- 1School of Post-baccalaureate Medicine, College of Medicine, Kaohsiung Medical University, Kaohsiung, Taiwan
- 2Division of Nephrology, Department of Internal Medicine, Kaohsiung Medical University Hospital, Kaohsiung Medical University, Kaohsiung, Taiwan
- 3Faculty of Medicine, College of Medicine, Kaohsiung Medical University, Kaohsiung, Taiwan
- 4Department of Internal Medicine, Kaohsiung Municipal Siaogang Hospital, Kaohsiung Medical University, Kaohsiung, Taiwan
- 5Research Center for Precision Environmental Medicine, Kaohsiung Medical University, Kaohsiung, Taiwan
- 6Division of Hepatobiliary, Department of Internal Medicine, Kaohsiung Medical University Hospital, Kaohsiung Medical University, Kaohsiung, Taiwan
- 7Department of Public Health and Environmental Medicine, School of Medicine, College of Medicine, Kaohsiung Medical University, Kaohsiung, Taiwan
- 8Department of Public Health, College of Health Sciences, Kaohsiung Medical University, Kaohsiung, Taiwan
- 9Institute of Environmental Engineering, College of Engineering, National Sun Yat-Sen University, Kaohsiung, Taiwan
- 10Department of Medical Research, Kaohsiung Medical University Hospital, Kaohsiung, Taiwan
- 11Department of Dermatology, Kaohsiung Medical University Hospital, Kaohsiung, Taiwan
- 12Department of Dermatology, College of Medicine, Kaohsiung Medical University, Kaohsiung, Taiwan
- 13Doctoral Degree Program, Department of International Business, National Kaohsiung University of Science and Technology, Kaohsiung, Taiwan
- 14Doctoral Degree Program, Department of Public Health, College of Health Sciences, Kaohsiung Medical University, Kaohsiung, Taiwan
- 15Division of Pulmonary and Critical Care Medicine, Department of Internal Medicine, Kaohsiung Medical University Hospital, Kaohsiung Medical University, Kaohsiung, Taiwan
- 16Department of Pediatrics, Kaohsiung Medical University Hospital, Kaohsiung Medical University, Kaohsiung, Taiwan
- 17Division of Gastroenterology, Department of Internal Medicine, Kaohsiung Medical University Hospital, Kaohsiung Medical University, Kaohsiung, Taiwan
Background: Chronic Obstructive lung diseases (COPD) are complex conditions influenced by various environmental, lifestyle, and genetic factors. Ambient air pollution has been identified as a potential risk factor, causing 4.2 million deaths worldwide in 2016, accounting for 25% of all COPD-related deaths and 26% of all respiratory infection-related deaths. This study aims to evaluate the associations among chronic lung diseases, air pollution, and meteorological factors.
Methods: This cross-sectional study obtained data from the Taiwan Biobank and Taiwan Air Quality Monitoring Database. We defined obstructive lung disease as patients with FEV1/FVC < 70%. Descriptive analysis between spirometry groups was performed using one-way ANOVA and the chi-square or Fisher’s exact test. A generalized additive model (GAM) was used to evaluate the relationship between SO2 and PM2.5/PM10 through equations and splines fitting.
Results: A total of 2,635 participants were enrolled. Regarding environmental factors, higher temperature, higher relative humidity, and lower rainfall were risk factors for obstructive lung disease. SO2 was positively correlated with PM10 and PM2.5, with correlation coefficients of 0.53 (p < 0.0001) and 0.52 (p < 0.0001), respectively. Additionally, SO2 modified the relative risk of obstructive impairment for both PM10 [β coefficient (β) = 0.01, p = 0.0052] and PM2.5 (β = 0.01, p = 0.0155). Further analysis per standard deviation (per SD) increase revealed that SO2 also modified the relationship for both PM10 (β = 0.11, p = 0.0052) and PM2.5 (β = 0.09, p = 0.0155). Our GAM analysis showed a quadratic pattern for SO2 (per SD) and PM10 (per SD) in model 1, and a quadratic pattern for SO2 (per SD) in model 2. Moreover, our findings confirmed synergistic effects among temperature, SO2 and PM2.5/PM10, as demonstrated by the significant associations of bivariate (SO2 vs. PM10, SO2 vs. PM2.5) thin-plate smoothing splines in models 1 and 2 with obstructive impairment (p < 0.0001).
