- Institute of Epidemiology and Health Statistics, School of Public Health, Lanzhou University, Lanzhou, China
Background: The association between exposure to trace elements mixture and the prevalence of kidney stones and the interactions between elements are unclear. The aim of this study was to explore the association between exposure to trace elements mixture and the prevalence of kidney stones and the interactions between the elements.
Methods: A total of 1,244 participants (139 kidney stone formers and 1,105 non-stone former participants) in NHANES 2017–2018 were included. The exposure to trace elements was evaluated by measuring their concentration in urine samples. Three methods, Logistic regression, quantile-based g computation (qgcomp), and Bayesian kernel machine regression (BKMR), were used for analysis.
Results: According to the results from qgcomp and BKMR, a negative association was found between exposure to the 13 trace elements and the prevalence of kidney stones [OR = 0.50 (0.32, 0.78)]. Subgroup analysis revealed that Co, As, and iodine in the whole population, Co, As, and Ni in males, and Cs, iodine, and Sb in females, were most strongly associated with kidney stones. Kidney stone was found to be positively correlated with Co and negatively correlated with the other elements. Besides, there were significant interactions between Ni and Pb in the whole population, Co and iodine in males, and Pb and iodine in females.
Conclusion: There was a negative association between exposure to the mixture of 13 trace elements and the prevalence of kidney stones.
1. Introduction
Kidney stones are widely prevalent, and the prevalence of kidney stones has shown an increasing trend over the years worldwide. The self-reported prevalence of kidney stones among adults aged 20 years and older in the United States was 3.8, 5.2% (1), 8.8% (2), and 11.0% (3) in 1976–1980, 1988–1994, 2007–2010, and 2015–2018, respectively. From 1976 to 2018, the prevalence increased nearly threefold. The incidence of kidney stones ranges from 114 to 720 per 100,000 persons, with a prevalence of 1.7 to 14.8%, and the incidence is rising in almost all nations, according to a retrospective study of epidemiologic data from seven countries (4). While kidney stones do not directly raise mortality rates in the general population (5), they are linked with excruciating pain, are prone to recurrence (6), and increase the risk of myocardial infarction in people who have them (7). In addition, in overweight people, kidney stones may also lead to renal impairment (8). All of the evidence above suggests that kidney stones need to be taken seriously.
Many factors contribute to the development of kidney stones, among which nutritional factors are very important (9). Reduced dietary calcium (Ca) intake (10, 11) and decreased urine output caused by insufficient water intake (12) are significant risk factors for kidney stone development, according to prior research. In addition to macronutrients such as calcium, which have an impact on the risk of kidney stones, exposure to many trace elements may also have an impact on kidney stones, for example, elevated dietary zinc (Zn) intake may be a protective factor for the risk of kidney stones (13, 14), and there may be an association between exposure concentrations of heavy metals such as cadmium (Cd), lead (Pb), arsenic (As), mercury (Hg), trimethyltin and the risk of kidney stones (15–18). The precise relationship between kidney stones and trace elements is unclear, due to discrepancies between recent studies on the topic. Human exposure to numerous trace elements is diverse and complicated, but prior research has only looked at a few hazardous trace elements, ignoring other less toxic or beneficial elements or mixed exposures to several trace elements, which may not accurately reflect actual exposure.
This study was based on data from the National Health and Nutrition Examination Survey (NHANES) 2017–2018. The impact of single trace elements was analyzed using multivariable logistic regression, and quantile-based g computation (qgcomp) and Bayesian kernel machine regression (BKMR) were applied to investigate the roles of trace elements co-exposure in kidney stones and the interactions between the trace elements.
2. Materials and methods
2.1. Study population
All study subjects in this study were from NHANES, an ongoing cross-sectional survey of nationally representative samples of the US civilian non-institutionalized population while using a complex multi-stage sampling method. The study analyzed data from the 2017–2018 NHANES cycle because urine nickel (Ni) was detected only in this cycle.
This cross-sectional study was performed under the Strengthening the Reporting of Observational Studies in Epidemiology (STROBE) guidelines (Supplementary Table S1). Participants were eligible if they met the following inclusion criteria: (1) aged above 20 years and (2) had available kidney stone status, urinary trace element measurements and all covariates. Figure 1 depicts a detailed flowchart of the selection process for eligible participants. Ultimately, 1,244 participants were enrolled in the study.
2.2. Assessment of kidney stones
Information on kidney stones was collected by the NHANES questionnaire. All adults older than 20 years were asked “Have you ever had kidney stones?” Subjects who answered “yes” were considered to be kidney stone patients.
2.3. Trace element measurement
The detection results of a total of 13 trace elements in urine were obtained from the 2017–2018 cycle of the NHANES. The urinary trace elements include Ni, molybdenum (Mo), thallium (Tl), Pb, barium (Ba), cobalt (Co), cesium (Cs), antimony (Sb), tin (Sn), tungsten (Tu), As, iodine (I), and Cd. Iodine was measured by inductively coupled plasma dynamic reaction cell mass spectrometry (ICP-DRC-MS) and the remaining elements were measured by inductively coupled plasma mass spectrometry (ICP-MS). For concentrations below the limit of detection (LOD), we used an imputed value of LOD/ according to the NHANES standard. The concentrations of metals in urine were all corrected by urinary creatinine in our analysis and were expressed as μg/g creatinine.
2.4. Covariates
According to previous studies, the following variables were included as covariates (19, 20): age, gender (male or female), race/ethnicity (Mexican American, Other Hispanic, non-Hispanic white, non-Hispanic black, other race/multiracial), education (under high school, high school or equivalent, above high school), the ratio of family income to poverty (PIR), marital status (married/cohabiting, widowed/divorced/separated, never married), drinking alcohol status (never, ever), body mass index (BMI), serum cotinine, and intakes of total energy, calcium, phosphate, sodium, potassium, magnesium, total fluid, alcohol, caffeine, and vitamins B6, C, and D. All covariates were collected by questionnaires (both demographic and dietary questionnaires) except for serum cotinine, which was measured by an isotope-dilution high-performance liquid chromatography-mass spectrometric (ID HPLC-MS) method. The average intakes of the above dietary factors from the two 24-h recalls were used in this analysis, or only the first day’s 24-h recall was applied if the second day’s recall was not available.
2.5. Statistical analysis
The statistical analysis accounted for the complex sample design of NHANES (21), using SPSS (version 26) and R (version 4.2.1), and Subsample A weight (WTSA2YR), which was the measurement weight of the urinary metal, was used as sample weight (22). For continuous variables, the Shapiro–Wilk test was used to explore whether the variables conformed to a normal distribution. Variables that conformed to a normal distribution were described by the mean ± SD, and those that did not conform to a normal distribution were described by the median [P25, P75]. Categorical variables were presented as No. (%). The urinary trace element concentrations were natural logarithm (Ln)-transformed, and Spearman correlation analysis was used to examine the correlations between each urinary trace element concentration. Subgroup analysis was stratified by gender.
Urinary trace element concentrations were divided into four quartiles (Q1, Q2, Q3, and Q4). The relationships between single elements and kidney stones were estimated by survey-weighted multivariable logistic regression, shown as odds ratios (OR) and 95% confidence intervals (CI) [OR, (95%CI)]. A linear trend test was performed with the median value of each quartile treated as a continuous variable in the models.
