- 1NICM Health Research Institute, Western Sydney University, Westmead, NSW, Australia
- 2School of Medical Sciences, Faculty of Medicine and Health, The University of Sydney, Camperdown, NSW, Australia
- 3Pharmacology Unit, School of Medicine, Western Sydney University, Campbelltown Campus, Sydney, NSW, Australia
Despite extensive research, determining the optimal level of sunlight exposure for human health remains a challenge, emphasizing the need for ongoing scientific inquiry into this critical aspect of human well-being. This review aims to elucidate how different components of the solar spectrum, particularly near-infrared (NIR) radiation and ultraviolet radiation (UVR) affect human health in diverse ways depending on factors such as time of day and duration of exposure. Sunlight has beneficial effects from the production of melatonin by NIR and vitamin D by UVB. Sunlight also causes harmful effects as evidenced by oxidative stress and DNA damage. Exposure to morning and evening sunlight when the UV index is below 3 is suggested to be beneficial for harnessing its positive effects while avoiding the harmful effects of UVR when the UV index is 3 or higher. Understanding the optimal timing and duration of sunlight exposure is crucial for harnessing its beneficial effects while minimizing its harmful consequences by adopting appropriate sun protection measures. By adhering to sun protection guidelines when the UV index is 3 or more and incorporating strategic exposure to NIR rays when the UV index is less than 3, individuals can optimize their health outcomes while mitigating the risks associated with sun exposure. Given that the effects of sun exposure can be both harmful and beneficial, and Australia’s unique geographical position where it experiences the highest levels of exposure to sunlight, it is vital to understand the appropriate level and timing of sun exposure to live healthy under the Australian sun.
1 Introduction
The complex dynamics of sunlight exposure concerning human health sheds light on its dual nature as a beneficial ally for autoimmune diseases, asthma, and cardiovascular diseases and its detrimental role in DNA damage, photodamage and a ubiquitous carcinogen in causing melanoma and non-melanoma (1).
Australia experiences some of the highest levels of ultraviolet radiation (UVR) radiation in the world due to its proximity to the equator (2). During summer, the Earth’s orbit also brings countries in the southern hemisphere, including Australia, closer to the sun than countries in the northern hemisphere (2). The distinctive geographical environment in Australia renders it particularly susceptible to the highest incidence rates of skin cancer as per 2022 statistics. The data reported by the International Agency for Research on Cancer in 2022 revealed that Australia’s skin cancer incidence rates of 42 in men and 31 in women per 100,000, starkly contrasts with Northern Europe’s comparatively lower rates of 17 in men and 18 in women (3). At the same time, mounting evidence shows that sunlight exposure also has beneficial effects on various diseases. These include autoimmune disorders, cardiovascular conditions, diabetes, and certain types of cancer (4–6). Additionally, there is a latitude gradient effect. This means that as we move away from the equator, the incidence of these diseases becomes more pronounced. This increase is due to reduced sunlight exposure.
Given that the effects of sun exposure can be both harmful and beneficial, and Australia’s unique geographical position, it is vital to understand the appropriate level and timing of sun exposure to live healthy under the Australian sun.
In this review, we summarize how different components of the solar spectrum, impact human health in various ways. We provide examples of how sunlight can help reduce the risk of autoimmune diseases such as multiple sclerosis (MS), diabetes, and cardiovascular conditions. We also address the harmful effects of sunlight, emphasizing the importance of understanding the optimal timing and duration of exposure based on the UV index.
2 UV index
The UV Index is a standardized measure used to express UVR intensity. The UV Index, produced by the Australian Radiation Protection and Nuclear Safety Agency (ARPANSA), is a calculated prediction of the amount of skin damaging UVR radiation that will reach a specific location (1m2) during the solar noon hour, 11:30 a.m. to 12:30 p.m. (local standard time). The World Meteorological Organization has designated one UV Index Unit as 25 mW/m2 or 90 J/m2/h. This prediction is derived from the combination of five elements: (1) latitude, (2) day of year, (3) total ozone overhead, (4) elevation above sea level, and (5) amount of cloud cover (7).
The UV index is equal to 40 times the erythemally effective power of the sun in W/m2. The UV Index at solar noon is generally in the range of 0–12 and values above 11 are considered extreme (8). In Australia, peak daily values in summer are regularly more than 12–14 and can reach 16–17 at more northern latitudes (2). When the forecast UV Index is ≥3, sun protection is required (9).
Daily sun protection times (the times when the UV Index is forecast to reach 3 or above) for locations across Australia can be accessed via the free SunSmart app (available for iPhone and Android phones/tablets), the SunSmart or Bureau of Meteorology websites and in daily newspapers. Real-time UVR levels for Australian capital cities can also be accessed via the app and at the Australian Radiation Protection and Nuclear Safety Agency (10). Analyzing the UV index for Alice Springs in Australia over the past 12 months using the ARPANSA UV index tool (Table 1), we observed a UV index generally below 3 before 8:15 am and after 5 pm. Thus, these times can be good to go outdoor to get exposure to beneficial near-infrared parts of the solar spectrum. However, it is recommended to use sun protection when the UV index is below 3 for outdoor workers who spend extended periods outdoors, those who work in alpine regions and those who work near highly reflective surfaces.
