- 1National Institute for Communicable Disease Control and Prevention, Chinese Center for Disease Control and Prevention, National Key Laboratory of Intelligent Tracking and Forecasting for Infectious Diseases, Beijing, China
- 2Affiliated Hospital of North China University of Science and Technology, Tangshan, China
Ticks are one of the most important vectors that can transmit pathogens to animals and human beings. This study investigated the dominant tick-borne bacteria carried by ticks and tick-borne infections in forestry populations in Arxan, Inner Mongolia, China. Ticks were collected by flagging from May 2020 to May 2021, and blood samples were collected from individuals at high risk of acquiring tick-borne diseases from March 2022 to August 2023. The pooled DNA samples of ticks were analyzed to reveal the presence of tick-borne bacteria using high-throughput sequencing of the 16S rDNA V3–V4 region, and species-specific polymerase chain reaction (PCR) related to sequencing was performed to confirm the presence of pathogenic bacteria in individual ticks and human blood samples. All sera samples were examined for anti-SFGR using ELISA and anti-B. burgdorferi using IFA and WB. A total of 295 ticks (282 Ixodes persulcatus and 13 Dermacentor silvarum) and 245 human blood samples were collected. Rickettsia, Anaplasma, Borrelia miyamotoi, and Coxiella endosymbiont were identified in I. persulcatus by high-throughput sequencing, while Candidatus R. tarasevichiae (89.00%, 89/100), B. garinii (17.00%, 17/100), B. afzelii (7.00%, 7/100), and B. miyamotoi (7.00%, 7/100) were detected in I. persulcatus, as well the dual co-infection with Candidatus R. tarasevichiae and B. garinii were detected in 13.00% (13/100) of I. persulcatus. Of the 245 individuals, B. garinii (4.90%, 12/245), R. slovaca (0.82%, 2/245), and C. burnetii (0.41%, 1/245) were detected by PCR, and the sequences of the target genes of B. garinii detected in humans were identical to those detected in I. persulcatus. The seroprevalence of anti-SFGR and anti-B. burgdorferi was 5.71% and 13.47%, respectively. This study demonstrated that Candidatus R. tarasevichiae and B. garinii were the dominant tick-borne bacteria in I. persulcatus from Arxan, and that dual co-infection with Candidatus R. tarasevichiae and B. garinii was frequent. This is the first time that B. miyamotoi has been identified in ticks from Arxan and R. solvaca has been detected in humans from Inner Mongolia. More importantly, this study demonstrated the transmission of B. garinii from ticks to humans in Arxan, suggesting that long-term monitoring of tick-borne pathogens in ticks and humans is important for the prevention and control of tick-borne diseases.
Introduction
Ticks are obligate hematophagous ectoparasites of terrestrial vertebrates that can transmit various pathogens to humans and are considered to be the second most notable vectors worldwide after mosquitoes (1). By the end of 2018, 103 tick-borne pathogens were detected in China, of which 65 were newly identified in the past two decades (2). Notable bacteria such as Rickettsia, Borrelia, Coxiella, Anaplasma, and Ehrlichia (2–4), have been detected in a wide range of tick species that can cause spread of relevant zoonotic diseases. Annually expanding geographic ranges and increasing populations of ticks, partly driven by climate change, demographic growth, and increased overseas travel and trade, have become serious threats to public health (5, 6). However, systematic and comprehensive investigations of tick-borne pathogens have not been conducted in tick habitats in China because of the limitations of investigation regions and research technology.
Inner Mongolia Autonomous Region, located in northern China, is well-known for its various landscapes and is one of the natural foci of various tick-borne diseases in China (7–10). Arxan, on the border between China and Mongolia in the northeastern Inner Mongolia, features abundant wildlife resources and a developed livestock industry that provides a suitable environment for ticks to survive and reproduce. Trade activities at border crossings and tourism have partly increased the risk of tick-borne diseases. For instance, the average annual incidence of tick-borne encephalitis (TBE) in Arxan from 2006 to 2013 was 188.48/1000000, indicating that Arxan has the highest incidence of TBE in China (11). However, tick-borne bacteria in Arxan have not been studied in recent years. Therefore, investigating the status of bacterial infections in ticks and humans plays an important role in the prevention and control of tick-borne diseases in Arxan.
In this study, we performed high-throughput sequencing of the 16S rDNA V3–V4 region to detect tick-borne bacteria carried by ticks, and species-specific polymerase chain reaction (PCR) was performed to confirm the presence of pathogenic bacteria in ticks and participants at a high risk of tick-borne diseases in Arxan. Furthermore, antibodies against dominant tick-borne bacteria were detected using Enzyme-Linked Immunosorbent Assay (ELISA), Indirect Immunofluorescence Assay (IFA), and Western Blotting (WB) assays, providing complete and comprehensive monitoring of tick-borne bacteria and a theoretical basis for the prevention and control of tick-borne diseases.
Materials and methods
Collection and identification of ticks
Free-living ticks were collected from ground vegetation by dragging white cloth in May 2020 and 2021 in Arxan, Inner Mongolia, China (Figure 1). All collected ticks were placed in breathable and moistened bottles and transported to the laboratory as soon as possible at room temperature (20–25°C). Tick species were identified based on morphological characterization and verified using sequences of mitochondrial 16S rDNA genes, as previously described (12). After identification, the ticks were stored at −80°C until use.
Collection of human blood samples
Patients with tick-borne diseases mostly work in the field, including as forestry workers (13–15). These workers had a high possibility of tick exposure and therefore, were defined as groups at high risk of acquiring tick-borne diseases. We simultaneously collected anticoagulated (EDTA blood) and non-anticoagulant blood samples from 245 participants (232 males and 13 females) working in the field from March 2022 to August 2023. The workplaces of these participants were Tianchi forest farm (8.57%, 21/245), Sandur forest farm (15.92%, 39/245), Lixin forest farm (16.33%, 40/245), Irsh forest farm (28.16%, 69/245), Xing’an Service Area (8.98%, 22/245), Jinjianggou Service Area (11.84%, 29/245) and Transportation Company (10.20%, 25/245), respectively. The median age of all participants was 53.00 years (range 23–60 years). All the participants provided written informed consent to participate in this study. Sera were isolated from non-anticoagulant blood samples and stored at −20°C for serological tests. Anticoagulated blood samples were used for DNA extraction.
DNA extraction
All ticks were washed with 75% ethanol and rinsed thrice with sterile water to remove environmental contaminants. Except for the 182 I. persulcatus samples used for the isolation and culture of B. burgdorferi, the remaining 100 I. persulcatus and 13 D. silvarum samples were used for DNA extraction. Individual tick samples were homogenized in 180 μL buffer ATL, then genomic DNA was extracted using the DNeasy Blood & Tissue Kit (QIAGEN, Germany) according to the manufacturer’s instructions. Similarly, the genomic DNA of human blood samples was extracted from anticoagulated blood using the DNeasy Blood & Tissue Kit (QIAGEN, Germany). Each time DNA extraction was performed, an extraction control (water) was added. All DNA samples from both ticks and human blood were stored at −80°C until use.
High-throughput sequencing
Individual DNA samples of ticks were mixed in an equal volume (10 μL) to prepare pooled DNA samples (3 or 4 DNA samples for a pool). One hundred I. persulcatus DNA samples were mixed to prepare 32 pools (N1–N24 and N29–N36), and 13 D. silvarum DNA samples were mixed for 4 pools (N25–N28). All pooled DNA samples were sent to Biomarker Technologies Co., Ltd. (Beijing, China) for high-throughput sequencing of the 16S rDNA V3–V4 region on an Illumina NovaSeq 6,000 platform (Illumina, San Diego, CA, United States) with a sequencing depth of 80,000. The bioinformatics analysis of raw data was performed with the aid of the BMK Cloud (Biomarker Technologies Co., Ltd., Beijing, China). Raw data were filtered according to the quality of a single nucleotide using Trimmomatic (version 0.33) (16). Primer sequences were identified and removed using Cutadapt (version 1.9.1) (17). Clean reads obtained from the previous steps were assembled using USEARCH (version 10) (18) and followed by chimera removal using UCHIME (version 8.1) (19). Effective reads were generated and used for subsequent analyses. Sequences with similarity ≥97% were clustered into the same operational taxonomic unit (OTU) by USEARCH (version 10). Taxonomic annotation of the OTUs was performed based on the Naive Bayes classifier in QIIME2 (20) using the SILVA database (release 132) (19), following which a relative abundance table and histogram for each sample at the level of the phylum, class, order, family, genus, and species were calculated and displayed. Alpha diversity, including Chao1, ACE, Shannon, and Simpson indices, were calculated and displayed using QIIME2 and R software.
Polymerase chain reaction
According to the results of high-throughput sequencing and tick-borne bacteria prevalently detected in Inner Mongolia, the presence of Borrelia burgdorferi, B. miyamotoi, Anaplasma phagocytophilum, spotted fever group rickettsia, Ehrlichia chaffeensis, and Coxiella burnetii were tested in ticks and human blood samples using nested PCR or traditional PCR. The PCR primer sequences are listed in Table 1. PCR was performed using a PCR system (SensoQuest, Germany). The PCR system was totally 25 μL, including 12.5 μL Premix Taq™ (TaKaRa), 1 μL upper primer (10 μm), 1 μL lower primer (10 μm), 5 μL each individual DNA sample and 5.5 μL ddH2O. Positive (recombinant plasmid containing the target gene), negative (water), and extraction controls were used for PCR amplification in each PCR experiment. For nested PCR, 1 μL of the primary PCR production was used as the template for the second round. All amplified products were electrophoresed on a 1.5% agarose gel and the positive products were purified and sequenced. The acquired nucleotide sequences were submitted to National Center for Biotechnology Information Genbank and compared with available sequences deposited using the Basic Local Assignment Search Tool.1 Additionally, human blood samples that were positive for SFGR based on nested PCR were tested using qPCR targeting gltA gene. qPCR was performed using a Probe qPCR mix (Premix Ex Taq™,TaKaRa) on a LightCycler 480 System (Roche Diagnostics, United States).
Phylogenetic analysis
Reference sequences of target genes downloaded from GenBank were aligned with the positive amplicon sequences using the ClustalW method with default parameters in MEGA11.0. One of the same nucleotide sequences detected in a tick or blood sample was selected as the representative sequence. Phylogenetic analysis was performed using the neighbor-joining algorithm, and bootstrap values were set for 1,000 replicates.
