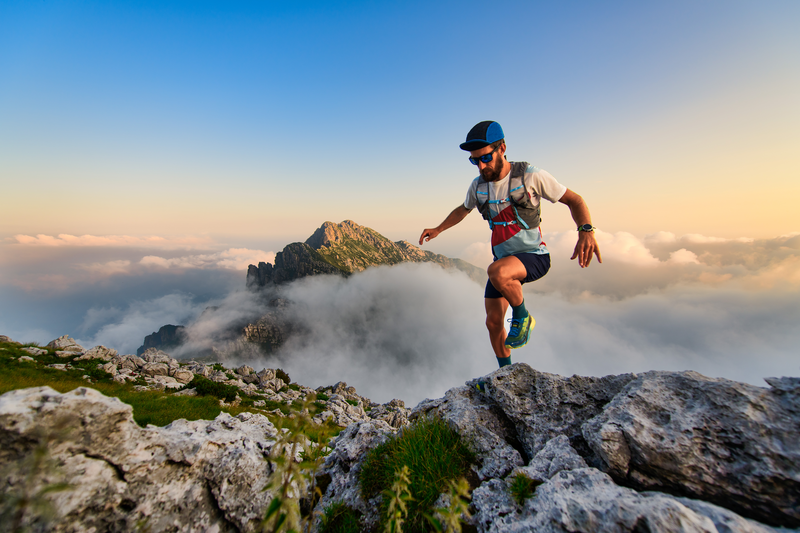
95% of researchers rate our articles as excellent or good
Learn more about the work of our research integrity team to safeguard the quality of each article we publish.
Find out more
MINI REVIEW article
Front. Public Health , 04 October 2024
Sec. Environmental Health and Exposome
Volume 12 - 2024 | https://doi.org/10.3389/fpubh.2024.1465837
This article is part of the Research Topic Toxicity Mechanisms of Environmental Pollutants and Health Risk Assessment View all 21 articles
Volcanic eruptions pose significant health risks to inhabitants of affected regions, with volcanic gases, including carbon dioxide (CO2), being a notable concern. This review examines the implications of long-term exposure to volcanic CO2 emissions on public health, highlighting the shift in understanding from acute to chronic health effects. Recent studies have underscored the need to reevaluate the adverse health impacts of CO2 beyond acute toxicity symptoms. While previous guidelines deemed an indoor (residential) acceptable long-term exposure range (ALTER) of ≤3,000 parts per million (ppm) in residential housing areas, emerging evidence suggests that even concentrations within the range of 3,000 to 1,000 ppm may induce deleterious health effects. International agencies now advocate for lower safe indoor CO2 levels (600–1,000 ppm), necessitating a reassessment of public health strategies in volcanic areas. This review argues for increased awareness among local and public health authorities about the chronic toxicity of CO2 exposure and emphasizes the importance of safeguarding populations from the adverse health effects induced by CO2 exposure.
Volcanism stands as one of the most potent geological phenomena, exemplifying the dynamic activity within the Earth’s interior and the shifts of its crust (1). During volcanic eruptions, vast quantities of pyroclastic material, ashes, and gases are expelled, often projected over considerable distances (2). Intriguingly, around 500 million people globally reside within potential exposure zones of volcanoes, which can also induce climatic changes with global ripple effects (3, 4).
Among the array of volcanic gases, carbon dioxide (CO2) is notably prevalent in volcanic and geothermal regions worldwide (5). It is emitted in substantial quantities by soils around active and post-eruptive volcanoes (6, 7). While CO2, a naturally occurring trace gas in the atmosphere, is involved in essential processes such as cellular respiration, organic matter fermentation, and the combustion of fossil fuels, its elevated concentrations can be hazardous (8). The current atmospheric concentration of CO2 is approximately 412 parts per million (ppm), or about 0.04% (9). Elevated levels of CO2 can lead to intoxication by impacting oxygen and serum bicarbonate levels (10), and it is classified as an asphyxiant by international standards (11).
The implications of CO2 on human health extend beyond asphyxiation (12); it poses significant risks to human health, manifesting both acute and chronic adverse effects (13). Considering that volcanic zones may continuously emit CO2 across vast areas over extended periods, it becomes imperative to assess the health risks for potentially exposed populations (14). This exposure is notably prevalent in occupational settings such as metallurgy, welding, and the production of carbonated beverages, where CO2 is commonly encountered (15, 16).
From a toxicological view, CO2 is absorbed passively through the lungs and is primarily excreted via the lungs after being transported in the blood as bicarbonate, a process facilitated by the enzyme carbonic anhydrase (17).
Cases of CO2 poisoning have been documented in medical literature since the 1950s (18), often resulting from accidental exposure to CO2 from sources such as dry ice, fermentation, or inadvertently opened liquid CO2 tanks (19). Additionally, cases of CO2 poisoning stemming from suicide attempts or even homicides have been reported (20). Notably, fatalities from asphyxiation due to the accumulation of CO2 from volcanic sources have also been recorded (3, 5, 21).
