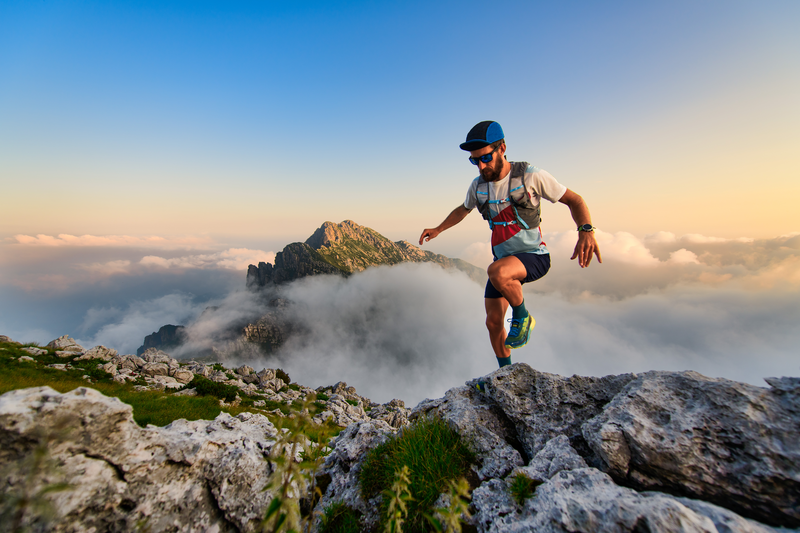
94% of researchers rate our articles as excellent or good
Learn more about the work of our research integrity team to safeguard the quality of each article we publish.
Find out more
OPINION article
Front. Public Health , 14 February 2024
Sec. Infectious Diseases: Epidemiology and Prevention
Volume 12 - 2024 | https://doi.org/10.3389/fpubh.2024.1366929
This article is part of the Research Topic Emerging and Re-emerging Viral Infections: Epidemiology, Pathogenesis and New Methods for Control and Prevention View all 31 articles
In the world of medical treatments, certain interventions carry hidden risks that are not always readily apparent. The use of equine serum in human therapies has raised substantial concerns, often overlooking, or minimizing the potential risks associated with these interventions. One prime example is the use of equine-derived antivenoms, crucial in treating venomous animal envenomations, such as those caused by snakes, scorpions, and spiders. A primary concern centers around the immunogenicity of equine serum components upon introduction into the human body. This can provoke immune responses ranging from mild allergic reactions to serum sickness and severe anaphylaxis, necessitating immediate medical intervention (1).
Likewise, equine serum-derived treatments may carry the risk of transmitting infections or diseases from the horse to the human recipient. The purification process involves pasteurizing horse IgGs. Typically, pasteurization occurs in the presence of stabilizers such as amino acids, sugars, or citrate to preserve protein functionality, preventing molecular changes and protein aggregation. These stabilizers also contribute to fortifying against viruses, underscoring the need to validate treatment conditions. Pasteurization can effectively deactivate a variety of viruses, both enveloped and non-enveloped (e.g., HIV, HBV, HCV, and HAV). However, there is limited data on the inactivation of resistant non-enveloped viruses like porcine parvovirus, SV 40, or reovirus type 3 in plasma products (2, 3). Thus, pasteurization has proven itself the only effective step toward assuring the virus safety of final product (4), although it is a process that do not have deliberately introduced viral inactivation, that could result in the parenteral transmission of zoonotic diseases. Caprylic acid treatment, formulation at acidic pH, and ion-exchange chromatography represent additional purification steps employed in the process. While these methods can effectively remove viruses from the serum, it's worth noting that they may not be as robust or comprehensive in their virus-removal capabilities (3, 5, 6).
Recent occurrences of zoonotic diseases serve as indicators of the interplay between humans and the reservoirs of biological agents harbored by animals (7). Moreover, these events underscore the inherent perils associated with the emergence of novel diseases such as the human immunodeficiency virus (HIV), Hantaan, Lassa, Ebola, Nipah, and a variety of paramyxoviruses. Furthermore, the list expands to encompass the equine morbilli virus, the West Nile virus, and notably, the strong likelihood of the inclusion of the severe acute respiratory syndrome coronavirus, including SARS-CoV-2 (8, 9). It is also important to highlight the actual endemic status of hepatitis E (HEV), which represents a significant public health concern. The transmission dynamics of this disease have been found to extend beyond conventional routes, potentially including blood transfusions as an important mode of spread (10).
Burnouf et al. (3) have put forward a list of 19 viruses, with the capacity to cause diseases in horses, and notably, 11 of these can also induce diseases in humans. The shared characteristics, encompassing attributes such as being enveloped or non-enveloped, DNA or RNA-based, etc., are depicted in Figure 1. Notably, Bornouf has proposed to include screening for at least these specific pathogens for sera derived from horses; however, these recommendations have not been implemented to date (3).
