- 1Department of Occupational and Environmental Health, Xiangya School of Public Health, Central South University, Changsha, China
- 2Hunan Children's Research Institute (HCRI), The Affiliated Children's Hospital of Xiangya School of Medicine, Central South University (Hunan Children's Hospital), Changsha, China
- 3Hunan Institute for Drug Control, Changsha, China
Background: Exposure to several metal elements has been found to be associated with thyroid hormone homeostasis. However, evidence for combined exposure is inconclusive, especially for children.
Objective: To examine the individual and joint effects of blood metal elements on thyroid hormones in children.
Methods: A total of 12,470 children aged 0–14 were collected from January 2018 to December 2021 in Hunan Children's Hospital. The concentrations of lead (Pb), iron (Fe), calcium (Ca), copper (Cu), zinc (Zn) and magnesium (Mg) in blood were detected via atomic absorption spectrometry (AAS). The levels of thyroid stimulating hormone (TSH), triiodothyronine (TT3, FT3) and total and free thyroxine (TT4, FT4) were measured by electrochemiluminescence immunoassay (ECLIA). Generalized linear regression (GLR) model and Quantile-based g-computation (QGC) were employed to estimate the association between metal exposure and thyroid hormone homeostasis.
Results: GLR model showed that a unit increase in ln-transformed Fe was associated with increases in TT3 (β = 0.163; PFDR < 0.001), TT4 (β = 12.255; PFDR < 0.001) and FT3 (β = 0.615; PFDR < 0.001), as well as decreases in TSH (β = −0.471; PFDR = 0.005) and FT4 (β = −1.938; PFDR < 0.001). The result of QGC analysis indicated a positive relationship of the ln-transformed concentration of metal mixture with the levels of TT3 (β = 0.018; P = 0.012), TT4 (β = 2.251; P < 0.001) and FT3 (β = 0.074; P < 0.001) in children. Fe was the predominant contributor among the metal mixture with positive contributions to TT3 (weight = 0.439), TT4 (weight = 0.502) and FT3 (weight = 0.450).
Conclusions: The combined metal exposure was associated with increased levels of TT3, TT4, and FT3 in children and Fe appeared to be the major contributor. Further studies are warranted to confirm our findings and elucidate the underlying mechanisms.
1 Introduction
Thyroid gland is the largest endocrine gland in human body, responsible for synthesis, storage and secretion of thyroid hormones (THs), such as Triiodothyronine (T3) and Thyroxine (T4) (1). T3 is the metabolically active form of THs while T4 is considered a precursor or prohormone, which are both tightly regulated through feedback inhibition by thyroid stimulating hormone (TSH) (2). THs are excitatory hormones that maintain cell growth and body metabolism, as well as mediate the development and differentiation of cardiovascular system, central nervous system and reproductive system (3, 4). Disrupted thyroid homeostasis has been associated with altered neural differentiation and cognitive deficits, as well as metabolic disorders (5–7).
In recent years, there has been increasing interest in the potential influence of element on the homeostasis of THs (8–10). Due to the unique property, lead (Pb) was used to make drinking water pipes and lead-acid battery, as well as added into paint (11, 12). Children in China were widely exposed to lead through drinking water and environmental sources with blood Pb level still higher than that in developed countries (13). Experimental studies shown that Pb could disturb endocrine function of thyroid through decreasing thyroid hormone secretion and endocrine-related gene expression as well as changing the tissue structure (14, 15). Iron (Fe), magnesium (Mg), copper (Cu), zinc (Zn) and calcium (Ca) are belong to essential element. Deficiency in essential element is the most common nutritional disorder detrimental to the neural, behavior and cognitive performance development of children (16) and probably alter thyroid hormone levels in experimental animals (17–20). Epidemiological studies regarding the effect of element on thyroid hormone homeostasis focused more on adults (21–23), and the results were quite different from those of children (24). Among children, the limited investigation did not reach consistent conclusions. Bucci et al. (25) found that Zn supplementation could reduce TSH level in hypozincemic Down children. Nevertheless, another investigation by Marreiro et al. (26) reported that intervention with Zn showed no influence on the metabolism of thyroid hormones among children with Down syndrome. Blood and hair Pb levels were positively correlated with TSH level while negatively associated with free thyroxine (FT4) level in children living in a rural community of Southern Brazil (27). Conversely, a recent study conducted in China showed that blood Pb level was inversely associated with the serum TSH level among school-age children living nearby a Pb-Zn mine (28).
Alterations in THs homeostasis during infancy, childhood and puberty, even subtle changes, may lead to growth delay, neuropsychological development delay and precocious puberty (29, 30). Therefore, there is a pressing need for research to explore the relationship between metal elements and thyroid hormones, especially in children (31). Notably, human body is simultaneously exposed to multiple metals, which result in a combined health effect quite different from the effect of single element exposure. However, few studies evaluated the joint effect of metal mixture exposure on THs in children (32).
In the present research, the physical examination data of 12,470 children aged 0–14 years in Hunan Children's Hospital from 2018 to 2021 were retrospectively analyzed. The impact of single selected metal (i.e., Pb, Fe, Ca, Cu, Zn, and Mg) on THs was analyzed by generalized linear model (GLM) and restricted cubic splines (RCS). Furthermore, quantile-based g-computation (QGC) model was applied to estimate the effect of metal co-exposure on THs and identify the most important individual metal in the mixture.
2 Materials and methods
2.1 Participants
This cross-sectional study was conducted in the Department of Children Health Care, Hunan Children's Hospital in Changsha city, China. Children who received medical examinations at outpatient clinic between 1st January 2018 and 31st December 2021 were recruited in this study based on the inclusion criteria: (1) between 0 and 14 years old, (2) they and their parents were residents of Hunan Province. Children's anthropometric data (height, weight) and blood samples were collected. Medical history and other basic information were collected by interviewing parents or guardians. Children with thyroid diseases (such as hyperthyroidism and hypothyroidism) and/or treated with any thyroid interfering drugs were excluded. At last, a total of 12,470 children were enrolled in this study. The flow chart of participant selection was shown in Supplementary Figure S1. All the processes were approved by the Ethics Committee of Hunan Children's Hospital.