Qgcomp can be used to assess the overall effect of multiple exposures on outcomes and to identify primary exposures (23). Qgcomp indices (ranging from-1 to 1) can be used to assess the strength and direction of the effect of trace elements mixture exposure on the odds of kidney stones. In addition, the relative weights of positive or negative associations between each trace element and kidney stones can be obtained using this method. However, the absolute values of relative weights are not directly comparable between positive and negative effects. The absolute weights of each trace element were obtained by multiplying each relative weight with the importance parameter of the positive/negative effect, and can be used to compare the importance of all elements on the effect of kidney stones. The qgcomp analysis was performed using the “qgcomp” package in R.
Given the potential non-linearity and non-additive dose–response relations among mixture exposure, BKMR was used to assess the joint effect of all trace elements on kidney stones (24). This method integrates Bayesian and statistical learning methods to estimate the nonlinear and/or interactions in the exposure-outcome association. Analyses were conducted using the bkmr package in R statistical software (25). We implemented the BKMR variable selection model with 50,000 iterations using a Markov chain Monte Carlo algorithm. We analyzed the dose–response relationships of single trace elements and the mixed exposure effects using BKMR. We examined whether there were two-way interactions among the primary exposures. We estimated an interactive effect as the difference in the first exposure health effect when the second exposure was fixed at the 90th percentile compared to fixing at the 10th percentile, and the remaining exposures were fixed to their median values. Due to the complexity of qgcomp and BKMR and the limitations of the survey package, we did not consider weights in our analysis with these two methods.
In this study, p < 0.05 was considered statistically significant.
Besides, multivariate imputation by chained equations (MICE) was applied to all missing covariates to test the bias of omitting observations with missing values by qgcomp.
3. Results
3.1. Basic characteristics and trace elements distribution
Among the 1,244 participants from the 2017–2018 NHANES, 139 (11.17%) were diagnosed with kidney stones. Table 1 presents the basic characteristics of adults with or without kidney stones in the United States.
The detection rates, median and interquartile range of each trace element are shown in Supplementary Table S2, and the detection rates of all elements were higher than 75%. Spearman correlation analysis (Supplementary Figure S1) revealed a strong correlation between Tl and Cs (r = 0.64). Moderate correlations were observed between Co and Ni (r = 0.51), Co and Ba (r = 0.49), and Pb and Cd (r = 0.43). There were only minor relationships between the other urine trace components (all r < 0.4).
3.2. Logistic regression results
Table 2 presents the survey-weighted logistic regression results. After dividing the data into four quartiles, it was found that compared with the first quartile (Q1) of each element, Mo [Q2: OR = 2.11 (1.09, 4.10)], Ba [Q2: OR = 3.19 (1.56, 6.51); Q4: OR = 2.50 (1.41, 4.44)], Co [Q2: OR = 4.22 (1.53, 11.68); Q3: OR = 3.69 (1.59, 8.58)] and Sn [Q2: OR = 1.73 (1.02, 2.95)] were positively associated with the odds of kidney stones. While Tl [Q4: OR = 0.19 (0.06, 0.59)], Cs [Q2: OR = 0.65 (0.43, 0.99); Q4: OR = 0.30 (0.15, 0.59)], As [Q2: OR = 0.45 (0.22, 0.94); Q3: OR = 0.49 (0.25, 0.98)], and iodine [Q4: OR = 0.32 (0.12, 0.85)] were negatively associated with the odds of kidney stones when these trace elements were present at certain exposure levels. Besides, the results of the trend test were significant on the association between Ba, Co, and Cs, and the odds of kidney stones (p < 0.05).
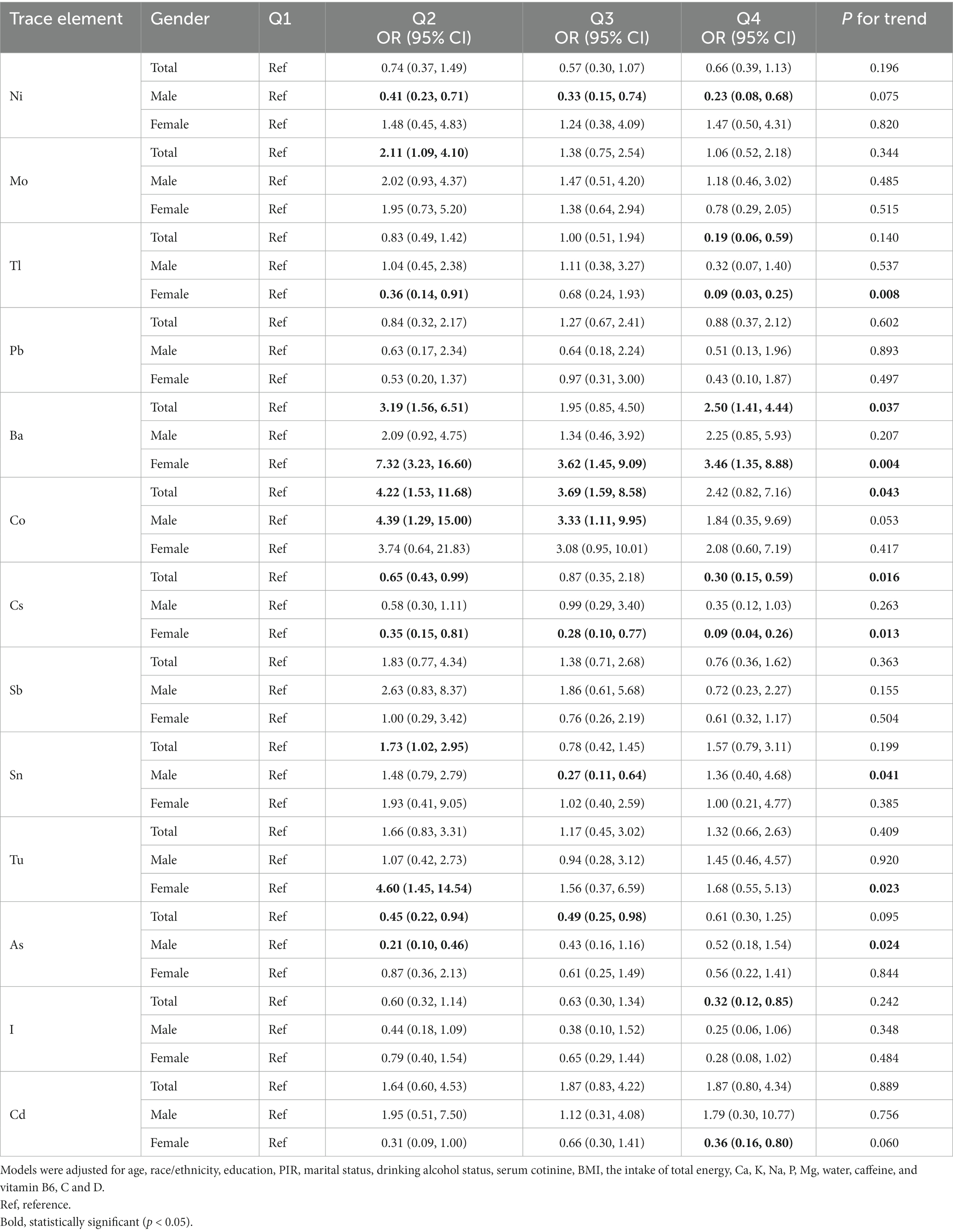
Table 2. Associations of single urinary trace elements with kidney stones and stratified by gender, NHANES, 2017–2018.
