- Institut de Radioprotection et de Sûreté Nucléaire (IRSN), PSE-SANTE/SESANE/LEPID, Fontenay-aux-Roses, France
Context and objective: To date, lung cancer is the only well-established health effect associated with radon exposure in humans. To summarize available evidence on other potential health effects of radon exposure, we performed a comprehensive qualitative and quantitative synthesis of the available literature on radon exposure and health effects other than lung cancer, in both occupational and general populations.
Method: Eligible studies published from January 1990 to March 2023, in English and French languages, were identified in PubMed, ScienceDirect, Scopus, ScieLo and HAL. In the meta-analysis, we estimated average weighted standardized incidence ratios (metaSIR), standardized mortality ratios (metaSMR), and risk ratio (metaRR) per 100 unit (Bq/m3 or Working level Month) increase in radon exposure concentration by combining estimates from the eligible studies using the random-effect inverse variance method. DerSimonian & Laird estimator was used to estimate the between-study variance. For each health outcome, analyses were performed separately for mine workers, children, and adults in the general population.
Results: A total of 129 studies were included in the systematic review and 40 distinct studies in the meta-analysis. For most of these health outcomes, the results of the meta-analyses showed no statistically significant association, and heterogeneity was only present among occupational studies, especially between those included in the metaSIR or metaSMR analyses. However, the estimated exposure-risk associations were positive and close to the statistical significance threshold for: lymphohematological cancer incidence in children (metaRR = 1.01; 95%CI: 1.00–1.03; p = 0.08); malignant melanoma mortality among adults in the general population (metaRR = 1.10; 95%CI: 0.99–1.21; p = 0.07); liver cancer mortality among mine workers (metaRR = 1.04; 95%CI: 1.00–1.10; p = 0.06); intestine and rectal cancer mortality combined among mine workers (metaRR = 1.02; 95%CI: 1.00–1.04; p = 0.06).
Conclusion: Although none of the exposure-risk associations estimated in the meta-analyses reached statistical significance, the hypothesis that radon may have other health effects apart from lung cancer could not be ruled-out and call for additional research. Larger and well-designed studies are needed to further investigate this question.
Systematic review registration:
1 Introduction
Radon is a natural radioactive noble gas originating from the decay series of uranium-238 present in rocks and soils. It is the most important source of natural background radiation (1). Epidemiological studies conducted in miners and in the general population have provided consistent evidence of the carcinogenic effect of radon on the lung (2–4). A recent systematic review and meta-analysis that included 24 single studies estimated a statistically significant 11% increase in the risk of lung cancer per 100 Becquerel/cubic meter [Bq/m3] increase in residential radon concentration, overall, and a 15% increased risk among lifelong never-smokers (5). A recent study conducted in the frame of the pooled uranium miners analysis (PUMA) consortium, composed of seven underground uranium miners cohorts from North America and Europe, estimated a 22% increase in lung cancer mortality risk per 100 working level months (WLM) (6). Since a few decades, a growing number of studies have investigated other potential health effects associated with radon exposure, but, individually, they did not allow for straightforward interpretations (7–9). Previously, several systematic reviews and meta-analyses were conducted on one or few diseases, and results were inconclusive (10–14). Most of these reviews and meta-analyses focused on only one type of radon exposure, occupational or residential, and were restricted to the child or adult population. To overcome these limitations, we carried out a comprehensive and up-to date systematic review and meta-analysis, covering both occupational and residential radon exposure, populations of children and adults, and incidence and mortality data for a wide range of malignant and non-malignant diseases, except lung cancer. This work was performed in the frame of the European project RadoNorm,1 which aims to manage risks from radon and Naturally-Occurring Radioactive Materials exposure situations to ensure effective radiation protection based on improved scientific evidence and social considerations.
2 Materials and methods
This work was carried out and reported in accordance with the Preferred Reporting Items for Systematic reviews and Meta-Analyses (PRISMA) (15, 16), and has been registered in the PROSPERO databases2 under the identification number CRD42023474542.
2.1 Information sources and search strategy
A comprehensive literature search was performed in March 2023 in five databases: PubMed, ScienceDirect, Scopus, ScieLo and HAL. The following bibliographic query, developed in collaboration with a professional librarian, was used: (“Radon exposure”) OR (“Exposure to radon”) OR (“Exposure of radon”) OR (“Exposure to Rn”) OR (“Exposure of Rn”) OR (“Exposed to radon”) OR (“Residential radon”) OR (“Radon concentration”) OR (“Working level month”) OR (“WLM”). We did not specify disease names on purpose, to enable identification of published articles on all possible malignant and non-malignant diseases in association with radon exposure. We applied restrictions to the language (English and French) and the period of publication (from 1990 to the time of the search in March 2023). Finally, we uploaded the identified references into a platform called RAYYAN,3 which is a cloud-based software application designed for researchers conducting systematic literature reviews and meta-analyses.
2.2 Eligibility criteria
Studies were included if all of the following criteria were fulfilled: (1) they focused on occupational exposure to radon such as in miners or on residential (air or water) exposure to radon in the general population, in children and/or adults; (2) the control group, except in case–control studies, was composed of persons with lower (ideally, minimal) levels of exposure to radon (internal control group) or representing a given reference population (for instance, miners inside a country were often compared with the national population from the same country); (3) the outcome of interest was morbidity (incidence/prevalence) or mortality due to any malignant and non-malignant disease excluding lung cancer; (4) the study design was a single or a pooled original cohort, case–control, case-cohort, cross-sectional, or ecological study.
Studies were excluded when: (1) there was lack of data specific to radon exposure history; (2) there was no ability to disentangle radon exposure from exposure to other sources of ionizing radiations; (3) the outcome of interest was overall cancer, i.e., including lung cancer; (4) the design was case-report, systematic review, and meta-analysis of original studies; (5) only simulated data were analyzed.
If several studies focused on the same population (with total or partial overlap), we only retained the study with the longest follow-up period or the largest sample size. Also, pooled studies were preferred to single studies.
2.3 Studies’ selection and data extraction process
After removal of duplicates, two authors (A.H. and E.C.) independently screened titles and if needed abstracts with regards to the eligibility criteria. Obviously irrelevant records were excluded. Disagreements between the two authors were resolved throughout discussions, and if necessary, the opinion of the third author (O.L.) was obtained. Full texts of the remaining potentially eligible studies were retrieved and carefully examined by one author (A.H.) for final inclusion or exclusion, and the other authors (E.C. and O.L.) were consulted in case of uncertainty. The reasons of exclusion at this stage were reported in Figure 1 and in Supplementary Table S1.
One author (A.H.) extracted relevant information from the included articles onto a spread sheet, including publication data (first author, year of publication, and location of investigation), follow-up period, study design, sample size, number of cases, and if relevant, number of controls, any other study population characteristics, health outcome(s) studied, characteristics of radon exposure assessment, variables included in statistical analyses to control for potential confounding, and main results.
If a study reported both incidence and mortality data, both were extracted. Results from multivariate/fully adjusted models were preferred for extraction to results from crude or more sparsely adjusted models. The overall study population results were preferred for extraction to the stratified ones (e.g., results per population subgroups). When several outcomes were studied and/or several effect measures were used within a same study, we extracted each of the results with a careful attention to avoid duplication or overlap with any other study.
2.4 Quality assessment of the included studies
One author (A.H.) assessed the quality of the included studies by using on the one hand, the New-castle Ottawa Scale (NOS) for cohort, case–control, and cross-sectional studies.4 On the other hand, an evaluation tool proposed by the United Nations Scientific Committee on the Effects of Atomic Radiation (UNSCEAR) in its 2017 report was also used (17). This UNSCEAR tool addresses methodological issues specific to radiation epidemiology studies, and applies to all the included studies, including the ecological ones which are not considered by the NOS scale. To control for potential subjectivity biases, a training and validation session was set during which two authors (A.H. and E.C.) independently assessed the quality of four randomly selected studies with different designs, using the two quality assessment tools. The overall and subdimension quality scores/tiers were compared, and any discordance was discussed to derive consolidated decision rules. The overall NOS score ranges from 0 to 9 for cohort and case–control studies, and from 0 to 10 for cross-sectional studies. Studies that obtained an overall NOS score of at least 6 (or at least 7 for cross-sectional studies) were considered to be of “high” quality. Those with an overall NOS score of 4–5 (or 5–6 for cross-sectional studies) were considered to be of “moderate” quality, otherwise, they were considered to be of “low” quality. The UNSCEAR quality assessment tool is composed of eight domains for which a study is appraised and judged to be of “very low, low, moderate or high” quality. To determine the overall quality tier of a study, we assigned a sub-score from 1 to 4 to each domain according to its quality tier. The sub-scores were then averaged, and the overall quality tier of the study was judged to be “very low, low, moderate, high” when the average score was “≤1.5, >1.5–2.5, >2.5–3.5, ≥3.5,” respectively.
2.5 Meta-analysis
2.5.1 Additional eligibility criteria specific to the meta-analysis step
Further criteria were defined for including studies in the meta-analysis. Studies that did not provide quantitative estimate of the effect were excluded. We also excluded ecological studies given their limitation to transpose their results at individual level. Studies that considered only drinking water as radon exposure source were excluded. The meta-analyses were limited to studies in which radon exposure estimates were treated in the regression model as a continuous variable since we did not plan to perform so-called “dose–response meta-analysis” combining results from categorical analyses to derive estimates for continuous exposure variables (18, 19) given the related uncertainties and the very large number of analyses to be done. No exclusion was made based on studies’ quality, since all the eligible studies showed at least moderate quality based on the NOS and UNSCEAR quality assessment tools (see Supplementary Tables S2, S3).
The measures of effect of interest were Standardized Incidence Ratio (SIR), and Standardized Mortality Ratio (SMR), Incidence Rate Ratio or Relative Risk (IRR), Excess Relative Risk (ERR), Odds Ratio (OR), and Hazard Ratio (HR). Whenever needed, exposure-risk relationships estimates were converted to Risk Ratio (RR) (10) and pooled together in this way, assuming they yield similar risk estimate under appropriate conditions (for instance, for ORs, rare health outcomes and true RR less than 2) (20, 21).
An additional exclusion criterion was applied to studies that reported SIRs and SMRs estimates: we excluded results/studies for which the value of SIR or SMR was null because the logarithm reached infinity, and therefore could not be properly handled in the analyses.
2.5.2 Data extraction and management for the meta-analysis
Estimates and their 95% confidence interval (CI) and/or p-value were retrieved from the eligible articles.
Regarding SIRs and SMRs, the 95%CIs were not provided in some cases. We then estimated them using Vanderbroucke method (22) with where a is the number of observed cases, λ the number of expected cases, Z the value of a unit-normal test statistic corresponding to α, the probability of a type 1 error (here, α = 0.05, meaning Z = 1.96 for a two-tailed test). For mine workers studies, risk estimates were all expressed for 100 WLM increase in radon concentration. For residential exposure studies, risk estimates for 100 Bq/m3 increase in radon concentration were preferred to those for 10 or 1,000 Bq/m3, and where necessary we computed the corresponding risk estimates for 100 Bq/m3 increase.
