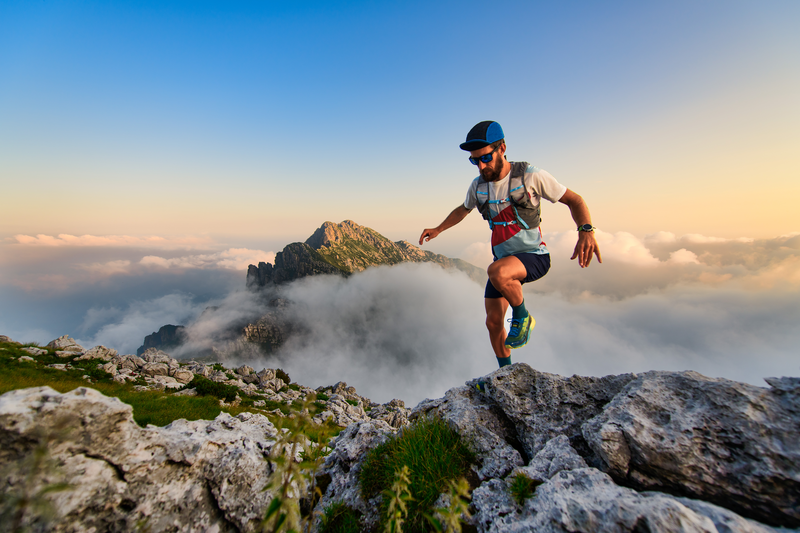
95% of researchers rate our articles as excellent or good
Learn more about the work of our research integrity team to safeguard the quality of each article we publish.
Find out more
REVIEW article
Front. Public Health , 23 November 2023
Sec. Environmental Health and Exposome
Volume 11 - 2023 | https://doi.org/10.3389/fpubh.2023.1285393
This article is part of the Research Topic Indoor Environment and Respiratory Diseases View all 5 articles
The quality of the indoor environment significantly impacts human health and productivity, especially given the amount of time individuals spend indoors globally. While chemical pollutants have been a focus of indoor air quality research, microbial contaminants also have a significant bearing on indoor air quality. This review provides a comprehensive overview of microbial contamination in built environments, covering sources, sampling strategies, and analysis methods. Microbial contamination has various origins, including human occupants, pets, and the outdoor environment. Sampling strategies for indoor microbial contamination include air, surface, and dust sampling, and various analysis methods are used to assess microbial diversity and complexity in indoor environments. The review also discusses the health risks associated with microbial contaminants, including bacteria, fungi, and viruses, and their products in indoor air, highlighting the need for evidence-based studies that can relate to specific health conditions. The importance of indoor air quality is emphasized from the perspective of the COVID-19 pandemic. A section of the review highlights the knowledge gap related to microbiological burden in indoor environments in developing countries, using India as a representative example. Finally, potential mitigation strategies to improve microbiological indoor air quality are briefly reviewed.
Around 90% of our time is spent indoors due to lifestyle changes and work habits; thus, indoor air quality is closely related to our health and comfort (1, 2). Many reports confirm that the concentration of pollutants can be 2 to 5 times higher inside than outside (3). In addition to the chemical pollutants, biological contaminants, such as bacteria, viruses, fungi, insects, mites, pollen, and pet dander, are also present in the indoor environment (4, 5). Microbial contaminants in the indoor environment can exist on surfaces, as suspended cells, or as bioaerosols (6). They show variations in numbers and types depending on the kind of indoor environment, sources of contamination, and other environmental factors. These chemical and biological pollutants may adversely impact indoor air quality leading to Indoor Air Pollution (IAP).
IAP is considered one of the five top risks influencing public health and presents a much higher risk than outdoor pollution. An estimated 3.2 million people die every year due to IAP (7, 8). Poor indoor air can have both immediate and long-term health effects, which are commonly referred to as Sick building syndrome (SBS), Building-related illnesses (BRIs), and Multiple chemical sensitivities (1, 9, 10). SBS refers to a collection of symptoms reported by the occupants or workers of a given building and is generally not attributed to a particular cause (10). These may include immediate or short-term effects such as irritation of the eyes, nose, and throat, headaches, dizziness, and fatigue (11, 12). This may also affect the nervous and cardiovascular systems and cause reduced fertility and congenital disabilities in the long term (8). BRI’s on the other hand refers to medical conditions such as hypersensitivities, asthma, and respiratory infections such as pneumonia, linked to a specific cause (13). Generally, exposure to microbes or their components is associated with three main groups of illness: toxicity, infections, and allergic reactions, including respiratory infections and other related diseases (1, 14, 15). Neonates, young children, older adults, and especially people suffering from co-morbid conditions are highly vulnerable to IAP (2, 16).
Particularly in developing nations, including India, the situation is more critical because of overpopulation and other socio-economic factors (17, 18). Tackling indoor air pollution is challenging and requires interdisciplinary efforts (19). There remains a significant knowledge gap and a lack of standards and guidelines, especially for assessing the microbiological quality of indoor air (20). Since the COVID-19 pandemic, IAP has been brought to the forefront because, globally, people are working remotely and spending more time indoors (21).
This review provides a comprehensive overview of microbial contaminants in diverse indoor environments, their sources, sampling and assessment strategies, the factors affecting their prevalence, and the associated health risks (Figure 1). The significance of IAP in the context of the COVID-19 pandemic is also highlighted. The severity of the problem is highlighted for developing countries with a particular reference to the scenario in India. The last section discusses various strategies to mitigate this challenge.
A thorough search of academic databases such as Google scholar and PubMed was conducted. We searched for specific terms such as ‘Microbial Indoor air quality,’ ‘Indoor air and Bioaerosols,’ ‘Bacteria in indoor air, ‘Fungi and molds in Indoor air,’ ‘Microbes in Indoor air and health’. The titles and abstracts of the articles were screened and assessed for the inclusion in the review. Articles were cross checked, duplicated and irrelevant articles were excluded. Further, relevant papers from the reference list of the included papers were also considered to ensure maximum coverage of the literature. A list of 430 papers were extensively discussed among the authors to resolve any discrepancies and reach a consensus. Finally, 314 references were included in this review, out of which 76% are from 2010 onwards.
Outdoor pollutants influence the number and kind of microorganisms that enter the indoor environment displaying a source-sink relationship (22, 23). Apart from these, various inherent sources in the built environment contribute to the abundant microbial numbers and variety. Abiotic factors like moisture, relative humidity, and temperature can influence indoor microbiology (24, 25).
Human occupancy and their activities affect the microbial numbers and diversity in the indoor environment (25, 26). In the indoor air, respirable particulate matter and bacterial DNA increased with human occupancy (27). It has been estimated that humans shed approximately a billion skin cells daily, likely influencing microbial concentrations in the indoor air (28). Abundant bacteria and viruses from human oral and respiratory fluids can become aerosolized via talking, breathing, coughing, sneezing, etc. and reach varying distances depending on the droplet size (29). The frequency of cooking, vacuum cleaning, showering, and other human activities also affect microbial numbers and diversity (30). Patients, doctors, visitors, and hospital staff and their activities contribute to the pathogen load in the hospital environment (5, 31). Fungal concentrations have been reported to be elevated due to increased human movement from outside to inside, specifically linked to increased dust levels (32).
Pets also influence the indoor air microbiome. Animal skin, saliva, hair, fecal matter, and fleas are expected to contribute to microbial diversity in indoor air (28, 33, 34). Bacterial diversity and community richness have been reported to increase in households owning dogs and cats (35, 36). In a study involving 70 pet dogs, about 44 fungal isolates were obtained from the hair and skin of these animals (37).
House dust consists of hair, cotton fibers, bacteria, molds, and other particulate matter (38–40). Dust-borne microbes can become resuspended into indoor air, increasing the risk of inhalation (41). Dust is reported to be dominated by skin-associated Gram-positive bacteria (35, 42, 43). Leppänen et al. (44) quantitatively assessed 259 house dust samples from both rural and urban homes in Finland. This study indicated that the fungal composition and seasonal variations correlated well between indoor air, settled, and reservoir dust samples. The latter showed reproducibility in repeated sampling over time. In another study by Wu et al. (45), bacterial composition and diversity in indoor dust samples were found to be affected by abiotic factors in the indoor environment. Marked differences in bacteria isolated from dust samples collected from university dormitory rooms and printing shops were reported. Human activities or strong air currents can also suspend respiratory viruses deposited in dust or indoor surfaces (46).
Building design and maintenance, moisture build-up, and inadequate ventilation are common triggers for microbial build-up in the indoor environment (47). Plumbing systems can impact indoor air quality by adding bioaerosols to the built environment. Toilet flushing can generate large numbers of aerosolized bacteria from human faces, especially in case of poor ventilation, and if pathogenic, they carry the risk of transmission to healthy occupants (48, 49). In a toilet-seeding experiment by Barker and Jones (50), colony-forming units of Serratia marcescens rose sharply from 0 to 1,370 CFU/m3 after the first flush. Plumbing faults or leaks, water-damped carpets, ceilings, walls, and cramped building design can lead to increased dampness in the indoor environment, further increasing mold growth, odor, and microbial counts (47, 51). Additionally, the events of flooding can substantially increase the dampness inside houses which may favor the growth and dispersal of mold and bacteria. This was observed in the aftermath of flooded houses due to hurricane Katrina in New Orleans, Louisiana, United States (52). Construction and other materials used in buildings significantly contribute as potential sources as they can degrade and convert into organic compounds that support the growth of microorganisms (5). Both natural and mechanical ventilation practices influence the microbial ecology of indoor air (26, 53). Heating, ventilation, and air-conditioning systems (HVAC), primarily installed for air exchange, cleaning, and thermal comfort, can favor microorganisms’ growth if not properly maintained and cleaned (54). These microbes can remain viable on the internal filters of air-conditioning units for a long time. They can re-enter the indoor environment due to filter clogging, inefficient operation, improper maintenance, and malfunction (55). Further, leakage or condensation can wet the filters, favoring mold and bacterial proliferation (56).
Microbial diversity and numbers are significantly contributed by indoor plants in the built environment (57, 58). Indoor plants can increase humidity levels and favor the excessive growth of molds and other airborne microorganisms (59). Due to agitation from the watering of plants or through the generation of strong air currents from fans, levels of airborne fungi were found to increase (60).
There is a lack of international consensus and standard operating procedures for collecting samples from indoor environments for both qualitative and quantitative estimation of microbial contaminants. International standards, including ISO 16000 series such as 16,000-17, 16,000-18, 16,000-19, 16,000-34, offer guidelines concerning sampling techniques, kinds of samplers, and sample analysis (47, 61, 62). Indoor air and dust samples are mainly used for microbial assessment of the indoor environment. Dust samples could be settled dust (on various surfaces like floors and tables) or reservoir dust (in mattresses, carpets, and bedding) (44). Dust collection is a quick but indirect method for sampling bioaerosols in the indoor environment. Various kinds of passive and active sampling techniques are widely and routinely used for the assessment of microbial contamination of indoor air.
Passive sampling using ‘agar settle plates’ is one of the most widely practiced procedures to collect settled dust samples under the force of gravity. The air sample is collected according to the 1/1/1 scheme, plates are incubated, and results are expressed as CFU/m3 using the equation described by Omeliansky (63, 64). It is a simple, inexpensive, and unobtrusive sampling method (65). It gives comparable results, requires no special powered instruments or personnel, and is not influenced by engineering factors. Further, it provides a valid risk assessment if passive sampling is performed in an operation theater or near a surgical site (63, 66).
A simple and cost-effective dustfall collector developed by Wurtz et al. (67) is useful for prolonged airborne dust collection to measure significant concentrations of culturable fungi. Passive samplers based on electrostatic attractions, such as electrostatic dust clothes and the recently invented Rutgers electrostatic sampler, are also used (68, 69). Also, a vacuum cleaner can collect reservoir dust like those from mattresses/carpets or settled dust samples above floor surfaces (44, 70). Swabs are used to collect surface dust from table tops, computers, doors, walls, and cupboards. However, it allows only a qualitative measure of microorganisms’ airborne concentrations, and the sample’s age is unknown (47).
Active sampling collects airborne microorganisms present in inhalable dust in the indoor environment. In this, an air sampler physically draws a pre-set volume of air with the help of a pump, through or over a particle collection device into a liquid or solid culture medium or a nitrocellulose membrane (71). Various types of active air samplers like Anderson, Active Casella slit, Surface air system, and Coriolis cyclone samplers are available commercially based on sampling techniques such as filtration, impaction, impingement, and cyclone, each with its own sets of advantages and limitations (4, 61, 65). Active samplers require trained operators and a power supply, which might constrain their use in remote areas. Frequent replacement of collection media can help avoid reducing sample viability and overloading (65). Massoudinejad et al. (72) stated that active sampling was more sensitive and precise than passive sampling. This sampling technique is mainly applicable when the concentration of microorganisms is not very high, such as in an operating theater of a hospital (73).
These two sampling procedures have shown a good correlation in some studies (68, 74, 75) while others reported no correlation (70, 73, 76, 77). These variations in results could occur due to the type of sample and sampler used, the volume of air sampled, and the place or time of sampling (61, 78). Frankel et al. (79) pointed towards an urgent need for standardized sampling methodologies as they observed significant differences in the levels of culturable fungi, bacteria, endotoxin, and total inflammatory potential, in various air and dust samples collected from homes.
Various culture-based and culture-independent methods employed to enumerate and identify microorganisms in the indoor environment are represented in Figure 2.
Microbial culturing techniques are simple, traditional, low-cost, and well-developed, primarily involving different types of microbiological media. Bacteria are routinely grown on general-purpose media such as nutrient agar, tryptic soy agar, blood agar, and casein soy peptone agar (80, 81). In addition to this, various selective media such as endo agar, eosin methylene blue agar, and mannitol salt agar are also employed (82). This method provides qualitative and quantitative measures of culturable bacteria and population diversity. Several broad-spectrum complex media, such as potato dextrose agar, malt extract agar, rose bengal agar, and dichloran glycerol 18 agar are used for fungal isolation and quantification (80, 81). Compared to bacteria, enumerating fungi is more complex and thus limits the comparison of data from different studies (7).
After processing the sample and using appropriate staining techniques, identification and enumeration of bacterial or fungal contaminants can be carried out by bright field microscopy (6). Alternatively, airborne microbial particles can be directly sampled onto glass slides, semisolid media, or membrane filters and microscopically examined (4). For enumeration and identification of fungi and their spores, hemocytometer chambers and adhesive tapes appressed onto indoor surfaces are also combined with microscopy (83). Both culturing and microscopic techniques are however time-consuming and tedious. Culturing methods show intrinsic constraints in obtaining actual microbial numbers and diversity. Direct microscopic counts are generally exceedingly higher than viable colony counts, commonly phrased as ‘the great plate count anomaly’ (84).
MALDI-TOF MS is a highly reliable and rapid spectrometric technique compared to traditional microbiological and molecular methods and is thus useful for high-throughput microbial identification (85). In this, the mass-to-charge (m/z) ratio of ribosomal protein/peptide (analyte of interest) of the given microorganism is measured by determining the time required for it to travel the length of the flight tube following ionization with a laser beam (86). A unique mass spectrum called peptide mass fingerprint is obtained for the organism quickly. Identification is made using an algorithm-based approach from databases containing the MS reference spectra of peptides and proteins extracted from known microorganisms (87, 88). This technique is gaining popularity for identifying bacteria and fungi from indoor dust and air samples (89–91). The microbial culture is transferred directly onto the sample target from the culture medium. MALDI-TOF MS is simple, cost-effective, and does not require highly skilled personnel (92).
LIF spectroscopy is a relatively novel technique for non-contact real-time online detection of bioaerosols. In this technique, the given bacterial or fungal culture is excited by laser light, showing the largest cross section with the selected wavelength (4, 93). Following de-excitation, the light of a longer wavelength is emitted, which is recorded by a photomultiplier tube resulting in the generation of a spectral fingerprint for each microbial species. Bioaerosols can be differentiated from other airborne particles based on their distinct fluorescence spectra and assessed quantitatively based on fluorescence intensity (94). It efficiently detects microbial contamination of surfaces in industrial and hospital cleanroom facilities, the food-processing industry, operating theaters, and as a biosafety measure in microbiological or medical laboratories or cases of suspicion of a deliberate or accidental release of biological warfare agents (95, 96). However, not all excited species may fluoresce, causing improper measurements (4). This technique has also been recently investigated for the first time for detecting Picorna viruses, thus showing the potential for rapid virological analysis with a substantial cost reduction (97, 98).