Populations residing in volcanic regions, whether active or dormant, face significant risks from diffuse CO2 emissions. Anomalous gas emissions are a common occurrence in areas with recent volcanic activity (7, 22, 23). Particularly vulnerable are areas where residential and tourist activities converge near emission sites, such as Colli Albani (Italy), and Stromboli and Vulcano Islands (Aeolian Archipelago, Italy) (23, 24), Rotorua city in New Zealand (25), La Palma Island in the Canary Islands (Spain) (26), and Sao Miguel Island in Azores Archipelago (Portugal) (27). Some of these areas feature sites of continuous high gas emission, with intermittent periods of increased release.
Considering these factors, the aim of this study is to assess the adverse effects experienced by populations inadvertently exposed to volcanic CO2. Given the scarcity of comprehensive data on health risks associated with volcanic CO2 exposure beyond its fatal outcomes, this critical review synthesizes both scientific and gray literature to assess the chronic and acute health risks to populations exposed to variable CO2 concentrations.
As previously discussed, CO2 is not inherently toxic, although it could also act as a toxicant. It constitutes a component of air (412 ppm), and it is generated and employed in many human processes.
The gas’s odorless and colorless nature renders detection without specific testing devices impossible, and its high density in comparison to oxygen and nitrogen results in high concentrations being condensed in the lower layers of both indoor and outdoor air, heightening its danger. CO2 may accumulate in lower spaces, leading to oxygen deficiency. Consequently, CO2 concentrations tend to be higher in environments such as mines, sugar refineries, distilleries, grain silos, and drains (28).
Although CO2 emissions from volcanic sites can indeed be fatal, such incidents are rare. However, it is crucial to remember that CO2 was implicated in the deaths of approximately 1,700 people in Cameroon, West Africa, in 1986 (as well as a similar event at Lake Monoun, also in Cameroon, in 1984) following a massive release of gas from these volcanic crater lakes (29, 30). Similarly, on February 20, 1979, in Dieng Plateau (Indonesia), 149 people perished and 1,000 others were injured after being enveloped in a gas cloud produced by a phreatic eruption (24, 31).
In addition to these major incidents, recent fatal accidents in Western countries have also been attributed to exposure to high concentrations of volcanic-origin CO2. Notably, during a period of significant degassing at La Fossa crater in the 1980s, two children and numerous small wild animals lost their lives on Vulcano Island, Italy. During this time, emissions reached up to 1,350 tons per day, with outdoor CO2 concentrations recorded as high as 9.8% (98,000 ppm) (32). Moreover, D’Alessandro and Kyriakopoulos (2013) reported three additional fatalities in the 1990s linked to CO2 accumulation in the Pausanias thermal baths on the northern coast of the Methana Peninsula (Greece) (14). In 1992, two fatalities were reported in the Azores archipelago, Portugal, inside a lava cave where diffusely released CO2 reached indoor concentrations of 10% (100,000 ppm) (21). Furthermore, in 1998, other fatality was reported in Mammoth Mountain (California, United States) when a cross-country skier fell into a snow well where CO2 concentration soared to 70% (33). Interestingly, in some of these cases, the recorded CO2 concentrations were not excessively high, suggesting that even initial exposure to low concentrations can be hazardous. This is attributed to the fact that exposure to gradually increasing CO2 concentrations, starting from atmospheric levels, can induce unconsciousness due to the narcotic properties of CO2, often without the victims perceiving any imminent danger (34, 35).
Additionally, the significant tourist activity in volcanic regions and geothermal fields globally necessitates attention to the risks faced by tourists in degassing zones. This is particularly crucial in coastal areas where tourists might be sunbathing close to the ground, a position that increases the risk of inhaling concentrated CO2 in the absence of wind. Monitoring activities that could lead to exposure to high CO2 concentrations is therefore essential for the safety of visiting tourists. Notably, cases of intoxication among tourists have been documented in volcanic regions. Documented cases of CO2 intoxication among tourists in volcanic regions underscore this risk. For instance, in April 2015, a 9-year-old French child experienced severe intoxication from gases emitted by an undersea vent near the shoreline on Vulcano Island, Italy (36). In 2019, three fatalities were reported among tourists visiting the “Solfatara,” geothermal area near Naples, Italy (37). Moreover, tourists in Sao Miguel (Azores Islands, Portugal), Hawaii (Hawaiian Islands, United States), and Mammoth Mountain (California, United States) have also suffered adverse health effects due to CO2 exposure (38–41).
The intoxications mentioned above can be attributed to the fact that CO2 poisoning typically involves life-threatening hypoxia and hypercapnia, resulting in varying levels of consciousness impairment ranging from drowsiness and confusion to deep coma and respiratory acidosis. In cases of inadvertent exposure to increasing CO2 levels, severe hypercapnia can lead to cerebral edema and respiratory center paralysis. Podlewski et al. have stated that CO2 concentrations of up to 1% (10,000 ppm) may induce drowsiness in some individuals, while concentrations above 3% (30,000 ppm) can disrupt gas exchange at the pulmonary membrane, altering pH and causing hypercapnia associated with brain damage and loss of consciousness. Breathing air with CO2 concentrations exceeding 5% (50,000 ppm) can lead to breathlessness, anxiety, and stimulation of the respiratory center. At levels between 7 and 10%, individuals may experience dizziness, headaches, visual and auditory impairments, and may rapidly become unconscious. It has been documented that CO2 concentrations of 9% inhaled for more than 10 min, and higher concentrations inhaled for less than 10 min, are poorly tolerated or not tolerated at all due to symptoms including exhaustion, anxiety, dissociation, or acidosis (pH < 7.2), despite normal oxygenation (42). Concentrations exceeding 10% are known to cause hallucinations and significantly impaired consciousness, potentially resulting in coma and convulsions. Furthermore, exposure to concentrations above 20% (200,000 ppm) typically results in death within minutes, while levels exceeding 30% (300,000 ppm) lead to instantaneous death (43). Acute effects of CO2 intoxication are shown in Table 1.
