001), and pulmonary tuberculosis (Prada-Medina et al., 2017), as well as with urinary tract infections (Nitzan et al., 2015).
Immune responses are dysfunctional in both young and elderly T2DM patients, leading to increased susceptibility to get infections and reduced responses to vaccination as compared to healthy age-matched controls, and these defects are exacerbated in older patients, due to their increased inflammatory condition (Pozzilli et al., 1986; Smith and Poland, 2000; Muller et al., 2005; McElhaney et al., 2015). Hyperglycemia in T2DM is considered to be a cause of dysfunctional immune responses, as it is associated with several pro-inflammatory and metabolic pathways, including the advanced glycated end products pathway (Singh et al., 2014) and the reactive oxygen intermediate pathway (Yan, 2014), which lead to increased intrinsic inflammation in immune cells and decreased function.
Metformin (dimethyl biguanide) is a synthetic product of guanidine, initially isolated from the extracts of a plant with anti-diabetic effects (Galega officinalis). Since its discovery more than 50 years ago, Metformin is the first-line medication for T2DM patients. Metformin is currently the only hypoglycemic and anti-inflammatory drug also influencing cellular processes associated with the development of chronic conditions of old age (inflammaging, oxidative damage, increased glycation of proteins, cell senescence, apoptosis). Recent findings in humans have shown that Metformin has anti-proliferative (Sabry et al., 2019), anti-fibrotic (Wang et al., 2020) and anti-oxidant (Esteghamati et al., 2013) effects, suggesting its potential use as an anti-aging molecule. Although not fully understood, the pleiotropic effects of Metformin are thought to be mediated primarily through the regulation of AMPK [5′-adenosine monophosphate (AMP)-activated protein kinase] and mTOR (mammalian target of rapamycin). AMPK activity is decreased in the liver, muscle, and adipose tissue of individuals with IR (Ruderman and Prentki, 2004).
We have previously shown that young and elderly T2DM patients treated with Metformin have optimal B cell function and humoral immunity to the seasonal influenza vaccine (Frasca et al., 2013a). In this paper, we have further evaluated B cell function and the metabolic requirements of B cell antibody responses in the following individuals: elderly T2DM patients (ET2DM) taking or not Metformin, healthy elderly (EH) and healthy young (YH) individuals. Results show that Metformin significantly increases in vivo influenza vaccine-specific serum antibodies in ET2DM patients. Metformin also decreases intrinsic inflammation in B cells from ET2DM and their hyper-metabolic phenotype needed to support the expression of markers of the senescence-associated secretory phenotype (SASP), as well as the secretion of pathogenic autoimmune antibodies.
Materials and Methods
Subjects
For this study, we recruited at the University of Miami Miller School of Medicine elderly patients with T2DM (ET2DM) taking Metformin (n = 14, age 74 ± 2) or not (n = 4, age 72 ± 2). Controls were healthy elderly (EH, n = 9, age 76 ± 3), and young (YH, n = 14, age 35 ± 1) individuals. The limited number of T2DM patients not taking Metformin is due to the mandatory rule of the University of Miami that patients diagnosed with T2DM must start treatment immediately after they have been diagnosed. After the establishment of this rule, it has been impossible to recruit treatment naïve T2DM patients. Both T2DM patients and healthy participants were screened for diseases known to alter the immune response or for consumption of medications that could alter the immune response. Subjects with autoimmune diseases, congestive heart failure, cardiovascular disease, chronic renal failure, malignancies, renal or hepatic diseases, infectious disease, trauma or surgery, pregnancy, or documented current substance and/or alcohol abuse were excluded. Dose of Metformin taken by ET2DM patients was 500 mg twice/day. ET2DM patients were on Metformin for at least 2 years before their recruitment.
All participants signed an informed consent. The study was reviewed and approved by our Institutional Review Board (IRB, protocols #20070481 and #20160542), which reviews all human research conducted under the auspices of the University of Miami.
Influenza Vaccination
Study participants were recruited during the 2011–2012, 2012–2013, and 2013–2014 influenza vaccine seasons. The 2011–2012 vaccine contained A/California/7/2009 (H1N1), A/Perth/16/2009 (H3N2), and B/Brisbane/60/2008. The 2012–2013 vaccine contained A/California/7/2009 (H1N1), A/Victoria/361/2011 (H3N2), and B/Wisconsin/1/2010-like (Yamagata lineage). The 2013–2014 vaccine contained A/California/7/2009 (H1N1), A(H3N2) virus antigenically like the cell-propagated prototype virus A/Victoria/361/2011 and B/Massachusetts/2/2012.
All participants at the time of enrollment were influenza-free, without symptoms associated with respiratory infections and did not contract flu-like symptoms within a 6-months follow-up period.
Blood samples were collected immediately before and 4 weeks after vaccination.
Hemagglutination Inhibition Assay
We evaluated H1N1-specific titers after vaccination, as we have previously described (Frasca et al., 2010; Frasca et al., 2012a; Frasca et al., 2013a; Frasca et al., 2013b), as the same H1N1 was repeated in the three consecutive seasons. The Hemagglutination Inhibition (HAI) assay is useful for the measurement of antibody titers in serum and is the most established correlate with vaccine protectiveness (Murasko et al., 2002; Skowronski et al., 2008).
PBMC Collection
Blood was drawn in Vacutainer CPT tubes (BD 362761). PBMC were isolated and cryopreserved. PBMC (1 × 106/ml) were thawed and cultured in complete medium (c-RPMI, RPMI 1640, supplemented with 10% FCS, 10 μg/ml Pen-Strep, 1mM Sodium Pyruvate, and 2 × 10−5 M 2-ME and 2 mM l-glutamine). After thawing the PBMC, viability is checked. We discard samples if viability is <75%, as evaluated by trypan blue counting.
B Cell Isolation and In vitro Stimulation
B cells were isolated from thawed PBMC by magnetic sorting using CD19 Microbeads (Miltenyi) and manufacturer’s instructions. Cell preparations were typically >95% pure. For autoimmune antibody production, B cells were stimulated for 10 days in c-RPMI with CpG (invivoGen ODN 2006, 10 μg/ml), then supernatants were collected and IgG antibodies measured by ELISA.
Flow Cytometry
After thawing, PBMC (2 × 106/ml) were stained for 20 min at room temperature with the following antibodies: anti-CD19 (BD 555415), anti-CD27 (BD 555441), and anti-IgD (BD 555778) to measure naive (IgD + CD27−), IgM memory (IgD + CD27+), switched memory (swIg, IgD-CD27+), and Double Negative (DN, IgD-CD27−) B cells.
To measure glucose uptake, we used the glucose fluorescent analog [2-(N-(7-Nitrobenz-2-oxa-1,3-diazol-4-yl)Amino)-2-Deoxyglucose] (2-NBDG, Thermo Fisher N13195). Briefly, PBMCs (106/ml) were left unstimulated and then 2-NBDG was added at a final concentration of 50 μM for 30 min.