In three studies (23–25), either IRR or OR per 1,000 Bq/m3-years were reported. We then computed the corresponding RR per 100 Bq/m3 using the following equation . In one study (26) a HR per 10 Bq/m3 was reported, we then computed the corresponding RR per 100 Bq/m3 using the following equation .
As much as possible, risk estimates from non-linear risk models were preferred to linear risk models because risk estimates that have been derived from linear risk models are more challenging to combined in a meta-analytic way due to difficulty of existing statistical methods to reasonably quantify study-specific variances. Richardson et al. (27) recently proposed an alternative approach to address this challenge, but it requires to know the maximum concentration recorded in each included study, which is not systematically reported. When only ERR was provided, the RR was computed based on the equation (28). This was also applied to 95%CI bounds. In some cases, the lower bound (l) of the 95%CI was not available, but the upper bound (u) was provided (29–32). We then estimated the lower bound using the equation , assuming the 95%CI was symmetric. When only the point estimate of the ERR and its associated p-value were given (29–35), the bounds of the 95%CI were computed based on the Wald statistic where α = 0.05, , and , the value of that corresponds to the associated p-value of the point estimate.
2.5.3 Health outcomes definition
In most studies, diseases or group of diseases were defined using the international classification of diseases (ICD). A careful attention was given to the ICD codes reported in the articles to ensure that only studies using a similar definition for a given disease were pooled together. When a study did not focus on a given disease, but rather on one of its subtypes, we included it as such. In addition, where appropriate, we aggregated different diseases to form relatively broad and homogeneous disease groups.
2.5.4 Statistical analysis
We investigated heterogeneity across studies using Cochrane’s Q test and the I-square index. A Q test with p-value of less than 0.1 was considered as “detecting heterogeneity,” and an I-square value about 25, 50% or 75% represented low, moderate or high heterogeneity, respectively (36).
We estimated average weighted SIR (metaSIR), average weighted SMR (metaSMR), and average weighted RR (metaRR) by combining at least two estimates from studies using the random-effect inverse variance method regardless of the heterogeneity tests results. DerSimonian & Laird estimator was used to estimate the between-study variance τ2 (37). For each health outcome, analyses were performed separately for mine workers exposed to radon, expressed in WLM, for children and for adults in the general population exposed to radon, expressed in annual average concentration in Bq/m3. Further, incidence and mortality data were analyzed separately.
We examined small-study effects and publication bias using Egger’s regression test (38) and Begg’s funnel plot (39). The existence of a publication bias was suspected if the p-value for Egger’s regression test was less than 0.05 and/or if Begg’s funnel plot showed an asymmetric shape.
In sensitivity analyses, for all metaRR close to the statistical significance threshold and whenever possible (i.e., with at least three studies), we investigated whether the result was driven by specific studies or estimates using the leave-one-out method (40). We also repeated all the average weighted effects estimation (metaSIR, metaSMR, and metaRR) using the fixed-effects inverse variance method.
All the statistical models were fitted using the meta and metafor packages in R, version 4.2.2.
3 Results
3.1 Systematic review
3.1.1 Literature search and selection results
In total 10,366 bibliographic references were identified from the electronic databases, and 129 were included in the review. Details about the selection process are shown in Figure 1.
3.1.2 Characteristics of the included studies
Regarding the exposure type, 43 studies focused on occupational radon exposure (9, 26, 29–35, 41–74) and 86 on residential radon exposure. Of these last ones, 24 were restricted to children (7, 23–25, 75–94) and 55 to adults in the general population (8, 95–148). The remaining seven studies included both children and adults from the general population (149–155), with results presented separately for children and adults in six of them. Regarding the design, studies on occupational radon exposure were predominantly cohorts (86%, n = 37), while those on residential radon exposure in adults were predominantly ecological studies (60%, n = 37); and finally, in children, most of the studies were ecological (50%, n = 15), followed by case–controls studies (30%, n = 9). In terms of geographical repartition, the studies were carried out worldwide, mainly in North America, Europe, and Asia. In terms of health outcomes, except for studies in children among which incidence data were mostly used, mortality data outweighed incidence data across studies including mine workers and adults in the general population.
Detailed information about the included studies, their repartition by design, exposure and population types, and their main findings are reported in Figure 2 and Supplementary Tables S2, S3. A qualitative summary of the studies’ results is provided in Supplementary Tables S4–S7, showing for each health outcome, the number of studies reporting no, negative, or positive statistically significant association. Overall, there was an apparently good agreement between findings for occupational and residential radon exposure, and, subsequently, between children and adults in the general population, with respect to the health outcomes that were studied in common in these different settings, especially regarding lymphohematological cancers which were the most common health outcomes. In most cases, results of individual studies pointed toward a lack of statistically significant association.
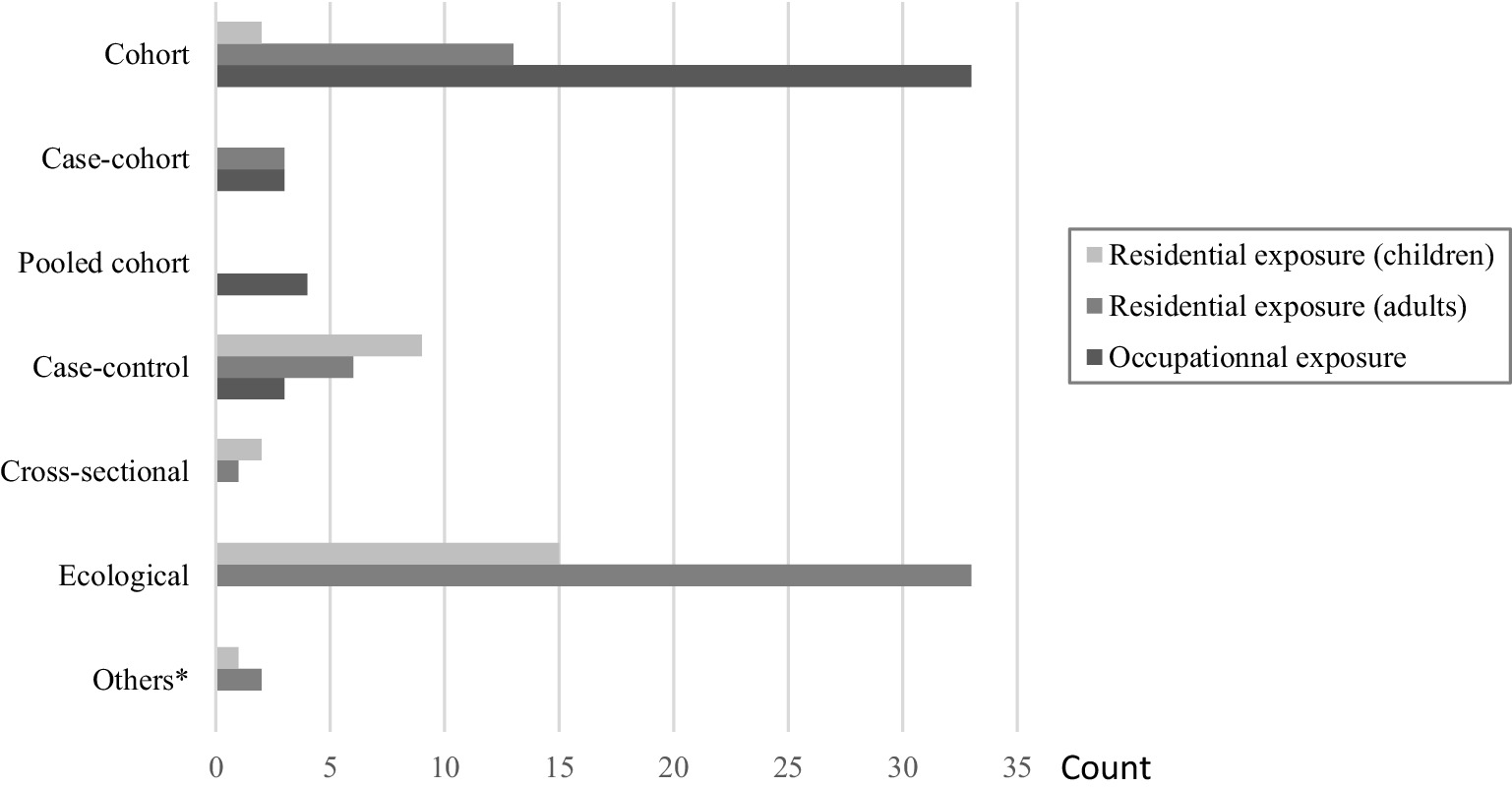
Figure 2. Repartition of studies included in the systematic review and meta-analysis by exposure type and design. *“Two-design in one” studies: ecological study & case-control study; Ecological study & case-only study; Ecological study & cohort study.
3.2 Meta-analyses
In total, 40 distinct studies were included in the meta-analysis, and the number of single estimates of SIR/SMR and RR included in the analyses for each health outcome studied was up to 34 and 7, respectively (Tables 1, 2). The quality score of studies ranged from moderate to high for both NOS and UNSCEAR quality assessment tools. Results of the meta-analysis are reported in Table 1 for SIRs and SMRs, and Table 2 for exposure-risk relationships.
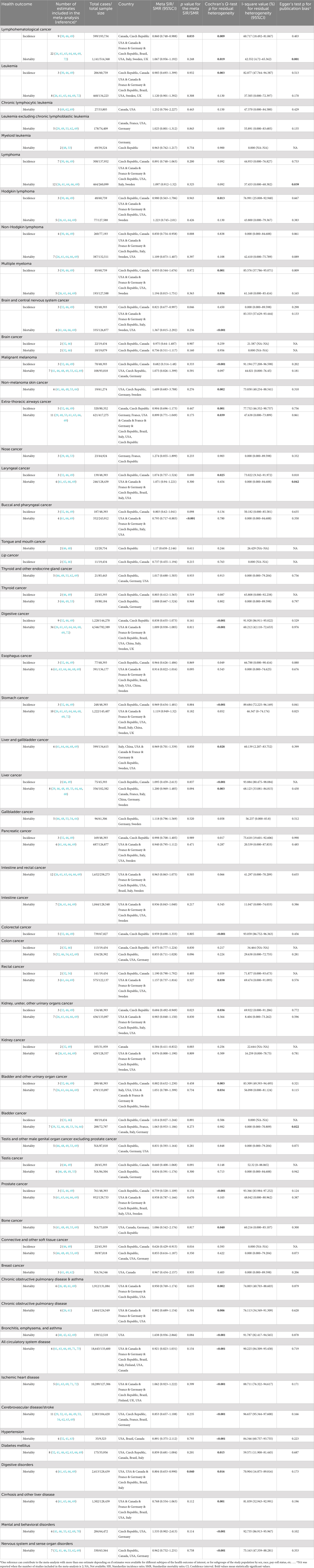
Table 1. Results of the meta-analyses for SIRs and SMRs of malignant and non-malignant health outcomes, except lung cancer, among mine workers using the random effect of DerSimonian & Laird (DL).
