- 1Department of Clinical Epidemiology, Shengjing Hospital of China Medical University, Shenyang, China
- 2Department of Otorhinolaryngology - Head and Neck Surgery, Shengjing Hospital of China Medical University, Shenyang, China
- 3Department of Clinical Laboratory, Shengjing Hospital of China Medical University, Shenyang, China
- 4Clinical Research Center, Shengjing Hospital of China Medical University, Shenyang, China
Background and aim: The current study aimed to clarify the association between household polluting cooking fuels and adverse birth outcomes using previously published articles.
Methods: In this systematic review and meta-analysis, a systematic literature search in PubMed, Embase, Web of Science, and Scopus databases were undertaken for relevant studies that had been published from inception to 16 January 2023. We calculated the overall odds ratio (OR) and 95% confidence interval (CI) for adverse birth outcomes [low birth weight (LBW), small for gestational age (SGA), stillbirth, and preterm birth (PTB)] associated with polluting cooking fuels (biomass, coal, and kerosene). Subgroup analysis and meta-regression were also conducted.
Results: We included 16 cross-sectional, five case–control, and 11 cohort studies in the review. Polluting cooking fuels were found to be associated with LBW (OR: 1.37, 95% CI: 1.24, 1.52), SGA (OR: 1.48, 95% CI: 1.13, 1.94), stillbirth (OR: 1.38, 95% CI: 1.23, 1.55), and PTB (OR: 1.27, 95% CI: 1.19, 1.36). The results of most of the subgroup analyses were consistent with the main results. In the meta-regression of LBW, study design (cohort study: P < 0.01; cross-sectional study: P < 0.01) and sample size (≥ 1000: P < 0.01) were the covariates associated with heterogeneity. Cooking fuel types (mixed fuel: P < 0.05) were the potentially heterogeneous source in the SGA analysis.
Conclusion: The use of household polluting cooking fuels could be associated with LBW, SGA, stillbirth, and PTB. The limited literature, observational study design, exposure and outcome assessment, and residual confounding suggest that further strong epidemiological evidence with improved and standardized data was required to assess health risks from particular fuels and technologies utilized.
Introduction
Household air pollution (HAP) is often considered to be a major public health problem in low- and middle-income countries (LMICs) (1). HAP has a direct impact on human health and is an important risk factor for increased morbidity and mortality (2). The inefficient combustion of solid fuels (wood, coal, charcoal, dung, and crop waste) and kerosene in simple stoves and devices is a major source of HAP (3–5). Approximately 2.4 billion people cook mainly with polluting fuels (solid fuels and kerosene) globally (6). The inefficient combustion of polluting fuels often emits a high level of air pollutants (7). It is estimated that nearly 3 million people die from HAP exposure every year, the vast majority of whom live in LMICs (1). Research has shown that exposure to HAP is associated with chronic obstructive pulmonary disease, lung cancer, acute respiratory infections, cerebrovascular disease, ischemic heart disease, and adverse birth outcomes (8–11). The mechanism involved in the cardiorespiratory effect includes inflammation and oxidative stress induced by reactive oxygen and nitrogen species generated by inhaled pollutants (12, 13). HAP has also been associated with epigenetic adverse effects, which change DNA expression and potentiate the inflammatory effects of pollutants (14).
Perinatal morbidity and mortality are majorly associated with adverse birth outcomes, such as low birth weight (LBW), small for gestational age (SGA), stillbirth, and preterm birth (PTB) (15). For example, 21.9% of neonatal deaths were attributable to being born SGA (16). The leading causes of death in children under 5 years old were PTB complications (17). The risk factors of adverse birth outcomes include both individual (e.g., smoking, diet, antenatal depression, and antenatal care) and environmental factors (e.g., air pollution, occupational exposure, and pesticides) (18–22). For example, evidence suggests that the combustion of polluting cooking fuels emits high levels of air pollutants, such as fine particulate matter (PM2.5), carbon monoxide (CO), and polycyclic aromatic hydrocarbons (PAHs), which can influence fetal development (23, 24).
A growing number of epidemiological studies focus on the association between HAP and adverse birth outcomes. Two meta-analyses have pooled the effects of solid fuel use and adverse birth outcomes (25, 26). Both mainly focused on LBW and stillbirth, and only one study conducted a subgroup analysis for LBW (26). In addition, the study did not address the question of whether the strength of any association between polluting fuel use and the risk of adverse birth outcomes was affected by different types of polluting fuel (26). Since then, many new studies have been conducted, focusing on a broader range of fuel types and adverse birth outcomes, and the conclusion is still inconsistent. One cohort study (27), two case–control studies (28, 29), and six cross-sectional studies (30–35) suggested that polluting fuel use was related to increased risk of adverse birth outcomes, while other studies (23, 24, 36–47) failed to reveal a significant correlation between them. A recent review of the subject qualitatively assessed the impact of unclean cooking fuels on adverse birth outcomes, but the review was short of quantitative combined research data (48). The HAP field is evolving rapidly. Confirming or disputing earlier findings is vital in this circumstance. Furthermore, a timely review of research methods and results can assist or guide further research as well as public health policy. Based on these considerations, we carried out a systematic review and meta-analysis to determine whether HAP was associated with LBW, stillbirth, PTB, and SGA. We also explored whether the strength of any association between polluting fuel use and the risk of adverse birth outcomes was affected by different types of polluting fuel. Subgroup and meta-regression analyses were conducted to explore the sources of heterogeneity.
Methods
The current systematic review and meta-analysis scrutinized the published adverse birth outcomes data related to polluting cooking fuel use from previous research publications using previously published search. We used the standard method of the preferred reporting items for systematic review and meta-analysis (PRISMA) protocols 2009 for conducting this review study (49). The study protocol was registered with PROSPERO (CRD42021269660).
Search strategy
We searched the PubMed, Embase, Web of Science, and Scopus databases for articles reporting HAP and adverse birth outcomes published up to 16 January 2023. In the present review, HAP is defined as chemical, biological, and physical contamination of house air and derived from the use of polluting fuel (wood, dung, crop residues, charcoal, coal, and kerosene) for cooking (31). An infant weighing < 2,500 g was defined as LBW (23), and SGA referred to a baby weighing less than the 10th percentile for a certain gestational age (35). Stillbirth was defined as delivering a baby without any sign of life after 20 weeks of gestation (27, 50), while PTB was defined as an infant born before 37 weeks (24). The search process has been focused on the following terms: (indoor air pollution or household air pollution or cooking fuel or unclean fuel or solid fuel or biomass or wood or coal or kerosene or cooking) and (pregnancy outcome or pregnancy complications or low birth weight or premature birth or stillbirth or small for gestational age). The detailed search strategies are presented in Supplementary Table 1. We also scanned the reference lists of retrieved articles and previous meta-analyses to identify additional studies.
Selection criteria
All studies obtained from the aforementioned resources were independently evaluated by two reviewers for inclusion and exclusion. First, the title and abstract of each study were reviewed, and the full text of the relevant studies was retrieved and assessed for inclusion eligibility. Studies were considered for inclusion if they were (1) original studies, (2) conducted in the human population, and (3) quantified the association between HAP exposure during pregnancy and adverse birth outcomes (LBW, SGA, stillbirth, and PTB). Studies were excluded for the following reasons: (1) the outcome was due to other factors (e.g., maternal age, educational level, and the house renovation) but not polluting cooking fuels (e.g., wood, dung, crop residues, charcoal, coal, and kerosene); (2) studies reported other outcomes those were not of interest; (3) the subjects from the control group were not exposed to clean fuels (e.g., electricity, liquid petroleum gas, natural gas, and biogas) or were exposed to polluting fuels; (4) studies did not report relative risk (RR) or odds ratio (OR); (5) conference abstract, letter, or protocol; (6) not published in English. Based on the inclusion and exclusion criteria, we excluded several unqualified studies from previous meta-analyses. The reasons for exclusion are as follows: reported the impact of other factors (e.g., cooking smoke and chimney stove) on adverse birth outcomes (n = 3) (51–53); the outcomes were not of interest (n = 2) (54, 55); did not report RR or OR (n = 3) (56–58); published in non-English (n = 2) (59, 60).