Up to 105 events in the B cell gate were acquired on an LSR-Fortessa (BD) and analyzed using FlowJo 10.5.3 software. In every experiment we included single color controls for compensation purposes as well as isotype antibodies to set up the gates.
mRNA Extraction and Quantitative PCR
To evaluate mRNA expression of the glucose transporter Glut1 and of SASP markers, the mRNA was extracted from B cells, using µMACS magnetic beads (Miltenyi) and manufacturer’s instructions. The mRNA was eluted into 75 µL of pre-heated (65°C) elution buffer, and stored at −80°C until use. Reverse Transcriptase (RT) reactions were performed in a Mastercycler Eppendorf Thermocycler to obtain cDNA. Briefly, 10 µL of mRNA +10 µL of RT-mix were used for cDNA synthesis. Conditions were: 40 min at 42°C and 5 min at 65°C.
Five µL of cDNA were used for qPCR. Reactions were conducted in MicroAmp 96-well plates and run in the ABI 7300 machine. Calculations were made with ABI software. For calculations, we determined the cycle number at which transcripts reached a significant threshold (Ct) for both target genes and for GAPDH (control). The difference in Ct values between GAPDH and each of the target genes was calculated as ΔCt. Then the relative amount of the target gene was expressed as 2−ΔCt and indicated as qPCR values. Reagents and Taqman primers, all from Life Technologies, were the following: GAPDH, Hs99999905_m1; TNF, Hs01113624_g1; IL-6, Hs00985639_m1; p16INK4 (CDKN2A), Hs00923894_m1; Glut1 (SLC2A1), Hs00892681_m1.
Metabolic Measurements
We used a Mitostress test to evaluate oxygen consumption rates (OCR), a measure of oxidative phosphorylation (OXPHOS), and extracellular acidification rates (ECAR), a measure of anaerobic glycolysis. The Mitostress test was conducted in a Seahorse XFp extracellular flux analyzer (Agilent). Briefly, we seeded B cells from ET2DM patients taking Metformin or not, as well as from EH and YH individuals, in a CellTAK (BD Biosciences)-coated plate, at the concentration of 2.5 × 105/well in XF DMEM medium supplemented with glutamine, glucose, and pyruvate (200 μL of each reagent in 20 ml of medium). Maximal respiratory capacity was measured by the addition of the following compounds: Oligomycin (1 μM) to block ATP production, and then FCCP (fluoro-carbonyl cyanide phenylhydrazone, 5 μM), an uncoupling agent, to dissipate proton gradients and allow electron transport and oxygen consumption to operate at maximal rate. Maximal respiration was then suppressed by the addition of Rotenone/Antimycin (1 μM), showing that respiration is mitochondrial.
ELISA to Measure Autoimmune Antibodies in Culture Supernatants
For dsDNA-specific IgG antibodies we used the Signosis EA-5002 kit, following manufacturer’s instructions.
Statistical Analyses
To examine differences between four groups, two-way ANOVA was used. Group-wise differences were analyzed afterwards with Bonferroni’s multiple comparisons test, with p < 0.05 set as criterion for significance. To examine differences between two groups, unpaired Student’s t tests (two-tailed) were used. To examine relationships between variables, bivariate Pearson’s correlation analyses were performed. GraphPad Prism version 8.4.3 software was used to construct all graphs.
Results and Discussion
Metformin Enhances the Serum H1N1-Specific Antibody Response of ET2DM Patients to the Levels Observed in EH and More Importantly in YH Individuals
We measured the serum H1N1-specific antibody response by HAI in ET2DM patients taking Metformin or not, as well as in EH and YH individuals. Results in Figure 1 show that the response is significantly decreased in EH as compared to YH individuals. The response is decreased even more in ET2DM patients as compared to EH individuals. Metformin significantly enhances the response of ET2DM patients to the levels observed in EH and more importantly in YH individuals.
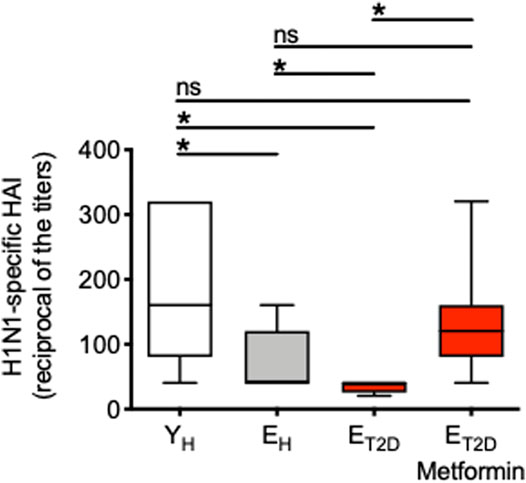
FIGURE 1. Metformin significantly enhances the serum H1N1-specific antibody response of ET2DM patients to the levels observed in EH and more importantly in YH individuals. Serum samples were collected from the 4 groups of individuals and evaluated by H1N1-specific HAI. Results show the reciprocal of the titers 4 weeks after vaccination. Mean comparisons between groups were performed by one-way ANOVA. *p < 0.05.
These results clearly indicate that T2DM superimposed on aging further decreases influenza vaccine responses in elderly individuals, and suggest the need to treat ET2DM patients with Metformin as soon as they are diagnosed in order to improve their response and protect them from the risk of infection.
Metformin Decreases the Frequencies of Pro-Inflammatory B Cell Subsets in the Blood of ET2DM Patients
The composition of the peripheral B cell pool influences the individual’s response to the influenza vaccine, and it has been shown that serum vaccine-specific antibody responses are reduced in EH as compared to YH individuals, in part due to decreased generation of the subset of swIg B cells (Amanna et al., 2007; Sasaki et al., 2008; Frasca et al., 2010; Frasca et al., 2012b; Frasca et al., 2013a) and of long-lived plasma cells residing in the bone marrow (Sasaki et al., 2011; Pritz et al., 2015). We therefore measured the frequencies of the major B cell subsets present in blood of ET2DM patients taking Metformin or not, and in EH and YH individuals. Previously published results have shown that aging increases the frequencies of naïve B cells (Chong et al., 2005; Shi et al., 2005; Frasca et al., 2016; Frasca et al., 2017a; Frasca et al., 2017b), although some studies have also reported the opposite result, a decrease in naïve B cell frequencies with age (Colonna-Romano et al., 2003; Buffa et al., 2013), likely due to differences among cohorts evaluated. Aging also increases the frequencies of DN B cells (Frasca et al., 2008; Colonna-Romano et al., 2009; Frasca et al., 2016; Frasca et al., 2017a; Frasca et al., 2017b) and concomitantly decreases the frequencies of IgM (Shi et al., 2005) and swIg (Shi et al., 2005; Frasca et al., 2008; Frasca et al., 2016; Frasca et al., 2017a; Frasca et al., 2017b) memory B cells. Results in Figure 2 confirm these previously published results and also show that T2DM induces further changes, exacerbating the effects of aging. Briefly, Figure 2A shows a representative experiment in which B cells isolated from the blood of the four groups of participants were stained with anti-CD19, anti-CD27, and anti-IgD antibodies to evaluate the frequencies of the major B cell subsets. Results from all the particpants are shown in Figure 2B. The composition of the peripheral B cell pool of ET2DM patients taking Metformin is comparable to that from YH individuals, and especially for the subsets of swIg and DN B cells.
