- 1Department of Laboratory Medicine, Ruijin Hospital, Shanghai Jiao Tong University School of Medicine, Shanghai, China
- 2Department of Clinical Microbiology, Ruijin Hospital, Shanghai Jiao Tong University School of Medicine, Shanghai, China
- 3Department of Clinical Laboratory, Xiangya Hospital, Central South University, Changsha, Hunan, China
Background: Pseudomonas aeruginosa (P. aeruginosa) accounts for high antimicrobial resistance and mortality rates of bloodstream infections (BSIs). We aim to investigate incidence, antimicrobial resistance and risk factors for mortality of P. aeruginosa BSIs among inpatients.
Methods: A retrospective cohort study were conducted at two tertiary hospitals in 2017–2021. Medical and laboratory records of all inpatients diagnosed with P. aeruginosa BSIs were reviewed. A generalized linear mixed model was used to identify risk factors for mortality.
Results: A total of 285 patients with P. aeruginosa BSIs were identified. Incidence of P. aeruginosa BSIs fluctuated between 2.37 and 3.51 per 100,000 patient-days over the study period. Out of 285 P. aeruginosa isolates, 97 (34.04%) were carbapenem-resistant (CR) and 75 (26.32%) were multidrug-resistant (MDR). These isolates showed low resistance to aminoglycosides (9.51–11.62%), broad-spectrum cephalosporins (17.19–17.61%), fluoroquinolones (17.25–19.43%), and polymyxin B (1.69%). The crude 30-day mortality rate was 17.89% (51/285). Healthcare costs of patients with MDR/CR isolates were significantly higher than those of patients with non-MDR/CR isolates (P < 0.001/=0.002). Inappropriate definitive therapy [adjusted odds ratio (aOR) 4.47, 95% confidence interval (95% CI) 1.35–14.77; P = 0.014], ICU stay (aOR 2.89, 95% CI: 1.26–6.63; P = 0.012) and corticosteroids use (aOR 2.89, 95% CI: 1.31–6.41; P = 0.009) were independently associated with 30-day mortality.
Conclusion: Incidence of P. aeruginosa BSIs showed an upward trend during 2017–2020 but dropped in 2021. MDR/CR P. aeruginosa BSIs are associated with higher healthcare costs. Awareness is required that patients with inappropriate definitive antimicrobial therapy, ICU stay and corticosteroids use are at higher risk of death from P. aeruginosa BSIs.
Introduction
Bloodstream infections (BSIs) are common fatal nosocomial infections and pose a significant healthcare issue as they are associated with increased risk of sepsis, hospitalization, healthcare costs and mortality (1, 2). Pseudomonas aeruginosa (P. aeruginosa) accounts for 5.90–15.78% of gram-negative BSIs worldwide (3, 4). A 13-year prospective cohort study conducted in US reported that P. aeruginosa was responsible for 5.90% of bacterial BSIs (4). BSIs caused by P. aeruginosa isolates are typically difficult to treat due to remarkable intrinsic antimicrobial resistance and ability to acquire resistance to multiple categories of antimicrobial agents (5). P. aeruginosa BSIs were associated with increased mortality relative to Staphylococcus aureus or other Gram-negative BSIs and this effect persisted after adjustment for patient, bacterial and treatment factors (4). Over the past decade, the overall mortality rates due to P. aeruginosa BSIs ranged between 1.38 and 37.30% (2, 6–12). These studies have revealed that the epidemiology of P. aeruginosa BSIs varies geographically.
According to the published data from Blood Bacterial Resistant Investigation Collaborative System in China, P. aeruginosa was responsible for 5.33% of gram-negative BSIs and the proportion showed an upward trend (13), with a mortality rate ranging from 26.8 to 28.4% (2, 9). There are few published papers on P. aeruginosa BSIs in China over the past decade (2, 9, 14, 15). Moreover, recent studies addressing risk factors for mortality of P. aeruginosa BSIs were either small or with focus on multidrug-resistant or carbapenem resistant isolates. To date, no comprehensive epidemiology study of P. aeruginosa BSIs has been conducted in Hunan Province and Shanghai. As such, we conducted this study with the following aims: (i) to examine incidence of P. aeruginosa BSIs; (ii) to investigate antimicrobial resistance profile of P. aeruginosa isolates causing BSIs; (iii) to determine risk factors for all-cause 30-day mortality of P. aeruginosa BSIs. Findings of this study will shed light on refining local screening and infection control policies for P. aeruginosa BSIs and thus will prevent further deterioration.
Methods
Ethics
The study was reviewed and approved by the participating hospitals (reference number: 202212318, KY2023-083). The need for informed consent was waived due to the observational retrospective nature of the study. The study was conducted in accordance with the Declaration of Helsinki.
Study design and setting
We performed a retrospective cohort study at two tertiary hospitals between January 1, 2017 and December 31, 2021. Xiangya Hospital is a 3,500-bed hospital located in Changsha, Central China and Ruijin Hospital is a 2,500-bed hospital located in Shanghai, East China. All consecutive hospitalized patients with P. aeruginosa BSIs admitted during the study period were included. Only the first episode of each patient was included and each patient was included only once. Patients with length of hospitalization < 48 h or incomplete data were excluded.