2.2 Collection of blood sample and determination of element concentration
About 2 mL of fasting venous blood was collected from each participant in the Department of Children Health Care by trained nurse, and then analyzed in the clinical laboratory of Hunan Children's Hospital. Before metal concentration measurement, standard curves of these selected metals were conducted by serial dilution of the stock standard solutions. Sample digestion was prepared by mixing 0.5 mL of blood sample with 4.5 mL of 0.5% Triton X-100 solution containing 1% HNO3 in a tube. Then after homogenization, the samples were detected immediately by a 7030A atomic absorption spectrometer (East-West Analytical Instruments Co., Beijing, China) for the concentrations of Fe, Ca, Cu, Zn and Mg. The content of Pb was determined by a Z-2700 atomic absorption spectrometer (Hitachi, Tokyo, Japan) through graphite furnace atomic absorption spectrometry. Coefficients of variation in both intra- and inter-assay were < 5.0%. Each sample was analyzed twice, and the average value was taken as the final value. ClinChek controls levels 1 and 2 (Recipe Chemicals, Munich, Germany) were used as quality control materials and the recovery rates were between 82% and 106%. Reference value ranges for the whole blood element concentrations in our laboratory were as follows: Fe: 363~474 μg/mL, Ca: 56~78.8 μg/mL, Cu: 0.75~2.50 μg/mL, Zn: 4.8~9.3 μg/mL, Mg: 27.1~45.5 μg/mL, Pb: 0~50 μg/L.
2.3 Estimation of thyroid hormones levels
Serum was separated from whole blood sample by centrifugation at 1,000 rpm, 10 min and stored at −20°C until analyses. Plasma concentrations of total and free T3 and T4 (TT3, FT3, TT4, and FT4) were all measured by using an automated electrochemiluminescence immunoassay (ECLIA) on the Roche Cobas e 411 analyzer as well as manufacturer-recommended reagents and calibrators (Roche Diagnostics GmbH, Mannheim, Germany). The TSH level was measured by using a 1-step sandwich method of ECLIA, according to the producer's instructions. The internal quality control serum samples were randomly measured along with the study samples to ensure the accuracy of the analytical methods. The analytical sensitivities of TSH, TT3, TT4, FT3 and FT4 were 0.27 μIU/mL, 0.3 nmol/L, 5.4 nmol/L, 0.4 pmol/L and 0.3 pmol/L, respectively.
2.4 Covariates
Potential confounding factors were adjusted in the analysis, including children's age (years), gender (girl/boy), parent smoking (yes/no), parent history of thyroid disease (yes/no), maternal education level (middle school or below/high school/college or above), urinary iodine concentration (μg/L), season (spring/summer/fall/winter) and BMI (kg/m2). Smoking was defined as smoking at least one cigarette a day for the past year. Thyroid disease included hyperthyroidism, hypothyroidism, thyroiditis, goiter and thyroid cancer. BMI was calculated as weight divided by squared height. Thyroid hormone levels fluctuated with season (33, 34), and therefore, the results of THs were classified into four groups based on the season for blood samples collection (spring: 1 March-31 May; summer: 1 June-31 August; fall: 1 September-30 November; winter: 1 December-28 or 29 February).
2.5 Statistical analysis
We calculated summary statistics to describe the basic characteristics for participants, FHs levels and the distribution of metal. For demographics, continuous variables were expressed as mean ± standard deviation (SD) or median (P25, P75), and categorical variables were presented as counts and percentages. Because of the skewness distribution of the metal concentrations, they were ln-transformed to make them normally distributed. Bivariate correlations between metallic element concentrations were explored by Spearman's rank test (continuous variables).
Generalized linear regression (GLR) model was used to calculate the regression coefficient (β) and 95% confidence intervals (CIs) for the associations of serum THs concentrations with both single metal and multiple metals. Metallic element and thyroid hormone were treated as continuous variables in the model and all the analyses were adjusted for sex, age, BMI and season. We used the Benjamini–Hochberg false discovery rate (FDR) to adjust all P values. Then, restricted cubic spline (RCS) was used to investigate the potential dose-response relationships between individual metal and thyroid hormones. The ln-transformed metal levels were treated as the independent variables and the thyroid hormone concentrations as the dependent variable. At last, QGC model was used to evaluate the association of metallic elements as a joint mixture with thyroid hormones, which yielded estimates of the effect for the increase in all the element exposures by one quantile. This analysis method, combining weighted quantile sum (WQS) regression and g-computation, relaxes the assumption that all chemical exposures are associated with the outcome in the same direction (35). Additionally, QGC model generally allows for flexible polynomial functions of the mixture exposure-response, though for simplicity we describe the implementation under linearity assumptions. The weight for each metal is calculated without bootstrapping, which represents the proportion of the contribution of a specific metal to thyroid hormone among the metals in the same direction, thus sum to 1 for positive and to −1 for negative direction, respectively. After adjusted for covariates, we investigated two different mixtures, i.e., a mixture containing 6 metallic elements and a mixture only containing 5 essential metal elements (Fe, Ca, Cu, Zn, and Mg). The overall mixture effect was interpreted as the effect on the outcome of increasing every ln-transformed exposure by one quantile (q = 4), the bootstrap variance with B was set as 500.
We performed three sensitivity analyses to test the reliability of our results. Firstly, considering that the QGC model may lead to counteracting effect among mixture (36), we also applied WQS to analyze the combined effects of metals exposure on thyroid hormones. Secondly, gender may modify the associations between metal elements and health outcomes (37, 38), we conducted stratified analyses among boys and girls, respectively, in the multiple-metal models. Similarly, we performed stratified analyzes separately for the four seasons in the multi-metal models.
GLR model was performed by using the Statistic Package for Social Sciences (SPSS), (v20.0; SPSS Inc., Chicago, IL, USA). We applied RCS, QGC and WQS analyses by R statistical software (v4.1.1; R Core Team 2021) by using R packages “rms,” “qgcomp,” and “gWQS,” respectively. In all statistical analyses, the criterion of significance was defined as P < 0.05.
3 Results
3.1 Characteristics of the study population and the distribution of metallic element
The demographic characteristics and the concentrations of THs among children were displayed in Table 1. A total of 12,470 children were recruited in this study with a mean age of 7.21 ± 3.64 years old, and the mean BMI was 15.69 ± 2.23 kg/m2. More than half of the participants were boys (61.8%) and the largest number of measurements were performed in summer (40.0%). The median levels for TSH, TT3, TT4, FT3, and FT4 were 2.57 uIU/mL, 2.30 nmol/L, 110.00 nmol/L,6.41 pmol/L and 15.93 pmol/L, respectively.
