- 1Faculty of Medicine, Team "Resist" UMR1184, "Immunology of Viral, Auto-Immune, Hematological and Bacterial Diseases (IMVA-HB)," INSERM, Université Paris-Saclay, CEA, Health and Therapeutic Innovation (HEALTHI), Le Kremlin-Bicêtre, France
- 2Laboratoire Microbiologie Santé et Environment (LMSE), Doctoral School of Sciences and Technology, Faculty of Public Health, Lebanese University, Tripoli, Lebanon
- 3Bacteriology-Hygiene Unit, Assistance Publique-Hôpitaux de Paris, AP-HP Paris-Saclay, Bicêtre Hospital Le Kremlin-Bicêtre, Paris, France
- 4Center for Food Safety and Department of Food Science and Technology, University of Georgia, Griffin, GA, United States
- 5French National Reference Center for Antibiotic Resistance, Clermont-Ferrand, France
- 6Cornell Atkinson Center for Sustainability, Cornell University, Ithaca, NY, United States
- 7Department of Public and Ecosystem Health, College of Veterinary Medicine, Cornell University, Ithaca, NY, United States
- 8Department of Neurosurgery, Yale University School of Medicine, New Haven, CT, United States
Background: Carbapenem- and extended-spectrum cephalosporin-resistant Enterobacterales (CR-E and ESCR-E, respectively) are increasingly isolated worldwide. Information about these bacteria is sporadic in Lebanon and generally relies on conventional diagnostic methods, which is detrimental for a country that is struggling with an unprecedented economic crisis and a collapsing public health system. Here, CR-E isolates from different Lebanese hospitals were characterized.
Materials and methods: Non-duplicate clinical ESCR-E or CR-E isolates (N = 188) were collected from three hospitals from June 2019 to December 2020. Isolates were identified by MALDI-TOF, and their antibiotic susceptibility by Kirby-Bauer disk diffusion assay. CR-E isolates (n = 33/188) were further analyzed using Illumina-based WGS to identify resistome, MLST, and plasmid types. Additionally, the genetic relatedness of the CR-E isolates was evaluated using an Infrared Biotyper system and compared to WGS.
Results: Using the Kirby-Bauer disk diffusion assay, only 90 isolates out of the 188 isolates that were collected based on their initial routine susceptibility profile by the three participating hospitals could be confirmed as ESCR-E or CR-E isolates and were included in this study. This collection comprised E. coli (n = 70; 77.8%), K. pneumoniae (n = 13; 14.4%), Enterobacter spp. (n = 6; 6.7%), and Proteus mirabilis (n = 1; 1.1%). While 57 were only ESBL producers the remaining 33 isolates (i.e., 26 E. coli, five K. pneumoniae, one E. cloacae, and one Enterobacter hormaechei) were resistant to at least one carbapenem, of which 20 were also ESBL-producers. Among the 33 CR-E, five different carbapenemase determinants were identified: blaNDM-5 (14/33), blaOXA-244 (10/33), blaOXA-48 (5/33), blaNDM-1 (3/33), and blaOXA-181 (1/33) genes. Notably, 20 CR-E isolates were also ESBL-producers. The analysis of the genetic relatedness revealed a substantial genetic diversity among CR-E isolates, suggesting evolution and transmission from various sources.
Conclusion: This study highlighted the emergence and broad dissemination of blaNDM-5 and blaOXA-244 genes in Lebanese clinical settings. The weak AMR awareness in the Lebanese community and the ongoing economic and healthcare challenges have spurred self-medication practices. Our findings highlight an urgent need for transformative approaches to combat antimicrobial resistance in both community and hospital settings.
1 Introduction
The order Enterobacterales includes the most common human bacterial pathogens responsible for community- and healthcare-associated infections. These species have the ability to rapidly evolve through horizontal gene transfer (e.g., mobile genetic elements) (1). This includes the ability to develop resistance to multiple antibiotics, which complicates the treatment of infections and increases, potentially mortality and morbidity in patients. Of particular concern is the emergence of carbapenem-resistant Enterobacterales (CR-E), which poses a major concern in clinical as well as community settings all across the globe (2). Carbapenems referred to as last-resort antibiotics, possess a broad spectrum of activity against most clinically-relevant Gram-negative bacteria (3, 4). Consequently, it is crucial to continuously monitor and assess the spread of carbapenem resistance, especially in low- and middle-income countries (LMICs) that face established challenges in antimicrobial stewardship and public health systems.
Available observations suggest that antimicrobial resistance (AMR) has been precipitously increasing in Lebanon, a country with a plethora of issues resulting from an unprecedented economic collapse (5–7). The latter has amplified critical issues such as access to medical care, sanitation, and nutritious and safe food, as well as promoted lax medical practices, including self-medication and the reliance on widely and easily available antibiotics as cheaper alternatives across the country. This is important because excessive and inappropriate use of antimicrobials in human and veterinary medicine and agriculture has been well-documented in Lebanon, even before the economic collapse (8–10). Taken together, these challenging conditions have been predicted to enhance the emergence of AMR and the cycle of complicated infections, especially in the most vulnerable populations in Lebanon (5–7, 10, 11). Nevertheless, studies on AMR in Lebanon are generally scant and, when available, can be limited, in scope or (e.g., low sample number) and/or descriptive (e.g., phenotypic AMR evaluation and absence of in-depth genomic analysis). Despite this, available studies have reported rates of extended-spectrum cephalosporin-resistant Enterobacterales (ESCR-E; ~50% of tested isolates) and CR-E (~3%) among clinical isolates in Lebanon between 2015 and 2019 (12, 13). The enzymatic nature of the carbapenem-resistance has also been evidenced, by the detection of several carbapenemase genes, including blaOXA-48, blaNDM-5, and blaNDM-19, in Escherichia coli, blaOXA-48, blaOXA-181, and blaNDM-5 in Klebsiella pneumoniae, blaOXA-48, blaVIM-1, blaVIM-4, and blaNDM-1 in Enterobacter cloacae, and blaOXA-48 in Citrobacter freundii isolated in Lebanese hospitals (12). In addition, high rates of multidrug-resistant (MDR) Enterobacterales (60.7%) and the dissemination of extended-spectrum ß-lactamase (ESBL) producing-, carbapenemase-producing (CP)-, and colistin-resistant E. coli isolates among healthy people in the Lebanese community (14), animals (15), and the environment (16) has been documented. Hence, in-depth studies to evaluate the emergence and spread of AMR in Lebanon, focusing on the determinants that contribute to the dissemination of ESBL-E and/or CP-E is now mandatory. Specifically, details of the sequence types of these bacteria, their resistome, and the plasmids carrying these genes may be crucial to identify the transmission routes and propose intervention strategies to limit their spread. To fill these gaps, we evaluated the occurrence of ESBL- and/or CP-E in three different hospitals in Lebanon and determined the underlying mechanisms of resistance, the population structure of the isolates, and the associated plasmid types using whole genome sequencing analysis (WGS), a powerful technique for investigating AMR, but still not commonly available in LMICs, such as Lebanon, due to its relative expensiveness and requirement of specialized equipment and skills (17).
This study focused only on hospital isolates, as (1) anecdotal evidence suggests that hospitals play an important role in the transmission of ESBL-E- and CP-E, and (2) hospitalized patients are more susceptible to infections/colonization with these species.
2 Materials and methods
2.1 Ethical approval
This study was approved by the Azm Center/Lebanese University ethical committee and the Lebanese Ministry of Public Health (CE-EDST-1-2020). All the specimens were analyzed anonymously, without any patient identifiers, and the patients were not physically involved in this study.
2.2 Isolation and identification of bacteria from clinical samples
A total of 188 clinical Enterobacterales isolates being either resistant to Expanded Spectrum Cephalosporin (ESCR) or Carbapenem Resistant were collected between 2019 and 2020 by the bacteriology laboratories of three hospitals, including El Youssef Hospital Center (50 isolates), the Nini Hospital (137 isolates), and the Tripoli Governmental Hospital (1 isolate), which are located in the Akkar and North governorates of Lebanon, respectively. These isolates were collected based on their susceptibility profile established in the hospitals as part of routine clinical testing. These clinical isolates were recovered from different sample types, including urine, pus, wound, rectal, axillary, pleural fluid, gastric fluid, and bronchial fluid. They were identified at the hospitals using the matrix-assisted laser desorption/ionization time-of-flight (MALDI-TOF) with VITEK MS protocol (bioMérieux, Version 3.0, Marcy L’Etoile, France). The isolates were subsequently stored at the Lebanese University bacterial bank (CMUL).