Figure 1. Main viruses identified in horses. Created with Biorender.com.
In fact, diseases like Eastern equine encephalitis (EEE), Western equine encephalitis (WEE), and Venezuelan-equine encephalitis (VEE) are highly infectious, spreading through aerosols. Venezuela and Colombia have seen continuous, fatal cases of VEE-induced encephalitis in horses and humans (11). On the other hand, Vesicular Stomatitis (VS), common among North American horses, poses zoonotic risks by causing encephalitis in children (12); while Hendra Virus (HeV) leads to respiratory and neurological diseases, fatal for humans and horses (13). West Nile fever has recently spread across new territories globally (14), being considered a epizootic emergence (15). Although rare in horses, rabies remains a grave public health concern (16, 17), in contrast with Equine Influenza, which apparently do not affect humans (18). Regarding SARS-CoV-2, although there is little evidence for horse natural infection (19), it was evidenced that a COVID-19 patient infected a horse, demonstrating in the horse the seroconversion following dayle contact during the development of clinical disease (20).
However, the diseases mentioned earlier are only the ones we're aware of; there could be numerous others being transmitted. In fact, it is estimated that 60% of emerging infectious diseases that are reported globally are zoonoses and over 30 new human pathogens have been detected in the last three decades, 75% of which have originated in animals (21).
This situation brings up a puzzling question: If we're not sure about the different diseases that could be in equine-derived serum, how can we know what illnesses might spread from it? Screening equine-derived serum for potential diseases with zoonotic implications may requires a multifaceted approach encompassing various techniques, including virus-specific PCR assays, serological tests, metagenomic sequencing, mass spectrometry, viral culture, next-generation sequencing (NGS), microarray analysis, immunofluorescence assays (IFA), proteomic analysis, nucleic acid amplification techniques (NAAT), cell culture-based assays, bioinformatics and computational analysis, lateral flow assays, digital PCR, and/or biosensors. As they are not applied so far, using serum from horses might not just bring new diseases to human but also create big outbreaks like the recent pandemic of COVID-19. This careless use of animal-based serums, such horses, is like “playing a risky game with public health”. Not being careful with these treatments ignores lessons from history and science.
We must not overlook the absence of transparency concerning these risks for patients and their families. Patients have the right to informed decision-making about their treatment choices, empowered by a thorough grasp of potential advantages and risks. And in this context, no information beyond the most common and rare side effects (i.e.,; allergies, serum sickness, fever, and anaphylaxis), that include the risks of contracting diseases, is described in the antivenom's label (22).
In the midst of the 21st century, with unprecedented achievements in science and pharmaceuticals, and with the glaring lessons imparted by the COVID-19 pandemic, one might wonder how we continue to endorse the archaic practice of using equine serum as a therapeutic option.
In the pursuit of alternatives to serum derived from horses, notable progress has been achieved, with a particularly promising pathway being the utilization of human monoclonal antibodies generated through phage display technology (23). The production of human monoclonal antibodies via phage display offers distinct advantages, including diminished immunogenicity and the capacity to customize antibodies for therapeutic applications (1). This methodology not only reduces dependence on equine serum but also provides a more individualized and human-centric solution, underscoring the transformative potential of cutting-edge technologies in advancing biotechnological alternatives (24). Currently, numerous researchers are actively exploring monoclonal antibodies and other alternatives to replace serum derived from horses (25–32).
While medical breakthroughs surge ahead, it is disconcerting that we overlook the pressing need to rigorously scrutinize the safety of treatments, particularly those produced by animal sources. Ignoring this matter not only dismisses the significant advancements in medical understanding but also puts the fundamental aspects of human health and wellbeing in danger.
MP: Writing—original draft, Writing—review & editing. AC: Writing—original draft, Writing—review & editing.
The author(s) declare financial support was received for the research, authorship, and/or publication of this article. This work was supported by Center for Contemporary Equine Studies, Woodside, CA, USA.
The authors declare that the research was conducted in the absence of any commercial or financial relationships that could be construed as a potential conflict of interest.
The author(s) declared that they were an editorial board member of Frontiers, at the time of submission. This had no impact on the peer review process and the final decision.
All claims expressed in this article are solely those of the authors and do not necessarily represent those of their affiliated organizations, or those of the publisher, the editors and the reviewers. Any product that may be evaluated in this article, or claim that may be made by its manufacturer, is not guaranteed or endorsed by the publisher.