Subgroup analysis by gender is also displayed in Table 2. In males, after dividing trace element concentrations into quartiles, Ni [Q2: OR = 0.41 (0.23, 0.71); Q3: 0.33 (0.15, 0.74); Q4: 0.23 (0.08, 0.68)], Sn [Q3: OR = 0.27 (0.11, 0.64)], and As [Q2: OR = 0.21 (0.10, 0.46)] were found to be negatively associated with kidney stones at certain exposure levels, and Co was found to be positively associated with the odds of kidney stones [Q2: OR = 4.39 (1.29, 15.00); Q3: OR = 3.33 (1.11, 9.95)]. The results of the trend test were significant on the association between Sn, and As, and the odds of kidney stones (p < 0.05).
In women, it was found that Ba [Q2: OR = 7.32 (3.23, 16.60); Q3: OR = 3.62 (1.45, 9.09); Q4: OR = 3.46 (1.35, 8.88)] and Tu [Q2: OR = 4.60 (1.45, 14.54)] were risk factors for kidney stones, and Tl [Q2: OR = 0.36 (0.14, 0.91); Q4: OR = 0.09 (0.03, 0.25)], Cs [Q2: OR = 0.35 (0.15, 0.81); Q3: OR = 0.28 (0.10, 0.77); Q4: OR = 0.09 (0.04, 0.26)], and Cd [Q4: OR = 0.36 (0.16, 0.80)] were negatively associated with kidney stones when these trace elements were present at certain exposure levels. The results of the trend test were significant on the association between Tl, Ba, Cs, and Tu, and the odds of kidney stones (p < 0.05) (Table 2).
3.3. Analysis of the association of trace elements co-exposure and kidney stones by the qgcomp model
As shown in Figure 2A, the OR of the trace elements was negatively associated with kidney stones in all [0.50 (0.32, 0.78)], male [0.51 (0.28, 0.93)] and female participants [0.54 (0.30, 0.93)], p < 0.05. In the whole population, the highest weight was given to Co, which was positively associated with the odds of kidney stones, followed by iodine and As, both of which were negatively associated with the odds of kidney stones (Figure 2B). In males, the trace element most significantly associated with kidney stones was Co, with a positive association, followed by As and Ni, both of which were negatively associated with the odds of kidney stones (Figure 2C). In females, the most significantly associated trace elements with kidney stones were Cs, Sb, and iodine, which were all negatively associated with the odds of kidney stones, followed by Ba, which was positively associated with the odds of kidney stones (Figure 2D).
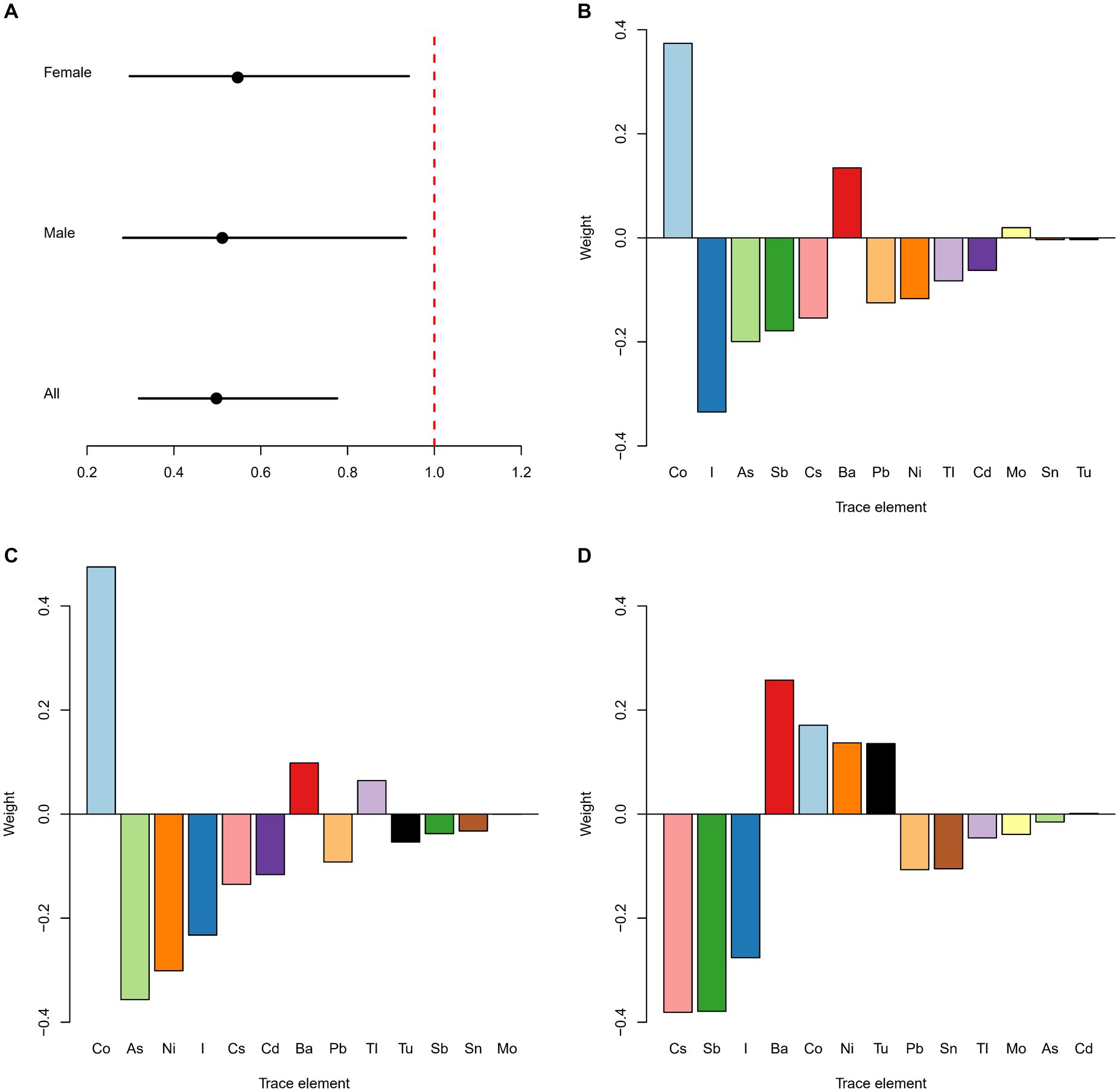
Figure 2. Estimated OR and weighted values of trace elements for kidney stones by qgcomp models. Associations of urinary trace elements with kidney stones odds in total population and different subgroups (A). Weighted values of urinary trace elements for kidney stones in qgcomp models for total population (B), males (C) and females (D). Models (total) were adjusted for gender, age, race/ethnicity, education, PIR, marital status, drinking alcohol status, serum cotinine, BMI, the intake of total energy, Ca, K, Na, P, Mg, water, caffeine, and vitamin B6, C and D.