Serological tests
Based on the results of the high-throughput sequencing and PCR assays, SFGR and B. burgdorferi were the dominant tick-borne bacteria in Arxan. All serum samples were tested anti-SFGR by ELISA and anti-B. burgdorferi using IFA and WB. ELISA kits acquired from Enzyme Immune Industrial Co., Ltd. (Jiangsu, China) were used to detect anti-SFGR IgG and IgM according to the manufacturer’s instructions. The cutoff value was the mean optical density (OD) of the negative control wells plus 0.15. Samples with OD values less than the cut-off value were considered negative. Samples with OD values greater than or equal to the cut-off value were considered positive.
IFA and WB were performed as previously described (29). First, all the serum samples were tested for anti-B. burgdorferi by IFA. A titer of ≥1/128 for IgG or 1:64 for IgM was considered positive. For IgG, samples that were positive at a titer of 1/64 but presented a weak fluorescence signal at a titer of 1/128 were considered equivocal. For IgM, samples that were positive at a titer of 1/32 but presented a weak fluorescence signal at a titer of 1/64 were considered equivocal. Subsequently, all sera with positive and equivocal (IgG or IgM) results were further analyzed by WB (both IgG and IgM). The positive criteria were at least one band of P83/100, P66, P58, P39, P30, OspC, OspA, and P17 in the IgG test and at least one band of P83/100, P58, P41, OspA, P30, OspC, and P17 in the IgM test (30).
Statistical analysis
Statistical analysis was performed using Pearson’s χ2 test and p < 0.05 was considered significant.
Results
Identification of ticks
A total of 295 adult hard ticks were identified as Ixodes persulcatus (n = 282) and Dermacentor silvarum (n = 13) based on morphological characteristics and mitochondrial 16S rDNA sequences. The nucleotide sequence data of mitochondrial 16S rDNA in this study will appear in GenBank under the following accession numbers: OR841358–OR841362 for I. persulcatus, OR841363–OR841367 for D. silvarum.
The overview of high-throughput sequencing
A total of 2,413,890 effective reads (range 69,960–76,736 reads, average 75434.06 reads) of high quality (Q20 > 98.94%, shown in Additional file 1: Supplementary Table S1) were obtained from 32 pooled I. persulcatus samples. Subsequently, the effective reads were clustered into 1,445 Operational Taxonomic Units (OTU), which belonged to 32 phyla, 79 classes, 191 orders, 348 families, and 594 genera. Moreover, 303,375 effective reads were acquired from four pooled DNA samples of D. silvarum (range 75,414–76,021 reads, average 75843.75 reads), all of which were of high quality (Q20 > 98.93%, shown in Additional file 1: Supplementary Table S1), and 1,430 OTUs were generated, belonging to 31 phyla, 78 classes, 190 orders, 346 families, and 588 genera.
Alpha diversity reflects species richness and diversity of individual samples. The Ace and Chao1 indices, which were calculated to measure species richness, were more than 1068.34 and 1098.66, respectively, in each pool (Additional file 2: Supplementary Table S2). Shannon and Simpson indices were used to measure species diversity, which were affected by species abundance and community evenness in the sample community. With the same species abundance, the greater the uniformity of each species in the community, the larger the Shannon and Simpson indices values, indicating higher species diversity in the sample. Shannon diversity rarefaction (Figure 2) reflects the microbial diversity of each sample at different sequencing amounts, and was used to determine whether the sequencing depth was sufficient. All pools were highly diverse, with Simpson indices more than 0.85 except for N23. The species accumulation curve reflects the relationship between the sample size and the number of annotated species, and can be used to determine whether the sample size was sufficient. The species accumulation curve of I. persulcatus at the genus level is shown in Figure 3. The number of D. silvarum pooled DNA samples was insufficient for the analysis of the species cumulative curve.
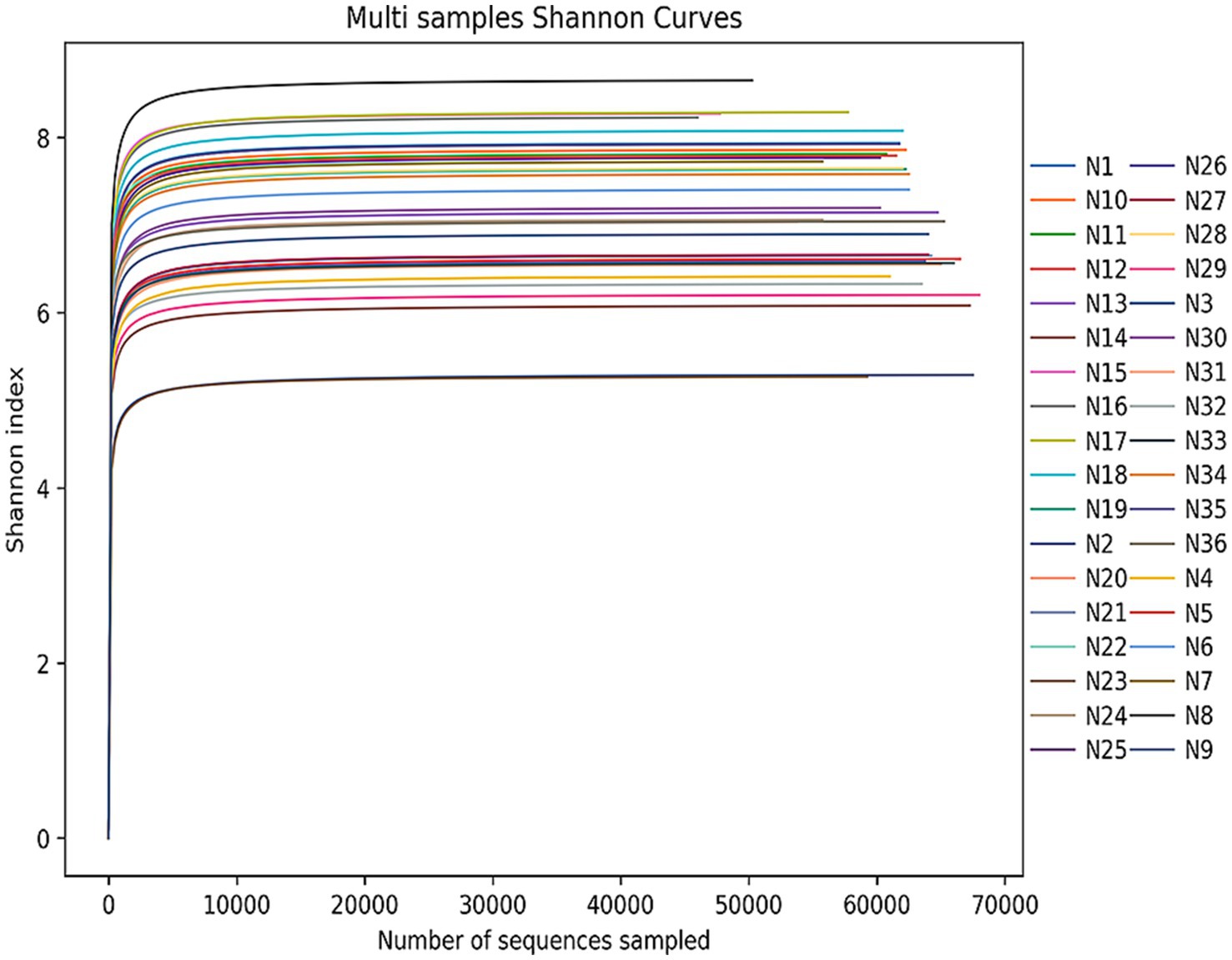
Figure 2. Shannon index rarefaction curve of 36 pools of I. persulcatus and D. silvarum. When the curve tends to be flat, it means that the sequencing data are sufficient for analysis, and the Shannon index will not increase with increasing sequencing data.
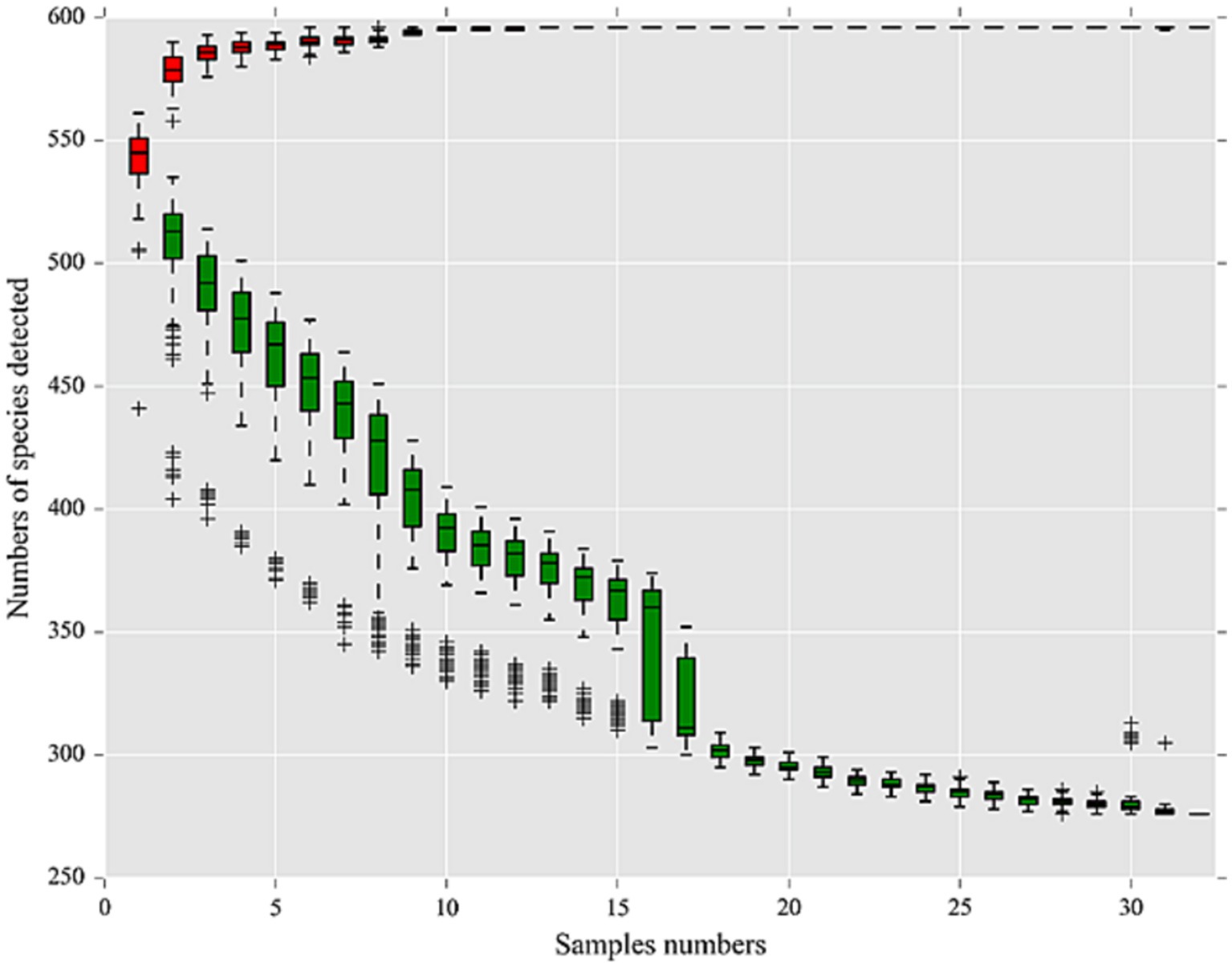
Figure 3. Species accumulation curve at genus level of 32 pools of I. persulcatus. Red boxes represent the accumulation curve of detected species numbers, and green boxes form the common curve of detected species numbers. When the curve tends to be flat, it means that the sample size is sufficient for the analysis.