LIBS is yet another rapid, flexible, and real-time monitoring technique based on the unique atomic composition of plasma produced when a high-power pulsed laser is focused on a minimal area of the sample surface (99). The sample gets ablated, generating a plasma plume with temperatures over 100,000 K, breaking the sample into excited ionic and atomic species. The plasma then expands and cools within a short time, emitting radiation. Thus, the characteristic emission lines give information about the sample (4). Saari et al. (94, 100) demonstrated the potential of LIBS and LIF techniques as promising tools for the online detection and differentiation of bioaerosol types.
Samples of airborne microbes collected in liquid buffer solutions or on membrane filters from the indoor environment are stained with fluorescent dyes and counted using epifluorescence microscopy (81, 101). DAPI and Acridine orange are popularly used for bioaerosol monitoring (4). It is a high throughput automated technique that allows the counting and differentiation of viable cells of culturable and non-culturable bacteria and fungi from non-viable cells or particles. Compared to conventional culturing, this technique gives much higher estimates of airborne microbial numbers for bacteria and fungi (102, 103), which was confirmed by Chi and Li (104) in a study conducted on bioaerosol concentrations and viability in swine buildings. However, the technique has the limited ability to differentiate between bacteria and fungi in case of size overlap and gives false positive results if the dye binds to the organic material (4, 102).
Flow cytometry is a rapid real-time technique for enumerating, detecting, and sorting microscopic particles suspended in a fluid stream with a high sample throughput (105, 106). It has been widely used for microbial analysis of environmental samples (107, 108). Different cell types cannot be distinguished only based on light scattering characteristics for bioaerosols, so using fluorescent dyes are recommended with this technique (106). After collecting indoor air samples in a liquid, flow cytometry with fluorescence is applied to quantify cells (4). The sample is hydrodynamically focused to create a single cell stream that passes in front of a laser beam, and the fluorescence emitted by each particle is then measured with photon detectors (105). The combination of FCM with fluorescence has been used to assess bacterial counts in hospital wards, reporting significantly higher counts than culture-based methods (109). This method can also detect and quantify airborne fungi (83). The significant drawbacks of FCM are high cost, the need for highly skilled labor, low-temperature requirements, and false positive results due to abiotic particles (4).
Normal or spontaneous Raman spectroscopy and its variations have been used for the spectroscopic analysis of biochemical components of microorganisms. It is an effective, non-invasive, and label-free technique to extract the chemical fingerprint of an individual microbial cell (110). However, the spectra are obscured in standard Raman spectroscopy due to intrinsic fluorescence. Also, a burning effect is caused due to high-energy UV photons in UV resonance Raman enhancement spectroscopy (111). Surface-enhanced Raman spectroscopy (SERS) increases the Raman signal by suppressing the fluorescence and is effective under infrared and visible excitation and has thus emerged as an upcoming rapid, cultivation-independent technique for selective and real-time detection of single microbial aerosols (111, 112). The method involves adsorption or proximity of the analyte to a roughened gold or silver surface or nanoparticles. Following excitation by the incident light, the total enhancement of the SERS process is due to chemical and electromagnetic enhancement effects that generate a sizeable spectroscopic signal for the analyte (113). The major limitation is the reproducibility of the spectral data, as the laser has to strike at a point where the substrate and the analyte are present at an appropriate geometry. This limitation is overcome by combining Raman spectroscopy with other techniques. One study involved the combination of powerful magnification provided by scanning electron microscope in conjunction with the Raman interface to successfully target SERS active regions of the sample matrix to produce reproducible spectra (113).
Similarly in another study, bioaerosols were impacted and transferred to colloidal silver nanoparticles to obtain efficient Raman spectra (111). Schwarzmeier et al. (114) employed a Coriolis μ wet particle sampler with SERS to detect E. coli aerosols, facilitated using an advanced microarray readout system. Distinct Raman spectra of spores from several fungi associated with a damp indoor environment were obtained, suggesting the need to develop a library of Raman spectra for maximal differentiation of fungal spores at the species level (115).
NanoSIMS uses a sufficiently small beam size of approximately 50 nm to analyze single microbial cells or their parts. It combines features of microbial imaging techniques, stable isotope probing, and molecular biomarkers to study environmental microbial communities and draw comparisons (116, 117). Both Raman microspectroscopy and NanoSIMS, though have the potential to dissect and compare microbial communities in environmental samples but have not been used widely for profiling microbes in the indoor environment.
Several culture-independent molecular methods have vastly improved the quality of data obtained for indoor air microbiomes in terms of greater microbial abundance and diversity and thus have changed the way we think about built environments (118, 119). The polymerase chain reaction is the most widely used molecular technique for environmental microbial samples. It is frequently used to amplify genes coding for 16S rRNA for prokaryotes and 18S rRNA and internal transcribed spacer region (ITS) for eukaryotes (28, 120). This technique is rapid and particularly useful when concentrations of airborne microorganisms are low. Indoor airborne bacteria and fungi associated have been analyzed by conventional PCR in many studies (22, 53, 121). In recent years, quantitative PCR (qPCR), also called real-time PCR, has gained popularity in providing accurate data on total microbial numbers in environmental samples (122). It has been used to assess bacterial (123) and fungal concentrations and types (70) in indoor air and dust samples. The limitations of PCR include a prior requirement of sequence knowledge to design the primers and non-specific binding of the primer to similar sequences on template DNA (124).
Amplicons obtained from PCR can be further analyzed using various genetic fingerprinting techniques like amplified ribosomal DNA restriction analysis, terminal restriction fragment length polymorphism (T-RFLP), automated ribosomal intergenic spacer analysis, denaturing gradient gel electrophoresis, temperature gradient gel electrophoresis, single-strand conformation polymorphism, and denaturing high-performance liquid chromatography (125). These techniques are widely used for studying microbial communities in environmental samples and have also been applied for indoor air microbial profiling (116). For instance, in a study by Weikl et al. (126) involving T-RFLP, the variation, and diversity of bacterial and fungal microbiomes were studied in dust samples from 286 households. The study indicated that both bacterial and fungal communities followed a temporal and seasonal pattern, with indoor and outdoor determinants affecting the fungal microbiome more strongly.
Our knowledge of indoor air microbiome diversity and community dynamics has been substantially enhanced by metagenomic-based sequencing, having the advantage of rapidly analyzing millions of samples in parallel with high sensitivity (127). The classic first-generation Sanger sequencing is often replaced by advanced sequencing approaches using platforms such as the second-generation Illumina MiSeq/HiSeq and Ion Torrent systems, third generation PacBio SMRT sequencing, and recently commercialized fourth-generation Oxford Nanopore MinION technology (128, 129).
These advanced identification methods are being increasingly applied to analyze microbial diversity in indoor air and dust samples (130, 131). In a study, indoor air samples from elementary schools and day-care centers in Korea showed the presence of a wide variety of taxa in microbial communities previously not identified by culture-based methods (132). Nygaard et al. (128) characterized microbiomes in the building-dust by comparing Nanopore MinION and Illumina MiSeq 16S rRNA gene sequencing. This study did not find significant differences in microbial composition between the two methods; however, a greater taxonomic resolution was achieved with MinION technology. Diverse indoor airborne viral communities associated with disparate hosts were also observed using metagenomic sequencing of dust samples obtained from the HVAC filters by Rosario et al. (133).
However, metagenomic-based sequencing studies of the indoor environment are limited by the availability of reference sequences in genome databases, low biomass of microbial aerosols leading to poor DNA extraction, and missing out the DNA of microbes present in low-abundance. Also, a significant deterrent to adopting these advanced methods for routine microbial surveillance is offset by the high cost of resources and machinery, especially in low- and middle-income countries (134, 135).
The indoor microflora is complex and dynamic, with their numbers and diversity guided by their sources and associated environmental factors. There are no uniform standards for the acceptable levels of microbial contaminants in the indoor environment. A study by the WHO expert group to assess the health risks of biological agents in indoor environments suggested that the total microbial concentration should not exceed 1,000 CFU/m3 (47). However, some recommend 300 CFU/m3 and 750 CFU/m3, the maximum limit for fungi and bacteria (136). Also, the standards followed may vary from one country to another and from one kind of indoor environment to another. Table 1 provides an insight into the vast diversity of microflora in various indoor environments such as homes, offices, schools, universities, libraries, healthcare facilities, shopping centers, public restrooms, and gyms. In comparison to bacteria and fungi, there are limited studies on viruses.
The most common bacterial genera observed in dust and air samples collected from various types of indoor environments are Acinetobacter, Actinobacteria, Arthrobacter, Alcaligenes, Bacillus, Corynebacterium, Kocuria, Micrococcus, Propionibacterium, Staphylococcus, and Streptococcus species (43, 45, 140, 147). Barberan et al. (35) indicated that there could be differences in bacterial types isolated depending on the gender occupancy of a particular environment. Relatively, a greater abundance of Corynebacterium, Dermabacter, and Roseburia spp. was found in homes dominated by males, whereas those occupied mainly by females had more Lactobacillus spp. Further, seasonal variations are observed in bacterial populations in the indoor environment. In a study by Rintala et al. (157), the relative abundance of alpha- and beta-proteobacteria increased slightly toward summer in house dust samples. The proportion of firmicutes and gamma-proteobacteria was highest in winter and that of actinobacteria, alpha- and beta-proteobacteria in spring or summer, whereas the diversity of Bacteroides peaked in fall. Barberan et al. (35) reported that species of Bacteroides, Porphyromonas, Arthrobacter, Moraxella, Blautia, and Neisseria were associated with dogs, while species of Prevotella, Porphyromonas, Jeotgalicoccus, Sporosarcina, Moraxella, and Bifidobacterium were associated with cats, in household dust samples.
The indoor air of hospitals and healthcare settings can also be a source of harmful bacteria like Mycobacterium tuberculosis, Staphylococcus aureus, Escherichia coli, Legionella pneumophila, and Pseudomonas aeruginosa. Consequently, patients, healthcare workers, visitors, and mainly immunocompromised patients are at a higher risk of acquiring nosocomial infections (138, 144, 158). In particular, Legionella pneumophila, is a highly concerning indoor pathogen, that can easily get aerosolised from toilet or sink faucets, shower heads, bathtubs, plumbing systems, and is abundantly found in various healthcare facilities (159, 160). It has also been found to contaminate the dust in air conditioning and HVAC systems. Both water and air sampling are performed to evaluate the pathogen exposure and to suggest measures for its control. In a study using real time TaqMan PCR, Legionella pneumophila was found in 66.7% of the dust samples collected from the air ducts of central air conditioning system (161). Montagna et al. (138) conducted a multicentre study in Italy, to detect airborne contamination of Legionella from the water sources. They found that the liquid impingement technique (using Coriolis®μ) was effective in the collection of airborne pathogens combined with molecular investigations in comparison to passive and active dust sampling. Transmission of airborne antibiotic-resistant bacteria like methicillin-resistant Staphylococcus aureus, beta-lactam-resistant Acinetobacter sp., vancomycin-resistant streptococci, and their inhalable antibiotic-resistance genes in the hospital environment are also a significant threat to public health (162). In a study assessing bacterial contamination of surfaces, medical equipment, and indoor air of the pediatric ward and neonatal intensive care unit of an Ethiopian hospital, a quarter of the bacteria isolated were found to be multi-drug resistant, and most were associated with nosocomial infections (163).
Exposure to fungi, their spores, hyphal fragments, and compounds like mycotoxins and beta-glucans in the indoor environment, is considered a major health risk mainly associated with hypersensitivity and allergic risks to the occupants (164). Outdoor air fungi mostly dominate the patterning of indoor air and can easily get suspended in the dust or settle on surfaces of any built environment (22, 32). The indoor temperature range of 10-35°C is highly conducive to the growth of fungi. Fungi can obtain nutrients from plant or animal matter in house dust, building, construction, and painting materials, cooking oil deposits, clothes, books, and paper (47). Dampness is a significant contributor to fungal growth and their spores, fragments, and allergens can easily get aerosolized in the indoor environment (47, 165). Both natural and mechanical ventilations are also considered as likely sources of fungal contamination. High humidity due to condensation of water and settled dust inside air-conditioning ducts, filters, and collecting trays are considered potential sites for high fungal contamination (165, 166). The prevalence of fungi in the indoor air of healthcare facilities poses a high risk of invasive fungal infections. This has been extensively studied and reviewed by Belizario et al. (167). The fungal genera predominating various indoor environments are Aspergillus, Penicillium, Fusarium, Alternaria, Cladosporium, Stachybotrys, Trichoderma, and yeasts like Candida spp. (137, 154, 168). They have frequently been related to hospital-acquired infections and exacerbation of respiratory symptoms (20, 167). The microbial content of rug dust and vacuum cleaner bag, dust samples determined by qPCR showed Penicillium, Aspergillus, and Paecilomyces to be present in the highest concentrations (169).
Though bacteria and fungi are monitored frequently in various indoor environments, not many efforts have been made to investigate the viral diversity of the indoor air (101, 133). Respiratory infections, like the common cold, bronchiolitis, and influenza, can transmit through aerosolized droplets (170, 171). Respiratory syncytial, influenza, parainfluenza, corona, and adeno viruses are implicated in these infections (172). Low relative humidity and cold temperatures are believed to increase the transmission of respiratory viruses (173, 174). Human activities like breathing, coughing, sneezing, talking, and laughing release viral aerosols abundantly in the indoor air (175). Moon et al. (176) showed that the indoor air of residential apartments in South Korea mainly contained adenoviruses and influenza A virus, with concentrations of adenoviruses higher in winter than in spring. In another study by Yang et al. (177), one-third of the samples from the health center and two-thirds from the day-care center were confirmed to contain aerosolized influenza A virus.
The diversity of viruses in the indoor environment could be beyond human viruses. Rosario et al. (133) used a metagenomic approach to investigate the diversity of DNA and RNA viruses in the dust samples accumulated in HVAC filters of university dormitory rooms. They detected a broad diversity of viruses, including human papillomaviruses (HPVs) and polyomaviruses (HPyVs), which were found to be associated with a range of hosts. Rare zoonotic diseases caused by deadly viruses like Marburg, Ebola, Hanta, and Lassa can pose a potential nosocomial threat (178). During the SARS outbreak of 2003, infectious viral droplets entered buildings via sewage and drainage systems due to inadequacies in plumbing (179). The profuse transmission of SARS-CoV-2 and cluster cases were primarily due to the confined, crowded, and poorly ventilated indoor environment (180).
Exposure to microbes in the indoor environment can lead to adverse health effects like respiratory illnesses, including allergies, microbial infections, acute toxic effects, and cancer, as described below. The major routes of human exposure to airborne microorganisms and their products are mainly inhalation followed by ingestion and dermal contact (15). Various health effects following microbial exposure depend on the dose, route, and timing of exposure and the person’s genetic disposition (181). Molds and bacteria can also produce components like endotoxin, beta-1-3-glucan, muramic acid, ergosterol, allergenic proteins, volatile organic compounds of microbial origin (MVOCs), and mycotoxins (32, 182, 183) which can also pose additional health hazards.
Exposure of the respiratory tract to microbes or their products can lead to mucous membrane irritation, allergic rhinitis, asthma, bronchitis, organic dust toxic syndrome (ODTS), chronic obstructive pulmonary disease (COPD), or allergic alveolitis (184, 185). Moldy and dusty environments are highly uncomfortable to susceptible individuals, with fungi such as Aspergillus, Penicillium, Cladosporium, Acremonium, Paecilomyces, and Mucor found to be commonly associated with respiratory infections and allergies (168). Asthma is common in children, with allergens and molds influencing the development of the disease (137). Fungal DNA has also been considered a risk factor for childhood asthma at home (181). However, according to hygiene hypothesis, exposure to microbes in early childhood could protect against allergies and asthma by stimulating the immune system, and that excessive cleanliness in the human environment might not necessarily be beneficial (186). Among fungi, Aspergillus species are the most commonly found indoor fungi. Aspergillus fumigatus and Aspergillus flavus are frequently encountered and responsible for sinusitis, allergic broncho-pulmonary aspergillosis (ABPA), and hospital-acquired infections (187, 188). High fungal or dust exposure has been linked to hypersensitive pneumonitis (189). Fungal metabolism produces volatile compounds such as 3-methyl furan that can irritate eyes, nose, and airways leading to headache, nausea, dizziness, and fatigue (40, 190). Inhalation of high doses of endotoxins, glucans, fungal spores, and mycotoxins can contribute to airway irritation and inflammation, decrease lung function, exacerbate asthma, and chronic conditions like ODTS and COPD (164, 191).