3.4. Assessment of trace elements co-exposure effects and interactions using the BKMR model
Table 3 shows the posterior inclusion probability (PIP) values for the effect of each trace element on kidney stones and Figure 3 and Supplementary Figures S2, S3 show the dose–response relationship of each trace element in the association with kidney stones. These graphs show that the trace elements with the highest PIP values (i.e., the highest contribution to kidney stones prevalence) in the overall population were Co (0.82), iodine (0.81) and As (0.66). The prevalence of kidney stones increased with rising concentrations and subsequently declined after reaching a specific threshold, forming an “inverted U-shaped” association between Co exposure and odds of kidney stones; in contrast, iodine and As exposure showed a “U-shaped” curve with the prevalence of kidney stones (Figure 3).
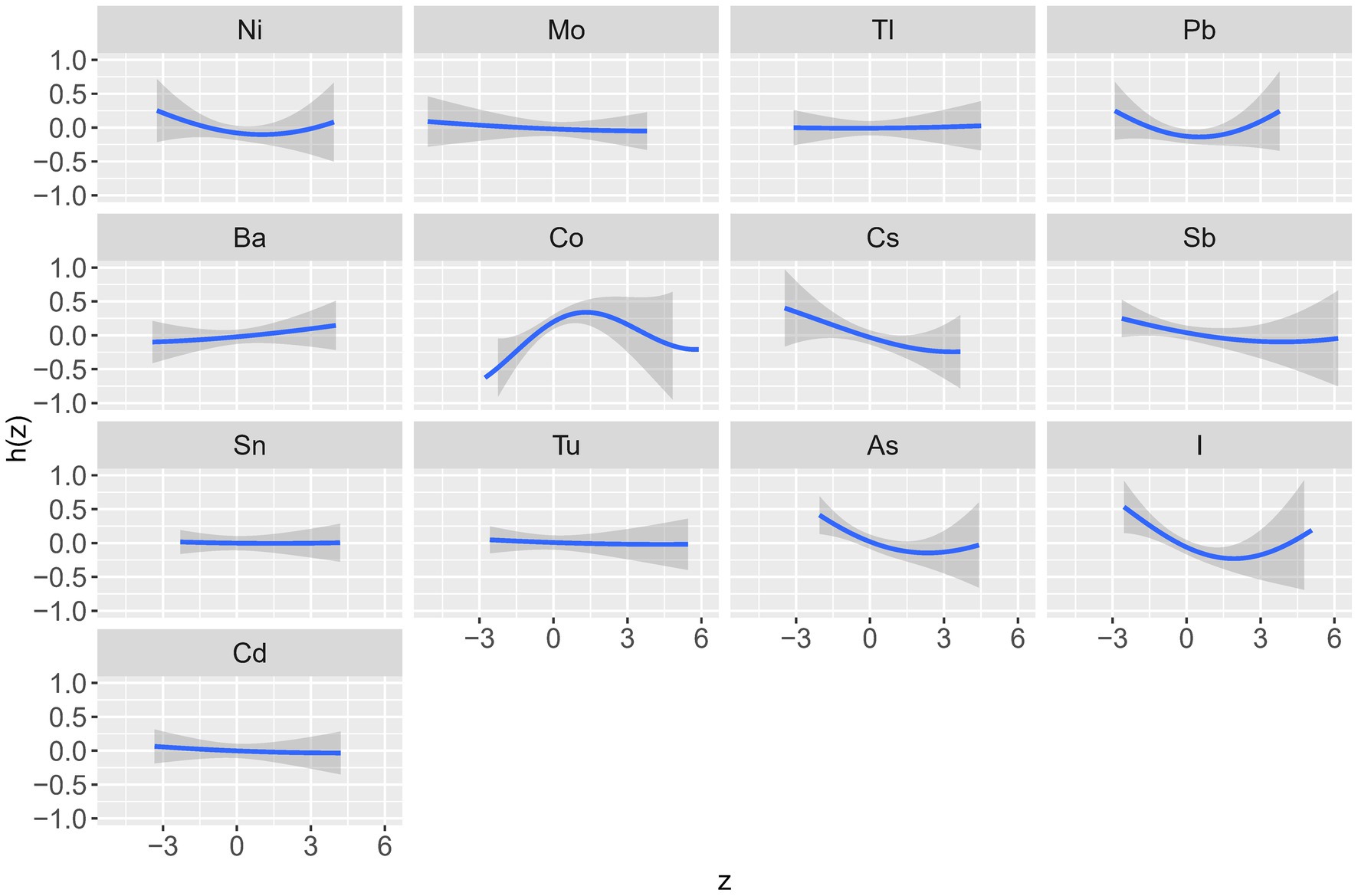
Figure 3. Univariate exposure-response functions for each trace element with other elements fixed at the median (total). The results were assessed by the Bayesian Kernel machine regression (BKMR) models. Models were adjusted for sex, age, race/ethnicity, education, PIR, marital status, drinking alcohol status, serum cotinine, BMI, the intake of total energy, Ca, K, Na, P, Mg, water, caffeine, and vitamin B6, C and D.
In males, the trace elements with the highest PIP values were As (0.82), Ni (0.47), and Co (0.41) (Table 3). The relationship between Co exposure and the odds of kidney stones showed an “inverted U-shaped” curve; the relationship between As exposure and the odds of kidney stones showed a “U-shaped” curve; and the relationship between Ni exposure and the odds of kidney stones showed a negative relationship (Supplementary Figure S2).
In females, the trace elements with the highest PIP values were Cs (0.66), I (0.65), and Sb (0.40), and all three exposure concentrations were negatively correlated with the odds of developing kidney stones (Supplementary Figures S3).
Figure 4 shows the overall association of the trace elements mixture with the odds of kidney stones. In all participants and the two subgroups, the overall exposure-response function indicated a significant and negative association of trace elements with kidney stones.
