- 1Department of Radiology, University Medical Center Utrecht and Utrecht University, Utrecht, Netherlands
- 2Julius Center for Health Sciences and Primary Care, University Medical Center Utrecht and Utrecht University, Utrecht, Netherlands
- 3Department of General Practice, Amsterdam UMC, Location University of Amsterdam, Amsterdam, Netherlands
- 4Amsterdam Public Health, Aging & Later Life, and Personalized Medicine, Amsterdam, Netherlands
- 5Amsterdam Neuroscience, Neurodegeneration, and Mood, Anxiety, Psychosis, Stress, and Sleep, Amsterdam, Netherlands
Background and purpose: Arterial calcifications on unenhanced CT scans and vessel wall lesions on MRI are often used interchangeably to portray intracranial arterial disease. However, the extent of pathology depicted with each technique is unclear. We investigated the presence and distribution of these two imaging findings in patients with a history of cerebrovascular disease.
Materials and methods: We analyzed CT and MRI data from 78 patients admitted for stroke or TIA at our institution. Vessel wall lesions were assessed on 7 T MRI sequences, while arterial calcifications were assessed on CT scans. The number of vessel wall lesions, severity of intracranial internal carotid artery (iICA) calcifications, and overall presence and distribution of the two imaging findings were visually assessed in the intracranial arteries.
Results: At least one vessel wall lesion or arterial calcification was assessed in 69 (88%) patients. Only the iICA and vertebral arteries (VA) showed a substantial number of both calcifications and vessel wall lesions. The other vessels showed almost exclusively vessel wall lesions. The number of vessel wall lesions was associated with the severity of iICA calcification (p = 0.013).
Conclusions: The number of vessel wall lesions increases with the severity of iICA calcifications. Nonetheless, the distribution of vessel wall lesions on MRI and arterial calcifications on CT shows remarkable differences. These findings support the need for a combined approach to examine intracranial arterial disease.
Introduction
Intracranial arterial disease is a major cause of ischemic cerebrovascular disease worldwide (1). While angiographic techniques are traditionally used to depict vessel lumen and identify stenosis, they are not suited to examine the arterial wall where the disease originates (2). Intracranial arterial disease can also manifest with non-stenosing lesions, and the composition of lesions can differ (3). Therefore, focusing exclusively on stenosis to define intracranial arterial disease can hamper progress in understanding this major disease (4).
Two imaging techniques are available that can provide information on the intracranial arterial wall. Unenhanced CT is the best method to visualize calcifications, while high-resolution intracranial vessel wall MR sequences are superior to CT angiography in visualizing vessel wall lesions, defined as focal or concentric thickenings of the arterial wall (5). These two imaging proxies of intracranial arterial disease are often used interchangeably to describe “intracranial atherosclerosis”. However, they depict various stages of arterial disease and sometimes different etiologies. Calcification occurs in atherosclerotic plaque formation, as well as in non-atherosclerotic diseases like Mönckeberg's calcification (6–8). In contrast, vessel wall lesions are due to infiltration of inflammatory mediators and lipid deposition in response to endothelial damage that marks the beginning of the atherosclerotic remodeling in most cases (9–12). Therefore, a clear understanding of intracranial arterial disease, as illustrated by these two imaging findings, is still required.
To shed light on the relation between intracranial calcifications and vessel wall lesions and their potential to depict intracranial arterial disease, the current study compared the presence and distribution of these two imaging findings in a cohort of patients with known cerebrovascular disease using unenhanced CT and 7 T MRI.
Materials and methods
Participants
For this study, we combined data from two clinical studies conducted at our institution: the Intracranial Vessel wall Imaging (IVI) study (62 patients) and the Posterior Intracranial Vessel wall Imaging (PIVI) study (16 patients). The IVI study included patients aged 18 years and older with a clinical history of acute ischemic stroke with a total or partial anterior circulation infarct or with a transient ischemic attack (TIA) which displayed symptoms related to the anterior circulation. The PIVI study included patients aged 18 years and older with ischemic stroke or TIA affecting the posterior cerebral circulation. Additional information on study inclusion and exclusion criteria, diagnostic criteria, and a flowchart of the study samples are provided in the Supplementary material. Both studies were approved by the review board of our institution, and written informed consent was obtained from all patients (10, 13).