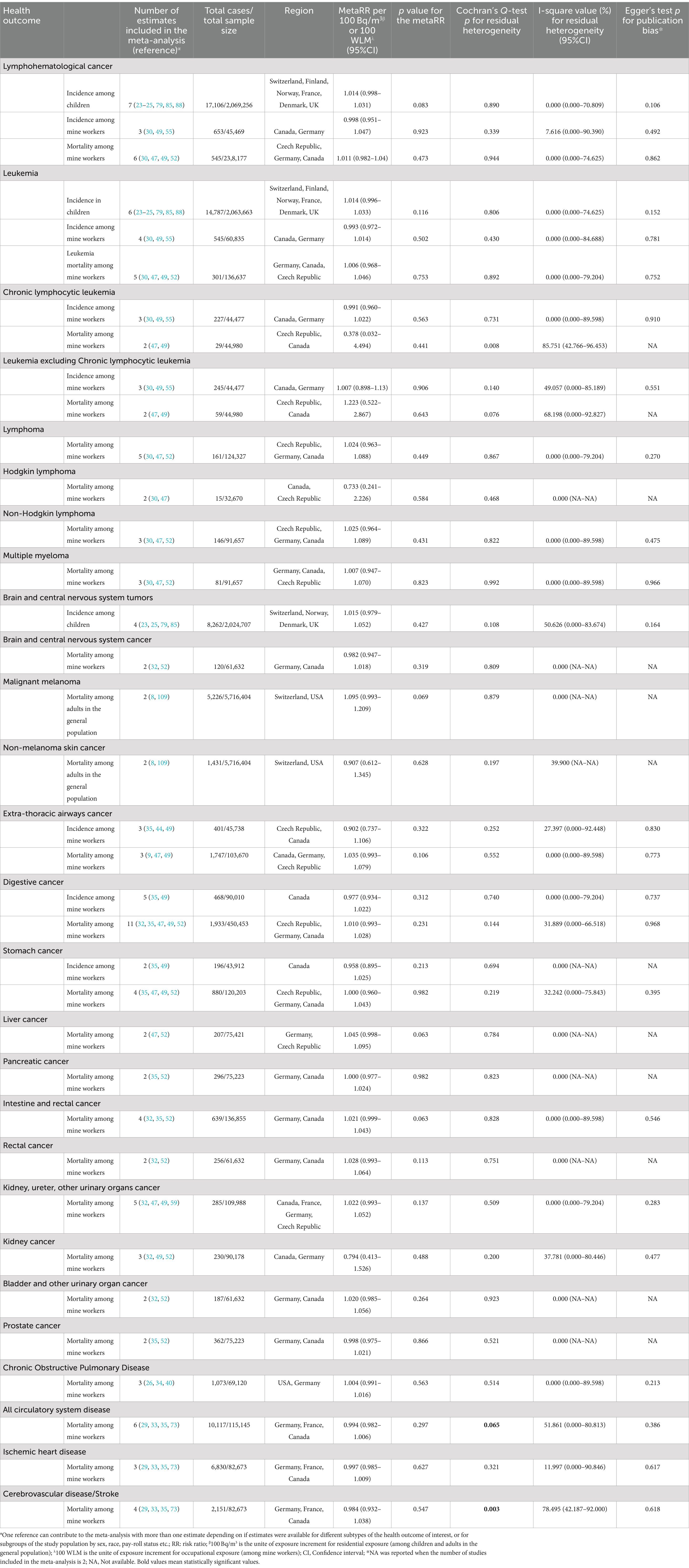
Table 2. Results of meta-analyses on exposure-risk relationships between radon exposure and malignant and non-malignant health outcomes, except lung cancer, among children, adults in the general population and mine workers, using random effect of DL.
3.2.1 Estimates for incidence and mortality rates compared with an external group (reference population)
Only studies on occupational exposure to radon among mine workers were considered.
3.2.1.1 Malignant health outcomes
Analyses were performed on incidence and/or mortality data for several cancer locations, including thyroid, other endocrine gland, brain, and central nervous system (CNS), breast, bone and connective tissue, lip, and different types of extra-thoracic airways, skin, digestive, genitourinary organs, and lymphohematological cancers. The metaSIRs indicate a statistically significant lower incidence rate than expected for lymphohematological cancer (especially for non-Hodgkin lymphoma), brain and CNS, kidney (only or combined with ureter and other urinary organs), and connective and soft tissue cancers. Analyses based on mortality data indicate a statistically significant lower mortality rate than expected only for buccal cavity and pharyngeal cancer [metaSMR = 0.80 (95%CI: 0.72–0.88); p < 0.001] (Table 1). In contrast to publication bias which was rare, substantial interstudy heterogeneity was detected for most health outcomes (Table 1 and Supplementary Figure S1). Sensitivity analyses using fixed-effect models suggest more statistically significant results than when a random-effect models was used, especially a statistically significant higher rate than expected for rectal cancer incidence [metaSIR = 1.33 (95%CI: 1.14–1.55); p < 0.001], stomach cancer mortality [metaSMR = 1.09 (95%CI: 1.03–1.16); p = 0.003], liver cancer mortality [metaSMR = 1.27 (95%CI: 1.15–1.41); p < 0.001], and liver and gallbladder cancer mortality combined [metaSMR = 1.12 (95%CI: 1.04–1.22); p = 0.004] (Supplementary Table S8).
3.2.1.2 Non-malignant health outcomes
Analyses included mortality data for diabetes mellitus, mental and behavioral disorders, nervous and sense organ disorders, and different type of non-malignant obstructive respiratory diseases, circulatory system diseases, and non-malignant digestive disorders (Table 1). Results suggest a statistically significant lower mortality rate than expected for digestive disorders [metaSMR = 0.80 (95%CI: 0.65–0.99), p = 0.04]. We found non-significantly increased mortality rates for bronchitis, emphysema, and asthma combined [metaSMR = 1.64 (95%CI: 0.94–2.87); p = 0.08], and for mental and behavioral disorders group [metaSMR = 1.54 (95%CI: 0.90–2.61); p = 0.11]. Heterogeneity between studies was high across the health outcomes studied. Both funnel plot and the Egger’s test indicate the presence of a publication bias for mortality risk from mental and behavioral disorders. Sensitivity analysis using fixed-effect models suggest substantial changes, including a statistically significant high mortality rate for bronchitis, emphysema, and asthma combined [metaSMR = 1.58 (95%CI: 1.36–1.84), p < 0.001], and a statistically significant mortality deficit for all circulatory system disease [metaSMR = 0.88 (95%CI: 0.87–0.89), p < 0.001], ischemic heart disease [metaSMR = 0.93 (95%CI: 0.91–0.95), p < 0.001], and nervous system and sense organ disorders [metaSMR = 0.88 (95%CI: 0.80–0.98), p = 0.02] (Supplementary Table S8 and Supplementary Figure S1).
3.2.2 Estimates for exposure-risk relationships
3.2.2.1 Malignant health outcomes risks
3.2.2.1.1 Among mine workers (occupational exposure)
Analyses were performed on incidence and/or mortality data for several cancer locations, including brain and CNS, extra-thoracic airways, and different types of lymphohematological, digestive, and male genito-urinary cancers. No statistically significant association was found. However, the metaRR per 100 WLM increase in radon exposure pointed toward an increased risk for several cancers: liver cancer mortality [metaRR = 1.05 (95% CI: 1.00–1.10); p = 0.06]; overall intestine and rectal cancer mortality [metaRR = 1.02 (95% CI: 1.00–1.04); p = 0.06]; leukemia excluding chronic lymphoblastic leukemia (non-CLL) mortality [metaRR = 1.22 (95%CI: 0.52–2.87); p = 0.64], etc. The lower bounds of the 95% CIs were close to 1 for mortality from several cancers (liver, intestine and rectal, and extra-thoracic airways, see Table 2). We found no influence of a specific study/estimate on the metaRR per 100 WLM for overall intestine and rectal cancer mortality, except an increase in the p-value when omitting one after the other the RRs of rectal cancer and intestine cancer retrieved from the study by Walsh et al. (53) (Supplementary Figure S3). The results remained unchanged when fixed-effect models were used (Supplementary Table S9). There was no evidence for residual heterogeneity among included studies for all health outcomes, except for chronic lymphoblastic leukemia (CLL) and non-CLL mortalities, Cochran’s Q-tests p = 0.008 and p = 0.076, respectively, and I-square value = 85.75% (95%CI: 42.77–96.45%), and 68.20% (95%CI: 0.00–92.83%), respectively. Overall, funnel plots and Egger’s tests suggest no evident publication bias (Table 2 and Supplementary Figure S2).
3.2.2.1.2 Among children (residential exposure)
Analyses among children in the general population included incidence data on leukemia, all lymphohematological cancer, and central nervous system tumors. The metaRRs per 100 Bq/m3 increase in residential radon concentration and their 95%CI suggest a marginally increased risk, but not statistically significant [1.01 (95%CI: 1.00–1.03), p = 0.126; 1.01 (95%CI: 1.00–1.03), p = 0.08; 1.02 (95%CI: 0.98–1.05), p = 0.43 for the three types of cancer, respectively]. Lower bounds of the 95%CI were close to, but remained inferior to 1 (which was not visible for some of them after 2-digit rounding). We found no influence of a specific study/estimate on the metaRR per Bq/m3 for all lymphohematological cancer incidence, except an increase in the p-value when omitting one after the other the study by Raaschou-Nielson et al. (25), and the RRs of leukemia and lymphoma retrieved from the study by Kendall et al. (23) (Supplementary Figure S4). Results did not change when fixed-effect models were used (Supplementary Table S9). There was no indication of inter-study heterogeneity, except for CNS tumors for which a moderate but not statistically significant residual heterogeneity was found among the included studies [Cochran’s Q-test p = 0.108; I-square value = 50.6% (0.00–83.67%)]. Neither funnel plots nor Egger’s test suggest evidence of publication bias (Table 2 and Supplementary Figure S2).
3.2.2.1.3 Among adults in the general population (residential exposure)
Only mortality from malignant melanoma and non-melanoma skin cancer were covered by a sufficient number of studies to be included in a meta-analysis. No statistically significant association was found. However, the metaRR per 100 Bq/m3 increase in residential radon concentration suggest a small decreased risk for non-melanoma skin cancer [0.907 (95%CI: 0.612–1.345); p = 0.069], whereas the metaRR for malignant melanoma was positive but not statistically significant [1.095 (95%CI: 0.993–1.209); p = 0.628]. The results did not change when fixed-effect model was used (Supplementary Table S9). There was no indication for residual heterogeneity among studies for both malignant melanoma and non-melanoma skin cancer. It was not possible to investigate for publication bias since the number of studies included in the analyses was less than three.