1. Laustsen AH, María Gutiérrez J, Knudsen C, Johansen KH, Bermúdez-Méndez E, Cerni FA, et al. Pros and cons of different therapeutic antibody formats for recombinant antivenom development. Toxicon. (2018) 146:151–75. doi: 10.1016/j.toxicon.2018.03.004
2. Burnouf-Radosevich M, Burnouf T, Huart JJ. A pasteurized therapeutic plasma. Infusionsther Transfusionsmed. (1992) 19:91–4. doi: 10.1159/000222590
3. Burnouf T, Griffiths E, Padilla A, Seddik S, Stephano MA, Gutiérrez J-M. Assessment of the viral safety of antivenoms fractionated from equine plasma. Biologicals. (2004) 32:115–28. doi: 10.1016/j.biologicals.2004.07.001
4. Gröner A, Broumis C, Fang R, Nowak T, Popp B, Schäfer W, et al. Effective inactivation of a wide range of viruses by pasteurization. Transfusion. (2018) 58:41–51. doi: 10.1111/trf.14390
5. Horowitz B, Piët MP, Prince AM, Edwards CA, Lippin A, Walakovits LA. Inactivation of lipid-enveloped viruses in labile blood derivatives by unsaturated fatty acids. Vox Sang. (1988) 54:14–20. doi: 10.1159/000461754
6. Burnouf T, Radosevich M. Affinity chromatography in the industrial purification of plasma proteins for therapeutic use. J Biochem Biophys Methods. (2001) 49:575–86. doi: 10.1016/S0165-022X(01)00221-4
7. Ivan A, Indrei LL. Emergence of transmissible disorders, a continuous process-a new type of viral meningoencephalitis. Rev Med Chir Soc Med Nat Iasi. (2000) 104:51–5.
8. Jo WK, de Oliveira-Filho EF, Rasche A, Greenwood AD, Osterrieder K, Drexler JF. Potential zoonotic sources of SARS-CoV-2 infections. Transbound Emerg Dis. (2021) 68:1824–34. doi: 10.1111/tbed.13872
9. Kaaden OR, Eichhorn W, Essbauer S. Recent developments in the epidemiology of virus diseases. J Vet Med B Infect Dis Vet Public Health. (2002) 49:3–6. doi: 10.1046/j.1439-0450.2002.00530.x
10. Arankalle VA, Chobe LP. Retrospective analysis of blood transfusion recipients: evidence for post-transfusion hepatitis E. Vox Sang. (2000) 79:72–4. doi: 10.1046/j.1423-0410.2000.7920072.x
11. Roy CJ, Reed DS, Wilhelmsen CL, Hartings J, Norris S, Steele KE. Pathogenesis of aerosolized Eastern Equine Encephalitis virus infection in guinea pigs. Virol J. (2009) 6:170. doi: 10.1186/1743-422X-6-170
12. Monath TP, Emerson JK, Kemp GE, Poland JD, Cholas G, Webb PA, et al. Epizootic vesicular stomatitis in Colorado, 1982: infection in occupational risk groups. Am J Trop Med Hyg. (1987) 36:177–82. doi: 10.4269/ajtmh.1987.36.177
13. Epstein JH, Baker ML, Zambrana-Torrelio C, Middleton D, Barr JA, DuBovi E, et al. Duration of maternal antibodies against canine distemper virus and hendra virus in pteropid bats. PLoS ONE. (2013) 8:e67584. doi: 10.1371/journal.pone.0067584
14. Chancey C, Grinev A, Volkova E, Rios M. The global ecology and epidemiology of West Nile Virus. Biomed Res Int. (2015) 2015:1–20. doi: 10.1155/2015/376230
15. Ziegler U, Santos PD, Groschup MH, Hattendorf C, Eiden M, Höper D, et al. West Nile virus epidemic in Germany triggered by epizootic emergence, 2019. Viruses. (2020) 12:448. doi: 10.3390/v12040448
16. Araujo FAA. Raiva Humana no Brasil, 1992 - 2001. (2002). Available online at: https://repositorio.ufmg.br/handle/1843/BUOS-8BWGGJ (accessed December 22, 2023).
17. Green SL, Smith LL, Vernau W, Beacock SM. Rabies in horses: 21 cases (1970-1990). J Am Vet Med Assoc. (1992) 200:1133–7. doi: 10.2460/javma.1992.200.08.1133
18. Kumar B, Manuja A, Gulati B, Virmani N, Tripathi BN. Zoonotic viral diseases of equines and their impact on human and animal health. Open Virol J. (2018) 12:80–98. doi: 10.2174/1874357901812010080
19. Legere RM, Allegro AR, Affram Y, da Silveira BP, Fridley JL, Wells KM, et al. Equine bronchial epithelial cells are susceptible to cell entry with a SARS-CoV-2 pseudovirus but reveal low replication efficiency. Am J Vet Res. (2023) 84:ajvr.23.06.0132. doi: 10.2460/ajvr.23.06.0132
20. Pusterla N, Chaillon A, Ignacio C, Smith DM, Barnum S, Lawton KOY, et al. SARS-CoV-2 seroconversion in an adult horse with direct contact to a COVID-19 individual. Viruses. (2022) 14:1047. doi: 10.3390/v14051047
21. Jones KE, Patel NG, Levy MA, Storeygard A, Balk D, Gittleman JL, et al. Global trends in emerging infectious diseases. Nature. (2008) 451:990–3. doi: 10.1038/nature06536
22. Butantan. Portal do Butantan. Soros e Bulas. (2023). Available online at: https://butantan.gov.br/soros-e-vacinas/soros (accessed August 31, 2023).