As part of the protocol for both the IVI and PIVI study, all patients underwent 7 T MRI, including an intracranial vessel wall MRI sequence, within three months of onset of cerebrovascular symptoms. To undergo the 7 T MRI protocol only TIA patients or patients with minor to moderate stroke could be included as reflected by a NIH stroke scale at 1 week from the cerebrovascular event in the IVI study population of 5 points. Additionally, all patients admitted to our hospital with a possible cerebrovascular event underwent an unenhanced CT and a CT angiography as part of the clinical work-up for suspected cerebrovascular events. The CT examinations performed at our institution were retrieved and included in this study for analysis.
MRI protocol
The MRI was conducted on a 7 T whole-body system (Philips Healthcare, Best, The Netherlands) with either a 16-channel (until May 2015) or 32-channel receive coil and volume transmit/receive coil for transmission (Nova Medical, Wilmington, MA, USA). Concerning the IVI study, for patients (n = 31) included until mid-July 2011 a reduced FOV 3D T1-weighted magnetization-prepared inversion recovery turbo spin echo (MPIR-TSE) sequence for visualization of the vessel wall was used, which excluded visualization of part of the basilar artery (BA) and vertebral arteries (VA). The scan parameters of this sequence have been described previously; in short, these were: FOV 220 × 180 × 13 mm3 in the transverse plane, acquired resolution 0.8 × 0.8 × 0.8 mm3, TSE factor+startup echoes 116, TR/TI/TE 6050/1770/80 ms, number of signal averages 2, and acquisition time ∼12 min (14). Due to technical developments, the remaining patients included in the IVI study (n = 31) and all patients in the PIVI study (n = 16) underwent a whole-brain 3D T1-weighted MPIR-TSE vessel wall sequence including the BA and VA. The scan parameters applied were: FOV 250 × 250 × 190 mm3 in sagittal plane, acquired resolution 0.8 × 0.8 × 0.8 mm3, TR/TI/TE 3952/1375/37 ms, TSE factor+startup echoes 168, number of signal averages 2, and acquisition time ∼11 min.
CT protocol
Unenhanced CT and CTA images were obtained using either a Philips Brilliance 64-slice or iCT 256-slice CT scanner (Philips Healthcare, Best, The Netherlands) at 80–120 kVP and 250 mAs (adjusted based on patient body composition).
MRI assessment
The detailed scoring method of vessel wall lesions and measures of agreement between the raters have been previously described. Results of the interim analyses have been published (10, 13). The presence of one or more vessel wall lesions was assessed at 23 sites throughout the intracranial arterial circulation, which were subsequently grouped in 7 artery types (Supplementary material). According to previous literature, these segments can be divided according to their dimensions in large (MCA, ICA, VA and BA), medium (ACA and PCA) and small (PCOM) (15). The definition of a vessel wall lesion was either a focal or diffuse thickening of the vascular wall compared to the healthy contralateral or contiguous wall. The presence or absence of enhancement after administration of Gadolinium contrast agent was also assessed in the original studies. However, since vessel wall enhancement can be observed in atherosclerosis, as well as in other inflammatory and non-inflammatory diseases of the brain vasculature (i.e., vasculitis, amyloid deposition) (16, 17), we decided to remove this from our current analysis. Figure 1 shows an example of an intracranial vessel wall lesion detected on 7 T MRI.