Data extraction and quality assessment
Data from all included studies were independently extracted by two reviewers and cross-checked to avoid errors. The following information was extracted from the publications: author, publication year, location of the study, study population, study design, study period, exposure, outcome, covariates adjustment, the frequency distribution of exposure and outcome, comparator, exposure, and outcome assessment method. We also extracted risk estimates of association relating polluting cooking fuel to adverse birth outcomes for pooled analysis. RR or OR with precision [95% confidence interval (CI)] was extracted from included studies. The fully adjusted effect estimates were used for analysis when both unadjusted and adjusted estimates were provided.
The methodological quality of cohort and case–control studies was assessed by the Newcastle–Ottawa scale (range, 0–9 stars) (61). Each study was awarded stars based on three dimensions [selection, comparability, and outcome (cohort studies) or exposure (case–control studies)] (61). A study awarded seven or more stars was considered high quality (62), whereas one awarded three or fewer stars was considered low quality (63). The 11-item checklist was used for methodological quality assessment of cross-sectional studies, which had been recommended by the Agency for Healthcare Research and Quality (range, 0–11 scores) (64). A study with an eight or higher score was deemed to be of high quality and that with a three or lower score was of low quality (64).
Statistical analysis
We assumed that RR was approximately equivalent to OR for our rare adverse birth outcomes (65, 66). To examine the association between polluting cooking fuels and adverse birth outcomes, we calculated ORs and corresponding 95% CIs. The I2 statistic was defined to assess the heterogeneity across studies. We reported the heterogeneity as low, moderate, or high with I2 values of 25, 50, or 75%, respectively (63). Publication bias was quantitatively assessed using Egger's tests. Moreover, the trim and fill method was adopted when publication bias existed. Meta-regression analysis was considered when there were 10 or more studies (67). Subgroup and meta-regression analyses were conducted by location (Asia, America, or Africa), type of study design (cohort, case–control, or cross-sectional), sample size (< 1,000 or ≥1,000), cooking fuel type [biomass fuels (wood, charcoal, crop residues, and animal dung), fossil fuels (coal and kerosene), or mixed fuels (biomass plus fossil fuels)], and outcome assessment method [direct assessment (measure and health/hospital records) or indirect assessment (maternal recall)]. Finally, sensitivity analyses were conducted to examine the influence of each study on the overall pooled estimate by the omission of each estimate one at a time (67). All the statistical analyses were performed using Stata statistical software version 14.0 (Copyright 1985–2015 StataCorp LP), and P-values < 0.05 were considered significant.
Results
Study selection
Figure 1 shows the literature screening processes in detail. In brief, 2,604 publications were identified through PubMed (n = 441), Embase (n = 421), Web of Science (n = 943), and Scopus (n = 798). An additional article was identified by a manual search of reference lists of included studies (n = 1). After excluding duplicates (n = 959), 1,536 records were excluded based on title and abstract. We obtained 109 articles for in-depth evaluation, of which 77 were excluded after reviewing the full text. Ultimately, 32 studies were enrolled in our final systematic review and meta-analysis.
Study characteristics
A summary of the characteristics of 32 publications is presented in Table 1. A total of 23 studies were conducted in Asia (9–11, 23, 24, 30–41, 43, 47, 68–71), three were conducted in America (28, 42, 72), five in Africa (29, 44–46, 73), and one study used a sample from more than one continent (27). Study designs included cohort studies (n = 11) (10, 11, 24, 27, 37, 40, 42, 44, 45, 47, 68), case–control studies (n = 5) (28, 29, 43, 70, 72), and cross-sectional studies (n = 16) (9, 23, 30–36, 38, 39, 41, 46, 69, 71, 73). Supplementary Table 2 quantitatively describes the association between exposure and outcome. Exposure to HAP during pregnancy was assessed through an interview or questionnaire survey by inquiring about the main cooking fuel type. In total, 13 studies focused on HAP from biomass fuels on adverse birth outcomes (9–11, 28, 35, 36, 38, 42, 45, 68, 70, 72, and 73). Six studies investigated biomass and fossil fuels and their impact on outcomes (24, 29, 30, 37, 40, and 71). In total, 13 studies classified fuels as polluting (biomass and fossil) or clean (23, 27, 31–34, 39, 41, 43, 44, 46, 47, 69) fuels. The comparator group was either exposure to clean fuels (electricity, liquid petroleum gas, natural gas, and biogas) (n = 29) (9, 10, 23, 24, 27–41, 43–47, 68, 69, and 71–73) or “no exposure” to polluting fuels (n = 3) (11, 42, and 70). In addition, three studies also directly measured the concentrations of kitchen air pollutants, such as CO (28), PM2.5 (40), and inhalable particles (PM10) (38), and quantified an exposure–response relationship between air pollutants and adverse birth outcomes. Seven studies directly measured birth outcomes (11, 24, 29, 33, 34, 36, and 70), eight relied on maternal recall (9, 30, 31, 38, 39, 41, 47, and 71), and 12 collected data from medical/health records (10, 28, 32, 35, 37, 40, 42, 43, 45, 68, 72, and 73), while the other five used a combination of maternal recall and medical records (23, 27, 44, 46, and 69). A total of 21 studies only reported one adverse birth outcome (9, 10, 23, 27–34, 40–43, 46, 47, 69–71, and 73), and 11 reported two or more outcomes (11, 24, 35–39, 44, 45, 68, and 72).
Detailed quality assessments are shown in Supplementary Tables 3–5. In general, six cohort studies were of high quality, while the rest were of moderate quality.
Pooled analysis
In this updated meta-analysis, we found polluting cooking fuels to be associated with LBW, SGA, stillbirth, and PTB. A total of 24 studies were included for investigating the relationship between polluting cooking fuels and LBW; the pooled OR was 1.37 (95% CI: 1.24, 1.52) with high heterogeneity (I2 = 75.0%, P < 0.001) (Figure 2). We found evidence of publication bias (Egger's test P < 0.001) (Supplementary Figure 1). After applying the trim and fill method, the corrected OR was 1.13 (95% CI: 1.01, 1.26). A total of 11 studies were explored for SGA; the pooled OR was 1.48 (95% CI: 1.13, 1.94), with high heterogeneity (I2 = 88.7%, P < 0.001) (Figure 3). No significant publication bias was found (Egger's test P = 0.468) (Supplementary Figure 2). For stillbirth, seven studies were explored, and the pooled OR was 1.38 (95% CI, 1.23, 1.55), with low statistical heterogeneity (I2 = 18.9%, P = 0.269) (Figure 4). We found no evidence of publication bias (Egger's test P = 0.584) (Supplementary Figure 3). Seven studies were explored for PTB; the pooled OR was 1.27 (95% CI: 1.19, 1.36), with low statistical heterogeneity (I2 = 0.0%, P = 0.464) (Figure 5). No evidence of publication bias was observed (Egger's test P = 0.568) (Supplementary Figure 4).