23. McCafferty J, Griffiths AD, Winter G, Chiswell DJ. Phage antibodies: filamentous phage displaying antibody variable domains. Nature. (1990) 348:552–4. doi: 10.1038/348552a0
24. Camphora A, Pucca M. Horses used for large-scale production of immunoglobulins: an inter-species approach. Int Anim Health J. (2022) 8:12–6. Available online at: https://international-animalhealth.com/horses-used-for-large-scale-production-of-immunoglobulins-an-inter-species-approach/
25. Pucca MB, Cerni FA, Peigneur S, Arantes EC, Tytgat J, Barbosa JE. Serrumab: a novel human single chain-fragment antibody with multiple scorpion toxin-neutralizing capacities. J Immunotoxicol. (2014) 11:133–40. doi: 10.3109/1547691X.2013.809175
26. Ahmadi S, Pucca MB, Jürgensen JA, Janke R, Ledsgaard L, Schoof EM, et al. An in vitro methodology for discovering broadly-neutralizing monoclonal antibodies. Sci Rep. (2020) 10:10765. doi: 10.1038/s41598-020-67654-7
27. Knudsen C, Ledsgaard L, Dehli RI, Ahmadi S, Sørensen CV, Laustsen AH. Engineering and design considerations for next-generation snakebite antivenoms. Toxicon. (2019) 167:67–75. doi: 10.1016/j.toxicon.2019.06.005
28. Sørensen CV, Fernández J, Adams AC, Wildenauer HHK, Schoffelen S, Ledsgaard L, et al. Antibody-dependent enhancement of toxicity of myotoxin II from Bothrops asper. Nat Commun. (2024) 15:173. doi: 10.1038/s41467-023-42624-5
29. Føns S, Ledsgaard L, Nikolaev MV, Vassilevski AA, Sørensen CV, Chevalier MK, et al. Discovery of a recombinant human monoclonal immunoglobulin G antibody against α-latrotoxin from the mediterranean Black Widow Spider (Latrodectus tredecimguttatus). Front Immunol. (2020) 11:587825. doi: 10.3389/fimmu.2020.587825
30. Ledsgaard L, Wade J, Jenkins TP, Boddum K, Oganesyan I, Harrison JA, et al. Discovery and optimization of a broadly-neutralizing human monoclonal antibody against long-chain α-neurotoxins from snakes. Nat Commun. (2023) 14:682. doi: 10.1038/s41467-023-36393-4
31. Campos LB, Pucca MB, Silva LC, Pessenda G, Filardi BA, Cerni FA, et al. Identification of cross-reactive human single-chain variable fragments against phospholipases A2 from Lachesis muta and Bothrops spp venoms. Toxicon. (2020) 184:116–21. doi: 10.1016/j.toxicon.2020.05.027
Keywords: infectious diseases, viral transmission, pandemic risks, infection control measures, health crisis, heterologous serum therapy
Citation: Pucca MB and Camphora AL (2024) The potential risks of equine serum therapy in transmitting new infectious diseases: lessons from a post-pandemic era. Front. Public Health 12:1366929. doi: 10.3389/fpubh.2024.1366929
Received: 07 January 2024; Accepted: 05 February 2024;
Published: 14 February 2024.
Edited by:
Manel Ben M'Hadheb, University of Monastir, TunisiaReviewed by:
Carlos Alberto M. Carvalho, Universidade do Estado do Pará, BrazilCopyright © 2024 Pucca and Camphora. This is an open-access article distributed under the terms of the Creative Commons Attribution License (CC BY). The use, distribution or reproduction in other forums is permitted, provided the original author(s) and the copyright owner(s) are credited and that the original publication in this journal is cited, in accordance with accepted academic practice. No use, distribution or reproduction is permitted which does not comply with these terms.
*Correspondence: Manuela B. Pucca, bWFudWVsYS5wdWNjYUB1bmVzcC5icg==
Disclaimer: All claims expressed in this article are solely those of the authors and do not necessarily represent those of their affiliated organizations, or those of the publisher, the editors and the reviewers. Any product that may be evaluated in this article or claim that may be made by its manufacturer is not guaranteed or endorsed by the publisher.
Research integrity at Frontiers
Learn more about the work of our research integrity team to safeguard the quality of each article we publish.