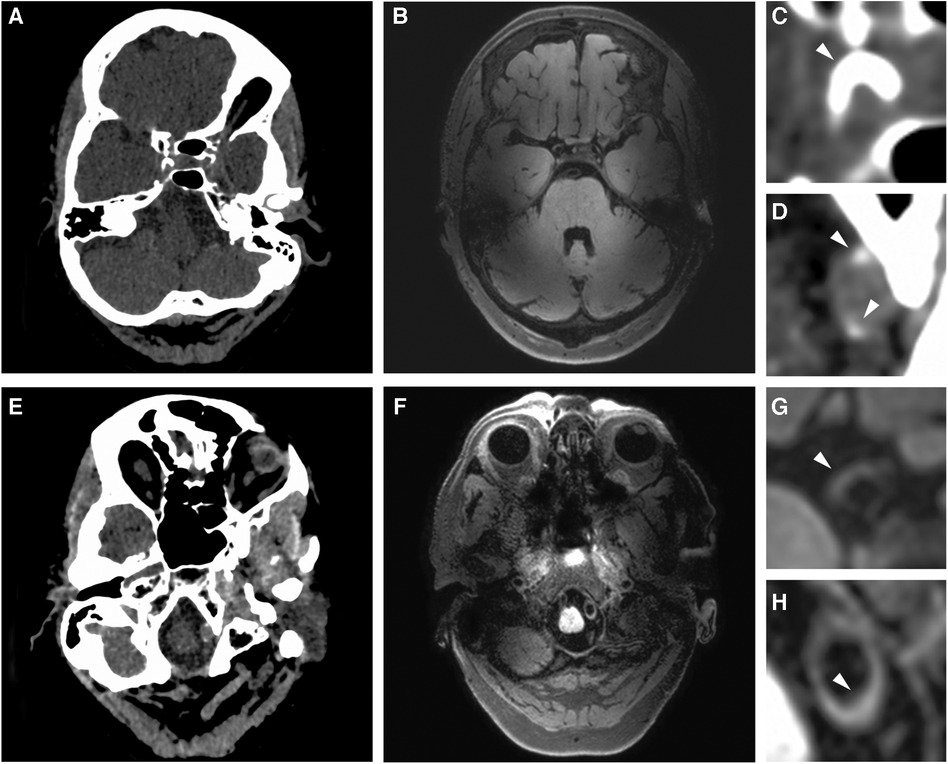
Figure 1. Imaging proxies of intracranial arterial disease. (A,E) Example of intracranial artery calcification on axial non-contrast head CT. (B,F) Example of vessel wall lesions on 7 T MRI. Zoomed images show: (C) a thick arterial calcification in the right ICA segment; (D) two calcified spots in the left VA segment; (G) a vessel wall lesion in the right ICA; (H) vessel wall lesion affecting a portion of the left VA.
CT assessment
We analyzed unenhanced CT images of the cranium from the skull base to the vertex, reconstructed with a slice thickness ranging from 0.625 to 1 mm. The presence of calcification was evaluated as yes or no in the 23 sites of the intracranial arterial circulation by one neuroradiologist (JWD—more than 10 years of experience). The CTA images were used to aid in identifying the specific arteries. In addition, the severity of calcification in the intracranial internal carotid artery (iICA) was determined by one rater (CL—3 years of experience), according to the Woodcock score [15]. This scale distinguishes four levels of calcification severity according to thickness and continuity: absent, mild, moderate, and severe. The score is easy to learn and has been thoroughly validated, showing extremely high intra- and inter-rater reliability (18, 19). Therefore, we decided not to repeat the scoring between raters. Both raters were blinded to the clinical data and the vessel wall MRI assessment. The images were analyzed with free adjustment of the viewing plane via MPR and window level settings, using Sectra Workstation IDS7 19.3 (Sectra AB, Linköping, Sweden). Figure 1 displays an example of intracranial arterial calcifications detected on CT.
Statistical analysis
First, we described the baseline patient characteristics of the study sample. Second, we assessed the overlap and differences in the distribution of vessel wall lesions and calcification for each arterial segment available, distinguishing absence of any imaging finding, exclusive vessel wall lesions, exclusive calcifications, or co-existence of the two. Third, to determine whether arterial disease as depicted by vessel wall lesions on MRI correlates with calcifications on CT, we used the Woodcock score in the ICA as a surrogate for the overall intracranial extent of calcifications. We then used the Jonckheere-Terpstra test (TJT) for ordered alternatives to test this relationship. Results were statistically significant at a p-value of ≤0.05. We used IBM SPSS Statistics (version 25 for Windows, IBM Corporation, Armonk, NY, USA) for the statistical analysis.
Results
The mean age of the study sample (n = 78) was 60 ± 13 years, and 44% were women. Table 1 presents the baseline characteristics of the IVI and PIVI study patients. Of all patients, 58 (75%) had a stroke, 19 (24%) had a TIA, and 1 (1%) had another ischemic event. One-third of patients had received thrombolytic treatment. The median time between symptoms and the 7 T MRI examination was 6 days (10–90 percentile, 2–65), and 0 days for the CT examination (10–90 percentile, 0–5).
Distribution of vessel wall lesions and arterial calcifications
The median number of vessel wall lesions per patient on MRI was 3 (range 0–23). Of patients, 89% (69/78) showed at least one arterial segment with a vessel wall lesion, and 89% (69/78) showed at least one calcified intracranial artery. ICA calcifications were mild in 22 patients, moderate in 38, and severe in 9 patients, while 9 patients presented with no calcifications at all. Supplementary material provides counts and percentages of vessel wall lesions on MRI and calcifications on CT for each artery type.