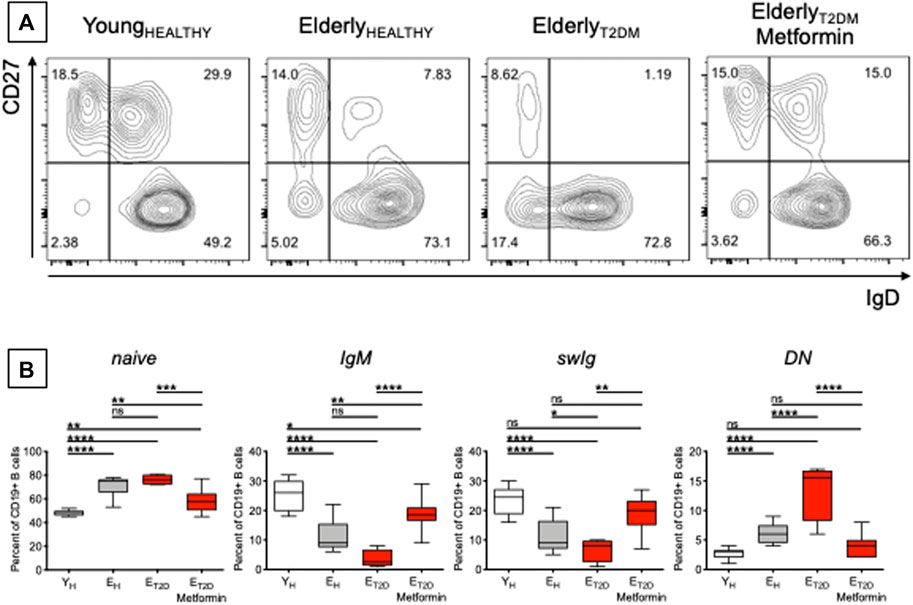
FIGURE 2. Metformin decreases the frequencies of pro-inflammatory B cell subsets in the blood of ET2DM patients. (A) Gating strategies and a representative contour plot from one individual/group. In this representative plot, naïve (IgD + CD27−) are in the lower right quadrant, IgG memory (IgD + CD27+) are in the upper right quadrant, swIg (IgD-CD27+) are in the upper left quadrant and DN (IgD-CD27-) are in the lower left quadrant. (B) Frequencies of the 4 B cell subsets in the 4 groups of individuals. Mean comparisons between groups were performed by one-way ANOVA. *p < 0.05, **p < 0.01, ***p < 0.001, ****p < 0.0001.
These are to our knowledge the first results showing decreased frequencies of DN B cells in the blood of ET2DM patients taking Metformin as compared to those not taking Metformin. DN B cells are the most pro-inflammatory B cell subset (Frasca et al., 2016; Frasca et al., 2019). These cells increase in the blood of individuals with inflammatory conditions and diseases, such as physiological aging (Colonna-Romano et al., 2009; Frasca et al., 2017a; Nevalainen et al., 2019), obesity (Frasca et al., 2016; Frasca et al., 2019; Frasca et al., 2021a), autoimmune diseases (Wehr et al., 2004; Saadoun et al., 2013; Martorana et al., 2014; Adlowitz et al., 2015; Claes et al., 2016; Wang et al., 2018; Golinski et al., 2020), chronic (Moir et al., 2008; Illingworth et al., 2013; Chang et al., 2017), and acute (Woodruff et al., 2020) infectious diseases. DN B cells are characterized by high RNA expression of several pro-inflammatory markers, such as cytokines (TNF, IL-6), chemokines (IL-8), micro-RNAs, miRs (miR-155, miR-16, miR-181a), and cell cycle inhibitors and markers of proliferation arrest (p16INK4, p21CIP1/WAF1, and p53) (Frasca et al., 2017a; Frasca et al., 2021b), thus contributing significantly to inflammaging. Based on the results in Figure 2, we can hypothesize that Metformin may be a valid anti-inflammatory treatment also for the above inflammatory conditions and diseases in which DN B cells play a crucial role in the maintenance of chronic inflammation.
Metformin Decreases mRNA Expression of SASP Markers in Ex Vivo-Isolated B Cells from ET2DM Patients
We have previously shown that serum antibodies generated in response to the influenza vaccine are negatively associated with TNF-α mRNA and protein expression in ex vivo-isolated B cells from both young and elderly individuals (Frasca et al., 2014; Frasca et al., 2016), as well as with the frequencies of DN B cells (Frasca et al., 2014; Frasca et al., 2017a). Therefore, we measured in B cells from ET2DM patients taking Metformin or not, as well as in B cells from EH and YH individuals, mRNA expression of the pro-inflammatory cytokines TNF-α and IL-6, and of the cell cycle inhibitor p16INK4, all markers of the SASP (Coppé et al., 2010). Results in Figure 3 show that Metformin significantly decreases mRNA expression of TNF-α and IL-6 as well as of p16INK4 in B cells from ET2DM patients to levels comparable to those observed in B cells from EH and YH individuals. This result is likely reflecting the effect of Metformin in reducing DN B cell frequencies. In the 4 groups of participants, TNF-α, IL-6, and p16INK4 levels were found negatively associated with the serum H1N1-specific antibody response (Pearson’s r = −0.61, p=0.012 for TNF-α; r = −0.72, p = 0.002 for IL6, r = −0.62, p = 0.010 for p16INK4).
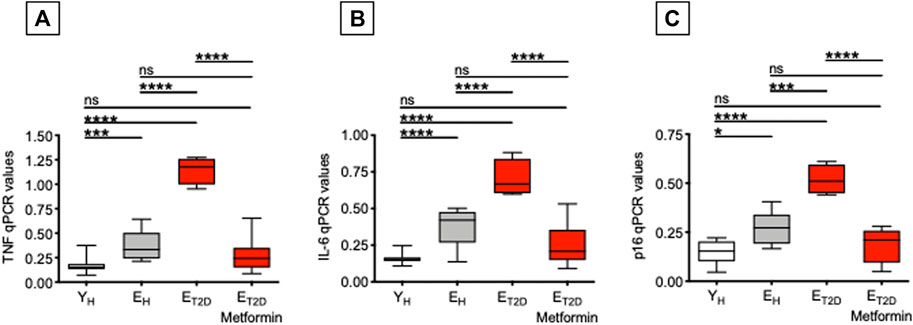
FIGURE 3. Metformin decreases mRNA expression of SASP markers in ex vivo-isolated B cells from ET2DM patients. B cells were resuspended in TRIzol, then the RNA was extracted and the expression of SASP markers detected by qPCR to evaluate expression of RNA for the pro-inflammatory cytokines TNF-α (A) and IL-6 (B), and for the cell cycle regulator p16INK4(C). qPCR values are measures of RNA expression of target genes, relative to the housekeeping gene GAPDH, calculated as 2−ΔCts. Mean comparisons between groups were performed by one-way ANOVA. *p < 0.05, ***p < 0.001, ****p < 0.0001.
These results demonstrate that Metformin is an effective treatment for T2DM-associated B cell intrinsic inflammation, known to negatively affect influenza vaccine responses (Frasca et al., 2014). Although not directly demonstrated in the present study, we hypothesize that Metformin may prevent negative effects of TNF-α on B cells, possibly through decreased TNF-α signaling and reduced NF-kB activation, as it has already been shown in other cell types (Isoda et al., 2006; Li et al., 2009; Gu et al., 2014; Sekino et al., 2018; Kanigur Sultuybek et al., 2019).