3.2.2.2 Non-malignant health outcomes risks
Analyses focused on mortality from chronic obstructive pulmonary disease, all circulatory system disease, ischemic heart disease, and cerebrovascular disease among mine workers. We found no statistically significant association, even with fixed effect models (Table 2 and Supplementary Table S9). Substantial heterogeneity was found among studies for all circulatory system disease and for cerebrovascular disease [Cochran’s Q-test p = 0.065 and 0.003, respectively, and I-square value = 51.86% (95%CI: 0.00–80.81%), and 78.50% (95%CI: 42.19–92.00%), respectively]. Both funnel plots and Egger’s tests do not support the existence of publication bias (see Table 2 and Supplementary Figure S2 for more details).
4 Discussion
We conducted a comprehensive systematic review and meta-analysis of the potential health effects other than lung cancer associated with radon exposure, covering occupational and residential radon exposure in children and adult populations, for both morbidity and mortality outcomes. This review covered a wide range of malignant and non-malignant diseases. Overall, regardless of the study design, there was an apparently good agreement between findings in children, adults in the general population with residential radon exposure, and mine workers with occupational radon exposure, across health outcomes that were studied in common in these populations, particularly lymphohematological cancers which were the most frequently studied. In most cases, individual study results pointed toward a lack of statistically significant association with radon exposure. Nevertheless, for some cancers, the average weighted estimates of exposure-risk associations were close to the statistical significance threshold, clearly justifying further research on their potential association with radon exposure. This was the case among mine workers for mortality from liver cancer, and also from “intestine and rectal” cancers combined. A positive estimate of exposure-risk association close to the statistical significance threshold was also observed among children for lymphohematological cancer incidence. Finally, this was also observed for malignant melanoma mortality among adults in the general population. It is worth noting that only two studies were included in the analyses for liver cancer mortality among mine workers and malignant melanoma mortality among adults in the general population, meaning these results should be interpreted with caution and their robustness would be improved by pooling with results from further studies in the future. Inter-study heterogeneity was present only in the occupational studies, especially among those included in the metaSIR or metaSMR estimation analyses.
While there is clear evidence that radon can cause lung cancer, even at low exposure levels (156), evidence from our study regarding other potential carcinogenic and non-carcinogenic effects of radon in humans is still inconsistent, whether among mine workers or children or adults in the general population, as it was found in previous reviews on health effects of radon exposure (157, 158). Yet, the hypothesis of radon exposure inducing cancer other than lung cancer is biological plausible. Inhalation of radon predominantly results in the exposure of cells in the lungs to alpha-particles, and only a very low proportion of inhaled radionuclides may enter the blood stream, and deliver dose to other organs like the red bone marrow, brain, heart, digestive system organs, etc. (159). Although direct DNA damage can only occur in cells traversed by alpha-particles after exposure to radon, damages may indirectly extend to the surrounding non-target cells trough molecular signals (160). In addition, radon exposure has been showed to induce systemic inflammation in uranium miners, which is known to increase risks of various cancers and non-cancerous diseases throughout the human body (161, 162).
Several factors may hinder the detection of associations by epidemiological studies. First, retrospective assessment of individual exposure is challenging in epidemiological studies, and many of them suffered from uncertainties in exposure assessment that can influence the exposure-risk relationships estimates toward the null if not correctly addressed (163). In the one hand, studies in the general population often used ecological approaches to assess radon exposure, and it is well known that radon concentrations may vary greatly within small geographical areas and across dwellings. While some case–control studies undertook direct short or long-term radon measurements, ranging from 3 days to 6 months or 1 year, no adjustment was made for seasonal variation in most cases. In addition, case–control studies involving contacts with participants could introduce selection bias due to the risk of low participation rates in controls. On the other hand, in most mine workplaces, the assessment of individual cumulative radon exposure for the earliest time periods was based on retrospective exposure reconstruction by experts and ambient dosimetry measurements. Individual dosimeters were generally introduced later. As a result, in both general population and occupational settings, there is a risk of measurement error, that may affect the health risk estimates toward the null if not correctly managed. However, such limitations did not greatly impair in the past the ability to detect strong associations between radon exposure and lung cancer risk (164). If weaker associations exist with other health effects, the uncertainties in exposure measurement might dilute such associations strongly enough that they cannot be detected anymore. Second, most of studies used mortality data. The use of mortality as a surrogate for incidence is likely to underestimate the true risk for diseases, especially for chronic diseases with relatively good survival rate (165). Finally, some studies did not adjust for important potential confounders, leading to difficulty to effectively disentangle radon health effects from those of exposure to other sources of ionizing radiation, including medical and gamma radiations, and other factors such as tobacco smoking. The statistically significant lower rates than expected (metaSIR or metaSMR) found for incidence of non-Hodgkin lymphoma, brain and CNS cancer, connective and other soft tissue cancer, kidney cancer alone or combined with “ureter and other urinary organs,” and for mortality from buccal and pharyngeal cancer, non-malignant digestive system disorders, are likely to reflect underestimations of the true risk due to the healthy worker effect, which is an issue inherent to occupational cohort studies (166), rather than a protective effect of radon exposure. Thus, these results should be interpreted with caution.
This is the first systematic review and meta-analysis to investigate many diseases at once as a potential health effect of radon exposure, without restriction on study population and radon exposure type. We pooled occupational and residential exposure data separately, since differences in exposure pattern (high exposure over a short duration versus low exposure over a continuous time scale) may result in differences in biological response and risk estimate. We also pooled data for children and adults separately due to the differences in response that may result from differences in baseline risks, latency periods, potential confounding factors or effect modifiers. Furthermore, we pooled incidence and mortality data separately, given the potential for risk underestimation that may be inherent to the use of mortality data. These approaches enable comparisons by exposure and population types, and we recommend this approach as part of future reviews and meta-analyses to better understand the underlying mechanisms that may explain any future epidemiological finding. Additionally, a careful attention was given to ICD codes reported in the included studies for homogeneity purposes, to make sure that studies using the same definition of a disease are pooled together, and that the right disease name is used. Another strength of this work was the use of a double quality assessment tool, the generic and validated NOS (see text footnote 4) and the UNSCEAR tool (17) which is specific to radiation epidemiology. None of the studies included in the meta-analysis was of low quality, moreover, we excluded ecological studies from the meta-analysis so that it may reflect a similar level of quality as the included studies.
Our systematic review and meta-analysis present some limits that need to be highlighted. First, few studies could be included in the meta-analysis for most health outcomes, especially regarding the exposure-risk relationships analyses based on incidence data. This may lead to a lack of power or consistency in results. Second, significant heterogeneity was estimated in studies on occupational exposure to radon, especially among those included in the metaSIR or metaSMR estimation analyses, which limits the interpretation of the average weighted estimates for the health outcomes that were concerned. We did not perform meta-regressions or more in-depth sensitivity analyses to investigate the sources of heterogeneity given the relatively limited number of studies/estimates involved in the analyses. However, for all metaRR close to the statistical significance threshold and whenever possible, we investigated the influence of each single study/estimate on the average weighted estimates, and no noticeable influence was found. Moreover, thanks to pooled studies on residential and occupational exposure to radon separately, and subsequently, studies involving children and adults, incidence data and mortality data may allow us to reduce heterogeneity that may arise from age, exposure level, and data validity differences across studies settings. Several potential reasons were thought to have contributed to the observed residual heterogeneity, including differences in the ways diseases are coded in practice in clinical sittings of each country; but this reason seems unlikely to introduce heterogeneity since this was rarely present among studies included in exposure-risk analyses in this work. One explanation may be differences in the cumulative radon dose received by mine workers in each cohort, depending on the type of mine (uranium, fluor, ore, zinc, etc.), the specific activity (underground or open pit mining, milling), and the environmental conditions. Other plausible explanations would be the possible variability in the baseline risks from one reference population to another (e.g., because cohorts of mine workers were set up in countries with contrasted socioeconomic levels), in mean age at first employment and follow-up duration from one cohort to another. Third, the health risks related to radon exposure that were considered in the meta-analyses were in the great majority based on studies on occupational exposure, and less on residential exposure given the predominance of ecological studies in the general population setting, which were not eligible for the meta-analysis. However, the qualitative summary tables (Supplementary Tables S4–S7) partly offset for this issue, and enables comparison of risks tendencies between children, adults in the general population, and mine workers, for a wide range of health outcomes, especially lymphohematological cancers which were the most studied health outcome in the three population groups.
5 Conclusion
While carcinogenic (other than lung cancer) and non-carcinogenic effects associated with radon exposure are biological plausible, the results of this systematic review and meta-analysis did not allow us to confirm radon-related risks other than lung cancer based on currently available epidemiological studies. Existing epidemiological studies are subject to several methodological limitations regarding radon exposure assessment, outcomes and confounding/modifying factors which may dilute the risk estimation, most likely toward the null. However, for several cancers, estimates of exposure-risk associations were close to statistical significance, clearly justifying further research on their potential association with radon exposure. As recommended by the UNSCEAR in the 2019 Report (167), larger and well-designed studies are needed to further investigate whether radon can cause diseases other than lung cancer in humans, and if so, to what extent, as well as potential modifiers such as gender, age or smoking.
Data availability statement
The original contributions presented in the study are included in the article/Supplementary material, further inquiries can be directed to the corresponding author.
Author contributions
AH: Conceptualization, Data curation, Formal analysis, Investigation, Methodology, Software, Writing – original draft, Writing – review & editing. OL: Conceptualization, Methodology, Supervision, Validation, Writing – original draft, Writing – review & editing. CM: Conceptualization, Validation, Writing – original draft, Writing – review & editing. EC: Conceptualization, Methodology, Supervision, Validation, Writing – original draft, Writing – review & editing.
Funding
The author(s) declare that financial support was received for the research, authorship, and/or publication of this article. Afi Mawulawoe Sylvie Henyoh PhD thesis is funded by the European project RadoNorm grant (2020–2025, Euratom-Grant Agreement n°900009). The publication fees of this article are entirely supported by the European project RadoNorm grant (2020–2025, Euratom-Grant Agreement n°900009).
Acknowledgments
The authors are grateful to Valérie Salmon from the IRSN for her help in building the bibliographic query. The authors are also grateful to Estelle Rage for answering to mine workers cohort-specific questions to effectively manage study population overlaps. The authors acknowledge Thi-Van-Trinh Tran, Kossi Abalo, and Marc Little for discussing some methodological issues.
Conflict of interest
The authors declare that the research was conducted in the absence of any commercial or financial relationships that could be construed as a potential conflict of interest.
Publisher’s note
All claims expressed in this article are solely those of the authors and do not necessarily represent those of their affiliated organizations, or those of the publisher, the editors and the reviewers. Any product that may be evaluated in this article, or claim that may be made by its manufacturer, is not guaranteed or endorsed by the publisher.