Species annotation and taxonomic analysis
Taxonomic assignment revealed that the relative abundances of the genera were highly variable in the microbiome of I. persulcatus (Figure 4). At least 594 genera were presented in I. persulcatus, including Rickettsia, Acinetobacter, Methylotenera, Methylomonas, Psychrobacter. Rickettsia was the most common genus, with a relative abundance >22.61% in the seven pools (Additional file 3: Supplementary Table S3). Borrelia, with a relative abundance of 0.15–0.35%, was detected in three pools and further identified as B. miyamotoi. Likewise, Coxiella (0.01–0.02%) was identified in five pools and further confirmed to be Coxiella endosymbiont. Anaplasma (0.01%) was present in two pools, but species-level annotation was lacking (Additional file 4, Supplementary Table S4).
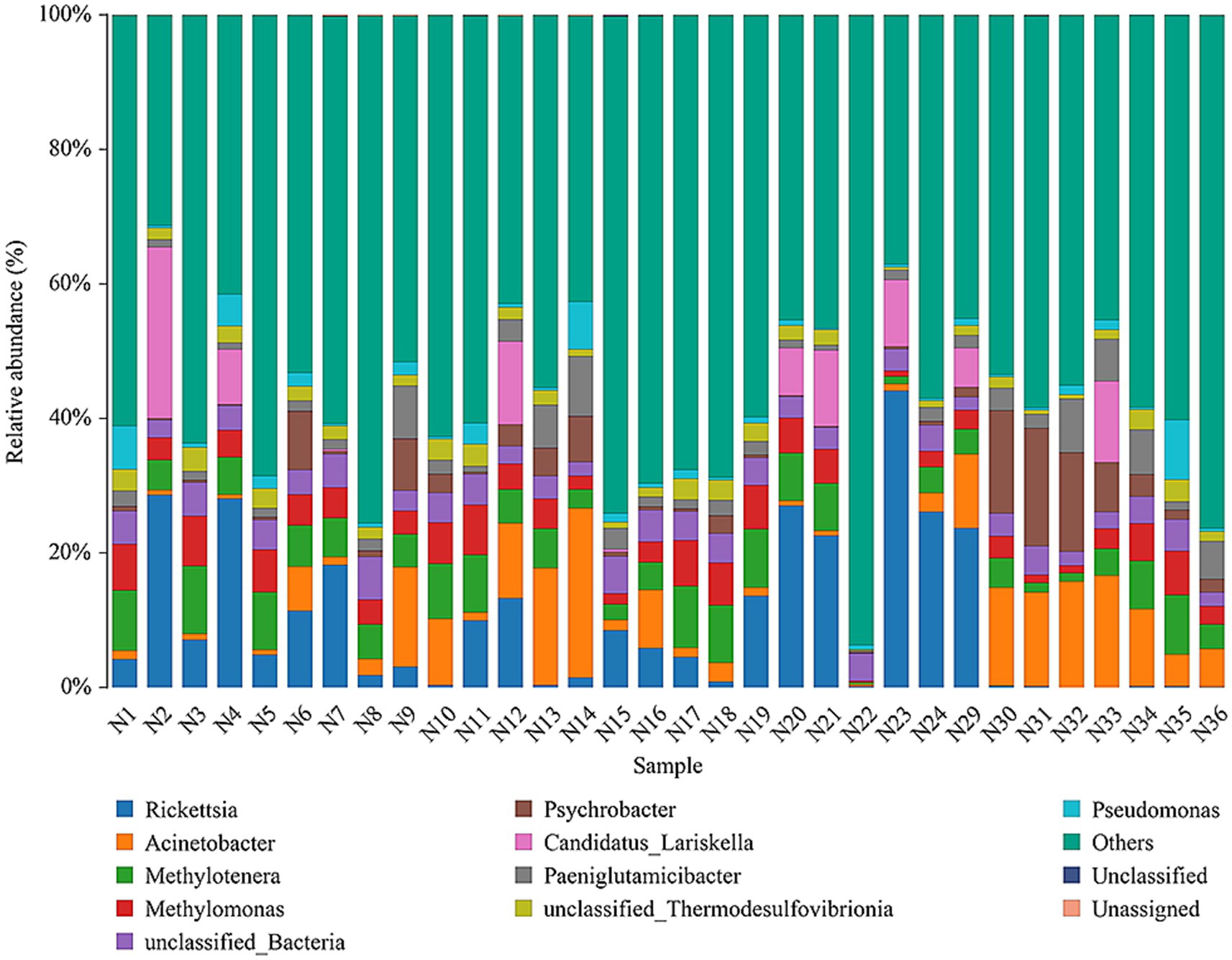
Figure 4. Relative abundances of top 10 microorganisms at the genus level in pooled I. persulcatus DNA samples.
Similarly, Rickettsia was the most abundant genus in D. silvarum (Additional file 3: Supplementary Table S3). Coxiella was identified in each D. silvarum pool and confirmed to be Coxiella endosymbiont at the species level (Additional file 4: Supplementary Table S4), whereas Borrelia was not detected in D. silvarum.
Prevalence of tick-borne bacteria in ticks and humans
Candidatus R. tarasevichiae (89.00%, 89/100), B. garinii (17.00%, 17/100), B. afzelii (7.00%, 7/100), and B. miyamotoi (7.00%, 7/100) were detected in I. persulcatus, while R. raoultii (69.23%, 9/13) was detected in D. silvarum (Table 2). More importantly, B. garinii (4.90%, 12/245), R. slovaca (0.82%, 2/245), and C. burnetii (0.41%, 1/245) were detected in the human blood samples. Neither Ehrlichia DNA nor Anaplasma DNA was detected in ticks or human blood samples. The qPCR results showed a cycle threshold value of 38.86 for one of those 2 individuals that were detected positive for R. slovaca using nested PCR targeting ompA gene.
Phylogenetic analysis
Phylogenetic tree based on ompA gene sequences of SFGR was shown in Figure 5. Of the 72 I. persulcatus positive for Candidatus R. tarasevichiae, all PCR amplicon sequences were identical (NMG-R1) and placed in a clade with Candidatus R. tarasevichiae from China (MN450410.2, MK576116.1). Two amplicon sequences (NMG-R102 and NMG-R105) detected in D. silvarum were closely related to strain Khabarovsk (AH015610.2, type strain of R. raoultii), and R. raoultii from China (KY474577.1 and MF511260.1). In particular, R. slovaca was detected in two individuals; both sequences were identical (H-R16) and clustered with strain 13-B (U43808.1, type strain of R. slovaca), and R. slovaca from China (MF002534.1), Russia (MT511330.1). In the phylogenetic tree based on gltA gene (Figure 6), 89 sequences amplified from I. persulcatus were identical (NMG-G1) and placed in a clade with Candidatus R. tarasevichiae from China (MN450396.2, JX996054.1). Two amplicon sequences (NMG-G102 and NMG-G105) detected in D. silvarum belonged to the same branch as R. raoultii from China (MF511250.1).
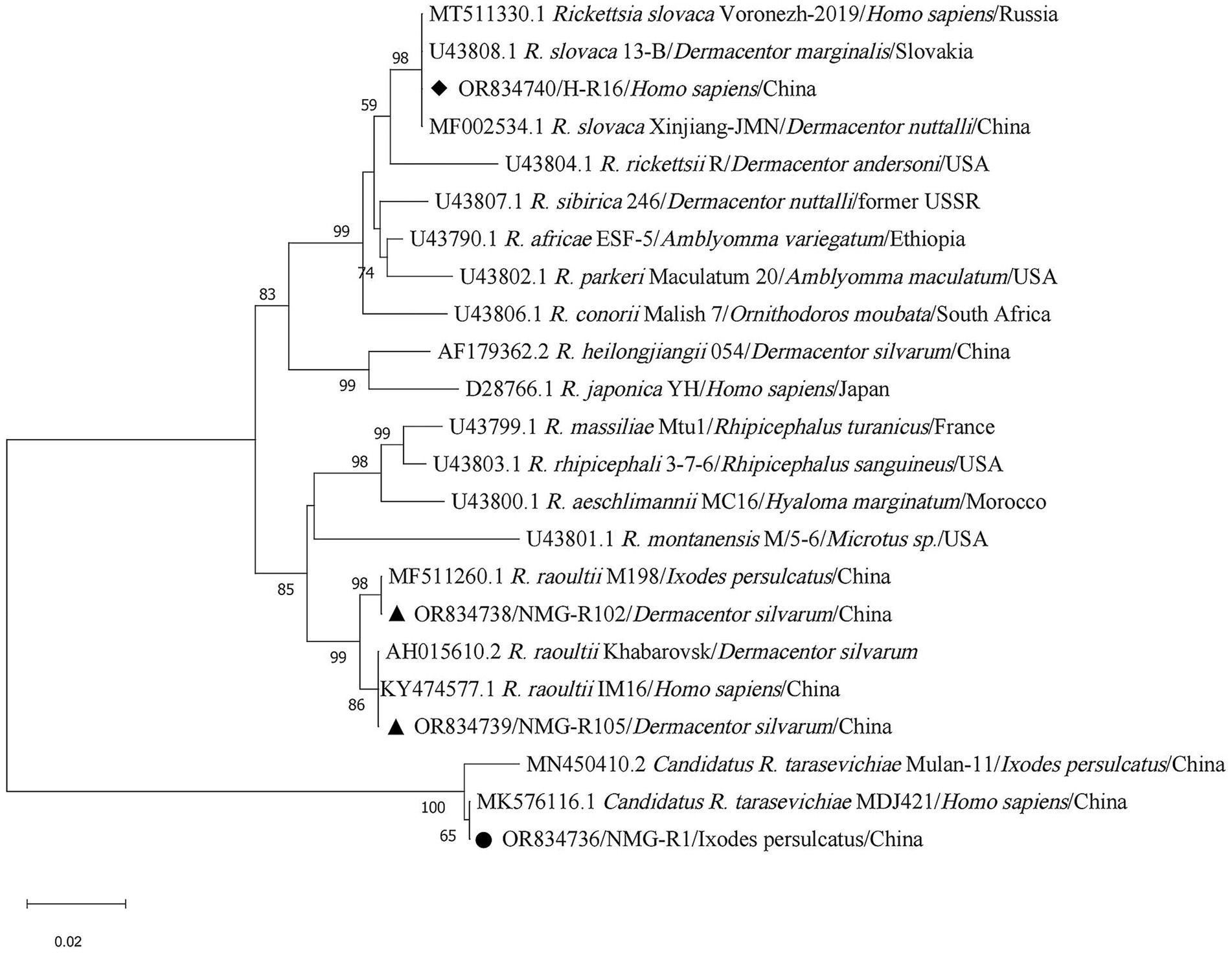
Figure 5. Phylogenetic analysis for ompA gene of SFGR. The sequences clustered as Candidatus R. tarasevichiae were marked as “●,” R. raoultii were marked as “▲,” R. slovaca were marked as “◆.”