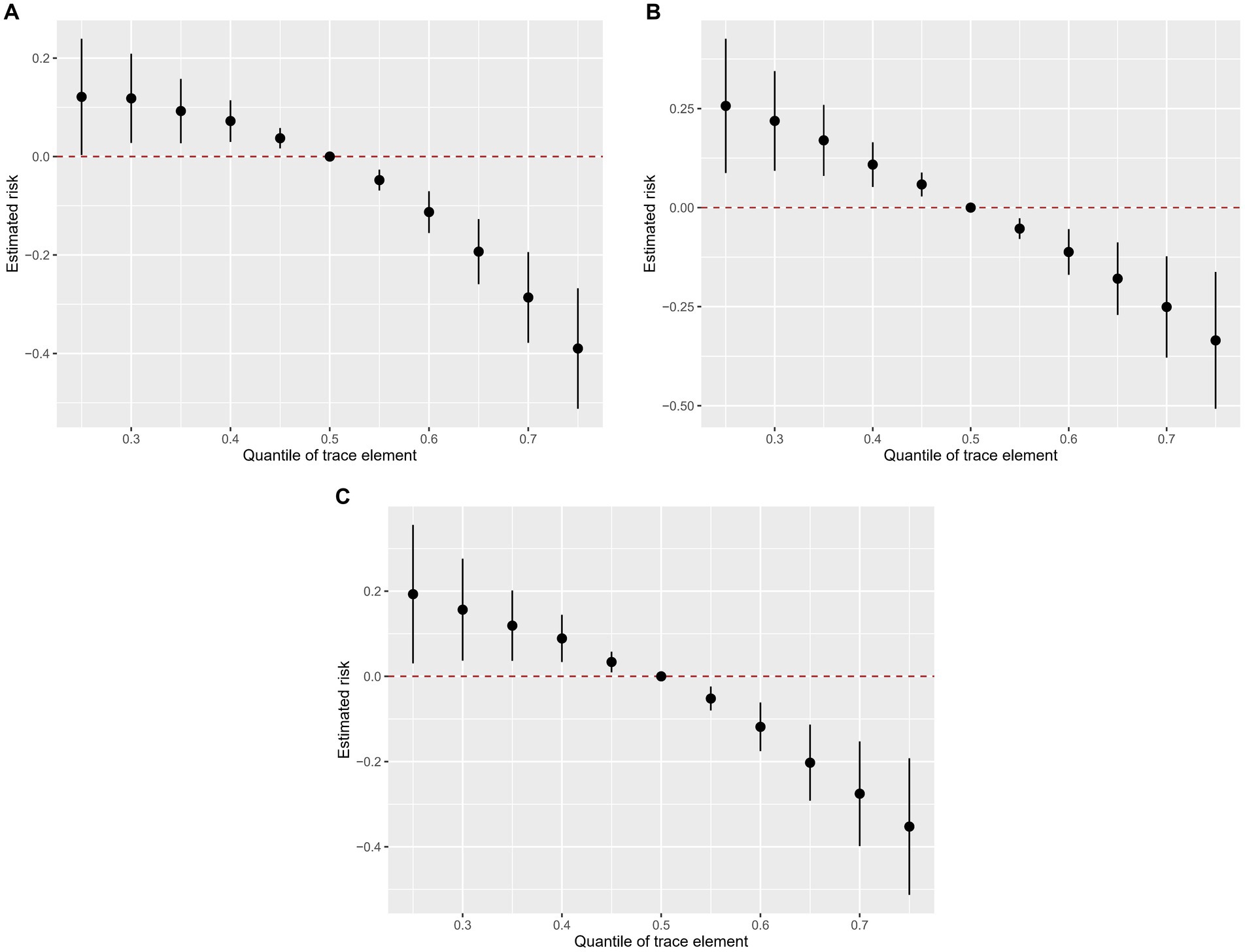
Figure 4. Combined effects of urinary trace elements mixture on kidney stones were estimated by BKMR models. (A) total population, (B) male subgroup, (C) female subgroups. Models (total) were adjusted for gender, age, race/ethnicity, education, PIR, marital status, drinking alcohol status, serum cotinine, BMI, the intake of total energy, Ca, K, Na, P, Mg, water, caffeine, and vitamin B6, C and D. This plot shows the estimated difference in kidney stones and 95%CI when all trace elements concentrations were held at particular percentiles compared to their medians.
In addition, the two-way interactions of trace elements were assessed, and 4–6 trace elements with high PIP values in each group were selected for inclusion in the two-way interactions analysis. There was a positive interaction between Ni and Pb in the whole population. The effect of Pb on kidney stones was more significant when Ni was at the higher percentile and vice versa (Supplementary Figure S4). In males, there was a negative interaction between Co and iodine. When iodine was at the higher percentile, the effect of Co on kidney stones was even less significant (Supplementary Figure S5). In females, there was an interaction between Pb and iodine. When Pb was at the higher percentile, the effect of iodine on kidney stones was more pronounced. When iodine was at the lower percentile, the association between Pb and kidney stones was positive, and as the iodine percentile increased, the association between Pb and kidney stones gradually became an “inverted U-shaped” curve (Supplementary Figure S6).
3.5. Sensitivity analysis
The results of sensitivity analysis are shown in Supplementary Figure S7, and the results did not substantially change after multiple imputation to all missing covariates. The OR of the trace elements was still negatively associated with kidney stones in all participants [OR = 0.57 (0.39, 0.81)]. The trace elements with higher weights are still Co, iodine, and As.
4. Discussion
This study analyzed the association between mixed exposure to multiple trace elements and the prevalence of kidney stones, and the interactions between elements. Combining the results of the three methods (logistic regression, qgcomp, and BKMR), we found that trace elements mixture exposure was negatively associated with the odds of kidney stones in the whole population and both male and female subgroups. Among the 13 trace elements, the elements that played the most significant role in the odds of kidney stones in the whole population were Co, iodine, and As, with a positive correlation for Co and a negative correlation for iodine and As. In males, the trace elements that played the most significant roles were As, Ni and Co, with a positive correlation for Co and negative correlations for As and Ni. In females, the trace elements that played the most important roles were Cs, iodine, and Sb, all of which were negatively correlated with the odds of kidney stones. In addition, there were interactions between iodine, Pb, and other trace elements mixtures, and two-way interactions between some trace elements.
Trace elements are ubiquitous, they exist in soil, water, air and food. The human body is constantly exposed to trace elements as a result of contact with these environmental media (26). Diseases, such as cancer and metabolic diseases may be related to excess or deficiency of trace elements (27). Excretion through the kidneys is the main way that trace elements are eliminated from the body (28). In this process, trace elements are incorporated into a urinary stone and can affect its properties (29). Trace elements also widely exist in various kidney stones. One study analyzed 31 different trace elements in 24 kidney stones using atomic absorption and found that calcium stones mainly contained iron (Fe), Pb, strontium (Sr), and zinc (Zn), and organic stones mainly contained As, Fe, and Zn (30), which means that those trace elements have an important influence on the formation of kidney stones. Zhao et al. have analyzed the associations of exposure to heavy metal mixtures with kidney stone. They got a positive association between the two (31), which was different from the result in our research. The reason for this contrast may be that their study included more toxic heavy metals like mercury, for example, while our study included potentially beneficial trace elements like iodine (which was also formalized in our study).
There have been more investigations on the link between As and Pb and kidney stones among the essential trace elements mentioned above. The toxicodynamics of As-induced kidney toxicity in experimental studies have been summarized elsewhere (32, 33). In terms of epidemiology, a previous study found a negative association between total urinary As and the risk of kidney stones in males, similar to the results of our study (17); in females, elevated As concentrations increased the risk of kidney stones (16), but our study did not find an association between the two in females, which may be related to the different number of mixed exposures in the two studies. In conclusion, there are significant differences between males and females in the correlation between kidney stones and urinary As exposure, and the specific mechanisms remain to be further elucidated.