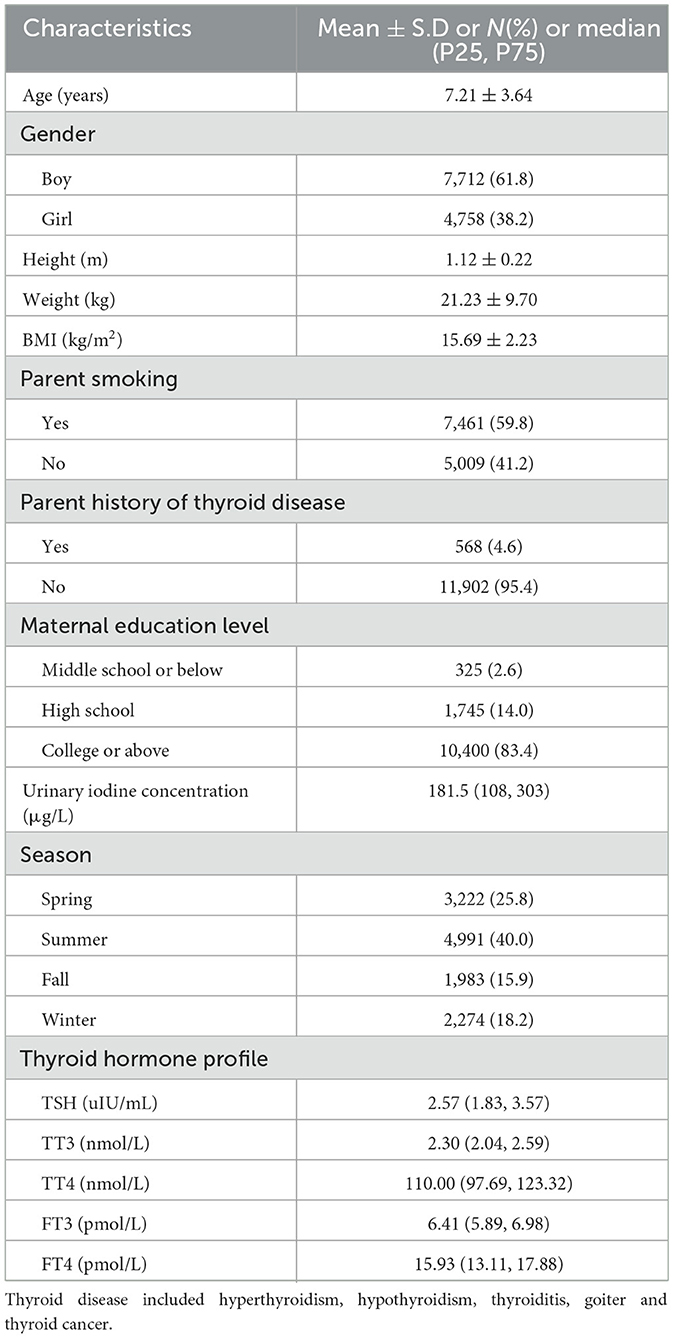
Table 1. Demographic characteristics and thyroid hormones levels of the study population (n = 12,470).
Table 2 demonstrated the distributions of metallic elements for the subjects. The median concentrations of Pb, Fe, Ca, Cu, Zn and Mg were 32.71 μg/L, 399.96 μg/mL, 63.47 μg/mL, 0.91 μg/mL, 6.39 μg/mL, and 35.94 μg/mL, respectively. Except for Cu and Fe, Cu and Ca, and Pb and Zn, other metals showed significantly weak correlations between each other (all P value < 0.05, the absolute value of r < 0.3, Supplementary Figure S2).
3.2 Associations between blood metals and serum thyroid hormones in single-metal and multiple-metal models
Table 3 showed the results of GLR model assessing the relationship between blood metals and serum thyroid hormones. In multiple-metal model, an ln-unit increase in Pb level was associated with higher TSH (β = 0.096; PFDR = 0.013) and FT4 (β = 0.227; PFDR < 0.001) after adjustment for the covariates. The concentration of Fe was positively associated with TT3 (β = 0.163; PFDR < 0.001), TT4 (β = 12.255; PFDR < 0.001) and FT3 (β = 0.615; PFDR < 0.001) while negatively associated with TSH (β = −0.471; PFDR = 0.005) and FT4 (β = −1.938; PFDR < 0.001). There was a positive association between ln-Cu level and TT4 concentration (β = 8.403; PFDR < 0.001). The concentration of ln-Mg was positively associated with TT3 (β = 0.079; PFDR = 0.044) and TT4 (β = 5.467; PFDR = 0.003). In addition, ln-Zn was negatively associated with TT3 (β = −0.083; PFDR < 0.001) and FT3 (β = −0.169; PFDR < 0.001) while positively associated with FT4 (β = 0.494; PFDR = 0.003). And all the results in multiple-metal models were basically in line with the results in single-metal models.