Airborne transmission is the most likely route for many microbial infections in the indoor environment. Legionella bacteria causing Pontiac fever and highly fatal pneumonia can rapidly spread in indoor environments through aerosolized contaminated water and inhalation of infected mist or vapor (138). Mycobacterium tuberculosis can be transmitted by inhaling the infectious droplet nuclei expectorated from sputum-positive patients in the indoor air of hospitals or other environments (192). Staphylococcus aureus, Pseudomonas aeruginosa, Enterococcus faecalis, Enterococcus faecium, Acinetobacter baumanii, and Escherichia coli are some of the most frequently encountered bacteria in the indoor air of hospitals, causing various nosocomial infections (193).
Immunocompromised patients are at potential risk for more severe opportunistic and systemic fungal infections caused by Blastomyces, Coccidioides, Cryptococcus, Histoplasma, Alternaria, Heliminthosporium, Cladosporium, Fusarium, Aspergillus, Phoma, and Penicillium (194, 195). Similarly, the presence of these fungi in the indoor air of critical hospital areas, especially neonatal and pediatric ICU, has been linked to the increased incidence of mucocutaneous colonization and a high risk of invasive fungal infections in neonates (167).
Human corona, respiratory syncytial, rhino, adeno, measles, mumps, rubella, and enteric viruses are readily transmitted through air droplets in the indoor environment causing various infections (196–198). The COVID-19 pandemic has recently shown the extreme contagiousness of the Severe Acute Respiratory Syndrome Coronavirus 2 (SARS-CoV-2) virus in the indoor environment, which has been responsible for high morbidity and mortality (199). A special section is dedicated here to the potential implications of poor indoor air quality in transmitting SARS-CoV2.
Several studies have tried to ascertain the link between cancer and bioaerosol exposure (200–202). Mycotoxins have been established as non-viral biological carcinogens (203, 204). Among them, aflatoxin from Aspergillus flavus is the best-known carcinogenic mycotoxin, particularly linked to liver cancer (205, 206). Ochratoxin A is also a possible human carcinogen (183, 207). The lung cancer risk of workers in the meat/poultry industry was reviewed with evidence pointing to oncogenic viruses associated with animals and heavy exposure to airborne material, including micro-organisms, fecal material, dander, and feathers (201).
The global pandemic of COVID-19 caused by the SARS-CoV-2 has left an unprecedented impact on various facets of human life, including health, lifestyle, economy, and the environment (208, 209). Terms like disinfection, masks, social distancing, and quarantine have become a part of our daily life. On the one hand, a few studies indicated improvement in ambient air quality in many cities during the lockdown, attributed to reduced industrial activities, vehicular movements, and various human activities (210–213). On the other hand, the reduced outdoor pollution did not contribute to any health gains, as prolonged exposure to polluted indoor air negated this effect (214, 215). Poor indoor air quality increased COVID-19 infection and viral transmission in enclosed spaces (216). It took several months before the WHO acknowledged the airborne transmission of SARS CoV2, especially when people spent prolonged periods in indoor, crowded, inadequately ventilated spaces (217). The aerosolized virus is stable for 3 h and can travel long distances in closed and open environments (214). These aerosols need to be controlled to reduce the risk of new infections. Also, in a study conducted in Bergamo, Northern Italy, viral RNA was isolated from the particulate matter, possibly explaining a higher COVID-19 burden in areas with high air pollution (218).
People were compelled to stay inside owing to home quarantine and lockdowns. This may have impacted indoor air quality due to insufficient ventilation, overcrowding, recirculation of polluted air, and increased household activities and office work (214, 215). Further, aerosolized virus released from infected people quarantined at homes, or hospitals could have increased the risk and deteriorated the indoor air (219). A study showed that the cough from an individual with a high SARS-CoV-2 load could contain 7.44 million viral copies/m3 (220). If the quality of the air is poor to begin with, people may be inadvertently exposed to various SBS-related symptoms (221) putting people with pre-existing medical conditions at a higher risk. Due to the high transmission of the SARS-CoV-2 virus in indoor spaces, IAP has thus become a table talk worldwide (180). The COVID-19 pandemic has thus strongly taught us to focus equally on sustainable and safe indoor spaces, as poor indoor air, can likely intensify the impact of air-borne viral outbreaks and other microbial infections (222, 223).
In developing countries, including India, people are exposed to the highest air pollution in the indoor environment (224, 225). There is a general lack of awareness among people in India as air pollution is only thought to be associated with the outdoor environment, and the inhabitants are considered safe indoors (18). Here, rural and urban poor populations still use simple solid fuels that release abundant amounts of suspended particulate matter, harmful chemicals and gasses and have been associated with severe respiratory and other health risks (226). Overcrowded and confined indoor spaces, improper ventilation, inadequacies in building design, and various socio-economic factors also aggravate poor indoor air quality (213, 227). Microbial contaminants also significantly contribute to IAP; however, in India, this aspect has not been dealt with extensively (228) and can pose various health hazards to the occupants of the indoor environment, as discussed earlier.
Further, there are no standard guidelines to monitor and assess various parameters related to IAP, including microbiological assessment (18). The Central Pollution Control Board (229) under the Ministry of Environment and Forests (MoEF), Government of India, does not include IAP in its agenda and mainly focuses on outdoor air pollution. Thus, this knowledge and skill gap is highly concerning (18). Damp, overcrowded houses, and poorly ventilated buildings are a hot spot for microbial contaminants and a source of respiratory illnesses like tuberculosis, contributing significantly to the national disease burden (230). India hosts diverse climatic conditions, and thus the indoor microflora diversity and concentration show a lot of seasonal variations (5, 231, 232). Some representative studies on the isolation and assessment of microflora in various indoor environments conducted in India’s urban and rural settings are highlighted in Table 2.
However, it is clear from these studies that primarily passive sampling procedures combined with conventional culturing methods have been used. Thus, a large proportion of non-culturable microbes still remain unassessed. There is a need to introduce advanced molecular and spectrometric techniques to routinely monitor microbiological indoor air quality and bridge this knowledge gap. Further, mainly bacterial and fungal contaminants have been studied, with only a few studies assessing viruses and microbial products such as endotoxins, mycotoxins, and microbial allergens in the indoor environment (252, 254).
Various mitigation strategies aim to control microorganisms and improve indoor air quality by optimizing ventilation systems, controlling emission sources, and developing air purification technologies, as discussed here (255).
Ventilation can help remove or dilute pollutants and control humidity or dampness in the built environment, thereby maintaining the health and comfort of the occupants (256, 257). International agencies such as ASHRAE (American Society of Heating, Refrigerating, and Air-Conditioning Engineers), WHO, REHVA (Representatives of European Heating and Ventilation Associations), and CDC (Center for Disease Control and Prevention) have also recognized that the transmission of airborne diseases can be effectively managed by ventilation (257–259). For instance, CDC (258) has provided detailed guidelines including cost considerations for improving ventilation in buildings. Ventilation can be provided by natural or mechanical means (260, 261). Natural or passive ventilation is achieved through airflow provided by doors, windows, louvers, and vents (7, 259). Various studies have reported that natural ventilation is effective in controlling the transmission of the infectious airborne microbes (260, 262). In low resource healthcare settings, Escombe et al. (263) demonstrated that it could be used as a low-cost and energy efficient measure to reduce the risk of transmission of nosocomial tuberculosis. Further, the rampant spread of corona virus brought natural ventilation strategy to the forefront of the discussion as a crucial factor that can help in lowering the viral build-up in the indoor spaces. In this context, Vignolo et al. (264) qualitatively assessed airborne viral transmission and natural ventilation in school classroom in Uruguay and reported that periodic ventilation can act as a useful strategy to reduce transmission of airborne microbes. However, in another study, no impact was reported (265). Despite the low cost, energy efficiency, low maintenance, and higher airflow changes, natural ventilation is difficult to maintain in a tight building without cross ventilation. Further, it is not a feasible strategy for healthcare facilities and countries with colder climates as natural ventilation can increase the thermal discomfort for the occupants (266). Thus, a cross-sectional study involving all the factors can potentially give an insight for optimal use of natural ventilation.
Mechanical ventilation uses an air handling system like HVAC that circulates fresh and recycled air via ducts (261). Healthcare facilities with efficiently maintained and operated mechanical air conditioning, and ventilation systems were found to be less contaminated than buildings with naturally ventilated systems (267). In a study by Gołofit-Szymczak and Górny (268), different ventilation systems were assessed for their impact on microbiological indoor air quality of 15 office buildings. Higher concentrations of both bacteria and fungi were observed in naturally ventilated office in comparisons with offices having air-conditioning and mechanical ventilation systems. Similarly, HVAC system was found to effectively remove fungal spores (269). However, it is essential to note that mechanical ventilation systems may be a source of biological contamination, as discussed earlier. Further, the high transmission of SARS-CoV-2 in indoor spaces has made us all think critically about indoor air quality, especially in hospitals, and the approaches to controlling nosocomial infections (270). Fonseca et al. (271) suggested that the mechanical ventilation systems in healthcare facilities could be complemented with natural ventilation to adequately control relative humidity and CO2 concentrations to minimize the risk of airborne infections. Both mechanical and natural ventilation systems combined in a hybrid technology can also provide sustainability in terms of energy efficiency (272), and the shortcomings of natural ventilation can be overcome by the mechanical components (273). The frequency of regular monitoring, cleaning, and maintenance procedures can be adjusted based on operating hours and human occupancy to achieve good air quality (20).
Studies show that HVAC filtration can effectively control pathogens penetrating the building envelope and their transmission in the indoor environment (274, 275). Thus, to improve the performance of HVAC systems, panel filters are installed in their ducts (274, 276, 277). These filters are installed as per the standard guidelines and used widely (278, 279). HEPA or High-Efficiency Particulate Air filters must be cleaned and replaced periodically to maintain proper function (280). By following the air route and sources of contamination through sequential sampling, Cabo Verde et al. (20) assessed the efficacy of HEPA filters installed in the ventilation systems of operation theaters and found an effective reduction in bacterial concentration from outdoors to indoors. These filters have the limited ability to inactivate the collected biological agents, which may regrow with adequate humidity levels (281). Nanofiber filters can overcome this problem and are very effective in indoor environments where stringent air purification is needed, such as hospitals, food, and pharmaceutical industries (282).
The inactivation of microbial contaminants in indoor air could also be brought about by utilizing other methods integrated with the HVAC system (275, 280, 283). These include ultraviolet germicidal irradiation (UVGI) (284, 285); chlorine dioxide gas (286); antimicrobial compounds (287); electrostatic technology (288); photocatalytic oxidation (289); plasma cluster ion technology (290); microwave heating (291); ozone sterilization (292, 293); and dispersion of atomized nanoparticles in the air (294). UVGI system uses UVC rays (200–280 nm) to form photo dimers in nucleic acids to bring about disinfection and has been widely recognized and recommended for installation, especially in healthcare facilities (295, 296). However, it is pertinent to mention that ozone-based technologies can themselves pose an additional health risk by releasing ozone as a harmful by-product (297). Recently, silver nanoparticles and multiwall carbon nanotubes coated with hybrid polypropylene nano-filter were tested in air-conditioning and found to be very effective in killing Pseudomonas aeruginosa, Salmonella enterica, and Staphylococcus aureus (298). Thus, two or more such technologies can be synergized to improve the efficient functioning of the HVAC systems to remove microbial contaminants (283, 299).
Air Purifiers/Cleaners (AP) have become essential for reducing IAP. There has been a considerable increase in sales of air purifiers from 0.8 million units in 2015 to almost 2 million units in 2018, with a similar projection for the future (300, 301). Portable air purifiers utilizing HEPA filtration are commonly used for homes. Air purifiers’ advantages are simple installation, portability, and the lack of harmful by-products (302). Their configuration generally employs a multilayer filter system consisting of a prefilter, a carbon filter, an antibacterial filter, and a HEPA filter (255, 303). Also, APs with disinfection capability are more effective than those with only HEPA filtration (216). In these, UVGI lights are installed within the body of the air cleaners. Combined HEPA and UVGI, air cleaning technologies could effectively remove airborne pathogens (304, 305). Air purifiers or cleaners based on ionization, electrostatic precipitation, cold plasma generation, and photocatalytic oxidation are emerging technologies (216, 255, 306–309). However, currently, no single technology can sufficiently achieve “cleaner” indoor air. Current research is focused on combined and innovative alternatives, such as plasma-catalytic hybrid systems, hybrid ozonation systems, and biofilter-adsorption systems, that can work synergistically to achieve optimum indoor air quality (310–312).
The frequency of housekeeping can also help in lowering the microbial content of the indoor air. This includes basic measures like dusting with a damp cloth or using electrostatic cloth, vacuuming, carpet and rug cleaning, and removal of shoes while entering the house. Persistent dampness and moldy growth on indoor surfaces and in building structures can be minimized. For instance, USEPA has provided guidelines for the control of moisture and mold in homes (313). Franke et al. (314) carried long-term monitoring of a building for biological, chemical, and particulate matter, following a standardized cleaning program. This improved house-keeping initiative was found to be effective in the reduction of culturable bacteria and fungi, airborne dust mass and total volatile organic compounds. However, barring a few reports, there are no direct studies that have ascertained the link between house-keeping and indoor microbial concentrations. This is definitely a thrust area that will also aim to increase awareness about the impact of poor indoor air quality on an individual’s health especially children and older people and those with underlying health conditions.
Rapid industrialization and urbanization have led people to spend most of their time indoors in tight-built environments. Thus, indoor air quality plays a significant role in their general state of health and comfort. They are exposed to a variety of pollutants, including microorganisms. A vast ecological niche has been created for these minuscule organisms leading to their presence in various microhabitats of the indoor environment, thus presenting us with a complex ecosystem that requires a more profound understanding. Compared to bacteria and fungi, there are limited studies on the viral diversity of the indoor environment. Protocols for sampling and assessing microorganisms need to be standardized and validated as the threshold limits can vary from one indoor environment to another, region to region, and country to country. Culture-independent methods can complement culture-based methods in comprehensively monitoring microbial composition and community structure. Advancements in metagenomic next-generation sequencing approaches have paved the way for better analysis of viral diversity which has been relatively unexplored in comparison to bacteria and fungi. To minimize the knowledge gap, a repository of microbial sequences obtained from different kinds of built environments must be maintained as a referential database source.
Developed countries mostly follow specific guidelines and standards for building design and management of indoor air quality. However, the situation is grave in developing countries with a high disease burden aggravated by various socio-economic factors. A concerted effort is needed to raise awareness about the issue, as simple source control measures can make a massive difference in the quality of the built environment. For example, basic housekeeping measures to prevent dust build-up or humidity control can curtail microbial growth, for which people need to be educated through awareness programs and surveys. Cross-sectional and evidence-based studies will provide a realistic analysis of the microbial impact and help design feasible mitigation strategies. We need to focus on hybrid air cleaning technologies and sustainable building architecture that combines energy saving with appropriate ventilation to minimize the buildup of indoor air pollutants. A multifactorial interdisciplinary approach will involve a synergy between microbiologists, public health experts, engineers, architects, environmental agencies, and the government to devise sustainable solutions to this serious public health issue.
HC: Writing – original draft, Data curation, Investigation. PA: Data curation, Writing – original draft, Writing – review & editing. KG: Investigation, Writing – original draft. NB: Data curation, Investigation, Writing – review & editing. SV: Investigation, Writing – review & editing. TB: Writing – original draft. AM: Writing – review & editing. RM: Conceptualization, Supervision, Writing – original draft.
The author(s) declare that no financial support was received for the research, authorship, and/or publication of this article.
The authors acknowledge the use of Biorender.com for creating the figures used in the manuscript.
The authors declare that the research was conducted in the absence of any commercial or financial relationships that could be construed as a potential conflict of interest.