Numerous studies on Pb have concentrated on how it affects renal function (34–36). Both modest and high levels of Pb exposure cause patients’ glomerular filtration rates to drastically decrease. Thus, the negative effects of Pb exposure on renal function are widely recognized and are supported by a large body of evidence. Regarding the association between Pb and kidney stones, there are contradictions in the existing studies. A cross-sectional survey of 1,293 Chinese participants found that high Pb exposure was positively associated with the risk of kidney stones in males and that co-exposure to Pb and Cd was also associated with the formation of kidney stones (37). In a prospective cohort study of 1,302 participants in Belgium, low-level Pb exposure was found to be associated with kidney stone formation (38). Similar findings to our analysis were reported in another investigation utilizing NHANES data: a “U-shaped” curve at higher levels of exposure and a negative correlation between Pb and kidney stone prevalence at lower levels of exposure. The reason for this phenomenon may be related to the different sensitivity to Pb in different ethnic groups, and further prospective studies are needed to figure out the relationship between Pb exposure and kidney stones in the US population.
In this study, Co was found to be strongly positively associated with kidney stone, which was similar to the previous study (31). However, analysis of the composition of trace elements in kidney stones revealed that the levels of Co in various kidney stones were very low, mostly below the detection limit (39). It indicates that Co may not be mainly involved in the composition of kidney stones, but promotes the formation of kidney stones through other pathways. It has been shown that cobalt may contribute to kidney stones by decreasing glomerular filtration rate (36) and causing acute kidney injury (40). But its specific mechanism still needs further study.
In addition, Cd was found to be significantly associated with kidney stones in previous studies, and most original studies and reviews have found a positive association between Cd exposure and kidney stones (15, 41, 42). However, the association between Cd and kidney stones occurs mostly in occupationally exposed populations (41), and dietary Cd exposure is unrelated to the prevalence of kidney stones (19). Other studies have found an “inverted U-shaped” curve relationship between the two (16, 17). However, no significant association was found between the two in this study, probably because we included more micronutrients and the different elements may interact with each other.
The results of this study also showed that iodine, Ni, and Cs all have important effects on kidney stones. However, there are few studies on the effects of these trace elements on kidney stones. Urinary Ni excretion was significantly lower in patients with active kidney stones compared to patients with previous kidney stones and healthy individuals who had never had kidney stones (43). Some Ni particles were also found in kidney stones (44), and Ni may inhibit the crystallization process of calcium oxalate (45, 46). All these results indicate the importance of Ni in the pathogenesis of new kidney stone formation. A study using NHANES 2011–2016 data found an interaction between Cs and manganese (Mn) in terms of their effects on kidney function (47). Few studies have examined the effects of iodine and Cs on kidney stones, but in our study, we found a negative association between iodine exposure and the odds of kidney stones in the whole population, and in both male and female subgroups; Cs was also negatively associated with the prevalence of kidney stones in females. We believe these findings warrant further prospective cohort studies.
Additionally, this study investigated the interactions of trace elements on the odds of kidney stones. Interactions were found between iodine and Pb and other trace elements mixture, and two-way interactions were found between many trace elements, such as Ni and Pb (whole population), Co and iodine (male), and Pb and iodine (female). However, we judged the interactions only by graphs and lack of interaction parameters, which can lead to subjective misjudgment. Other more suitable methods could be chosen to further investigate the interactions of trace elements on kidney stones specifically.
In subgroup analysis, different elements affecting differences between females and males. The reason might be the compositions of kidney stones are different between males and females. Compared to men, women have a lower proportion of CaOx stones and uric acid (UA) stones and a higher proportion of hydroxyapatite (HA) in kidney stones (48, 49). The amount of trace elements contained in different types of kidney stones is also different and it was statistically significant (39). Besides, the difference might be mediated through sex hormones. Different trace elements had different effects on the production of sex hormones in men or women (50), and changes in sex hormones could further promote or inhibit the development of kidney stones (51). The root reason needs further mechanistic researches.
Our study has some strengths. Firstly, 3 methods, logistic regression, qgcomp, and BKMR, were used to analyze the effect of trace elements on kidney stones. Secondly, all urinary trace element concentrations available in the NHANES database with a detection rate > 70% were included in the analysis, which is similar to real human exposure. Thirdly, the impact of each trace element on kidney stones was in the same direction by the three methods and the results were similar through the three different methods, especially in the elements with higher contributions to kidney stone. Fourthly, the three methods produced generally consistent results, demonstrating the robustness of the findings.
Inevitably, this study also has some limitations: firstly, the data in the NHANES database are cross-sectional, so it is difficult to infer a causal relationship between trace elements exposure and the odds of kidney stones. Secondly, since the trace elements we intended to study include Ni, only data from NHANES 2017–2018 were included. Thirdly, the concentrations of trace elements in urine may not reflect the actual exposure of the human body. Fourthly, self-reported kidney stones diagnosis may potentially affect the credibility of the results. Fifthly, BKMR and qgcomp cannot consider the sample weights for complex sampling, which may lead to inaccurate results. Sixthly, some unknown or residual mixes cannot be fully adjusted due to information acquisition limitations.
5. Conclusion
We found a negative association between trace elements mixture exposure and the prevalence of kidney stones. We also identified elements such as Co, As, iodine, Ni, and Cs that were significantly associated with the odds of kidney stones and found that there was a positive interaction between Ni and Pb in the whole population. Given the limitations of this study and the fact that the research was an exploratory study, these results need to be interpreted with caution and further investigations are needed to support our findings.
Data availability statement
Publicly available datasets were analyzed in this study. This data can be found here: [NHANES] repository (https://www.cdc.gov/nchs/nhanes/).
Ethics statement
The studies involving humans were approved by the studies involving human participants were reviewed and approved by the [National Center for Health Statistics (NCHS) Ethics Review Board, Centers for Disease Control and Prevention, 1,600 Clifton Road Atlanta, GA 30329–4,027 USA]. The studies were conducted in accordance with the local legislation and institutional requirements. The participants provided their written informed consent to participate in this study.
Author contributions
XW: Conceptualization, Methodology, Software, Visualization, Writing – original draft, Data curation. JZ: Software, Validation, Writing – review & editing. ZM: Writing – original draft. YY: Writing – original draft. YD: Writing – original draft. SC: Writing – original draft. XS: Writing – original draft. CO: Writing – original draft. JP: Writing – original draft. XH: Supervision.
Funding
The author(s) declare that no financial support was received for the research, authorship, and/or publication of this article.
Acknowledgments
The authors thank the National Center for Health Statistics of the Centers for Disease Control and Prevention for sharing the data.
Conflict of interest
The authors declare that the research was conducted in the absence of any commercial or financial relationships that could be construed as a potential conflict of interest.
Publisher’s note
All claims expressed in this article are solely those of the authors and do not necessarily represent those of their affiliated organizations, or those of the publisher, the editors and the reviewers. Any product that may be evaluated in this article, or claim that may be made by its manufacturer, is not guaranteed or endorsed by the publisher.