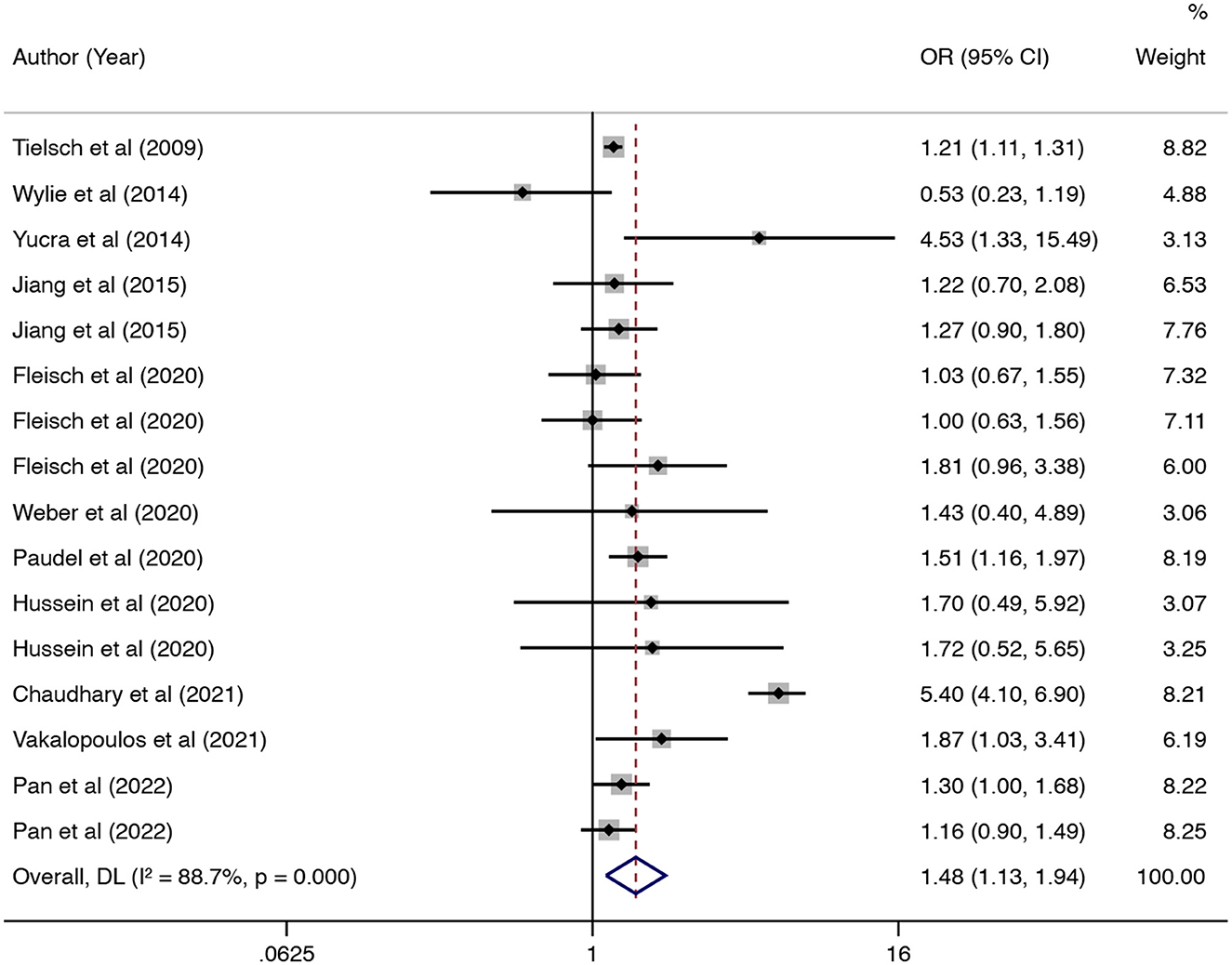
Figure 3. Forest plot showing the effect of polluting cooking fuels on small for gestational age (SGA).
Subgroup and meta-regression analyses
To explore possible sources of heterogeneity across eligible studies, we performed subgroup analyses for LBW and SGA (Table 2). Notably, the association between polluting cooking fuels and the risk of LBW was consistent in all subgroups examined, except for the subgroup conducted according to fuel types. In the subgroup analysis of cooking fuel type, biomass (OR: 1.57; 95% CI: 1.34–1.84) and fossil fuels (OR: 1.49; 95% CI: 1.10–2.02), but not mixed fuels (OR: 1.14; 95% CI: 0.98–1.32), were associated with LBW. Subgroup analysis based on location, the association between polluting cooking fuels, and risk of SGA was significant in Asia (OR: 1.47; 95% CI: 1.03–2.10), not in America (OR: 1.37; 95% CI: 0.87–2.18) or Africa (OR: 1.61; 95% CI: 0.79–3.28). In the subgroup analysis of study design, the association was significant in cohort studies (OR: 1.21; 95% CI: 1.13–1.30) not in cross-sectional studies (OR: 1.77; 95% CI: 0.73–4.32). Among cooking fuel types, both biomass (OR: 1.22; 95% CI: 1.05–1.42) and fossil fuels (OR: 1.29; 95% CI: 1.05–1.59), but not mixed fuels (OR: 2.40; 95% CI: 0.84–6.88), were associated with SGA.
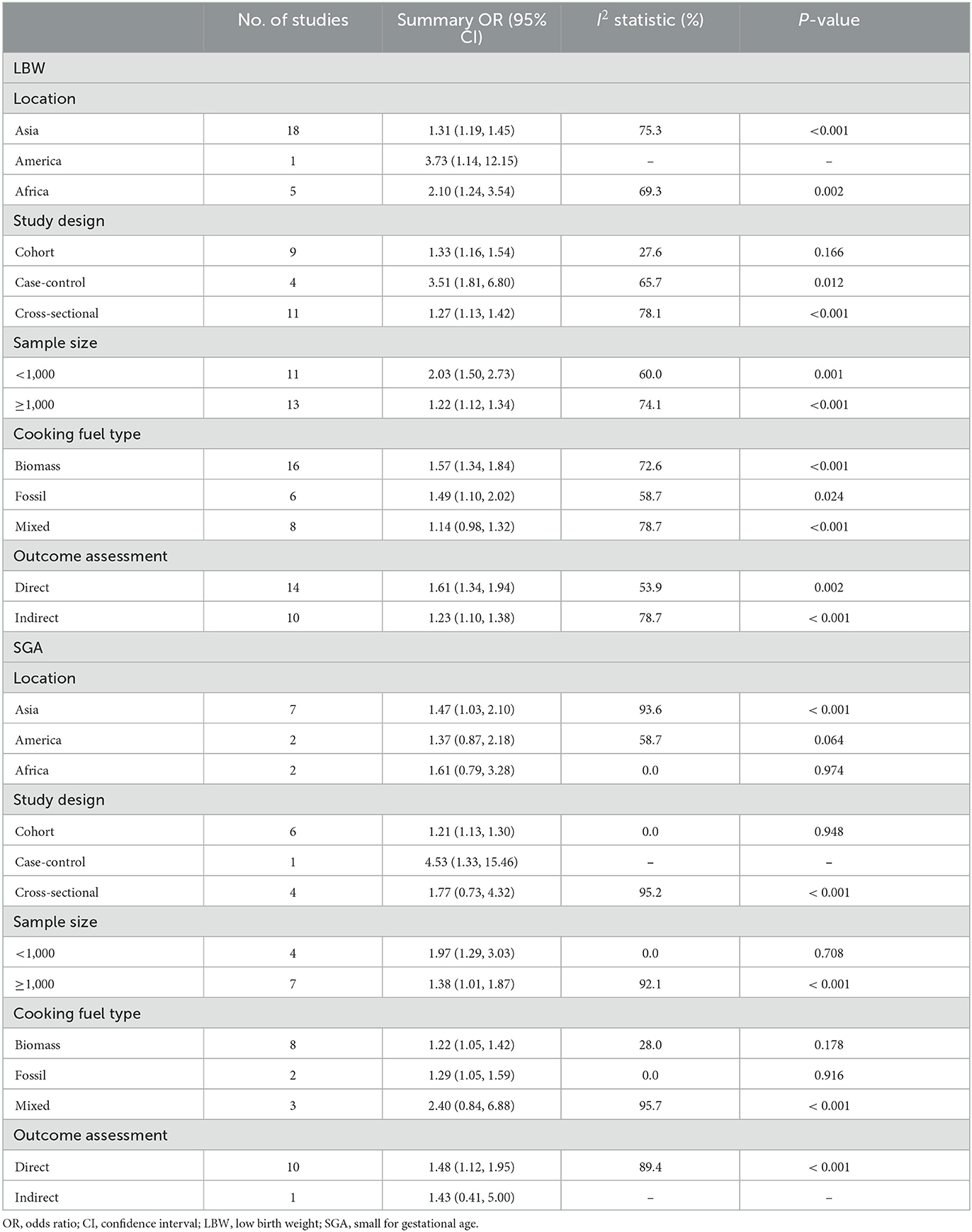
Table 2. Pooled estimates for the association of polluting cooking fuels with LBW and SGA stratified according to the study characteristics.