Definitions
P. aeruginosa BSIs were defined as the presence of a positive blood culture of P. aeruginosa with simultaneous clinical signs and symptoms of infections (16). Onset of P. aeruginosa BSIs was defined as the moment of taking the first positive blood culture of P. aeruginosa. A case was defined as a patient diagnosed with P. aeruginosa BSIs. Incidence of P. aeruginosa BSIs was defined as the number of cases per 100,000 patient-days. Nosocomial BSIs were defined as blood samples taken more than 48 h after hospital admission and no presence of any clinical signs or symptoms of infections between hospital admission and onset of P. aeruginosa BSIs (6). Polymicrobial BSIs were defined as recovery of multiple bacterial species from a blood specimen in addition to P. aeruginosa (11). Source of infection was defined as the most possible origin of infection responsible for P. aeruginosa BSIs according to both medical records and US Centers for Disease Control and Prevention guidelines (9, 17), including an “Unknown” origin if no source was identified. Crude 30-day mortality rate was defined as the number of deaths by any cause within 30 days of onset of P. aeruginosa BSIs per 100 cases. Inappropriate empirical antimicrobial therapy was defined as no administration of any anti-pseudomonal agent with in-vitro activity before blood culture report, whereas inappropriate definitive antimicrobial therapy refers to the moment of receiving antimicrobial susceptibility test results (2, 18).
Both hospitals follow international guideline to collect and process blood cultures (19). Identification of P. aeruginosa isolates was performed using MALDITOF MS (bioMérieux, Marcy l'Etoile, France or Zybio Inc., Chongqing, China). Antimicrobial susceptibility test was performed by VITEK®2 Compact (bioMérieux, Marcy l'Etoile, France) (20). The sensitivity of polymyxin B was detected using broth microdilution method. The results were interpreted according to the Clinical and Laboratory Standards Institute guidelines (21). Antibiotics tested included amikacin, gentamicin, tobramycin, imipenem, meropenem, ceftazidime, cefepime, ciprofloxacin, levofloxacin, piperacillin/tazobactam, cefoperazone/sulbactam, polymyxin B, and aztreonam. Newer antibiotics such as ceftolozane and ceftazidime/avibactam were not tested as they were either not approved or not widely applied in clinical use during the study period. Multidrug-resistant (MDR) P. aeruginosa was defined as isolates non-susceptible in-vitro to least one agent in three or more antipseudomonal antimicrobial categories (22, 23). Carbapenem-resistant (CR) P. aeruginosa was defined as isolates non-susceptible in-vitro to imipenem or meropenem.
Data collection
Data were extracted from medical records via the electronic hospital and laboratory information system. All the data were place in one of six categories: (i) age, gender and ward; (ii) comorbidities; (iii) healthcare exposure in the prior 90 days before onset of P. aeruginosa BSIs including time at risk, intensive care unit (ICU) stay, length of ICU stay and length of hospital stay; (iv) invasive procedures in the prior 90 days before onset of P. aeruginosa BSIs; (v) drug use 90 days before onset of P. aeruginosa BSIs until discharge including corticosteroids, immunosuppressor and antibiotics; (vi) date of taking the first positive blood culture of P. aeruginosa, source of infection and antimicrobial susceptibility results. For risk factor analysis, groups (i)–(v) were considered as potential risk factors. Definition of each variable corresponding to these data was listed in Supplementary Table 1.
Statistical analysis
The resistance rates were compared between antimicrobial resistant and non-resistant phenotypes using Pearson's Chi-squared test or Fisher's exact test as appropriate. The Kaplan-Meier method was used to plot 30-day survival curves and differences between survival curves by antimicrobial resistant phenotypes were evaluated by the log-rank test (23). A generalized linear mixed model with hospital as a random effect was used to determine risk factors for 30-day mortality and to compare total length of hospital stay and healthcare costs between cases infected with antimicrobial resistant P. aeruginosa isolates and cases infected with non-resistant P. aeruginosa isolates. For risk factor analysis, univariate analysis was performed first. Correlation and relevant interactions between variables with P < 0.10 in univariate analysis were checked. After removing variables with high-level correlation (correlation coefficient ≥0.70), the remaining variables were considered for inclusion in the multivariate model and selected using lease absolute shrinkage and selection operator (LASSO) penalty (lambda used to choose variables = lambda.1se, the lambda that minimizes cross validation error plus one standard error) (24). The selected variables were included in the final multivariate analysis to determine the independent associations. Odds ratio (OR) and 95% confidence interval (95%CI) were calculated to determine the strengths of these associations. All the analyses were performed using R version 4.2.1 and a P-value < 0.05 was considered statistically significant. To test the stability of the final multivariate model, variables in the model were removed in turn and the significance of the remaining variables were checked (25).
Results
Overview of the study
A total of 288 cases were diagnosed with P. aeruginosa BSIs. Two patients with length of hospitalization < 48 h and one patient with incomplete data were excluded, hence only 285 cases were included in the study. The number of cases identified each year was 48, 54, 58, 65 and 60, respectively. Clinical characteristics of P. aeruginosa BSIs cases are listed in Table 1. The median age of the 285 cases was 55 years and 29.12% were 65 years or older. There were more male cases (190/285, 66.67%). Majority of the 285 cases were nosocomial BSIs (242/285, 84.91%) and 24.56% (70/285) were polymicrobial BSIs. The details of other bacterial species in polymicrobial BSIs cases were listed in Supplementary Table 2. Of the 84 (29.47%) cases with known source, respiratory tract was the most common source (32/285, 11.23%), followed by skin and soft tissue (27/285, 9.47%). Forty-two (14.74%) cases were from ICU, but 30.53% (87/285) had been admitted to ICU in the prior 90 days of P. aeruginosa BSIs onset. Nearly half (132/285, 46.32%) of all the cases had malignancy. Most of these patients had antimicrobial exposure in the prior 90 days of P. aeruginosa BSIs onset (240/285, 84.21%).
Incidence
Annual incidence of P. aeruginosa BSIs fluctuated between 2.37 per 100,000 patient-days and 3.51 per 100,000 patient-days over the study period, showing an upward trend between 2017 and 2020 (Figure 1).