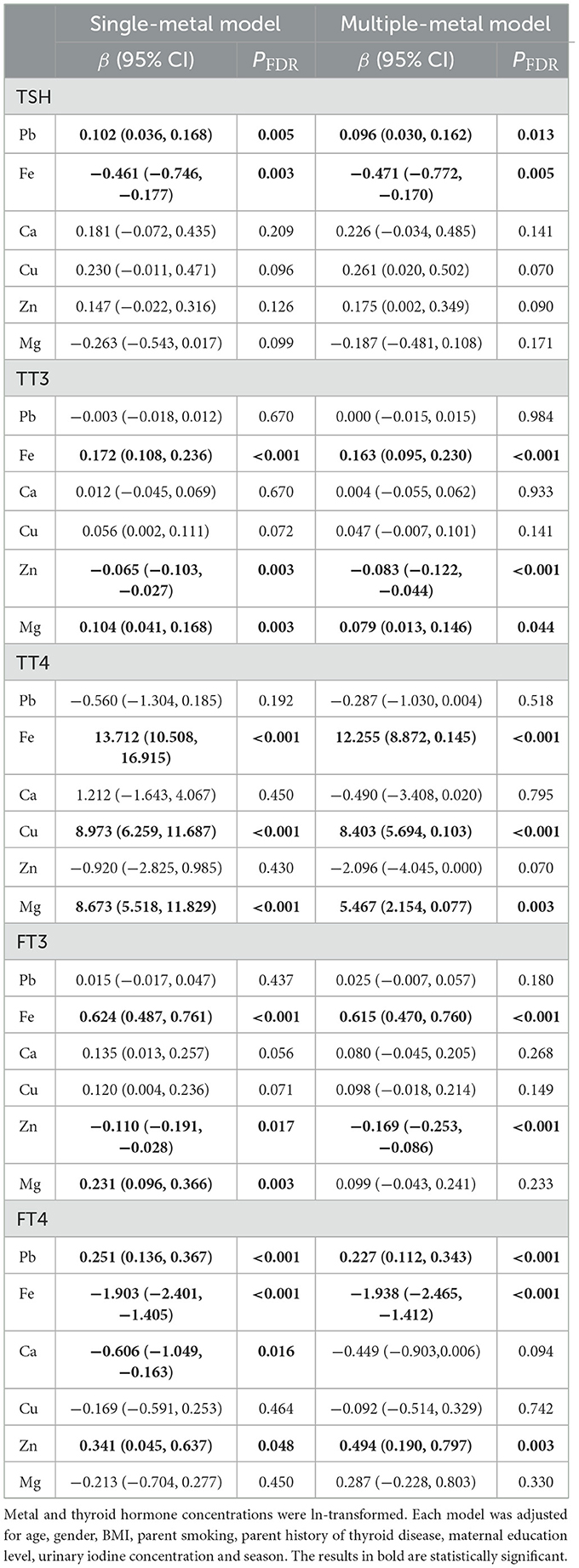
Table 3. Associations of the ln-transformed blood metal levels with serum thyroid hormones analyzed by the generalized linear regression.
The RCS models showed significant non-linear associations for Fe with TT4 and FT4, Cu with TT4 and FT4 (all P for non-linear < 0.05, Supplementary Figure S3). The association between Cu and FT4 showed an inverse U-shape (Supplementary Figure S3).
3.3 Association between metal mixture exposure and serum thyroid hormones analyzed by QGC model
Figure 1 showed the associations between combined six metals exposures and serum thyroid hormones for each higher quartile compared to the lowest quartile. After adjusting the relevant covariates, one quartile increase in selected metal mixtures generated a 0.018-SD increase in TT3 (95% CI: 0.004, 0.031), a 2.251-SD increase in TT4 (95% CI: 1.535, 2.967), and a 0.074-SD increase in FT3 (95% CI: 0.044, 0.104). In Figure 2, the estimated weights of metals for each QGC index in positive and negative direction were indicated, respectively. Fe was the most important positively weighted metal in TT3 (w = 0.439), TT4 (w = 0.502) and FT3 (w = 0.450). Zn was the most important negatively weighted metal in TT3 (w = 1.000), TT4 (w = 0.493) and FT3 (w = 1.000). Fe was both the largest weighted factor for TSH and FT4 (w = 0.709 or 0.786).
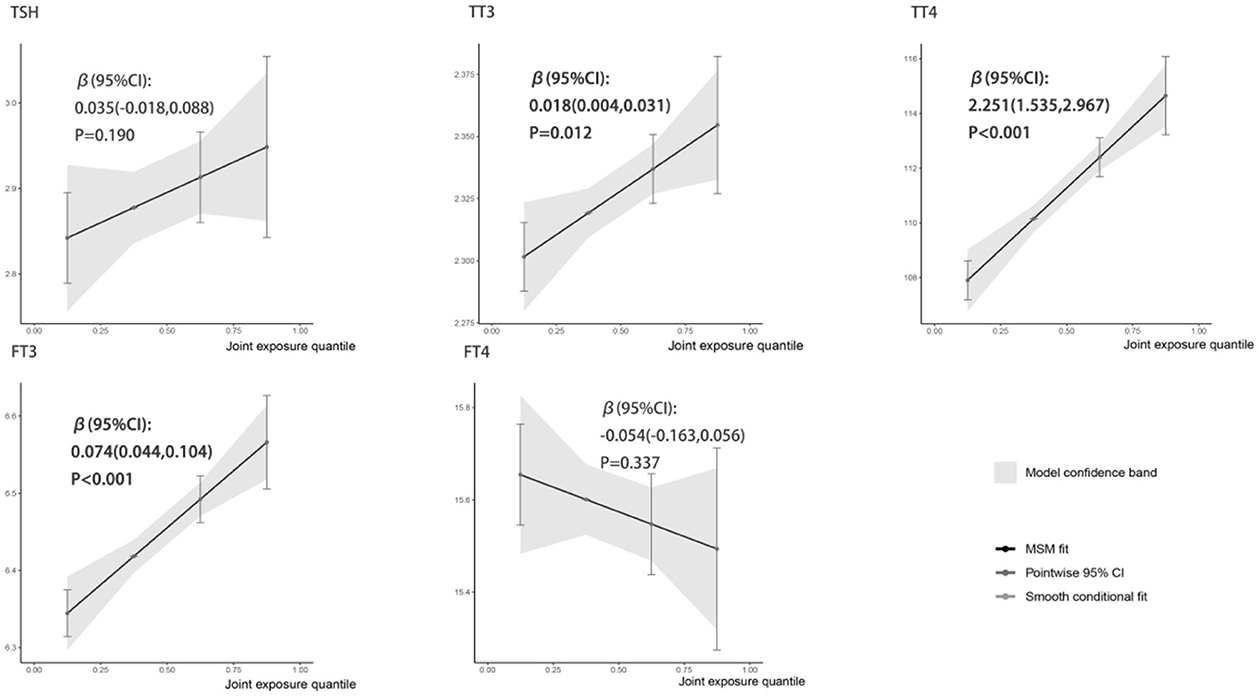
Figure 1. Linear association of the metal mixture with thyroid hormones analyzed by QGC regression. All metal levels were ln-transformed and the models were adjusted by age, gender, BMI, parent smoking, parent history of thyroid disease, maternal education level, urinary iodine concentration and season. Boot = 500: the models with 500 times bootstraps and the results were presented as β (95% CIs).