Correlation between the imaging proxies
Sixty-two of the 78 patients presented with both vessel wall lesions and arterial calcifications: 14 patients showed calcification without any vessel wall lesion (n = 7) or vessel wall lesion(s) without calcification (n = 7); 2 patients did not show any presence of intracranial arterial disease. Figure 2 shows a significantly higher median burden of vessel wall lesions in patients with severe calcification of the iICA on the Woodcock score (TJT-statistic = 1.26, z = 2.48, p = 0.013). No statistical difference was noted between the burden and vessel wall lesions and absent, mild, and moderate levels of iICA calcification as assessed by the Woodcock score.
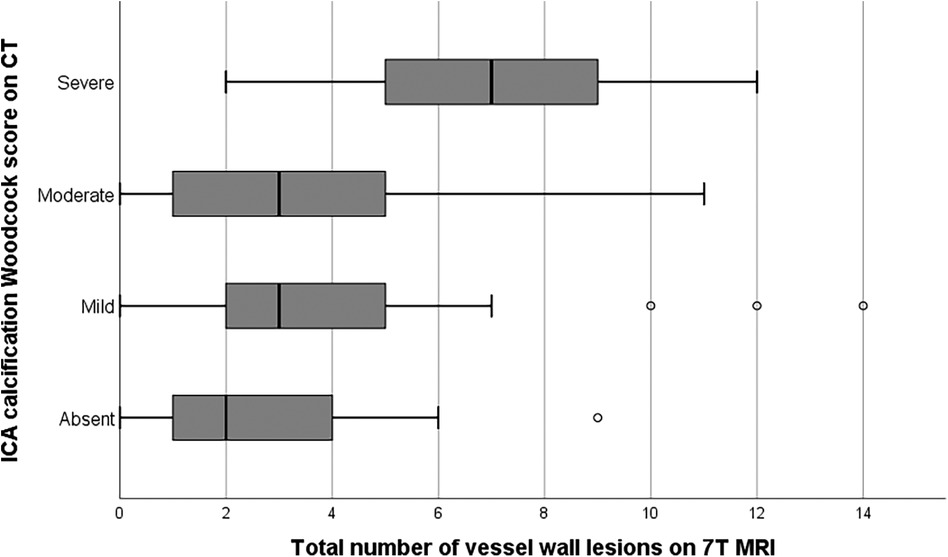
Figure 2. Bar graph showing the relation between vessel wall lesion burden and calcification severity in the internal carotid artery. Extreme values have been excluded from the image for better illustration of the trend of the association.
Overlap and differences between the imaging proxies
Figure 3 shows the minor overlap and major differences between the two imaging proxies of intracranial arterial disease. Only the iICA and the VA showed a substantial number of calcifications as well as vessel wall lesions while the other vessels (ACA, MCA, PCA, and PCOM) showed almost exclusively vessel wall lesions. 32% of the iICA arterial segments showed both calcifications and vessel wall lesions, 51% showed only calcification, and few segments (3%) showed exclusive vessel wall lesions. In 22% of the VA, both imaging proxies occurred together, 29% showed exclusively vessel wall lesions, and 12% manifested exclusive calcifications. 3% of the BA segments presented exclusive calcifications, while 57% showed only vessel wall lesions.
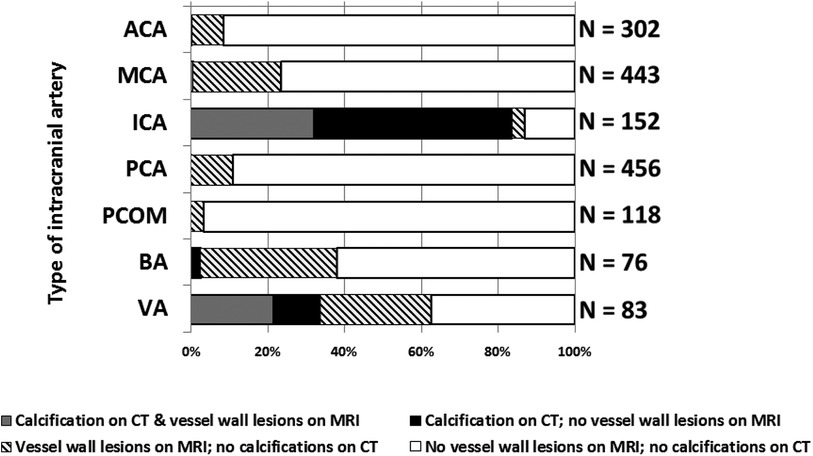
Figure 3. Graph showing the distribution of intracranial vessel wall lesions and arterial calcifications in 7 different arterial segments. Data from left and right segments are showed together. ACA, anterior cerebral artery; MCA, middle cerebral artery; PCA, posterior cerebral artery; PCOM, posterior communicating artery; BA, basilar artery; VA, vertebral artery. Left and right segments taken together (if applicable).