Metformin Decreases Glucose Uptake in Ex Vivo-Isolated B Cells From Elderly T2DM Patients
Our recently published results in mice have shown that the higher expression of SASP markers in B cells isolated from the spleens of old mice is associated with a hyper-metabolic profile needed to support their pro-inflammatory phenotype (Frasca et al., 2021c). Splenic B cells from old mice, as compared to those from young mice, are characterized by higher glucose uptake and higher mRNA expression of key metabolic enzymes involved in anaerobic glycolysis and OXPHOS, such as lactate dehydrogenase that converts pyruvate into lactate and represents a measure of anerobic glycolysis and pyruvate dehydrogenase that converts pyruvate into acetyl-CoA and represents a measure of OXPHOS and mitochondrial function.
Therefore, we measured glucose uptake in ex vivo-isolated B cells from ET2DM patients taking Metformin or not (four patients/group), as well as in B cells from EH and YH individuals (four individuals/group). We used flow cytometry and the 2-NBDG glucose fluorescent analog. Results in Figure 4 show that Metformin decreases glucose uptake in B cells from ET2DM patients to levels observed in B cells from EH and YH individuals (A). Metformin also decreases the mRNA expression of the glucose transporter Glut1 (B).
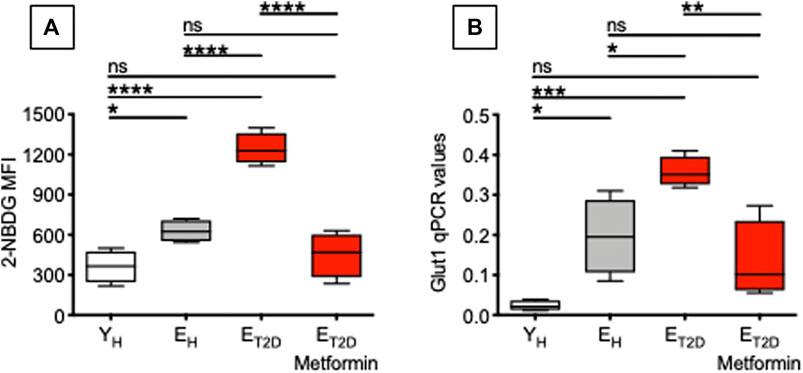
FIGURE 4. Metformin decreases glucose uptake in ex vivo-isolated B cells from ET2DM patients. Glucose uptake was measured by flow cytometry and the glucose fluorescent analog 2-NBDG. (A) MFI (mean fluorescence intensity) of 2-NBDG staining in B cells from the 4 groups of individuals. (B) qPCR values of Glut1 mRNA expression, relative to GAPDH, calculated as 2−ΔCts in the same B cells in (A). Mean comparisons between groups were performed by one-way ANOVA. *p < 0.05, **p < 0.01, ***p < 0.001, ****p < 0.0001.
Glucose uptake is associated with a higher metabolic phenotype. Therefore, we evaluated the metabolic profile of B cells from the same patients and healthy controls in Figure 4. We performed a mitostress test comparing B cells from ET2DM patients taking Metformin or not, as well as from EH and YH individuals (4 individuals/group). Magnetic beads-sorted B cells from the 4 groups of participants were seeded into the wells of an extracellular flux analyzer to evaluate real-time changes in OCR and ECAR. Seahorse technology gives the possibility to perform several measures of mitochondrial function, including maximal respiration and spare respiratory capacity with a relatively high throughput. Results in Figure 5 show, as expected, higher OCR (A, left) and ECAR (B) in B cells from ET2DM patients not taking Metformin as compared to those from ET2DM patients on Metformin, confirming the higher inflammatory profile of B cells from ET2DM patients not taking Metformin. Moreover, Metformin decreases OCR and ECAR in B cells from ET2DM patients to levels observed in B cells from YH individuals, and lower as compared to B cells from EH individuals. Major differences in OCR among the 4 groups were observed in maximal respiration (A, right).
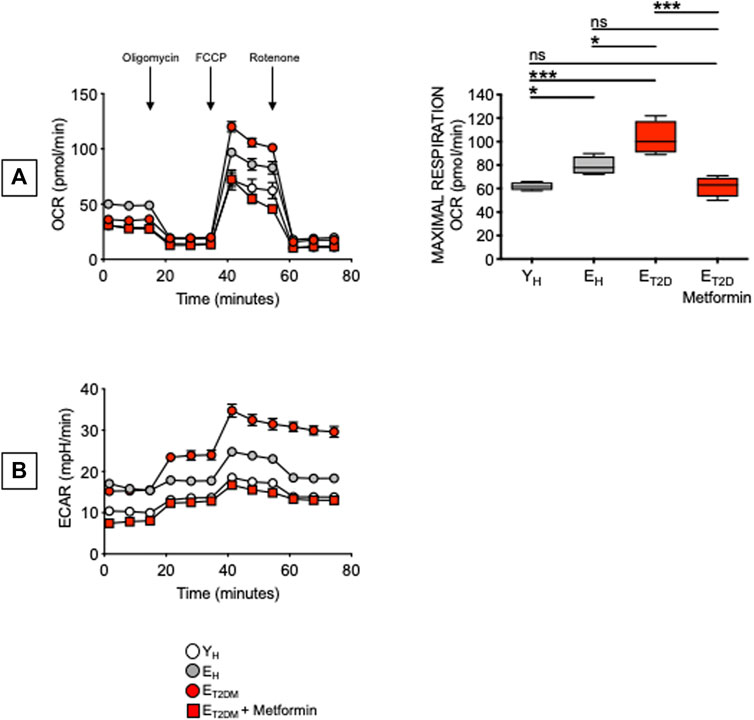
FIGURE 5. Metformin decreases OCR and ECAR in ex vivo-isolated B cells from ET2DM patients. B cells were seeded into the wells of an extracellular flux analyzer at the concentration of 2 × 105/well in triplicate and run in a mitostress test. (A) OCR results (left) and maximal respiration results (right) are shown. Mean comparisons between groups were performed by one-way ANOVA. *p < 0.05, ***p < 0.001. (B) ECAR results.
These results are the first to our knowledge to show that Metformin decreases glucose uptake, OCR and ECAR in B cells from ET2DM patients. In the 4 groups of participants, 2-NBDG MFI measures and maximal respiration were also found negatively associated with the serum H1N1-specific antibody response (Pearson’s r = −0.61, p = 0.015, and r = −0.59, p = 0.015, respectively). It was recently shown that Metformin controls intrinsic inflammation by decreasing OCR as well as OCR:ECAR ratio in Th17 cells from ET2DM patients (Bharath et al., 2020). Importantly, in this T cell study Metformin was effective in shifting measures of mitochondrial function and intrinsic inflammation to values indistinguishable from those observed in cells from young individuals. These findings together with ours in Figure 5 highlight the relationship between metabolism and intrinsic inflammation and provide examples of how metabolic pathways can represent potential novel therapeutic targets, supporting the importance of clinical trials to improve health span with Metformin.
Metformin Decreases Autoimmune Antibody Secretion in CpG-Stimulated B Cells From Elderly Type-2 Diabetes Mellitus Patients
Our previously published work has shown that a higher metabolic phenotype is associated with the secretion of IgG antibodies with autoimmune specificities in both mice (Frasca et al., 2021c; Caro-Maldonado et al., 2014) and humans (Frasca et al., 2019). Here, we measured the secretion of anti-dsDNA IgG antibodies in culture supernatants of CpG-stimulated B cells isolated from ET2DM patients taking Metformin or not, as well as of B cells isolated from EH and YH individuals (same patients and healthy controls in Figure 4). Although T2DM is not a typical autoimmune disease, autoimmune antibodies with different specificities can be detected in the majority of these patients (de Candia et al., 2019). Results in Figure 6 show that Metformin decreases autoimmune IgG secretion in B cells from ET2DM patients as compared to B cells from EH and YH individuals (A). As expected, glucose uptake and autoimmune IgG antibodies were positively associated (B).