Conclusion: Our study showed high temperature, humidity, and low rainfall increased the risk of obstructive lung disease. Synergistic effects were observed among temperature, SO2, and PM2.5/PM10. The impact of air pollutants on obstructive lung disease should consider these interactions.
1. Introduction
Obstructive lung diseases such as asthma, chronic obstructive pulmonary disease (COPD), and bronchiectasis are complex heterogeneous diseases resulting from interactions among environmental, lifestyle, and genotype factors. In 2015, around 358.2 and 174.5 million individuals worldwide had asthma and COPD, respectively, and 0.4 and 3.2 million people died from the diseases (1). The high prevalence and mortality associated with obstructive lung disease result in significant medical and social costs (2, 3) and therefore it is crucial to determine the risk factors and comorbidities that cause obstructive lung disease.
Ambient air pollution has been identified as a potential risk factor for obstructive lung disease. Air pollution is a mixture of hazardous substances, including particulate matter (PM10, PM2.5), sulfur dioxide (SO2), nitrogen monoxide (NO), nitrogen dioxide (NO2), nitrogen oxides (NOx), carbon monoxide (CO), and ozone (O3). Aerosol-like air pollutants are transported to the alveoli by inhalation, and PM is subsequently deposited in the respiratory tract. These air pollutants can induce the release of inflammatory mediators and lead to the development of obstructive lung disease. Previous studies have revealed associations between exposure to air pollutants and daily admissions for COPD (4) and increased mortality and morbidity (5, 6). In 2016, ambient air pollution was reported to cause 4.2 million deaths worldwide, including 25% of all COPD deaths and 26% of all respiratory infection-related deaths (7, 8). Ambient air pollution has also been associated with cardiovascular (9, 10) and central nervous system diseases (11). Furthermore, air pollution is correlated with meteorological factors (12). A previous study demonstrated an additive interaction between high temperature and air pollution (13), and another study found that a decrease in lung function was related to high temperature and humidity (14).
Air pollution usually contains many harmful components, and interactions between these components are possible. For example, Yun et al. found a synergistic effect between PM10 and SO2. In their study, cell damage and apoptosis occurred at low exposure to both PM10 and SO2, however these effects were not observed when exposed to either PM10 or SO2 alone at the same concentration (15). In addition, Ku et al. reported that low exposure to both PM2.5 and SO2 could lead to neurodegeneration (16). Moreover, interactions between fine particles with NO2 or O3 have also been associated with adverse effects such as cardiovascular diseases (17, 18) and respiratory diseases (19), as well as an increased risk of preterm birth (20). Taken together, interactions between air pollutants can affect health even at a low concentrations, and therefore it is important to understand the synergistic impact of air pollutants on health.
In this study, we aimed to evaluate the relationships among chronic lung diseases, air pollution, meteorological factors and anthropometric indices, and also the synergistic effect of SO2 and PM2.5/PM10. We hypothesized that exposure to SO2 and PM2.5/PM10 air pollution may be associated with lower lung function and higher prevalence of obstructive lung disease, even at relatively lower concentrations of PM2.5 and PM10.