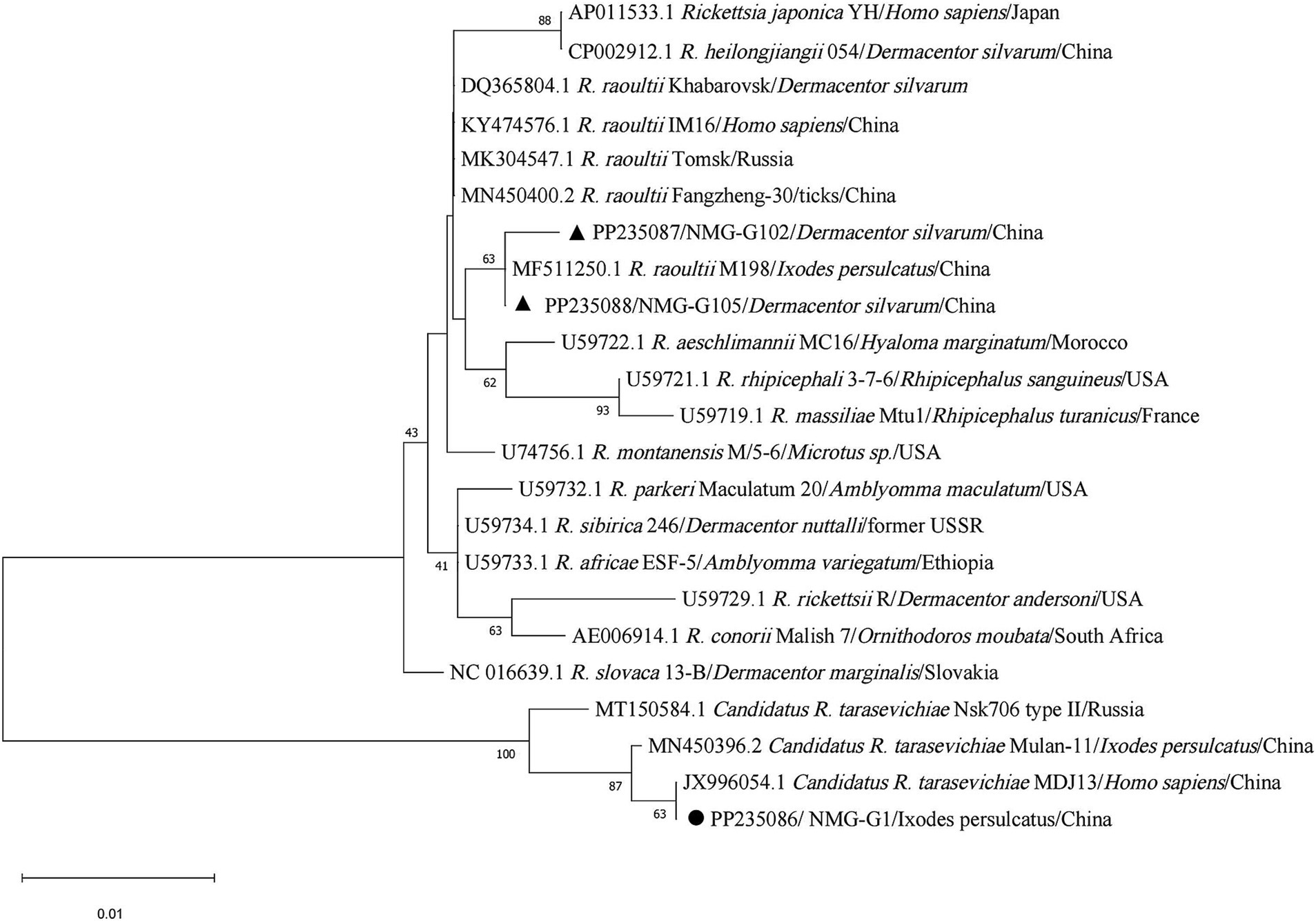
Figure 6. Phylogenetic analysis for gltA gene of SFGR. The sequences clustered as Candidatus R. tarasevichiae were marked as “●,” R. raoultii were marked as “▲.”
Seventeen I. persulcatus were positive for B. garinii. After removing duplicate sequences, the remaining 12 sequences were used to build the phylogenetic tree of B. burgdorferi. The 11 sequences were found to be closely related to strain 20047 (CP028861.1, type strain of B. garinii), B. garinii from China (JX888456.1, DQ156524.1, and HQ434240.1), and Russia (AM748051.1), another sequence (NMG-B49) was clustered with B. garinii (KY273110.1) from France. Similarly, 12 human blood samples were identified as positive for B. garinii, and all sequences were identical to each other (H-B1) and matched 100% with the sequences (NMG-B61) from I. persulcatus and B. garinii strain PD91 (HQ434240.1). We detected B. afzelii in seven I. persulcatus samples; two sequences (NMG-B12 and NMG-B50) fell into the same branch as strain VS461 (L30135.1, type strain of B. afzelii), B. afzelii from China (HQ434325.1) and Germany (MW489229.1) after the elimination of equal sequences (Figure 7).
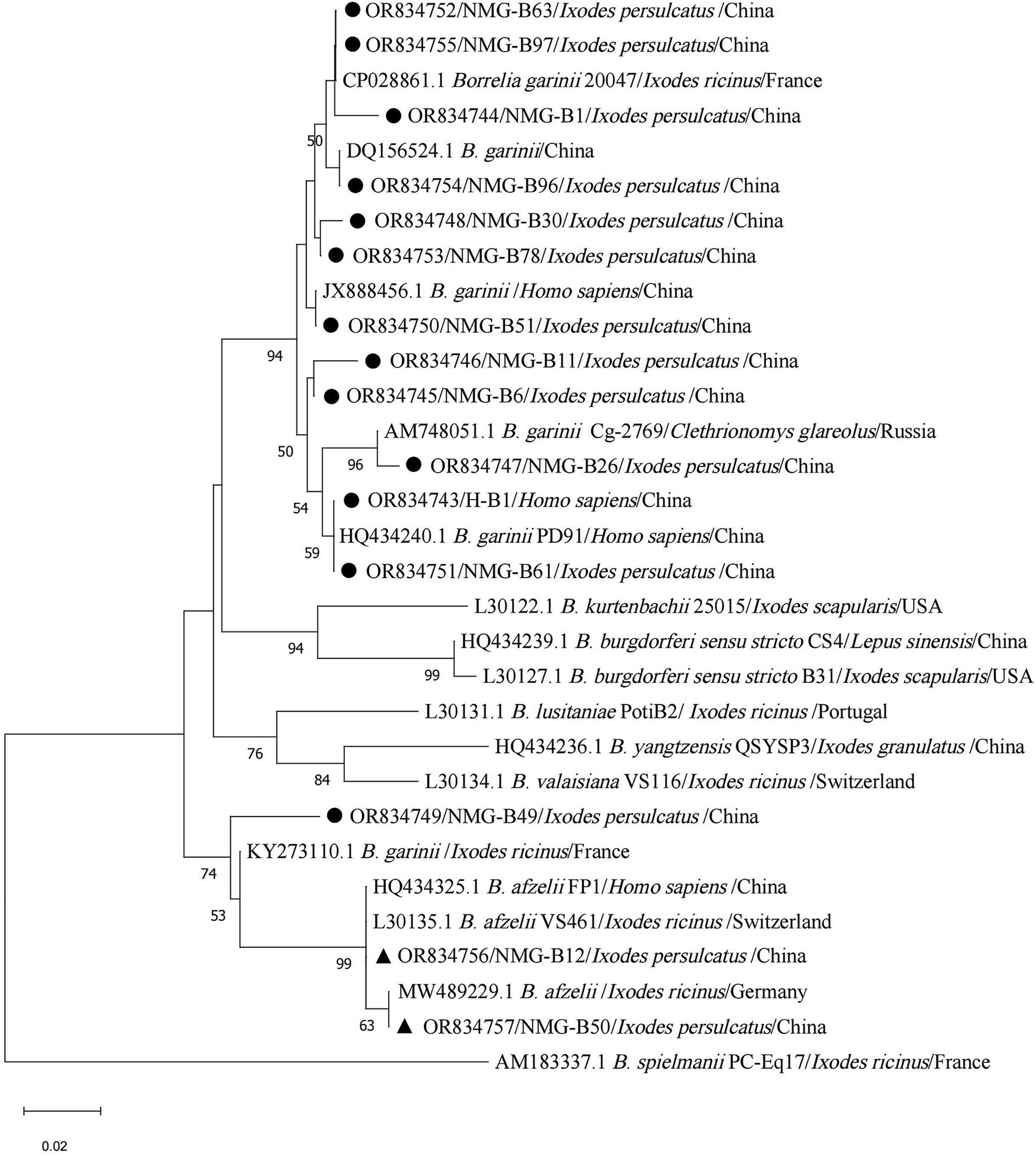
Figure 7. Phylogenetic analysis for 5S-23S rRNA intergenic spacer region of B. burgdorferi sensu lato. The sequences clustered as B. garinii were marked as “●,” B. afzelii were marked as “▲.”
Among the seven I. persulcatus samples positive for B. miyamotoi, all sequences were identical (NMG-M6) and closely related to strain HT31 (AB900798.1, type strain of B. miyamotoi), B. miyamotoi from China (KU749386.1), Japan (CP004217.2), and Russia (MK955928.1, KJ950108.1), which belong to the Siberian type (Figure 8).