3 Solar Spectrum and its impact on health
Solar radiation reaching the Earth encompasses various wavelengths, categorized into UVR, visible, and infrared radiation (IR). UVR makes up about 6.8% of the solar spectrum, while visible light constitutes 38.9%, and IR accounts for 54.3% (11). IR consists of wavelengths longer than 760 nm up to 1 mm and is divided into three bands: IR-A (760–1,400 nm), IR-B (1400–3,000 nm), and IR-C (3,000 nm-1 mm) (12). The wavelengths 760–3,000 nm are considered NIR radiation and 3,000 to 1 mm are far infrared (FIR). The wavelengths of UVR range from 100 to 400 nm and can be subdivided into UVA (315–400 nm), UVB (280–315 nm), and UVC (100–280 nm) (13). The UVR component of terrestrial radiation from the midday sun consists of about 95% UVA and 5% UVB (14). The stratospheric ozone layer typically blocks UVC and a significant portion of UVB radiation, but their levels can be altered based on factors such as altitude and the extent of ozone depletion (15). UVB is mainly absorbed in the epidermis, and longer wavelength UVA penetrates the dermis’ inner layers (16). NIR radiation can penetrate deeply into the subcutaneous layer of skin (12). UVA and UVB-exposed skin showed significantly different features. Histological changes of the skin under UVA exposure showed an increase of dermis thickness and breakdown, as well as the disorganization of collagen fiber, which indicated the potential loss of skin integrity in the dermal layer (17). Meanwhile the UVB enhance the sunburn, carbonylation of various proteins and proliferation of keratinocytes (17). UVB is 1,000 times more potent than UVA in causing sunburn (18). Tanning response from UVA and UVB are also exerted through different mechanisms. UVA-induced tanning stems from processes like melanin oxidation or redistribution of melanosomes (organelles containing melanin) within the skin layers. Conversely, UVB-induced tanning entails an increase in melanin content through enhanced reuptake and differentiation of melanocytes (19). Chronic exposure to UVR, particularly UVB, instigates immunosuppression, largely triggered by UVB-induced DNA damage (20, 21). Different components of the solar spectrum affect human health in different ways contingent upon the wavelength, time of day and the level of exposure consequently affecting the cellular metabolism in different ways.
In natural sunlight, over 70% of the photons impinging on the body are NIR photons (22). NIR plays a crucial role in producing melatonin within cells. Melatonin has broad spectrum antioxidative effect against oxidative stress (22), DNA damage repair, and apoptosis (23). In circumstances when the UV index is below 3, sunlight exposure can be beneficial for infra-red exposure that facilitates the production of melatonin, a potent antioxidant (22). Exposure to UVB is the primary source of vitamin D synthesis (24). Vitamin D has been shown to reduce UV-induced cell death and DNA damage along with its well-known function to regulate blood calcium levels, nearly 1,000 genes are regulated by vitamin D highlights its role in maintaining good health (24, 25).
Exposure to the UVA and UVB region of the solar spectrum, when the UV index is 3 or above, it can induce significant oxidative damage in skin cells by production of ROS (26). Cellular redox balance is maintained by intrinsic antioxidant systems, including enzymes like superoxide dismutase and catalase, as well as non-enzymatic antioxidants such as glutathione and vitamins C and E (27). UVR exposure can decrease the activity of these antioxidant systems, leading to an accumulation of ROS which may contribute to inflammation and oxidative stress (28, 29). This imbalance can damage DNA, proteins, and membranes, ultimately leading to cell death and contributing to both photo carcinogenesis and photoaging (24). UVC does not reach the earth as it is absorbed by the ozone layer.
4 Beneficial effects of sunlight on the synthesis of biomolecules
Two vital biomolecules vitamin D and cellular melatonin are directly related to sunlight exposure, where cellular melatonin is produced under the influence of NIR, and vitamin D is produced under the influence of UVB.
4.1 Melatonin
Melatonin, long recognized as a key component in circadian rhythms and traditionally associated solely with the pineal gland, is now understood to be generated in substantial quantities by mitochondria within various cells on exposure to NIR (22). This subcellular melatonin, although not subject to circadian rhythm or bloodstream circulation, functions as a localized antioxidant (22).
The NIR segment of natural sunlight triggers the proliferation of melatonin within each of our healthy cells (22). This cumulative effect fortifies the body’s capacity to combat changing conditions promptly and locally throughout the day (23). Advanced three-dimensional bio-optical models illustrate how NIR sunlight profoundly affects numerous cells throughout the body, including delicate regions such as blood vessels, retina, brain, and skin by triggering melatonin production on the cellular level (22).
Nuclear factor erythroid 2 related factor 2 (Nrf2) is the master regulator of cellular antioxidant defense. The activation of Nrf2 pathways can lead to the upregulation of various antioxidant enzymes such as glutathione to combat excessive oxidative damage. Melatonin is a potential modulator of Nrf2 pathways (30) where melatonin can target Nrf2 signaling pathways and exert positive effects against oxidative stress. Melatonin improves nuclear translocation and expression of Nrf2 to diminish oxidative stress markers such as ROS by increasing the glutathione production that serves as a first line of cellular defense against oxidative damage (31).
However, modern lifestyles, characterized by prolonged exposure to visible light and minimal exposure to NIR radiation, pose a threat to this homeostasis where oxidative damage is not balanced by the body’s natural antioxidant defenses (24). Urban concrete structures do not reflect NIR rays, thus artificial lighting and living in urban concrete structures disrupt this vital process of getting enough NIR radiation (22). This also explains the health benefits of the great outdoors (32). NIR exposure is also known for its influence on gut microbiota that is related to several diseases including Parkinson’s’ and Alzheimer’s (33).