2.3 Antimicrobial susceptibility testing
The isolates were screened for ESBL and CR phenotypes using the Kirby-Bauer disk diffusion assay (including Ticarcillin, Ticarcillin/clavulanic acid, cefoxitin, ceftazidime, cefepime, temocillin, and imipenem). As for the CR-E isolates, a total of 15 β-lactams (amoxicillin, amoxicillin/clavulanic acid, ticarcillin, ticarcillin/clavulanic acid, piperacillin, piperacillin/tazobactam, cefoxitin, cefotaxime, cefepime, ceftazidime, aztreonam, ertapenem, imipenem, meropenem, and temocillin) antibiotics of human interest were tested. The minimum inhibitory concentrations (MICs) were also determined by E-test (bioMérieux) for ertapenem, imipenem, and meropenem, while temocillin and colistin MICs were assessed using the broth microdilution method.
Additionally, 13 CP-E. coli isolates were selected according to their resistance phenotype, genotype, and MLST type for further determination of MIC values using broth microdilution (BMD) test (Sensititre, ThermoFisher, Grenoble, France) for a complementary list of novel beta-lactam and non-beta-lactam antibiotics of clinical and veterinary interest (i.e., ceftazidime/avibactam, ceftiofur, ceftaroline, ceftobiprole, aztreonam-avibactam, mecillinam, imipenem/relebactam, meropenem/vaborbactam, cefiderocol, eravacyclin, apramycin, gentamicin, neomycin, streptomycin, sulfonamides, and nitrofurantoin). Susceptibility patterns were interpreted according to the European Committee on Antimicrobial Susceptibility Testing (EUCAST)1 guidelines when available (18). For aztreonam/avibactam, interpretation was done using aztreonam breakpoints alone (18). For ceftiofur, apramycin, neomycin and streptomycin veterinary breakpoints were used.2
2.4 Evaluation of the ESCR- and CR-E isolates using enzymatic assays
The β LACTA™ test (Bio-Rad, Marne-la-Coquette, France) was used to further evaluate the ESCR-E isolates. Briefly, this test is based on the hydrolysis of a chromogenic cephalosporin that turns red upon hydrolysis. Notably, the chromogenic cephalosporin is not hydrolyzed by acquired penicillinases (e.g., SHV-1, TEM-1) but by ESBL, carbapenemase, and acquired AmpC (19). Furthermore, the NG-Test® CTX-M MULTI (NG Biotech, Guipry, France) immunochromatographic assay (ICA) was performed on the β LACTA™ positive isolates to infer the presence of CTX-M-type ESBLs (20). The CR-E isolates were also evaluated using the Carba NP hydrolysis test, which detects carbapenemase activity based on in vitro hydrolysis of imipenem (21). The NG-Test® CARBA-5 ICA (NG Biotech) was used to detect members of the five main families of carbapenemases (i.e., KPC-, NDM-, VIM-, IMP-, and OXA-48-like enzymes) produced by the CR-E isolates as described in the manufacturers’ instructions (22).
2.5 Molecular characterization of the ESCR- and CR-E isolates
The ESCR-E isolates (positive using the β-LACTA™ and NG-Test® CTX-M MULTI tests) were screened by PCR and subsequent Sanger sequencing to identify the blaCTX-M allele as described previously (23, 24). For the ESCR-E isolates (positive using the β-LACTA™ but negative with the NG-Test® CTX-M MULTI) and the CR-E isolates, the total DNA was extracted using the PureLink™ Genomic DNA Mini-Kit (ThermoFisher Scientific, Waltham, MA, United States) following the manufacturer’s instructions and stored at -20°C. Genomic DNA was used for library preparation using the NEBNext Ultra II FS DNA Library Prep Kit for Illumina (NEB, France) according to the manufacturer’s instructions. Whole genome sequencing was performed on an Illumina NextSeq 500 instrument (Illumina). After sequencing, raw data were assembled de novo using the CLC genomics 10.2 program (Qiagen, Les Ulis, France), and the genomes were analyzed online using software available at the Center for Genomic Epidemiology-CGE.3 The latter included multilocus sequence typing (MLST) with the CGE MLST 2.0 software to determine sequence types (ST), and acquired resistance gene determinations using ResFinder 4.1 (25–27). Similarly, plasmid replicon types and virulence genes were identified using PlasmidFinder 2.0 (26, 28) and VirulenceFinder 2.0., respectively (26, 29, 30). Reference plasmids sequence were retrieved from the NCBI database, using a local BLAST algorithm. Reads and/or Contigs carrying carbapenemase genes were mapped to reference plasmids, using the CLC genomics 10.2 program (Qiagen).
2.6 Plasmids from CR-E isolates
Plasmids were extracted from the carbapenem-resistant isolates by the Kieser method as previously described (31). Transfer of plasmid-borne resistance markers was assessed by electroporation of the plasmids into electro-competent E. coli TOP10 (Invitrogen, Saint-Aubin, France). Transformants were selected on Trypticase soy agar (TSA) supplemented with ticarcillin (100 μg/mL). Transformants were PCR screened for the carbapenemase genes likely transferred to the recipient E. coli. Plasmids were visualized using electrophoresis on 0.7% agarose. E. coli NCTC 50192, which harbors four plasmids (7, 48, 66, and 154 kb) was used as size markers during electrophoresis (31).
2.7 Typing of the CR-E isolates using infrared spectrometry
The CR-E isolates were typed using the Bruker IR Biotyper spectrometer (IRBT, Bruker, Hamburg, Germany). An amount of 1 μL of bacterial colonies selected from the confluent part of the culture was re-suspended in 50 μL of 70% ethanol solution in an IR Biotyper suspension vial. After vortexing, 50 μL of deionized water was added, and the solution was mixed by pipetting. The bacterial suspensions (15 μL) were spotted in three technical replicates onto the 96-spot silicon IR Biotyper target and let dry for 15–20 min at 35°C ± 2°C. In each run, prior to sample spectra acquisition, quality control was performed with the Infrared Test Standards (IRTS 1 and 2) of the IR Biotyper kit. IRTS 1 and IRTS 2 were re-suspended in 90 μL deionized water and 90 μL of absolute ethanol was added and mixed. Subsequently, 12 μL of the suspension was spotted onto the IR Biotyper target and let dry as previously described. The relationships between the isolates were analyzed using the Bruker IR Biotyper Software (version 2.1.0.195, Bruker) (32). An online tool4 was used to assess the quantitative data of discriminatory power and concordance of the typing methods. Simpson’s index of diversity (SID) was used to evaluate the discriminatory power of the typing method, calculating the probability that two unrelated isolates from the test strain set will be clustered into different typing groups. Adjusted Rand index (ARI) with 95% confidence intervals was used to evaluate the concordance of IRBT typing results (33).
3 Results
3.1 Bacterial isolates
Based on the susceptibility profile (Resistance to ESC and/or carbapenems) derived from the microbiology laboratories of the three participating hospitals, 188 isolates, E. coli (n = 151; 80.3%), K. pneumoniae (n = 20; 10.6%), Enterobacter spp. (n = 7; 3.7%), Proteus mirabilis (n = 6; 3.2%), Salmonella spp. (n = 3; 1.6%), and Serratia marcescens (n = 1; 0.5%) were collected. Using the Kirby-Bauer disk diffusion assay, only 90 isolates could be confirmed and were included in this study. This collection comprised E. coli (n = 70; 77.8%), K. pneumoniae (n = 13; 14.4%), Enterobacter spp. (n = 6; 6.7%), and Proteus mirabilis (n = 1; 1.1%). Routine antimicrobial susceptibility testing revealed that out of these 90 isolates, 57 were only ESBL producers (e.g., fully susceptible to carbapenems), while the remaining 33 (i.e., 26 E. coli, five K. pneumoniae, one E. cloacae, and one Enterobacter hormaechei) were resistant to at least one carbapenem, of which 20 were also ESBL-producers, as revealed by synergy images between ESCs and clavulanic acid containing disks.
3.2 Susceptibility testing and ESBL gene characterization among the ESBL-producers
The 57 ESBL-producing isolates (Table S1) were composed of E. coli (n = 44; 77.2%), K. pneumoniae (n = 8; 14%), Enterobacter spp. (n = 4; 7%), and Proteus mirabilis (n = 1; 1.8%). These isolates were MDR and remained consistently susceptible only to carbapenems.
The NG-Test® CTX-M MULTI showed that all but two isolates were CTX-M positive (Table S1). PCR amplification of the entire blaCTX-M-gene and subsequent sequencing revealed that blaCTX-M-15 was predominant (91.2%, 52/55), followed by blaCTX-M-55 (3.5%, 2/55) and blaCTX-M-3 gene (1.8%, 1/55). The whole genome sequencing analysis showed that the two non-CTX-M producing ESBL isolates: one E. coli isolate harboring a blaSHV-12 ESBL gene and one K. pneumoniae isolate harboring a chromosomally encoded blaSHV-187 gene.