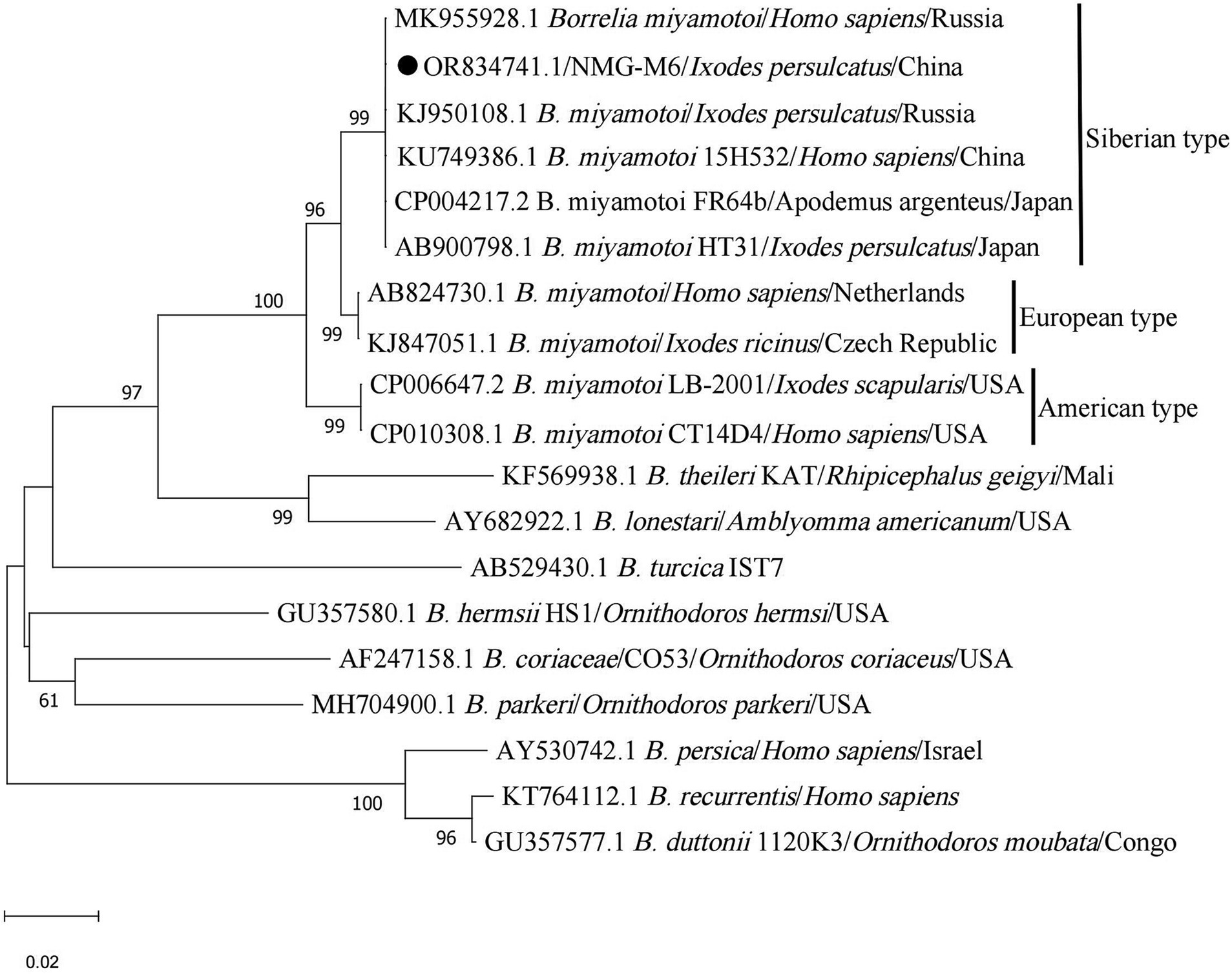
Figure 8. Phylogenetic analysis for glpQ gene of B. miyamotoi. The sequence clustered as B. miyamotoi was marked as “●.”
In addition, the sequence (H-C74) detected in a blood sample fell into the same branch as strain Namibia belonging to genomic group IV (CP007555.1), C. burnetii from China (KR697576.1) (Figure 9).
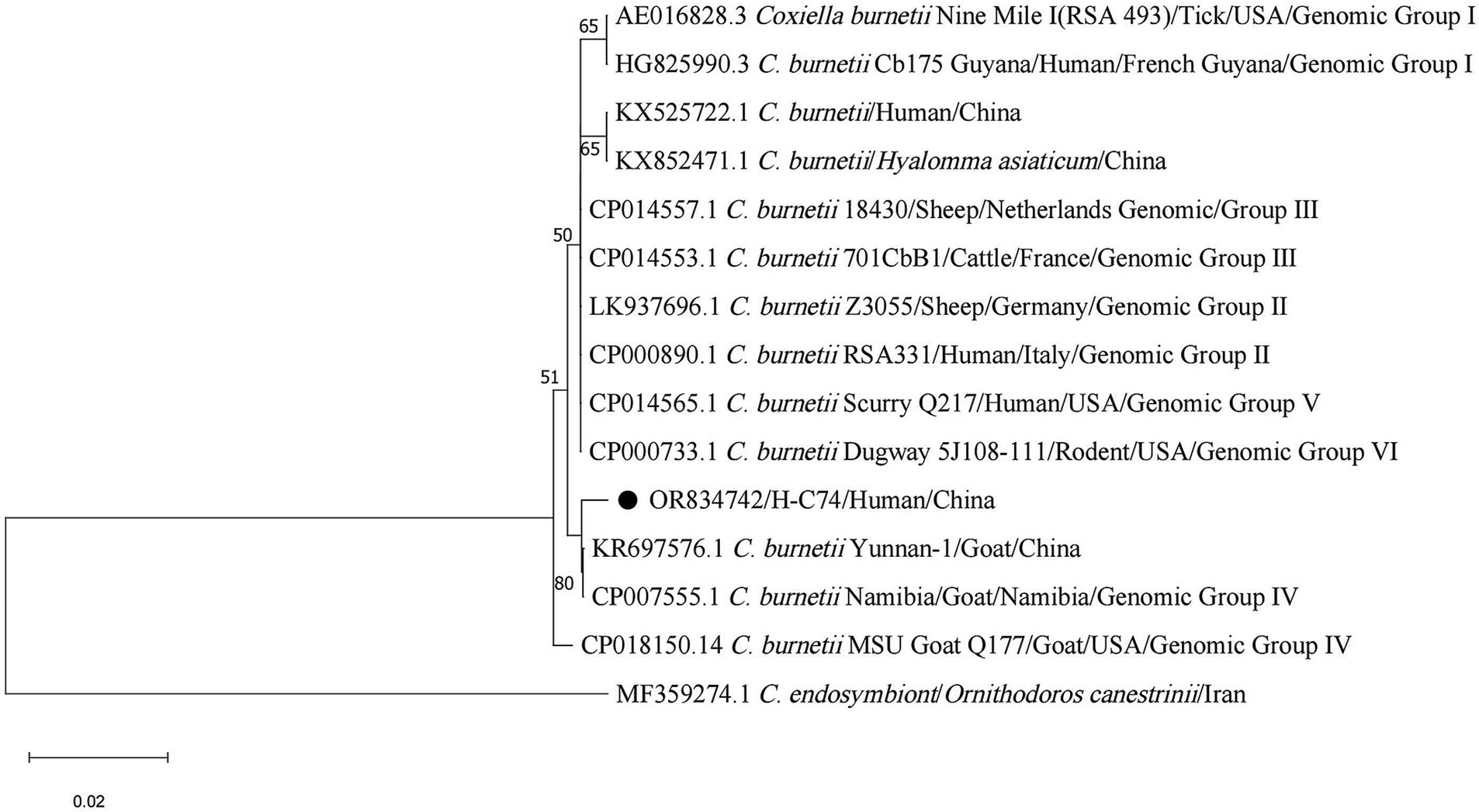
Figure 9. Phylogenetic analysis for IS1111 gene of C. burnetii. The sequence clustered as C. burnetii was marked as “●.”
Co-infection of pathogenic bacteria in ticks
In the present study, 27 I. persulcatus samples (27.00%, 27/100) were found to be co-infected with more than one bacterium (Table 3). Dual co-infection with B. garinii and Candidatus R. tarasevichiae (13.00%, 13/100) was most frequent in I. persulcatus. There was no co-infection in the participants according to PCR results.
Seroprevalence of SFGR and Borrelia burgdorferi
Among the 245 serum samples, 14 were positive for anti-SFGR by ELISA, of which four were only positive for IgG and 10 were positive for IgM and IgG (Table 4). Both IgG and IgM antibodies against SFGR were negative in those 2 individuals who were detected positive for R. slovaca using PCR. Forty serum samples were seropositive for B. burgdorferi using IFA, and positive samples were further detected by WB; 33 of them were positive for anti-B. burgdorferi IgG (Table 4). The representative image of positive western blotting reactions was displayed in Additional file 5 (Supplementary Figure S1). Two serum samples were positive for both anti-B. burgdorferi IgG and anti-SFGR IgG.
No significant difference in the seroprevalence of antibodies against SFGR and B. burgdorferi according to sex, age, or workplace was observed (Table 5).