Understanding the intricate interplay between exposure to NIR and the production of melatonin that can bolster our innate antioxidant defenses can help us effectively combat oxidative damage throughout the day and opens a new avenue to combat oxidative degenerative diseases.
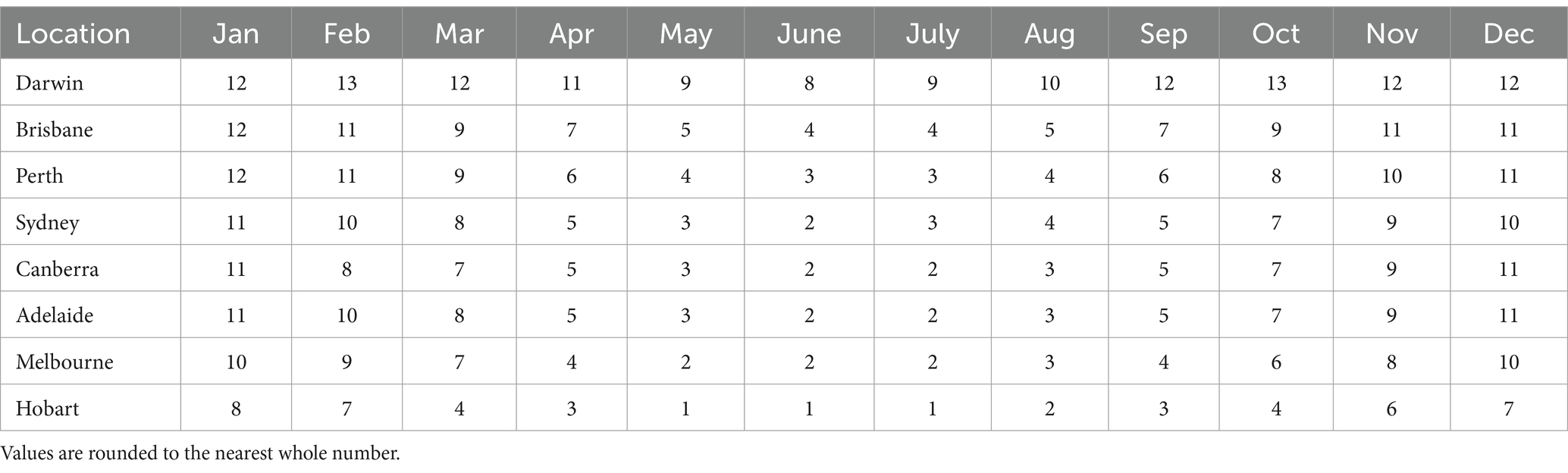
Table 1. Australian capital cities’ average daily maximum UVR levels by month (72).
4.2 Vitamin D
Exposure to UVB is the primary source of vitamin D synthesis (24). Nearly 1,000 genes governing virtually every tissue in the body are now thought to be regulated by 1,25-dihydroxy vitamin D3 (1,25[OH]2D3), the active form of vitamin D, including several involved in calcium metabolism and neuromuscular and immune system functioning (25). Vitamin D has been shown to reduce UV-induced cell death and DNA damage along with its well-known function to regulate blood calcium levels and maintain good health (24). The amount of sunlight needed for adequate vitamin D levels depends on factors such as skin phototype, UVR levels, age, and exposure duration (34).
Short frequent exposure to UVR is better as it is sufficient for vitamin D synthesis, while any further exposure degrades vitamin D as excess vitamin D is broken down into inactive metabolites (35). Furthermore, this short frequent exposure rather than long exposure also reduces erythema and the development of skin cancer (36–38) In particular, the findings indicate that reducing clothing cover and increasing the amount of skin exposure is a more effective way to increase vitamin D levels rather than increasing the time spent outdoors (39).
People with naturally darker skin may require more sun exposure to achieve adequate vitamin D levels due to reduced UVR penetration (40, 41) but all skin types need to protect against excessive UVR to prevent damage. Older people have less 7-dehydrocholesterol, which is the precursor molecule in the skin for vitamin D production, in their skin and therefore need more exposure to produce sufficient vitamin D. Recognition of this fact is crucial for the older people, who often expose only a limited area of skin and rely on this exposure for their vitamin D requirements (42).
5 Beneficial effects of sunlight on human diseases
Sunlight has beneficial effects in the context of autoimmune diseases including MS, cardiovascular diseases (CVD), diabetes, and various kinds of cancers. NIR is beneficial for melatonin production and UV light is beneficial for Vitamin D production. However, these two are not the only beneficial effects of sunlight exposure. There is a correlation between the decrease in the incidence of autoimmune diseases with sunlight exposure. This sunlight effect seems to be sunlight exposure dependent but vitamin D-independent (1).
5.1 Multiple sclerosis
Multiple sclerosis (MS) arises from repeated waves of immune cells infiltrating the central nervous system (CNS), triggering inflammation and the demyelination of neurons (43). Compelling epidemiological data link sun exposure and vitamin D levels to MS incidence. A key argument supporting the impact of UVR on MS rates is the latitude gradient – a notable correlation between MS prevalence and global latitudes (4). This trend is consistent across various autoimmune diseases, such as type 1 diabetes and potentially rheumatoid arthritis, all of which exhibit increased incidence further from the equator (1). The most pronounced correlation with latitude is observed in CNS, autoimmune diseases like MS (44). This phenomenon, termed the “latitude gradient effect,” is replicated globally (45). Remarkably, this sunlight effect seems to be UVR-dependent but vitamin D-independent, a recurring theme in the field of photo immunology (1).