All claims expressed in this article are solely those of the authors and do not necessarily represent those of their affiliated organizations, or those of the publisher, the editors and the reviewers. Any product that may be evaluated in this article, or claim that may be made by its manufacturer, is not guaranteed or endorsed by the publisher.
1. Van, TV, Park, D, and Lee, YC. Indoor air pollution, related human diseases, and recent trends in the control and improvement of indoor air quality. Int J Environ Res Public Health. (2020) 17:2927. doi: 10.3390/ijerph17082927
2. Sundell, J. On the history of indoor air quality and health. Indoor Air. (2004) 14:51–8. doi: 10.1111/j.1600-0668.2004.00273.x
3. Smith, KR. National burden of disease in India from indoor air pollution. Proc Natl Acad Sci. (2000) 97:13286–93. doi: 10.1073/pnas.97.24.13286
4. Ghosh, B, Lal, H, and Srivastava, A. Review of bioaerosols in indoor environment with special reference to sampling, analysis and control mechanisms. Environ Int. (2015) 85:254–72. doi: 10.1016/j.envint.2015.09.018
5. Kumar, P, MohdA, K, Singh, AB, and Singh, R. Biological contaminants in the indoor air environment and their impacts on human health. Air Qual Atmos. Health. (2021) 14:1723–36. doi: 10.1007/s11869-021-00978-z
6. Stetzenbach, LD, Buttner, MP, and Cruz, P. Detection and enumeration of airborne biocontaminants. Curr Opin Biotechnol. (2004) 15:170–4. doi: 10.1016/j.copbio.2004.04.009
7. Flannigan, B, Samson, RA, and David, MJ. Microorganisms in home and indoor work environments. Boca Raton, FL: CRC Press (2002).
9. Spachos, P, and Plataniotis, K. Environmental monitoring for smart buildings In: P Spachos, editor. Transportation and power grid in smart cities. Chichester, UK: John Wiley and Sons, Ltd. (2018). 327–54.
10. Nag, PK. Sick building syndrome and other building-related illnesses. Berlin: Springer, pp. 53–103. (2019).
11. USEPA. Introduction to indoor air quality. United States Environmental Protection Agency, United States Government (2021) Available at: https://www.epa.gov/indoor-air-quality-iaq/introduction-indoor-air-quality.
12. Berglund, B, Brunekreef, B, Knoppe, H, Lindvall, T, Maroni, M, Molhave, L, et al. Effects of indoor air pollution on human health. Indoor Air. (1992) 2:2–25. doi: 10.1111/j.1600-0668.1992.02-21.x
13. Horvath, EP. Building-related illness and sick building syndrome: from the specific to the vague. Cleve Clin J Med. (1997) 64:303–9. doi: 10.3949/ccjm.64.6.303
14. Bernstein, JA, Alexis, N, Bacchus, H, Bernstein, IL, Fritz, P, Horner, E, et al. The health effects of nonindustrial indoor air pollution. J Allergy Clin Immunol. (2008) 121:585–91. doi: 10.1016/j.jaci.2007.10.045
15. Srikanth, P, Sudharsanam, S, and Steinberg, R. Bio-aerosols in indoor environment: composition, health effects and analysis. Indian J Med Microbiol. (2008) 26:302–12. doi: 10.1016/S0255-0857(21)01805-3
16. Holgate, S, Grigg, J, Arshad, H, Carslaw, N, Cullinan, P, Dimitroulopoulou, S, et al. Health effects of indoor air quality on children and young people In: S Holgate, editor. Issues in environmental science and technology. London, UK: Royal Society of Chemistry (2020). 151–88.
17. Bruce, N, Perez-Padilla, R, and Albalak, R. Indoor air pollution in developing countries: a major environmental and public health challenge. Bull World Health Organ. (2000) 78:1078–92.
18. Goyal, R, and Khare, M. Indoor air quality: current status, missing links and future road map for India. J Civil Environ Eng. (2012) 2:118. doi: 10.4172/2165-784X.1000118
19. Baecher, C, Sohui, F, Ball, L, and Masson, O. Indoor air quality: a multifaceted public health problem. Field Actions Sci Rep. (2020) 2020:6–7.
20. Cabo Verde, S, Almeida, SM, Matos, J, Guerreiro, D, Meneses, M, Faria, T, et al. Microbiological assessment of indoor air quality at different hospital sites. Res Microbiol. (2015) 166:557–63. doi: 10.1016/j.resmic.2015.03.004
21. Mumtaz, R, Zaidi, SMH, Shakir, MZ, Shafi, U, Malik, MM, Haque, A, et al. Internet of things (IoT) based indoor air quality sensing and predictive analytic–a COVID-19 perspective. Electronics (Basel). (2021) 10:184. doi: 10.3390/electronics10020184
22. Adams, RI, Miletto, M, Taylor, JW, and Bruns, TD. Dispersal in microbes: fungi in indoor air are dominated by outdoor air and show dispersal limitation at short distances. ISME J. (2013) 7:1262–73. doi: 10.1038/ismej.2013.28
23. Miletto, M, and Lindow, SE. Relative and contextual contribution of different sources to the composition and abundance of indoor air bacteria in residences. Microbiome. (2015) 3:61. doi: 10.1186/s40168-015-0128-z
24. Prussin, AJ, and Marr, LC. Sources of airborne microorganisms in the built environment. Microbiome. (2015) 3:78. doi: 10.1186/s40168-015-0144-z
25. Adams, RI, Bhangar, S, Pasut, W, Arens, EA, Taylor, JW, Lindow, SE, et al. Chamber bioaerosol study: outdoor air and human occupants as sources of indoor airborne microbes. PLoS One. (2015) 10:e0128022. doi: 10.1371/journal.pone.0128022
26. Meadow, JF, Altrichter, AE, Kembel, SW, Kline, J, Mhuireach, G, Moriyama, M, et al. Indoor airborne bacterial communities are influenced by ventilation, occupancy, and outdoor air source. Indoor Air. (2014) 24:41–8. doi: 10.1111/ina.12047
27. Hospodsky, D, Qian, J, Nazaroff, WW, Yamamoto, N, Bibby, K, Rismani-Yazdi, H, et al. Human occupancy as a source of indoor airborne Bacteria. PLoS One. (2012) 7:e34867. doi: 10.1371/journal.pone.0034867
28. Kelley, ST, and Gilbert, JA. Studying the microbiology of the indoor environment. Genome Biol. (2013) 14:202. doi: 10.1186/gb-2013-14-2-202
29. Xie, X, Li, Y, Sun, H, and Liu, L. Exhaled droplets due to talking and coughing. J R Soc Interface. (2009) 6:S703–14. doi: 10.1098/rsif.2009.0388.focus
30. Chen, Q, and Hildemann, LM. The effects of human activities on exposure to particulate matter and bioaerosols in residential homes. Environ Sci Technol. (2009) 43:4641–6. doi: 10.1021/es802296j
31. Nevalainen, A, and Seuri, M. Of microbes and men. Indoor Air. (2005) 15:58–64. doi: 10.1111/j.1600-0668.2005.00344.x
32. Nevalainen, A, Täubel, M, and Hyvärinen, A. Indoor fungi: companions and contaminants. Indoor Air. (2015) 25:125–56. doi: 10.1111/ina.12182
33. Fujimura, KE, Johnson, CC, Ownby, DR, Cox, MJ, Brodie, EL, Havstad, SL, et al. Man’s best friend? The effect of pet ownership on house dust microbial communities. J Allergy Clin Immunol. (2010) 126:410–412.e3. doi: 10.1016/j.jaci.2010.05.042
34. Kettleson, EM, Adhikari, A, Vesper, S, Coombs, K, Indugula, R, and Reponen, T. Key determinants of the fungal and bacterial microbiomes in homes. Environ Res. (2015) 138:130–5. doi: 10.1016/j.envres.2015.02.003
35. Barberán, A, Dunn, RR, Reich, BJ, Pacifici, K, Laber, EB, Menninger, HL, et al. The ecology of microscopic life in household dust. Proc R Soc B Biol Sci. (2015) 282:20151139. doi: 10.1098/rspb.2015.1139
36. Mäki, JM, Kirjavainen, PV, Täubel, M, Piippo-Savolainen, E, Backman, K, Hyvärinen, A, et al. Associations between dog keeping and indoor dust microbiota. Sci Rep. (2021) 11:5341. doi: 10.1038/s41598-021-84790-w
37. Jang, K-S, Yun, Y-H, Yoo, H-D, and Kim, SH. Molds isolated from pet dogs. Mycobiology. (2007) 35:100. doi: 10.4489/MYCO.2007.35.2.100
38. Harrison, RM, Jones, AM, Biggins, PDE, Pomeroy, N, Cox, CS, Kidd, SP, et al. Climate factors influencing bacterial count in background air samples. Int J Biometeorol. (2005) 49:167–78. doi: 10.1007/s00484-004-0225-3
39. Maron, P-A, Lejon, DPH, Carvalho, E, Bizet, K, Lemanceau, P, Ranjard, L, et al. Assessing genetic structure and diversity of airborne bacterial communities by DNA fingerprinting and 16S rDNA clone library. Atmos Environ. (2005) 39:3687–95. doi: 10.1016/j.atmosenv.2005.03.002
40. Korpi, A, Pasanen, A-L, Pasanen, P, and Kalliokoski, P. Microbial growth and metabolism in house dust. Int Biodeterior Biodegradation. (1997) 40:19–27. doi: 10.1016/S0964-8305(97)00032-2
41. Ferro, AR, Kopperud, RJ, and Hildemann, LM. Source strengths for indoor human activities that Resuspend particulate matter. Environ Sci Technol. (2004) 38:1759–64. doi: 10.1021/es0263893
42. Pakarinen, J, Hyvärinen, A, Salkinoja-Salonen, M, Laitinen, S, Nevalainen, A, Mäkelä, MJ, et al. Predominance of gram-positive bacteria in house dust in the low-allergy risk Russian Karelia. Environ Microbiol. (2008) 10:3317–25. doi: 10.1111/j.1462-2920.2008.01723.x
43. Rintala, H, Pitkäranta, M, and Täubel, M. Microbial communities associated with house dust. Adv Appl Microbiol. (2012) 78:75–120. doi: 10.1016/B978-0-12-394805-2.00004-X
44. Leppänen, HK, Täubel, M, Jayaprakash, B, Vepsäläinen, A, Pasanen, P, and Hyvärinen, A. Quantitative assessment of microbes from samples of indoor air and dust. J Expo Sci Environ Epidemiol. (2018) 28:231–41. doi: 10.1038/jes.2017.24
45. Wu, Z, Lyu, H, Ma, X, Ren, G, Song, J, Jing, X, et al. Comparative effects of environmental factors on bacterial communities in two types of indoor dust: potential risks to university students. Environ Res. (2022) 203:111869. doi: 10.1016/j.envres.2021.111869
46. Rawat, MS, and Ferro, AR. Respiratory virus deposition and resuspension from indoor surfaces In: D Barry and H Kanematsu, editors. Studies to combat COVID-19 using science and engineering. Singapore: Springer Nature Singapore (2022). 107–18.
48. Lai, ACK, Tan, TF, Li, WS, and Ip, DKM. Emission strength of airborne pathogens during toilet flushing. Indoor Air. (2018) 28:73–9. doi: 10.1111/ina.12406
49. Lou, M, Liu, S, Gu, C, Hu, H, Tang, Z, Zhang, Y, et al. The bioaerosols emitted from toilet and wastewater treatment plant: a literature review. Environ Sci Pollut Res. (2021) 28:2509–21. doi: 10.1007/s11356-020-11297-8
50. Barker, J, and Jones, MV. The potential spread of infection caused by aerosol contamination of surfaces after flushing a domestic toilet. J Appl Microbiol. (2005) 99:339–47. doi: 10.1111/j.1365-2672.2005.02610.x
51. Cho, SJ, Cox-Ganser, JM, and Park, J-H. Observational scores of dampness and mold associated with measurements of microbial agents and moisture in three public schools. Indoor Air. (2016) 26:168–78. doi: 10.1111/ina.12191
52. Solomon, GM, Hjelmroos-Koski, M, Rotkin-Ellman, M, and Hammond, SK. Airborne Mold and endotoxin concentrations in New Orleans, Louisiana, after flooding, October through November 2005. Environ Health Perspect. (2006) 114:1381–6. doi: 10.1289/ehp.9198
53. Kwan, SE, Shaughnessy, R, Haverinen-Shaughnessy, U, Kwan, TA, and Peccia, J. The impact of ventilation rate on the fungal and bacterial ecology of home indoor air. Build Environ. (2020) 177:106800. doi: 10.1016/j.buildenv.2020.106800
54. Stanley, HO, Onwuna, B, and Ugboma, CJ. Microbial assessment of indoor air quality of ventilation systems. Asian J Adv Res Rep. (2019) 2019:1–7. doi: 10.9734/ajarr/2019/v3i430095
55. Lee, BU, Yun, SH, Ji, J-H, and Bae, G-N. Inactivation of S. epidermidis, B. subtilis, and E. coli bacteria bioaerosols deposited on a filter utilizing airborne silver nanoparticles. J Microbiol Biotechnol. (2008) 18:176–82.
56. Hassan Al-abdalall, A, Abdullah Al-dakheel, S, and Abdulhadi, A-AH. Impact of air-conditioning filters on microbial growth and indoor air pollution In: T Morosuk and M Sultan, editors. Low-temperature technologies. London: IntechOpen (2020)
57. Mahnert, A, Moissl-Eichinger, C, and Berg, G. Microbiome interplay: plants alter microbial abundance and diversity within the built environment. Front Microbiol. (2015) 6:887. doi: 10.3389/fmicb.2015.00887
58. Berg, G, Mahnert, A, and Moissl-Eichinger, C. Beneficial effects of plant-associated microbes on indoor microbiomes and human health? Front Microbiol. (2014) 5:15. doi: 10.3389/fmicb.2014.00015
59. Wolverton, BC, and Wolverton, JD. Interior plants: their influence on airborne microbes inside energy-efficient buildings. J Miss Acad Sci. (1996) 41:100–5.
60. Burge, HA, Solomon, WR, and Muilenberg, ML. Evaluation of indoor plantings as allergen exposure sources. J Allergy Clin Immunol. (1982) 70:101–8. doi: 10.1016/0091-6749(82)90236-6
61. Napoli, C, Marcotrigiano, V, and Montagna, MT. Air sampling procedures to evaluate microbial contamination: a comparison between active and passive methods in operating theatres. BMC Public Health. (2012) 12:594. doi: 10.1186/1471-2458-12-594
62. Saffell, J, and Nehr, S. Improving indoor air quality through standardization. Standards. (2023) 3:240–67. doi: 10.3390/standards3030019
63. Viani, I, Colucci, ME, Pergreffi, M, Rossi, D, Veronesi, L, Bizzarro, A, et al. Passive air sampling: the use of the index of microbial air contamination. Acta Biomed. (2020) 91:92–105. doi: 10.23750/abm.v91i3-S.9434
64. Kotgire, S, Akhtar, R, Damle, A, Siddiqui, S, and Padekar, H. Bioaerosol assessment of indoor air in hospital wards from a tertiary care hospital. Indian J Microbiol Res. (2020) 7:28–34. doi: 10.18231/j.ijmr.2020.007
65. Haig, CW, Mackay, WG, Walker, JT, and Williams, C. Bioaerosol sampling: sampling mechanisms, bioefficiency and field studies. J Hosp Infect. (2016) 93:242–55. doi: 10.1016/j.jhin.2016.03.017
66. French, MLV, Eitzen, HE, Ritter, MA, and Leland, DS. Wound healing and wound infection In: TK Hunt, editor. Environmental control of microbial contamination in the operating room. New York: Appleton-Century Crois (1980). 254–61.