Supplementary material
The Supplementary material for this article can be found online at: https://www.frontiersin.org/articles/10.3389/fpubh.2023.1251637/full#supplementary-material
References
1. Stamatelou, KK, Francis, ME, Jones, CA, Nyberg, LM Jr, and Curhan, GC. Time trends in reported prevalence of kidney stones in the United States: 1976–1994. Kidney Int. (2003) 63:1817–23. doi: 10.1046/j.1523-1755.2003.00917.x
2. Scales, CD Jr, Smith, AC, Hanley, JM, and Saigal, CS. Prevalence of kidney stones in the United States. Eur Urol. (2012) 62:160–5. doi: 10.1016/j.eururo.2012.03.052
3. Hill, AJ, Basourakos, SP, Lewicki, P, Wu, X, Arenas-Gallo, C, Chuang, D, et al. Incidence of kidney stones in the United States: the continuous National Health and nutrition examination survey. J Urol. (2022) 207:851–6. doi: 10.1097/JU.0000000000002331
4. Romero, V, Akpinar, H, and Assimos, DG. Kidney stones: a global picture of prevalence, incidence, and associated risk factors. Rev Urol. (2010) 12:e86–96.
5. Tang, J, Mettler, P, McFann, K, and Chonchol, M. The association of prevalent kidney stone disease with mortality in US adults: the National Health and nutrition examination survey III, 1988-1994. Am J Nephrol. (2013) 37:501–6. doi: 10.1159/000350691
6. Moe, OW. Kidney stones: pathophysiology and medical management. Lancet. (2006) 367:333–44. doi: 10.1016/S0140-6736(06)68071-9
7. Alexander, RT, Hemmelgarn, BR, Wiebe, N, Bello, A, Samuel, S, Klarenbach, SW, et al. Kidney stones and cardiovascular events: a cohort study. Clin J Am Soc Nephrol. (2014) 9:506–12. doi: 10.2215/CJN.04960513
8. Gillen, DL, Worcester, EM, and Coe, FL. Decreased renal function among adults with a history of nephrolithiasis: a study of NHANES III. Kidney Int. (2005) 67:685–90. doi: 10.1111/j.1523-1755.2005.67128.x
9. Siener, R. Nutrition and kidney stone disease. Nutrients. (2021) 13:1917. doi: 10.3390/nu13061917
10. Curhan, GC, Willett, WC, Knight, EL, and Stampfer, MJ. Dietary factors and the risk of incident kidney stones in younger women: nurses' health study II. Arch Intern Med. (2004) 164:885–91. doi: 10.1001/archinte.164.8.885
11. Curhan, GC, Willett, WC, Rimm, EB, and Stampfer, MJ. A prospective study of dietary calcium and other nutrients and the risk of symptomatic kidney stones. N Engl J Med. (1993) 328:833–8. doi: 10.1056/NEJM199303253281203
12. Siener, R, and Hesse, A. Fluid intake and epidemiology of urolithiasis. Eur J Clin Nutr. (2003) 57:S47–51. doi: 10.1038/sj.ejcn.1601901
13. Tang, J, McFann, K, and Chonchol, M. Dietary zinc intake and kidney stone formation: evaluation of NHANES III. Am J Nephrol. (2012) 36:549–53. doi: 10.1159/000345550
14. Sun, Y, Wang, Y, Wang, D, and Zhou, Q. Dietary zinc intake, supplemental zinc intake and serum zinc levels and the prevalence of kidney stones in adults. J Trace Elem Med Biol. (2020) 57:126410. doi: 10.1016/j.jtemb.2019.126410
15. Sorokin, I, Mamoulakis, C, Miyazawa, K, Rodgers, A, Talati, J, and Lotan, Y. Epidemiology of stone disease across the world. World J Urol. (2017) 35:1301–20. doi: 10.1007/s00345-017-2008-6
16. Sun, Y, Zhou, Q, and Zheng, J. Nephrotoxic metals of cadmium, lead, mercury and arsenic and the odds of kidney stones in adults: an exposure-response analysis of NHANES 2007-2016. Environ Int. (2019) 132:105115. doi: 10.1016/j.envint.2019.105115
17. Liu, Y, Zhang, C, Qin, Z, Yang, Q, Lei, J, Tang, X, et al. Analysis of threshold effect of urinary heavy metal Elements on the high prevalence of nephrolithiasis in men. Biol Trace Elem Res. (2022) 200:1078–88. doi: 10.1007/s12011-021-02740-z
18. Tang, X, Li, N, Kang, L, Dubois, AM, Gong, Z, Wu, B, et al. Chronic low level trimethyltin exposure and the risk of developing nephrolithiasis. Occup Environ Med. (2013) 70:561–7. doi: 10.1136/oemed-2012-101261
19. Thomas, LD, Elinder, CG, Tiselius, HG, Wolk, A, and Åkesson, A. Dietary cadmium exposure and kidney stone incidence: a population-based prospective cohort study of men & women. Environ Int. (2013) 59:148–51. doi: 10.1016/j.envint.2013.06.008
20. Ferraro, PM, Taylor, EN, Gambaro, G, and Curhan, GC. Caffeine intake and the risk of kidney stones. Am J Clin Nutr. (2014) 100:1596–603. doi: 10.3945/ajcn.114.089987
21. Chen, TC, Clark, J, Riddles, MK, Mohadjer, LK, and Fakhouri, THI. National Health and nutrition examination survey, 2015-2018: sample design and estimation procedures. Vital Health Stat. (2020) 2:1–35.
22. Fakhouri, T, Martin, CB, Chen, TC, Akinbami, LJ, Ogden, CL, Paulose-Ram, R, et al. An investigation of nonresponse bias and survey location variability in the 2017-2018 National Health and nutrition examination survey. Vital Health Stat. (2020) 2:1–36.