3.3 Carbapenemase detection and susceptibility testing of CR-E isolates
The Carba NP hydrolysis test and the NG-Test® CARBA-5, are displayed in Table 1 and Table S2. Among the 33 CR-E isolates, 23 were positive using the Carba NP test, while the remaining isolates were repeatedly negative. Using the NG-Test® CARBA-5 ICA, all 33 isolates were CPs: 17 isolates (51.5%) were positive for NDM and 16 (48.5%) for OXA-48-like enzymes. Out of the 26 E. coli isolates different AMR phenotypes were observed (Figure S1). NDM (n = 14) and OXA-48-like (n = 12) were the only carbapenemases detected in these isolates. Three K. pneumoniae isolates were positive for OXA-48-like, and two for NDM. The two E. cloacae complex isolates produced either an OXA-48-like or an NDM.
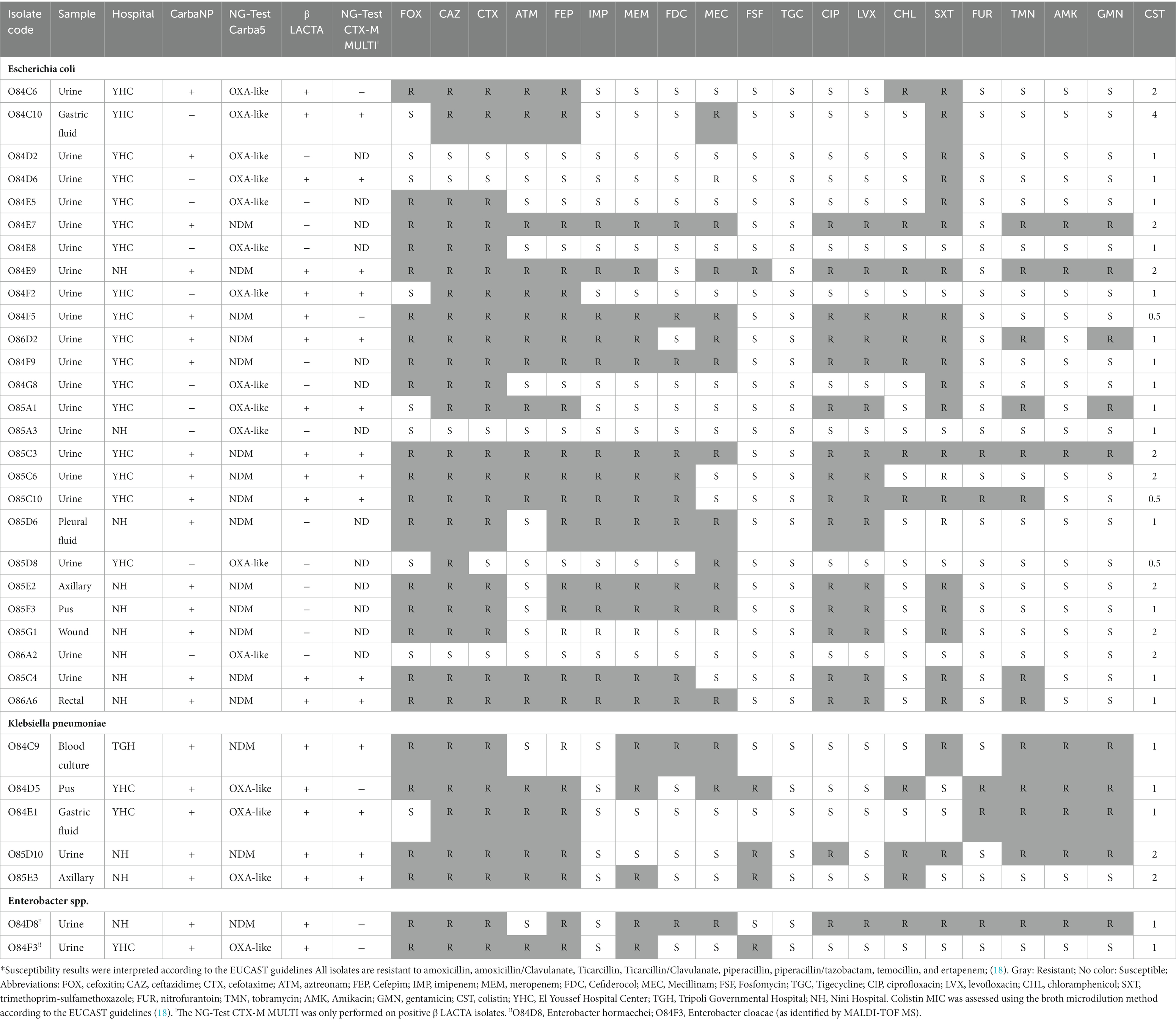
Table 1. Antibiotic susceptibility patterns using Kirby-Bauer disk diffusion of carbapenemase-producing Enterobacterales (expect colistin).
Using disk diffusion antibiograms, all CR-E isolates were found to be resistant to nearly all antibiotics tested routinely in Lebanon, including ertapenem (n = 33; 100%). However, 31% (8/26) of these E. coli isolates were found susceptible to ertapenem (i.e., MIC ≤0.5 mg/L) using the broth microdilution method; of them, six isolates were negative with the Carba NP test, while all were positive by ICA for OXA-48. While NDM- and some OXA-48-like- producing E. coli displayed high MIC levels for temocillin, surprisingly most isolates (75%, 9/12) carrying blaOXA-48-like had relatively low MICs (≤ 64 μg/mL) for this antibiotic (as compared to 14.3%; 2/14) of the NDM-producing E. coli isolates.
Colistin MIC results revealed that almost all CR-E isolates (97%, 32/33) remained consistently susceptible to this antibiotic (MIC ≤2 mg/L). Furthermore, the activity of different last-resort beta-lactam and non-beta-lactam antibiotics was assessed against a subpopulation of CR-E. coli isolates (6 blaNDM and 7 blaOXA-48-like-producers). All the tested isolates were resistant to ceftaroline and ceftobiprole but susceptible to eravacyclin, nitrofurantoin, apramycin, and tigecycline. Resistance to cefiderocol, ceftazidime/avibactam, imipenem/relebactam, and meropenem/vaborbactam was observed among the blaNDM-producing isolates (Table 2). Additionally, increased MICs to aztreonam/avibactam (4, 8, and 16 mg/L) were noticed among blaNDM-producing E. coli isolates.
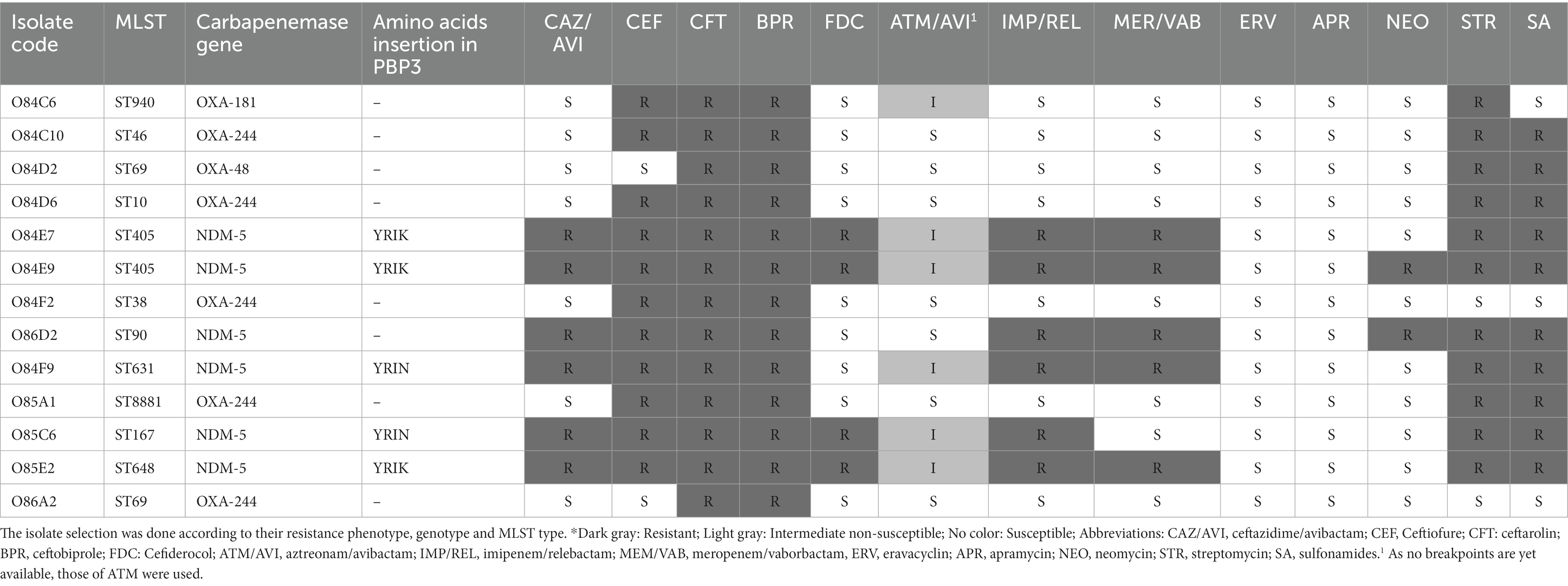
Table 2. Antibiotic susceptibility patterns of selected carbapenemase-producing Escherichia coli isolates for antibiotics considered last resort molecules using broth microdilution test (Sensititre), and interpreted according to the EUCAST guidelines (18).