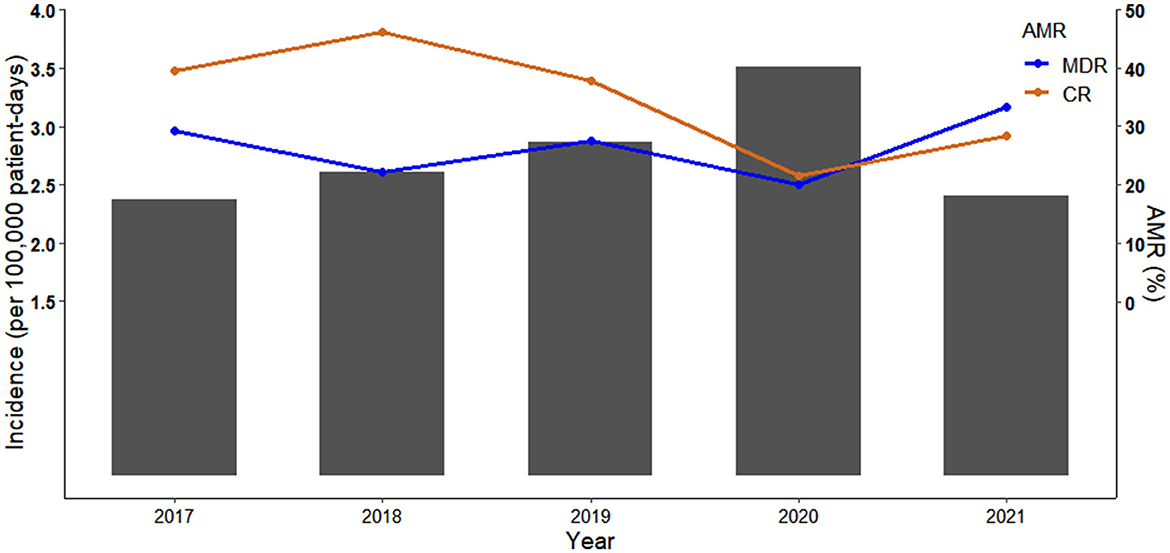
Figure 1. Incidence of Pseudomonas aeruginosa bloodstream infections (P. aeruginosa BSIs) and percentage of antimicrobial resistant phenotypes (AMR) between 2017 and 2021. Bar chart represents the incidence of P. aeruginosa BSIs. Scatter plot and line chart illustrate percentage of multidrug resistant (MDR) and carbapenem resistant (CR) P. aeruginosa isolates.
Antimicrobial resistance
The MDR and CR phenotypes were present in 26.32% (75/285) and 34.04% (97/285) of the 285 P. aeruginosa isolates, respectively. The percentage of CR P. aeruginosa isolates was generally higher than that of MDR isolates in 2017-2020, but there were more MDR isolates than CR isolates in 2021 (Figure 1). All isolates showed low resistance to aminoglycosides (9.51–11.62%), broad-spectrum cephalosporins (17.19–17.61%), fluoroquinolones (17.25–19.43%), and polymyxin B (1.69%) (Figure 2, Supplementary Table 3). By contrast, resistance rate was the highest to aztreonam (39.44%). For all the antibiotics tested, resistance rates of MDR isolates were significantly higher than those of non-MDR isolates (Figure 2, Supplementary Table 3). Also, CR isolates showed significant higher resistance to most antibiotics than non-CR isolates except for polymyxin B (Figure 2, Supplementary Table 3). It is notable that resistance rates of MDR isolates were higher to majority of the antibiotics than those of CR isolates except for carbapenems (Figure 2).
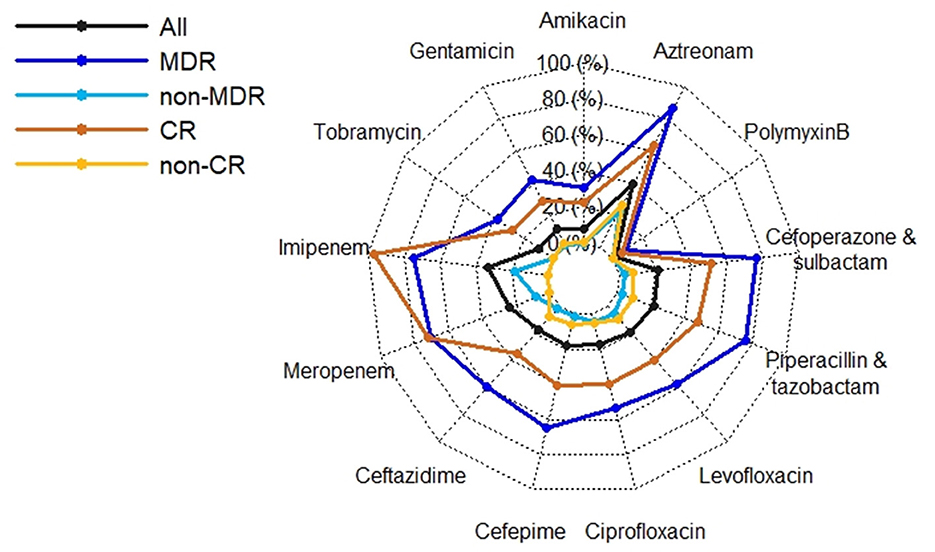
Figure 2. Radar plot of antimicrobial resistance rates of 285 P. aeruginosa isolates by antimicrobial resistant phenotypes. MDR, multidrug resistant; non-MDR, non-multidrug resistant; CR, carbapenem resistant; non-CR, non-carbapenem resistant.
Mortality and risk factors for crude 30-day mortality
Crude 30-day mortality rate was 17.89% (51/285). Crude 30-day survival of cases with MDR and CR P. aeruginosa isolates were significantly lower than those of cases with non-MDR and non-CR isolates (P = 0.003/ < 0.001; Figure 3). There were no differences of total length of hospital stay between cases with MDR/CR isolates and cases with non-MDR/non-CR isolates (Table 2). However, healthcare costs of cases with MDR/CR isolates were significantly higher than those of cases with non-MDR/non-CR isolates (Table 2).