23. Keil, AP, Buckley, JP, O’Brien, KM, Ferguson, KK, Zhao, S, and White, AJ. A quantile-based g-computation approach to addressing the effects of exposure mixtures. Environ Health Perspect. (2020) 128:47004. doi: 10.1289/EHP5838
24. Bobb, JF, Valeri, L, Claus Henn, B, Christiani, DC, Wright, RO, Mazumdar, M, et al. Bayesian kernel machine regression for estimating the health effects of multi-pollutant mixtures. Biostatistics. (2015) 16:493–508. doi: 10.1093/biostatistics/kxu058
25. Bobb, JF, Claus Henn, B, Valeri, L, and Coull, BA. Statistical software for analyzing the health effects of multiple concurrent exposures via Bayesian kernel machine regression. Environ Health. (2018) 17:67. doi: 10.1186/s12940-018-0413-y
26. Ali, H, Khan, E, and Ilahi, I. Environmental chemistry and ecotoxicology of hazardous heavy metals: environmental persistence, toxicity, and bioaccumulation. J Chem. (2019) 2019:305. doi: 10.1155/2019/6730305
27. Cannas, D, Loi, E, Serra, M, Firinu, D, Valera, P, and Zavattari, P. Relevance of essential trace Elements in nutrition and drinking water for human health and autoimmune disease risk. Nutrients. (2020) 12:2074. doi: 10.3390/nu12072074
28. Singh, VK, and Rai, PK. Kidney stone analysis techniques and the role of major and trace elements on their pathogenesis: a review. Biophys Rev. (2014) 6:291–310. doi: 10.1007/s12551-014-0144-4
29. Hesse, A, and Siener, R. Trace Elements in Urolithiasis. Urolithiasis. (2012) 227–30. doi: 10.1007/978-1-4471-4387-1_27
30. Joost, J, and Tessadri, R. Trace element investigations in kidney stone patients. Eur Urol. (1987) 13:264–70. doi: 10.1159/000472792
31. Zhao, H, Fang, L, Chen, Y, Ma, Y, Xu, S, Ni, J, et al. Associations of exposure to heavy metal mixtures with kidney stone among US adults: a cross-sectional study. Environ Sci Pollut Res. (2023) 30:96591–603. doi: 10.1007/s11356-023-29318-7
32. Orr, SE, and Bridges, CC. Chronic kidney disease and exposure to nephrotoxic metals. Int J Mol Sci. (2017) 18:1039. doi: 10.3390/ijms18051039
33. Rana, MN, Tangpong, J, and Rahman, MM. Toxicodynamics of Lead, cadmium, mercury and arsenic-induced kidney toxicity and treatment strategy: a mini review. Toxicol Rep. (2018) 5:704–13. doi: 10.1016/j.toxrep.2018.05.012
34. Barry, V, Todd, AC, and Steenland, K. Bone lead associations with blood lead, kidney function and blood pressure among US, lead-exposed workers in a surveillance programme. Occup Environ Med. (2019) 76:349–54. doi: 10.1136/oemed-2018-105505
35. Harari, F, Sallsten, G, Christensson, A, Petkovic, M, Hedblad, B, Forsgard, N, et al. Blood Lead levels and decreased kidney function in a population-based cohort. Am J Kidney Dis. (2018) 72:381–9. doi: 10.1053/j.ajkd.2018.02.358
36. Nan, YX, Yang, J, Yang, J, Wei, L, and Bai, Y. Associations between individual and combined metal exposures in whole blood and kidney function in US adults aged 40 years and older. Biol Trace Elem Res. (2023). doi: 10.1007/s12011-023-03722-z
37. Huang, JL, Mo, ZY, Li, ZY, Liang, GY, Liu, HL, Aschner, M, et al. Association of lead and cadmium exposure with kidney stone incidence: a study on the non-occupational population in Nandan of China. J Trace Elem Med Biol. (2021) 68:126852. doi: 10.1016/j.jtemb.2021.126852
38. Hara, A, Yang, WY, Petit, T, Zhang, ZY, Gu, YM, Wei, FF, et al. Incidence of nephrolithiasis in relation to environmental exposure to lead and cadmium in a population study. Environ Res. (2016) 145:1–8. doi: 10.1016/j.envres.2015.11.013
39. Kuta, J, Machát, J, Benová, D, Červenka, R, Zeman, J, and Martinec, P. Association of minor and trace elements with mineralogical constituents of urinary stones: a hard nut to crack in existing studies of urolithiasis. Environ Geochem Health. (2013) 35:511–22. doi: 10.1007/s10653-013-9511-5
40. Liu, WN, Gan, YJ, Ding, Y, Zhang, LN, Jiao, XJ, Liu, L, et al. Autophagy promotes GSDME-mediated pyroptosis via intrinsic and extrinsic apoptotic pathways in cobalt chloride-induced hypoxia reoxygenation-acute kidney injury. Ecotoxicol Environ Saf. (2022) 242:113881. doi: 10.1016/j.ecoenv.2022.113881
41. Guo, ZL, Wang, JY, Gong, LL, Gan, S, Gu, CM, and Wang, SS. Association between cadmium exposure and urolithiasis risk: a systematic review and meta-analysis. Medicine. (2018) 97:e9460. doi: 10.1097/MD.0000000000009460
42. Ferraro, PM, Bonello, M, Frigo, AC, D’Addessi, A, Sturniolo, A, and Gambaro, G. Cadmium exposure and kidney stone formation in the general population--an analysis of the National Health and nutrition examination survey III data. J Endourol. (2011) 25:875–80. doi: 10.1089/end.2010.0572
43. Hofbauer, J, Steffan, I, Höbarth, K, Vujicic, G, Schwetz, H, Reich, G, et al. Trace elements and urinary stone formation: new aspects of the pathological mechanism of urinary stone formation. J Urol. (1991) 145:93–6. doi: 10.1016/s0022-5347(17)38256-3
44. Moser, R, Zaccarini, F, Moser, W, Schrittwieser, R, and Kerbl, R. Metals in human gall, bladder, and kidney stones based on an electron microprobe investigation. Microsc Microanal. (2015) 21:1167–72. doi: 10.1017/S1431927615000690
45. Munoz, JA, and Valiente, M. Effects of trace metals on the inhibition of calcium oxalate crystallization. Urol Res. (2005) 33:267–72. doi: 10.1007/s00240-005-0468-4
46. Sutor, DJ. Growth studies of calcium oxalate in the presence of various ions and compounds. Br J Urol. (1969) 41:171–8. doi: 10.1111/j.1464-410x.1969.tb09919.x
47. Yang, J, Yang, A, Cheng, N, Huang, W, Huang, P, Liu, N, et al. Sex-specific associations of blood and urinary manganese levels with glucose levels, insulin resistance and kidney function in US adults: national health and nutrition examination survey 2011-2016. Chemosphere. (2020) 258:126940. doi: 10.1016/j.chemosphere.2020.126940
48. Gillams, K, Juliebø-Jones, P, Juliebø, SØ, and Somani, BK. Gender differences in kidney stone disease (KSD): findings from a systematic review. Curr Urol Rep. (2021) 22:50. doi: 10.1007/s11934-021-01066-6
49. Lieske, JC, Rule, AD, Krambeck, AE, Williams, JC, Bergstralh, EJ, Mehta, RA, et al. Stone composition as a function of age and sex. Clin J Am Soc Nephrol. (2014) 9:2141–6. doi: 10.2215/Cjn.05660614
50. Tao, CZ, et al. Independent and combined associations of urinary heavy metals exposure and serum sex hormones among adults in NHANES 2013-2016. Environ Pollut. (2021) 281:117097. doi: 10.1016/j.envpol.2021.117097
Keywords: trace element, kidney stone, NHANES, BKMR, qgcomp
Citation: Wang X, Zhang J, Ma Z, Yang Y, Dang Y, Cao S, Shi X, Ouyang C, Pan J and Hu X (2023) Association and interactions between mixed exposure to trace elements and the prevalence of kidney stones: a study of NHANES 2017–2018. Front. Public Health. 11:1251637. doi: 10.3389/fpubh.2023.1251637
Edited by:
Alica Pizent, Institute for Medical Research and Occupational Health, CroatiaReviewed by:
Ozden Gokdemir, İzmir University of Economics, TürkiyeYuan Xue, Zhengzhou University, China
Copyright © 2023 Wang, Zhang, Ma, Yang, Dang, Cao, Shi, Ouyang, Pan and Hu. This is an open-access article distributed under the terms of the Creative Commons Attribution License (CC BY). The use, distribution or reproduction in other forums is permitted, provided the original author(s) and the copyright owner(s) are credited and that the original publication in this journal is cited, in accordance with accepted academic practice. No use, distribution or reproduction is permitted which does not comply with these terms.
*Correspondence: Xiaobin Hu, lzhuxb@126.com