The whole genome sequencing of the 33 CR-E isolates was performed. After read assembly, the contigs were submitted to ResFinder 4.0. In total, 47 different resistance genes were identified (Table S3), which code for resistance determinants to clinically-important classes of antibiotics, including β-lactams, aminoglycosides, tetracycline, quinolones, trimethoprim-sulfamethoxazole, fosfomycin, and sulfonamides. Among E. coli isolates, the most frequently identified carbapenemase was NDM-5 (53.8%; 14/26), followed by OXA-244 (38.5%; 10/26), OXA-181 (3.8%; 1/26), and OXA-48 (3.8%, 1/26). OXA-48 has also been identified in three K. pneumoniae and one E. cloacae isolates, while NDM-1 (n = 3, 9.1%) was detected in two K. pneumoniae and one E. hormaechei isolates (Table 2). Notably, two plasmid-encoded cephalosporinases, blaCMY-145 and blaDHA-1 genes that confer resistance to ESCs were detected in three E. coli isolates. ESBL genes such as blaCTX-M-15 (n = 15), blaCTX-M-27 (n = 1), blaTEM-35 (n = 1), blaSHV-33 (n = 1), blaSHV-12 (n = 2), blaSHV-26 (n = 1), were also identified. Specifically, the blaCTX-M-15 gene was the most frequently detected in E. coli (n = 11; including one isolate co-harboring two CTX-M alleles: blaCTX-M-15 and blaCTX-M-27) and K. pneumoniae (n = 4) isolates. 16S RNA methylase genes were detected among 6 isolates including 5 blaNDM-5-producing E. coli and one blaNDM-1-producing E. hormaechei isolate.
MLST analysis using the whole genome sequence showed that the CR-E. coli belonged to 11 different STs; including ST69 (n = 6 isolates), followed by ST648 (n = 4), ST167 (n = 3), ST361 (n = 3), ST405 (n = 3), ST10 (n = 2), ST90 (n = 1), ST940 (n = 1), ST38 (n = 1), ST46 (n = 1), and ST8881 (n = 1). Additionally, the CR-K. pneumoniae isolates belonged to four different STs, namely ST35, ST37, ST45, and ST1770. E. cloacae and E. hormaechei belonged to ST1006 and ST182, respectively (Table S3).
Furthermore, alignment of the ftsI gene sequences with that of a wild-type gene (E. coli NCTC 9022, accession number LR134237) revealed a four amino-acid insertion in PBP3 after residue 333 in 13 of 14 NDM-5-producing E. coli isolates. Two types of insertions were detected: YRIN (n = 7) and YRIK (n = 6). YRIN insertion was found among isolates with ST167 and ST361, and YRIK insertion was present in isolates with ST648 and ST405. ST90 isolate contained neither YRIN nor YRIK insertions. These 4 AA insertions could be correlated with increased MICs to aztreonam/avibactam (4, 8, and 16 mg/L) and cefiderocol among blaNDM-5 producing E. coli isolates, as compared to similar isolates lacking a 4 AA insertion (0.06 mg/mL).
3.4 Virulence determinants and plasmids in the CR-Escherichia coli isolates
Virulence factors (VFs) in the CR-E. coli isolates were identified using the CGE VirulenceFinder 2.0. TraT protein, previously shown to mediate resistance to bacterial killing by serum, was detected in 14 E. coli isolates, including a blaOXA-48-positive isolate belonging to ST69. Genes encoding adhesins (ipfA, fimH, afaA, afaC, afaD, afaE) were found in five E. coli that were positive for blaOXA-244 (Table 3). Additionally, iss encoding an outer membrane lipoprotein that enhances serum resistance, was detected only in one blaOXA-244-positive E. coli isolate. The capsular genes, kpsE and kpsM, were both detected in eight isolates, of which three were blaNDM-5-positive (ST648), and 5 were blaOXA-244-positive (ST69). Most VFs were detected in two isolates belonging to ST69.
Using PlasmidFinder 2.1, 9 plasmid replicon types were identified. Specifically, the following plasmid types were detected in the E. coli isolates; Col (n = 9 isolates), IncFII (n = 18), IncX3/X4 (n = 4), IncI1-I (n = 3), and IncFIA (n = 11). Additionally, in the K. pneumoniae isolates, IncL (n = 3), IncM2 (n = 1), and IncFIB (n = 4) were detected. The E. cloacae and E. hormaechei isolates carried IncFIB/II and IncL/X3, respectively (Table 4). Transformants were obtained after Kieser plasmid extraction and electro-transformation for 25/33 isolates. For 8 blaOXA-244- producing isolates, even with repeated attempts no plasmids were observed on Kieser gel, and no transformants were obtained suggesting a chromosomal location. For K. pneumoniae and E. hormaechi, the blaOXA-48 gene was carried on an IncL plasmid.
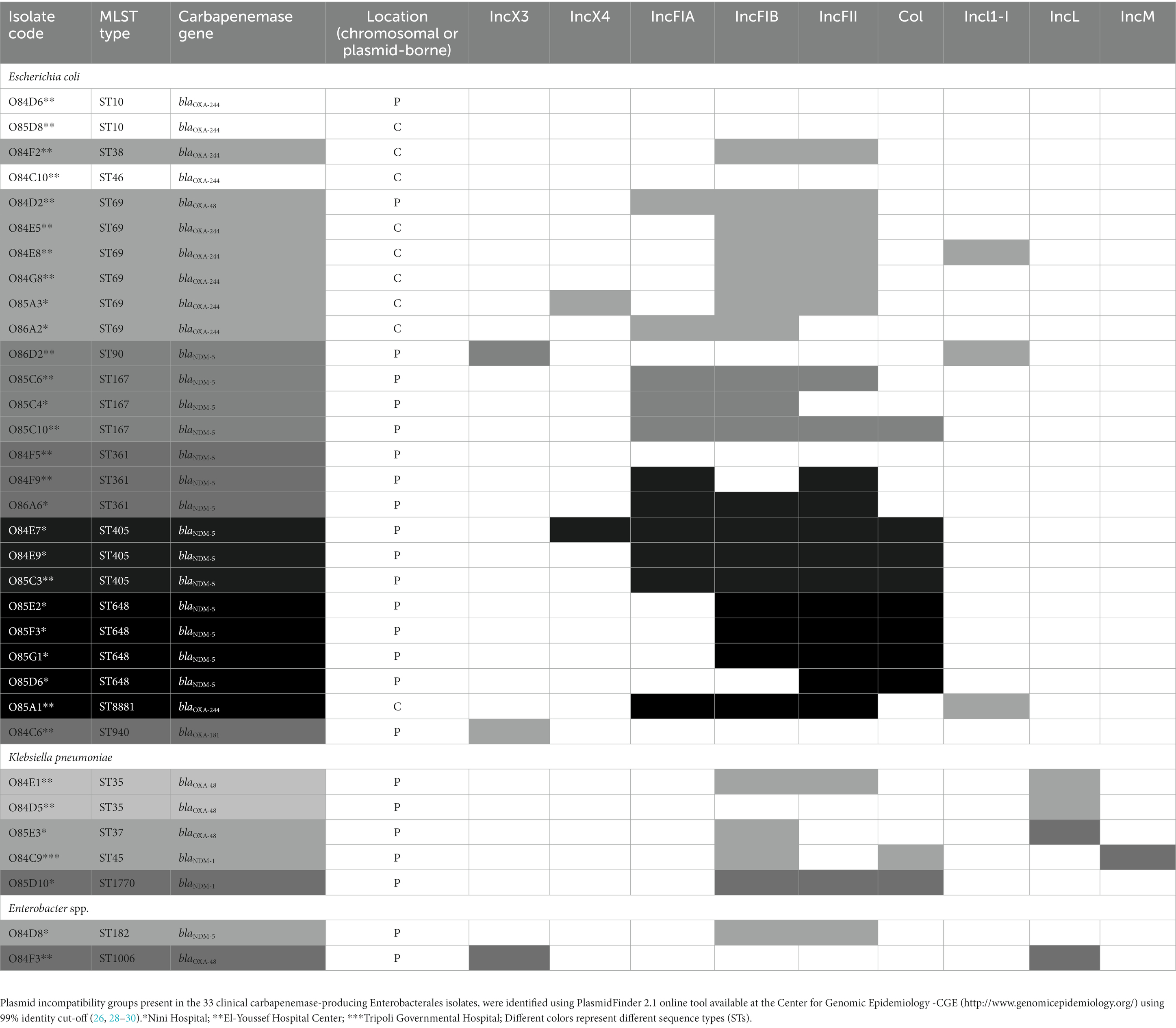
Table 4. Carbapenemase gene location was determined by plasmid extraction using Kieser technique and by applying a BLASTN algorithm for carbapenemase-genes-carrying contigs and then these contigs were mapped to reference plasmids using CLC genomics.