Supplementary material
The Supplementary material for this article can be found online at: https://www.frontiersin.org/articles/10.3389/fpubh.2024.1439355/full#supplementary-material
Footnotes
2. ^https://www.crd.york.ac.uk/prospero/
4. ^https://www.ohri.ca/programs/clinical_epidemiology/oxford.asp
References
1. United Nations. Scientific committee on the effects of atomic radiation. UNSCEAR 2006 Report volume II. Available at: https://www.unscear.org/unscear/en/publications/2006_2.html (Accessed March 26, 2024).
2. WHO. Handbook on indoor radon: a public health perspective. Available at: https://www.who.int/publications-detail-redirect/9789241547673 (Accessed January 5, 2024).
3. Malinovsky, G, Yarmoshenko, I, and Vasilyev, A. Meta-analysis of case–control studies on the relationship between lung cancer and indoor radon exposure. Radiat Environ Biophys. (2019) 58:39–47.
5. Cheng, ES, Egger, S, Hughes, S, Weber, M, Steinberg, J, Rahman, B, et al. Systematic review and meta-analysis of residential radon and lung cancer in never-smokers. Eur Respir Rev. (2021) 30:200230. doi: 10.1183/16000617.0230-2020
6. Kelly-Reif, K, Bertke, SJ, Rage, E, Dermers, PA, Fenske, N, Deffner, V, et al. Radon and lung cancer in the pooled uranium miners analysis (PUMA): highly exposed early miners and all miners. Occup Environ Med. (2023) 80:385–91. doi: 10.1136/oemed-2022-108532
7. Peckham, EC, Scheurer, ME, Danysh, HE, Lubega, J, Langlois, PH, and Lupo, PJ. Residential radon exposure and incidence of childhood lymphoma in Texas, 1995-2011. Int J Environ Res Public Health. (2015) 12:12110–26.
8. Boz, S, Berlin, C, Kwiatkowski, M, Bochud, M, Bulliard, JL, Zwahlen, M, et al. A prospective cohort analysis of residential radon and UV exposures and malignant melanoma mortality in the Swiss population. Environ Int. (2022) 169:107437. doi: 10.1016/j.envint.2022.107437
9. Kreuzer, M, Dufey, F, Marsh, JW, Nowak, D, Schnelzer, M, and Walsh, L. Mortality from cancers of the extra-thoracic airways in relation to radon progeny in the Wismut cohort, 1946-2008. Int J Radiat Biol. (2014) 90:1030–5.
10. Lu, L, Zhang, Y, Chen, C, Field, RW, and Kahe, K. Radon exposure and risk of cerebrovascular disease: a systematic review and meta-analysis in occupational and general population studies. Environ Sci Pollut Res Int. (2022) 29:45031–43.
11. Gómez-Anca, S, and Barros-Dios, JM. Radon exposure and neurodegenerative disease. Int J Environ Res Public Health. (2020) 17:7439. doi: 10.3390/ijerph17207439
12. Ruano-Ravina, A, Dacosta-Urbieta, A, Barros-Dios, JM, and Kelsey, KT. Radon exposure and tumors of the central nervous system. Gac Sanit. (2018) 32:567–75.
13. Moon, J, and Yoo, H. Residential radon exposure and leukemia: a meta-analysis and dose-response meta-analyses for ecological, case-control, and cohort studies. Environ Res. (2021) 202:111714. doi: 10.1016/j.envres.2021.111714
14. Chen, B, Yuan, TW, Wang, AQ, Zhang, H, Fang, LJ, Wu, QQ, et al. Exposure to radon and kidney cancer: a systematic review and meta-analysis of observational epidemiological studies. Biomed Environ Sci. (2018) 31:805–15. doi: 10.3967/bes2018.108
15. Page, MJ, Moher, D, Bossuyt, PM, Boutron, I, Hoffmann, TC, Mulrow, CD, et al. PRISMA 2020 explanation and elaboration: updated guidance and exemplars for reporting systematic reviews. BMJ. (2021) 372:n160. doi: 10.1136/bmj.n160
16. Page, MJ, McKenzie, JE, Bossuyt, PM, Boutron, I, Hoffmann, TC, Mulrow, CD, et al. Statement: an updated guideline for reporting systematic reviews. BMJ. (2020) 2021:n71. doi: 10.1136/bmj.n71
17. United Nations. Scientific committee on the effects of atomic radiation. UNSCEAR 2017 report. Available at: http://www.unscear.org/unscear/en/publications/2017.html (Accessed January 3, 2024).
18. Greenland, S, and Longnecker, MP. Methods for trend estimation from summarized dose-response data, with applications to meta-analysis. Am J Epidemiol. (1992) 135:1301–9.
19. Orsini, N, Bellocco, R, and Greenland, S. Generalized least squares for trend estimation of summarized dose–response data. Stata J. (2006) 6:40–57.
20. Callas, PW, Pastides, H, and Hosmer, DW. Empirical comparisons of proportional hazards, poisson, and logistic regression modeling of occupational cohort data. Am J Ind Med. (1998) 33:33–47. doi: 10.1002/(SICI)1097-0274(199801)33:1<33::AID-AJIM5>3.0.CO;2-X
21. King, G, and Zeng, L. Estimating risk and rate levels, ratios and differences in case-control studies. Stat Med. (2002) 21:1409–27.
22. Vandenbroucke, JP. A shortcut method for calculating the 95 per cent confidence interval of the standardized mortality ratio. Am J Epidemiol. (1982) 115:303–4.
23. Kendall, GM, Little, MP, Wakeford, R, Bunch, KJ, Miles, JCH, Vincent, TJ, et al. A record-based case-control study of natural background radiation and the incidence of childhood leukaemia and other cancers in Great Britain during 1980–2006. Leukemia. (2013) 27:3–9. doi: 10.1038/leu.2012.151
24. Nikkilä, A, Arvela, H, Mehtonen, J, Raitanen, J, Heinäniemi, M, Lohi, O, et al. Predicting residential radon concentrations in Finland: model development, validation, and application to childhood leukemia. Scand J Work Environ Health. (2020) 46:278–92. doi: 10.5271/sjweh.3867
25. Raaschou-Nielsen, O, Andersen, CE, Andersen, HP, Gravesen, P, Lind, M, Schüz, J, et al. Domestic radon and childhood cancer in Denmark. Epidemiology. (2008) 19:536–43. doi: 10.1097/EDE.0b013e318176bfcd
26. Silver, SR, Bertke, SJ, Hein, MJ, Daniels, RD, Fleming, DA, Anderson, JL, et al. Mortality and ionising radiation exposures among workers employed at the Fernald feed materials production center (1951–1985). Occup Environ Med. (2013) 70:453–63. doi: 10.1136/oemed-2012-100768
27. Richardson, DB, Abalo, K, Bernier, MO, Rage, E, Leuraud, K, Laurier, D, et al. Meta-analysis of published excess relative risk estimates. Radiat Environ Biophys. (2020) 59:631–41. doi: 10.1007/s00411-020-00863-w
29. Rage, E, Caër-Lorho, S, and Laurier, D. Low radon exposure and mortality among Jouac uranium miners an update of the French cohort (1946-2007). J Radiol Prot. (2018) 38:92–108. doi: 10.1088/1361-6498/aa8d97
30. Zablotska, LB, Lane, RSD, Frost, SE, and Thompson, PA. Leukemia, lymphoma and multiple myeloma mortality (1950-1999) and incidence (1969-1999) in the Eldorado uranium workers cohort. Environ Res. (2014) 130:43–50.
31. Zablotska, LB, Fenske, N, Schnelzer, M, Zhivin, S, Laurier, D, and Kreuzer, M. Analysis of mortality in a pooled cohort of Canadian and German uranium processing workers with no mining experience. Int Arch Occup Environ Health. (2018) 91:91–103.
32. Zablotska, LB, Lane, RSD, and Frost, SE. Mortality (1950–1999) and cancer incidence (1969–1999) of workers in the Port Hope cohort study exposed to a unique combination of radium, uranium and γ-ray doses. BMJ Open. (2013) 3:e002159. doi: 10.1136/bmjopen-2012-002159
33. Kreuzer, M, Grosche, B, Schnelzer, M, Tschense, A, Dufey, F, and Walsh, L. Radon and risk of death from cancer and cardiovascular diseases in the German uranium miners cohort study: follow-up 1946–2003. Radiat Environ Biophys. (2010) 49:177–85.
34. Kreuzer, M, Sogl, M, Brüske, I, Möhner, M, Nowak, D, Schnelzer, M, et al. Silica dust, radon and death from non-malignant respiratory diseases in German uranium miners. Occup Environ Med. (2013) 70:869–75. doi: 10.1136/oemed-2013-101582
35. Lane, RSD, Frost, SE, Howe, GR, and Zablotska, LB. Mortality (1950–1999) and cancer incidence (1969–1999) in the cohort of Eldorado uranium workers. Radiat Res. (2010) 174:773–85. doi: 10.1667/RR2237.1
36. Higgins, JPT, Thompson, SG, Deeks, JJ, and Altman, DG. Measuring inconsistency in meta-analyses. BMJ. (2003) 327:557–60. doi: 10.1136/bmj.327.7414.557
37. Der Simonian, R, and Laird, N. Meta-analysis in clinical trials. Control Clin Trials. (1986) 7:177–88.
38. Egger, M, Davey Smith, G, Schneider, M, and Minder, C. Bias in meta-analysis detected by a simple, graphical test. BMJ. (1997) 315:629–34. doi: 10.1136/bmj.315.7109.629
39. Song, F, Khan, KS, Dinnes, J, and Sutton, AJ. Asymmetric funnel plots and publication bias in meta-analyses of diagnostic accuracy. Int J Epidemiol. (2002) 31:88–95. doi: 10.1093/ije/31.1.88
40. Viechtbauer, W, and Cheung, MWL. Outlier and influence diagnostics for meta-analysis. Res Synth Methods. (2010) 1:112–25.