In the meta-regression analysis of LBW (Supplementary Table 6), we found that study design (cohort study: P < 0.01; cross-sectional study: P < 0.01) and sample size (≥1,000: P < 0.01) were the covariates associated with heterogeneity. Cooking fuel types (mixed fuel: P < 0.05) were covariates associated with the heterogeneity in the SGA analysis.
Sensitivity analysis
Sensitivity analysis results showed that excluding one study at a time did not significantly alter the overall effect of polluting fuel use on LBW (OR altered between 1.33 and 1.41) (Supplementary Table 7) and SGA (OR altered between 1.26 and 1.56) (Supplementary Table 8). Regarding the studies reporting SGA, heterogeneity was greatly reduced when Chaudhary's study (34) was excluded (I2 reduced to 15.9%) (Supplementary Table 8).
Discussion
In a previous meta-analysis, Pope et al. reported that indoor air pollution from solid fuel use was associated with a 38% increased risk of LBW and a 51% increased risk of stillbirth (25). Amegah et al. reported that solid fuel use was associated with a 35% increased risk of LBW, a 30% increased risk of PTB, and a 29% increased risk of stillbirth (26). These results are consistent with ours.
This is the first meta-analysis to summarize the available evidence relating to polluting cooking fuels and SGA, and we found a positive association between them. The combustion of polluting cooking fuels emits high levels of pollutants, such as particulate matter (PM) and nitrogen dioxide (NO2), which have been shown to be associated with SGA in several meta-analyses (74–76). We included 11 relevant studies exploring the effect of polluting cooking fuels on SGA; further eligible studies are needed to verify the association.
In the subgroup analysis, the summary OR varied between different locations for SGA. This may be attributed to the different cooking fuel choices in the studies. For example, the majority of women in South Asia use wood (49.1–89.7%), and most of the women in Africa use charcoal (85.4–93.5%), whereas women in Latin America mainly use liquefied petroleum gas (69.1–97.6%) (77). However, this study only included five studies in South Asia (11, 33–36), two studies in Africa (44, 45), and one study in Latin America (28) to detect the association between polluting fuels and SGA. Therefore, more studies are needed to explore regional differences.
Different fuel types may influence the strength of the association between polluting fuels with LBW and SGA. The results demonstrated that biomass fuels had a larger pooled OR for the association with LBW than fossil fuels. However, when various polluting cooking fuels were grouped together, the association was not significant. Studies have shown that the concentrations of pollutants released from coal are lower than those released from biomass (78, 79). A laboratory assessment has also shown that the concentrations of pollutants released from fossil fuels (including kerosene) are lower than those from biomass (80). Therefore, the pooled OR could be higher between biomass fuels and LBW. However, we found the pooled OR of the association between fossil fuels and SGA was higher than that with biomass fuels. The result was consistent with the included studies (24, 37). In addition, the results of the included studies also showed higher OR values between biomass and LBW than fossil fuels. The two cohort studies were both conducted in China, and more studies are needed to confirm this conclusion and explore the potential biological mechanism. Studies have reported that the concentrations of pollutants are reduced when people use mixed fuels (78, 81). This could be attributed to the occasional use of relatively low polluting fuels (e.g., kerosene) (81), which masked the relationship between one type of polluting cooking fuel (biomass or fossil fuels) and adverse birth outcomes.
There was high heterogeneity in the present study, although the meta-regression analyses found several covariates associated with heterogeneity, which was still uncontrolled after subgroup analyses. Moreover, sensitivity analysis was performed to explore the potential source of heterogeneity in LBW and SGA. However, the source of heterogeneity was not found in LBW. This may be attributed to the number of included studies that were not sufficient to detect the source of heterogeneity. In the future, more qualified original research is needed to explore the source of heterogeneity. For SGA, sensitivity analysis revealed that after excluding Chaudhary's study (34), the heterogeneity was reduced greatly. In this cross-sectional study, mothers of SGA infants had higher rates of exposure to polluting fuels during pregnancy, and mothers with appropriate size for gestational age infants had lower rates of exposure. Therefore, the population with different exposure rates may be a source of heterogeneity. Furthermore, the adjustment for different covariates may be a source of heterogeneity. Compared with other studies, Chaudhary's study had additionally adjusted high carbohydrate snacks, pregnancy-induced hypertension, gestational diabetes, cardiovascular diseases, polyhydramnios, hypothyroid, and anemia.
Combustion of polluting cooking fuels emits high levels of pollutants, such as PM, CO, NO2, sulfur dioxide (SO2), and PAHs (37). Exposure to PM induces maternal systemic and placental oxidative stress and inflammation (82), which could result in suboptimal placentation, and subsequent fetal growth restriction (83). It is known that PAHs are linked to developmental and reproductive toxicity (84). They can result in inadequate transplacental nutrient exchange (85). Carbon monoxide binds to hemoglobin to form carboxyhemoglobin (86), which leads to inadequate oxygen supply to the fetus and hence fetal growth retardation (35). Moreover, it may cross the placental barrier, where it can act directly to affect fetal health and development (44). In short, both PM and toxic chemicals affect the growth and development of the fetus through various mechanisms, leading to adverse birth outcomes.
The current study had some strengths. In this updated meta-analysis, we provided the first quantitative assessment of the association between polluting cooking fuels and SGA. Compared with the previous study (26), this meta-analysis included 23 new studies and conducted subgroup and meta-regression analyses for LBW and SGA. In addition, we explored whether different types of polluting fuels use had a different impact on the risk of adverse birth outcomes.