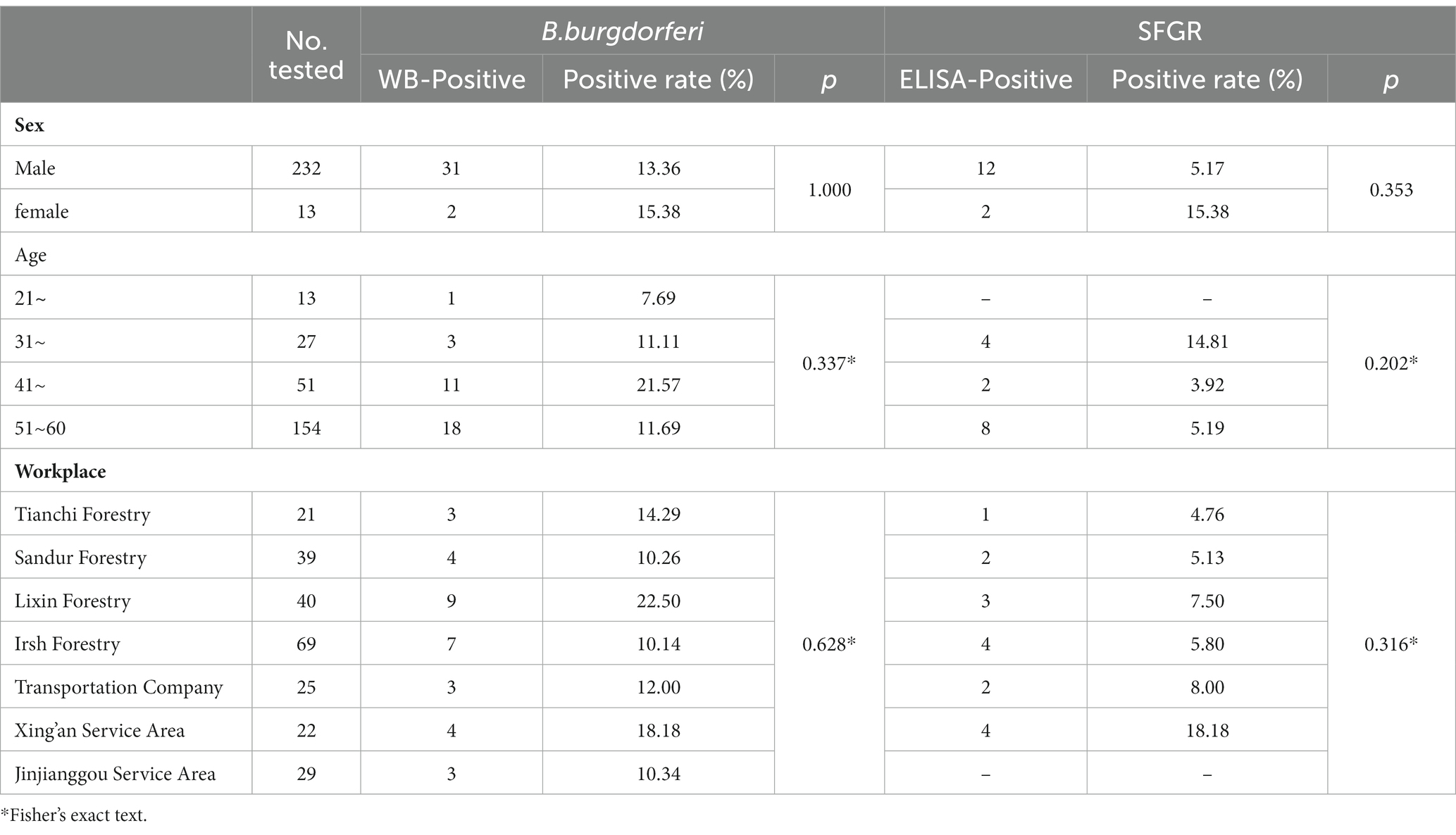
Table 5. Differences in seroprevalence of antibodies against B. burgdorferi and SFGR according to sex, age, and workplace.
Discussion
Ticks are recognized as one of the most important vectors of a wide variety of diseases in humans and animals. In recent years, an increasing number of tick-borne infections have stimulated the investigation of ticks and tick-borne pathogens (31, 32). In this study, we applied 16S rDNA V3–V4 region high-throughput sequencing combined with species-specific PCR to detect tick-borne bacteria in free-living ticks and humans at high risk of tick-borne diseases in Arxan, Inner Mongolia, China. Subsequently, the seroprevalence of antibodies against SFGR and B. burgdorferi was determined to understand the infection rate of major tick-borne bacteria in the participants.
In this survey, the collected free-living ticks were mainly I. persulcatus with a small number of D. silvarum. Arxan City is located in northeastern China, which is mainly covered by coniferous and broad-leaved forests and is characterized by strong seasonality in temperature, providing a favorable habitat for I. persulcatus. The natural habitats of D. silvarum were characterized by middle to high elevations, shrub grasslands, and low precipitation during the driest month (2). In addition, we collected ticks in May 2020 and May 2021. The peak for I. persulcatus adults occurred in late May (33), while D. silvarum peaked in mid-April (34). Due to the limitation of the sampling season, sampling sites, and the number of ticks collected, only a small number of D. silvarum were collected.
High-throughput sequencing of 16S rDNA has been widely used to study microbial communities in ticks. For example, Runlsi et al. identified 189 genera in Haemaphysalis longicornis through high-throughput sequencing at the Illumina HiSeq platform, including Anaplasma, Rickettsia, and Ehrlichia (35). Jun et al. used high-throughput sequencing to study the microbial diversity of D. nuttalli and revealed the presence and relative abundance of the bacterial genera Rickettsia, Anaplasma and Coxiella (8). In the present study, at least 32 phyla, 79 classes, 191 orders, 348 families, and 594 genera were detected in pooled DNA samples of I. persulcatus, and the Ace and Chao1 indices were more than 1068.34 and 1098.66, respectively, in each pool indicating that all pools were of highly species richness. Simpson indices were more than 0.85 except for N23 indicating that almost all pools were highly diverse. Of 594 genera, Rickettsia, Anaplasma, Coxiella, and Borrelia, were detected in pooled DNA samples of I. persulcatus through high-throughput sequencing of the 16S rDNA V3–V4 region, Rickettsia was recognized as the most common genus. Candidatus R. tarasevichiae and three Borrelia species (B. garinii, B. afzelii and B. miyamotoi) were identified in I. persulcatus by nested PCR, whereas only R. raoultii was detected in D. silvarum.
Candidatus R. tarasevichiae was first detected in I. persulcatus collected from the southern Urals and Siberia in 2003 (36) and was then found in Haemaphysalis japonica and D. silvarum from the Russian Far East (37, 38). Human cases of Candidatus R. tarasevichiae infection were first identified using laboratory molecular testing in eastern China in 2012 (39). In the present study, Candidatus R. tarasevichiae was detected in 89.00% of I. persulcatus, which was considered the dominant genotype of SFGR carried by I. persulcatus in Arxan.
Rickettsia solvaca was first isolated from D. marginatus ticks in Slovakia in 1968, and subsequent investigations reported that R. solvaca is widely distributed in Dermacentor ticks (40). Tian et al. firstly detected R. slovaca in D. silvarum collected from Xinjiang Autonomous Region, China in 2012 (41). In the present study, R. slovaca was detected in two participants using nested PCR targeting ompA gene. This is the first study to report the presence of R. slovaca has been found in humans in Inner Mongolia, China.
Rickettsia raoultii was first detected in Dermacentor ticks collected in Russia in 1999 (42) and then isolated from Dermacentor ticks in France, and named in 2008 (43). Similar to R. slovaca, R. raoultii was more common among Dermacentor ticks. Other hard ticks infected with R. raoultii have been reported by several groups in recent years, including the Haemaphysalis, Hyalomma, and Ixodes ticks (44–46). In this study, R. raoultii was detected in nine D. silvarum using nested PCR.
Candidatus R. tarasevichiae, R. slovaca, and R. raoultii were all pathogenic genotypes of spotted fever group Rickettsia. The SFG patients present with rashes, eschar, fever, fatigue, anorexia, nausea, and local lymph node enlargement. A few patients had neurological manifestations such as coma, neck stiffness, and Kernig’s sign (47). Combined with the presence of anti-SFGR antibodies among participants, public health workers in the area need to be aware of the risk of SFGR infection; regularly conduct education campaigns on tick bite prevention; and monitor populations, ticks, and animal hosts.
Borrelia burgdorferi, first isolated from I. persulcatus in 1982 (48), was the causative pathogen of Lyme disease. In our previous study, two B. garinii strains were isolated from ticks in Arxan, suggesting that this area was the natural focus of Lyme disease (49). Through nested PCR targeting 5S-23S rRNA IGS, B. garinii was the dominant genotype of B. burgdorferi carried by I. persulcatus collected from Arxan, followed by B. afzelii, and the two genotypes were dominant causative genotypes of B. burgdorferi in China. We also detected B. garinii in 12 participants, and these 12 sequences were identical to the sequence (NMG-B61) detected in I. persulcatus, indicating the transmission of B. garinii from ticks to humans in Arxan. The positivity rate of anti-B. burgdorferi in participants was 13.47% based on the IFA results and confirmed by Western Blot assay, which was similar to the positive rate of forestry populations in Inner Mongolia (15). Human Lyme disease generally occurs in stages, from the early localized stage of erythema migrans, fatigue, chills, and fever, to a late disseminated stage of intermittent bouts of arthritis with severe joint pain and swelling and neurological symptoms (29). Thus, Lyme disease should be considered by clinicians with the forestry workers who had correlated symptoms with Lyme disease in Arxan.
B. miyamotoi, initially identified and isolated in Japan in 1994 (50), is the causative agent of B. miyamotoi disease with generalized flu-like symptoms. Human cases of B. miyamotoi infection have been reported in European countries (51, 52), the United States (53, 54), Australia (55), and Japan (56). A survey of B. miyamotoi in Inner Mongolia suggested that 2.6% of I. persulcatus carried B. miyamotoi and 1.7% of patients bitten by ticks were infected with B. miyamotoi (57). In this study, B. miyamotoi which belongs to the Siberian type, was detected in 7% of I. persulcatus. This is the first report of this bacterium that has been detected in ticks from Arxan, indicating that local forestry populations are at risk of B. miyamotoi infection.
Coxiella burnetii, the causative agent of Q fever, is transmitted to humans via inhalation of infected aerosols or tick bites (58). In our study, C. burnetii was detected in one participant, indicating that extensive and in-depth monitoring of C. burnetii in ticks and animal hosts should be conducted to identify the risk of infection in the local population.
Ticks can acquire multiple pathogenic species during blood feeding on their vertebrate hosts, and humans may be infected by more than one pathogen carried by ticks. In this study, Candidatus R. tarasevichiae and B. garinii were the dominant pathogens detected in I. persulcatus, which, to some extent, explains why the rate of co-infection with B. burgdorferi and SFGR was the highest in I. persulcatus. Two participants tested positive for antibodies against both B. burgdorferi and SFGR, indicating that cases of coinfection with B. burgdorferi and SFGR were present in Arxan, Inner Mongolia. In recent years, concurrent infections with multiple tick-borne agents have been reported, including co-infection with B. burgdorferi s.l and A. phagocytophilum (59), Babesia microti and B. burgdorferi s.l (60), SFGR and severe fever with thrombocytopenia syndrome virus (61), etc. Pathogens may behave synergistically, indifferently, or antagonistically within common human hosts, thus prolonging the duration of symptoms and modulating disease severity (31). Additionally, the presence of concurrent infections may be neglected when multiple pathogens are encountered, which results in missed diagnoses and poses an additional challenge for a definitive diagnosis. Therefore, when treating patients with suspected tick-borne diseases, clinicians in this area should consider the possibility of coinfection. Long-term monitoring of tick-borne pathogens in ticks and humans should be investigated in the future.
Data availability statement
The datasets presented in this study can be found in online repositories. The names of the repository/repositories and accession number(s) can be found at: [https://www.ncbi.nlm.nih.gov/ AND OR841358–OR841362 for I. persulcatus, OR841363–OR841367 for D. silvarum].