Discussion
This study assessed the intracranial distribution of arterial calcifications on unenhanced CT and vessel wall lesions on 7 T MRI in patients with known cerebrovascular disease. We found major differences between the distribution of these imaging findings that are frequently used as intracranial atherosclerotic disease indicators and only a significant association of severe iICA calcification as defined by the Woodcock score and the overall burden of MRI vessel wall lesions.
Calcifications vs. vessel wall lesions
The overall burden of vessel wall lesions in the intracranial arterial circulation is associated with the severity of iICA calcifications. Severe carotid artery calcification in stroke patients could help identify patients eligible for an MRI vessel wall examination in the clinical setting given the higher burden of non-calcified intracranial arterial disease these patients might carry. However, vessel wall pathology assessed with MRI showed a profoundly different distribution pattern compared to arterial calcification on unenhanced CT. Advanced atherosclerotic plaques (fibrous and calcified) affect mainly the large arteries of the brain (namely iICA, VA, BA, and MCA) while medium and small arteries (ACA, PCA, and PCOM) are more affected by early-stage atherosclerotic lesions (manifesting only as intimal thickening and fatty streaks).
The iICA showed calcification in 84% of cases vs. approximately 35% that showed vessel wall lesions, while in the VA approximately 30% of segments presented with calcifications vs. ca. 50% that had vessel wall lesions. The discrepancy between anterior and posterior circulation could be due to the reliability of MR vessel wall lesion assessment in the internal carotid artery, which has the lowest reliability due to its tortuous course and location at the skull base. Severe calcifications could also hamper evaluation of vessel wall thickness. Arterial wall lesions in the anterior brain vasculature could be left unidentified and cause underestimation of total disease burden.
Distribution of imaging proxies of intracranial arterial disease
From previous literature, overall prevalence of arterial calcifications is highest in the iICA (∼60%) followed by the VA (∼20%), MCA (∼5%), and BA (∼5%), while the PCA and ACA are rarely affected (18). The distribution of arterial calcification in our study sample follows the same pattern, albeit with an overall higher frequency of calcifications due to the selection of patients with known cerebrovascular disease. Intracranial artery calcifications are more prevalent in stroke patients and are recognized as an independent risk factor for stroke (20–22). A study of patients with internal carotid artery stenosis ≥60% found that calcified atherosclerotic plaques in the carotid artery were 21 times less likely to be symptomatic compared to non-calcified plaques, even after adjusting for traditional cardiovascular risk factors (5, 23). This evidence suggests that the presence of calcification alone is not sufficient for accurately predicting cerebrovascular disease.
Intracranial vessel wall imaging has seen a recent growth in popularity for the characterization of vessel wall pathology (24). The prevalence of at least one intracranial vessel wall lesion was estimated to be around 40% in a recent population-based study focusing on vessel wall MRI (25). In the current study, performed in patients with known history of ischemic stroke or TIA, we found an overall prevalence of 89%. This may be due to the use of 7 T MRI data, which has shown to be more sensitive to subtle thickening of the intracranial vessel wall (26). Additionally, patients with known cerebrovascular disease were selected, which increases the likelihood of a higher burden of intracranial arterial disease (27). Therefore the use of vessel wall MRI is certainly valuable for secondary prevention of ischemic events. However, the prognostic value of vessel wall lesions assessment in vivo in still asymptomatic patients has not yet been established.
Strengths and limitations
This study is among the first to investigate two imaging proxies of intracranial arterial disease thanks to the availability of both clinically acquired unenhanced CT and vessel wall MRI data in patients with known cerebrovascular disease. While recent 3 T studies also showed the ability to depict vessel wall lesions with high accuracy, 7 T MRI proved to be more sensitive to small vessel wall lesions due to its higher spatial resolution and contrast-to-noise ratio (27, 28).