Study limitations and future perspectives
Our study also had some limitations. First, because of the observational nature of included studies, recall and selection bias cannot be eliminated. Although cohort studies are less vulnerable to such bias, only 11 cohort studies were included in the final analysis. In addition, cross-sectional and case–control studies have difficulties in determining the temporality between exposure and outcome. Consequently, our current results should be interpreted cautiously, and more prospective studies are needed to thoroughly investigate the association between polluting cooking fuels and adverse birth outcomes. Second, there is a possibility of misclassification of exposure and outcome assessment. All included studies collected information regarding primary cooking fuels through interviews. However, there is considerable variability in exposure, such as multiple fuel use and temporal changes in fuel use. Using only qualitative indicators (such as reported cooking fuel use) could produce considerable exposure misclassification for exposure settings and inaccurate correlation assessments between exposure and outcomes. By directly measuring and recording the concentration of kitchen air pollutants, we can avoid misclassification of exposures and obtain accurate exposure–response relationships. However, only three of included studies directly measured the concentration of kitchen air pollutants (28, 38, 40). It is important for future studies to employ more accurate methods, such as household or portable air quality monitors, to assess household air pollutant exposure. Moreover, the baby's birth size was used as a proxy for birth weight when the information could not be retrieved from a health card or maternal recall. However, previous findings demonstrated that the demographic and health survey data on birth size could be used as an alternative to birth weight (23). Third, although some potential confounding factors were taken into account in the original studies, other confounding factors, such as the type of cooking stoves, personal exposure, and the availability of windows or chimneys, still remained uncontrolled in some studies. A study conducted in Indonesia demonstrated that pollutant concentrations remained high even when the houses were adequately ventilated (31); however, the residual confounding effects of other important factors could not be eliminated, which might cause inaccurate evaluations of their effects on the risk of adverse birth outcomes. Finally, there was considerable heterogeneity in the current study. After conducting subgroup and meta-regression analyses, the heterogeneity was still uncontrolled. Moreover, sensitivity analysis suggested that no individual study significantly affected the pooled effect size. Therefore, the heterogeneity is the cause for caution concerning the conclusion. Strong epidemiological evidence estimating health risks from particular fuels and technologies utilized, and improved and standardized data collection capturing the fuels and technologies used in the home for all key end-uses, such as cooking, heating, and lighting, are needed. The understanding of exposure to total household air pollution will be improved through increased monitoring efforts combined with future modeling that takes stove stacking into account. This will better inform policy and programmatic decision-making as well as the global monitoring of health and environmental impacts.
Conclusion
Our findings suggest that polluting cooking fuel use is associated with an increased risk of LBW, SGA, stillbirth, and PTB. Different polluting fuel types may influence the strength of the association between polluting fuel use and LBW and SGA. Moving forward, we encourage more prospective studies to thoroughly investigate the association between polluting cooking fuel use and adverse birth outcomes. On exposure assessment, a more direct method of measuring, such as household or portable air quality monitors, will help quantify exposure information and an exposure–response relationship.
Data availability statement
The original contributions presented in the study are included in the article/Supplementary material, further inquiries can be directed to the corresponding authors.
Author contributions
ML: conceptualization, data curation, software, formal analysis, and writing—original draft. TL, CM, JF, ZZ, and YW: writing—reviewing and editing. YX: writing—reviewing and editing and supervising. YZ: writing—reviewing and editing and funding acquisition. CJ: conceptualization, writing—reviewing and editing, supervision, and funding acquisition. All authors contributed to the article and approved the submitted version.
Funding
This study was supported by the JieBangGuaShuai Project of Liaoning Province (2021JH1/10400050), Precise prevention, diagnosis, and treatment for metabolic diseases, the National Key Research and Development Program of China (grant number 2017YFC0907403), the Projects of Shenyang Science and Technology Bureau (grant number 20-205-4-029n), Doctoral Research Foundation Project of Liaoning Province (grant number 2022-BS-130), and the 345 Talent Project of Shengjing Hospital of China Medical University (grant numbers M0700 and M0937).
Conflict of interest
The authors declare that the research was conducted in the absence of any commercial or financial relationships that could be construed as a potential conflict of interest.
Publisher's note
All claims expressed in this article are solely those of the authors and do not necessarily represent those of their affiliated organizations, or those of the publisher, the editors and the reviewers. Any product that may be evaluated in this article, or claim that may be made by its manufacturer, is not guaranteed or endorsed by the publisher.
Supplementary material
The Supplementary Material for this article can be found online at: https://www.frontiersin.org/articles/10.3389/fpubh.2023.978556/full#supplementary-material
References
1. Luo J, Aschebrook-Kilfoy B, Olopade CO. Maps of solid-fuel use and household air pollution. Lancet Glob Health. (2022) 10:e1361–e2. doi: 10.1016/S2214-109X(22)00386-2
2. Santos UP, Arbex MA, Braga ALF, Mizutani RF, Cançado JED, Terra-Filho M, et al. environmental air pollution: respiratory effects. J Bras Pneumol. (2021) 47:e20200267. doi: 10.36416/1806-3756/e20200267
3. Chowdhury S, Chafe ZA, Pillarisetti A, Lelieveld J, Guttikunda S, Dey S. The Contribution of Household Fuels to Ambient Air Pollution in India—A Comparison of Recent Estimates. (2019). Available online at: https://ccapc.org.in/policy-briefs/2019/5/30/the-contribution-of-household-fuels-to-ambient-air-pollution-in-india-a-comparison-of-recent-estimates (accessed January 16, 2023).
4. Islam MA, Hasan MN, Ahammed T, Anjum A, Majumder A, Siddiqui MN, et al. Association of household fuel with acute respiratory infection (Ari) under-five years Children in Bangladesh. Front Public Health. (2022) 10:985445. doi: 10.3389/fpubh.2022.985445
5. Stoner O, Lewis J, Martínez IL, Gumy S, Economou T, Adair-Rohani H. Household cooking fuel estimates at global and country level for 1990 to 2030. Nat Commun. (2021) 12:5793. doi: 10.1038/s41467-021-26036-x
6. IEA IRENA UNSD World Bank WHO. Tracking SDG7 The Energy Progress Report. (2022). Available online at: https://trackingsdg7.esmap.org/data/files/download-documents/sdg7-report2022-full_report.pdf (accessed January 16, 2023).