3.5 Genetic relatedness as revealed by IR Biotyper in comparison to WGS
Sixteen CR-E. coli and the five CR-K. pneumoniae isolates positive with the Carba NP test were assessed with the IR Biotyper for strain typing and the results were compared with those obtained by WGS analysis (Figures S2, S3). Overall IRBT results corroborated WGS for the typing of the five CR K. pneumoniae, which were classified into four IR types (Figure S2). However, IRBT differentiated 16 E. coli isolates that belonged to 7 STs into 11 IR types, with 8 IR types comprising only a single isolate (Table 5). The Simpson Index of Diversity (SID) was used to determine the discriminatory power of the typing methods. WGS had the highest discriminatory power (0.967) followed by IRBT (0.933), while the lowest SID was for MLST (0.875). Main discrepancies were observed between IRBT and WGS with E. coli ST-648 isolates (O85D6, O85E2, O85G1, and O85F3), which clustered into IR type 7 (Figure S3), but differed from each other by 53, 400, and 1,360 SNIPs.
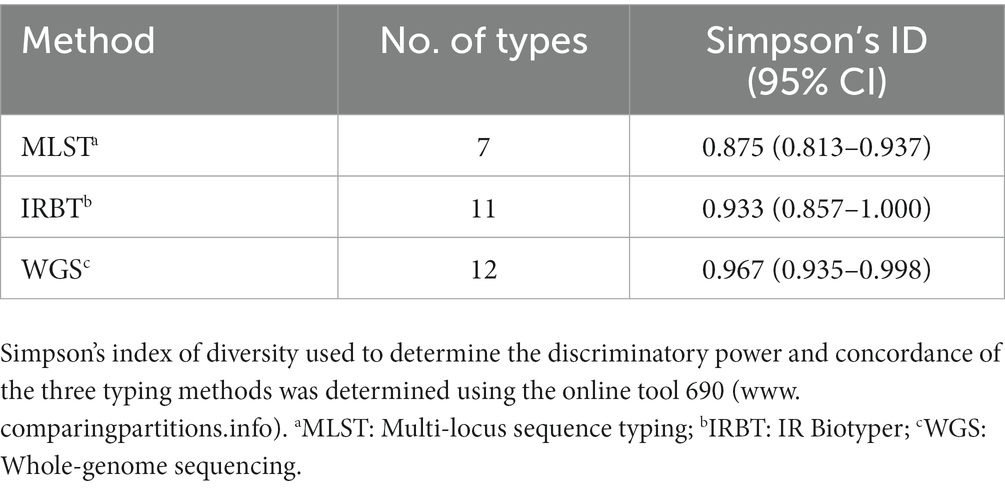
Table 5. Comparison of different typing methods for carbapenemase-producing Escherichia coli clinical isolates.
4 Discussion
The increase in ESCR- and CR-E isolates has been observed in hospitals worldwide. In Lebanon, two nationwide hospital-based retrospective studies documented a high prevalence of MDR pathogens, including ESBL-producing Enterobacterales (34% in 2016) (5). Recently, a community-based study revealed that approximately half of the population carries ESCR-E. coli (14), with an important dynamic of acquisition and loss of MDR strains and limited plasmid spread. The occurrence of Enterobacterales with decreased susceptibility to carbapenems, raised from 0.4% in 2008–2010 to 1.6% in 2012 and 3.3% in 2019 in hospitalized patients (12, 35). Despite the concerning increase and reports, there is a lack of molecular data on the epidemiology of ESBL- and CR-E in Lebanon.
Our results highlighted the predominance of the blaCTX-M-15 gene (52/57, 91.2%) among ESBL-producing isolates regardless of the co-existence of other ß-lactam resistance determinants, as observed globally (36). CTX-M-15-producing Enterobacterales clones have been widely reported in Lebanon among human, animal, and environmental sources (14, 15, 37–39). The finding that one single E. coli isolate may carry two CTX-M (−15 and − 27) variants capable of strongly hydrolyzing ceftazidime and belonging to two different groups of CTX-Ms (Group 1 and 9) is another example of concentration of restriction mechanisms.
Our findings further support the shift from blaOXA-48-like variants toward blaNDM-5 among E. coli in hospital settings in Lebanon (12, 35). OXA-48-like enzymes were the most prevalent carbapenemases among Enterobacterales in Lebanon over the last decade while NDM-5 was uncommon in hospital and community settings (12, 40). In our study, the blaNDM-5 gene has become the predominant carbapenemase gene (14 of 26 E. coli isolates). The blaOXA-244 gene (10/26) came in second place, while blaOXA-48 (1/33), and blaOXA-181 (1/33) genes are rare. OXA-244 producing E. coli isolates are increasingly described worldwide (41), and represents a threat to public health because of the difficulties in their detection using classical screening media based on carbapenems and temocillin (42). Indeed, our results confirmed that OXA-244-producing isolates have lower MICs to temocillin and carbapenems as compared to other CP-E, which results in the absence of growth on screening media and thus an underestimation and silent spread. Furthermore, these isolates were negative for the Carba NP test, and only the use of an ICA (here the NG-Test Carba5) revealed a positive signal for OXA-48-like carbapenemase, suggesting that both tests should be used together to maximize the chances of detecting all OXA-48-like carbapenemases. Additionally, the CR K. pneumoniae isolates harbored blaOXA-48 (3/5) and blaNDM-1 (2/5), while the E. cloacae and E. hormaechei isolates harbored blaOXA-48 and blaNDM-1, respectively.
MLST results demonstrated several distinct genetic backgrounds for the CR-E isolates, suggesting horizontal gene transfer of the carbapenemase gene carrying plasmids, particularly those carrying the blaNDM-5 gene, rather than a clonal spread of a single clone. Interestingly, we found that the carbapenemase genes were plasmid-borne (except in eight blaOXA-244-producing E. coli) and on different plasmid types (Table 4). Of the NDM-5-producing E. coli isolates, six and seven had the insertion of YRIN or YRIK in PBP3, respectively, which resulted in a significant increase of MICs to aztreonam/avibactam and cefiderocol as well as to other PBP3-targeting ß-lactams (34). Surprisingly, the blaOXA-244 gene was chromosomally encoded in 8 isolates, while it was plasmid-mediated in only two isolates belonging to ST69 and ST10. The chromosomal location of the blaOXA-244 gene was previously reported in ST38 and ST69 in France (43). Taken together, the occurrence of different resistance markers in diverse genetic backgrounds as well as plasmid types constitute a risk to patients, potentially highlighting a more severe problem and highlighting an urgent need to monitor and control the spread of resistance in hospitals in Lebanon.
The study identified various MLST types, including ST648, ST167, ST361, and ST405, in blaNDM-5-producing E. coli. These STs are recognized as high-risk global clones that contribute to the widespread dissemination of drug resistance determinants among Enterobacterales (44). For instance, ST648 is recognized as a major global ESBL-producing E. coli clone (45, 46), particularly associated with blaCTX-M-15, in humans, birds, and companion animals (47). Additionally, ST648 was identified in Lebanon among clinical CRE (48), including blaNDM-5-producing E. coli (49). Besides, ST167 has been linked to the global spread of blaNDM in humans, animals, and food (50). ST405 has been detected in several countries, including the United States (51), Japan (52), and Lebanon (48), allowing the transmission of blaCTX-M-15 and aac(6′)-Ib-cr genes (53). Notably, both ST361 and ST648 have been reported among Syrian refugees in Lebanon (54), and ST90 was found in the effluent of Al-Qaa refugee camp (55). Additionally, we identified ST38 among blaOXA-244-producing E. coli, previously described in estuary water in Lebanon (56). Several European countries have reported increased dissemination of the blaOXA-244 gene (57, 58), with ST38 being the most common sequence type among blaOXA-244-producing E. coli isolates (59). Moreover, ST38 blaOXA-48-producing E. coli has also been found in fowls in Lebanon (60). As observed in our study, ST940 E. coli carrying the blaOXA-181 gene was previously reported at the American University of Beirut Medical Center (61). Among the K. pneumoniae isolates, there were five isolates belonging to four different sequence types. One of them, K. quasipneumoniae subsp. similipneumoniae ST1770, has been previously reported in hospital wastewater effluents in Japan (62), but had not been reported in Lebanon. Additionally, clinical K. pneumoniae isolates ST35 and ST45 have been documented in Lebanon (63, 64). ST37 has also been closely associated with ESBLs (65). Furthermore, the ST182 blaNDM-1 E. hormaechei isolate, previously reported in Lebanon (66), has been frequently isolated from clinical specimens in China, Mexico, the Czech Republic, and the United States (67).