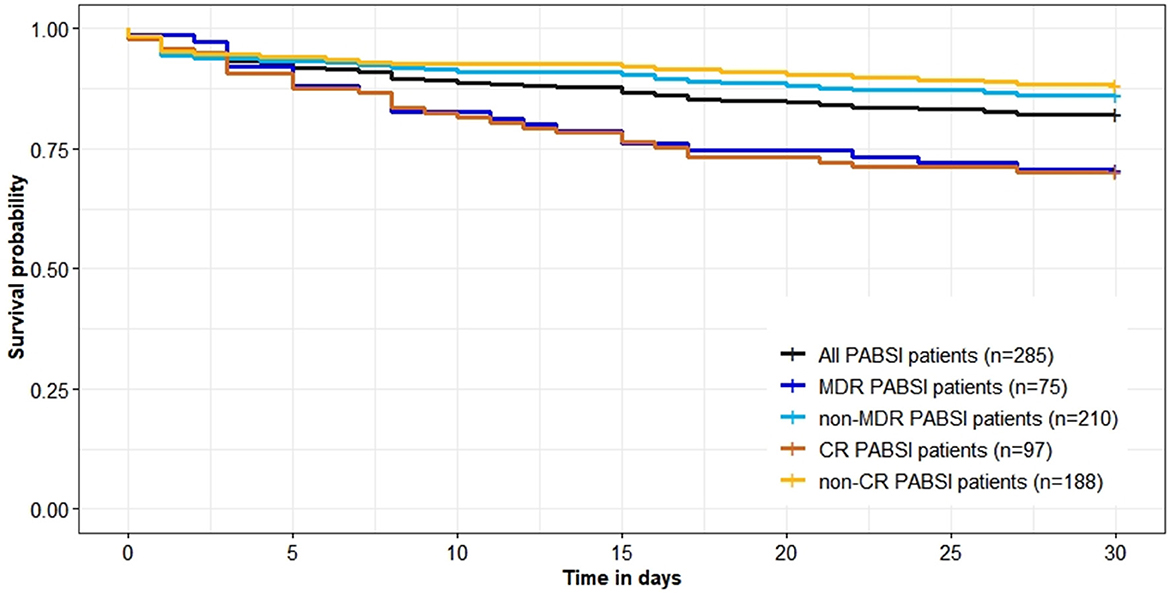
Figure 3. Survival (in days) of 285 Pseudomonas aeruginosa bloodstream infections (PABSI) cases and comparison by antimicrobial resistant phenotypes. MDR, multidrug resistant; non-MDR, non-multidrug resistant; CR, carbapenem resistant; non-CR, non-carbapenem resistant.

Table 2. Comparison of total length of hospital stay and healthcare costs of 285 Pseudomonas aeruginosa bloodstream infections cases by antimicrobial resistant phenotypes.
The univariate analysis showed that polymicrobial BSIs, antimicrobial resistant phenotypes (MDR or CR), burns, several healthcare exposure and treatment factors in the prior 90 days before BSIs onset (ICU stay, length of ICU stay, invasive ventilation, indwelling catheterization, corticosteroids, carbapenems, and quantity of carbapenems) and inappropriate empiric and definitive therapies were associated with crude 30-day mortality (Table 3). The multivariate analysis indicated that inappropriate definitive therapy [adjusted odds ratio (aOR) 4.47, 95% confidence interval (95% CI) 1.35–14.77; P = 0.014], ICU stay (aOR 2.89, 95% CI: 1.26–6.63; P = 0.012) and corticosteroids use (aOR 2.89, 95% CI: 1.31–6.41; P = 0.009) in the prior 90 days were independent risk factors for crude 30-day mortality (Table 3).
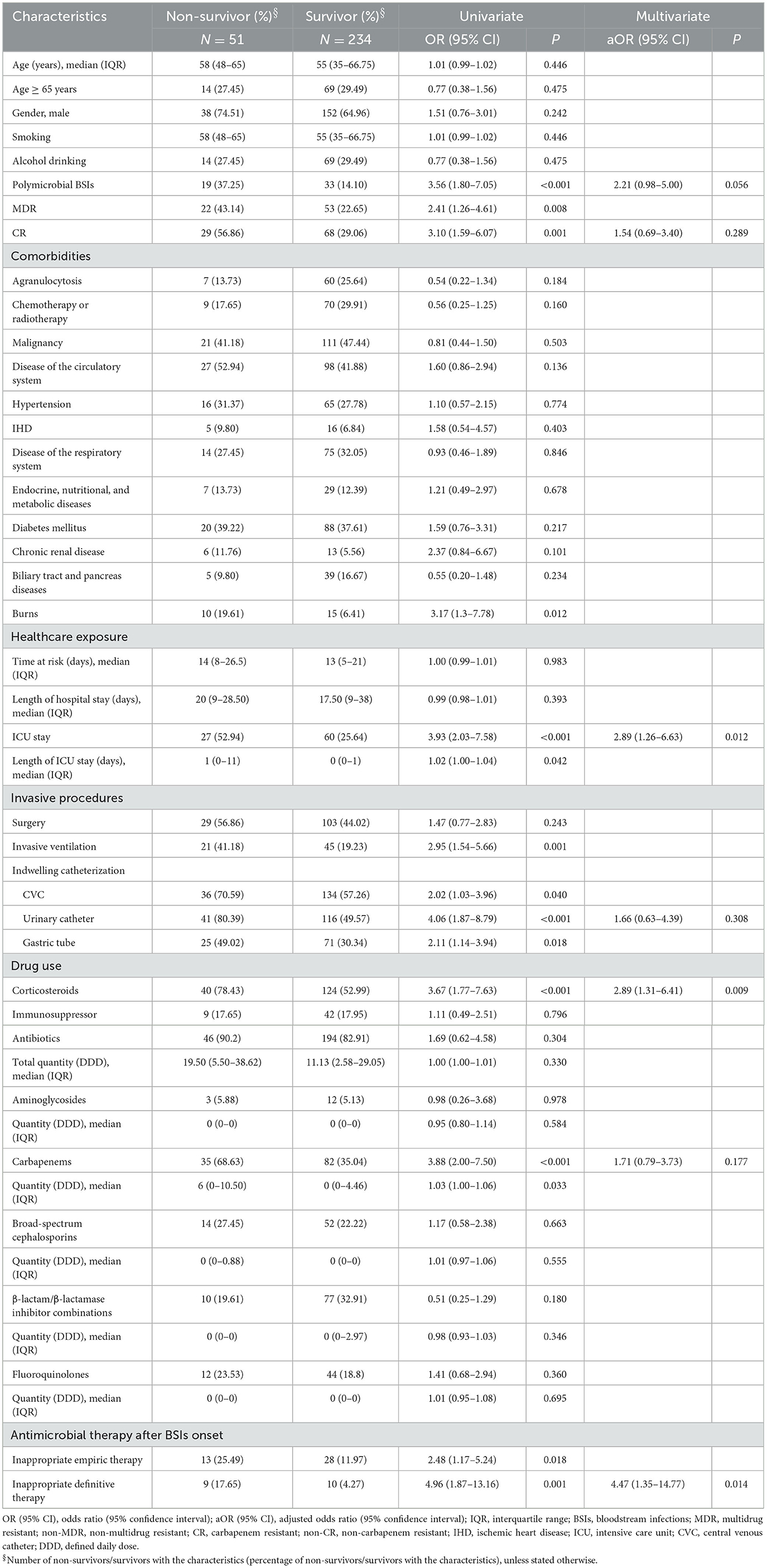
Table 3. Risk factors associated with crude 30-day mortality of 285 Pseudomonas aeruginosa bloodstream infections cases.
Discussion
This study conducted an in-depth epidemiological analysis of P. aeruginosa BSIs at individual level hence to provide a comprehensive understanding of characteristics underlying factors and related morbidity and mortality associated with P. aeruginosa BSIs. To the authors' knowledge, this is the first epidemiological study of P. aeruginosa BSIs conducted in these two different administrative regions of China.
Incidence of P. aeruginosa BSIs fluctuated between 2.37 and 3.51 per 100,000 patient-days. This finding is similar to a previous study conducted in Southeast China reporting that the incidence was between 2.70 and 6.20 per 100,000 patient-days (2). According to the management policy of coronavirus disease 2019 (COVID-19) over the study period, all COVID-19 patients were closed loop transferred to designated hospitals immediately after positive PCR test at admission. Therefore, none of the 285 P. aeruginosa BSIs were COVID-19 patients. Also, it is difficult to evaluate impact of the pandemic on P. aeruginosa BSIs. Incidence of P. aeruginosa BSIs showed an upward trend during the pre-pandemic (2017–2019) and pandemic period (2020) but dropped in 2021. Some studies observed a higher incidence of BSIs during the COVID-19 pandemic (26–28). As management of COVID-19 has been downgraded in China, impact of the COVID-19 pandemic on the incidence of P. aeruginosa BSIs warrants more local research in future study. Crude 30-day mortality rate (17.89%) of P. aeruginosa BSIs was lower than that of studies conducted in other parts of China (2, 9), Europe (6, 10, 29), and Australia (12).