Ethics statement
The studies involving humans were approved by the ethics committee of National Institute for Communicable Disease Control and Prevention, Chinese Center for Disease Control and Prevention, Beijing, China. The studies were conducted in accordance with the local legislation and institutional requirements. The participants provided their written informed consent to participate in this study. The manuscript presents research on animals that do not require ethical approval for their study.
Author contributions
LD: Data curation, Formal analysis, Validation, Visualization, Writing – original draft. LZ: Investigation, Formal analysis. XH: Investigation, Methodology. ZB: Methodology. YZ: Investigation. LH: Methodology. ZL: Methodology. HZ: Supervision, Writing – review & editing. QH: Project administration, Supervision, Investigation, Formal analysis, Writing – original draft, Writing – review & editing. AD: Supervision, Writing – review & editing.
Funding
The author(s) declare financial support was received for the research, authorship, and/or publication of this article. This study was supported by the National Science and Technology Major Project of China (2017ZX10303404006003), an autonomous project for the National Institute for Communicable Disease Control and Prevention, Chinese Center for Disease Control and Prevention (32055), and operation of public health emergency response mechanism-prevention and control of infectious diseases (2100410029).
Acknowledgments
We thank the National Key Laboratory of Intelligent Tracking and Forecasting for Infectious Diseases, the National Institute for Communicable Disease Control and Prevention, and the Chinese Center for Disease Control and Prevention for their technical support. We would like to thank Editage (www.editage.cn) for English language editing.
Conflict of interest
The authors declare that the research was conducted in the absence of any commercial or financial relationships that could be construed as a potential conflict of interest.
Publisher’s note
All claims expressed in this article are solely those of the authors and do not necessarily represent those of their affiliated organizations, or those of the publisher, the editors and the reviewers. Any product that may be evaluated in this article, or claim that may be made by its manufacturer, is not guaranteed or endorsed by the publisher.
Supplementary material
The Supplementary material for this article can be found online at: https://www.frontiersin.org/articles/10.3389/fpubh.2024.1302133/full#supplementary-material
Footnotes
References
1. Dantas-Torres, F, Chomel, BB, and Otranto, D. Ticks and tick-borne diseases: a one health perspective. Trends Parasitol. (2012) 28:437–46. doi: 10.1016/j.pt.2012.07.003
2. Zhao, GP, Wang, YX, Fan, ZW, Ji, Y, Liu, M-J, Zhang, W-H, et al. Mapping ticks and tick-borne pathogens in China. Nat Commun. (2021b) 12:1075. doi: 10.1038/s41467-021-21375-1
3. Al-Hosary, A, Răileanu, C, Tauchmann, O, Fischer, S, Nijhof, AM, and Silaghi, C. Tick species identification and molecular detection of tick-borne pathogens in blood and ticks collected from cattle in Egypt. Ticks Tick-Borne Diseases. (2021) 12:101676. doi: 10.1016/j.ttbdis.2021.101676
4. Capligina, V, Seleznova, M, Akopjana, S, Freimane, L, Lazovska, M, Krumins, R, et al. Large-scale countrywide screening for tick-borne pathogens in field-collected ticks in Latvia during 2017–2019. Parasit Vectors. (2020) 13:351. doi: 10.1186/s13071-020-04219-7
5. Gilbert, L . The impacts of climate change on ticks and tick-borne disease risk. Annu Rev Entomol. (2021) 66:373–88. doi: 10.1146/annurev-ento-052720-094533
6. Madison-Antenucci, S, Kramer, LD, Gebhardt, LL, and Kauffman, E. Emerging tick-borne diseases. Clin Microbiol Rev. (2020) 33:e18. doi: 10.1128/CMR.00083-18
7. Gaowa, W, Wulantuya,, Sato, K, Liu, D, Cui, Y, Yin, X, et al. Surveillance of Borrelia miyamotoi-carrying ticks and genomic analysis of isolates in Inner Mongolia. China Paras Vectors. (2021) 14:368. doi: 10.1186/s13071-021-04809-z
8. Jiao, J, Lu, Z, Yu, Y, Ou, Y, Fu, M, Zhao, Y, et al. Identification of tick-borne pathogens by metagenomic next-generation sequencing in Dermacentor nuttalli and Ixodes persulcatus in Inner Mongolia. China Parasit Vectors. (2021) 14:287. doi: 10.1186/s13071-021-04740-3
9. Kong, Y, Zhang, G, Jiang, L, Wang, P, Zhang, S, Zheng, X, et al. Metatranscriptomics reveals the diversity of the tick Virome in Northwest China. Microbiol Spect. (2022) 10:e0111522. doi: 10.1128/spectrum.01115-22
10. Liu, D, Wulantuya, FH, Fan, H, Li, F, Li, F, Gao, T, et al. Co-infection of tick-borne bacterial pathogens in ticks in Inner Mongolia. PLoS Negl Trop Dis. (2023) 17:e0011121. doi: 10.1371/journal.pntd.0011121
11. Sun, R . Study on Spatiotenporal distribution of tick-borne encephalitis in China. [dissertation/master’s thesis]. Hefei (AH): Anhui Medical University (2016).
12. Black, WC, and Piesman, J. Phylogeny of hard-and soft-tick taxa (Acari: Ixodida) based on mitochondrial 16S rDNA sequences. Proc Natl Acad Sci USA. (1994) 91:10034–8. doi: 10.1073/pnas.91.21.10034
13. De Keukeleire, M, Robert, A, Luyasu, V, Kabamba, B, and Vanwambeke, SO. Seroprevalence of Borrelia burgdorferi in Belgian forestry workers and associated risk factors. Parasit Vectors. (2018) 11:277. doi: 10.1186/s13071-018-2860-2
14. Stark, JH, Li, X, Zhang, JC, Burn, L, Valluri, SR, Liang, J, et al. Systematic review and Meta-analysis of Lyme disease data and Seropositivity for Borrelia burgdorferi, China, 2005–2020. Emerg Infect Dis. (2022) 28:2389–97. doi: 10.3201/eid2812.212612
15. Zhang, Z, Wan, K, Zhang, J, Zhu, G, Dou, G, Li, M, et al. Study on Epidemiology and Etiology of Lyme Disease in China. Chin J Epidemiol. (1997) 18:8–11.
16. Bolger, AM, Lohse, M, and Usadel, B. Trimmomatic: a flexible trimmer for Illumina sequence data. Bioinformatics. (2014) 30:2114–20. doi: 10.1093/bioinformatics/btu170
17. Callahan, BJ, McMurdie, PJ, Rosen, MJ, Han, AW, Johnson, AJA, and Holmes, SP. DADA2: high-resolution sample inference from Illumina amplicon data. Nat Methods. (2016) 13:581–3. doi: 10.1038/nmeth.3869
18. Edgar, RC . UPARSE: highly accurate OTU sequences from microbial amplicon reads. Nat Methods. (2013) 10:996–8. doi: 10.1038/nmeth.2604
19. Quast, C, Pruesse, E, Yilmaz, P, Gerken, J, Schweer, T, Yarza, P, et al. The SILVA ribosomal RNA gene database project: improved data processing and web-based tools. Nucleic Acids Res. (2013) 41:D590–6. doi: 10.1093/nar/gks1219
20. Bolyen, E, Rideout, JR, Dillon, MR, Bokulich, NA, Abnet, CC, Al-Ghalith, GA, et al. Reproducible, interactive, scalable and extensible microbiome data science using QIIME 2. Nat Biotech. (2019) 37:852. doi: 10.1038/s41587-019-0209-9.
21. Zhang, L, Miao, G, Hou, X, Li, B, and Hao, Q. Evaluation of nested PCR and real-time PCR in host surveillance of Lyme disease. Chin J Vector Biol & Control. (2018) 29:425–7. doi: 10.11853/j.issn.1003.8280.2018.05.001
22. Schwan, TG, Schrumpf, ME, Hinnebusch, BJ, Anderson, DE, and Konkel, ME. GlpQ: an antigen for serological discrimination between relapsing fever and Lyme borreliosis. J Clin Microbiol. (1996) 34:2483–92. doi: 10.1128/jcm.34.10.2483-2492.1996
23. Roux, V, Fournier, PE, and Raoult, D. Differentiation of spotted fever group rickettsiae by sequencing and analysis of restriction fragment length polymorphism of PCR-amplified DNA of the gene encoding the protein rOmpA. J Clin Microbiol. (1996) 34:2058–65. doi: 10.1128/jcm.34.9.2058-2065.1996
24. Socolovschi, C, Mediannikov, O, Sokhna, C, Tall, A, Diatta, G, Bassene, H, et al. Rickettsia felis-associated uneruptive fever. Senegal Emerg Infect Diseases. (2010) 16:1140–2. doi: 10.3201/eid1607.100070
25. Overzier, E, Pfister, K, Thiel, C, Herb, I, Mahling, M, and Silaghi, C. Anaplasma phagocytophilum in questing Ixodes ricinus ticks: comparison of prevalences and partial 16S rRNA gene variants in urban, pasture, and natural habitats. Appl Environ Microbiol. (2013) 79:1730–4. doi: 10.1128/AEM.03300-12
26. Anderson, BE, Sumner, JW, Dawson, JE, Tzianabos, T, Greene, CR, Olson, JG, et al. Detection of the etiologic agent of human ehrlichiosis by polymerase chain reaction. J Clin Microbiol. (1992) 30:775–80. doi: 10.1128/jcm.30.4.775-780.1992
27. Dawson, JE, Stallknecht, DE, Howerth, EW, Warner, C, Biggie, K, Davidson, WR, et al. Susceptibility of white-tailed deer (Odocoileus virginianus) to infection with Ehrlichia chaffeensis, the etiologic agent of human ehrlichiosis. J Clin Microbiol. (1994) 32:2725–8. doi: 10.1128/jcm.32.11.2725-2728.1994
28. Fenollar, F, Fournier, PE, and Raoult, D. Molecular detection of Coxiella burnetii in the sera of patients with Q fever endocarditis or vascular infection. J Clin Microbiol. (2004) 42:4919–24. doi: 10.1128/JCM.42.11.4919-4924.2004
29. Zhang, L, Zhu, X, Hou, X, Li, H, Yang, X, Chen, T, et al. Prevalence and prediction of Lyme disease in Hainan province. PLoS Negl Trop Dis. (2021) 15:e0009158. doi: 10.1371/journal.pntd.0009158
30. Jiang, Y, Wan, K, Geng, Z, and Hou, X. Standard criteria of Western blot for the diagnosis of Lyme disease caused by Borrelia garinii in China. Chin J Microbiol Immunol. (2005) 7:594–8. doi: 10.3760/j:issn:0254-5101.2005.07.020
31. Cutler, SJ, Vayssier-Taussat, M, Estrada-Peña, A, Potkonjak, A, Mihalca, AD, and Zeller, H. Tick-borne diseases and co-infection: current considerations. Ticks Tick-Borne Diseases. (2021) 12:101607. doi: 10.1016/j.ttbdis.2020.101607
32. Rodino, KG, Theel, ES, and Pritt, BS. Tick-borne diseases in the United States. Clin Chem. (2020) 66:537–48. doi: 10.1093/clinchem/hvaa040
33. Xu, S . Studies on population ecology of Ixodes persulcatus and its infection with the tick-borne pathogens. [dissertation/master’s thesis]. Shijiazhuang (HB): Hebei Normal University (2015).