41. Kelly-Reif, K, Bertke, S, Daniels, RD, Richardson, DB, and Schubauer-Berigan, MK. Nonmalignant respiratory disease mortality in male Colorado plateau uranium miners, 1960-2016. Am J Ind Med. (2022) 65:773–82. doi: 10.1002/ajim.23419
42. Schubauer-Berigan, MK, Daniels, RD, and Pinkerton, LE. Radon exposure and mortality among white and American Indian uranium miners: an update of the Colorado plateau cohort. Am J Epidemiol. (2009) 169:718–30. doi: 10.1093/aje/kwn406
43. Roscoe, RJ. An update of mortality from all causes among white uranium miners from the Colorado plateau study group. Am J Ind Med. (1997) 31:211–22. doi: 10.1002/(SICI)1097-0274(199702)31:2<211::AID-AJIM11>3.0.CO;2-4
44. Řeřicha, V, Kulich, M, Řeřicha, R, Shore, DL, and Sandler, DP. Incidence of leukemia, lymphoma, and multiple myeloma in Czech uranium miners: a case–cohort study. Environ Health Perspect. (2006) 114:818–22. doi: 10.1289/ehp.8476
45. Kelly-Reif, K, Sandler, DP, Shore, D, Schubauer-Berigan, M, Troester, M, Nylander-French, L, et al. Lung and extrathoracic cancer incidence among underground uranium miners exposed to radon progeny in the Příbram region of the Czech Republic: a case-cohort study. Occup Environ Med. (2022) 79:102–8. doi: 10.1136/oemed-2021-107392
46. Kulich, M, Reřicha, V, Reřicha, R, Shore, DL, and Sandler, DP. Incidence of non-lung solid cancers in Czech uranium miners: a case-cohort study. Environ Res. (2011) 111:400–5. doi: 10.1016/j.envres.2011.01.008
47. Kelly-Reif, K, Sandler, DP, Shore, D, Schubauer-Berigan, M, Troester, MA, Nylander-French, L, et al. Mortality and cancer incidence among underground uranium miners in the Czech Republic 1977-1992. Occup Environ Med. (2019) 76:511–8. doi: 10.1136/oemed-2018-105562
48. Kelly-Reif, K, Sandler, DP, Shore, D, Schubauer-Berigan, MK, Troester, MA, Nylander-French, L, et al. Radon and cancer mortality among underground uranium miners in the Příbram region of the Czech Republic. Am J Ind Med. (2020) 63:859–67. doi: 10.1002/ajim.23167
49. Tomásek, L, Darby, SC, Swerdlow, AJ, Placek, V, and Kunz, E. Radon exposure and cancers other than lung cancer among uranium miners in West Bohemia. Lancet. (1993) 341:919–23.
50. Navaranjan, G, Berriault, C, Do, M, Villeneuve, PJ, and Demers, PA. Cancer incidence and mortality from exposure to radon progeny among Ontario uranium miners. Occup Environ Med. (2016) 73:oemed-2016-103836–45. doi: 10.1136/oemed-2016-103836
51. Zeng, X, Berriault, C, Arrandale, VH, DeBono, NL, Harris, MA, and Demers, PA. Radon exposure and risk of neurodegenerative diseases among male miners in Ontario, Canada: a cohort study. Am J Ind Med. (2023) 66:132–41. doi: 10.1002/ajim.23449
52. Kreuzer, M, Kreisheimer, M, Kandel, M, Schnelzer, M, Tschense, A, and Grosche, B. Mortality from cardiovascular diseases in the German uranium miners cohort study, 1946–1998. Radiat Environ Biophys. (2006) 45:159–66.
53. Walsh, L, Dufey, F, Tschense, A, Schnelzer, M, Grosche, B, and Kreuzer, M. Radon and the risk of cancer mortality—internal poisson models for the German uranium miners cohort. Health Phys. (2010) 99:292–300. doi: 10.1097/HP.0b013e3181cd669d
54. Kreuzer, M, Deffner, V, Schnelzer, M, and Fenske, N. Mortality in underground miners in a former uranium ore mine–results of a cohort study among former employees of Wismut AG in Saxony and Thuringia. Dtsch Arztebl Int. (2021) 118:41–8.
55. Kreuzer, M, Dufey, F, Laurier, D, Nowak, D, Marsh, JW, Schnelzer, M, et al. Mortality from internal and external radiation exposure in a cohort of male German uranium millers, 1946–2008. Int Arch Occup Environ Health. (2015) 88:431–41. doi: 10.1007/s00420-014-0973-2
56. Möhner, M, Lindtner, M, Otten, H, and Gille, HG. Leukemia and exposure to ionizing radiation among German uranium miners. Am J Ind Med. (2006) 49:238–48.
57. Möhner, M, Lindtner, M, and Otten, H. Ionizing radiation and risk of laryngeal cancer among German uranium miners. Health Phys. (2008) 95:725.
58. Nusinovici, S, Vacquier, B, Leuraud, K, Metz-Flamant, C, Caër-Lorho, S, Acker, A, et al. Mortality from circulatory system diseases and low-level radon exposure in the French cohort study of uranium miners, 1946-1999. Scand J Work Environ Health. (2010) 36:373–83. doi: 10.5271/sjweh.2896
59. Drubay, D, Caër-Lorho, S, Laroche, P, Laurier, D, and Rage, E. Mortality from circulatory system diseases among French uranium miners a nested case-control study. Radiat Res. (2015) 183:550–62. doi: 10.1667/RR13834.1
60. Drubay, D, Ancelet, S, Acker, A, Kreuzer, M, Laurier, D, and Rage, E. Kidney cancer mortality and ionizing radiation among French and German uranium miners. Radiat Environ Biophys. (2014) 53:505–13.
61. Golden, AP, Milder, CM, Ellis, ED, Anderson, JL, Boice, JD Jr, Bertke, SJ, et al. Cohort profile: four early uranium processing facilities in the US and Canada. Int J Radiat Biol. (2021) 97:833–47. doi: 10.1080/09553002.2021.1917786
62. Richardson, DB, Rage, E, Demers, PA, Do, MT, DeBono, N, Fenske, N, et al. Mortality among uranium miners in North America and Europe: the pooled uranium miners analysis (PUMA). Int J Epidemiol. (2021) 50:633–43. doi: 10.1093/ije/dyaa195
63. Jr, JDB, Cohen, SS, Mumma, MT, Chadda, B, and Blot, WJ. A cohort study of uranium millers and miners of Grants, New Mexico, 1979–2005. J Radiol Prot. (2008) 28:303–25. doi: 10.1088/0952-4746/28/3/002
64. Veiga, LHS, Amaral, ECS, Colin, D, and Koifman, S. A retrospective mortality study of workers exposed to radon in a Brazilian underground coal mine. Radiat Environ Biophys. (2006) 45:125–34. doi: 10.1007/s00411-006-0046-3
65. Darby, SC, Radford, EP, and Whitley, E. Radon exposure and cancers other than lung cancer in Swedish iron miners. Environ Health Perspect. (1995) 103:45–7. doi: 10.1289/ehp.95103s245
66. Darby, SC, Whitley, E, Howe, GR, Hutchings, SJ, Kusiak, RA, Lubin, JH, et al. Radon and cancers other than lung cancer in underground miners: a collaborative analysis of 11 studies. J Natl Cancer Inst. (1995) 87:378–84. doi: 10.1093/jnci/87.5.378
67. Cocco, PL, Carta, P, Belli, S, Picchiri, GF, and Flore, MV. Mortality of Sardinian lead and zinc miners: 1960-88. Occup Environ Med. (1994) 51:674–82.
68. Xiang-Zhen, X, Lubin, JH, Jun-Yao, L, Li-Fen, Y, Sheng, LQ, Lan, Y, et al. A cohort study in southern China of tin miners exposed to radon and radon decay products. Health Phys. (1993) 64:120–31. doi: 10.1097/00004032-199302000-00001
69. Chen, SY, Hayes, RB, Liang, SR, Li, QG, Stewart, PA, and Blair, A. Mortality experience of haematite mine workers in China. Br J Ind Med. (1990) 47:175–81.
70. Golden, AP, Ellis, ED, Cohen, SS, Mumma, MT, Leggett, RW, Wallace, PW, et al. Updated mortality analysis of the Mallinckrodt uranium processing workers, 1942–2012. Int J Radiat Biol. (2022) 98:701–21. doi: 10.1080/09553002.2019.1569773
71. Tomásek, L, Swerdlow, AJ, Darby, SC, Placek, V, and Kunz, E. Mortality in uranium miners in West Bohemia: a long-term cohort study. Occup Environ Med. (1994) 51:308–15.
72. Ahlman, K, Koskela, RS, Kuikka, P, Koponen, M, and Annanmäki, M. Mortality among sulfide ore miners. Am J Ind Med. (1991) 19:603–17.
73. Hodgson, JT, and Jones, RD. Mortality of a cohort of tin miners 1941-86. Occup Environ Med. (1990) 47:665–76. doi: 10.1136/oem.47.10.665
74. Villeneuve, PJ, Morrison, HI, Volesky, K, and Lane, RSD. Circulatory system disease mortality and occupational exposure to radon progeny in the cohort of Newfoundland fluorspar miners between 1950 and 2016. Int Arch Occup Environ Health. (2023) 96:411–8. doi: 10.1007/s00420-022-01932-x
75. Langlois, PH, Lee, M, Lupo, PJ, Rahbar, MH, and Cortez, RK. Residential radon and birth defects: a population-based assessment. Birth Defects Res A Clin Mol Teratol. (2016) 106:5–15. doi: 10.1002/bdra.23369
76. Steinbuch, M, Weinberg, CR, Buckley, JD, Robison, LL, and Sandler, DP. Indoor residential radon exposure and risk of childhood acute myeloid leukaemia. Br J Cancer. (1999) 81:900–6.
77. Lubin, JH, Linet, MS, Boice, JD, Buckley, J, Conrath, SM, Hatch, EE, et al. Case-control study of childhood acute lymphoblastic leukemia and residential radon exposure. J Natl Cancer Inst. (1998) 90:294–300. doi: 10.1093/jnci/90.4.294
78. Collman, GW, Loomis, DP, and Sandler, DP. Childhood cancer mortality and radon concentration in drinking water in North Carolina. Br J Cancer. (1991) 63:626–9. doi: 10.1038/bjc.1991.143
79. Hauri, D, Spycher, B, Huss, A, Zimmermann, F, Grotzer, M, von der Weid, N, et al. Domestic radon exposure and risk of childhood cancer: a prospective census-based cohort study. Environ Health Perspect. (2013) 121:1239–44. doi: 10.1289/ehp.1306500
80. Kohli, S, Noorlind, B, and Lofman, O. Childhood leukaemia in areas with different radon levels: a spatial and temporal analysis using GIS. J Epidemiol Community Health. (2000) 54:822–6.
81. Kaletsch, U, Kaatsch, P, Meinert, R, Schüz, J, Czarwinski, R, and Michaelis, J. Childhood cancer and residential radon exposure – results of a population-based case-control study in Lower Saxony (Germany). Radiat Environ Biophys. (1999) 38:211–5.
82. Thorne, R, Foreman, NK, and Mott, MG. Radon in Devon and Cornwall and paediatric malignancies. Eur J Cancer. (1996) 32:282–5.
83. Foreman, NK, Thorne, R, Berry, PJ, Oakhill, A, and Mott, MG. Childhood malignancies in the south-west region of England, 1976-1985. Med Pediatr Oncol. (1994) 23:14–9. doi: 10.1002/mpo.2950230104
84. Chen, J, and Xie, L. Domestic radon exposure and childhood leukaemia and lymphoma: a population-based study in Canada. Radiat Prot Dosim. (2019) 184:486–92.
85. Del Risco, KR, Blaasaas, KG, and Claussen, B. Risk of leukaemia or cancer in the central nervous system among children living in an area with high indoor radon concentrations: results from a cohort study in Norway. Br J Cancer. (2014) 111:1413–20.
86. Yoshinaga, S, Tokonami, S, Akiba, S, Nitta, H, and Kabuto, M. Case-control study of residential radon and childhood leukemia in Japan: results from preliminary analyses. Int Congr Ser. (2005) 1276:233–5.