5.2 Diabetes
Diabetes is a condition characterized by consistently high blood glucose levels and is divided into two main forms: type 1 and type 2. Type 1 diabetes results from damage to insulin-producing cells because of an autoimmune response, leading to a lack of insulin production. Type 2 diabetes involves insulin resistance, where insulin is produced but fails to effectively lower blood glucose levels. Type 2 diabetes is more common (46).
While low vitamin D levels are associated with both type 1 and type 2 diabetes (47), the useful effects of UVR in diabetes extend beyond vitamin D production (5). For type 1 diabetes, UVR exposure may decrease the autoimmune attack on insulin-producing cells (48). For type 2 diabetes, UV radiation exposure has been found to reduce insulin resistance independently of vitamin D levels, suggesting an additional mechanism independent of vitamin D that contributes to protective effects against diabetes (49, 50).
5.3 Cardiovascular diseases
Sunlight induced benefits to CVD which may involve UVA-induced release of NO from endothelial nitric oxide synthase from the skin, lowering blood pressure and possibly reducing the risk of stroke (6). This may contribute to seasonal variations in hypertension and overall cardiovascular health (6).
While UVR reduces hypertension, vitamin D supplements show limited evidence of a similar effect (6). This leads to the hypothesis that UVR’s beneficial impact on blood pressure may occur through pathways unrelated to vitamin D. Seasonal variations in cardiovascular disease, such as higher occurrences in later winter and early spring, correlate with latitude, but not necessarily temperature drops or increased respiratory infections (6).
5.4 Cancer
Heightened sunlight exposure or elevated vitamin levels have been associated with reduced risks of breast cancer, ovarian cancer, prostate cancer, and non-Hodgkin lymphoma, among others (51). Sunlight exposure exhibit a stronger association with reduced cancer risk than circulating vitamin D concentrations, implying that sunlight might safeguard against cancers and other maladies through pathways independent of vitamin D. Nevertheless, sunlight still exerts beneficial effects through vitamin D-dependent mechanisms as well (51).
6 Harmful effects of sunlight
Oxidative stress and DNA damage can lead to many harmful effects of UVR. Some of the evident harmful effects include sunburn, premature skin aging, immunosuppression, and skin cancer. The pathological effects of NIR, such as damage to skin collagen content via an increase in matrix metalloproteinase-1 activity, are mainly due to high dose exposure (12). Again, highlighting the importance of understanding the duration of exposure.
6.1 Sunburn
Sunburn signals overexposure to UVR (52), and is linked to melanoma risk (53). It manifests as an acute skin inflammation, erythema (redness) and warmth (54). Individual factors like skin type, site of burn, age, and previous UVR exposure influence the severity of sunburn. Environmental variables such as UVR wavelength, dose, geographical factors (Altitude, latitude, time of day), presence of UVR reflective surfaces (snow, water), and climatic conditions (wind, temperature, humidity) also play significant roles (54). Compared to fair skin types moderately pigmented skin necessitates 3–5 times more UVR exposure for sunburn, while darkly pigmented skin may require up to 30 times exposure (54), as per the Fitzpatrick skin phototype classification (55).
In regions like Australia, where fine January days are characterized by intense UVR, sunburn can develop in as little as 8 min (56) of exposure. Moreover, UVR penetrates water easily offering minimal protection against sunburn while swimming in the sea or open-air pools (57, 58).
6.2 Premature skin aging
Prolonged exposure to UVR accelerates skin aging, manifesting in increased wrinkling, fine lines, hyperpigmentation, diminished skin tone, and reduced elasticity (26). Both UVA and UVB rays generate ROS, leading to collagen degradation and connective tissue damage (26, 59). There is a compelling correlation between self-reported lifetime sun exposure and skin damage (60).
6.3 Immunosuppression
UV photons directly interact with DNA to cause direct DNA photo lesions, while indirect DNA photo lesions result from the formation of ROS (24). These molecular changes disrupt the production of crucial immune system molecules such as IL-10, IL-4, and prostaglandin E2. Consequently, systemic immune responses are modulated, fostering deficiencies in cellular immunity (61). Furthermore, chronic exposure to UVR, particularly UVB, instigates immunosuppression, largely triggered by UVB-induced DNA damage (20, 21). This immunosuppressive state amplifies the carcinogenic potential of UVR by altering immune responses (62).
6.4 Skin cancer
Australia has the highest reported incidences of non-melanocytic skin cancer (NMSC) in the world. Deaths from NMSC have been recorded since 1971 and have increased more than five-fold in the 50 years to 2021. There is no sign of a reduction in the increasing incidence of deaths from NMSC. Most deaths from NMSC are due to cutaneous squamous cell carcinoma (SCC). It is estimated that 1 in 260 cutaneous SCCs will metastasize and cause death (63).
Skin cancers are classified based on the type of skin cell from which they originate. These include basal cell carcinoma (BCC), squamous cell carcinoma (SCC), and melanoma. Melanoma, while the least common form of skin cancer, poses the greatest threat due to its potential to metastasize (64, 65) often resulting from UV-induced skin damage (66). Non-melanocytic skin cancers (NMSC), comprising BCC and SCC, are more prevalent, particularly in Australia, where the incidence of NMSC surpasses that of all other cancers combined (67). BCC the most common type of NMSC (68), is often associated with sun exposure (69). While UVR remains a primary environmental risk factor for skin cancer, individual factors and the specifics regarding the role of UV such as quantity, timing, and pattern of exposure require further investigation (70, 71).