There are several limitations to the current investigation that deserve mention. Our analyses were performed on a relatively small group of stroke/TIA patients with good physical status, which may not reflect the severe stroke population. Nonetheless, including healthier patients would only dilute the correlation we found, not invalidate our results. Additionally, obtaining histological data in patients with intracranial atherosclerosis is virtually impossible, which limits the possibility of confirming the nature of our imaging findings. We focus only on intracranial arteries without providing information about extracranial arterial disease which is known to be a significant risk factor for stroke (29). However, previous research shows that the risk factors for intracranial and extracranial arterial disease are different (30) and therefore might depict different causes of arterial disease. Future studies are needed to assess the distribution and the relation between these imaging findings in intracranial and extracranial arteries.
Conclusion
We discovered a significant relationship between the severity of arterial calcifications and vessel wall lesions in intracranial arteries. That being said, we also noticed significant differences in the distribution of intracranial arterial calcification on CT scans and arterial lesion assessments on vessel wall MRI. The differences suggest that these two imaging findings might depict different types of intracranial diseases, in light of the recent findings of intracranial non-atherosclerotic medial calcification, or various stages of atherosclerotic disease. Therefore, a combination of both imaging modalities could provide complementary information to non-invasively depict the real burden of intracranial arterial disease considering its relation with an increasing risk of stroke.
Data availability statement
The data analyzed in this study is subject to the following licenses/restrictions: Patient data. Requests to access these datasets should be directed to Jeroen Hendrikse, j.hendrikse@umcutrecht.nl.
Ethics statement
The studies involving humans were approved by Ethics committee of UMC Utrecht. The studies were conducted in accordance with the local legislation and institutional requirements. The participants provided their written informed consent to participate in this study.
Author contributions
CL: Conceptualization, Data curation, Formal Analysis, Investigation, Methodology, Project administration, Visualization, Writing – original draft. IR: Conceptualization, Formal Analysis, Investigation, Methodology, Project administration, Writing – review & editing. RAPT: Conceptualization, Investigation, Methodology, Writing – review & editing. AGvdK: Conceptualization, Investigation, Methodology, Writing – review & editing. AH: Conceptualization, Investigation, Methodology, Writing – review & editing. JWD: Conceptualization, Investigation, Methodology, Writing – review & editing. MIG: Conceptualization, Investigation, Methodology, Supervision, Writing – review & editing. PAdJ: Conceptualization, Investigation, Methodology, Supervision, Writing – review & editing. JH: Conceptualization, Funding acquisition, Investigation, Methodology, Project administration, Supervision, Writing – review & editing.
Funding
The author(s) declare financial support was received for the research, authorship, and/or publication of this article.
This research has been made possible by the Dutch Heart Foundation and the Netherlands Organization for Scientific Research (NWO), as part of their joint strategic research program: “Earlier recognition of cardiovascular diseases”. This project is partially financed by the PPP Allowance made available by Top Sector Life Sciences & Health to the Dutch Heart foundation to stimulate public-private partnerships.
Acknowledgments
We thank Arjen Lindenholz and Nikki Dieleman for their support in data collection and files management.
Conflict of interest
The authors declare that the research was conducted in the absence of any commercial or financial relationships that could be construed as a potential conflict of interest.
Publisher's note
All claims expressed in this article are solely those of the authors and do not necessarily represent those of their affiliated organizations, or those of the publisher, the editors and the reviewers. Any product that may be evaluated in this article, or claim that may be made by its manufacturer, is not guaranteed or endorsed by the publisher.