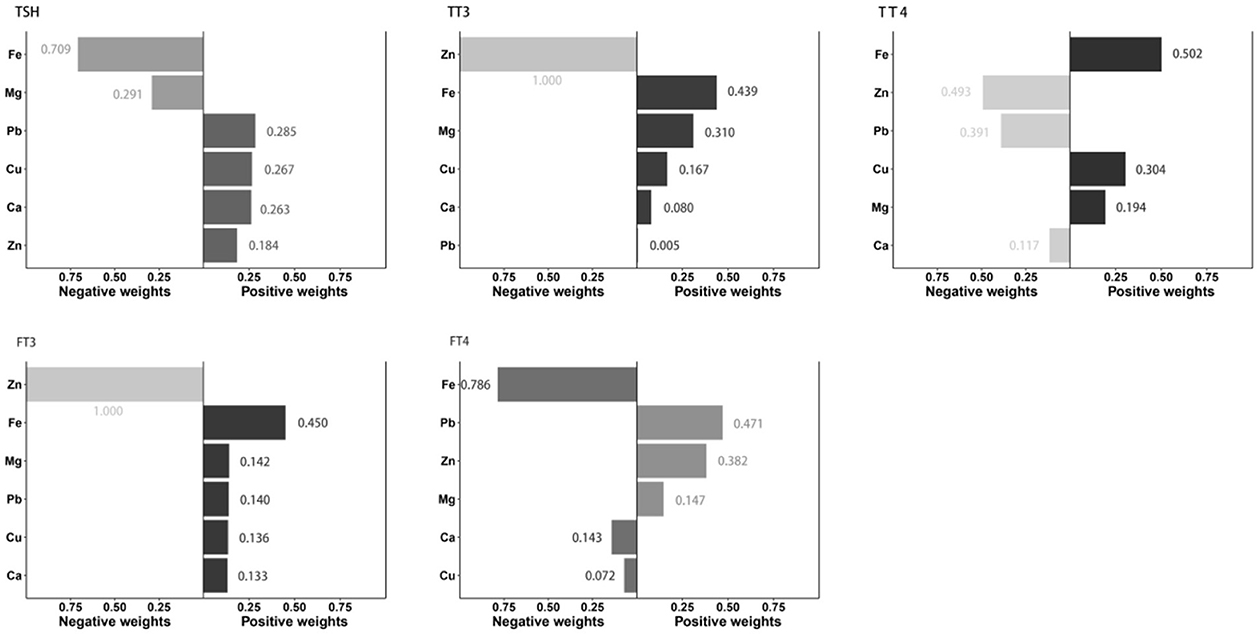
Figure 2. Estimation of individual weight for metal in the mixture associated with thyroid hormone levels. All metal levels were ln-transformed and the models were adjusted by age, gender, BMI, parent smoking, parent history of thyroid disease, maternal education level, urinary iodine concentration and season.
Supplementary Table S1 presented the association of the 5 essential metal mixture (Fe, Ca, Cu, Zn, and Mg) on thyroid hormones and the weights for each metal in QGC models. One quartile increase in selected metal mixtures generated a 0.019-SD increase in TT3 (95% CI: 0.007, 0.032), a 2.459-SD increase in TT4 (95% CI: 1.848, 3.070), a 0.058-SD increase in FT3 (95% CI: 0.032, 0.084), and a 0.158-SD decrease in FT4 (95% CI: −0.253, −0.062). Fe was the most important positively weighted metal in TT3 (w = 0.402), TT4 (w = 0.489) and FT3 (w = 0.524).
3.4 Sensitivity analysis
The results of WQS analyses indicated that concentrations of metal mixture were positively associated with serum TT3 (β = 0.014; P < 0.001), TT4 (β = 0.171; P < 0.001) and FT3 (β = 0.045; P < 0.001) with Fe contributing most to the overall mixture effect (Supplementary Figure S4), which was consistent with the results of QGC regression. Additionally, the concentration of metal mixtures has a significant impact on serum FT4 in both positive (β = 0.064; P = 0.002) and negative (β = −0.080; P < 0.001) directions. The results of subgroup analyses were similar to the main results and we found no gender-specific and season-specific association in this study (Supplementary Tables S2, S3).
4 Discussion
In this cross-sectional study, we explored both individual and joint associations of the selected metallic elements with thyroid hormone profiles in 12,470 children aged 0–14 years. We found that several selected blood metals (Pb, Fe, Cu, Zn and Mg) were associated with changes in one or more parameters of thyroid hormones in the GLR models. Furthermore, we used QGC models to estimate associations of metal mixture and thyroid hormones. Significant positive associations of the metal mixture with TT3, TT4 and FT3 were observed and Fe was identified as the most important contributors, which remained robust in WQS model and stratified by gender and season.
Fe is an essential metallic element that plays critical roles in multiple physiological processes. Our results suggested that Fe was the most influential metallic element for thyroid hormone homeostasis, being positively correlated with TT3, TT4 and FT3 while negatively correlated with TSH and FT4. Similarly, a study conducted in Iran reported that iron supplementation increased TT3 and TT4 levels in iron-deficient non-anemic children (39). A randomized controlled trial in goitrous children showed that Fe-treatment could significantly decrease thyroid size (40). Besides, results among pregnant women showed that blood Fe level was associated with significant increases in FT3 and FT4, as well as decrease in TSH (41). In an animal study, when pregnant rat dams were subjected to Fe deficient from early gestation through weaning, the serum TT3 and TT4 and whole-brain T3 decreased in pups (42). Mechanistic studies shown that Fe could mediate the synthesis of thyroid hormone and conversion of T4 to T3 through increasing the activities of thyroid peroxidase and T4-deiodinase, respectively (43).
Zn probably acts as an essential element involved in the metabolism of thyroid hormones. Research evidence showed that carboxypeptidase, a zinc-dependent enzyme, participated in the synthesis of thyrotropin-releasing hormone (TRH) (44). In children with hypozincemia, Zn sulfate supplementation could improve thyroid function, manifested as decreased TSH level and increased triiodothyronine level (25, 45). Consistently, results of the present study found a positive association between blood Zn and FT4 level. However, there were other investigation with adverse results both among adults and children (26, 46). The present research have not reached a conclusive result yet, probably attribute to the differences in physiological condition and genetic background of participants as well as statistical power and research design.