7. Dhital S, Rupakheti D, Rupakheti M, Yin X, Liu Y, Mafiana JJ, et al. A scientometric analysis of indoor air pollution research during 1990–2019. J Environ Manage. (2022) 320:115736. doi: 10.1016/j.jenvman.2022.115736
8. Lee KK, Bing R, Kiang J, Bashir S, Spath N, Stelzle D, et al. Adverse health effects associated with household air pollution: a systematic review, meta-analysis, and burden estimation study. Lancet Global Health. (2020) 8:e1427–e34. doi: 10.1016/S2214-109X(20)30343-0
9. Mishra V, Retherford RD, Smith KR. Cooking smoke and tobacco smoke as risk factors for stillbirth. Int J Environ Health Res. (2005) 15:397–410. doi: 10.1080/09603120500288913
10. Siddiqui AR, Gold EB, Yang X, Lee K, Brown KH, Bhutta ZA. Prenatal exposure to wood fuel smoke and low birth weight. Environ Health Perspect. (2008) 116:543–9. doi: 10.1289/ehp.10782
11. Tielsch JM, Katz J, Thulasiraj RD, Coles CL, Sheeladevi S, Yanik EL, et al. Exposure to indoor biomass fuel and tobacco smoke and risk of adverse reproductive outcomes, mortality, respiratory morbidity and growth among newborn infants in South India. Int J Epidemiol. (2009) 38:1351–63. doi: 10.1093/ije/dyp286
12. Rao X, Zhong J, Brook RD, Rajagopalan S. Effect of particulate matter air pollution on cardiovascular oxidative stress pathways. Antioxid Redox Signal. (2018) 28:797–818. doi: 10.1089/ars.2017.7394
13. Al-Kindi SG, Brook RD, Biswal S, Rajagopalan S. Environmental determinants of cardiovascular disease: lessons learned from air pollution. Nat Rev Cardiol. (2020) 17:656–72. doi: 10.1038/s41569-020-0371-2
14. Schraufnagel DE, Balmes JR, Cowl CT, De Matteis S, Jung SH, Mortimer K, et al. Air pollution and noncommunicable diseases: a review by the forum of international respiratory societies' environmental committee, part 1: the damaging effects of air pollution. Chest. (2019) 155:409–16. doi: 10.1016/j.chest.2018.10.042
15. Price JT, Vwalika B, Rittenhouse KJ, Mwape H, Winston J, Freeman BL, et al. Adverse birth outcomes and their clinical phenotypes in an urban Zambian cohort. Gates Open Res. (2020) 3:1533. doi: 10.12688/gatesopenres.13046.2
16. Lee AC, Kozuki N, Cousens S, Stevens GA, Blencowe H, Silveira MF, et al. Estimates of burden and consequences of infants born small for gestational age in low and middle income countries with intergrowth-21st standard: analysis of Cherg datasets. BMJ. (2017) 358:j4229. doi: 10.1136/bmj.j4229
17. Liu L, Oza S, Hogan D, Chu Y, Perin J, Zhu J, et al. Global, regional, and national causes of under-5 mortality in 2000–15: an updated systematic analysis with implications for the sustainable development goals. Lancet. (2016) 388:3027–35. doi: 10.1016/S0140-6736(16)31593-8
18. Grazuleviciene R, Danileviciute A, Nadisauskiene R, Vencloviene J. Maternal smoking, gstm1 and gstt1 polymorphism and susceptibility to adverse pregnancy outcomes. Int J Environ Res Public Health. (2009) 6:1282–97. doi: 10.3390/ijerph6031282
19. Fekadu Dadi A, Miller ER, Mwanri L. Antenatal depression and its association with adverse birth outcomes in low and middle-income countries: a systematic review and meta-analysis. PLoS ONE. (2020) 15:e0227323. doi: 10.1371/journal.pone.0227323
20. Degno S, Lencha B, Aman R, Atlaw D, Mekonnen A, Woldeyohannes D, et al. Adverse birth outcomes and associated factors among mothers who delivered in bale zone hospitals, Oromia region, Southeast Ethiopia. J Int Med Res. (2021) 49:03000605211013209. doi: 10.1177/03000605211013209
21. Yang J, Wang M, Tobias DK, Rich-Edwards JW, Darling AM, Abioye AI, et al. Dietary diversity and diet quality with gestational weight gain and adverse birth outcomes, results from a prospective pregnancy cohort study in Urban Tanzania. Matern Child Nutr. (2022) 18:e13300. doi: 10.1111/mcn.13300
22. Nieuwenhuijsen MJ, Dadvand P, Grellier J, Martinez D, Vrijheid M. Environmental risk factors of pregnancy outcomes: a summary of recent meta-analyses of epidemiological studies. Environ Health. (2013) 12:6. doi: 10.1186/1476-069X-12-6
23. Islam S, Mohanty SK. Maternal exposure to cooking smoke and risk of low birth weight in India. Sci Total Environ. (2021) 774:145717. doi: 10.1016/j.scitotenv.2021.145717
24. Pan D, Liu S, Huang D, Zeng X, Zhang Y, Pang Q, et al. Effects of household environmental exposure and ventilation in association with adverse birth outcomes: a prospective cohort study in rural China. Sci Total Environ. (2022) 822:153519. doi: 10.1016/j.scitotenv.2022.153519
25. Pope DP, Mishra V, Thompson L, Siddiqui AR, Rehfuess EA, Weber M, et al. Risk of low birth weight and stillbirth associated with indoor air pollution from solid fuel use in developing countries. Epidemiol Rev. (2010) 32:70–81. doi: 10.1093/epirev/mxq005
26. Amegah AK, Quansah R, Jaakkola JJ. Household air pollution from solid fuel use and risk of adverse pregnancy outcomes: a systematic review and meta-analysis of the empirical evidence. PLoS ONE. (2014) 9:e113920. doi: 10.1371/journal.pone.0113920
27. Patel AB, Meleth S, Pasha O, Goudar SS, Esamai F, Garces AL, et al. Impact of exposure to cooking fuels on stillbirths, perinatal, very early and late neonatal mortality—a multicenter prospective cohort study in rural communities in India, Pakistan, Kenya, Zambia and Guatemala. Matern Health Neonatol Perinatol. (2015) 1:18. doi: 10.1186/s40748-015-0019-0
28. Yucra S, Tapia V, Steenland K, Naeher LP, Gonzales GF. Maternal exposure to biomass smoke and carbon monoxide in relation to adverse pregnancy outcome in two high altitude cities of Peru. Environ Res. (2014) 130:29–33. doi: 10.1016/j.envres.2014.01.008
29. Demelash H, Motbainor A, Nigatu D, Gashaw K, Melese A. Risk factors for low birth weight in bale zone hospitals, South–East Ethiopia: a case-control study. BMC Pregnancy Childbirth. (2015) 15:264. doi: 10.1186/s12884-015-0677-y
30. Haider MR, Rahman MM, Islam F, Khan MM. Association of low birthweight and indoor air pollution: biomass fuel use in Bangladesh. J Health Pollut. (2016) 6:18–25. doi: 10.5696/2156-9614-6-11.18
31. Suryadhi MAH, Abudureyimu K, Kashima S, Yorifuji T. Effects of household air pollution from solid fuel use and environmental tobacco smoke on child health outcomes in Indonesia. J Occup Environ Med. (2019) 61:335–9. doi: 10.1097/JOM.0000000000001554
32. Gurung A, Wrammert J, Sunny AK, Gurung R, Rana N, Basaula YN, et al. Incidence, risk factors and consequences of preterm birth—findings from a multi-centric observational study for 14 months in Nepal. Arch Public Health. (2020) 78:64. doi: 10.1186/s13690-020-00446-7
33. Gautam Paudel P, Sunny AK, Gurung R, Gurung A, Malla H, Budhathoki SS, et al. Prevalence, risk factors and consequences of newborns born small for gestational age: a multisite study in Nepal. BMJ Paediatr Open. (2020) 4:e000607. doi: 10.1136/bmjpo-2019-000607