As other studies reported, there were more male cases than female cases (8, 9, 29, 30), however, gender is not associated with adverse clinical outcomes. Yoon et al. have claimed that 26.70% of P. aeruginosa BSIs patients were with polymicrobial BSIs and this was not associated with mortality though (11). The rate of polymicrobial BSIs in this study was slightly lower (24.56%) than the above study, however, polymicrobial BSIs was a risk factor for crude 30-day mortality. Our data showed that the percentage of ICU stay among cases with polymicrobial BSIs was higher than that among cases only with P. aeruginosa BSIs (42.31 vs. 27.90%), indicating that polymicrobial BSIs may be a surrogate marker of critical illness and higher risk of death. Respiratory tract (32/84, 38.10%) was the most common probable source among cases with known source of BSIs which agreed with other studies (2, 29, 30). Interestingly, more than half of the 32 cases (20/32, 62.50%) with BSIs stemmed from respiratory tract infection had invasive ventilation support. The mucosal barrier injury of respiratory tract would decrease the capacity for bacterial clearance and increase probabilities of bacterial colonization and/or infection (31).
Almost all of comorbidities were not linked to mortality with the exception of burns (Table 3). Burned patients are more likely to have invasive treatments and are more debilitated and prone to subsequent infections as burn wounds are favorable sites for bacterial colonization until they are closed (32). Our study identified that mechanical ventilation, CVC, urinary catheter and gastric tube were predictors for crude 30-day mortality (Table 3). Invasive indwelling devices or procedures have been widely investigated as risk factors for mortality caused by bacterial infections as bypass the innate host mechanical defenses and provide a niche for microorganisms, facilitating progression of infections (29, 30). Mechanical ventilation is a treatment option that is often necessary in critical ill patients (ICU patients in particular). All the above factors should be interpreted with caution as they may present surrogate markers of critical illness and extensive healthcare exposure rather than reflecting a direct association. Consistent with this interpretation, our study also identified that ICU stay was independently associated with crude 30-day mortality which has been reported by multiple other studies (8, 11, 29). Nevertheless, the exist of P. aeruginosa isolates in the manmade environment is a prerequisite for the infection to occur, so implementation and good compliance of aseptic technique during invasive procedures and infection control measures during daily medical work.