In this study, blood metals of Pb, Cu and Mg were positively associated with one or more parameters of thyroid hormones including TSH, TT3, TT4 and FT4. In a study of 54 children aged 5–16 years in Slovakia, blood and hair levels of Pb were positively associated with thyroid TSH concentration while negatively associated with FT4 level in the low exposure period (27). Besides, results from children with congenital hypothyroidism in Germany showed that serum Cu levels were associated with significant increases in T3 and T4 levels (47). However, there were also other inconsistent reports (48, 49).
Previous evidence indicated that the combined effect of a multi-metal exposure might differ from the individual effect of single metal, which was more closer to the real exposure scene of human (50). Recently, a cross-sectional investigation among 1,067 adults found no association between metal mixture (Zn, Fe, Ca, Cu, Mg, and Mn) and thyroid function biomarkers (51). Conversely, Gustin et al. reported a positive relationship of joint exposure to iodine, selenium and Zn with TSH, with Zn being the most significant contributor among participants (52). In addition, result of 2007–2012 National Health and Nutrition Examination Survey (NHANES) survey showed that the combined effect of 12 metals was sex-specifically associated with T3, T4 and T3:T4 ratio in adults (53). The present investigation indicated that the joint increases in blood metal concentrations were associated with increased levels of TT3, TT4, and FT3 among children, adding new evidence to the joint effect of metal mixture exposure on thyroid hormone homeostasis. Notably, the results of this study found both significantly positive and negative correlation between metal mixture and FT4 in the WQS regression analysis, which was presumably attributed to complex interactions between elements. Investigation with more refined design and multidisciplinary collaboration are warranted to elucidate the influence of element co-exposure on thyroid hormone level.
Our study has several strengths. Firstly, the relatively large sample size ensures enough statistical power to detect subtle difference. Secondly, to our knowledge, this is the first study to examine the association between multiple metals co-exposures and thyroid hormones in children and identified the most prominent contributor. The result is robust to the adjustment for several covariates and stratification by gender and season. However, our study also has several limitations. Due to the cross-sectional nature, the causal relationships of the blood metal mixture with thyroid hormones remain unclear. Only six metallic elements were included in the model and the potential influence of diabetes status on thyroid hormones was not estimated in this study.
5 Conclusion
This study indicated that metal mixture exposure was significantly associated with increased levels of three thyroid function biomarkers (TT3, TT4, and FT3), and Fe was the main contributors among a total of 12,470 Chinese children, which provided evidence for the development of health promotion strategy. However, further prospective cohort and mechanism studies are needed to warrant the conclusions.
Data availability statement
The original contributions presented in the study are included in the article/Supplementary material, further inquiries can be directed to the corresponding authors.
Ethics statement
The studies involving humans were approved by Ethics Committee of Hunan Children's Hospital. The studies were conducted in accordance with the local legislation and institutional requirements. Written informed consent for participation in this study was provided by the participants' legal guardians/next of kin.
Author contributions
YC: Investigation, Methodology, Writing – original draft. SX: Investigation, Writing – original draft, Funding acquisition. YD: Investigation, Methodology, Writing – original draft. MC: Investigation, Writing – original draft. RX: Investigation, Writing – original draft. QL: Supervision, Validation, Writing – original draft. JQ: Supervision, Validation, Writing – original draft. YD: Conceptualization, Writing – review & editing.
Funding
The author(s) declare financial support was received for the research, authorship, and/or publication of this article. This investigation was financially supported by the clinical research project of Hunan Children's Hospital (No. 2023CR01), the Natural Science Foundation of Hunan Province, China (No. 2023JJ30319), research project of Hunan Provincial Health Commission (No. 202112011874), and High level Talent Research Project of Hunan Provincial Health Commission.
Conflict of interest
The authors declare that the research was conducted in the absence of any commercial or financial relationships that could be construed as a potential conflict of interest.
Publisher's note
All claims expressed in this article are solely those of the authors and do not necessarily represent those of their affiliated organizations, or those of the publisher, the editors and the reviewers. Any product that may be evaluated in this article, or claim that may be made by its manufacturer, is not guaranteed or endorsed by the publisher.
Supplementary material
The Supplementary Material for this article can be found online at: https://www.frontiersin.org/articles/10.3389/fpubh.2025.1387702/full#supplementary-material
References
1. Mullur R, Liu YY, Brent GA. Thyroid hormone regulation of metabolism. Physiol Rev. (2014) 94:355–82. doi: 10.1152/physrev.00030.2013
2. Stathatos N. Thyroid physiology. Med Clin North Am. (2012) 96:165–73. doi: 10.1016/j.mcna.2012.01.007
3. Mondal S, Raja K, Schweizer U, Mugesh G. Chemistry and biology in the biosynthesis and action of thyroid hormones. Angew Chem Int Ed Engl. (2016) 55:7606–30. doi: 10.1002/anie.201601116
5. Zoeller TR, Dowling AL, Herzig CT, Iannacone EA, Gauger KJ, Bansal R. Thyroid hormone, brain development, and the environment. Environ Health Perspect. (2002) 110:355–61. doi: 10.1289/ehp.02110s3355
6. Ribeiro MO. Effects of thyroid hormone analogs on lipid metabolism and thermogenesis. Thyroid. (2008) 18:197–203. doi: 10.1089/thy.2007.0288
7. Farasat T, Cheema AM, Khan MN. Hyperinsulinemia and insulin resistance is associated with low t(3)/t(4) ratio in pre diabetic euthyroid Pakistani subjects. J Diab Complicat. (2012) 26:522–5. doi: 10.1016/j.jdiacomp.2012.05.017
8. Krieg EJ, A. meta-analysis of studies investigating the effects of occupational lead exposure on thyroid hormones. Am J Ind Med. (2016) 59:583–90. doi: 10.1002/ajim.22591
9. O'Kane SM, Mulhern MS, Pourshahidi LK, Strain JJ, Yeates AJ. Micronutrients, iodine status and concentrations of thyroid hormones: a systematic review. Nutr Rev. (2018) 76:418–31. doi: 10.1093/nutrit/nuy008
10. Boas M, Main KM, Feldt-Rasmussen U. Environmental chemicals and thyroid function: an update. Curr Opin Endocrinol Diabetes Obes. (2009) 16:385–91. doi: 10.1097/MED.0b013e3283305af7
11. Swaringen BF, Gawlik E, Kamenov GD, Mctigue NE, Cornwell DA, Bonzongo JJ. Children's exposure to environmental lead: a review of potential sources, blood levels, and methods used to reduce exposure. Environ Res. (2022) 204:112025. doi: 10.1016/j.envres.2021.112025
12. Huang J, Zeng Z, Xu X, Tian Q, Zheng K, Huo X. Blood lead levels of children exposed to e-waste: a systematic review and meta-analysis. Environ Sci Pollut Res Int. (2023) 30:64860–71. doi: 10.1007/s11356-023-27114-x
13. He K, Wang S, Zhang J. Blood lead levels of children and its trend in China. Sci Total Environ. (2009) 407:3986–93. doi: 10.1016/j.scitotenv.2009.03.018
14. Lu Y, Zhang X, Chen J, Cao J, Feng C, Yun S, et al. Sex-specific effects of fluoride and lead on thyroid endocrine function in zebrafish (Danio rerio). Chem Biol Interact. (2022) 367:110151. doi: 10.1016/j.cbi.2022.110151
15. Der R, Yousef M, Fahim Z, Fahim M. Effects of lead and cadmium on adrenal and thyroid functions in rats. Res Commun Chem Pathol Pharmacol. (1977) 17:237–53.