67. Wurtz, H, Sigsgaard, T, Valbjorn, O, Doekes, G, and Meyer, HW. The dustfall collector - a simple passive tool for long-term collection of airborne dust: a project under the Danish Mould in buildings program (DAMIB). Indoor Air. (2005) 15:33–40. doi: 10.1111/j.1600-0668.2005.00342.x
68. Manibusan, S, and Mainelis, G. Passive bioaerosol samplers: a complementary tool for bioaerosol research. A review. J Aerosol Sci. (2022) 163:105992. doi: 10.1016/j.jaerosci.2022.105992
69. Therkorn, J, Thomas, N, Scheinbeim, J, and Mainelis, G. Field performance of a novel passive bioaerosol sampler using polarized ferroelectric polymer films. Aerosol Sci Technol. (2017) 51:787–800. doi: 10.1080/02786826.2017.1316830
70. Cox, J, Indugula, R, Vesper, S, Zhu, Z, Jandarov, R, and Reponen, T. Comparison of indoor air sampling and dust collection methods for fungal exposure assessment using quantitative PCR. Environ Sci Process Impacts. (2017) 19:1312–9. doi: 10.1039/C7EM00257B
71. Saha, R, Agarawal, S, and Khan, AM. Air sampling procedures to evaluate microbial contamination: a comparison between active and passive methods at high-risk areas in a tertiary care hospital of Delhi. J Patient Saf Infect Control. (2017) 5:18–23. doi: 10.4103/jpsic.jpsic_12_17
72. Massoudinejad, MR, Ghajari, A, Hezarkhani, N, and Aliyari, A. Survey of fungi bioaersols in ICU ward of Taleghani hospital in Tehran by petri-dish trapping technique and bioaerosol sampler in 2013. J Saf Promot Injury Prevent. (2015) 3:147–54.
73. Petti, S, Iannazzo, S, and Tarsitani, G. Comparison between different methods to monitor the microbial level of indoor air contamination in the dental office. Ann Ig. (2003) 15:725–33.
74. Balyan, P, Ghosh, C, Das, S, and Banerjee, BD. Comparison of efficiency of active and passive methods of bioaerosols’ estimation In: P Balyan, editor. Lecture notes in civil engineering. Berlin: Springer (2020). 85–94. doi: 10.1007/978-981-15-1334-3_10
75. Canha, N, Almeida, SM, Freitas Do, CM, and Wolterbeek, HT. Assessment of bioaerosols in urban and rural primary schools using passive and active sampling methodologies. Archives of. Environ Prot. (2015) 41:11–22. doi: 10.1515/aep-2015-0034
76. Sudharsanam, S, Swaminathan, S, Ramalingam, A, Thangavel, G, Annamalai, R, Steinberg, R, et al. Characterization of indoor bioaerosols from a hospital ward in a tropical setting. Afr Health Sci. (2012) 12:217–25. doi: 10.4314/ahs.v12i2.22
77. Dallongeville, A, le Cann, P, Zmirou-Navier, D, Chevrier, C, Costet, N, Annesi-Maesano, I, et al. Concentration and determinants of molds and allergens in indoor air and house dust of French dwellings. Sci Total Environ. (2015) 536:964–72. doi: 10.1016/j.scitotenv.2015.06.039
78. Pasquarella, C, Albertini, R, Dall’aglio, P, Saccani, E, Sansebastiano, GE, and Signorelli, C. Air microbial sampling: the state of the art. Ig Sanita Pubbl. (2008) 64:79–120.
79. Frankel, M, Timm, M, Hansen, EW, and Madsen, AM. Comparison of sampling methods for the assessment of indoor microbial exposure. Indoor Air. (2012) 22:405–14. doi: 10.1111/j.1600-0668.2012.00770.x
80. Jensen, PA, and Schafer, MP. Sampling and characterization of bioaerosols. In: PA Jensen. NIOSH Manual of Analytical Methods. Atlanta, Georgia: CDC pp. 82–112. (1998).
81. Mandal, J, and Brandl, H. Bioaerosols in indoor environment–a review with special reference to residential and occupational locations. Open Environ Biol Monit J. (2011) 4:83–96. doi: 10.2174/1875040001104010083
82. Kalogerakis, N, Paschali, D, Lekaditis, V, Pantidou, A, Eleftheriadis, K, and Lazaridis, M. Indoor air quality—bioaerosol measurements in domestic and office premises. J Aerosol Sci. (2005) 36:751–61. doi: 10.1016/j.jaerosci.2005.02.004
83. Prigione, V, Lingua, G, and Marchisio, VF. Development and use of flow cytometry for detection of airborne Fungi. Appl Environ Microbiol. (2004) 70:1360–5. doi: 10.1128/AEM.70.3.1360-1365.2004
84. Hugenholtz, P. Exploring prokaryotic diversity in the genomic era. Genome Biol. (2002) 3:REVIEWS0003. doi: 10.1186/gb-2002-3-2-reviews0003
85. Rahi, P, Prakash, O, and Shouche, YS. Matrix-assisted laser desorption/ionization time-of-flight mass-spectrometry (MALDI-TOF MS) based microbial identifications: challenges and scopes for microbial ecologists. Front Microbiol. (2016) 7:1359. doi: 10.3389/fmicb.2016.01359
86. Singhal, N, Kumar, M, Kanaujia, PK, and Virdi, JS. MALDI-TOF mass spectrometry: an emerging technology for microbial identification and diagnosis. Front Microbiol. (2015) 6:791. doi: 10.3389/fmicb.2015.00791
87. Ashfaq, MY, Da’na, DA, and Al-Ghouti, MA. Application of MALDI-TOF MS for identification of environmental bacteria: a review. J Environ Manag. (2022) 305:114359. doi: 10.1016/j.jenvman.2021.114359
88. Jang, K-S, and Kim, YH. Rapid and robust MALDI-TOF MS techniques for microbial identification: a brief overview of their diverse applications. J Microbiol. (2018) 56:209–16. doi: 10.1007/s12275-018-7457-0
89. Fykse, EM, Tjärnhage, T, Humppi, T, Eggen, VS, Ingebretsen, A, Skogan, G, et al. Identification of airborne bacteria by 16S rDNA sequencing, MALDI-TOF MS and the MIDI microbial identification system. Aerobiologia (Bologna). (2015) 31:271–81. doi: 10.1007/s10453-015-9363-9
90. Fox, K, Fox, A, Elßner, T, Feigley, C, and Salzberg, D. MALDI-TOF mass spectrometry speciation of staphylococci and their discrimination from micrococci isolated from indoor air of schoolrooms. J Environ Monit. (2010) 12:917–23. doi: 10.1039/b925250a
91. Motteu, N, Goemaere, B, Bladt, S, and Packeu, A. Implementation of MALDI-TOF mass spectrometry to identify Fungi from the indoor environment as an added value to the classical morphology-based identification tool. Front Allergy. (2022) 3:826148. doi: 10.3389/falgy.2022.826148
92. Fenselau, CC. Rapid characterization of microorganisms by mass spectrometry–what can be learned and how? J Am Soc Mass Spectrom. (2013) 24:1161–6. doi: 10.1007/s13361-013-0660-7
93. Geng, Z, Yang, W, Wei, S, Wang, L, and Pan, J. Verification of real-time microbial monitoring technology based on the principle of laser-induced fluorescence under USP <1223> standard and prospective application in our hospital PIVAS clean room. Int J Pept Res Ther. (2022) 28:4. doi: 10.1007/s10989-021-10315-8
94. Saari, S, Järvinen, S, Reponen, T, Mensah-Attipoe, J, Pasanen, P, Toivonen, J, et al. Identification of single microbial particles using electro-dynamic balance assisted laser-induced breakdown and fluorescence spectroscopy. Aerosol Sci Technol. (2016) 50:126–32. doi: 10.1080/02786826.2015.1134764
95. Babichenko, S, Gala, J, Bentahir, M, Piette, A, Poryvkina, L, Rebane, O, et al. Real-time laser-induced fluorescence detection and monitoring of microbial contaminants on solid surfaces before, during and after decontamination. J Biosens Bioelectron. (2018) 9:255. doi: 10.4172/2155-6210.1000255
96. Leone, N, D’Arthur, G, Adam, P, and Amouroux, J. Detection of bacterial deposits and bio aerosols by time-resolved laser-induced breakdown spectroscopy (trelibs). High Temp Mater Proc. (2004) 8:1–22. doi: 10.1615/HighTempMatProc.v8.i1.10
97. Gabbarini, V, Rossi, R, Ciparisse, J-F, Malizia, A, Divizia, A, de Filippis, P, et al. Laser-induced fluorescence (LIF) as a smart method for fast environmental virological analyses: validation on picornaviruses. Sci Rep. (2019) 9:12598. doi: 10.1038/s41598-019-49005-3
98. Owoicho, O, Olwal, CO, and Quaye, O. Potential of laser-induced fluorescence-light detection and ranging for future stand-off virus surveillance. Microb Biotechnol. (2021) 14:126–35. doi: 10.1111/1751-7915.13698
99. Singh, VK, Sharma, J, Pathak, AK, Ghany, CT, and Gondal, MA. Laser-induced breakdown spectroscopy (LIBS): a novel technology for identifying microbes causing infectious diseases. Biophys Rev. (2018) 10:1221–39. doi: 10.1007/s12551-018-0465-9
100. Saari, S, Putkiranta, MJ, and Keskinen, J. Fluorescence spectroscopy of atmospherically relevant bacterial and fungal spores and potential interferences. Atmos Environ. (2013) 71:202–9. doi: 10.1016/j.atmosenv.2013.02.023
101. Prussin, AJ, Garcia, EB, and Marr, LC. Total concentrations of virus and Bacteria in indoor and outdoor air. Environ Sci Technol Lett. (2015) 2:84–8. doi: 10.1021/acs.estlett.5b00050
102. Li, C-S, and Huang, T-Y. Fluorochrome in monitoring indoor bioaerosols. Aerosol Sci Technol. (2006) 40:237–41. doi: 10.1080/02786820500543308
103. Fabian, MP, Miller, SL, Reponen, T, and Hernandez, MT. Ambient bioaerosol indices for indoor air quality assessments of flood reclamation. J Aerosol Sci. (2005) 36:763–83. doi: 10.1016/j.jaerosci.2004.11.018
104. Chi, M-C, and Li, C-S. Fluorochrome and fluorescent in situ hybridization to monitor bioaerosols in swine buildings. Aerosol Sci Technol. (2005) 39:1101–10. doi: 10.1080/02786820500421539
105. Bergquist, PL, Hardiman, EM, Ferrari, BC, and Winsley, T. Applications of flow cytometry in environmental microbiology and biotechnology. Extremophiles. (2009) 13:389–401. doi: 10.1007/s00792-009-0236-4
106. Lange, JL, Thorne, PS, and Lynch, N. Application of flow cytometry and fluorescent in situ hybridization for assessment of exposures to airborne bacteria. Appl Environ Microbiol. (1997) 63:1557–63. doi: 10.1128/aem.63.4.1557-1563.1997
107. Wang, Y, Hammes, F, de Roy, K, Verstraete, W, and Boon, N. Past, present and future applications of flow cytometry in aquatic microbiology. Trends Biotechnol. (2010) 28:416–24. doi: 10.1016/j.tibtech.2010.04.006
108. Chen, P-S, and Li, C-S. Bioaerosol characterization by flow cytometry with fluorochrome. J Environ Monit. (2005) 7:950–9. doi: 10.1039/b505224f
109. Nimra, A, Ali, Z, Sultan, S, Nasir, ZA, Sidra, S, and Hussain, A. Molecular sequencing and phylogenetic analysis of bioaerosols in hospital wards with different ventilation conditions. J Infect Dev Countr. (2022) 16:157–65. doi: 10.3855/jidc.13682
110. Jayan, H, Pu, H, and Sun, D-W. Recent developments in Raman spectral analysis of microbial single cells: techniques and applications. Crit Rev Food Sci Nutr. (2022) 62:4294–308. doi: 10.1080/10408398.2021.1945534
111. Sengupta, A, Laucks, ML, Dildine, N, Drapala, E, and Davis, EJ. Bioaerosol characterization by surface-enhanced Raman spectroscopy (SERS). J Aerosol Sci. (2005) 36:651–64. doi: 10.1016/j.jaerosci.2004.11.001
112. Witkowska, E, Jagielski, T, Kamińska, A, Kowalska, A, Hryncewicz-Gwóźdź, A, and Waluk, J. Detection and identification of human fungal pathogens using surface-enhanced Raman spectroscopy and principal component analysis. Anal Methods. (2016) 8:8427–34. doi: 10.1039/C6AY02957D
113. Jarvis, RM, Brooker, A, and Goodacre, R. Surface-enhanced Raman spectroscopy for bacterial discrimination utilizing a scanning Electron microscope with a Raman spectroscopy Interface. Anal Chem. (2004) 76:5198–202. doi: 10.1021/ac049663f
114. Schwarzmeier, K, Knauer, M, Ivleva, NP, Niessner, R, and Haisch, C. Bioaerosol analysis based on a label-free microarray readout method using surface-enhanced Raman scattering. Anal Bioanal Chem. (2013) 405:5387–92. doi: 10.1007/s00216-013-6984-0
115. Ghosal, S, Macher, JM, and Ahmed, K. Raman microspectroscopy-based identification of individual fungal spores as potential indicators of indoor contamination and moisture-related building damage. Environ Sci Technol. (2012) 46:6088–95. doi: 10.1021/es203782j
116. Su, C, Lei, L, Duan, Y, Zhang, K-Q, and Yang, J. Culture-independent methods for studying environmental microorganisms: methods, application, and perspective. Appl Microbiol Biotechnol. (2012) 93:993–1003. doi: 10.1007/s00253-011-3800-7
117. Kuypers, MMM, and Jørgensen, BB. The future of single-cell environmental microbiology. Environ Microbiol. (2007) 9:6–7. doi: 10.1111/j.1462-2920.2006.01222_5.x
118. Adams, RI, Bateman, AC, Bik, HM, and Meadow, JF. Microbiota of the indoor environment: a meta-analysis. Microbiome. (2015) 3:49. doi: 10.1186/s40168-015-0108-3
119. Stephens, B. What have we learned about the microbiomes of indoor environments? mSystems. (2016) 1:16. doi: 10.1128/mSystems.00083-16
120. Georgakopoulos, DG, Després, V, Fröhlich-Nowoisky, J, Psenner, R, Ariya, PA, Pósfai, M, et al. Microbiology and atmospheric processes: biological, physical and chemical characterization of aerosol particles. Biogeosciences. (2009) 6:721–37. doi: 10.5194/bg-6-721-2009
121. Leung, MHY, Wilkins, D, Li, EKT, Kong, FKF, and Lee, PKH. Indoor-air microbiome in an urban Subway network: diversity and dynamics. Appl Environ Microbiol. (2014) 80:6760–70. doi: 10.1128/AEM.02244-14
122. Smith, CJ, and Osborn, AM. Advantages and limitations of quantitative PCR (Q-PCR)-based approaches in microbial ecology. FEMS Microbiol Ecol. (2009) 67:6–20. doi: 10.1111/j.1574-6941.2008.00629.x
123. Kärkkäinen, PM, Valkonen, M, Hyvärinen, A, Nevalainen, A, and Rintala, H. Determination of bacterial load in house dust using qPCR, chemical markers and culture. J Environ Monit. (2010) 12:759. doi: 10.1039/b917937b
124. Garibyan, L, and Avashia, N. Polymerase chain reaction. J Investig Dermatol. (2013) 133:1–4. doi: 10.1038/jid.2013.1
125. Nocker, A, Burr, M, and Camper, AK. Genotypic microbial community profiling: a critical technical review. Microb Ecol. (2007) 54:276–89. doi: 10.1007/s00248-006-9199-5
126. Weikl, F, Tischer, C, Probst, AJ, Heinrich, J, Markevych, I, Jochner, S, et al. Fungal and bacterial communities in indoor dust follow different environmental determinants. PLoS One. (2016) 11:4131. doi: 10.1371/journal.pone.0154131
127. Thomas, T, Gilbert, J, and Meyer, F. Metagenomics–a guide from sampling to data analysis. Microb Inform Exp. (2012) 2:3. doi: 10.1186/2042-5783-2-3