87. Berlivet, J, Hémon, D, Cléro, É, Ielsch, G, Laurier, D, Faure, L, et al. Residential exposure to natural background radiation at birth and risk of childhood acute leukemia in France, 1990–2009. J Environ Radioact. (2021) 233:106613. doi: 10.1016/j.jenvrad.2021.106613
88. Demoury, C, Marquant, F, Ielsch, G, Goujon, S, Debayle, C, Faure, L, et al. Residential exposure to natural background radiation and risk of childhood acute leukemia in France, 1990–2009. Environ Health Perspect. (2017) 125:714–20. doi: 10.1289/EHP296
89. Berlivet, J, Hémon, D, Cléro, É, Ielsch, G, Laurier, D, Guissou, S, et al. Ecological association between residential natural background radiation exposure and the incidence rate of childhood central nervous system tumors in France, 2000-2012. J Environ Radioact. (2020) 211:106071. doi: 10.1016/j.jenvrad.2019.106071
90. Mukharesh, L, Greco, KF, Banzon, T, Koutrakis, P, Li, L, Hauptman, M, et al. Environmental radon and childhood asthma. Pediatr Pulmonol. (2022) 57:3165–8. doi: 10.1002/ppul.26143
91. Sheehan, A, Freni Sterrantino, A, Fecht, D, Elliott, P, and Hodgson, S. Childhood type 1 diabetes: an environment-wide association study across England. Diabetologia. (2020) 63:964–76. doi: 10.1007/s00125-020-05087-7
92. UK Childhood Cancer Study Investigators. The United Kingdom childhood cancer study of exposure to domestic sources of ionising radiation: 1: radon gas. Br J Cancer. (2002) 86:1721–6. doi: 10.1038/sj.bjc.6600276
93. McLaughlin, JR, King, WD, Anderson, TW, Clarke, EA, and Ashmore, JP. Paternal radiation exposure and leukaemia in offspring: the Ontario case-control study. BMJ. (1993) 307:959–66. doi: 10.1136/bmj.307.6910.959
94. Muirhead, CR, Butland, BK, Green, BMR, and Draper, GJ. Childhood leukaemia and natural radiation. Lancet. (1991) 337:503–4. doi: 10.1016/0140-6736(91)93451-E
95. Kim, SH, Park, JM, and Kim, H. The prevalence of stroke according to indoor radon concentration in South Koreans: nationwide cross section study. Medicine (Baltimore). (2020) 99:e18859. doi: 10.1097/MD.0000000000018859
96. Teras, LR, Diver, WR, Turner, MC, Krewski, D, Sahar, L, Ward, E, et al. Residential radon exposure and risk of incident hematologic malignancies in the cancer prevention study-II nutrition cohort. Environ Res. (2016) 148:46–54. doi: 10.1016/j.envres.2016.03.002
97. Ruano-Ravina, A, Cameselle-Lago, C, Torres-Durán, M, Pando-Sandoval, A, Dacal-Quintas, R, Valdés-Cuadrado, L, et al. Indoor radon exposure and COPD, synergic association? A multicentric, hospital-based case-control study in a radon-prone area. Arch Bronconeumol. (2021) 57:630–6.
98. Barbosa-Lorenzo, R, Ruano-Ravina, A, Ramis, R, Aragonés, N, Kelsey, KT, Carballeira-Roca, C, et al. Residential radon and COPD. An ecological study in Galicia, Spain. Int J Radiat Biol. (2017) 93:222–30. doi: 10.1080/09553002.2017.1238526
99. Ruano-Ravina, A, Aragonés, N, Kelsey, KT, Pérez-Ríos, M, Piñeiro-Lamas, M, López-Abente, G, et al. Residential radon exposure and brain cancer: an ecological study in a radon prone area (Galicia, Spain). Sci Rep. (2017) 7:3595. doi: 10.1038/s41598-017-03938-9
100. López-Abente, G, Núñez, O, Fernández-Navarro, P, Barros-Dios, JM, Martín-Méndez, I, Bel-Lan, A, et al. Residential radon and cancer mortality in Galicia, Spain. Sci Total Environ. (2018) 610–611:1125–32. doi: 10.1016/j.scitotenv.2017.08.144
101. Ruano-Ravina, A, Aragonés, N, Pérez-Ríos, M, López-Abente, G, and Barros-Dios, JM. Residential radon exposure and esophageal cancer. An ecological study from an area with high indoor radon concentration (Galicia, Spain). Int J Radiat Biol. (2014) 90:299–305. doi: 10.3109/09553002.2014.886792
102. Monastero, RN, and Meliker, JR. Incidence of brain and spinal cord cancer and county-level radon levels in New Jersey, Wisconsin, Minnesota, Pennsylvania, and Iowa, USA. Environ Geochem Health. (2020) 42:389–95. doi: 10.1007/s10653-019-00368-6
103. Bräuner, EV, Andersen, ZJ, Andersen, CE, Pedersen, C, Gravesen, P, Ulbak, K, et al. Residential radon and brain tumour incidence in a Danish cohort. PLoS One. (2013) 8:e74435. doi: 10.1371/journal.pone.0074435
104. Bräuner, EV, Loft, S, Sørensen, M, Jensen, A, Andersen, CE, Ulbak, K, et al. Residential radon exposure and skin cancer incidence in a prospective Danish cohort. PLoS One. (2015) 10:e0135642. doi: 10.1371/journal.pone.0135642
105. Schwartz, GG, and Klug, MG. Incidence rates of chronic lymphocytic leukemia in US states are associated with residential radon levels. Future Oncol. (2016) 12:165–74.
106. Oancea, SC, Rundquist, BC, Simon, I, Swartz, S, Zheng, Y, Zhou, X, et al. County level incidence rates of chronic lymphocytic leukemia are associated with residential radon levels. Future Oncol. (2017) 13:1873–81. doi: 10.2217/fon-2017-0165
107. Wheeler, BW, Allen, J, Depledge, MH, and Curnow, A. Radon and skin cancer in Southwest England: an ecologic study. Epidemiology. (2012) 23:44–52. doi: 10.1097/EDE.0b013e31823b6139
108. Papatheodorou, S, Yao, W, Vieira, CLZ, Li, L, Wylie, BJ, Schwartz, J, et al. Residential radon exposure and hypertensive disorders of pregnancy in Massachusetts, USA: a cohort study. Environ Int. (2021) 146:106285. doi: 10.1016/j.envint.2020.106285
109. VoPham, T, DuPré, N, Tamimi, RM, James, P, Bertrand, KA, Vieira, V, et al. Environmental radon exposure and breast cancer risk in the nurses’ health study II. Environ Health. (2017) 16:97. doi: 10.1186/s12940-017-0305-6
110. Turner, MC, Krewski, D, Chen, Y, Pope, CA III, Gapstur, SM, and Thun, MJ. Radon and nonrespiratory mortality in the American Cancer Society cohort. Am J Epidemiol. (2012) 176:808–14.
111. Turner, MC, Krewski, D, Chen, Y, Pope, CA, Gapstur, SM, and Thun, MJ. Radon and COPD mortality in the American Cancer Society cohort. Eur Respir J. (2012) 39:1113–9.
112. Barbosa-Lorenzo, R, Barros-Dios, JM, Raíces Aldrey, M, Cerdeira Caramés, S, and Ruano-Ravina, A. Residential radon and cancers other than lung cancer: a cohort study in Galicia, a Spanish radon-prone area. Eur J Epidemiol. (2016) 31:437–41. doi: 10.1007/s10654-016-0134-x
113. Pando-Sandoval, A, Ruano-Ravina, A, Torres-Durán, M, Dacal-Quintas, R, Valdés-Cuadrado, L, Hernández-Hernández, JR, et al. Residential radon and characteristics of chronic obstructive pulmonary disease. Sci Rep. (2022) 12:1381. doi: 10.1038/s41598-022-05421-6
114. Nilles, JD, Lim, D, Boyer, MP, Wilson, BD, Betar, RA, Showalter, HA, et al. The occurrence of bone and joint cancers and their association with rural living and radon exposure in Iowa. Environ Geochem Health. (2022) 45:925–40. doi: 10.1007/s10653-022-01261-5
115. Mauriz-Barreiro, V, Barreiro-de Acosta, M, Bastón-Rey, I, Ferreiro-Iglesias, R, Calviño-Suárez, C, Barros-Dios, JM, et al. Radon exposure and inflammatory bowel disease in a radon prone area. Rev Esp Enferm Dig. (2022) 114:405–9. doi: 10.17235/reed.2021.8239/2021
116. Abaszadeh Fathabadi, Z, Ehrampoush, MH, Mirzaei, M, Mokhtari, M, Nadi Sakhvidi, M, Rahimdel, A, et al. The relationship of indoor radon gas concentration with multiple sclerosis: a case-control study. Environ Sci Pollut Res. (2020) 27:16350–61. doi: 10.1007/s11356-020-08147-y
117. Messier, KP, and Serre, ML. Lung and stomach cancer associations with groundwater radon in North Carolina, USA. Int J Epidemiol. (2017) 46:676–85.
118. Schwartz, GG, and Klug, MG. Motor neuron disease mortality rates in U.S. states are associated with well water use. Amyotrophic Lateral Scler Frontotemporal Degener. (2016) 17:528–34. doi: 10.1080/21678421.2016.1195409
119. Groves-Kirkby, CJ, Denman, AR, Campbell, J, Crockett, RGM, Phillips, PS, and Rogers, S. Is environmental radon gas associated with the incidence of neurodegenerative conditions? A retrospective study of multiple sclerosis in radon affected areas in England and Wales. J Environ Radioact. (2016) 154:1–14. doi: 10.1016/j.jenvrad.2015.12.003
120. Goyal, N, Camacho, F, Mangano, J, and Goldenberg, D. Evaluating for a geospatial relationship between radon levels and thyroid cancer in Pennsylvania. Laryngoscope. (2015) 125:E45–9.
121. Wheeler, BW, Kothencz, G, and Pollard, AS. Geography of non-melanoma skin cancer and ecological associations with environmental risk factors in England. Br J Cancer. (2013) 109:235–41. doi: 10.1038/bjc.2013.288
122. Auvinen, A, Kurttio, P, Pekkanen, J, Pukkala, E, Ilus, T, and Salonen, L. Uranium and other natural radionuclides in drinking water and risk of leukemia: a case–cohort study in Finland. Cancer Causes Control. (2002) 13:825–9.
123. Auvinen, A, Salonen, L, Pekkanen, J, Pukkala, E, Ilus, T, and Kurttio, P. Radon and other natural radionuclides in drinking water and risk of stomach cancer: a case-cohort study in Finland. Int J Cancer. (2005) 114:109–13. doi: 10.1002/ijc.20680
124. Kurttio, P, Salonen, L, Ilus, T, Pekkanen, J, Pukkala, E, and Auvinen, A. Well water radioactivity and risk of cancers of the urinary organs. Environ Res. (2006) 102:333–8.