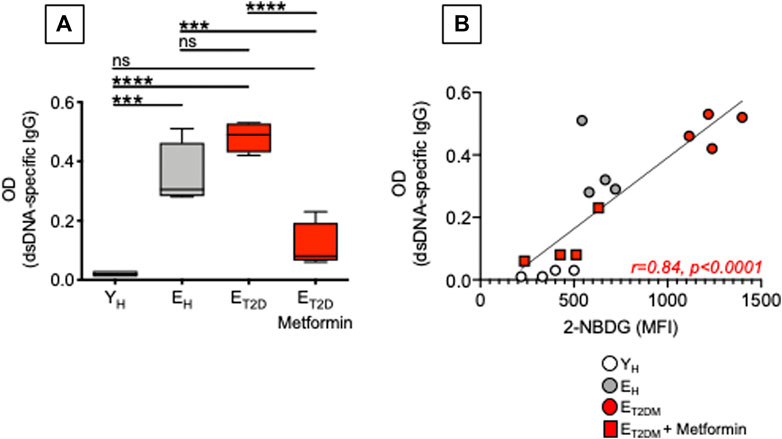
FIGURE 6. Metformin decreases autoimmune antibody secretion in CpG-stimulated B cells from ET2DM patients. (A) Results show OD values at 1:200 sample dilution. (B) Correlations of anti-dsDNA IgG (OD values) with 2-NBDG MFI. Pearson’s regression coefficients and p values are indicated at the bottom of the figure.
Although Metformin has been shown to be effective in reducing inflammation in various models of autoimmune diseases such as systemic lupus erythematosus (Yin et al., 2015), colitis (Lee et al., 2015), and experimental autoimmune arthritis (Son et al., 2014), its effects on autoimmune B cells have not been evaluated yet. Our results herein are particularly important as it is known that upregulation of glucose uptake and glycolysis are essential for B cell-mediated generation of pathogenic autoimmune Th cells, and immunotherapy with anti-CD20 antibodies, that specifically depletes B cells, completely abrogates glucose uptake in Th cells and suppresses disease progression in patients with autoimmune diseases (Zeng et al., 2020).
Conclusion
Results herein show that Metformin significantly increases the antibody response to the influenza vaccine in ET2DM patients to the levels observed in EH, and more importantly in YH individuals, likely by decreasing B cell intrinsic inflammation that is negatively associated with protective responses to infections and vaccination. Our results also show that Metformin induces changes in the metabolic phenotype of B cells, reducing their hyper-metabolic status that is supporting age-associated B cell intrinsic inflammation, the expression of SASP markers and the secretion of pathogenic autoimmune antibodies. Although many studies have already outlined the importance of Metformin in the regulation of metabolic requirements of T cells, studies on the effects of Metforming on B cell metabolism are lacking. Our results therefore add important findings indicating that Metformin represents an effective therapeutic option to rewire metabolic pathways also in B cells, leading to improved humoral immunity and reduced inflammation and autoimmunity.
Data Availability Statement
The raw data supporting the conclusion of this article will be made available by the authors, without undue reservation.
Ethics Statement
The studies involving human participants were reviewed and approved by IRB, protocols #20070481 and #20160542 (University of Miami Miller School of Medicine). The patients/participants provided their written informed consent to participate in this study.
Author Contributions
DF wrote the paper. AD, MR, and DF performed the experiments, acquire and analyze data. DF and BB were involved in funding acquisition. All authors reviewed and edited the paper.
Funding
This study was supported by NIH awards AG32576, AG059719, AG023717.
Conflict of Interest
The authors declare that the research was conducted in the absence of any commercial or financial relationships that could be construed as a potential conflict of interest.
Publisher’s Note
All claims expressed in this article are solely those of the authors and do not necessarily represent those of their affiliated organizations, or those of the publisher, the editors and the reviewers. Any product that may be evaluated in this article, or claim that may be made by its manufacturer, is not guaranteed or endorsed by the publisher.
References
Adlowitz, D. G., Barnard, J., Biear, J. N., Cistrone, C., Owen, T., Wang, W., et al. (2015). Expansion of Activated Peripheral Blood Memory B Cells in Rheumatoid Arthritis, Impact of B Cell Depletion Therapy, and Biomarkers of Response. PLoS One 10 (6), e0128269. doi:10.1371/journal.pone.0128269
Alberti, K. G. M. M., and Zimmet, P. Z. (1998). Definition, Diagnosis and Classification of Diabetes Mellitus and its Complications. Part 1: Diagnosis and Classification of Diabetes Mellitus. Provisional Report of a WHO Consultation. Diabet. Med. 15 (7), 539–553. doi:10.1002/(sici)1096-9136(199807)15:7<539::aid-dia668>3.0.co;2-s
Amanna, I. J., Carlson, N. E., and Slifka, M. K. (2007). Duration of Humoral Immunity to Common Viral and Vaccine Antigens. N. Engl. J. Med. 357 (19), 1903–1915. doi:10.1056/nejmoa066092
Bharath, L. P., Agrawal, M., McCambridge, G., Nicholas, D. A., Hasturk, H., Liu, J., et al. (2020). Metformin Enhances Autophagy and Normalizes Mitochondrial Function to Alleviate Aging-Associated Inflammation. Cel Metab. 32 (1), 44–55. doi:10.1016/j.cmet.2020.04.015
Bonomini, F., Rodella, L. F., and Rezzani, R. (2015). Metabolic Syndrome, Aging and Involvement of Oxidative Stress. A&D 6 (2), 109–120. doi:10.14336/ad.2014.0305
Bryl, E., Vallejo, A. N., Weyand, C. M., and Goronzy, J. J. (2001). Down-Regulation of CD28 Expression by TNF-α. J. Immunol. 167 (6), 3231–3238. doi:10.4049/jimmunol.167.6.3231
Buffa, S., Pellicanò, M., Bulati, M., Martorana, A., Goldeck, D., Caruso, C., et al. (2013). A Novel B Cell Population Revealed by a CD38/CD24 Gating Strategy: CD38−CD24− B Cells in Centenarian Offspring and Elderly People. Age 35 (5), 2009–2024. doi:10.1007/s11357-012-9488-5
Caro-Maldonado, A., Wang, R., Nichols, A. G., Kuraoka, M., Milasta, S., Sun, L. D., et al. (2014). Metabolic Reprogramming Is Required for Antibody Production that Is Suppressed in Anergic but Exaggerated in Chronically BAFF-Exposed B Cells. J.I. 192 (8), 3626–3636. doi:10.4049/jimmunol.1302062
Chang, L.-Y., Li, Y., and Kaplan, D. E. (2017). Hepatitis C Viraemia Reversibly Maintains Subset of Antigen-specific T-Bet+ Tissue-like Memory B Cells. J. Viral Hepat. 24 (5), 389–396. doi:10.1111/jvh.12659
Chong, Y., Ikematsu, H., Yamaji, K., Nishimura, M., Nabeshima, S., Kashiwagi, S., et al. (2005). CD27+ (Memory) B Cell Decrease and Apoptosis-Resistant CD27- (Naive) B Cell Increase in Aged Humans: Implications for Age-Related Peripheral B Cell Developmental Disturbances. Int. Immunol. 17 (4), 383–390. doi:10.1093/intimm/dxh218