34. Chaudhary N, Yadav SN, Kalra SK, Pathak S, Gupta BK, Shrestha S, et al. Prognostic factors associated with small for gestational age babies in a tertiary care hospital of western Nepal: a cross-sectional study. Health Sci Rep. (2021) 4:e250. doi: 10.1002/hsr2.250
35. Vakalopoulos A, Dharmage SC, Dharmaratne S, Jayasinghe P, Lall O, Ambrose I, et al. Household air pollution from biomass fuel for cooking and adverse fetal growth outcomes in rural Sri Lanka. Int J Environ Res Public Health. (2021) 18:1878. doi: 10.3390/ijerph18041878
36. Wylie BJ, Coull BA, Hamer DH, Singh MP, Jack D, Yeboah-Antwi K, et al. Impact of biomass fuels on pregnancy outcomes in central East India. Environ Health. (2014) 13:1. doi: 10.1186/1476-069X-13-1
37. Jiang M, Qiu J, Zhou M, He X, Cui H, Lerro C, et al. Exposure to cooking fuels and birth weight in Lanzhou, China: a birth cohort study. BMC Public Health. (2015) 15:712. doi: 10.1186/s12889-015-2038-1
38. Mukherjee S, Siddique S, Chakraborty S, Bhattacharya P, Banerjee M, Roychoudhury S, et al. Adverse reproductive health outcomes in pre-menopausal indian women chronically exposed to biomass smoke. J Public Health. (2015) 23:363–72. doi: 10.1007/s10389-015-0690-7
39. Khan MN CZ BN, Mofizul Islam M, Islam MR, Rahman MM. Household air pollution from cooking and risk of adverse health and birth outcomes in Bangladesh: a nationwide population-based study. Environ Health. (2017) 16:57. doi: 10.1186/s12940-017-0272-y
40. Balakrishnan K, Ghosh S, Thangavel G, Sambandam S, Mukhopadhyay K, Puttaswamy N, et al. Exposures to fine particulate matter (PM[[sb]]25[[/s]]) and birthweight in a rural-urban, mother-child cohort in Tamil Nadu, India. Environ Res. (2018) 161:524–31. doi: 10.1016/j.envres.2017.11.050
41. Nisha MK, Alam A, Raynes-Greenow C. Variations in perinatal mortality associated with different polluting fuel types and kitchen location in Bangladesh. Int J Occup Environ Health. (2018) 24:47–54. doi: 10.1080/10773525.2018.1507868
42. Fleisch AF, Seshasayee SM, Garshick E, Chipman JW, Koutrakis P, Baker ER, et al. Assessment of maternal glycemia and newborn size among pregnant women who use wood stoves in Northern New England. JAMA Netw Open. (2020) 3:e206046. doi: 10.1001/jamanetworkopen.2020.6046
43. Basel CA. Singh S. Low birth weight and its associated risk factors: health facility-based case-control study. PLoS ONE. (2020) 15:e0234907. doi: 10.1371/journal.pone.0234907
44. Weber E, Adu-Bonsaffoh K, Vermeulen R, Klipstein-Grobusch K, Grobbee DE, Browne JL, et al. Household fuel use and adverse pregnancy outcomes in a Ghanaian cohort study. Reprod Health. (2020) 17:29. doi: 10.1186/s12978-020-0878-3
45. Hussein H, Shamsipour M, Yunesian M, Hasanvand MS, Fotouhi A. Association of adverse birth outcomes with exposure to fuel type use: a prospective cohort study in the Northern Region of Ghana. Heliyon. (2020) 6:e04169. doi: 10.1016/j.heliyon.2020.e04169
46. Kanno GG, Anbesse AT, Shaka MF, Legesse MT, Andarge SD. Effect of biomass fuel use and kitchen location on maternal report of birth size: cross-sectional analysis of 2016 Ethiopian demographic health survey data. Public Health Pract. (2021) 2:100211. doi: 10.1016/j.puhip.2021.100211
47. Lu C, Xiao F, Yang X, Zhang YP Li BZ, Zhao ZH, et al. Long-term exposure to mould/damp stains and mouldy odour increases low birth weight. Build Environ. (2022) 222:109418. doi: 10.1016/j.buildenv.2022.109418
48. Younger A, Alkon A, Harknett K, Jean Louis R, Thompson LM. Adverse birth outcomes associated with household air pollution from unclean cooking fuels in low- and middle-income countries: a systematic review. Environ Res. (2022) 204:112274. doi: 10.1016/j.envres.2021.112274
49. Moher D, Liberati A, Tetzlaff J, Altman DG, Group P. Preferred reporting items for systematic reviews and meta-analyses: the prisma statement. BMJ. (2009) 339:b2535. doi: 10.1136/bmj.b2535
51. Thompson LM, Bruce N, Eskenazi B, Diaz A, Pope D, Smith KR. Impact of reduced maternal exposures to wood smoke from an introduced chimney stove on newborn birth weight in rural Guatemala. Environ Health Perspect. (2011) 119:1489–94. doi: 10.1289/ehp.1002928
52. Mavalankar DV, Trivedi CR, Gray RH. Levels and risk-factors for perinatal-mortality in Ahmedabad, India. Bull World Health Organ. (1991) 69:435–42.
53. Mavalankar DV, Gray RH, Trivedi C. Risk factors for preterm and term low birthweight in Ahmedabad, India. Int J Epidemiol. (1992) 21:263–72. doi: 10.1093/ije/21.2.263
54. Li Z, Zhang L, Ye R, Pei L, Liu J, Zheng X, et al. Indoor air pollution from coal combustion and the risk of neural tube defects in a rural population in Shanxi Province, China. Am J Epidemiol. (2011) 174:451–8. doi: 10.1093/aje/kwr108
55. Samaraweera Y, Abeysena C. Maternal sleep deprivation, sedentary lifestyle and cooking smoke: risk factors for miscarriage: a case control study. Aust N Z J Obstet Gynaecol. (2010) 50:352–7. doi: 10.1111/j.1479-828X.2010.01190.x
56. Boy E, Bruce N, Delgado H. Birth weight and exposure to kitchen wood smoke during pregnancy in rural Guatemala. Environ Health Perspect. (2002) 110:109–14. doi: 10.1289/ehp.02110109
57. Mishra V, Dai X, Smith KR, Mika L. Maternal exposure to biomass smoke and reduced birth weight in Zimbabwe. Ann Epidemiol. (2004) 14:740–7. doi: 10.1016/j.annepidem.2004.01.009
58. Lakshmi PV, Virdi NK, Sharma A, Tripathy JP, Smith KR, Bates MN, et al. Household air pollution and stillbirths in India: analysis of the Dlhs-Ii national survey. Environ Res. (2013) 121:17–22. doi: 10.1016/j.envres.2012.12.004
59. Stanković A, Mitrović V, Živadinović R. Influence of air pollution on birth weight. Srp Arh Celok Lek. (2011) 139:651–6. doi: 10.2298/SARH1110651S
60. Stanković A, Mitrović V, Živadinović R. Influence of air pollution on pregnant women's health and pregnancy outcomes. Med Pregl. (2011) 64:279–84. doi: 10.2298/MPNS1106279S
61. Stang A. Critical evaluation of the Newcastle–Ottawa scale for the assessment of the quality of nonrandomized studies in meta-analyses. Eur J Epidemiol. (2010) 25:603–5. doi: 10.1007/s10654-010-9491-z
62. Yuhara H, Steinmaus C, Cohen SE, Corley DA, Tei Y, Buffler PA. Is diabetes mellitus an independent risk factor for colon cancer and rectal cancer? Am J Gastroenterol. (2011) 106:1911–21. doi: 10.1038/ajg.2011.301
63. Liu YS, Wu QJ, Xia Y, Zhang JY, Jiang YT, Chang Q, et al. Carbohydrate intake and risk of metabolic syndrome: a dose-response meta-analysis of observational studies. Nutr Metab Cardiovasc Dis. (2019) 29:1288–98. doi: 10.1016/j.numecd.2019.09.003