International recommendations and occupational guidelines regarding indoor CO2 levels suggest a time-weighted average (TWA) of 5,000 ppm (0.5%; for an 8-h weighted average) or a short-term TWA (15 min) of 30,000 ppm (44, 45). However, considering that elevated CO2 levels may disrupt placental development, it is essential to provide specific protection for pregnant women. Consequently, the US Navy’s toxicity experts have lowered short-term exposure limits and 24-h emergency exposure limits for submarines with female crew members to 0.8% (8,000 ppm) (46). In fact, most countries have set references values between 1,000 ppm to 1800 ppm in school, and working environments (47).
Although low levels of CO2 have long been regarded as a toxicant with no immediate lethal effects, recent studies have sparked interest in its potential health implications, particularly concerning chronic and/or intermittent long-term exposure. Such exposure has been associated with pathological conditions, including DNA alterations, nasal inflammation, and pulmonary inflammation (48). Notably, during the SARS-CoV-2 pandemic, the wearing of face masks has contributed to increased resistance and dead space volume, resulting in the re-breathing of CO2. Elevated blood CO2 levels are a key feature of the Mask-Induced Exhaustion Syndrome (49). In addition, rising indoor CO2 concentrations have been associated with symptoms characteristic of Sick Building Syndrome. These symptoms include headache, drowsiness, lethargy, tiredness, mental fatigue, reduction in decision-making performance, dizziness, as well as upper respiratory and mucosal symptoms, and skin irritation, such as itching, stinging, or dryness (50–52).
Nevertheless, given the current global trend of rising outdoor atmospheric CO2 concentrations, it is imperative to re-evaluate the effects of low-level CO2 exposure on human health. Indeed, it has been documented that CO2 can produce various hazardous health effects even at relatively low concentrations (13, 51). Long-term exposure to air polluted with CO2 from soil diffuse degassing sites has been linked to an increased risk of developing pulmonary restrictive diseases and exacerbating chronic obstructive pulmonary diseases, particularly among asthmatic and older adults (53). Residents of volcanic areas chronically exposed to high concentrations of CO2, such as Furnas in the Azores Islands, Portugal, exhibit a high incidence of chronic bronchitis and other respiratory disorders (54). Likewise, a high prevalence of non-infectious respiratory diseases has been observed among residents living in geothermal areas, such as Rotorua, New Zealand (25). Even more so, recent studies suggest that increased CO2 exposure may contribute to obesity, potentially playing a role in the rising trends of obesity and diabetes after chronic exposures to around 500 ppm CO2 (55–57).
Considering these findings, it is worth noting that while an acceptable long-term exposure range (ALTER) of ≤3,000 ppm for CO2 in residential indoor air had initially been established (58), Satish reported that even indoor concentrations ranging from 3,000 to 1,000 ppm may lead to deleterious health effects. These effects include mucous membrane or respiratory symptoms, decreased test performance, and neurophysiological symptoms (e.g., headache, fatigue) (51). Gall et al. define a level of concern for CO2 exposure, particularly regarding adverse cognitive impacts, as average personal exposure exceeding 1,000 ppm for exposures greater than 2.5 h (59). Consequently, international agencies have adopted an acceptable long-term exposure range (ALTER) of ≤1,000 ppm for CO2 in residential indoor air (54, 56).
Furthermore, although not directly related to the harmful effects of CO2, it is pertinent to note that CO2 naturally carries radioactive radon gas, a well-known carcinogen (60). Long-term inhalation exposure to radon has been linked to respiratory disorders and lung cancer (61, 62). Higher cancer incidence rates have been reported in volcanic areas compared to non-volcanic areas, with CO2 potentially acting as a carrier for radon gas (63). Populations residing in volcanic areas with chronic exposure to environmental factors resulting from volcanic activity show a higher risk of certain cancers, such as lip, oral cavity, pharynx, and breast cancers (64). Indeed, biomonitoring studies in the Azores Islands, Portugal, have revealed a higher incidence of micronucleated cells in oral epithelium (a recognized predictive biomarker of cancer risk) in individuals inhabiting volcanically active environments, suggesting an increased risk of cancer (65), thus underscoring the need for further investigation into the health effects of low levels of CO2 long-term exposure. Potential adverse health effects related to long-term CO2 exposure are summarized in Table 2.
Table 2. Potential chronic adverse health effects related to long-term (chronic/intermittent) CO2 exposure.
In light of these considerations, akin to urban populations residing in highly polluted regions, it is evident that safeguarding the inhabitants of volcanic areas from the adverse effects associated with relatively low-dose/long-term CO2 inadvertent exposure is imperative.