Supplementary material
The Supplementary Material for this article can be found online at: https://www.frontiersin.org/articles/10.3389/fradi.2024.1338418/full#supplementary-material
References
1. Arenillas JF. Intracranial atherosclerosis. Stroke. (2011) 42:S20–3. doi: 10.1161/STROKEAHA.110.597278
2. Holmstedt CA, Turan TN, Chimowitz MI. Atherosclerotic intracranial arterial stenosis: risk factors, diagnosis, and treatment. Lancet Neurol. (2013) 12(11):1106–14. doi: 10.1016/S1474-4422(13)70195-9
3. Qureshi AI, Caplan LR. Intracranial atherosclerosis. Lancet. (2014) 383(9921):984–98. doi: 10.1016/S0140-6736(13)61088-0
4. Leng X, Wong KS, Liebeskind DS. Evaluating intracranial atherosclerosis rather than intracranial stenosis. Stroke. (2014) 45(2):645–51. doi: 10.1161/STROKEAHA.113.002491
5. Saba L, Yuan C, Hatsukami TS, Balu N, Qiao Y, DeMarco JK, et al. Carotid artery wall imaging: perspective and guidelines from the ASNR vessel wall imaging study group and expert consensus recommendations of the American society of neuroradiology. Am J Neuroradiol. (2018) 39(2):E9–E31. doi: 10.3174/ajnr.A5488
6. Wen-Jie Y, Ka-Sing W, Xiang-Yan C. Intracranial atherosclerosis: from microscopy to high-resolution magnetic resonance imaging. J Stroke. (2017) 19(3):249–60. doi: 10.5853/jos.2016.01956
7. Vos A, Van Hecke W, Spliet WGM, Goldschmeding R, Isgum I, Kockelkoren R, et al. Predominance of nonatherosclerotic internal elastic lamina calcification in the intracranial internal carotid artery. Stroke. (2016) 47(1):221–3. doi: 10.1161/STROKEAHA.115.011196
8. Kockelkoren R, Vos A, Van Hecke W, Vink A, Bleys RLAW, Verdoorn D, et al. Computed tomographic distinction of intimal and medial calcification in the intracranial internal carotid artery. PLoS One. (2017) 12(1):e0168360. doi: 10.1371/journal.pone.0168360
9. Dieleman N, van der Kolk AG, Zwanenburg JJM, Harteveld AA, Biessels GJ, Luijten PR, et al. Imaging intracranial vessel wall pathology with magnetic resonance imaging current prospects and future directions. Circulation. (2014) 130(2):192–201. doi: 10.1161/CIRCULATIONAHA.113.006919
10. Harteveld AA, van der Kolk AG, van der Worp HB, Dieleman N, Zwanenburg JJM, Luijten PR, et al. Detecting intracranial vessel wall lesions with 7T-magnetic resonance imaging: patients with posterior circulation ischemia versus healthy controls. Stroke. (2017) 48(9):2601–4. doi: 10.1161/STROKEAHA.117.017868
11. van der Kolk AG, Zwanenburg JJM, Denswil NP, Vink A, Spliet WGM, Daemen MJAP, et al. Imaging the intracranial atherosclerotic vessel wall using 7T MRI: initial comparison with histopathology. Am J Neuroradiol. (2015) 36(4):694–701. doi: 10.3174/ajnr.A4178
12. Mandell DM, Mossa-Basha M, Qiao Y, Hess CP, Hui F, Matouk C, et al. Intracranial vessel wall MRI: principles and expert consensus recommendations of the American society of neuroradiology. Am J Neuroradiol. (2017) 38(2):218–29. doi: 10.3174/ajnr.A4893
13. van der Kolk AG, Zwanenburg JJM, Brundel M, Biessels GJ, Visser F, Luijten PR, et al. Distribution and natural course of intracranial vessel wall lesions in patients with ischemic stroke or TIA at 7.0 tesla MRI. Eur Radiol. (2015) 25(6):1692–700. doi: 10.1007/s00330-014-3564-4
14. van der Kolk AG, Hendrikse J, Brundel M, Biessels GJ, Smit EJ, Visser F, et al. Multi-sequence whole-brain intracranial vessel wall imaging at 7.0 tesla. Eur Radiol. (2013) 23(11):2996–3004. doi: 10.1007/s00330-013-2905-z
15. Denswil NP, van der Wal AC, Ritz K, de Boer OJ, Aronica E, Troost D, et al. Atherosclerosis in the circle of Willis: spatial differences in composition and in distribution of plaques. Atherosclerosis. (2016) 251:78–84. doi: 10.1016/j.atherosclerosis.2016.05.047
16. Arenillas JF, Dieleman N, Bos D. Intracranial arterial wall imaging: techniques, clinical applicability, and future perspectives. Int J Stroke. (2019) 14(6):564–73. doi: 10.1177/1747493019840942
17. Hao Q, Tsankova NM, Shoirah H, Kellner CP, Nael K. Vessel wall MRI enhancement in noninflammatory cerebral amyloid angiopathy. Am J Neuroradiol. (2020) 41(3):446–8. doi: 10.3174/AJNR.A6445
18. Woodcock RJ, Goldstein JH, Kallmes DF, Cloft HJ, Phillips CD. Angiographic correlation of CT calcification in the carotid siphon. Am J Neuroradiol. (1999) 20(3):495–9.10219418