7 Discussion
Implementing photoprotection strategies such as seeking shade, applying sunscreen with SPF 50, and wearing protective clothing, during periods of heightened UVR when UV index is 3 or higher (10), along with incorporating exposure to NIR from sunlight during the morning and evening hours when the UV index is less than 3 can offer beneficial health effects. The knowledge of safe exposure to sunlight with UV index as a guiding factor is essential for safely enjoying the Australian sun.
During the morning and evening hours, NIR is abundant as the sun angle is low at this time allowing NIR to penetrate the atmosphere easily while UVR remains limited. Moreover, going outside in the green spaces during these hours further increases the NIR exposure as green spaces reflect and amplify NIR exposure more than UVR, unlike concrete and built environments. Indoor artificial light lacks beneficial NIR, which is essential for cellular melatonin production (22). Short, frequent exposure to UVR is sufficient for vitamin D synthesis, and further exposure does not provide more vitamin D yet increases the risk of skin damage (37).
Sunlight exposure can present opportunities to prevent various kinds of cancer development, autoimmune diseases such as MS, CVD and diabetes but it also poses significant risk for skin cancer development (1). By using the UV index as a guide in determining sun exposure duration and timing, and by adhering to recommended sun protection measures, individuals can balance the sun’s benefits while minimizing its harmful effects on the skin.
Author contributions
KS: Conceptualization, Writing – original draft. KD: Writing – review & editing. GM: Writing – review & editing. DC: Writing – review & editing. XZ: Supervision, Writing – review & editing.
Funding
The author(s) declare financial support was received for the research, authorship, and/or publication of this article. Dr Zhou’s research is supported by the Research Support Program Fellowship, Western Sydney University.
Acknowledgments
We are grateful to Unilever for being the industry partner in the research.
Conflict of interest
The authors declare that the research was conducted in the absence of any commercial or financial relationships that could be construed as a potential conflict of interest.
Generative AI statement
The authors declare that no Gen AI was used in the creation of this manuscript.
Publisher’s note
All claims expressed in this article are solely those of the authors and do not necessarily represent those of their affiliated organizations, or those of the publisher, the editors and the reviewers. Any product that may be evaluated in this article, or claim that may be made by its manufacturer, is not guaranteed or endorsed by the publisher.
References
1. Byrne, SN. How much sunlight is enough? Photochem Photobiol Sci. (2014) 13:840–52. doi: 10.1039/c4pp00051j
2. Gies, P, Roy, C, Javorniczky, J, Henderson, S, Lemus-Deschamps, L, and Driscoll, C. Global solar UV index: Australian measurements, forecasts and comparison with the UK. Photochem Photobiol. (2004) 79:32–9. doi: 10.1111/j.1751-1097.2004.tb09854.x
3. Terrasse, V.World Health Organization. (2022). Global burden of cutaneous melanoma in 2020 and projections to 2040. Available at:. (https://www.iarc.who.int/wp-content/uploads/2022/03/pr311_E.pdf).
4. van der Mei, IA, Ponsonby, A-L, Dwyer, T, Blizzard, L, Simmons, R, Taylor, B, et al. Past exposure to sun, skin phenotype, and risk of multiple sclerosis: case-control study. BMJ. (2003) 327:316. doi: 10.1136/bmj.327.7410.316
5. Gorman, S, Lucas, RM, Allen-Hall, A, Fleury, N, and Feelisch, M. Ultraviolet radiation, vitamin D and the development of obesity, metabolic syndrome and type-2 diabetes. Photochem Photobiol Sci. (2017) 16:362–73. doi: 10.1039/c6pp00274a
6. Weller, RB. The health benefits of UV radiation exposure through vitamin D production or non-vitamin D pathways. Blood pressure and cardiovascular disease. Photochem Photobiol Sci. (2017) 16:374–80. doi: 10.1039/c6pp00336b
7. Kinney, JP, Long, CS, and Geller, AC. The ultraviolet index: a useful tool. Dermatol Online J. (2000) 6:2. doi: 10.5070/D35925W4HQ
8. World Health Organization. IARC monographs on the evaluation of carcinogenic risks to humans. Geneva: World Health Organization (1992).
9. Allinson, S, Asmuss, M, Baldermann, C, Bentzen, J, Buller, D, Gerber, N, et al. Validity and use of the UV index: report from the UVI working group, Schloss Hohenkammer, Germany, 5–7 December 2011. Health Phys. (2012) 103:301–6. doi: 10.1097/HP0b013e31825b581e
10. Cancer Council. (2020). UV radiation skin Cancer statistics and issues prevention policy. Available at:. (https://www.cancer.org.au/about-us/policy-and-advocacy/prevention-policy/national-cancer-prevention-policy/skin-cancer-statistics-and-issues/uv-radiation).
11. Kochevar, IE, Pathak, MA, and Parrish, JA. Photophysics, photochemistry and photobiology In: TB Fitzpatrick, AZ Eisen, K Wolff, IM Freedberg, and KF Austen, editors. Dermatology in General Medicine. New York: McGraw-Hill (1987)
12. Barolet, D, Christiaens, F, and Hamblin, MR. Infrared and skin: friend or foe. J Photochem Photobiol B Biol. (2016) 155:78–85. doi: 10.1016/j.jphotobiol.2015.12.014
13. International Organization for Standardization. ISO 21348 definitions of solar irradiance spectral categories. Environment. (2007) 5:6–7.