In volcanic regions featuring degassing sites, all the subtle/chronic effects of CO2 must be duly considered by Public Health Authorities when implementing measures aimed at reducing gas concentrations and mitigating the associated health risks. While ventilation with ambient air is a common strategy for reducing indoor CO2 levels, it may not always be suitable in volcanic degassing sites where outdoor CO2 levels are also elevated. Consequently, in such instances, evacuating volcanic/geothermal areas may be necessary, mirroring the evacuation ordered in the Port of Volcano (Volcano Island, Italy) in 2021 when outdoor CO2 levels of approximately 12.5% (125.000 ppm) were recorded (66). This proactive approach should be prioritized by Public Health Authorities in volcanic regions where sporadic and unpredictable releases of CO2 may occur, resulting in inadvertent population exposure to escalating CO2 levels (27).
Moreover, the existence of population subgroups more susceptible to the toxic effects of CO2 must also be taken into consideration. For instance, the exposure of older adults, pregnant women, cardiac or pulmonary patients, and children to CO2 warrants careful oversight (46, 49).
In this scenario, in volcanic regions it would be necessary to be able to set dangerous levels at which chronic CO2 exposure capable of producing subtle health effects is occurring. Unfortunately, measurement of bicarbonate levels or blood pH in populations chronically exposed to increasing CO2 concentrations is neither useful, nor possible, as arterial blood gasometry tests are invasive and cannot be repeated continuously for an individual tracking. Similarly, while the quantification of exhaled CO2 through capnography is valuable for assessing hypoventilation, there is no scientific evidence supporting its efficacy in diagnosing CO2 intoxication or exposure. In fact, to diagnose chronic or even acute CO2 intoxication, exposure to this gas must be confirmed in a manner akin to practices in forensic and occupational medicine (15, 19), namely, by quantifying the CO2 levels in the suspected area. In any case, in long-term exposures, it may be possible to rely on the presence of neurophysiological symptoms, (e.g., headache, or fatigue), and to perform decision-making tests, as described by Satish et al.
Implementing measures to protect the population from anthropogenic high indoor CO2 levels is feasible, given the extensive regulations and recommendations governing indoor CO2 exposure levels both internationally and occupationally. However, mitigating the effects or controlling emissions from outdoor CO2 sources poses significant challenges, being enormously complex and often hardly feasible.
Indeed, outdoor CO2 concentrations are not typically regulated, and research into the toxic effects of CO2 in open environments remains scarce. In the context of volcanic degassing sites, Public Health faces several challenges. These include the inability to control emission sources, which often produce unpredictable peaks of extremely high CO2 levels, and the absence of regulatory thresholds. This is due to limited evidence on the health effects of CO2 exposure on the general population, both short- and long-term, which complicates decision-making processes.
Further prospective studies are warranted to advance our understanding of the chronic health effects of volcanic emissions, including gases like CO2, as well as aerosols and ashes. Ongoing studies, such as the studies on the island of La Palma (Spain), could be instrumental in defining safe versus dangerous concentrations of CO2 for the population (65, 66). This would enable International Agencies and institutions to establish guidelines and recommendations for protecting health in outdoor areas affected by volcanic gas emissions. Such insights would be invaluable for Local Authorities in conducting health impact assessments and making informed planning decisions, especially concerning the placement of human settlements in volcanic zones.
In summary, although the scientific evidence on lethal incidents due to CO2 degassing in volcanic zones is generally limited, the potential for such incidents cannot be ignored, particularly in active volcanic areas where extraordinarily high concentrations of CO2 have been recorded both indoors and outdoors. This is particularly concerning in areas with significant residential and tourist activity.
Considering the “precautionary principle” in health impact assessments, which emphasizes the avoidance of unnecessary risks to population health (67). In case of population exposed to volcanic emissions, Public Health Authorities have adopted several measures to prevent or minimize individual hazards. These measures included: (a) Establishing a sensor network to continuously monitor CO2 concentrations indoors and outdoors (68); (b) Strictly controlling access to areas with the highest CO2 concentrations to mitigate the risk of accidental exposures in areas with elevated (potentially lethal) CO2 levels (66); (c) Prioritizing efforts to keep vulnerable populations (children, older adults, pregnant women, individuals with pre-existing respiratory or cardiovascular conditions) away from such areas (49); (d) Enhancing information dissemination to affected populations (including tourists) through informative meetings to foster cooperation and shared responsibility for the implemented measures (40). Thus, raising awareness and disseminating information about the “hidden” dangers of CO2 is essential.
Finally, as highlighted by Pefferkorn et al., it is crucial not to overlook the importance of adhering to safety protocols and utilizing appropriate respiratory protection near eruptive zones, even in open environments, due to the potential invisible dangers posed by volcanic areas (69).
LB: Conceptualization, Formal analysis, Investigation, Methodology, Project administration, Supervision, Validation, Visualization, Writing – original draft, Writing – review & editing. KS-R: Formal analysis, Funding acquisition, Investigation, Methodology, Supervision, Validation, Visualization, Writing – original draft, Writing – review & editing. CR-P: Writing – original draft, Writing – review & editing. MF-F: Writing – original draft, Writing – review & editing. LH-H: Writing – original draft, Writing – review & editing. EL-V: Writing – original draft, Writing – review & editing. EA-L: Writing – original draft, Writing – review & editing.