In contrast to another study conducted in China (33), our study showed that P. aeruginosa isolates retained susceptibility to aminoglycosides, broad-spectrum cephalosporins, fluoroquinolones, and polymyxin B which was consistent with the findings of studies conducted in Spain and Korea (10, 34). Discrepancies between studies may reflect different antimicrobial prescribing practices and antimicrobial mechanisms, highlighting the importance of understanding local anti-biograms to preserve antibiotic utility and rational treatment. MDR and CR phenotypes were widely reported as predictors of poorer outcome as very few effective therapeutic options are available to treatment (2, 6, 9, 10, 29). Although antimicrobial phenotypes were not independently associated with mortality in this study (Table 3) which agrees with Montero et al. (35), they had significant adverse impact on the crude 30-day survival (Figure 3).
We found that prior carbapenems use, quantity of carbapenems, and inappropriate empirical/definitive antimicrobial therapy were linked to mortality (Table 3). Again, this could be explained by critical illness as carbapenems are often used as last resort antibiotics for treatments of MDR infections. It has been well-proved that inappropriate empirical and/or definitive antimicrobial therapy was associated with increased mortality outcomes (2, 8, 10, 30). Moreover, another concern is the higher percentage of MDR isolates over that of CR isolates in 2021 (Figure 1) given that resistance rates of MDR isolates were higher to majority of the antibiotics than those of CR isolates (Figure 2, Supplementary Table 3). These findings would trigger antimicrobial stewardship programs to monitor antimicrobial use and contain antimicrobial resistance in patients with P. aeruginosa BSIs. In addition, we identified corticosteroids administration as an independent predictor of crude 30-day mortality (Table 3). Corticosteroids are well-recognized as the marker of immunocompromised status which is vulnerable to severe infections. Therefore, past work has widely demonstrated an association between corticosteroids administration and higher mortality risk (2, 8, 12, 29).
Interestingly, we found that total length of hospital stay did not differ significantly between cases with MDR/CR phenotypes and cases with non-MDR/CR phenotypes while healthcare costs of cases with MDR/CR phenotypes were significantly higher than those of cases with non-MDR/non-CR phenotypes (Table 2). Increased healthcare costs may stem from higher costs of agents needed to treat MDR/CR BSIs, greater likelihood for procedures such as line placement for intravenous antibiotics, and complications from these agents and procedures (36–38).
There are some limitations of the study. First, for antimicrobial susceptibility test, not all the isolates were tested for the same agents. Second, no genomic data were available to identify mechanisms of antimicrobial resistance, possible clonal spread and virulence. Bioinformatic and phylogenetic study is warranted to better understand of the phylogeny and pathogenicity of these P. aeruginosa isolates in the future.
Conclusion
In conclusion, annual incidence of P. aeruginosa BSIs was fluctuating over the study period. P. aeruginosa BSIs cases with non-MDR/CR phenotypes had a survival advantage over cases with MDR/CR phenotypes which resulted in higher healthcare costs. Awareness is required that patients with inappropriate definitive therapy, ICU stay and corticosteroids use are at higher risk of death from P. aeruginosa BSIs. P. aeruginosa isolates retained susceptibility to aminoglycosides, broad-spectrum cephalosporins, fluoroquinolones, and polymyxin B which may be alternative therapeutic options.
Data availability statement
The original contributions presented in the study are included in the article/Supplementary material, further inquiries can be directed to the corresponding author.
Ethics statement
The studies involving humans were approved by Medical Ethics Committee of Xiangya Hospital Central South University and Ruijin Hospital Ethics Committee (Shanghai Jiao Tong University School of Medicine). The studies were conducted in accordance with the local legislation and institutional requirements. The human samples used in this study were acquired from a by- product of routine care or industry. Written informed consent for participation was not required from the participants or the participants' legal guardians/next of kin in accordance with the national legislation and institutional requirements.
Author contributions
SX: Data curation, Formal analysis, Investigation, Methodology, Resources, Software, Validation, Writing—original draft. XL: Data curation, Formal analysis, Investigation, Methodology, Resources, Software, Validation, Writing—original draft. LH: Resources, Validation, Writing—review & editing. SZ: Conceptualization, Formal analysis, Methodology, Project administration, Resources, Supervision, Validation, Writing—review & editing.
Funding
The author(s) declare that no financial support was received for the research, authorship, and/or publication of this article.
Acknowledgments
The authors wish to thank Hongying Tang, Yaolei Wang, and Zhaohui Jin for the guidance and training of using hospital information system.
Conflict of interest
The authors declare that the research was conducted in the absence of any commercial or financial relationships that could be construed as a potential conflict of interest.
Publisher's note
All claims expressed in this article are solely those of the authors and do not necessarily represent those of their affiliated organizations, or those of the publisher, the editors and the reviewers. Any product that may be evaluated in this article, or claim that may be made by its manufacturer, is not guaranteed or endorsed by the publisher.