14. Romanhole, R, Ataide, J, Moriel, P, and Mazzola, P. Update on ultraviolet a and B radiation generated by the sun and artificial lamps and their effects on skin. Int J Cosmet Sci. (2015) 37:366–70. doi: 10.1111/ics.12219
15. International Agency for Research on Cancer. IARC monographs on the evaluation of carcinogenic risks to humans. France: International Agency for Research on Cancer (2012).
16. Finlayson, L, Barnard, IR, McMillan, L, Ibbotson, SH, Brown, CTA, Eadie, E, et al. Depth penetration of light into skin as a function of wavelength from 200 to 1000 nm. Photochem Photobiol. (2022) 98:974–81. doi: 10.1111/php.13550
17. Wang, P-W, Hung, Y-C, Lin, T-Y, Fang, J-Y, Yang, P-M, Chen, M-H, et al. Comparison of the biological impact of UVA and UVB upon the skin with functional proteomics and immunohistochemistry. Antioxidants. (2019) 8:569. doi: 10.3390/antiox8120569
18. McKinlay, AF, and Diffey, BL. A reference action spectrum for ultraviolet induced erythema in human skin In: WF Passchier and BFM Bosnjakovic, editors. Human Exposure to ultraviolet radiation: risks and regulations. Amsterdam: Elsevier Science Publishers (1987)
19. Miyamura, Y, Coelho, SG, Schlenz, K, Batzer, J, Smuda, C, Choi, W, et al. The deceptive nature of UVA tanning versus the modest protective effects of UVB tanning on human skin. Pigment Cell Melanoma Res. (2011) 24:136–47. doi: 10.1111/j.1755-148X.2010.00764.x
20. Schwarz, T. Mechanisms of UV-induced immunosuppression. Keio J Med. (2005) 54:165–71. doi: 10.2302/kjm.54.165
21. Damian, D, Matthews, Y, Phan, T, and Halliday, G. An action spectrum for ultraviolet radiation-induced immunosuppression in humans. Br J Dermatol. (2011) 164:657–9. doi: 10.1111/j.1365-2133.2010.10161.x
22. Zimmerman, S, and Reiter, RJ. Melatonin and the optics of the human body. Melatonin Res. (2019) 2:138–60. doi: 10.32794/mr11250016
23. Cezary Skobowiat, AAB, Janjetovic, Z, Jeayeng, S, Oak, ASW, Kim, T-K, Panich, U, et al. Melatonin and its derivatives counteract the ultraviolet B radiation-induced damage in human and porcine skin ex vivo. J Pineal Res. (2018) 65:12501. doi: 10.1111/jpi.12501
24. Mason, RS, and Dixon, KM. Sunlight, skin cancer and vitamin D In: M Hewison, R Bouillon, E Giovannucci, D Goltzman, M Meyer, and JE Welsh, editors. Feldman and Pike's Vitamin D. New York: Academic Press (2024). 967–93.
25. Mead, MN. Benefits of sunlight: a bright spot for human health. Environ Health Perspect. (2008) 116:A160–7. doi: 10.1289/ehp.116-a160
26. Scharffetter-Kochanek, K, Brenneisen, P, Wenk, J, Herrmann, G, Ma, W, Kuhr, L, et al. Photoaging of the skin from phenotype to mechanisms. Exp Gerontol. (2000) 35:307–16. doi: 10.1016/S0531-5565(00)00098-X
27. Valko, M, Rhodes, C, Moncol, J, Izakovic, M, and Mazur, M. Free radicals, metals and antioxidants in oxidative stress-induced cancer. Chem Biol Interact. (2006) 160:1–40. doi: 10.1016/j.cbi.2005.12.009
28. Halliday, GM, Byrne, SN, and Damian, DL. Ultraviolet A radiation: its role in immunosuppression and carcinogenesis. Semin Cutan Med Surg. (2011) 30:214–21. doi: 10.1016/j.sder.2011.08.002
29. Leccia, MT, Yaar, M, Allen, N, Gleason, M, and Gilchrest, B. Solar simulated irradiation modulates gene expression and activity of antioxidant enzymes in cultured human dermal fibroblasts. Exp Dermatol. (2001) 10:272–9. doi: 10.1034/j.1600-0625.2001.100407.x
30. Ahmadi, Z, and Ashrafizadeh, M. Melatonin as a potential modulator of Nrf2. Fundam Clin Pharmacol. (2020) 34:11–9. doi: 10.1111/fcp.12498
31. Janjetovic, Z, Jarrett, SG, Lee, EF, Duprey, C, Reiter, RJ, and Slominski, AT. Melatonin and its metabolites protect human melanocytes against UVB-induced damage: involvement of NRF2-mediated pathways. Sci Rep. (2017) 7:1274. doi: 10.1038/s41598-017-01305-2
32. Athokpam, V, Chamroy, T, and Ngairangbam, H. The role of urban green spaces in mitigating climate change: an integrative review of ecological, social, and health benefits. Environ Rep. (2024) 6:10–4. doi: 10.51470/ER.2024.6.1.10
33. Liebert, A, Bicknell, B, Johnstone, DM, Gordon, LC, Kiat, H, and Hamblin, MR. “Photobiomics”: can light, including photobiomodulation, alter the microbiome? Photobiomodul Photomed Laser Surg. (2019) 37:681–93. doi: 10.1089/photob.2019.4628