16. Farkhondeh T, Mansouri B, Binkowski LJ, Blaszczyk M, Pirsaheb M, Azadi NA, et al. Blood lead concentrations in children with iron deficiency anemia: a systematic review and meta-analysis. Environ Sci Pollut Res Int. (2022) 29:3199–212. doi: 10.1007/s11356-021-17301-z
17. Zhong L, Zhang H, Wu L, Ru H, Wei N, Yao F, et al. Copper and zinc treatments alter the thyroid endocrine system in zebrafish embryos/larvae. Toxics. (2022) 10:756. doi: 10.3390/toxics10120756
18. Mahoney CP, Alster FA, Carew LJ. Growth, thyroid function, and serum macromineral levels in magnesium-deficient chicks. Poult Sci. (1992) 71:1669–79. doi: 10.3382/ps.0711669
19. Chandra AK, Goswami H, Sengupta P. Effects of magnesium on cytomorphology and enzyme activities in thyroid of rats. Indian J Exp Biol. (2014) 52:787–92.
20. Beard J, Tobin B, Green W. Evidence for thyroid hormone deficiency in iron-deficient anemic rats. J Nutr. (1989) 119:772–8. doi: 10.1093/jn/119.5.772
21. Zevenbergen C, Korevaar TI, Schuette A, Peeters RP, Medici M, Visser TJ, et al. Association of antiepileptic drug usage, trace elements and thyroid hormone status. Eur J Endocrinol. (2016) 174:425–32. doi: 10.1530/EJE-15-1081
22. Kim MJ, Kim S, Choi S, Lee I, Moon MK, Choi K, et al. Association of exposure to polycyclic aromatic hydrocarbons and heavy metals with thyroid hormones in general adult population and potential mechanisms. Sci Total Environ. (2021) 762:144227. doi: 10.1016/j.scitotenv.2020.144227
23. Wada L, King JC. Effect of low zinc intakes on basal metabolic rate, thyroid hormones and protein utilization in adult men. J Nutr. (1986) 116:1045–53. doi: 10.1093/jn/116.6.1045
24. Chen A, Kim SS, Chung E, Dietrich KN. Thyroid hormones in relation to lead, mercury, and cadmium exposure in the national health and nutrition examination survey, 2007–2008. Environ Health Perspect. (2013) 121:181–6. doi: 10.1289/ehp.1205239
25. Bucci I, Napolitano G, Giuliani C, Lio S, Minnucci A, Di Giacomo F, et al. Zinc sulfate supplementation improves thyroid function in hypozincemic down children. Biol Trace Elem Res. (1999) 67:257–68. doi: 10.1007/BF02784425
26. Marreiro DN, de Sousa AF, Nogueira NN, Oliveira FE. Effect of zinc supplementation on thyroid hormone metabolism of adolescents with down syndrome. Biol Trace Elem Res. (2009) 129:20–7. doi: 10.1007/s12011-008-8280-y
27. Nascimento S, Goethel G, Gauer B, Sauer E, Nardi J, Cestonaro L, et al. Exposure to environment chemicals and its possible role in endocrine disruption of children from a rural area. Environ Res. (2018) 167:488–98. doi: 10.1016/j.envres.2018.07.039
28. Cai QL, Peng DJ. Lin-Zhao, Chen JW, Yong-Li, Luo HL, et al. Impact of lead exposure on thyroid status and IQ performance among school-age children living nearby a lead-zinc mine in China. Neurotoxicology. (2021) 82:177–85. doi: 10.1016/j.neuro.2020.10.010
29. Freire C, Ramos R, Amaya E, Fernandez MF, Santiago-Fernandez P, Lopez-Espinosa MJ, et al. Newborn TSH concentration and its association with cognitive development in healthy boys. Eur J Endocrinol. (2010) 163:901–9. doi: 10.1530/EJE-10-0495
30. Papi G, Uberti ED, Betterle C, Carani C, Pearce EN, Braverman LE, et al. Subclinical hypothyroidism. Curr Opin Endocrinol Diabetes Obes. (2007) 14:197–208. doi: 10.1097/MED.0b013e32803577e7
31. Hu MJ, Zhu JL, Zhang Q, He JL, Yang WJ, Zhu ZY, et al. Thyroid hormones in relation to polybrominated diphenyl ether and metals exposure among rural adult residents along the Yangtze river, China. Int J Hyg Environ Health. (2021) 236:113800. doi: 10.1016/j.ijheh.2021.113800
32. Weisskopf MG, Seals RM, Webster TF. Bias amplification in epidemiologic analysis of exposure to mixtures. Environ Health Perspect. (2018) 126:47003. doi: 10.1289/EHP2450
33. Mahwi TO, Abdulateef DS. Relation of different components of climate with human pituitary-thyroid axis and ft3/ft4 ratio: a study on euthyroid and SCH subjects in two different seasons. Int J Endocrinol. (2019) 2019:2762978. doi: 10.1155/2019/2762978
34. Wang D, Cheng X, Yu S, Qiu L, Lian X, Guo X, et al. Data mining: seasonal and temperature fluctuations in thyroid-stimulating hormone. Clin Biochem. (2018) 60:59–63. doi: 10.1016/j.clinbiochem.2018.08.008
35. Keil AP, Buckley JP, O'Brien KM, Ferguson KK, Zhao S, White AJ, et al. quantile-based g-computation approach to addressing the effects of exposure mixtures. Environ Health Perspect. (2020) 128:47004. doi: 10.1289/EHP5838
36. Carrico C, Gennings C, Wheeler DC, Factor-Litvak P. Characterization of weighted quantile sum regression for highly correlated data in a risk analysis setting. J Agric Biol Environ Stat. (2015) 20:100–20. doi: 10.1007/s13253-014-0180-3
37. Gochfeld M. Factors influencing susceptibility to metals. Environ Health Perspect. (1997) 105:817–22. doi: 10.1289/ehp.97105s4817