Although there is a paramount need to monitor the spread of critical AMR strains in hospitals in Lebanon, these efforts are complicated by the unavailability of resources. Molecular typing methods such as whole genome sequencing are relatively time-consuming and expensive (32). Therefore, we evaluated the Bruker IR Biotyper for reliable detection of the relatedness and discrimination between strains. Our findings showed that Fourier Transform Infrared (FT-IR) technology is a powerful tool for strain typing, showing slightly better results to MLST and comparable results to WGS among CR-E. coli and K. pneumoniae isolates. Its advantages are summed up in the simple preparation of samples, ease of use, and low running costs. Together with its relatively high discriminatory power, the FT-IR seems to be a good tool for outbreaks real-time surveillance and infection control in clinical settings.
In conclusion, our study showed that in-depth studies are crucial to better understand the emergence and dissemination of drug-resistant determinants within and across healthcare institutions. Specifically, we highlighted an unprecedented diversity of ESBL- and CR-E determinants compared to other studies in Lebanon. Furthermore, the MLST and the associated plasmid types suggested that these determinants were circulating in diverse strains, complicating the control efforts and suggesting the need for evidence-based antimicrobial stewardship programs. In the distressing situation of Lebanon, the accessibility to novel antibiotic molecules remains a major concern, impacting public health. The economic crisis had a profound impact leading to significant challenges in antibiotics procurement and even more so to new molecules, which are unavailable and not yet routinely tested. Furthermore, our data support that the fight against MDR bacteria in LMICs, such as Lebanon, requires a comprehensive One Health approach because of the diffuse sources and factors that affect the spread of resistance in hospitals and the community. The latter corroborates our previous research that showed a wide reliance on and sometimes indiscriminate use of critically important antibiotics in healthcare settings, agriculture, and the community in Lebanon (8, 9). This approach and science-based interventions are urgently needed to control the spread of AMR in Lebanon, which represents an essential threat nationally and globally as AMR is known to spill across international borders.
Data availability statement
The datasets presented in this study can be found in online repositories. The names of the repository/repositories and accession number(s) can be found at http://www.ncbi.nlm.nih.gov/bioproject and PRJNA973232.
Ethics statement
The studies involving humans were approved by Azm Center/Lebanese University ethical committee and the Lebanese Ministry of Public Health (CE-EDST-1-2020). The studies were conducted in accordance with the local legislation and institutional requirements. The human samples used in this study were acquired from bacterial isolates collected based on susceptibility profiles from three hospitals. Written informed consent for participation was not required from the participants or the participants’ legal guardians/next of kin in accordance with the national legislation and institutional requirements.
Author contributions
DD: Conceptualization, Formal analysis, Investigation, Writing – original draft, Writing – review & editing. SO: Supervision, Validation, Writing – review & editing. MR: Investigation, Writing – review & editing. IK: Investigation, Resources, Writing – review & editing. HM: Investigation, Writing – review & editing. AB: Formal analysis, Validation, Writing – review & editing. DG: Formal analysis, Investigation, Writing – review & editing. MH: Methodology, Writing – review & editing. FD: Formal analysis, Investigation, Writing – review & editing. MO: Conceptualization, Funding acquisition, Investigation, Methodology, Project administration, Validation, Writing – original draft, Writing – review & editing. TN: Conceptualization, Funding acquisition, Investigation, Methodology, Writing – original draft, Writing – review & editing, Project administration, Validation.
Funding
The author(s) declare financial support was received for the research, authorship, and/or publication of this article. This work was supported by the Assistance Publique-Hôpitaux de Paris, the transdisciplinary Health and Therapeutic Innovation object (HEALTHI) of the Université Paris-Saclay, through a grant from the French National research Agency (ANR-10-LABX-33), and the ANR-BMBF French-German bilateral project Natural-Arsenal (ANR-19-AMRB-0004). Marwan Osman and Dina Daaboul were supported by the Atkinson Postdoctoral Fellowship (Cornell University) and SAFAR Doctoral Scholarship (Lebanese University and French Embassy in Lebanon), respectively.
Acknowledgments
We are grateful to NG Biotech for providing the NG-Test® CARBA-5 and CTX-M MULTI free of charge.
Conflict of interest
The authors declare that the research was conducted in the absence of any commercial or financial relationships that could be construed as a potential conflict of interest.
Publisher’s note
All claims expressed in this article are solely those of the authors and do not necessarily represent those of their affiliated organizations, or those of the publisher, the editors and the reviewers. Any product that may be evaluated in this article, or claim that may be made by its manufacturer, is not guaranteed or endorsed by the publisher.
Supplementary material
The Supplementary material for this article can be found online at: https://www.frontiersin.org/articles/10.3389/fpubh.2023.1290912/full#supplementary-material
Footnotes
1. ^ https://www.eucast.org/clinical_breakpoints
2. ^ https://www.sfm-microbiologie.org/wp-content/uploads/2021/12/CASFM_VET2021.pdf
References
1. Nordmann, P, Naas, T, and Poirel, L. Global spread of Carbapenemase-producing Enterobacteriaceae. Emerg Infect Dis. (2011) 17:1791–8. doi: 10.3201/eid1710.110655
2. Matar, GM, Andremont, A, and Bazzi, W. Editorial: combating antimicrobial resistance - a one health approach. Front Cell Infect Microbiol. (2019) 9:458. doi: 10.3389/fcimb.2019.00458
3. Iovleva, A, and Doi, Y. Carbapenem-Resistant Enterobacteriaceae. Clin Lab Med. (2017) 37:303–15. doi: 10.1016/j.cll.2017.01.005
4. Papp-Wallace, KM, Endimiani, A, Taracila, MA, and Bonomo, RA. Carbapenems: past, present, and future. Antimicrob Agents Chemother. (2011) 55:4943–60. doi: 10.1128/AAC.00296-11
5. Osman, M, Kasir, D, Kassem, II, and Hamze, M. Shortage of appropriate diagnostics for antimicrobial resistance in Lebanese clinical settings: a crisis amplified by COVID-19 and economic collapse. J Glob Antimicrob Resist. (2021) 27:72–4. doi: 10.1016/j.jgar.2021.08.008
6. Kassem, II, and Osman, M. A brewing storm: the impact of economic collapse on the access to antimicrobials in Lebanon. J Glob Antimicrob Resist. (2022) 29:313–5. doi: 10.1016/j.jgar.2022.04.023
7. Osman, M, Cummings, KJ, El Omari, K, and Kassem, II. Catch-22: war, refugees, COVID-19, and the scourge of antimicrobial resistance. Front Med (Lausanne). (2022) 9:921921. doi: 10.3389/fmed.2022.921921
8. Al Omari, S, Al Mir, H, Wrayde, S, Merhabi, S, Dhaybi, I, Jamal, S, et al. First Lebanese antibiotic awareness week campaign: knowledge, attitudes and practices towards antibiotics. J Hosp Infect. (2019) 101:475–9. doi: 10.1016/j.jhin.2018.07.009
9. Kassem, II, Hijazi, MA, and Saab, R. On a collision course: the availability and use of colistin-containing drugs in human therapeutics and food-animal farming in Lebanon. J Glob Antimicrob Resist. (2019) 16:162–4. doi: 10.1016/j.jgar.2019.01.019
10. Osman, M, Rafei, R, Ismail, MB, Omari, SA, Mallat, H, Dabboussi, F, et al. Antimicrobial resistance in the protracted Syrian conflict: halting a war in the war. Future Microbiol. (2021) 16:825–45. doi: 10.2217/fmb-2021-0040
11. Kassem, II, Osman, M, Jaafar, H, and El Omari, K. Refugee settlements, sewage pollution, COVID-19 and the unfolding cholera outbreak in Lebanon. J Travel Med. (2022) 29:taac142. doi: 10.1093/jtm/taac142
12. Rima, M, Oueslati, S, Dabos, L, Daaboul, D, Mallat, H, Bou Raad, E, et al. Prevalence and molecular mechanisms of carbapenem resistance among gram-negative bacilli in three hospitals of northern Lebanon. Antibiotics (Basel). (2022) 11:1295. doi: 10.3390/antibiotics11101295
13. Moghnieh, R, Araj, GF, Awad, L, Daoud, Z, Mokhbat, JE, Jisr, T, et al. A compilation of antimicrobial susceptibility data from a network of 13 Lebanese hospitals reflecting the national situation during 2015-2016. Antimicrob Resist Infect Control. (2019) 8:41. doi: 10.1186/s13756-019-0487-5
14. Al-Mir, H, Osman, M, Drapeau, A, Hamze, M, Madec, JY, and Haenni, M. Spread of ESC-, carbapenem- and colistin-resistant Escherichia coli clones and plasmids within and between food workers in Lebanon. J Antimicrob Chemother. (2021) 76:3135–43. doi: 10.1093/jac/dkab327
15. Al-Mir, H, Osman, M, Drapeau, A, Hamze, M, Madec, JY, and Haenni, M. WGS analysis of clonal and plasmidic epidemiology of colistin-resistance mediated by mcr genes in the poultry sector in Lebanon. Front Microbiol. (2021) 12:624194. doi: 10.3389/fmicb.2021.624194
16. Nasser, NA, Mann, D, Li, S, Deng, X, and Kassem, II. Draft genome sequences of colistin-resistant and mcr-1.1-carrying Escherichia coli strains isolated from irrigation water. Microbiol Resour Announc. (2021) 10:e00120-21. doi: 10.1128/MRA.00120-21
17. Gilchrist, CA, Turner, SD, Riley, MF, Petri, WA Jr, and Hewlett, EL. Whole-genome sequencing in outbreak analysis. Clin Microbiol Rev. (2015) 28:541–63. doi: 10.1128/CMR.00075-13