34. Nowson, CA, McGrath, JJ, Ebeling, PR, Haikerwal, A, Daly, RM, Sanders, KM, et al. Vitamin D and health in adults in Australia and New Zealand: a position statement. Med J Aust. (2012) 196:686–7. doi: 10.5694/mja11.10301
35. Gilchrest, BA. Sun exposure and vitamin D sufficiency. Am J Clin Nutr. (2008) 88:570S–7S. doi: 10.1093/ajcn/88.2.570S
36. Van Der Pols, JC, Russell, A, Bauer, U, Neale, RE, Kimlin, MG, and Green, AC. Vitamin D status and skin cancer risk independent of time outdoors: 11-year prospective study in an Australian community. J Invest Dermatol. (2013) 133:637–41. doi: 10.1038/jid.2012.346
37. Webb, AR, and Engelsen, O. Ultraviolet exposure scenarios: Balancing risks of erythema and benefits of cutaneous vitamin D synthesis. Adv Exp Med Biol. (2020) 1268:387–405. doi: 10.1007/978-3-030-46227-7_20
38. Norman, AW. Sunlight, season, skin pigmentation, vitamin D, and 25-hydroxyvitamin D: integral components of the vitamin D endocrine system. Am J Clin Nutr. (1998) 67:1108–10. doi: 10.1093/ajcn/67.6.1108
39. Kimlin, MG, Lucas, RM, Harrison, SL, Van Der Mei, I, Armstrong, BK, Whiteman, DC, et al. The contributions of solar ultraviolet radiation exposure and other determinants to serum 25-hydroxyvitamin D concentrations in Australian adults: the AusD study. Am J Epidemiol. (2014) 179:864–74. doi: 10.1093/aje/kwt446
40. Clemens, T, Henderson, S, Adams, J, and Holick, M. Increased skin pigment reduces the capacity of skin to synthesise vitamin D3. Lancet. (1982) 319:74–6. doi: 10.1016/S0140-6736(82)90214-8
41. Armas, LA, Dowell, S, Akhter, M, Duthuluru, S, Huerter, C, Hollis, BW, et al. Ultraviolet-B radiation increases serum 25-hydroxyvitamin D levels: the effect of UVB dose and skin color. J Am Acad Dermatol. (2007) 57:588–93. doi: 10.1016/j.jaad.2007.03.004
42. MacLaughlin, J, and Holick, MF. Aging decreases the capacity of human skin to produce vitamin D3. J Clin Invest. (1985) 76:1536–8. doi: 10.1172/JCI112134
43. Correale, J, Marrodan, M, and Ysrraelit, MC. Mechanisms of neurodegeneration and axonal dysfunction in progressive multiple sclerosis. Biomedicines. (2019) 7:14. doi: 10.3390/biomedicines7010014
44. McLeod, JG, Hammond, SR, and Hallpike, JF. Epidemiology of multiple sclerosis in Australia: with NSW and SA survey results. Med J Aust. (1994) 160:117–22. doi: 10.5694/j.1326-5377.1994.tb126553.x
45. Simpson, S, Blizzard, L, Otahal, P, Van der Mei, I, and Taylor, B. Latitude is significantly associated with the prevalence of multiple sclerosis: a meta-analysis. J Neurol Neurosurg Psychiatry. (2011) 82:1132–41. doi: 10.1136/jnnp.2011.240432
46. Zaccardi, F, Webb, DR, Yates, T, and Davies, MJ. Pathophysiology of type 1 and type 2 diabetes mellitus: a 90-year perspective. Postgrad Med J. (2016) 92:63–9. doi: 10.1136/postgradmedj-2015-133281
47. Hansen, C, Fleischer, J, Vistisen, D, Ridderstråle, M, Jensen, J, and Jørgensen, ME. High and low vitamin D level is associated with cardiovascular autonomic neuropathy in people with type 1 and type 2 diabetes. Diabet Med. (2017) 34:364–71. doi: 10.1111/dme.13269
48. Ponsonby, AL, Lucas, R, and van der Mei, IA. UVR, vitamin D and three autoimmune diseases—multiple sclerosis, type 1 diabetes, rheumatoid arthritis. Photochem Photobiol. (2005) 81:1267–75. doi: 10.1562/2005-02-15-IR-441
49. Mohr, S, Garland, C, Gorham, E, and Garland, F. The association between ultraviolet B irradiance, vitamin D status and incidence rates of type 1 diabetes in 51 regions worldwide. Diabetologia. (2008) 51:1391–8. doi: 10.1007/s00125-008-1061-5
50. LC, S. Use of cod liver oil during the first year of life is associated with lower risk of childhood-onset type 1 diabetes: a large, population-based, case control study. Am J Clin Nutr. (2003) 78:1128–34. doi: 10.1093/ajcn/78.6.1128
51. Lin, SW, Wheeler, DC, Park, Y, Cahoon, EK, Hollenbeck, AR, Freedman, DM, et al. Prospective study of ultraviolet radiation exposure and risk of cancer in the United States. Int J Cancer. (2012) 131:E1015–23. doi: 10.1002/ijc.27619
52. Armstrong, BK. How sun exposure causes skin cancer: an epidemiological perspective In: D Hill, JM Elwood, and DR English, editors. Prevention of skin cancer. Dordrecht: Springer (2004). 89–116.