128. Nygaard, AB, Tunsjø, HS, Meisal, R, and Charnock, C. A preliminary study on the potential of Nanopore MinION and Illumina MiSeq 16S rRNA gene sequencing to characterize building-dust microbiomes. Sci Rep. (2020) 10:3209. doi: 10.1038/s41598-020-59771-0
129. Madsen, AM, Zervas, A, Tendal, K, and Nielsen, JL. Microbial diversity in bioaerosol samples causing ODTS compared to reference bioaerosol samples as measured using Illumina sequencing and MALDI-TOF. Environ Res. (2015) 140:255–67. doi: 10.1016/j.envres.2015.03.027
130. Nazzal, Y, Howari, FM, Yaslam, A, Iqbal, J, Maloukh, L, Ambika, LK, et al. A methodological review of tools that assess dust microbiomes, Metatranscriptomes and the particulate chemistry of indoor dust. Atmosphere (Basel). (2022) 13:1276. doi: 10.3390/atmos13081276
131. Gangneux, J-P, Sassi, M, Lemire, P, and Le Cann, P. Metagenomic characterization of indoor dust bacterial and fungal microbiota in homes of asthma and non-asthma patients using next generation sequencing. Front Microbiol. (2020) 11:1671. doi: 10.3389/fmicb.2020.01671
132. Shin, S-K, Kim, J, Ha, S, Oh, H-S, Chun, J, Sohn, J, et al. Metagenomic insights into the bioaerosols in the indoor and outdoor environments of childcare facilities. PLoS One. (2015) 10:e0126960. doi: 10.1371/journal.pone.0126960
133. Rosario, K, Fierer, N, Miller, S, Luongo, J, and Breitbart, M. Diversity of DNA and RNA viruses in indoor air as assessed via metagenomic sequencing. Environ Sci Technol. (2018) 52:1014–27. doi: 10.1021/acs.est.7b04203
134. Ko, KKK, Chng, KR, and Nagarajan, N. Metagenomics-enabled microbial surveillance. Nat Microbiol. (2022) 7:486–96. doi: 10.1038/s41564-022-01089-w
135. Behzad, H, Gojobori, T, and Mineta, K. Challenges and opportunities of airborne metagenomics. Genome Biol Evol. (2015) 7:1216–26. doi: 10.1093/gbe/evv064
136. Cappitelli, F, Fermo, P, Vecchi, R, Piazzalunga, A, Valli, G, Zanardini, E, et al. Chemical–physical and microbiological measurements for indoor air quality assessment at the ca’ Granda historical archive, Milan (Italy). Water Air Soil Pollut. (2009) 201:109–20. doi: 10.1007/s11270-008-9931-5
137. Fsadni, P, Frank, B, Fsadni, C, and Montefort, S. The impact of microbiological pollutants on school indoor air quality. J Geosci Environ Prot. (2017) 5:54–65. doi: 10.4236/gep.2017.55004
138. Montagna, MT, De Giglio, O, Cristina, ML, Napoli, C, Pacifico, C, Agodi, A, et al. Evaluation of Legionella air contamination in healthcare facilities by different sampling methods: An Italian multicenter study. Int J Environ Res Public Health. (2017) 14:670. doi: 10.3390/ijerph14070670
139. Solomon, FB, Wadilo, F, Tufa, EG, and Mitiku, M. Extended spectrum and metalo beta-lactamase producing airborne Pseudomonas aeruginosa and Acinetobacter baumanii in restricted settings of a referral hospital: a neglected condition. Antimicrob Resist Infect Control. (2017) 6:106. doi: 10.1186/s13756-017-0266-0
140. Asif, A, Zeeshan, M, Hashmi, I, Zahid, U, and Bhatti, MF. Microbial quality assessment of indoor air in a large hospital building during winter and spring seasons. Build Environ. (2018) 135:68–73. doi: 10.1016/j.buildenv.2018.03.010
141. Brągoszewska, E, and Biedroń, I. Indoor air quality and potential health risk impacts of exposure to antibiotic resistant Bacteria in an office rooms in southern Poland. Int J Environ Res Public Health. (2018) 15:2604. doi: 10.3390/ijerph15112604
142. Choi, JY, Zemke, J, Philo, SE, Bailey, ES, Yondon, M, and Gray, GC. Aerosol sampling in a hospital emergency room setting: a complementary surveillance method for the detection of respiratory viruses. Front Public Health. (2018) 6:174. doi: 10.3389/fpubh.2018.00174
143. Kozdrój, J, Frączek, K, and Ropek, D. Assessment of bioaerosols in indoor air of glasshouses located in a botanical garden. Build Environ. (2019) 166:106436. doi: 10.1016/j.buildenv.2019.106436
144. Sornboot, J, Aekplakorn, W, Ramasoota, P, Bualert, S, Tumwasorn, S, and Jiamjarasrangsi, W. Detection of airborne Mycobacterium tuberculosis complex in high-risk areas of health care facilities in Thailand. Int J Tuberc Lung Dis. (2019) 23:465–73. doi: 10.5588/ijtld.18.0218
145. Awad, AHA, Saeed, Y, Shakour, AA, Abdellatif, NM, Ibrahim, YH, Elghanam, M, et al. Indoor air fungal pollution of a historical museum, Egypt: a case study. Aerobiologia. (2020) 36:197–209. doi: 10.1007/s10453-019-09623-w
146. Vornanen-Winqvist, C, Järvi, K, Andersson, MA, Duchaine, C, Létourneau, V, Kedves, O, et al. Exposure to indoor air contaminants in school buildings with and without reported indoor air quality problems. Environ Int. (2020) 141:105781. doi: 10.1016/j.envint.2020.105781
147. Baudet, A, Baurès, E, Guegan, H, Blanchard, O, Guillaso, M, Le Cann, P, et al. Indoor air quality in healthcare and care facilities: chemical pollutants and microbiological contaminants. Atmosphere (Basel). (2021) 12:1337. doi: 10.3390/atmos12101337
148. Cox, J, Stone, T, Ryan, P, Burkle, J, Jandarov, R, Mendell, MJ, et al. Associations of observed home dampness and mold with the fungal and bacterial dust microbiomes. Environ Sci Process Impacts. (2021) 23:491–500. doi: 10.1039/D0EM00505C
149. Habibi, N, Uddin, S, Al-Salameen, F, Al-Amad, S, Kumar, V, Al-Otaibi, M, et al. SARS-CoV-2, other respiratory viruses and bacteria in aerosols: Report from Kuwait’s hospitals. Indoor Air. (2021) 31:1815–25. doi: 10.1111/ina.12871
150. Lu, R, Pørneki, AD, Lindgreen, JN, Li, Y, and Madsen, AM. Species of Fungi and pollen in the PM1 and the inhalable fraction of indoor air in homes. Atmosphere (Basel). (2021) 12:404. doi: 10.3390/atmos12030404
151. Sibanda, T, Selvarajan, R, Ogola, HJ, Obieze, CC, and Tekere, M. Distribution and comparison of bacterial communities in HVAC systems of two university buildings: implications for indoor air quality and public health. Environ Monit Assess. (2021) 193:47. doi: 10.1007/s10661-020-08823-z
152. Vosoughi, M, Karami, C, Dargahi, A, Jeddi, F, Jalali, KM, Hadisi, A, et al. Investigation of SARS-CoV-2 in hospital indoor air of COVID-19 patients’ ward with impinger method. Environ Sci Pollut Res. (2021) 28:50480–8. doi: 10.1007/s11356-021-14260-3
153. Zavieh, FS, Mohammadi, MJ, Vosoughi, M, Abazari, M, Raesee, E, Fazlzadeh, M, et al. Assessment of types of bacterial bio-aerosols and concentrations in the indoor air of gyms. Environ Geochem Health. (2021) 43:2165–73. doi: 10.1007/s10653-020-00774-1
154. Maphossa, V, Langa, JC, Simbine, S, Maússe, FE, Kenga, D, Relvas, V, et al. Environmental bacterial and fungal contamination in high touch surfaces and indoor air of a paediatric intensive care unit in Maputo central hospital, Mozambique in 2018. Infect Prevent Pract. (2022) 4:100250. doi: 10.1016/j.infpip.2022.100250
155. An, X-L, Xu, J-X, Xu, M-R, Zhao, C-X, Li, H, Zhu, Y-G, et al. Dynamics of microbial community and potential microbial pollutants in shopping malls. mSystems. (2023) 8:e0057622. doi: 10.1128/msystems.00576-22
156. Kim, J, Bae, S, Park, S, Shukla, SK, and Yoo, K. Investigation of surface bacterial diversities and compositions in the global Subway facilities. Atmosphere (Basel). (2023) 14:140. doi: 10.3390/atmos14010140
157. Rintala, H, Pitkaranta, M, Toivola, M, Paulin, L, and Nevalainen, A. Diversity and seasonal dynamics of bacterial community in indoor environment. BMC Microbiol. (2008) 8:56. doi: 10.1186/1471-2180-8-56
158. Bonadonna, L, Briancesco, R, Coccia, AM, Meloni, P, La Rosa, G, and Moscato, U. Microbial air quality in healthcare facilities. Int J Environ Res Public Health. (2021) 18:6226. doi: 10.3390/ijerph18126226
159. Chang, CW, Chou, FC, and Hung, PY. Evaluation of bioaerosol sampling techniques for Legionella pneumophila coupled with culture assay and quantitative PCR. J Aerosol Sci. (2010) 41:1055–65. doi: 10.1016/j.jaerosci.2010.09.002
160. Spagnolo, AM, Cristina, ML, Casini, B, and Perdelli, F. Legionella pneumophila in healthcare facilities. Rev Med Microbiol. (2013) 24:70–80. doi: 10.1097/MRM.0b013e328362fe66
161. Zhang, BY, Liu, F, and Chen, XD. The effect of Legionella Pneumophila contamination in the surface dust of the air ducts of central air conditioning Systemson indoor air quality. Int J Vent. (2015) 14:231–40. doi: 10.1080/14733315.2015.11684083
162. Lee, G, and Yoo, K. A review of the emergence of antibiotic resistance in bioaerosols and its monitoring methods. Rev Environ Sci Biotechnol. (2022) 21:799–827. doi: 10.1007/s11157-022-09622-3
163. Bitew, K, Gidebo, DD, and Ali, MM. Bacterial contamination rates and drug susceptibility patterns of bacteria recovered from medical equipment, inanimate surfaces, and indoor air of a neonatal intensive care unit and pediatric ward at Hawassa university comprehensive specialized hospital, Ethiopia. IJID Reg. (2021) 1:27–33. doi: 10.1016/j.ijregi.2021.09.005
164. Douwes, J, Thorne, P, Pearce, N, and Heederik, D. Bioaerosol health effects and exposure assessment: Progress and prospects. Ann Occup Hyg. (2003) 47:187–200. doi: 10.1093/annhyg/meg032
165. Li, X, Liu, D, and Yao, J. Aerosolization of fungal spores in indoor environments. Sci Total Environ. (2022) 820:153003. doi: 10.1016/j.scitotenv.2022.153003
166. Liu, Z, Yin, H, Ma, S, Wei, B, Jensen, B, and Cao, G. Effect of environmental parameters on culturability and viability of dust accumulated fungi in different HVAC segments. Sustain Cities Soc. (2019) 48:101538. doi: 10.1016/j.scs.2019.101538
167. Belizario, JA, Lopes, LG, and Pires, RH. Fungi in the indoor air of critical hospital areas: a review. Aerobiologia (Bologna). (2021) 37:379–94. doi: 10.1007/s10453-021-09706-7
168. Khalaf, NF, Al-Obaidi, MJ, Mohammed, SW, Al-Malkey, MK, Nayyef, HJ, Al-Hur, FJA, et al. Indoor house dust-borne fungi and risk of allergic respiratory diseases in Baghdad city. Rev Fr Allergol. (2022) 62:401–6. doi: 10.1016/j.reval.2021.05.002
169. Kaarakainen, P, Rintala, H, Vepsäläinen, A, Hyvärinen, A, Nevalainen, A, and Meklin, T. Microbial content of house dust samples determined with qPCR. Sci Total Environ. (2009) 407:4673–80. doi: 10.1016/j.scitotenv.2009.04.046
170. Barker, J, Stevens, D, and Bloomfield, SF. Spread and prevention of some common viral infections in community facilities and domestic homes. J Appl Microbiol. (2001) 91:7–21. doi: 10.1046/j.1365-2672.2001.01364.x
171. López, A, Fuentes, E, Yusà, V, López-Labrador, F, Camaró, M, Peris-Martinez, C, et al. Indoor air quality including respiratory viruses. Toxics. (2021) 9:274. doi: 10.3390/toxics9110274
172. Ciencewicki, J, and Jaspers, I. Air pollution and respiratory viral infection. Inhal Toxicol. (2007) 19:1135–46. doi: 10.1080/08958370701665434
173. Clements, N, Binnicker, MJ, and Roger, VL. Indoor environment and viral infections. Mayo Clin Proc. (2020) 95:1581–3. doi: 10.1016/j.mayocp.2020.05.028
174. Myatt, TA, Kaufman, MH, Allen, JG, MacIntosh, DL, Fabian, MP, and McDevitt, JJ. Modeling the airborne survival of influenza virus in a residential setting: the impacts of home humidification. Environ Health. (2010) 9:55. doi: 10.1186/1476-069X-9-55
175. Johnson, GR, and Morawska, L. The mechanism of breath aerosol formation. J Aerosol Med Pulm Drug Deliv. (2009) 22:229–37. doi: 10.1089/jamp.2008.0720
176. Moon, KW, Huh, EH, and Jeong, HC. Seasonal evaluation of bioaerosols from indoor air of residential apartments within the metropolitan area in South Korea. Environ Monit Assess. (2014) 186:2111–20. doi: 10.1007/s10661-013-3521-8
177. Yang, W, Elankumaran, S, and Marr, LC. Concentrations and size distributions of airborne influenza a viruses measured indoors at a health Centre, a day-care Centre and on aeroplanes. J R Soc Interface. (2011) 8:1176–84. doi: 10.1098/rsif.2010.0686
178. Weinstein, RA, Weber, DJ, and Rutala, WA. Risks and prevention of nosocomial transmission of rare zoonotic diseases. Clin Infect Dis. (2001) 32:446–56. doi: 10.1086/318509
179. Yu, ITS, Li, Y, Wong, TW, Tam, W, Chan, AT, Lee, JHW, et al. Evidence of airborne transmission of the severe acute respiratory syndrome virus. N Engl J Med. (2004) 350:1731–9. doi: 10.1056/NEJMoa032867
180. Qian, H, Miao, T, Liu, L, Zheng, X, Luo, D, and Li, Y. Indoor transmission of SARS-CoV-2. Indoor Air. (2021) 31:639–45. doi: 10.1111/ina.12766
181. Norbäck, D, and Cai, GH. Microbial agents in the indoor environment: associations with health. Environmental Science, pp. 179–198. (2020).
182. Kim, K-H, Kabir, E, and Jahan, SA. Airborne bioaerosols and their impact on human health. J Environ Sci. (2018) 67:23–35. doi: 10.1016/j.jes.2017.08.027
183. Humbal, C, Gautam, S, and Trivedi, U. A review on recent progress in observations, and health effects of bioaerosols. Environ Int. (2018) 118:189–93. doi: 10.1016/j.envint.2018.05.053
184. Pomés, A, Chapman, MD, and Wünschmann, S. Indoor allergens and allergic respiratory disease. Curr Allergy Asthma Rep. (2016) 16:43. doi: 10.1007/s11882-016-0622-9
185. Sun, Y, Meng, Y, Ou, Z, Li, Y, Zhang, M, Chen, Y, et al. Indoor microbiome, air pollutants and asthma, rhinitis and eczema in preschool children–a repeated cross-sectional study. Environ Int. (2022) 161:107137. doi: 10.1016/j.envint.2022.107137
186. Brooks, C, Pearce, N, and Douwes, J. The hygiene hypothesis in allergy and asthma. Curr Opin Allergy Clin Immunol. (2013) 13:70–7. doi: 10.1097/ACI.0b013e32835ad0d2
187. Sham, NM, Ahmad, NI, Pahrol, MA, and Leong, Y-H. Fungus and mycotoxins studies in hospital environment: a scoping review. Build Environ. (2021) 193:107626. doi: 10.1016/j.buildenv.2021.107626
188. Oshikata, C, Watanabe, M, Saito, A, Ishida, M, Kobayashi, S, Konuma, R, et al. Allergic bronchopulmonary mycosis due to exposure to Eurotium herbariorum after the great East Japan earthquake. Prehosp Disaster Med. (2017) 32:688–90. doi: 10.1017/S1049023X17006914
189. Sarkingobir, Y, Zayyanu, MM, and Sarkingobir, S. Sick building syndrome: a review of related literatures. Front Knowl J. (2017) 1:1–11.