The authors declare that no financial support was received for the research and authorship. However, the publication fees related to this manuscript was financed by Pontificia Universidad Católica del Ecuador (PUCE).
The authors declare that this research was carried out in the absence of any commercial or financial relationships that could be perceived as a potential conflict of interest.
All claims expressed in this article are solely those of the authors and do not necessarily represent those of their affiliated organizations, or those of the publisher, the editors and the reviewers. Any product that may be evaluated in this article, or claim that may be made by its manufacturer, is not guaranteed or endorsed by the publisher.
CO2, carbon dioxide; ppm, parts per million; WHO, World Health Organization; ILO, International Labor Organization; OECD, Organization for Economic Co-operation and Development; OSHA, Occupational Safety and Health Administration; NIOSH, National Institute for Occupational Safety & Health; CDC, Centers for Disease Control and Prevention; ALTER, acceptable long-term exposure range; TWA, time-weighted average; EPA, Environmental Protection Agency.
1. Fabricio Neta, A d B, do Nascimento, CWA, Biondi, CM, van Straaten, P, and Bittar, SMB. Natural concentrations and reference values for trace elements in soils of a tropical volcanic archipelago. Environ Geochem Health. (2018) 40:163–73. doi: 10.1007/s10653-016-9890-5
2. Ruggieri, F, Saavedra, J, Fernandez-Turiel, JL, Gimeno, D, and Garcia-Valles, M. Environmental geochemistry of ancient volcanic ashes. J Hazard Mater. (2010) 183:353–65. doi: 10.1016/j.jhazmat.2010.07.032
3. Hansell, AL, Horwell, CJ, and Oppenheimer, C. The health hazards of volcanoes and geothermal areas. Occup Environ Med. (2006) 63:149–25. doi: 10.1136/oem.2005.022459
4. Sasidharan, S, and Dhillon, HS. Health hazards from a volcano eruption. Am J Emerg Med. (2022) 56:254–6. doi: 10.1016/j.ajem.2021.06.063
5. Hansell, A, and Oppenheimer, C. Health hazards from volcanic gases: a systematic literature review. Archives Environ Heal: Int J. (2004) 59:628–39. doi: 10.1080/00039890409602947
6. Allard, P, Carbonnelle, J, Dajlevic, D, Bronec, JL, Morel, P, Robe, MC, et al. Eruptive and diffuse emissions of CO2 from Mount Etna. Nature. (1991) 351:387–91. doi: 10.1038/351387a0
7. Chiodini, G, Cioni, R, Guidi, M, Raco, B, and Marini, L. Soil CO2 flux measurements in volcanic and geothermal areas. Appl Geochem. (1998) 13:543–52. doi: 10.1016/S0883-2927(97)00076-0
8. International volcanic health Hazard network (IVHHN). Health impacts of volcanic gases. (2020). Available from: https://www.ivhhn.org/information/health-impacts-volcanic-gases (Accessed on 2024 Feb 26)
9. Cui, Y, Schubert, BA, and Jahren, AH. A 23 m.y. record of low atmospheric CO2. Geology. (2020) 48:888–92. doi: 10.1130/G47681.1
10. Dunford, JV, Lucas, J, Vent, N, Clark, RF, and Cantrell, FL. Asphyxiation due to dry ice in a walk-in freezer. J Emerg Med. (2009) 36:353–6. doi: 10.1016/j.jemermed.2008.02.051
11. Organisation for economic co-operation and development (OECD). Guidelines for the testing of chemicals. (2023). Available from: https://www.oecd.org/chemicalsafety/testing/oecdguidelinesforthetestingofchemicals.htm (Accessed on 2024 Feb 26)
12. Permentier, K, Vercammen, S, Soetaert, S, and Schellemans, C. Carbon dioxide poisoning: a literature review of an often forgotten cause of intoxication in the emergency department. Int J Emerg Med. (2017) 10:14. doi: 10.1186/s12245-017-0142-y
13. Azuma, K, Kagi, N, Yanagi, U, and Osawa, H. Effects of low-level inhalation exposure to carbon dioxide in indoor environments: a short review on human health and psychomotor performance. Environ Int. (2018) 121:51–6. doi: 10.1016/j.envint.2018.08.059
14. D’Alessandro, W, and Kyriakopoulos, K. Preliminary gas hazard evaluation in Greece. Nat Hazards. (2013) 69:1987–2004. doi: 10.1007/s11069-013-0789-5
15. Oyama, I, Tajima, Y, Ojima, T, and Iida, A. Exposure to high concentrations of carbon dioxide during transporting a cadaver preserved with dry ice inside an ambulance vehicle. Forensic Toxicol. (2023) 41:179–82. doi: 10.1007/s11419-022-00644-8
16. Kettner, M, Ramsthaler, F, Juhnke, C, Bux, R, and Schmidt, P. A fatal case ofCO2Intoxication in a fermentation tank. J Forensic Sci. (2013) 58:556–8. doi: 10.1111/1556-4029.12058