53. Pfahlberg, A, and Kölmel, KFGefeller For The Febim Study Group, O. Timing of excessive ultraviolet radiation and melanoma: epidemiology does not support the existence of a critical period of high susceptibility to solar ultraviolet radiation-induced melanoma. Br J Dermatol. (2001) 144:471–5. doi: 10.1046/j.1365-2133.2001.04070.x
54. Hönigsmann, H. Erythema and pigmentation. Photodermatol Photoimmunol Photomed. (2002) 18:75–81. doi: 10.1034/j.1600-0781.2002.180204.x
55. Fitzpatrick, TB. The validity and practicality of sun-reactive skin types I through VI. Arch Dermatol. (1988) 124:869–71. doi: 10.1001/archderm.1988.01670060015008
56. Samanek, AJ, Croager, EJ, Gies, P, Milne, E, Prince, R, and AJ, MM. Estimates of beneficial and harmful sun exposure times during the year for major Australian population centres. Med J Aust. (2006) 184:338–41. doi: 10.5694/j.1326-5377.2006.tb00267.x
58. Lerche, C, Philipsen, P, and Wulf, H. UVR: sun, lamps, pigmentation and vitamin D. Photochem Photobiol Sci. (2017) 16:291–301. doi: 10.1039/c6pp00277c
60. Kimlin, MG, and Guo, Y. Assessing the impacts of lifetime sun exposure on skin damage and skin aging using a non-invasive method. Sci Total Environ. (2012) 425:35–41. doi: 10.1016/j.scitotenv.2012.02.080
61. Gonzales, CM, Dalmolin, LF, da Silva, KA, Slade, NBL, Lopez, RFV, Moreto, JA, et al. New insights of turmeric extract-loaded PLGA nanoparticles: development, characterization and in vitro evaluation of antioxidant activity. Plant Foods Hum Nutr. (2021) 76:507–15. doi: 10.1007/s11130-021-00929-0
62. Matsumura, Y, and Ananthaswamy, HN. Toxic effects of ultraviolet radiation on the skin. Toxicol Appl Pharmacol. (2004) 195:298–308. doi: 10.1016/j.taap.2003.08.019
63. Czarnecki, D. Mortality from nonmelanoma skin Cancer in Australia from 1971 to 2021. Cancers. (2024) 16:867. doi: 10.3390/cancers16050867
64. Marks, R. An overview of skin cancers. Cancer. (1995) 75:607–12. doi: 10.1002/1097-0142(19950115)75:2+<607::AID-CNCR2820751402>3.0.CO;2-8
65. Gilchrest, BA, and Eller, MS. DNA photodamage stimulates melanogenesis and other photoprotective responses. J Investig Dermatol Symp Proc. (1999) 4:35–40. doi: 10.1038/sj.jidsp.5640178
66. Jhappan, C, Noonan, FP, and Merlino, G. Ultraviolet radiation and cutaneous malignant melanoma. Oncogene. (2003) 22:3099–112. doi: 10.1038/sj.onc.1206450
67. Staples, MP, Elwood, M, Burton, RC, Williams, JL, Marks, R, and Giles, GG. Non-melanoma skin cancer in Australia: the 2002 national survey and trends since 1985. Med J Aust. (2006) 184:6–10. doi: 10.5694/j.1326-5377.2006.tb00086.x
68. Lomas, A, Leonardi-Bee, J, and Bath-Hextall, F. A systematic review of worldwide incidence of nonmelanoma skin cancer. Br J Dermatol. (2012) 166:1069–80. doi: 10.1111/j.1365-2133.2012.10830.x
69. Bauer, A, Diepgen, T, and Schmitt, J. Is occupational solar ultraviolet irradiation a relevant risk factor for basal cell carcinoma? A systematic review and meta-analysis of the epidemiological literature. Br J Dermatol. (2011) 165:612–25. doi: 10.1111/j.1365-2133.2011.10425.x
70. Wong, C, Strange, R, and Lear, J. Basal cell carcinoma. BMJ. (2003) 327:794–8. doi: 10.1136/bmj.327.7418.794
71. Albert, MR, and Weinstock, MA. Keratinocyte Carcinoma. CA Cancer J Clin. (2003) 53:292–302. doi: 10.3322/canjclin.53.5.292
Keywords: ultraviolet radiation, skin cancer, oxidative stress, melatonin, UV index
Citation: Sharma K, Dixon KM, Münch G, Chang D and Zhou X (2024) Ultraviolet and infrared radiation in Australia: assessing the benefits, risks, and optimal exposure guidelines. Front. Public Health. 12:1505904. doi: 10.3389/fpubh.2024.1505904
Edited by:
Rakesh C. Ramola, Hemwati Nandan Bahuguna Garhwal University, IndiaReviewed by:
Katharina Maria Rolfes, Leibniz-Institut für Umweltmedizinische Forschung (IUF), GermanyShuyu Zhang, Sichuan University, China
Copyright © 2024 Sharma, Dixon, Münch, Chang and Zhou. This is an open-access article distributed under the terms of the Creative Commons Attribution License (CC BY). The use, distribution or reproduction in other forums is permitted, provided the original author(s) and the copyright owner(s) are credited and that the original publication in this journal is cited, in accordance with accepted academic practice. No use, distribution or reproduction is permitted which does not comply with these terms.
*Correspondence: Kirti Sharma, MjIwNTkxMDBAc3R1ZGVudC53ZXN0ZXJuc3lkbmV5LmVkdS5hdQ==; Xian Zhou, cC56aG91QHdlc3Rlcm5zeWRuZXkuZWR1LmF1