Claes, N., Fraussen, J., Vanheusden, M., Hellings, N., Stinissen, P., Van Wijmeersch, B., et al. (2016). Age-Associated B Cells with Proinflammatory Characteristics Are Expanded in a Proportion of Multiple Sclerosis Patients. J.I. 197 (12), 4576–4583. doi:10.4049/jimmunol.1502448
Colonna-Romano, G., Bulati, M., Aquino, A., Pellicanò, M., Vitello, S., Lio, D., et al. (2009). A Double-Negative (IgD−CD27−) B Cell Population Is Increased in the Peripheral Blood of Elderly People. Mech. Ageing Dev. 130 (10), 681–690. doi:10.1016/j.mad.2009.08.003
Colonna-Romano, G., Bulati, M., Aquino, A., Scialabba, G., Candore, G., Lio, D., et al. (2003). B Cells in the Aged: CD27, CD5, and CD40 Expression. Mech. Ageing Dev. 124 (4), 389–393. doi:10.1016/s0047-6374(03)00013-7
Coppé, J.-P., Desprez, P.-Y., Krtolica, A., and Campisi, J. (2010). The Senescence-Associated Secretory Phenotype: the Dark Side of Tumor Suppression. Annu. Rev. Pathol. Mech. Dis. 5, 99–118. doi:10.1146/annurev-pathol-121808-102144
de Candia, P., Prattichizzo, F., Garavelli, S., De Rosa, V., Galgani, M., Di Rella, F., et al. (2019). Type 2 Diabetes: How Much of an Autoimmune Disease?. Front. Endocrinol. (Lausanne) 10, 451. doi:10.3389/fendo.2019.00451
Esteghamati, A., Eskandari, D., Mirmiranpour, H., Noshad, S., Mousavizadeh, M., Hedayati, M., et al. (2013). Effects of Metformin on Markers of Oxidative Stress and Antioxidant reserve in Patients with Newly Diagnosed Type 2 Diabetes: a Randomized Clinical Trial. Clin. Nutr. 32 (2), 179–185. doi:10.1016/j.clnu.2012.08.006
Franceschi, C., Bonafè, M., Valensin, S., Olivieri, F., De Luca, M., Ottaviani, E., et al. (2000). Inflamm-aging. An Evolutionary Perspective on Immunosenescence. Ann. N. Y Acad. Sci. 908, 244–254. doi:10.1111/j.1749-6632.2000.tb06651.x
Frasca, D., Diaz, A., Romero, M., and Blomberg, B. B. (2021). Phenotypic and Functional Characterization of Double Negative B Cells in the Blood of Individuals with Obesity. Front. Immunol. 12, 616650. doi:10.3389/fimmu.2021.616650
Frasca, D., Diaz, A., Romero, M., Mendez, N. V., Landin, A. M., and Blomberg, B. B. (2013). Effects of Age on H1N1-specific Serum IgG1 and IgG3 Levels Evaluated during the 2011-2012 Influenza Vaccine Season. Immun. Ageing 10 (1), 14. doi:10.1186/1742-4933-10-14
Frasca, D., Diaz, A., Romero, M., Phillips, M., Mendez, N. V., Landin, A. M., et al. (2012). Unique Biomarkers for B-Cell Function Predict the Serum Response to Pandemic H1N1 Influenza Vaccine. Int. Immunol. 24 (3), 175–182. doi:10.1093/intimm/dxr123(
Frasca, D., Diaz, A., Romero, M., Thaller, S., and Blomberg, B. B. (2019). Metabolic Requirements of Human Pro-inflammatory B Cells in Aging and Obesity. PLoS One 14 (7), e0219545. doi:10.1371/journal.pone.0219545
Frasca, D., Romero, M., Diaz, A., Garcia, D., Thaller, S., and Blomberg, B. B. (2021). B Cells with a Senescent-Associated Secretory Phenotype Accumulate in the Adipose Tissue of Individuals with Obesity. Int. J. Mol. Sci. 22 (4). doi:10.3390/ijms22041839
Frasca, D., Romero, M., Garcia, D., Diaz, A., and Blomberg, B. B. (2021). Hyper-metabolic B Cells in the Spleens of Old Mice Make Antibodies with Autoimmune Specificities. Immun. Ageing 18 (1), 9. doi:10.1186/s12979-021-00222-3
Frasca, D., Diaz, A., Romero, M., and Blomberg, B. B. (2017). Human Peripheral Late/exhausted Memory B Cells Express a Senescent-Associated Secretory Phenotype and Preferentially Utilize Metabolic Signaling Pathways. Exp. Gerontol. 87 (Pt A), 113–120. doi:10.1016/j.exger.2016.12.001
Frasca, D., Diaz, A., Romero, M., D'Eramo, F., and Blomberg, B. B. (2017). Aging Effects on T-Bet Expression in Human B Cell Subsets. Cell Immunol. 321, 68–73. doi:10.1016/j.cellimm.2017.04.007
Frasca, D., Diaz, A., Romero, M., Landin, A. M., and Blomberg, B. B. (2014). High TNF-α Levels in Resting B Cells Negatively Correlate with Their Response. Exp. Gerontol. 54, 116–122. doi:10.1016/j.exger.2014.01.004
Frasca, D., Diaz, A., Romero, M., Landin, A. M., Phillips, M., Lechner, S. C., et al. (2010). Intrinsic Defects in B Cell Response to Seasonal Influenza Vaccination in Elderly Humans. Vaccine 28 (51), 8077–8084. doi:10.1016/j.vaccine.2010.10.023
Frasca, D., Diaz, A., Romero, M., Mendez, N. V., Landin, A. M., Ryan, J. G., et al. (2013). Young and Elderly Patients with Type 2 Diabetes Have Optimal B Cell Responses to the Seasonal Influenza Vaccine. Vaccine 31 (35), 3603–3610. doi:10.1016/j.vaccine.2013.05.003
Frasca, D., Diaz, A., Romero, M., Phillips, M., Mendez, N. V., Landin, A. M., et al. (2012). Unique Biomarkers for B-Cell Function Predict the Serum Response to Pandemic H1N1 Influenza Vaccine. Int. Immunol. 24 (3), 175–182. doi:10.1093/intimm/dxr123
Frasca, D., Ferracci, F., Diaz, A., Romero, M., Lechner, S., and Blomberg, B. B. (2016). Obesity Decreases B Cell Responses in Young and Elderly Individuals. Obesity 24 (3), 615–625. doi:10.1002/oby.21383
Frasca, D., Landin, A. M., Lechner, S. C., Ryan, J. G., Schwartz, R., Riley, R. L., et al. (2008). Aging Down-Regulates the Transcription Factor E2A, Activation-Induced Cytidine Deaminase, and Ig Class Switch in Human B Cells. J. Immunol. 180 (8), 5283–5290. doi:10.4049/jimmunol.180.8.5283
Golinski, M. L., Demeules, M., Derambure, C., Riou, G., Maho-Vaillant, M., Boyer, O., et al. (2020). CD11c(+) B Cells Are Mainly Memory Cells, Precursors of Antibody Secreting Cells in Healthy Donors. Front. Immunol. 11, 32. doi:10.3389/fimmu.2020.00032
Gu, J., Ye, S., Wang, S., Sun, W., and Hu, Y. (2014). Metformin Inhibits Nuclear Factor-Κb Activation and Inflammatory Cytokines Expression Induced by High Glucose via Adenosine Monophosphate-Activated Protein Kinase Activation in Rat Glomerular Mesangial Cells In Vitro. Chin. Med. J. (Engl) 127 (9), 1755–1760.