64. Hu J, Dong Y, Chen X, Liu Y, Ma D, Liu X, et al. Prevalence of suicide attempts among chinese adolescents: a meta-analysis of cross-sectional studies. Compr Psychiatry. (2015) 61:78–89. doi: 10.1016/j.comppsych.2015.05.001
65. Van Gerwen OT, Craig-Kuhn MC, Jones AT, Schroeder JA, Deaver J, Buekens P, et al. Trichomoniasis and adverse birth outcomes: a systematic review and meta-analysis. BJOG. (2021) 128:1907–15. doi: 10.1111/1471-0528.16774
66. Cummings P. The relative merits of risk ratios and odds ratios. Arch Pediatr Adolesc Med. (2009) 163:438–45. doi: 10.1001/archpediatrics.2009.31
67. Higgins JP, Thomas J, Chandler J, Cumpston M, Li T, Page MJ, et al. (2019) Cochrane Handbook for Systematic Reviews of Interventions. Chichester: John Wiley and Sons. doi: 10.1002/9781119536604
68. Siddiqui A, Gold E, Brown K, Lee K, Bhutta Z. Preliminary Analyses of Indoor Air Pollution and Low Birth Weight (LBW) in Southern Pakistan. Indoor air pollution from solid fuels and risk of low birth weight and stillbirth Report from a symposium held at the Annual Conference of the International Society for Environmental Epidemiology (ISEE): 2005. Johannesburg, South Africa (2005). doi: 10.1097/00001648-200509000-00215
69. Sreeramareddy CT, Shidhaye RR, Sathiakumar N. Association between biomass fuel use and maternal report of child size at birth—an analysis of 2005-06 India demographic health survey data. BMC Public Health. (2011) 11:403. doi: 10.1186/1471-2458-11-403
70. Abusalah A, Gavana M, Haidich AB, Smyrnakis E, Papadakis N, Papanikolaou A, et al. Low birth weight and prenatal exposure to indoor pollution from tobacco smoke and wood fuel smoke: a matched case-control study in Gaza strip. Matern Child Health J. (2012) 16:1718–27. doi: 10.1007/s10995-011-0851-4
71. Epstein MB, Bates MN, Arora NK, Balakrishnan K, Jack DW, Smith KR. Household fuels, low birth weight, and neonatal death in India: the separate impacts of biomass, kerosene, and coal. Int J Hyg Environ Health. (2013) 216:523–32. doi: 10.1016/j.ijheh.2012.12.006
72. Yucra S, Tapia V, Steenland K, Naeher LP, Gonzales GF. Association between biofuel exposure and adverse birth outcomes at high altitudes in Peru: a matched case-control study. Int J Occup Environ Health. (2011) 17:307–13. doi: 10.1179/oeh.2011.17.4.307
73. Amegah AK, Jaakkola JJ, Quansah R, Norgbe GK, Dzodzomenyo M. Cooking fuel choices and garbage burning practices as determinants of birth weight: a cross-sectional study in Accra, Ghana. Environ Health. (2012) 11:78. doi: 10.1186/1476-069X-11-78
74. Guo LQ, Chen Y, Mi BB, Dang SN, Zhao DD, Liu R, et al. Ambient air pollution and adverse birth outcomes: a systematic review and meta-analysis. J Zhejiang Univ Sci B. (2019) 20:238–52. doi: 10.1631/jzus.B1800122
75. Yuan L, Zhang Y, Gao Y, Tian Y. Maternal fine particulate matter (PM[[sb]]25[[/s]]) exposure and adverse birth outcomes: an updated systematic review based on cohort studies. Environ Sci Pollut Res Int. (2019) 26:13963–83. doi: 10.1007/s11356-019-04644-x
76. Pun VC, Dowling R, Mehta S. Ambient and household air pollution on early-life determinants of stunting—a systematic review and meta-analysis. Environ Sci Pollut Res Int. (2021) 28:26404–12. doi: 10.1007/s11356-021-13719-7
77. Kadir MM, McClure EM, Goudar SS, Garces AL, Moore J, Onyamboko M, et al. Exposure of pregnant women to indoor air pollution: a study from nine low and middle income countries. Acta Obstet Gynecol Scand. (2010) 89:540–8. doi: 10.3109/00016340903473566
78. Edwards RD, Liu Y, He G, Yin Z, Sinton J, Peabody J, et al. Household Co and Pm measured as part of a review of China's national improved stove program. Indoor Air. (2007) 17:189–203. doi: 10.1111/j.1600-0668.2007.00465.x
79. Mestl HE, Aunan K, Seip HM, Wang S, Zhao Y, Zhang D. Urban and rural exposure to indoor air pollution from domestic biomass and coal burning across China. Sci Total Environ. (2007) 377:12–26. doi: 10.1016/j.scitotenv.2007.01.087
80. Bilsback KR, Dahlke J, Fedak KM, Good N, Hecobian A, Herckes P, et al. A laboratory assessment of 120 air pollutant emissions from biomass and fossil fuel cookstoves. Environ Sci Technol. (2019) 53:7114–25. doi: 10.1021/acs.est.8b07019
81. Titcombe ME. Simcik M. Personal and indoor exposure to PM[[sb]]25[[/s]] and polycyclic aromatic hydrocarbons in the Southern highlands of Tanzania: a pilot-scale study. Environ Monit Asses. (2011) 180:461–76. doi: 10.1007/s10661-010-1799-3
82. Johnson NM, Hoffmann AR, Behlen JC, Lau C, Pendleton D, Harvey N, et al. Air pollution and children's health-a review of adverse effects associated with prenatal exposure from fine to ultrafine particulate matter. Environ Health Prev Med. (2021) 26:72. doi: 10.1186/s12199-021-00995-5
83. van den Hooven EH, Pierik FH, de Kluizenaar Y, Hofman A, van Ratingen SW, Zandveld PY, et al. Air pollution exposure and markers of placental growth and function: the generation R study. Environ Health Perspect. (2012) 120:1753–9. doi: 10.1289/ehp.1204918
84. Yin S, Tang M, Chen F, Li T, Liu W. Environmental exposure to polycyclic aromatic hydrocarbons (Pahs): the correlation with and impact on reproductive hormones in umbilical cord serum. Environ Pollut. (2017) 220:1429–37. doi: 10.1016/j.envpol.2016.10.090
85. Saha P, Johny E, Dangi A, Shinde S, Brake S, Eapen MS, et al. Impact of maternal air pollution exposure on children's lung health: an Indian perspective. Toxics. (2018) 6:68. doi: 10.3390/toxics6040068
Keywords: cooking fuels, low birth weight, meta-analysis, preterm birth, small for gestational age, stillbirth
Citation: Luo M, Liu T, Ma C, Fang J, Zhao Z, Wen Y, Xia Y, Zhao Y and Ji C (2023) Household polluting cooking fuels and adverse birth outcomes: An updated systematic review and meta-analysis. Front. Public Health 11:978556. doi: 10.3389/fpubh.2023.978556
Received: 26 June 2022; Accepted: 06 February 2023;
Published: 03 March 2023.
Edited by:
Fabrizio Bianchi, National Research Council (CNR), ItalyReviewed by:
Hong Liang, Shanghai Institute for Biomedical and Pharmaceutical Technologies, ChinaLu Ma, Wuhan University, China
Prosun Bhattacharya, Royal Institute of Technology, Sweden
Copyright © 2023 Luo, Liu, Ma, Fang, Zhao, Wen, Xia, Zhao and Ji. This is an open-access article distributed under the terms of the Creative Commons Attribution License (CC BY). The use, distribution or reproduction in other forums is permitted, provided the original author(s) and the copyright owner(s) are credited and that the original publication in this journal is cited, in accordance with accepted academic practice. No use, distribution or reproduction is permitted which does not comply with these terms.
*Correspondence: Yuhong Zhao, emhhb3l1aG9uZ0Bzai1ob3NwaXRhbC5vcmc=; Chao Ji, amljaGFvQGNtdS5lZHUuY24=
†These authors have contributed equally to this work