Supplementary material
The Supplementary Material for this article can be found online at: https://www.frontiersin.org/articles/10.3389/fpubh.2023.1294141/full#supplementary-material
References
1. Rudd KE, Johnson SC, Agesa KM, Shackelford KA, Tsoi D, Kievlan DR, et al. Global, regional, and national sepsis incidence and mortality, 1990-2017: analysis for the Global Burden of Disease Study. Lancet. (2020) 395:200–11. doi: 10.1016/S0140-6736(19)32989-7
2. Shi Q, Huang C, Xiao T, Wu Z, Xiao Y. A retrospective analysis of Pseudomonas aeruginosa bloodstream infections: prevalence, risk factors, and outcome in carbapenem-susceptible and -non-susceptible infections. Antimicrob Resist Infect Control. (2019) 8:68. doi: 10.1186/s13756-019-0520-8
3. Diekema DJ, Hsueh PR, Mendes RE, Pfaller MA, Rolston KV, Sader HS, et al. The microbiology of bloodstream infection: 20-year trends from the SENTRY antimicrobial surveillance program. Antimicrob Agents Chemother. (2019) 63:e00355-19. doi: 10.1128/AAC.00355-19
4. Thaden JT, Park LP, Maskarinec SA, Ruffin F, Fowler VG Jr, van Duin D. Results from a 13-year prospective cohort study show increased mortality associated with bloodstream infections caused by Pseudomonas aeruginosa compared to other bacteria. Antimicrob Agents Chemother. (2017) 61:e02671–16. doi: 10.1128/AAC.02671-16
5. Reynolds D, Kollef M. The epidemiology and pathogenesis and treatment of Pseudomonas aeruginosa infections: an update. Drugs. (2021) 81:2117–31. doi: 10.1007/s40265-021-01635-6
6. Rolo M, Martín-Higuera MC, Viedma E, Villa J, Mancheño-Losa M, Lora-Tamayo J, et al. Clinical impact of time-to-positivity of blood cultures on mortality in patients with Pseudomonas aeruginosa bacteremia. J Glob Antimicrob Resist. (2022) 30:269–75. doi: 10.1016/j.jgar.2022.06.026
7. Sid Ahmed MA, Hamid JM, Husain AA, Hadi HA, Skariah S, Sultan AA, et al. Clinical outcomes, molecular epidemiology and resistance mechanisms of multidrug-resistant Pseudomonas aeruginosa isolated from bloodstream infections from Qatar. Ann Med. (2021) 53:2345–53. doi: 10.1080/07853890.2021.2012588
8. Ababneh MA, Rababa'h AM, Almomani BA, Ayoub AM, Al-Azzam SI. A ten-year surveillance of P aeruginosa bloodstream infections in a tertiary care hospital: trends and risk factors for mortality. Int J Clin Practice. (2021) 75:e14409. doi: 10.1111/ijcp.14409
9. Zhang Y, Li Y, Zeng J, Chang Y, Han S, Zhao J, et al. Risk factors for mortality of inpatients with Pseudomonas aeruginosa bacteremia in China: impact of resistance profile in the mortality. Infect Drug Resist. (2020) 13:4115–23. doi: 10.2147/IDR.S268744
10. Recio R, Mancheño M, Viedma E, Villa J, Orellana M, Lora-Tamayo J, et al. Predictors of mortality in bloodstream infections caused by Pseudomonas aeruginosa and impact of antimicrobial resistance and bacterial virulence. Antimicrob Agents Chemother. (2020) 64:e01759-19. doi: 10.1128/AAC.01759-19
11. Yoon EJ, Kim D, Lee H, Lee HS, Shin JH, Park YS, et al. Mortality dynamics of Pseudomonas aeruginosa bloodstream infections and the influence of defective OprD on mortality: prospective observational study. J Antimicrob Chemother. (2019) 74:2774–83. doi: 10.1093/jac/dkz245
12. McCarthy KL, Paterson DL. Long-term mortality following Pseudomonas aeruginosa bloodstream infection. J Hosp Infection. (2017) 95:292–9. doi: 10.1016/j.jhin.2016.11.014
13. Chen Y, Ji J, Ying C, Liu Z, Yang Q, Kong H, et al. BRICS report of 2020: the bacterial composition and antimicrobial resistance profile of clinical isolates from bloodstream infections in China. Chin J Clin Infect Dis. (2021) 14:413–26.
14. Zhang Y, Chen XL, Huang AW, Liu SL, Liu WJ, Zhang N, et al. Mortality attributable to carbapenem-resistant Pseudomonas aeruginosa bacteremia: a meta-analysis of cohort studies. Emerg Microbes Infect. (2016) 5:e27. doi: 10.1038/emi.2016.22
15. Su TY, Ye JJ, Hsu PC, Wu HF, Chia JH, Lee MH. Clinical characteristics and risk factors for mortality in cefepime-resistant Pseudomonas aeruginosa bacteremia. J Microbiol Immunol Infect. (2015) 48:175–82. doi: 10.1016/j.jmii.2013.07.009
16. Garner JS, Jarvis WR, Emori TG, Horan TC, Hughes JM. CDC definitions for nosocomial infections, 1988. Am J Infect Control. (1988) 16:128–40.
17. Horan TC, Andrus M, Dudeck MA. CDC/NHSN surveillance definition of health care-associated infection and criteria for specific types of infections in the acute care setting. Am J Infect Control. (2008) 36:309–32. doi: 10.1016/j.ajic.2008.03.002
18. Xiao T, Zhu Y, Zhang S, Wang Y, Shen P, Zhou Y, et al. A retrospective analysis of risk factors and outcomes of carbapenem-resistant Klebsiella pneumoniae bacteremia in nontransplant patients. J Hosp Infect. (2020) 221:S174–83. doi: 10.1093/infdis/jiz559
19. Baron EJ, Miller JM, Weinstein MP, Richter SS, Gilligan PH, Thomson RB Jr, et al. A guide to utilization of the microbiology laboratory for diagnosis of infectious diseases: 2013 recommendations by the Infectious Diseases Society of America (IDSA) and the American Society for Microbiology (ASM)(a). Clin Infect Dis. (2013) 57:e22–121. doi: 10.1093/cid/cit278
20. Patil S, Chen X, Dong S, Mai H, Lopes BS, Liu S, et al. Resistance genomics and molecular epidemiology of high-risk clones of ESBL-producing Pseudomonas aeruginosa in young children. Front Cell Infect Microbiol. (2023) 13:1168096. doi: 10.3389/fcimb.2023.1168096
21. Wayne PA. Clinical and Laboratory Standards Institute (CLSI): Performance Standards for Antimicrobial Susceptibility Testing. 31st ed. Wayne, PA: CLSI supplement M100 (2021).