38. Ge X, Yang A, Huang S, Luo X, Hou Q, Huang L, et al. Sex-specific associations of plasma metals and metal mixtures with glucose metabolism: an occupational population-based study in China. Sci Total Environ. (2021) 760:143906. doi: 10.1016/j.scitotenv.2020.143906
39. Eftekhari MH, Simondon KB, Jalali M, Keshavarz SA, Elguero E, Eshraghian MR, et al. Effects of administration of iron, iodine and simultaneous iron-plus-iodine on the thyroid hormone profile in iron-deficient adolescent Iranian girls. Eur J Clin Nutr. (2006) 60:545–52. doi: 10.1038/sj.ejcn.1602349
40. Hess SY, Zimmermann MB, Adou P, Torresani T, Hurrell RF. Treatment of iron deficiency in goitrous children improves the efficacy of iodized salt in cote d'ivoire. Am J Clin Nutr. (2002) 75:743–8. doi: 10.1093/ajcn/75.4.743
41. Wu W, Lu J, Ruan X, Ma C, Lu W, Luo Y, et al. Maternal essential metals, thyroid hormones, and fetal growth: association and mediation analyses in Chinese pregnant women. J Trace Elem Med Biol. (2021) 68:126809. doi: 10.1016/j.jtemb.2021.126809
42. Bastian TW, Prohaska JR, Georgieff MK, Anderson GW. Perinatal iron and copper deficiencies alter neonatal rat circulating and brain thyroid hormone concentrations. Endocrinology. (2010) 151:4055–65. doi: 10.1210/en.2010-0252
43. Zimmermann MB, Kohrle J. The impact of iron and selenium deficiencies on iodine and thyroid metabolism: biochemistry and relevance to public health. Thyroid. (2002) 12:867–78. doi: 10.1089/105072502761016494
44. Severo JS, Morais J, de Freitas T, Andrade A, Feitosa MM, Fontenelle LC, et al. The role of zinc in thyroid hormones metabolism. Int J Vitam Nutr Res. (2019) 89:80–8. doi: 10.1024/0300-9831/a000262
45. Licastro F, Mocchegiani E, Zannotti M, Arena G, Masi M, Fabris N. Zinc affects the metabolism of thyroid hormones in children with down's syndrome: normalization of thyroid stimulating hormone and of reversal triiodothyronine plasmic levels by dietary zinc supplementation. Int J Neurosci. (1992) 65:259–68. doi: 10.3109/00207459209003299
46. Jain RB. Thyroid function and serum copper selenium, and zinc in general U.S. Population. Biol Trace Elem Res. (2014) 159:87–98. doi: 10.1007/s12011-014-9992-9
47. Blasig S, Kuhnen P, Schuette A, Blankenstein O, Mittag J, Schomburg L. Positive correlation of thyroid hormones and serum copper in children with congenital hypothyroidism. J Trace Elem Med Biol. (2016) 37:90–5. doi: 10.1016/j.jtemb.2016.05.007
48. Nakhaee S, Rezayee M, Mansouri B, Hadianfar A, Zadeh AA, Zardast M, et al. Comparison of thyroid function in lead-poisoned patients and healthy individuals in eastern Iran. Biol Trace Elem Res. (2022) 200:3097–102. doi: 10.1007/s12011-021-02935-4
49. Cayir A, Doneray H, Kurt N, Orbak Z, Kaya A, Turan MI, et al. Thyroid functions and trace elements in pediatric patients with exogenous obesity. Biol Trace Elem Res. (2014) 157:95–100. doi: 10.1007/s12011-013-9880-8
50. Altenburger R. Understanding combined effects for metal co-exposure in ecotoxicology. Met Ions Life Sci. (2011) 8:1–26. doi: 10.1039/9781849732116-00001
51. Ye Y, Li Y, Ma Q, Li Y, Zeng H, Luo Y, et al. Association of multiple blood metals with thyroid function in general adults: a cross-sectional study. Front Endocrinol (Lausanne). (2023) 14:1134208. doi: 10.3389/fendo.2023.1134208
52. Gustin K, Vahter M, Barman M, Jacobsson B, Skroder H, Filipsson NH, et al. Assessment of joint impact of iodine, selenium, and zinc status on women's third-trimester plasma thyroid hormone concentrations. J Nutr. (2022) 152:1737–46. doi: 10.1093/jn/nxac081
Keywords: metals, thyroid hormones, children, mixture exposure, quantile-based g-computation (QGC)
Citation: Cao Y, Xiang S, Du Y, Chen M, Xue R, Li Q, Qiu J and Duan Y (2025) Associations of combined exposure to selected metal mixtures with thyroid hormones in children: a cross-sectional study in China. Front. Public Health 13:1387702. doi: 10.3389/fpubh.2025.1387702
Received: 20 February 2024; Accepted: 06 January 2025;
Published: 22 January 2025.
Edited by:
Akira Sugawara, Tohoku University, JapanReviewed by:
Ozgur Karcioglu, University of Health Sciences, TürkiyeTan Long, Tianjin Medical University, China
Copyright © 2025 Cao, Xiang, Du, Chen, Xue, Li, Qiu and Duan. This is an open-access article distributed under the terms of the Creative Commons Attribution License (CC BY). The use, distribution or reproduction in other forums is permitted, provided the original author(s) and the copyright owner(s) are credited and that the original publication in this journal is cited, in accordance with accepted academic practice. No use, distribution or reproduction is permitted which does not comply with these terms.
*Correspondence: Jun Qiu, cWl1anVudHJldm9yQDE2My5jb20=; Yanying Duan, ZHVhbnlAY3N1LmVkdS5jbg==
†These authors have contributed equally to this work