18. CASFM-European Society of Clinical Microbiology and Infectious Disease Guidelines. Recommandations. (2023).
19. Renvoisé, A, Decré, D, Amarsy-Guerle, R, Huang, TD, Jost, C, Podglajen, I, et al. Evaluation of the βLacta test, a rapid test detecting resistance to third-generation cephalosporins in clinical strains of Enterobacteriaceae. J Clin Microbiol. (2013) 51:4012–7. doi: 10.1128/JCM.01936-13
20. Bernabeu, S, Ratnam, KC, Boutal, H, Gonzalez, C, Vogel, A, Devilliers, K, et al. A lateral flow immunoassay for the rapid identification of CTX-M-producing Enterobacterales from culture plates and positive blood cultures. Diagnostics (Basel). (2020) 10:764. doi: 10.3390/diagnostics10100764
21. Nordmann, P, Poirel, L, and Dortet, L. Rapid detection of carbapenemase-producing Enterobacteriaceae. Emerg Infect Dis. (2012) 18:1503–7. doi: 10.3201/eid1809.120355
22. Boutal, H, Vogel, A, Bernabeu, S, Devilliers, K, Creton, E, Cotellon, G, et al. A multiplex lateral flow immunoassay for the rapid identification of NDM-, KPC-, IMP- and VIM-type and OXA-48-like carbapenemase-producing Enterobacteriaceae. J Antimicrob Chemother. (2018) 73:909–15. doi: 10.1093/jac/dkx521
23. Karim, A, Poirel, L, Nagarajan, S, and Nordmann, P. Plasmid-mediated extended-spectrum beta-lactamase (CTX-M-3 like) from India and gene association with insertion sequence ISEcp1. FEMS Microbiol Lett. (2001) 201:237–41. doi: 10.1111/j.1574-6968.2001.tb10762.x
24. Poirel, L, Decousser, JW, and Nordmann, P. Insertion sequence ISEcp1B is involved in expression and mobilization of a Bla(CTX-M) beta-lactamase gene. Antimicrob Agents Chemother. (2003) 47:2938–45. doi: 10.1128/AAC.47.9.2938-2945.2003
25. Bortolaia, V, Kaas, RS, Ruppe, E, Roberts, MC, Schwarz, S, Cattoir, V, et al. ResFinder 4.0 for predictions of phenotypes from genotypes. J Antimicrob Chemother. (2020) 75:3491–500. doi: 10.1093/jac/dkaa345
26. Camacho, C, Coulouris, G, Avagyan, V, Ma, N, Papadopoulos, J, Bealer, K, et al. BLAST+: architecture and applications. BMC bioinformatics. (2009) 10:421. doi: 10.1186/1471-2105-10-421
27. Zankari, E, Allesøe, R, Joensen, KG, Cavaco, LM, Lund, O, and Aarestrup, FM. PointFinder: a novel web tool for WGS-based detection of antimicrobial resistance associated with chromosomal point mutations in bacterial pathogens. J Antimicrob Chemother. (2017) 72:2764–8. doi: 10.1093/jac/dkx217
28. Carattoli, A, Zankari, E, García-Fernández, A, Voldby Larsen, M, Lund, O, Villa, L, et al. In silico detection and typing of plasmids using PlasmidFinder and plasmid multilocus sequence typing. Antimicrob Agents Chemother. (2014) 58:3895–903. doi: 10.1128/AAC.02412-14 (Accessed November 2, 2023).
29. Joensen, KG, Scheutz, F, Lund, O, Hasman, H, Kaas, RS, Nielsen, EM, et al. Real-time whole-genome sequencing for routine typing, surveillance, and outbreak detection of verotoxigenic Escherichia coli. J Clin Microbiol. (2014) 52:1501–10. doi: 10.1128/JCM.03617-13
30. Malberg Tetzschner, AM, Johnson, JR, and Johnston, BD. In silico genotyping of Escherichia coli isolates for extraintestinal virulence genes by use of whole-genome sequencing data. J Clin Microbiol. (2020) 58:e01269-20. doi: 10.1128/JCM.01269-20
31. Kieser, T. Factors affecting the isolation of CCC DNA from Streptomyces lividans and Escherichia coli. Plasmid. (1984) 12:19–36. doi: 10.1016/0147-619X(84)90063-5
32. Alhasabi, J, Balakrishnan, I, and Wey, E. Application of the Bruker IR Biotyper for strain typing during an outbreak. Access Microbiol. (2020) 2. doi: 10.1099/acmi.fis2019.po0086
33. Carriço, JA, Silva-Costa, C, Melo-Cristino, J, Pinto, FR, de Lencastre, H, Almeida, JS, et al. Illustration of a common framework for relating multiple typing methods by application to macrolide-resistant Streptococcus pyogenes. J Clin Microbiol. (2006) 44:2524–32. doi: 10.1128/JCM.02536-05
34. Patino-Navarrete, R, Rosinski-Chupin, I, Cabanel, N, Gauthier, L, Takissian, J, Madec, JY, et al. Stepwise evolution and convergent recombination underlie the global dissemination of carbapenemase-producing Escherichia coli. Genome Med. (2020) 12:10. doi: 10.1186/s13073-019-0699-6
35. Beyrouthy, R, Robin, F, Dabboussi, F, Mallat, H, Hamze, M, and Bonnet, R. Carbapenemase and virulence factors of Enterobacteriaceae in North Lebanon between 2008 and 2012: evolution via endemic spread of OXA-48. J Antimicrob Chemother. (2014) 69:2699–705. doi: 10.1093/jac/dku181
36. Bevan, ER, Jones, AM, and Hawkey, PM. Global epidemiology of CTX-M β-lactamases: temporal and geographical shifts in genotype. J Antimicrob Chemother. (2017) 72:2145–55. doi: 10.1093/jac/dkx146
37. Diab, M, Hamze, M, Madec, JY, and Haenni, M. High prevalence of non-ST131 CTX-M-15-producing Escherichia coli in healthy cattle in Lebanon. Microb Drug Resist. (2017) 23:261–6. doi: 10.1089/mdr.2016.0019
38. Al-Mir, H, Osman, M, Azar, N, Madec, JY, Hamze, M, and Haenni, M. Emergence of clinical mcr-1-positive Escherichia coli in Lebanon. J Glob Antimicrob Resist. (2019) 19:83–4. doi: 10.1016/j.jgar.2019.08.019
39. Kassem, II, Hassan, J, Esseili, MA, Mann, D, Osman, M, Li, S, et al. Draft genome sequences of multidrug-resistant Escherichia coli isolated from river water. Microbiol Resour Announc. (2022) 11:e0082722. doi: 10.1128/mra.00827-22
40. Fadlallah, M, Salman, A, and Salem-Sokhn, E. Updates on the status of carbapenem-resistant Enterobacterales in Lebanon. Int J Microbiol. (2023) 2023:1–10. doi: 10.1155/2023/8831804
41. Pitout, JDD, Peirano, G, Kock, MM, Strydom, K-A, and Matsumura, Y. The global ascendency of OXA-48-type carbapenemases. Clin Microbiol Rev. (2019) 33:e00102-19. doi: 10.1128/cmr.00102-19
42. Rima, M, Emeraud, C, Bonnin, RA, Gonzalez, C, Dortet, L, Iorga, BI, et al. Biochemical characterization of OXA-244, an emerging OXA-48 variant with reduced β-lactam hydrolytic activity. J Antimicrob Chemother. (2021) 76:2024–8. doi: 10.1093/jac/dkab142
43. Emeraud, C, Girlich, D, Bonnin, RA, Jousset, AB, Naas, T, and Dortet, L. Emergence and polyclonal dissemination of OXA-244-producing Escherichia coli. France Emerg Infect Dis. (2021) 27:1206–10. doi: 10.3201/eid2704.204459
44. Mathers, AJ, Peirano, G, and Pitout, JD. The role of epidemic resistance plasmids and international high-risk clones in the spread of multidrug-resistant Enterobacteriaceae. Clin Microbiol Rev. (2015) 28:565–91. doi: 10.1128/CMR.00116-14
45. Ewers, C, Bethe, A, Stamm, I, Grobbel, M, Kopp, PA, Guerra, B, et al. CTX-M-15-D-ST648 Escherichia coli from companion animals and horses: another pandemic clone combining multiresistance and extraintestinal virulence? J Antimicrob Chemother. (2014) 69:1224–30. doi: 10.1093/jac/dkt516
46. Peirano, G, van der Bij, AK, Gregson, DB, and Pitout, JD. Molecular epidemiology over an 11-year period (2000 to 2010) of extended-spectrum beta-lactamase-producing Escherichia coli causing bacteremia in a centralized Canadian region. J Clin Microbiol. (2012) 50:294–9. doi: 10.1128/JCM.06025-11
47. Liu, X, Thungrat, K, and Boothe, DM. Occurrence of OXA-48 carbapenemase and other beta-lactamase genes in ESBL-producing multidrug resistant Escherichia coli from dogs and cats in the United States, 2009-2013. Front Microbiol. (2016) 7:1057. doi: 10.3389/fmicb.2016.01057
48. Dagher, C, Salloum, T, and Alousi, S. Molecular characterization of carbapenem resistant Escherichia coli recovered from a tertiary hospital in Lebanon. PLoS One. (2018) 13:e0203323. doi: 10.1371/journal.pone.0203323
49. Hodroj, Z. Utilizing NGS in AMR surveillance in North Lebanon - a one health approach American University of Beirut (2023) 12:3981–85.