22. Hattemer A, Hauser A, Diaz M, Scheetz M, Shah N, Allen JP, et al. Bacterial and clinical characteristics of health care- and community-acquired bloodstream infections due to Pseudomonas aeruginosa. Antimicrob Agents Chemother. (2013) 57:3969–75. doi: 10.1128/AAC.02467-12
23. Mantel N. Evaluation of survival data and two new rank order statistics arising in its consideration. Cancer Chemother Rep. (1966) 50:163–70.
24. Friedman J, Hastie T, Tibshirani R. Regularization paths for generalized linear models via coordinate descent. J Stat Softw. (2010) 33:1–22.
25. Zhao S, Perry MR, Kennedy S, Wilson J, Chase-Topping ME, Anderson E, et al. Risk factors for carbapenemase-producing organisms among inpatients in Scotland: a national matched case-control study. Infect Control Hosp Epidemiol. (2021) 42:968–77. doi: 10.1017/ice.2020.1351
26. Valik JK, Hedberg P, Holmberg F, van der Werff SD, Naucler P. Impact of the COVID-19 pandemic on the incidence and mortality of hospital-onset bloodstream infection: a cohort study. Br Med J Qual Saf. (2022) 31:379–82. doi: 10.1136/bmjqs-2021-014243
27. Baker MA, Sands KE, Huang SS, Kleinman K, Septimus EJ, Varma N, et al. The impact of coronavirus disease 2019 (COVID-19) on healthcare-associated infections. Clin Infect Dis. (2022) 74:1748–54. doi: 10.1093/cid/ciab688
28. Pasquini Z, Barocci I, Brescini L, Candelaresi B, Castelletti S, Iencinella V, et al. Bloodstream infections in the COVID-19 era: results from an Italian multi-centre study. Int J Infect Dis. (2021) 111:31–6. doi: 10.1016/j.ijid.2021.07.065
29. Babich T, Naucler P, Valik JK, Giske CG, Benito N, Cardona R, et al. Risk factors for mortality among patients with Pseudomonas aeruginosa bacteraemia: a retrospective multicentre study. Int J Antimicrob Agents. (2020) 55:105847. doi: 10.1016/j.ijantimicag.2019.11.004
30. Tan TL, Chiew SC, Laang ST, Umabalan, Khor SH, Lee LY. Pseudomonas aeruginosa bacteraemia: a five-year analysis of epidemiology, clinical profiles, and outcome in a Malaysian district hospital. Med J Malaysia. (2021) 76:630–6.
31. Zhao S, Kennedy S, Perry MR, Wilson J, Chase-Topping M, Anderson E, et al. Epidemiology of and risk factors for mortality due to carbapenemase-producing organisms (CPO) in healthcare facilities. J Hosp Infect. (2021) 110:184–93. doi: 10.1016/j.jhin.2021.01.028
32. Coban YK. Infection control in severely burned patients. World J Crit Care Med. (2012) 1:94–101. doi: 10.5492/wjccm.v1.i4.94
33. Yin S, Chen P, You B, Zhang Y, Jiang B, Huang G, et al. Molecular typing and carbapenem resistance mechanisms of Pseudomonas aeruginosa isolated from a Chinese Burn Center From 2011 to 2016. Front Microbiol. (2018) 9:1135. doi: 10.3389/fmicb.2018.01135
34. Kang JS, Moon C, Mun SJ, Lee JE, Lee SO, Lee S, et al. Antimicrobial susceptibility trends and risk factors for antimicrobial resistance in Pseudomonas aeruginosa bacteremia: 12-year experience in a tertiary hospital in Korea. J Korean Med Sci. (2021) 36:e273. doi: 10.3346/jkms.2021.36.e273
35. Montero MM, López Montesinos I, Knobel H, Molas E, Sorlí L, Siverio-Parés A, et al. Risk factors for mortality among patients with Pseudomonas aeruginosa bloodstream infections: what is the influence of XDR phenotype on outcomes? J Clin Med. (2020) 9:514. doi: 10.3390/jcm9020514
36. Thaden JT, Li Y, Ruffin F, Maskarinec SA, Hill-Rorie JM, Wanda LC, et al. Increased costs associated with bloodstream infections caused by multidrug-resistant gram-negative bacteria are due primarily to patients with hospital-acquired infections. Antimicrob Agents Chemother. (2017) 61:e01709-16. doi: 10.1128/AAC.01709-16
37. Ng E, Earnest A, Lye DC, Ling ML, Ding Y, Hsu LY. The excess financial burden of multidrug resistance in severe gram-negative infections in Singaporean hospitals. Ann Acad Med Singapore. (2012) 41:189–93.
Keywords: Pseudomonas aeruginosa, bloodstream infections, incidence, mortality, antimicrobial resistance
Citation: Xiao S, Liang X, Han L and Zhao S (2024) Incidence, antimicrobial resistance and mortality of Pseudomonas aeruginosa bloodstream infections among hospitalized patients in China: a retrospective observational multicenter cohort study from 2017 to 2021. Front. Public Health 11:1294141. doi: 10.3389/fpubh.2023.1294141
Received: 14 September 2023; Accepted: 13 December 2023;
Published: 05 January 2024.
Edited by:
Soheil Ebrahimpour, Babol University of Medical Sciences, IranReviewed by:
Kate McCarthy, Royal Brisbane and Women's Hospital, AustraliaMai Mahmoud Zafer, Ahram Canadian University, Egypt
Copyright © 2024 Xiao, Liang, Han and Zhao. This is an open-access article distributed under the terms of the Creative Commons Attribution License (CC BY). The use, distribution or reproduction in other forums is permitted, provided the original author(s) and the copyright owner(s) are credited and that the original publication in this journal is cited, in accordance with accepted academic practice. No use, distribution or reproduction is permitted which does not comply with these terms.
*Correspondence: Shengyuan Zhao, c2hlbmd5dWFuemhhb0Bjc3UuZWR1LmNu
†These authors have contributed equally to this work and share first authorship