Guralnik, J. M., Ferrucci, L., Balfour, J. L., Volpato, S., and Di Iorio, A. (2001). Progressive versus Catastrophic Loss of the Ability to Walk: Implications for the Prevention of Mobility Loss. J. Am. Geriatr. Soc. 49 (11), 1463–1470. doi:10.1046/j.1532-5415.2001.4911238.x
Hotamisligil, G. S. (2017). Inflammation, Metaflammation and Immunometabolic Disorders. Nature 542 (7640), 177–185. doi:10.1038/nature21363
Huang, E. S., Laiteerapong, N., Liu, J. Y., John, P. M., Moffet, H. H., and Karter, A. J. (2014). Rates of Complications and Mortality in Older Patients with Diabetes Mellitus. JAMA Intern. Med. 174 (2), 251–258. doi:10.1001/jamainternmed.2013.12956
Illingworth, J., Butler, N. S., Roetynck, S., Mwacharo, J., Pierce, S. K., Bejon, P., et al. (2013). Chronic Exposure to Plasmodium Falciparum Is Associated with Phenotypic Evidence of B and T Cell Exhaustion. J.I. 190 (3), 1038–1047. doi:10.4049/jimmunol.1202438
Isoda, K., Young, J. L., Zirlik, A., MacFarlane, L. A., Tsuboi, N., Gerdes, N., et al. (2006). Metformin Inhibits Proinflammatory Responses and Nuclear Factor-Κb in Human Vascular Wall Cells. Atvb 26 (3), 611–617. doi:10.1161/01.atv.0000201938.78044.75
Kanigur Sultuybek, G., Soydas, T., and Yenmis, G. (2019). NF‐κB as the Mediator of Metformin's Effect on Ageing and Ageing‐related Diseases. Clin. Exp. Pharmacol. Physiol. 46 (5), 413–422. doi:10.1111/1440-1681.13073
Lee, S. Y., Lee, S. H., Yang, E. J., Kim, E. K., Kim, J. K., Shin, D. Y., et al. (2015). Metformin Ameliorates Inflammatory Bowel Disease by Suppression of the STAT3 Signaling Pathway and Regulation of the between Th17/Treg Balance. PLoS One 10 (9), e0135858. doi:10.1371/journal.pone.0135858
Li, S.-N., Wang, X., Zeng, Q.-T., Feng, Y.-B., Cheng, X., Mao, X.-B., et al. (2009). Metformin Inhibits Nuclear Factor κB Activation and Decreases Serum High-Sensitivity C-Reactive Protein Level in Experimental Atherogenesis of Rabbits. Heart Vessels 24 (6), 446–453. doi:10.1007/s00380-008-1137-7
Martorana, A., Balistreri, C. R., Bulati, M., Buffa, S., Azzarello, D. M., Camarda, C., et al. (2014). Double Negative (CD19+IgG+IgD−CD27−) B Lymphocytes: A New Insight from Telomerase in Healthy Elderly, in Centenarian Offspring and in Alzheimer's Disease Patients. Immunol. Lett. 162 (1 Pt B), 303–309. doi:10.1016/j.imlet.2014.06.003
McElhaney, J. E., Garneau, H., Camous, X., Dupuis, G., Pawelec, G., Baehl, S., et al. (2015). Predictors of the Antibody Response to Influenza Vaccination in Older Adults with Type 2 Diabetes. BMJ Open Diabetes Res. Care 3 (1), e000140. doi:10.1136/bmjdrc-2015-000140
Moir, S., Ho, J., Malaspina, A., Wang, W., DiPoto, A. C., O'Shea, M. A., et al. (2008). Evidence for HIV-Associated B Cell Exhaustion in a Dysfunctional Memory B Cell Compartment in HIV-Infected Viremic Individuals. J. Exp. Med. 205 (8), 1797–1805. doi:10.1084/jem.20072683
Muller, L. M. A. J., Gorter, K. J., Hak, E., Goudzwaard, W. L., Schellevis, F. G., Hoepelman, A. I. M., et al. (2005). Increased Risk of Common Infections in Patients with Type 1 and Type 2 Diabetes Mellitus. Clin. Infect. Dis. 41 (3), 281–288. doi:10.1086/431587
Murasko, D. M., Bernstein, E. D., Gardner, E. M., Gross, P., Munk, G., Dran, S., et al. (2002). Role of Humoral and Cell-Mediated Immunity in protection from Influenza Disease after Immunization of Healthy Elderly. Exp. Gerontol. 37 (2-3), 427–439. doi:10.1016/s0531-5565(01)00210-8
Muriach, M., Flores-Bellver, M., Romero, F. J., and Barcia, J. M. (2014). Diabetes and the Brain: Oxidative Stress, Inflammation, and Autophagy. Oxid Med. Cel Longev 2014, 102158. doi:10.1155/2014/102158
Nevalainen, T., Autio, A., Kummola, L., Salomaa, T., Junttila, I., Jylha, M., et al. (2019). CD27- IgD- B Cell Memory Subset Associates with Inflammation and Frailty in Elderly Individuals but Only in Males. Immun. Ageing 16, 19. doi:10.1186/s12979-019-0159-6
Nitzan, O., Elias, M., Chazan, B., and Saliba, W. (2015). Urinary Tract Infections in Patients with Type 2 Diabetes Mellitus: Review of Prevalence, Diagnosis, and Management. Diabetes Metab. Syndr. Obes. 8, 129–136. doi:10.2147/DMSO.S51792
Parish, S. T., Wu, J. E., and Effros, R. B. (2009). Modulation of T Lymphocyte Replicative Senescence via TNF-α Inhibition: Role of Caspase-3. J. Immunol. 182 (7), 4237–4243. doi:10.4049/jimmunol.0803449
Pozzilli, P., Gale, E. A. M., Visallil, N., Baroni, M., Crovari, P., Frighi, V., et al. (1986). The Immune Response to Influenza Vaccination in Diabetic Patients. Diabetologia 29 (12), 850–854. doi:10.1007/bf00870139
Prada-Medina, C. A., Fukutani, K. F., Pavan Kumar, N., Gil-Santana, L., Babu, S., Lichtenstein, F., et al. (2017). Systems Immunology of Diabetes-Tuberculosis Comorbidity Reveals Signatures of Disease Complications. Sci. Rep. 7 (1), 1999. doi:10.1038/s41598-017-01767-4
Prattichizzo, F., De Nigris, V., La Sala, L., Procopio, A. D., Olivieri, F., and Ceriello, A. (2016). Inflammaging" as a Druggable Target: A Senescence-Associated Secretory Phenotype-Centered View of Type 2 Diabetes. Oxid Med. Cel Longev 2016, 1810327. doi:10.1155/2016/1810327
Prattichizzo, F., De Nigris, V., Spiga, R., Mancuso, E., La Sala, L., Antonicelli, R., et al. (2018). Inflammageing and Metaflammation: The Yin and Yang of Type 2 Diabetes. Ageing Res. Rev. 41, 1–17. doi:10.1016/j.arr.2017.10.003