190. Wålinder, R, Ernstgård, L, Johanson, G, Norbäck, D, Venge, P, and Wieslander, G. Acute effects of a fungal volatile compound. Environ Health Perspect. (2005) 113:1775–8. doi: 10.1289/ehp.8193
191. Haleem Khan, AA, and Karuppayil, SM. Fungal pollution of indoor environments and its management. Saudi J Biol Sci. (2012) 19:405–26. doi: 10.1016/j.sjbs.2012.06.002
192. Nardell, EA. Indoor environmental control of tuberculosis and other airborne infections. Indoor Air. (2016) 26:79–87. doi: 10.1111/ina.12232
193. Solomon, FB, Wadilo, FW, Arota, AA, and Abraham, YL. Antibiotic resistant airborne bacteria and their multidrug resistance pattern at university teaching referral Hospital in South Ethiopia. Ann Clin Microbiol Antimicrob. (2017) 16:29. doi: 10.1186/s12941-017-0204-2
194. Singh, J. Toxic Moulds and indoor air quality. Indoor Built Environ. (2005) 14:229–34. doi: 10.1177/1420326X05054015
195. Holý, O, Matoušková, I, Kubátová, A, Hamal, P, Svobodová, L, Jurásková, E, et al. Monitoring of microscopic filamentous Fungi in indoor air of transplant unit. Cent Eur J Public Health. (2015) 23:331–4. doi: 10.21101/cejph.a4062
197. Li, Y, Huang, X, Yu, ITS, Wong, TW, and Qian, H. Role of air distribution in SARS transmission during the largest nosocomial outbreak in Hong Kong. Indoor Air. (2005) 15:83–95. doi: 10.1111/j.1600-0668.2004.00317.x
198. Ijaz, MK, Zargar, B, Wright, KE, Rubino, JR, and Sattar, SA. Generic aspects of the airborne spread of human pathogens indoors and emerging air decontamination technologies. Am J Infect Control. (2016) 44:S109–20. doi: 10.1016/j.ajic.2016.06.008
199. Allen, JG, and Ibrahim, AM. Indoor air changes and potential implications for SARS-CoV-2 transmission. JAMA. (2021) 325:2112. doi: 10.1001/jama.2021.5053
200. Hayleeyesus, SF, Ejeso, A, and Derseh, FA. Quantitative assessment of bio-aerosols contamination in indoor air of university dormitory rooms. Int J Health Sci (Qassim). (2015) 9:249–56.
201. Johnson, ES, and Choi, K-M. Lung Cancer risk in Workers in the Meat and Poultry Industries - a review. Zoonoses Public Health. (2012) 59:303–13. doi: 10.1111/j.1863-2378.2012.01459.x
202. Hoppin, JA, Umbach, DM, Long, S, Rinsky, JL, Henneberger, PK, Salo, PM, et al. Respiratory disease in United States farmers. Occup Environ Med. (2014) 71:484–91. doi: 10.1136/oemed-2013-101983
203. Hendry, KM, and Cole, EC. A review of mycotoxins in indoor air. J Toxicol Environ Health. (1993) 38:183–98. doi: 10.1080/15287399309531711
204. Claeys, L, Romano, C, De Ruyck, K, Wilson, H, Fervers, B, Korenjak, M, et al. Mycotoxin exposure and human cancer risk: a systematic review of epidemiological studies. Compr Rev Food Sci Food Saf. (2020) 19:1449–64. doi: 10.1111/1541-4337.12567
205. Bray, GA, and Ryan, DH, Pennington Biomedical Research Center. Mycotoxins, cancer, and health. Baton Rouge: Louisiana State University Press (1991).
206. Jarvis, BB, and Miller, JD. Mycotoxins as harmful indoor air contaminants. Appl Microbiol Biotechnol. (2005) 66:367–72. doi: 10.1007/s00253-004-1753-9
207. Clark, HA, and Snedeker, SM. Ochratoxin a: its Cancer risk and potential for exposure. J Toxicol Environ Health B. (2006) 9:265–96. doi: 10.1080/15287390500195570
208. Verma, AK, and Prakash, S. Impact of COVID-19 on environment and society. J Glob Biosci. (2020) 9:7352–63.
209. Nazir, R, Ali, J, Rasul, I, Widemann, E, and Shafiq, S. Eco-environmental aspects of COVID-19 pandemic and potential control strategies. Int J Environ Res Public Health. (2021) 18:3488. doi: 10.3390/ijerph18073488
210. Mahato, S, Pal, S, and Ghosh, KG. Effect of lockdown amid COVID-19 pandemic on air quality of the megacity Delhi, India. Sci Total Environ. (2020) 730:139086. doi: 10.1016/j.scitotenv.2020.139086
211. Shrestha, AM, Shrestha, UB, Sharma, R, Bhattarai, S, Ngoc, H, Tran, T, et al. Lockdown caused by COVID-19 pandemic reduces air pollution in cities worldwide. EarthArXiv. (2020) 2020:4. doi: 10.31223/osf.io/edt4j
212. Ching, J, and Kajino, M. Rethinking air quality and climate change after COVID-19. Int J Environ Res Public Health. (2020) 17:5167. doi: 10.3390/ijerph17145167
213. Rani, R, Arokiasamy, P, and Sikarwar, A. Household air pollution during COVID-19 pandemic: a concern in India. J Public Aff. (2021) 21:e2729. doi: 10.1002/pa.2729
214. Du, W, and Wang, G. Indoor air pollution was nonnegligible during COVID-19 lockdown. Aerosol Air Qual Res. (2020) 20:1851–5. doi: 10.4209/aaqr.2020.06.0281
215. Zhang, Y, Zhao, B, Jiang, Y, Xing, J, Sahu, SK, Zheng, H, et al. Non-negligible contributions to human health from increased household air pollution exposure during the COVID-19 lockdown in China. Environ Int. (2022) 158:106918. doi: 10.1016/j.envint.2021.106918
216. Agarwal, N, Meena, CS, Raj, BP, Saini, L, Kumar, A, Gopalakrishnan, N, et al. Indoor air quality improvement in COVID-19 pandemic: review. Sustain Cities Soc. (2021) 70:102942. doi: 10.1016/j.scs.2021.102942
217. Lewis, D. Why the WHO took two years to say COVID is airborne. Nature. (2022) 604:26–31. doi: 10.1038/d41586-022-00925-7
218. Setti, L, Passarini, F, de Gennaro, G, Barbieri, P, Licen, S, Perrone, MG, et al. Potential role of particulate matter in the spreading of COVID-19 in northern Italy: first observational study based on initial epidemic diffusion. BMJ Open. (2020) 10:e039338. doi: 10.1136/bmjopen-2020-039338
219. Schijven, J, Vermeulen, LC, Swart, A, Meijer, A, Duizer, E, and de Roda Husman, AM. Quantitative microbial risk assessment for airborne transmission of SARS-CoV-2 via breathing, speaking, singing, coughing, and sneezing. Environ Health Perspect. (2021) 129:047002. doi: 10.1289/EHP7886
220. Riediker, M, and Tsai, D-H. Estimation of viral aerosol emissions from simulated individuals with asymptomatic to moderate coronavirus disease 2019. JAMA Netw Open. (2020) 3:e2013807. doi: 10.1001/jamanetworkopen.2020.13807
221. Hosseini, MR, Fouladi-Fard, R, and Aali, R. COVID-19 pandemic and sick building syndrome. Indoor Built Environ. (2020) 29:1181–3. doi: 10.1177/1420326X20935644
222. D’Alessandro, D, Gola, M, Appolloni, L, Dettori, M, Fara, GM, Rebecchi, A, et al. COVID-19 and living space challenge. Well-being and public health recommendations for a healthy, safe, and sustainable housing. Acta Biomed. (2020) 91:61–75. doi: 10.23750/abm.v91i9-S.10115
223. Ciric, L. Microbes in the built environment. Sci Rep. (2022) 12:8732. doi: 10.1038/s41598-022-12254-w
224. Smith, KR, Samet, JM, Romieu, I, and Bruce, N. Indoor air pollution in developing countries and acute lower respiratory infections in children. Thorax. (2000) 55:518–32. doi: 10.1136/thorax.55.6.518
225. Bruce, N, Perez-Padilla, R, and Albalak, R. The health effects of indoor air pollution exposure in developing countries (no. WHO/SDE/OEH/02.05). (2002). Available at: https://apps.who.int/iris/handle/10665/67496.
226. Jindal, SK, Aggarwal, AN, and Jindal, A. Household air pollution in India and respiratory diseases. Curr Opin Pulm Med. (2020) 26:128–34. doi: 10.1097/MCP.0000000000000642
227. Maharana, S, Paul, B, Garg, S, Dasgupta, A, and Bandyopadhyay, L. Exposure to indoor air pollution and its perceived impact on health of women and their children: a household survey in a slum of Kolkata, India. Indian J Public Health. (2018) 62:182–7. doi: 10.4103/ijph.IJPH_259_18
228. Roy, R, Jan, R, Joshi, U, Bhor, R, Pai, K, and Satsangi, PG. Characterization, pro-inflammatory response and cytotoxic profile of bioaerosols from urban and rural residential settings in Pune, India. Environ Pollut. (2020) 264:114698. doi: 10.1016/j.envpol.2020.114698
229. Central Pollution Control Board. National Ambient air Quality Status and Trends 2019 (NAAQMS/45/2019-2020). pp. 1–164. (2020).
230. Manivannan, S, Venugopal, V, Kindo, AJ, and Ravinder, T. Role of residential indoor dampness and mold as determinants of respiratory symptoms among adults. Indian J Public Health Res Dev. (2019) 10:91–7. doi: 10.5958/0976-5506.2019.01245.2
231. Dhar, DG, Dhar, P, Das, AK, and Uddin, N. Assessment of airborne Fungi in Childrens hospital located in Kolkata India. Def Life Sci J. (2021) 6:228–34. doi: 10.14429/dlsj.6.16731
232. Kumar, P, Singh, AB, and Singh, R. Seasonal variation and size distribution in the airborne indoor microbial concentration of residential houses in Delhi and its impact on health. Aerobiologia (Bologna). (2021) 37:719–32. doi: 10.1007/s10453-021-09718-3
233. Bali, R, Sharma, P, Nagrath, S, and Gupta, P. Microbial isolations from maxillofacial operation theatre and its correlation to fumigation in a teaching Hospital in India. J Maxillofac Oral Surg. (2012) 13:128–32. doi: 10.1007/s12663-012-0458-3
234. Naruka, K, and Gaur, J. Microbial air contamination in a school. Int J Curr Microbiol Appl Sci. (2013) 2:404–10.
235. Ghosh, B, Lal, H, Kushwaha, R, Hazarika, N, Srivastava, A, and Jain, VK. Estimation of bioaerosol in indoor environment in the university library of Delhi. Sustain Environ Res. (2013) 23:199–207.
236. Kumar, B, Prakash Gupta, G, Singh, S, and Kulshrestha, UC. Study of abundance and characterization of Culturable bioaerosol at Delhi, India. Int J Environ Eng Manage. (2013) 4:219–26.
237. Mohan, KNM, Ramprasad, S, and Maruthi, YA. Microbiological air quality of indoors in primary and secondary schools of Visakhapatnam, India. Int J Curr Microbiol Appl Sci. (2014) 3:880–7.
238. Paul, D, Biswas, K, Sengupta, C, and Narayan, SS. Studies on environmental monitoring of Aeromicroflora in a Hospital at Kalyani, West Bengal, India. Front Environ Microbiol. (2015) 1:47–50. doi: 10.11648/j.fem.20150103.13
239. Banerjee, B, Mukhopadhyay, C, Ke, V, Bupendra, A, and Varma, M. “News on air!” Air surveillance REPORT from intensive care units of a tertiary care hospital. Asian J Pharm Clin Res. (2016) 9:247. doi: 10.22159/ajpcr.2016.v9s3.14867
240. Meraj-Ul-Haque, BM, and Nitnawre, S. A study of fungal flora of house dust in Nagpur district, India. Mycopathologia. (2016) 14:43–5.
241. Anjum, KMR, Sivakumari, K, Ashok, K, and Rajesh, S. A pilot investigation into associations between various indoor airborne fungal particles in Triplicane public toilet of Chennai, Tamil Nadu. Int J Curr Pharm Res. (2017) 9:50–3. doi: 10.22159/ijcpr.2017v9i3.18891
242. Gaur, J, and Naruka, K. Assessment of airborne fungal pollution in a hospital room. Int Res J Biol Sci. (2017) 6:1–6.
243. Quadir, R, Chauhan, PP, and Sofi, R. Airborne microbial population in different health care units of Dehrad. Bio Bulletin. (2017) 3:8–13.
244. Kumar, H, Kaur, A, Kaur, B, Gupta, RK, Dhanjal, DS, Kaur, A, et al. Assessment of microbial contamination in indoor air of private maternity homes in Moga, Punjab. J Clin Diagn Res. (2018) 12:DC01–5. doi: 10.7860/JCDR/2018/34209.11480
245. Pawar, A, Dhapte, V, Kalrao, V, Godha, I, Jacob, J, and Raut, A. Profiling of microbial contamination in internal atmosphere of hospital Ward. Res Pediatr Neonatol. (2018) 2:531. doi: 10.31031/RPN.2018.02.000531
246. Priyamvada, H, Priyanka, C, Singh, RK, Akila, M, Ravikrishna, R, and Gunthe, SS. Assessment of PM and bioaerosols at diverse indoor environments in a southern tropical Indian region. Build Environ. (2018) 137:215–25. doi: 10.1016/j.buildenv.2018.04.016
247. Sharma, R, Sharma, S, and Rampal, RK. Comparative assessment of indoor air quality within office environments with reference to indoor biopollutants, suspended particulate matter and relative humidity. JK Knowl Initiat. (2018) 2:54–63.
248. Balyan, P, Ghosh, C, Das, S, and Banerjee, B. Spatio-temporal variations of indoor bioaerosols in different socio-economic zones of an urban Metropolis. Pol J Environ Stud. (2019) 28:4087–97. doi: 10.15244/pjoes/81272
249. Raksha, SG. Operation theaters: a source of nosocomial infection. Era’s J Med Res. (2019) 6:09–13. doi: 10.24041/ejmr2019.103
250. Valentina, Y, and Umadevi, S. Phenotypic detection and quality assessment of indoor air-borne microorganisms using passive air sampling technique (settle plate) at a tertiary care teaching Hospital in Puducherry. J Pure Appl Microbiol. (2019) 13:241–5. doi: 10.22207/JPAM.13.1.25
251. Kumari, H, Chakraborti, T, Singh, M, Chakrawarti, MK, and Mukhopadhyay, K. Prevalence and antibiogram of coagulase negative staphylococci in bioaerosols from different indoors of a university in India. BMC Microbiol. (2020) 20:211. doi: 10.1186/s12866-020-01875-8
252. Akila, M, Earappa, R, and Qureshi, A. Ambient concentration of airborne microbes and endotoxins in rural households of southern India. Build Environ. (2020) 179:106970. doi: 10.1016/j.buildenv.2020.106970
253. Kumar, P, Singh, AB, and Singh, R. Comprehensive health risk assessment of microbial indoor air quality in microenvironments. PLoS One. (2022) 17:e0264226. doi: 10.1371/journal.pone.0264226
254. Balasubramanian, R, Nainar, P, and Rajasekar, A. Airborne bacteria, fungi, and endotoxin levels in residential microenvironments: a case study. Aerobiologia (Bologna). (2012) 28:375–90. doi: 10.1007/s10453-011-9242-y
255. Szczotko, M, Orych, I, Mąka, Ł, and Solecka, J. A review of selected types of indoor air purifiers in terms of microbial air contamination reduction. Atmosphere (Basel). (2022) 13:800. doi: 10.3390/atmos13050800
256. Qian, H, and Zheng, X. Ventilation control for airborne transmission of human exhaled bio-aerosols in buildings. J Thorac Dis. (2018) 10:S2295–304. doi: 10.21037/jtd.2018.01.24
257. Nair, AN, Anand, P, George, A, and Mondal, N. A review of strategies and their effectiveness in reducing indoor airborne transmission and improving indoor air quality. Environ Res. (2022) 213:113579. doi: 10.1016/j.envres.2022.113579
258. Centers for Disease Control and Prevention. Ventilation in buildings. (2023). Available at: https://www.cdc.gov/coronavirus/2019-ncov/community/ventilation.html (Accessed October 16, 2023).