50. Baloch, Z, Lv, L, Yi, L, Wan, M, Aslam, B, Yang, J, et al. Emergence of almost identical F36:A-:B32 plasmids carrying Bla(NDM-5) and qepA in Escherichia coli from both Pakistan and Canada. Infect Drug Resist. (2019) 12:3981–5. doi: 10.2147/IDR.S236766
51. Tian, GB, Rivera, JI, Park, YS, Johnson, LE, Hingwe, A, Adams-Haduch, JM, et al. Sequence type ST405 Escherichia coli isolate producing QepA1, CTX-M-15, and RmtB from Detroit, Michigan. Antimicrob Agents Chemother. (2011) 55:3966–7. doi: 10.1128/AAC.00652-11
52. Matsumura, Y, Yamamoto, M, Nagao, M, Ito, Y, Takakura, S, and Ichiyama, S. Association of fluoroquinolone resistance, virulence genes, and IncF plasmids with extended-spectrum-β-lactamase-producing Escherichia coli sequence type 131 (ST131) and ST405 clonal groups. Antimicrob Agents Chemother. (2013) 57:4736–42. doi: 10.1128/AAC.00641-13
53. Coque, TM, Novais, A, Carattoli, A, Poirel, L, Pitout, J, Peixe, L, et al. Dissemination of clonally related Escherichia coli strains expressing extended-spectrum beta-lactamase CTX-M-15. Emerg Infect Dis. (2008) 14:195–200. doi: 10.3201/eid1402.070350
54. Azour, A, Al-Bayssari, C, Dagher, TN, Fajloun, F, Fajloun, M, and Rolain, JM. Clonal dissemination of plasmid-mediated carbapenem and colistin resistance in refugees living in overcrowded camps in North Lebanon. Antibiotics (Basel). (2021) 10:1478. doi: 10.3390/antibiotics10121478
55. Tokajian, S, Moghnieh, R, Salloum, T, Arabaghian, H, Alousi, S, Moussa, J, et al. Extended-spectrum beta-lactamase-producing Escherichia coli in wastewaters and refugee camp in Lebanon. Future Microbiol. (2018) 13:81–95. doi: 10.2217/fmb-2017-0093
56. Diab, M, Hamze, M, Bonnet, R, Saras, E, Madec, JY, and Haenni, M. Extended-spectrum beta-lactamase (ESBL)- and carbapenemase-producing Enterobacteriaceae in water sources in Lebanon. Vet Microbiol. (2018) 217:97–103. doi: 10.1016/j.vetmic.2018.03.007
57. Falgenhauer, L, Nordmann, P, Imirzalioglu, C, Yao, Y, Falgenhauer, J, Hauri, AM, et al. Cross-border emergence of clonal lineages of ST38 Escherichia coli producing the OXA-48-like carbapenemase OXA-244 in Germany and Switzerland. Int J Antimicrob Agents. (2020) 56:106157. doi: 10.1016/j.ijantimicag.2020.106157
58. Hammerum, AM, Porsbo, LJ, Hansen, F, Roer, L, Kaya, H, Henius, A, et al. Surveillance of OXA-244-producing Escherichia coli and epidemiologic investigation of cases, Denmark, January 2016 to august 2019. Euro Surveill. (2020) 25:1900742. doi: 10.2807/1560-7917.ES.2020.25.18.1900742
59. Potron, A, Poirel, L, Dortet, L, and Nordmann, P. Characterisation of OXA-244, a chromosomally-encoded OXA-48-like β-lactamase from Escherichia coli. Int J Antimicrob Agents. (2016) 47:102–3. doi: 10.1016/j.ijantimicag.2015.10.015
60. Al Bayssari, C, Olaitan, AO, Dabboussi, F, Hamze, M, and Rolain, JM. Emergence of OXA-48-producing Escherichia coli clone ST38 in fowl. Antimicrob Agents Chemother. (2015) 59:745–6. doi: 10.1128/AAC.03552-14
61. Bitar, I, Dagher, C, Salloum, T, Araj, G, and Tokajian, S. First report of an Escherichia coli from Lebanon carrying an OXA-181 carbapenemase resistance determinant. J Glob Antimicrob Resist. (2018) 12:113–4. doi: 10.1016/j.jgar.2018.01.002
62. Takizawa, S, Soga, E, Hayashi, W, Sakaguchi, K, Koide, S, Tanabe, M, et al. Genomic landscape of Bla(GES-5)- and Bla(GES-24)-harboring gram-negative bacteria from hospital wastewater: emergence of class 3 integron-associated Bla(GES-24) genes. J Glob Antimicrob Resist. (2022) 31:196–206. doi: 10.1016/j.jgar.2022.09.005
63. Arabaghian, H, Salloum, T, Alousi, S, Panossian, B, Araj, GF, and Tokajian, S. Molecular characterization of carbapenem resistant Klebsiella pneumoniae and Klebsiella quasipneumoniae isolated from Lebanon. Sci Rep. (2019) 9:531. doi: 10.1038/s41598-018-36554-2
64. Nawfal Dagher, T, Al-Bayssari, C, Chabou, S, Baron, S, Hadjadj, L, Diene, SM, et al. Intestinal carriage of colistin-resistant Enterobacteriaceae at Saint Georges Hospital in Lebanon. J Glob Antimicrob Resist. (2020) 21:386–90. doi: 10.1016/j.jgar.2019.12.001
65. Deng, J, Li, YT, Shen, X, Yu, YW, Lin, HL, Zhao, QF, et al. Risk factors and molecular epidemiology of extended-spectrum β-lactamase-producing Klebsiella pneumoniae in Xiamen. China J Glob Antimicrob Resist. (2017) 11:23–7. doi: 10.1016/j.jgar.2017.04.015
66. Merhi, G, Amayri, S, Bitar, I, Araj, GF, and Tokajian, S. Whole genome-based characterization of multidrug resistant Enterobacter and Klebsiella aerogenes isolates from Lebanon. Microbiol Spectr. (2023) 11:e0291722. doi: 10.1128/spectrum.02917-22
Keywords: Enterobacterales, antimicrobial resistance, one health, ESBL, carbapenemases
Citation: Daaboul D, Oueslati S, Rima M, Kassem II, Mallat H, Birer A, Girlich D, Hamze M, Dabboussi F, Osman M and Naas T (2023) The emergence of carbapenemase-producing Enterobacterales in hospitals: a major challenge for a debilitated healthcare system in Lebanon. Front. Public Health. 11:1290912. doi: 10.3389/fpubh.2023.1290912
Edited by:
Spyros Pournaras, National and Kapodistrian University of Athens, GreeceReviewed by:
Konstantina Dafopoulou, University Hospital of Larissa, GreeceBlanca Pérez Viso, Ramón y Cajal University Hospital, Spain
Copyright © 2023 Daaboul, Oueslati, Rima, Kassem, Mallat, Birer, Girlich, Hamze, Dabboussi, Osman and Naas. This is an open-access article distributed under the terms of the Creative Commons Attribution License (CC BY). The use, distribution or reproduction in other forums is permitted, provided the original author(s) and the copyright owner(s) are credited and that the original publication in this journal is cited, in accordance with accepted academic practice. No use, distribution or reproduction is permitted which does not comply with these terms.
*Correspondence: Thierry Naas, dGhpZXJyeS5uYWFzQGFwaHAuZnI=; Marwan Osman, bWFyd2FuLm9zbWFuQHlhbGUuZWR1
†Present address Thierry Naas Associated French National Reference Center for Antibiotic Resistance: Carbapenemase-Producing Enterobacteriaceae, Le Kremlin-Bicêtre, France
‡These authors have contributed equally to this work