125. Forastiere, F, Sperati, A, Cherubini, G, Miceli, M, Biggeri, A, and Axelson, O. Adult myeloid leukaemia, geology, and domestic exposure to radon and gamma radiation: a case control study in Central Italy. Occup Environ Med. (1998) 55:106–10.
126. Puskin, JS. Smoking as a confounder in ecologic correlations of cancer mortality rates with average county radon levels. Health Phys. (2003) 84:526–32. doi: 10.1097/00004032-200304000-00012
127. Boice, JD, Cohen, SS, Mumma, MT, Chadda, B, and Blot, WJ. Mortality among residents of Uravan, Colorado who lived near a uranium mill, 1936-84. J Radiol Prot. (2007) 27:299–319.
128. Jr, JDB, Mumma, M, Schweitzer, S, and Blot, WJ. Cancer mortality in a Texas county with prior uranium mining and milling activities, 1950–2001. J Radiol Prot. (2003) 23:247–62. doi: 10.1088/0952-4746/23/3/302
129. Smith, BJ, Zhang, L, and Field, RW. Iowa radon leukaemia study: a hierarchical population risk model for spatially correlated exposure measured with error. Stat Med. (2007) 26:4619–42. doi: 10.1002/sim.2884
130. Ye, W, Sobue, T, Lee, VS, Tanooka, H, Mifune, M, Suyama, A, et al. Mortality and cancer incidence in Misasa, Japan, a spa area with elevated radon levels. Jpn J Cancer Res. (1998) 89:789–96. doi: 10.1111/j.1349-7006.1998.tb00630.x
131. Mifune, M, Sobue, T, Arimoto, H, Komoto, Y, Kondo, S, and Tanooka, H. Cancer mortality survey in a spa area (Misasa, Japan) with a high radon background. Jpn J Cancer Res. (1992) 83:1–5.
132. Suzuki, Y, Honjo, S, Kawamura, H, Koishi, F, Suzuki, T, and Hirohata, T. Cancer mortality in low radon spa area. Jpn J Cancer Res. (1994) 85:1063–6.
133. Lehrer, S, Rheinstein, PH, and Rosenzweig, KE. Association of radon background and total background ionizing radiation with Alzheimer’s disease deaths in U.S. States. J Alzheimer’s Dis. (2017) 59:737–41. doi: 10.3233/JAD-170308
134. Reddy, NK, and Bhutani, MS. Racial disparities in pancreatic cancer and radon exposure: a correlation study. Pancreas. (2009) 38:391–5. doi: 10.1097/MPA.0b013e31819b03a8
135. Etherington, DJ, Pheby, DF, and Bray, FI. An ecological study of cancer incidence and radon levels in south West England. Eur J Cancer. (1996) 32:1189–97. doi: 10.1016/0959-8049(96)00060-3
136. Neilson, S, Robinson, I, and Rose, FC. Ecological correlates of motor neuron disease mortality: a hypothesis concerning an epidemiological association with radon gas and gamma exposure. J Neurol. (1996) 243:329–36.
137. Tomášek, L, Müller, T, Kunz, E, Heribanová, A, Matzner, J, Plaček, V, et al. Czech residential radon study. Int Congr Ser. (2002) 1225:239–45. doi: 10.1016/S0531-5131(01)00522-2
138. Viel, JF. Radon exposure and Leukaemia in adulthood. Int J Epidemiol. (1993) 22:627–31. doi: 10.1093/ije/22.4.627
139. Cohen, BL. Relationship between exposure to radon and various types of cancer. Health Phys. (1993) 65:529–31. doi: 10.1097/00004032-199311000-00009
140. Miller, AS, Harwick, RD, Alfaro-Miranda, M, and Sundararajan, M. Search for correlation of radon levels and incidence of salivary gland tumors. Oral Surg Oral Med Oral Pathol. (1993) 75:58–63. doi: 10.1016/0030-4220(93)90407-u
141. Miller, D, Morrison, H, Semenciw, R, and Mao, Y. Leukemia and residential exposure to radon. Can J Public Health. (1993) 84:205–6.
142. Neuberger, JS, and Field, RW. Radon and breast cancer. Risk Anal. (1996) 16:729–30. doi: 10.1111/j.1539-6924.1996.tb00823.x
144. Bølviken, B, Celius, EG, Nilsen, R, and Strand, T. Radon: a possible risk factor in multiple sclerosis. Neuroepidemiology. (2003) 22:87–94.
145. Schwartz, GG, and Klug, MG. Thyroid cancer incidence rates in North Dakota are associated with land and water use. Int J Environ Res Public Health. (2019) 16:3805. doi: 10.3390/ijerph16203805
146. Law, GR, Kane, EV, Roman, E, Smith, A, and Cartwright, R. Residential radon exposure and adult acute leukaemia. Lancet. (2000) 355:1888.
147. Kjellberg, S, and Wiseman, JS. The relationship of radon to gastrointestinal malignancies. Am Surg. (1995) 61:822–5.
148. Forastiere, F, Quiercia, A, Cavariani, F, Miceli, M, Perucci, CA, and Axelson, O. Cancer risk and radon exposure. Lancet. (1992) 339:1115.
149. Boice, JD, Mumma, MT, and Blot, WJ. Cancer and noncancer mortality in populations living near uranium and vanadium mining and milling operations in Montrose County, Colorado, 1950–2000. rare. (2007) 167:711–26.
150. Ha, M, Hwang, SS, Kang, S, Park, NW, Chang, BU, and Kim, Y. Geographical correlations between indoor radon concentration and risks of lung cancer, non-Hodgkin’s lymphoma, and leukemia during 1999–2008 in Korea. Int J Environ Res Public Health. (2017) 14:344. doi: 10.3390/ijerph14040344
151. Boice, JD, Mumma, MT, and Blot, WJ. Cancer incidence and mortality in populations living near uranium milling and mining operations in Grants, New Mexico, 1950–2004. rare. (2010) 174:624–36.
152. Henshaw, DL, Eatough, JP, and Richardson, RB. Radon as a causative factor in induction of myeloid leukaemia and other cancers. Lancet. (1990) 335:1008–12. doi: 10.1016/0140-6736(90)91071-H
153. Lucie, NP. Radon and acute lymphoblastic leukaemia. Leuk Lymphoma. (1990) 3:213–6. doi: 10.3109/10428199009050998
154. Zlobina, A, Farkhutdinov, I, Carvalho, FP, Wang, N, Korotchenko, T, Baranovskaya, N, et al. Impact of environmental radiation on the incidence of cancer and birth defects in regions with high natural radioactivity. Int J Environ Res Public Health. (2022) 19:8643. doi: 10.3390/ijerph19148643
155. Eatough, JP, and Henshaw, DL. Radon and monocytic leukaemia in England. J Epidemiol Community Health. (1993) 47:506–7.
156. Darby, S, Hill, D, Deo, H, Auvinen, A, Barros-Dios, JM, Baysson, H, et al. Residential radon and lung cancer—detailed results of a collaborative analysis of individual data on 7148 persons with lung cancer and 14 208 persons without lung cancer from 13 epidemiologic studies in Europe. Scand J Work Environ Health. (2006) 32:1–84.
157. Kang, JK, Seo, S, and Jin, YW. Health effects of radon exposure. Yonsei Med J. (2019) 60:597–603. doi: 10.3349/ymj.2019.60.7.597
158. Al-Zoughool, M, and Krewski, D. Health effects of radon: a review of the literature. Int J Radiat Biol. (2009) 85:57–69.
159. Kendall, GM, and Smith, TJ. Doses from radon and its decay products to children. J Radiol Prot. (2005) 25:241–56. doi: 10.1088/0952-4746/25/3/002
160. Heeran, AB, Berrigan, HP, and O’Sullivan, J. The radiation-induced bystander effect (RIBE) and its connections with the hallmarks of cancer. rare. (2019) 192:668–79. doi: 10.1667/RR15489.1
161. Popp, W, Plappert, U, Müller, WU, Rehn, B, Schneider, J, Braun, A, et al. Biomarkers of genetic damage and inflammation in blood and bronchoalveolar lavage fluid among former German uranium miners: a pilot study. Radiat Environ Biophys. (2000) 39:275–82. doi: 10.1007/s004110000072
162. Li, K, Chen, Y, Li, X, Lei, S, Chen, Q, Liu, J, et al. Alteration of cytokine profiles in uranium miners exposed to long-term low dose ionizing radiation. Sci World J. (2014) 2014:216408:1–5. doi: 10.1155/2014/216408
163. Allodji, RS, Leuraud, K, Thiébaut, ACM, Henry, S, Laurier, D, and Bénichou, J. Impact of measurement error in radon exposure on the estimated excess relative risk of lung cancer death in a simulated study based on the French uranium miners’ cohort. Radiat Environ Biophys. (2012) 51:151–63.
164. Richardson, DB, Rage, E, Demers, PA, Do, MT, Fenske, N, Deffner, V, et al. Lung cancer and radon: pooled analysis of uranium miners hired in 1960 or later. Environ Health Perspect. (2022) 130:57010. doi: 10.1289/EHP10669
165. Bray, F, Laversanne, M, Sung, H, Ferlay, J, Siegel, RL, Soerjomataram, I, et al. Global cancer statistics 2022: GLOBOCAN estimates of incidence and mortality worldwide for 36 cancers in 185 countries. CA Cancer J Clin. (2024) 74:229–63. doi: 10.3322/caac.21834
166. Chowdhury, R, Shah, D, and Payal, AR. Healthy worker effect phenomenon: revisited with emphasis on statistical methods – a review. Indian J Occup Environ Med. (2017) 21:2–8. doi: 10.4103/ijoem.IJOEM_53_16
167. United Nations. Scientific committee on the effects of atomic radiation. UNSCEAR 2019 report. Available at: https://www.unscear.org/unscear/en/publications/2019.html
Keywords: radon, occupational exposure, residential exposure, cancer, non-malignant disease, cardiovascular disease, neurodegenerative disease, meta-analysis
Citation: Henyoh AMS, Laurent O, Mandin C and Clero E (2024) Radon exposure and potential health effects other than lung cancer: a systematic review and meta-analysis. Front. Public Health. 12:1439355. doi: 10.3389/fpubh.2024.1439355
Edited by:
Sara Antignani, National Institute of Health (ISS), ItalyReviewed by:
Nezahat Hunter, Public Health England, United KingdomMiroslaw Janik, National Institutes for Quantum and Radiological Science and Technology, Japan
Copyright © 2024 Henyoh, Laurent, Mandin and Clero. This is an open-access article distributed under the terms of the Creative Commons Attribution License (CC BY). The use, distribution or reproduction in other forums is permitted, provided the original author(s) and the copyright owner(s) are credited and that the original publication in this journal is cited, in accordance with accepted academic practice. No use, distribution or reproduction is permitted which does not comply with these terms.
*Correspondence: Afi Mawulawoe Sylvie Henyoh, YWZpLmhlbnlvaEBpcnNuLmZy; Enora Clero, ZW5vcmEuY2xlcm9AaXJzbi5mcg==