17. Langford, NJ. Carbon dioxide poisoning. Toxicol Rev. (2005) 24:229–35. doi: 10.2165/00139709-200524040-00003
19. Scott, JL, Kraemer, DG, and Keller, RJ. Occupational hazards of carbon dioxide exposure. J Chem Health Saf. (2009) 16:18–22. doi: 10.1016/j.jchas.2008.06.003
20. Sautter, J, Gapert, R, Tsokos, M, and Oesterhelweg, L. Murder-suicide by carbon dioxide (CO2) poisoning: a family case from Berlin, Germany. Forensic Sci Med Pathol. (2014) 10:97–102. doi: 10.1007/s12024-013-9495-6
21. Viveiros, F, Gaspar, JL, Ferreira, T, and Silva, C. Hazardous indoor CO2 concentrations in volcanic environments. Environ Pollut. (2016) 214:776–86. doi: 10.1016/j.envpol.2016.04.086
22. Barberi, F, and Carapezza, ML. Helium and CO2 soil gas emission from Santorini (Greece). Bull Volcanol. (1994) 56:335–42. doi: 10.1007/BF00326460
23. Carapezza, ML, Badalamenti, B, Cavarra, L, and Scalzo, A. Gas hazard assessment in a densely inhabited area of Colli Albani volcano (cava Dei Selci, Roma). J Volcanol Geotherm Res. (2003) 123:81–94. doi: 10.1016/S0377-0273(03)00029-5
24. Madonia, P, Cangemi, M, Colajanni, M, and Winkler, A. Atmospheric concentration of CO2 and PM2.5 at Salina, Stromboli, and Vulcano Islands (Italy): how anthropogenic sources, ordinary volcanic activity and unrests affect air quality. Int J Environ Res Public Health. (2022) 19:4833. doi: 10.3390/ijerph19084833
25. Durand, M, and Wilson, JG. Spatial analysis of respiratory disease on an urbanized geothermal field. Environ Res. (2006) 101:238–45. doi: 10.1016/j.envres.2005.08.006
26. Padilla, GD, Barrancos, J, Hernández, PA, Díaz, AJÁ, Pérez, NM, Pérez, AMG, et al. Air CO2 monitoring network in the urban areas of Puerto naos and La Bombilla, La Palma, Canary Islands. Copernicus Meetings; (2023). Available from: https://meetingorganizer.copernicus.org/EGU23/EGU23-4188.html (Accessed on 2024 Mar 12)
27. Viveiros, F, Gaspar, JL, Ferreira, T, Silva, C, Marcos, M, and Hipólito, A. Chapter 14 mapping of soil CO2diffuse degassing at São Miguel Island and its public health implications. Geol Soc Lond Mem. (2015) 44:185–95. doi: 10.1144/M44.14
28. World Health Organization/International Labour Organization (WHO/ILO). International Chemical Safety Cards, Card 0021: carbon dioxide. (2018). Available from: https://www.ilo.org/dyn/icsc/showcard.display?p_lang=en&p_card_id=0021&p_version=2 (Accessed on 2024 Feb 26)
29. Baxter, PJ, Kapila, M, and Mfonfu, D. Lake Nyos disaster, Cameroon, 1986: the medical effects of large scale emission of carbon dioxide? BMJ. (1989) 298:1437–41. doi: 10.1136/bmj.298.6685.1437
30. Baxter, PJ, Tedesco, D, Miele, G, Baubron, JC, and Cliff, K. Health hazards of volcanic gases. Lancet. (1990) 336:176. doi: 10.1016/0140-6736(90)91695-7
31. International volcanic health Hazard network (IVHHN). Volcanic gases and aerosols guidelines. (2022). Available from: https://www.ivhhn.org/images/pdf/gas_guidelines.pdf (Accessed on 2024 Mar 12)
32. Carapezza, ML, Barberi, F, Ranaldi, M, Ricci, T, Tarchini, L, Barrancos, J, et al. Diffuse CO2 soil degassing and CO2 and H2S concentrations in air and related hazards at Vulcano Island (Aeolian arc, Italy). J Volcanol Geotherm Res. (2011) 207:130–44. doi: 10.1016/j.jvolgeores.2011.06.010
33. Hill, PM. Possible asphyxiation from carbon dioxide of a cross-country skier in eastern California: a deadly volcanic hazard. Wilderness Environ Med. (2000) 11:192–5. doi: 10.1580/1080-6032(2000)011[0192:PAFCDO]2.3.CO;2
35. Gill, M, Natoli, MJ, Vacchiano, C, MacLeod, DB, Ikeda, K, Qin, M, et al. Effects of elevated oxygen and carbon dioxide partial pressures on respiratory function and cognitive performance. J Appl Physiol. (2014) 117:406–12. doi: 10.1152/japplphysiol.00995.2013
36. Diliberto, IS, Cangemi, M, Gagliano, AL, Inguaggiato, S, Jacome Paz, MP, Madonia, P, et al. Volcanic gas Hazard assessment in the Baia di Levante area (Vulcano Island, Italy) inferred by geochemical investigation of passive fluid degassing. Geosciences. (2021) 11:478. doi: 10.3390/geosciences11110478
37. Carfora, A, Campobasso, CP, Cassandro, P, la Sala, F, Maiellaro, A, Perna, A, et al. Fatal inhalation of volcanic gases in three tourists of a geothermal area. Forensic Sci Int. (2019) 297:e1–7. doi: 10.1016/j.forsciint.2019.01.044
38. Sorey, ML, Evans, WC, Kennedy, BM, Farrar, CD, Hainsworth, LJ, and Hausback, B. Carbon dioxide and helium emissions from a reservoir of magmatic gas beneath Mammoth Mountain, California. J Geophys Res Solid Earth. (1998) 103:15303–23. doi: 10.1029/98JB01389