19. Subedi D, Zishan US, Chappell F, Gregoriades M, Sudlow C, Sellar R, et al. Intracranial carotid calcification on cranial computed tomography: visual scoring methods, semiautomated scores, and volume measurements in patients with stroke. Stroke. (2015) 46(9):2504–9. doi: 10.1161/STROKEAHA.115.009716
20. Wu XH, Chen XY, Wang LJ, Wong KS. Intracranial artery calcification and its clinical significance. J Clin Neurol. (2016) 12(3):253–61. doi: 10.3988/jcn.2016.12.3.253
21. Chen XY, Lam WWM, Ng HK, Fan YH, Wong KS. The frequency and determinants of calcification in intracranial arteries in Chinese patients who underwent computed tomography examinations. Cerebrovasc Dis. (2006) 21(1–2):91–7. doi: 10.1159/000090206
22. Bos D, Portegies MLP, van der Lugt A, Bos MJ, Koudstaal PJ, Hofman A, et al. Intracranial carotid artery atherosclerosis and the risk of stroke in whites: the Rotterdam study. JAMA Neurol. (2014) 71(4):405–11. doi: 10.1001/jamaneurol.2013.6223
23. Quiney B, Ying SM, Hippe DS, Balu N, Urdaneda-Moncada AR, Mossa-Basha M. The association of intracranial vascular calcification and stenosis with acute ischemic cerebrovascular events. J Comput Assist Tomogr. (2017) 41(6):849–53. doi: 10.1097/RCT.0000000000000629
24. Lindenholz A, van der Kolk AG, Zwanenburg JJM, Hendrikse J. The use and pitfalls of intracranial vessel wall imaging: how we do it. Radiology. (2018) 286(1):12–28. doi: 10.1148/radiol.2017162096
25. Nandalur KR, Baskurt E, Hagspiel KD, Phillips CD, Kramer CM. Calcified carotid atherosclerotic plaque is associated less with ischemic symptoms than is noncalcified plaque on MDCT. AJR Am J Roentgenol. (2005) 184(1):295–8. doi: 10.2214/ajr.184.1.0184029
26. Zwartbol MHT, van der Kolk AG, Ghaznawi R, van der Graaf Y, Hendrikse J, Geerlings MI. Intracranial vessel wall lesions on 7T MRI (magnetic resonance imaging). Stroke. (2018) 50:88–94. doi: 10.1161/STROKEAHA.118.022509
27. van der Kolk AG, Hendrikse J, Luijten PR. Ultrahigh-field magnetic resonance imaging: the clinical potential for anatomy, pathogenesis, diagnosis, and treatment planning in brain disease. Neuroimaging Clin N Am. (2012) 22(2):343–62. doi: 10.1016/j.nic.2012.02.004
28. Schenck JF. The role of magnetic susceptibility in magnetic resonance imaging: MRI magnetic compatibility of the first and second kinds. Med Phys. (1996) 23(6):815–50. doi: 10.1118/1.597854
29. Abbot AL. Extra-cranial carotid artery stenosis: an objective analysis of the available evidence. Front Neurol. (2022) 13. doi: 10.3389/fneur.2022.739999
Keywords: intracranial arterial calcification, stroke, vessel wall imaging MRI, CT scan (CT), magnetic resonance imaging (MRI)
Citation: Lucci C, Rissanen I, Takx RAP, van der Kolk AG, Harteveld AA, Dankbaar JW, Geerlings MI, de Jong PA and Hendrikse J (2024) Imaging of intracranial arterial disease: a comparison between MRI and unenhanced CT. Front. Radiol. 4:1338418. doi: 10.3389/fradi.2024.1338418
Received: 14 November 2023; Accepted: 29 January 2024;
Published: 15 February 2024.
Edited by:
Nico Sollmann, University of California, San Francisco, United StatesReviewed by:
Agostino Tessitore, University of Messina, ItalyCamilla Russo, Santobono-Pausilipon Children’s Hospital, Italy
© 2024 Lucci, Rissanen, Takx, van der Kolk, Harteveld, Dankbaar, Geerlings, de Jong and Hendrikse. This is an open-access article distributed under the terms of the Creative Commons Attribution License (CC BY). The use, distribution or reproduction in other forums is permitted, provided the original author(s) and the copyright owner(s) are credited and that the original publication in this journal is cited, in accordance with accepted academic practice. No use, distribution or reproduction is permitted which does not comply with these terms.
*Correspondence: Carlo Lucci c.lucci@umcutrecht.nl
Abbreviations iICA, intracranial internal carotid artery; BA, basilar artery; VA, vertebral artery; ACA, anterior cerebral artery; MCA, middle cerebral artery; PCA, posterior cerebral artery; PCOM, posterior communicating artery; IVI, Intracranial Vessel wall Imaging study; PIVI, Posterior Intracranial Vessel wall Imaging study.