259. Walker, A. Natural Ventilation. National Renewable Energy Laboratory, Whole Building Design Guide. (2016). Available at: https://www.wbdg.org/resources/natural-ventilation (Accessed September 30, 2022).
260. Qian, H, Li, Y, Seto, WH, Ching, P, Ching, WH, and Sun, HQ. Natural ventilation for reducing airborne infection in hospitals. Build Environ. (2010) 45:559–65. doi: 10.1016/j.buildenv.2009.07.011
261. Luongo, JC, Fennelly, KP, Keen, JA, Zhai, ZJ, Jones, BW, and Miller, SL. Role of mechanical ventilation in the airborne transmission of infectious agents in buildings. Indoor Air. (2016) 26:666–78. doi: 10.1111/ina.12267
262. Escombe, AR, Oeser, CC, Gilman, RH, Navincopa, M, Ticona, E, Pan, W, et al. Natural ventilation for the prevention of airborne contagion. PLoS Med. (2007) 4:e68. doi: 10.1371/journal.pmed.0040068
263. Escombe, AR, Ticona, E, Chávez-Pérez, V, Espinoza, M, and Moore, DAJ. Improving natural ventilation in hospital waiting and consulting rooms to reduce nosocomial tuberculosis transmission risk in a low resource setting. BMC Infect Dis. (2019) 19:88. doi: 10.1186/s12879-019-3717-9
264. Vignolo, A, Gómez, AP, Draper, M, and Mendina, M. Quantitative assessment of natural ventilation in an elementary school classroom in the context of COVID-19 and its impact in airborne transmission. Appl Sci. (2022) 12:9261. doi: 10.3390/app12189261
265. Núñez, A, and García, AM. Effect of the passive natural ventilation on the bioaerosol in a small room. Build Environ. (2022) 207:108438. doi: 10.1016/j.buildenv.2021.108438
266. Atkinson, J, Chartier, Y, Pessoa-Silva, CL, Jensen, P, Li, Y, and Seto, W-H. Natural ventilation for infection control in health-care settings. 2nd ed. Geneva: World Health Organization (2009).
267. Dai, R, Liu, S, Li, Q, Wu, H, Wu, L, and Ji, C. A systematic review and meta-analysis of indoor bioaerosols in hospitals: the influence of heating, ventilation, and air conditioning. PLoS One. (2021) 16:e0259996. doi: 10.1371/journal.pone.0259996
268. Gołofit-Szymczak, M, and Górny, RL. Microbiological air quality in office buildings equipped with dventilation systems. Indoor Air. (2018) 28:792–805. doi: 10.1111/ina.12495
269. Lin, W-R, Ho, Y-H, Lee, WK, Cheng, H-M, and Wang, P-H. Spatiotemporal distribution and the passive dispersal of fungal spores through HVAC systems. Aerobiologia (Bologna). (2022) 38:13–21. doi: 10.1007/s10453-021-09730-7
270. Mousavi, ES, Kananizadeh, N, Martinello, RA, and Sherman, JD. COVID-19 outbreak and hospital air quality: a systematic review of evidence on air filtration and recirculation. Environ Sci Technol. (2021) 55:4134–47. doi: 10.1021/acs.est.0c03247
271. Fonseca, A, Abreu, I, Guerreiro, M, Abreu, C, Silva, R, and Barros, N. Indoor air quality and sustainability management–case study in three Portuguese healthcare units. Sustainability. (2018) 11:101. doi: 10.3390/su11010101
272. Chenari, B, Dias Carrilho, J, and Gameiro da Silva, M. Towards sustainable, energy-efficient and healthy ventilation strategies in buildings: a review. Renew Sust Energ Rev. (2016) 59:1426–47. doi: 10.1016/j.rser.2016.01.074
273. Vornanen-Winqvist, C, Salonen, H, Järvi, K, Andersson, M, Mikkola, R, Marik, T, et al. Effects of ventilation improvement on measured and perceived indoor air quality in a school building with a hybrid ventilation system. Int J Environ Res Public Health. (2018) 15:1414. doi: 10.3390/ijerph15071414
274. Azimi, P, and Stephens, B. HVAC filtration for controlling infectious airborne disease transmission in indoor environments: predicting risk reductions and operational costs. Build Environ. (2013) 70:150–60. doi: 10.1016/j.buildenv.2013.08.025
275. Song, L, Zhou, J, Wang, C, Meng, G, Li, Y, Jarin, M, et al. Airborne pathogenic microorganisms and air cleaning technology development: a review. J Hazard Mater. (2022) 424:127429. doi: 10.1016/j.jhazmat.2021.127429
276. Polidori, A, Fine, PM, White, V, and Kwon, PS. Pilot study of high-performance air filtration for classroom applications. Indoor Air. (2013) 23:185–95. doi: 10.1111/ina.12013
277. Medical Advisory Secretariat. Air cleaning technologies: an evidence-based analysis. Ont Health Technol Assess Ser. (2005) 5:1–52.
278. ANSI. ANSI/ASHRAE standard 52.2-2017. Method of testing general ventilation air-cleaning devices for removal efficiency by particle size. (2017). Available at: https://www.ashrae.org/technology.
279. Bastani, A, Lee, C-S, Haghighat, F, Flaherty, C, and Lakdawala, N. Assessing the performance of air cleaning devices–a full-scale test method. Build Environ. (2010) 45:143–9. doi: 10.1016/j.buildenv.2009.05.008
280. Navaratnam, S, Nguyen, K, Selvaranjan, K, Zhang, G, Mendis, P, and Aye, L. Designing post COVID-19 buildings: approaches for achieving healthy buildings. Buildings. (2022) 12:74. doi: 10.3390/buildings12010074
281. Xu, Z, Wu, Y, Shen, F, Chen, Q, Tan, M, and Yao, M. Bioaerosol science, technology, and engineering: past, present, and future. Aerosol Sci Technol. (2011) 45:1337–49. doi: 10.1080/02786826.2011.593591
282. de Almeida, DS, Martins, LD, and Aguiar, ML. Air pollution control for indoor environments using nanofiber filters: a brief review and post-pandemic perspectives. Chem Eng J Adv. (2022) 11:100330. doi: 10.1016/j.ceja.2022.100330
283. Shamim, JA, Hsu, W-L, and Daiguji, H. Review of component designs for post-COVID-19 HVAC systems: possibilities and challenges. Heliyon. (2022) 8:e09001. doi: 10.1016/j.heliyon.2022.e09001
284. Luo, H, and Zhong, L. Ultraviolet germicidal irradiation (UVGI) for in-duct airborne bioaerosol disinfection: review and analysis of design factors. Build Environ. (2021) 197:107852. doi: 10.1016/j.buildenv.2021.107852
285. de Souza, SO, Cardoso, AA Jr, Sarmento, ASC, and d’Errico, F. Effectiveness of a UVC air disinfection system for the HVAC of an ICU. Eur Phys J Plus. (2022) 137:37. doi: 10.1140/epjp/s13360-021-02240-y
286. Hsu, C-S, and Huang, D-J. Disinfection efficiency of chlorine dioxide gas in student cafeterias in Taiwan. J Air Waste Manage Assoc. (2013) 63:796–805. doi: 10.1080/10962247.2012.735212
287. Joubert, A, Abd Ali, SAZ, Frossard, M, and Andrès, Y. Dust and microbial filtration performance of regular and antimicrobial HVAC filters in realistic conditions. Environ Sci Pollut Res. (2021) 28:39907–19. doi: 10.1007/s11356-021-13330-w
288. Elsaid, AM, and Ahmed, MS. Indoor air quality strategies for air-conditioning and ventilation systems with the spread of the global coronavirus (COVID-19) epidemic: improvements and recommendations. Environ Res. (2021) 199:111314. doi: 10.1016/j.envres.2021.111314
289. Sánchez, B, Sánchez-Muñoz, M, Muñoz-Vicente, M, Cobas, G, Portela, R, Suárez, S, et al. Photocatalytic elimination of indoor air biological and chemical pollution in realistic conditions. Chemosphere. (2012) 87:625–30. doi: 10.1016/j.chemosphere.2012.01.050
290. Adhi, PM, Rahman, H, Nuriskasari, I, and Syamsudin, SA. A simple and low-cost photocatalytic air purification test method. J Phys Conf Ser. (2022) 2243:012075. doi: 10.1088/1742-6596/2243/1/012075
291. Kang, Y, and Kato, S. A study on the effectiveness of microwave heating for disinfection of humidifier elements. HVAC&R Res. (2014) 20:113–20. doi: 10.1080/10789669.2013.834780
292. Khurana, A. Ozone treatment for prevention of microbial growth in air conditioning systems. [Master’s Thesis]. Florida: University of Florida (2003).
293. Chan, DWT, Law, KC, Kwan, CHS, and Chiu, WY. Application of an air purification system to control air-borne bacterial contamination in a university clinic. HKIE Trans. (2005) 12:17–21. doi: 10.1080/1023697X.2005.10667988
294. Bolashikov, ZD, and Melikov, AK. Methods for air cleaning and protection of building occupants from airborne pathogens. Build Environ. (2009) 44:1378–85. doi: 10.1016/j.buildenv.2008.09.001
295. USDHHS, CDC. Guidelines for environmental infection control in health-care facilities recommendations of CDC and the healthcare infection control practices advisory committee (HICPAC), Atlanta. (2019). Available at: https://www.cdc.gov/infectioncontrol/guidelines/environmental/index.htm (Accessed August 25, 2022).
296. De Robles, D, and Kramer, SW. Improving indoor air quality through the use of ultraviolet Technology in Commercial Buildings. Proc Eng. (2017) 196:888–94. doi: 10.1016/j.proeng.2017.08.021
297. Zhong, L, and Haghighat, F. Ozonation air purification technology in HVAC applications. ASHRAE Trans (2014). AVailable at: https://link.gale.com/apps/doc/A371282980/AONE?u=anon~197f2a56&sid=googleScholar&xid=0f07a9a8 (Accessed October 16, 2023).
298. Haider, AJ, Sukkar, KA, Abdalsalam, AH, Ali, AF, Jaber, SH, and Ridha, TTA. Enhancement of the air quality and heat transfer rate of an air-conditioning system using a hybrid polypropylene nanofilter. Process Saf Environ Prot. (2021) 149:56–66. doi: 10.1016/J.PSEP.2020.10.041
299. Al-Rawi, M, Ikutegbe, CA, Auckaili, A, and Farid, MM. Sustainable technologies to improve indoor air quality in a residential house–a case study in Waikato, New Zealand. Energ Buildings. (2021) 250:111283. doi: 10.1016/j.enbuild.2021.111283
300. Kim, W, Ko, S, Oh, M, Choi, I, and Shin, J. Is an incentive policy for energy efficient products effective for air purifiers? Case South Korea Energies (Basel). (2019) 12:1664. doi: 10.3390/en12091664
301. Brągoszewska, E, and Biedroń, I. Efficiency of air purifiers at removing air pollutants in educational facilities: a preliminary study. Front. Environ Sci. (2021) 9:718. doi: 10.3389/fenvs.2021.709718
302. Cooper, E, Wang, Y, Stamp, S, Nijsen, T, de Graaf, P, Hofman, J, et al. Why do people use portable air purifiers? Evidence from occupant surveys and air quality monitoring in homes in three European cities. Build Res Inf. (2022) 50:213–29. doi: 10.1080/09613218.2021.2001303
303. Vijayan, V, Paramesh, H, Salvi, S, and Dalal, AK. Enhancing indoor air quality–the air filter advantage. Lung India. (2015) 32:473. doi: 10.4103/0970-2113.164174
304. Kujundzic, E, Matalkah, F, Howard, CJ, Hernandez, M, and Miller, SL. UV air cleaners and upper-room air ultraviolet germicidal irradiation for controlling airborne Bacteria and fungal spores. J Occup Environ Hyg. (2006) 3:536–46. doi: 10.1080/15459620600909799
305. Nguyen, TT, Johnson, GR, Bell, SC, and Knibbs, LD. A systematic literature review of indoor air disinfection techniques for airborne bacterial respiratory pathogens. Int J Environ Res Public Health. (2022) 19:1197. doi: 10.3390/ijerph19031197
306. Grinshpun, SA, Mainelis, G, Trunov, M, Adhikari, A, Reponen, T, and Willeke, K. Evaluation of ionic air purifiers for reducing aerosol exposure in confined indoor spaces. Indoor Air. (2005) 15:235–45. doi: 10.1111/j.1600-0668.2005.00364.x
307. Jakober, C, and Phillips, T. Evaluation of ozone emissions from portable indoor air cleaners: Electrostatic precipitators and ionizers. Sacramento, CA. (2008). Available at: http://www.arb.ca.gov/research/indoor/ozone.htm.
308. Riaz, N, Khan, MS, Bilal, M, Ullah, S, and Al-Sehemi, AG. Photocatalytic inactivation of bioaerosols: a short review on emerging technologies. Curr Anal Chem. (2020) 17:31–7. doi: 10.2174/1573411016999200729115254
309. Ruwan Jayakantha, DNP, Bandara, HMN, Gunawardana, NM, Jayantha Rajapakse, RPV, Thilakarathne, DS, Comini, E, et al. Design and construction of a low cost air purifier for killing harmful airborne microorganisms using a combination of a strong multi-directional electric-field and an ultra violet light. HardwareX. (2022) 11:e00279. doi: 10.1016/j.ohx.2022.e00279
310. Luengas, A, Barona, A, Hort, C, Gallastegui, G, Platel, V, and Elias, A. A review of indoor air treatment technologies. Rev Environ Sci Biotechnol. (2015) 14:499–522. doi: 10.1007/s11157-015-9363-9
311. González-Martín, J, Kraakman, NJR, Pérez, C, Lebrero, R, and Muñoz, R. A state–of–the-art review on indoor air pollution and strategies for indoor air pollution control. Chemosphere. (2021) 262:128376. doi: 10.1016/j.chemosphere.2020.128376
312. Kaushik, AK, and Dhau, JS. Photoelectrochemical oxidation assisted air purifiers; perspective as potential tools to control indoor SARS-CoV-2 exposure. Appl Surf Sci Adv. (2022) 9:100236. doi: 10.1016/j.apsadv.2022.100236
313. USEPA. Mold cleanup in your home. (2023). Available at: https://www.epa.gov/mold/mold-cleanup-your-home (Accessed October 16, 2023).
Keywords: indoor environments, indoor air quality, public health, bioaerosols, microbial diversity
Citation: Chawla H, Anand P, Garg K, Bhagat N, Varmani SG, Bansal T, McBain AJ and Marwah RG (2023) A comprehensive review of microbial contamination in the indoor environment: sources, sampling, health risks, and mitigation strategies. Front. Public Health. 11:1285393. doi: 10.3389/fpubh.2023.1285393
Received: 29 August 2023; Accepted: 25 October 2023;
Published: 23 November 2023.
Edited by:
Jianbang Xiang, Sun Yat-sen University, ChinaReviewed by:
Gaetano Settimo, Istituto Superiore di Sanità, ItalyCopyright © 2023 Chawla, Anand, Garg, Bhagat, Varmani, Bansal, McBain and Marwah. This is an open-access article distributed under the terms of the Creative Commons Attribution License (CC BY). The use, distribution or reproduction in other forums is permitted, provided the original author(s) and the copyright owner(s) are credited and that the original publication in this journal is cited, in accordance with accepted academic practice. No use, distribution or reproduction is permitted which does not comply with these terms.
*Correspondence: Ruchi Gulati Marwah, cnVjaGkuZ3VsYXRpQGJjYXMuZHUuYWMuaW4=
‡ORCID: Andrew J. McBain, https://orcid.org/0000-0002-5255-5425
Ruchi Gulati Marwah, https://orcid.org/0000-0001-8945-7055
†These authors have contributed equally to this work and share first authorship
Disclaimer: All claims expressed in this article are solely those of the authors and do not necessarily represent those of their affiliated organizations, or those of the publisher, the editors and the reviewers. Any product that may be evaluated in this article or claim that may be made by its manufacturer is not guaranteed or endorsed by the publisher.
Research integrity at Frontiers
Learn more about the work of our research integrity team to safeguard the quality of each article we publish.