39. Baxter, PJ, Baubron, JC, and Coutinho, R. Health hazards and disaster potential of ground gas emissions at Furnas volcano, São Miguel, Azores. J Volcanol Geotherm Res. (1999) 92:95–106. doi: 10.1016/S0377-0273(99)00070-0
40. Heggie, TW. Reported fatal and non-fatal incidents involving tourists in Hawaii volcanoes National Park, 1992–2002. Travel Med Infect Dis. (2005) 3:123–31. doi: 10.1016/j.tmaid.2004.09.004
41. Heggie, TW. Geotourism and volcanoes: health hazards facing tourists at volcanic and geothermal destinations. Travel Med Infect Dis. (2009) 7:257–61. doi: 10.1016/j.tmaid.2009.06.002
42. van der Schrier, R, van Velzen, M, Roozekrans, M, Sarton, E, Olofsen, E, Niesters, M, et al. Carbon dioxide tolerability and toxicity in rat and man: a translational study. Front Toxicol. (2022) 4:1001709. doi: 10.3389/ftox.2022.1001709
43. Podlewski, R, Płotek, W, Grześkowiak, M, Małkiewicz, T, Frydrysiak, K, and Żaba, Z. Carbon dioxide as a potential danger to medical rescue teams at work–a case study. Med Pr. (2017) 68:135–8. doi: 10.13075/mp.5893.00408
44. Occupational safety and health administration (OSHA). Permissible Exposure Limits. (2019). Available from: https://www.osha.gov/annotated-pels/table-z-1 (Accessed on 2024 Mar 12)
45. Centers for Disease Control and Prevention (CDC). National Institute for Occupational Safety and Health (NIOSH) - pocket guide to chemical hazards - carbon dioxide. (2019). Available from: https://www.cdc.gov/niosh/npg/npgd0103.html (Accessed on 2024 Mar 12)
46. Howard, WR, Wong, B, Yeager, KSB, Stump, DG, Edwards, T, Arden James, R, et al. Submarine exposure guideline recommendations for carbon dioxide based on the prenatal developmental effects of exposure in rats. Birth Defects Res. (2019) 111:26–33. doi: 10.1002/bdr2.1417
47. Settimo, G, Yu, Y, Gola, M, Buffoli, M, and Capolongo, S. Challenges in IAQ for indoor spaces: a comparison of the reference guideline values of indoor air pollutants from the governments and international institutions. Atmosfera. (2023) 14:633. doi: 10.3390/atmos14040633
48. Guais, A, Brand, G, Jacquot, L, Karrer, M, Dukan, S, Grévillot, G, et al. Toxicity of carbon dioxide: a review. Chem Res Toxicol. (2011) 24:2061–70. doi: 10.1021/tx200220r
49. Kisielinski, K, Wagner, S, Hirsch, O, Klosterhalfen, B, and Prescher, A. Possible toxicity of chronic carbon dioxide exposure associated with face mask use, particularly in pregnant women, children and adolescents – a scoping review. Heliyon. (2023) 9:e14117. doi: 10.1016/j.heliyon.2023.e14117
50. Apte, MG, Fisk, WJ, and Daisey, JM. Associations between indoor CO2Concentrations and sick building syndrome symptoms in U.S. office buildings: an analysis of the 1994-1996 BASE study data. Indoor Air. (2000) 10:246–57. doi: 10.1034/j.1600-0668.2000.010004246.x
51. Satish, U, Mendell, MJ, Shekhar, K, Hotchi, T, Sullivan, D, Streufert, S, et al. Is CO2 an indoor pollutant? Direct effects of low-to-moderate CO2 concentrations on human decision-making performance. Environ Health Perspect. (2012) 120:1671–7. doi: 10.1289/ehp.1104789
52. Tsai, DH, Lin, JS, and Chan, CC. Office workers’ sick building syndrome and indoor carbon dioxide concentrations. J Occup Environ Hyg. (2012) 9:345–51. doi: 10.1080/15459624.2012.675291
53. Linhares, D, Garcia, PV, Viveiros, F, and Ferreira, T. Air pollution by hydrothermal volcanism and human pulmonary function. Biomed Res Int. (2015) 2015:e326794:1–9. doi: 10.1155/2015/326794
54. Navarro-Sempere, A, Segovia, Y, Rodrigues, AS, Garcia, PV, Camarinho, R, and García, M. First record on mercury accumulation in mice brain living in active volcanic environments: a cytochemical approach. Environ Geochem Health. (2021) 43:171–83. doi: 10.1007/s10653-020-00690-4
55. Hersoug, LG, Sjödin, A, and Astrup, A. A proposed potential role for increasing atmospheric CO2 as a promoter of weight gain and obesity. Nutr & Diabetes. (2012) 2:e31–1. doi: 10.1038/nutd.2012.2
56. Zheutlin, AR, Adar, SD, and Park, SK. Carbon dioxide emissions and change in prevalence of obesity and diabetes in the United States: an ecological study. Environ Int. (2014) 73:111–6. doi: 10.1016/j.envint.2014.07.012
57. Chen, JK, Wu, C, and Su, TC. Positive association between indoor gaseous air pollution and obesity: an observational study in 60 households. Int J Environ Res Public Health. (2021) 18:11447. doi: 10.3390/ijerph182111447