Pritz, T., Lair, J., Ban, M., Keller, M., Weinberger, B., Krismer, M., et al. (2015). Plasma Cell Numbers Decrease in Bone Marrow of Old Patients. Eur. J. Immunol. 45 (3), 738–746. doi:10.1002/eji.201444878
Ruderman, N., and Prentki, M. (2004). AMP Kinase and Malonyl-CoA: Targets for Therapy of the Metabolic Syndrome. Nat. Rev. Drug Discov. 3 (4), 340–351. doi:10.1038/nrd1344
Saadoun, D., Terrier, B., Bannock, J., Vazquez, T., Massad, C., Kang, I., et al. (2013). Expansion of Autoreactive Unresponsive CD21−/lowB Cells in Sjögren's Syndrome-Associated Lymphoproliferation. Arthritis Rheum. 65 (4), 1085–1096. doi:10.1002/art.37828
Sabry, D., Abdelaleem, O. O., El Amin Ali, A. M., Mohammed, R. A., Abdel-Hameed, N. D., Hassouna, A., et al. (2019). Anti-proliferative and Anti-apoptotic Potential Effects of Epigallocatechin-3-Gallate And/or Metformin on Hepatocellular Carcinoma Cells: In Vitro Study. Mol. Biol. Rep. 46 (2), 2039–2047. doi:10.1007/s11033-019-04653-6
Sasaki, S., He, X. S., Holmes, T. H., Dekker, C. L., Kemble, G. W., Arvin, A. M., et al. (2008). Influence of Prior Influenza Vaccination on Antibody and B-Cell Responses. PloS one 3 (8), e2975. doi:10.1371/journal.pone.0002975
Sasaki, S., Sullivan, M., Narvaez, C. F., Holmes, T. H., Furman, D., Zheng, N.-Y., et al. (2011). Limited Efficacy of Inactivated Influenza Vaccine in Elderly Individuals Is Associated with Decreased Production of Vaccine-specific Antibodies. J. Clin. Invest. 121 (8), 3109–3119. doi:10.1172/jci57834
Sekino, N., Kano, M., Matsumoto, Y., Sakata, H., Akutsu, Y., Hanari, N., et al. (2018). Antitumor Effects of Metformin Are a Result of Inhibiting Nuclear Factor Kappa B Nuclear Translocation in Esophageal Squamous Cell Carcinoma. Cancer Sci. 109 (4), 1066–1074. doi:10.1111/cas.13523
Shi, Y., Yamazaki, T., Okubo, Y., Uehara, Y., Sugane, K., and Agematsu, K. (2005). Regulation of Aged Humoral Immune Defense against Pneumococcal Bacteria by IgM Memory B Cell. J. Immunol. 175 (5), 3262–3267. doi:10.4049/jimmunol.175.5.3262
Singh, V. P., Bali, A., Singh, N., and Jaggi, A. S. (2014). Advanced Glycation End Products and Diabetic Complications. Korean J. Physiol. Pharmacol. 18 (1), 1–14. doi:10.4196/kjpp.2014.18.1.1
Skowronski, D. M., Tweed, S. A., and De Serres, G. (2008). Rapid Decline of Influenza Vaccine-Induced Antibody in the Elderly: Is it Real, or Is it Relevant?. J. Infect. Dis. 197 (4), 490–502. doi:10.1086/524146
Smith, S. A., and Poland, G. A. (2000). Use of Influenza and Pneumococcal Vaccines in People with Diabetes. Diabetes Care 23 (1), 95–108. doi:10.2337/diacare.23.1.95
Son, H. J., Lee, J., Lee, S. Y., Kim, E. K., Park, M. J., Kim, K. W., et al. (2014). Metformin Attenuates Experimental Autoimmune Arthritis through Reciprocal Regulation of Th17/Treg Balance and Osteoclastogenesis. Mediators Inflamm. 2014, 973986. doi:10.1155/2014/973986
Wang, S., Wang, J., Kumar, V., Karnell, J. L., Naiman, B., Gross, P. S., et al. (2018). IL-21 Drives Expansion and Plasma Cell Differentiation of Autoreactive CD11c(hi)T-Bet(+) B Cells in SLE. Nat. Commun. 9 (1), 1758. doi:10.1038/s41467-018-03750-7
Wang, Y., Lin, C., Han, R., Lu, C., Li, L., Hu, C., et al. (2020). Metformin Attenuates TGF-Beta1-Induced Pulmonary Fibrosis through Inhibition of Transglutaminase 2 and Subsequent TGF-Beta Pathways. 3 Biotech. 10 (6), 287. doi:10.1007/s13205-020-02278-2
Wehr, C., Eibel, H., Masilamani, M., Illges, H., Schlesier, M., Peter, H.-H., et al. (2004). A New CD21low B Cell Population in the Peripheral Blood of Patients with SLE. Clin. Immunol. 113 (2), 161–171. doi:10.1016/j.clim.2004.05.010
Woodruff, M. C., Ramonell, R. P., Nguyen, D. C., Cashman, K. S., Saini, A. S., Haddad, N. S., et al. (2020). Extrafollicular B Cell Responses Correlate with Neutralizing Antibodies and Morbidity in COVID-19. Nat. Immunol. 21 (12), 1506–1516. doi:10.1038/s41590-020-00814-z
Yan, L. J. (2014). Pathogenesis of Chronic Hyperglycemia: from Reductive Stress to Oxidative Stress. J. Diabetes Res. 2014, 137919. doi:10.1155/2014/137919
Yin, Y., Choi, S.-C., Xu, Z., Perry, D. J., Seay, H., Croker, B. P., et al. (2015). Normalization of CD4 + T Cell Metabolism Reverses Lupus. Sci. Transl. Med. 7 (274), 274ra18. doi:10.1126/scitranslmed.aaa0835
Keywords: aging, Type-2 Diabetes Mellitus, B cells, inflammation, autoimmunity
Citation: Frasca D, Diaz A, Romero M and Blomberg BB (2021) Metformin Enhances B Cell Function and Antibody Responses of Elderly Individuals With Type-2 Diabetes Mellitus. Front. Aging 2:715981. doi: 10.3389/fragi.2021.715981
Received: 27 May 2021; Accepted: 12 July 2021;
Published: 23 July 2021.
Edited by:
Jenna M Bartley, University of Connecticut Health Center, United StatesReviewed by:
Niharika Arora Duggal, University of Birmingham, United KingdomCarey Shive, Louis Stokes Cleveland VA Medical Center, United States
Copyright © 2021 Frasca, Diaz, Romero and Blomberg. This is an open-access article distributed under the terms of the Creative Commons Attribution License (CC BY). The use, distribution or reproduction in other forums is permitted, provided the original author(s) and the copyright owner(s) are credited and that the original publication in this journal is cited, in accordance with accepted academic practice. No use, distribution or reproduction is permitted which does not comply with these terms.
*Correspondence: Daniela Frasca, ZGZyYXNjYUBtZWQubWlhbWkuZWR1