34. Yu, Z . Studies of population ecology of Dermacentor silvarum and antimicrobial Molecales of egg wax in Amblyomma hebraeum [dissertation/master’s thesis]. Shijiazhuang (HB): Heibei Normal University (2011).
35. Cao, R, Ren, Q, Luo, J, Tian, Z, Liu, W, Zhao, B, et al. Analysis of microorganism diversity in from Shaanxi, China, based on metagenomic sequencing. Front Genet. (2021) 12:723773. doi: 10.3389/fgene.2021.723773
36. Shpynov, S, Fournier, P-E, Rudakov, N, and Raoult, D. Candidatus Rickettsia tarasevichiae in Ixodes persulcatus ticks collected in Russia. Ann N Y Acad Sci. (2003) 990:162–72. doi: 10.1111/j.1749-6632.2003.tb07358.x
37. Igolkina, Y, Rar, V, Vysochina, N, Ivanov, L, Tikunov, A, Pukhovskaya, N, et al. Genetic variability of Rickettsia spp. in Dermacentor and Haemaphysalis ticks from the Russian Far East. Ticks Tick-Borne Diseases. (2018) 9:1594–603. doi: 10.1016/j.ttbdis.2018.07.015
38. Mediannikov, O, Sidelnikov, Y, Ivanov, L, Fournier, PE, Tarasevich, I, and Raoult, D. Far eastern tick-borne rickettsiosis: identification of two new cases and tick vector. Ann N Y Acad Sci. (2006) 1078:80–8. doi: 10.1196/annals.1374.010
39. Jia, N, Zheng, YC, Jiang, JF, Ma, L, and Cao, WC. Human infection with Candidatus Rickettsia tarasevichiae. N Engl J Med. (2013) 369:1178–80. doi: 10.1056/NEJMc1303004
40. Parola, P, Paddock, CD, and Raoult, D. Tick-borne rickettsioses around the world: emerging diseases challenging old concepts. Clin Microbiol Rev. (2005) 18:719–56. doi: 10.1128/CMR.18.4.719-756.2005
41. Tian, ZC, Liu, GY, Shen, H, Xie, J-R, Luo, J, and Tian, M-Y. First report on the occurrence of Rickettsia slovaca and Rickettsia raoultii in Dermacentor silvarum in China. Parasit Vectors. (2012) 5:19. doi: 10.1186/1756-3305-5-19
42. Rydkina, E, Roux, V, Rudakov, N, Gafarova, M, Tarasevich, I, and Raoult, D. New Rickettsiae in ticks collected in territories of the former Soviet Union. Emerg Infect Dis. (1999) 5:811–4. doi: 10.3201/eid0506.990612
43. Mediannikov, O, Matsumoto, K, Samoylenko, I, Drancourt, M, Roux, V, Rydkina, E, et al. Rickettsia raoultii sp. nov., a spotted fever group rickettsia associated with Dermacentor ticks in Europe and Russia. Int J Syst Evol Microbiol. (2008) 58:1635–9. doi: 10.1099/ijs.0.64952-0
44. Guo, W-P, Wang, Y-H, Lu, Q, Xu, G, Luo, Y, Ni, X, et al. Molecular detection of spotted fever group rickettsiae in hard ticks, northern China. Transbound Emerg Dis. (2019) 66:1587–96. doi: 10.1111/tbed.13184
45. Lu, M, Ji, Y, Zhao, H, Wang, W, Tian, J, Duan, C, et al. Circulation of multiple Rickettsiales bacteria in ticks from Sichuan province. Southwest China Microbial Pathogenesis. (2023) 183:106313. doi: 10.1016/j.micpath.2023.106313
46. Teng, Z, Shi, Y, Zhao, N, Zhang, X, Jin, X, He, J, et al. Molecular detection of tick-borne bacterial and protozoan pathogens in Haemaphysalis longicornis (Acari: Ixodidae) Ticks from free-ranging domestic sheep in Hebei Province, China. Pathogens. (2023) 12:763. doi: 10.3390/pathogens12060763
47. Fang, L-Q, Liu, K, Li, X-L, Liang, S, Yang, Y, Yao, H-W, et al. Emerging tick-borne infections in mainland China: an increasing public health threat. Lancet Infect Dis. (2015) 15:1467–79. doi: 10.1016/S1473-3099(15)00177-2
48. Burgdorfer, W, Barbour, AG, Hayes, SF, Benach, JL, Grunwaldt, E, and Davis, JP. Lyme disease-a tick-borne spirochetosis? Science. (1982) 216:1317–9. doi: 10.1126/science.7043737
49. Duan, LK, Hou, XX, Zhang, L, Bao, ZH, Liu, ZL, Shi, QY, et al. Detection and genotyping of Borrelia burgdorferi from free-living ticks in Arxan area, Inner Mongolia. China Chin J Vector Biol Control. (2022) 33:642–7. doi: 10.11853/j.issn.1003.8280.2022.05.006
50. Fukunaga, M, Takahashi, Y, Tsuruta, Y, Matsushita, O, Ralph, D, McClelland, M, et al. Genetic and phenotypic analysis of Borrelia miyamotoi sp. nov., isolated from the ixodid tick Ixodes persulcatus, the vector for Lyme disease in Japan. Int J Syst Bacteriol. (1995) 45:804–10. doi: 10.1099/00207713-45-4-804
51. Hovius, JWR, Wever, B, Sohne, M, Brouwer, MC, Coumou, J, Wagemakers, A, et al. A case of meningoencephalitis by the relapsing fever spirochaete Borrelia miyamotoi in Europe. Lancet. (2013) 382:658. doi: 10.1016/S0140-6736(13)61644-X
52. Hrnková, J, Golovchenko, M, Musa, AS, Needham, T, Italiya, J, Ceacero, F, et al. Borrelia spirochetes in European exotic farm animals. Front Vet Sci. (2022) 9:996015. doi: 10.3389/fvets.2022.996015
53. Krause, PJ, Narasimhan, S, Wormser, GP, Rollend, L, Fikrig, E, Lepore, T, et al. Human Borrelia miyamotoi infection in the United States. N Engl J Med. (2013) 368:291–3. doi: 10.1056/NEJMc1215469
54. Xu, G, Luo, C-Y, Ribbe, F, Pearson, P, Ledizet, M, and Rich, SM. Borrelia miyamotoi in human-biting ticks, United States, 2013–2019. Emerg Infect Dis. (2021) 27:3193–5. doi: 10.3201/eid2712.204646
55. Tobudic, S, Burgmann, H, Stanek, G, Winkler, S, Schötta, A-M, Obermüller, M, et al. Human Borrelia miyamotoi infection, Austria. Emerg Infect Dis. (2020) 26:2201–4. doi: 10.3201/eid2609.191501
56. Kumagai, Y, Sato, K, Taylor, KR, Zamoto-Niikura, A, Imaoka, K, Morikawa, S, et al. A relapsing fever group Borrelia sp. is widely distributed among wild deer in Japan. Ticks Tick-Borne Diseases. (2018) 9:465–70. doi: 10.1016/j.ttbdis.2017.12.016
57. Gao, Y, Lv, X-L, Han, S-Z, Wang, W, Liu, Q, and Song, M. First detection of Borrelia miyamotoi infections in ticks and humans from the northeast of Inner Mongolia. China Acta Tropica. (2021) 217:105857. doi: 10.1016/j.actatropica.2021.105857
58. Melenotte, C, Million, M, and Raoult, D. New insights in Coxiella burnetii infection: diagnosis and therapeutic update. Expert Rev Anti-Infect Ther. (2020) 18:75–86. doi: 10.1080/14787210.2020.1699055
59. Moniuszko-Malinowska, A, Dunaj, J, Andersson, MO, Chmielewski, T, Czupryna, P, Groth, M, et al. Anaplasmosis in Poland – analysis of 120 patients. Ticks Tick-Borne Diseases. (2021) 12:101763. doi: 10.1016/j.ttbdis.2021.101763
60. Diuk-Wasser, MA, Vannier, E, and Krause, PJ. Coinfection by Ixodes tick-borne pathogens: ecological, epidemiological, and clinical consequences. Trends Parasitol. (2016) 32:30–2. doi: 10.1016/j.pt.2015.09.008
61. Zhao, B, Hou, H, Gao, R, Tian, B, and Deng, B. Mononucleosis-like illnesses due to co-infection with severe fever with thrombocytopenia syndrome virus and spotted fever group rickettsia:a case report. BMC Infect Dis. (2021a) 21:829. doi: 10.1186/s12879-021-06434-8
Keywords: tick-borne bacteria, Ixodes persulcatus , co-infection, spotted fever group Rickettsia, Inner Mongolia
Citation: Duan L, Zhang L, Hou X, Bao Z, Zeng Y, He L, Liu Z, Zhou H, Hao Q and Dong A (2024) Surveillance of tick-borne bacteria infection in ticks and forestry populations in Inner Mongolia, China. Front. Public Health. 12:1302133. doi: 10.3389/fpubh.2024.1302133
Edited by:
Omid Teymournejad, University of Illinois Chicago, United StatesReviewed by:
Xin-Ru Wang, Upstate Medical University, United StatesMarina Eremeeva, Georgia Southern University, United States
Copyright © 2024 Duan, Zhang, Hou, Bao, Zeng, He, Liu, Zhou, Hao and Dong. This is an open-access article distributed under the terms of the Creative Commons Attribution License (CC BY). The use, distribution or reproduction in other forums is permitted, provided the original author(s) and the copyright owner(s) are credited and that the original publication in this journal is cited, in accordance with accepted academic practice. No use, distribution or reproduction is permitted which does not comply with these terms.
*Correspondence: Qin Hao, aGFvcWluQGljZGMuY24=; Aiying Dong, ZG9uZ2FpeWluZzY4QDEyNi5jb20=
†These authors have contributed equally to this work and share first authorship