58. Health Canada. Government of Canada. (2021). Residential indoor air quality guidelines: Carbon dioxide. Available from: https://www.canada.ca/en/health-canada/services/publications/healthy-living/residential-indoor-air-quality-guidelines-carbon-dioxide.html (Accessed on 2024 Mar 12)
59. Gall, E, Cheung, T, Luhung, I, Schiavon, S, and Nazaroff, W. Real-time monitoring of personal exposures to carbon dioxide. Build Environ. (2016) 104:59–67. doi: 10.1016/j.buildenv.2016.04.021
60. Sóki, E, Gyila, S, and Csige, I. Origin and transport of radon in a dry and in a wet mofette of COVASNA, Romania. J Environ Radioact. (2022) 251:106962. doi: 10.1016/j.jenvrad.2022.106962
61. Field, RW, Steck, DJ, Smith, BJ, Brus, CP, Fisher, EL, Neuberger, JS, et al. Residential radon gas exposure and lung Cancer: the Iowa radon lung Cancer study. Am J Epidemiol. (2000) 151:1091–102. doi: 10.1093/oxfordjournals.aje.a010153
62. Field, RW, Steck, DJ, Smith, BJ, Brus, CP, Fisher, EF, Neuberger, JS, et al. The Iowa radon lung cancer study — phase I: residential radon gas exposure and lung cancer. Sci Total Environ. (2001) 272:67–72. doi: 10.1016/S0048-9697(01)00666-0
63. Putri, RGP, Ysrafil, Y, and Awisarita, W. Cancer incidence in volcanic areas: a systematic review. Asian Pac J Cancer Prev. (2022) 23:1817–26. doi: 10.31557/APJCP.2022.23.6.1817
64. Amaral, A, Rodrigues, V, Oliveira, J, Pinto, C, Carneiro, V, Sanbento, R, et al. Chronic exposure to volcanic environments and cancer incidence in the Azores, Portugal. Sci Total Environ. (2006) 367:123–8. doi: 10.1016/j.scitotenv.2006.01.024
65. Rodrigues, AS, Arruda, MSC, and Garcia, PV. Evidence of DNA damage in humans inhabiting a volcanically active environment: a useful tool for biomonitoring. Environ Int. (2012) 49:51–6. doi: 10.1016/j.envint.2012.08.008
66. Inguaggiato, S, Vita, F, Diliberto, IS, Inguaggiato, C, Mazot, A, Cangemi, M, et al. The volcanic activity changes occurred in the 2021–2022 at Vulcano island (Italy), inferred by the abrupt variations of soil CO2 output. Sci Rep. (2022) 12:21166. doi: 10.1038/s41598-022-25435-4
67. Environmental Protection Agency (EPA). Emisiones de dióxido de carbono. (2021). Available from: https://espanol.epa.gov/la-energia-y-el-medioambiente/emisiones-de-dioxido-de-carbono (Accessed on 2024 Mar 13)
68. Viveiros, F, Silva, C, Pacheco, J, Moreno, L, Ferreira, T, and Gaspar, JL. “A permanent indoor CO2 monitoring system installed in a degassing area of the Azores archipelago.” In: International conference on urban risks (ICUR2016). Lisboa; (2016). 907–914.
Keywords: volcanic CO2 emissions, health risks, chronic exposure, public health, mitigation strategies
Citation: Boada LD, Simbaña-Rivera K, Rodríguez-Pérez C, Fuentes-Ferrer M, Henríquez-Hernández LA, López-Villarrubia E and Alvarez-León EE (2024) Assessing the hidden dangers of volcanic CO2 exposure: a critical review of health impacts. Front. Public Health. 12:1465837. doi: 10.3389/fpubh.2024.1465837
Received: 16 July 2024; Accepted: 23 September 2024;
Published: 04 October 2024.
Edited by:
Pu Xia, University of Birmingham, United KingdomReviewed by:
Gaetano Settimo, Istituto Superiore di Sanità, ItalyCopyright © 2024 Boada, Simbaña-Rivera, Rodríguez-Pérez, Fuentes-Ferrer, Henríquez-Hernández, López-Villarrubia and Alvarez-León. This is an open-access article distributed under the terms of the Creative Commons Attribution License (CC BY). The use, distribution or reproduction in other forums is permitted, provided the original author(s) and the copyright owner(s) are credited and that the original publication in this journal is cited, in accordance with accepted academic practice. No use, distribution or reproduction is permitted which does not comply with these terms.
*Correspondence: Katherine Simbaña-Rivera, a2F0aGVyaW5lLnNpbWJhbmExMDFAYWx1LnVscGdjLmVz
Disclaimer: All claims expressed in this article are solely those of the authors and do not necessarily represent those of their affiliated organizations, or those of the publisher, the editors and the reviewers. Any product that may be evaluated in this article or claim that may be made by its manufacturer is not guaranteed or endorsed by the publisher.
Research integrity at Frontiers
Learn more about the work of our research integrity team to safeguard the quality of each article we publish.