2. Materials and methods
2.1. Data source and study population
This cross-sectional study used data from two large databases: the Taiwan Biobank (TWB) and the Taiwan Air Quality Monitoring Database (TAQMD), both of which were obtained from the Taiwan Environmental Protection Administration (TEPA). The Taiwan Biobank (TWB) is the largest biobank in Taiwan, consisting of biological samples and associated data collected from volunteers aged between 30 and 70 years old who do not have a history of cancer. Prior to participation, every individual provided informed consent and underwent a face-to-face comprehensive interview, physical examination, blood sampling, and completed a questionnaire covering personal information and lifestyle factors. These procedures ensured that a detailed and comprehensive set of data could be collected for analysis, contributing to the understanding of health and disease in the Taiwanese population. We used data from 74 air quality monitoring stations located throughout Taiwan, as recorded by the TAQMD on a daily basis. The TAQMD was established by the Executive Yuan of the Taiwan Environmental Protection Administration, and is comprised of daily air pollutant concentration data at the study period of data collection. PM2.5 and PM10 were detected by β-ray attenuation method, SO2 was detected by ultraviolet fluorescence method, CO was determined by nondispersive infrared method, O3 was calculated by ultraviolet absorption method, NOx was detected by chemiluminescence method. All air pollutant data is stored in the cloud every hour for free. The average concentrations of air pollutants in a selected year were obtained before analysis.
By utilizing both the TWB and TAQMD, we were able to determine the nearest air quality monitoring station to the residential addresses of the participants using a three-step procedure. First, we used Google geocoding to determine the exact geoposition of each residential address. Second, we determined the interpolation point between each residential address and the nearest air quality monitoring station. Lastly, we selected data from the air quality monitoring station recorded during the year leading up to the survey date and calculated the average values of air pollutants including PM2.5, PM10, CO, NO, NO2, NOx, SO2, and O3 for the chosen year (21).
2.2. Variables
The following variables were recorded: demographic characteristics including age, gender, smoking and alcohol consumption; anthropometric parameters including height, weight, body mass index (BMI), body adiposity index (BAI), and body roundness index (BRI); comorbidities including hypertension, type 2 diabetes, renal failure, metabolic syndrome, and coronary artery disease; region of Taiwan, including northern, central, and southern regions; and meteorological factors including temperature (in Celsius), relative humidity (in percentage), and rainfall (in millimeters).
2.3. Lung function status
Pulmonary function parameters including forced expiratory volume in one second (FEV1), forced vital capacity (FVC), FEV1/FVC% ratio, FVC-predicted value, and FEV1-predicted value, were recorded in the TWB. Technicians used MicroLab spirometers and Spida 5 software (Micro Medical Ltd., Rochester, Kent, UK) (22) to perform spirometry measurements. Obstructive lung diseases including asthma, COPD, and bronchiectasis were defined as patients with FEV1/FVC < 70%, according to the American Thoracic Society and European Respiratory Society guidelines.
2.4. Statistical analysis
We used one-way ANOVA and the chi-square or Fisher’s exact tests as appropriate. Multinomial logistic regression was used to estimate crude odds ratios (ORs) and 95% confidence intervals (CIs). Stepwise multinomial logistic regression was used to calculate adjusted ORs and 95% CIs. In addition, for the factors showing a significant association in the crude analysis, estimated adjusted ORs and 95% CIs were further used to evaluate associations between covariant factors and obstructive lung disease. Pearson’s correlation analysis was used to evaluate the relationships between variables (temperature, relative humidity, rainfall, PM10, PM2.5, and SO2). As correlations between SO2 and PM2.5 and SO2 and PM10 were found, a generalized additive model (GAM) was further used to evaluate the relationships between SO2 and PM2.5 and SO2 and PM10 to fit equations and splines, and to explore linear and nonlinear effects of SO2 and PM2.5 or PM10 on the outcomes of obstructive impairment. All data analyses were performed using SAS software version 9.4 (SAS Institute Inc., Cary, NC, USA).
3. Results
3.1. Profiles of the participants
The mean age of the 2,635 enrolled participants was 49.80 ± 10.53 years. Of these participants, 1,225 (46.5%) were men, and 1,410 (53.5%) were women. The participants were stratified into two groups according to lung function test results: the control group (normal spirometry group) and chronic lung disease group (obstructive impairment). Overall, 72.2% (1902/2635) of the participants were classified into the control group, and 27.8% (733/2635) were classified into the chronic lung disease group. Propensity score matching (1:2) was performed to balance the baseline characteristics between the two groups. Table 1 shows the results of baseline characteristics before and after propensity score matching.
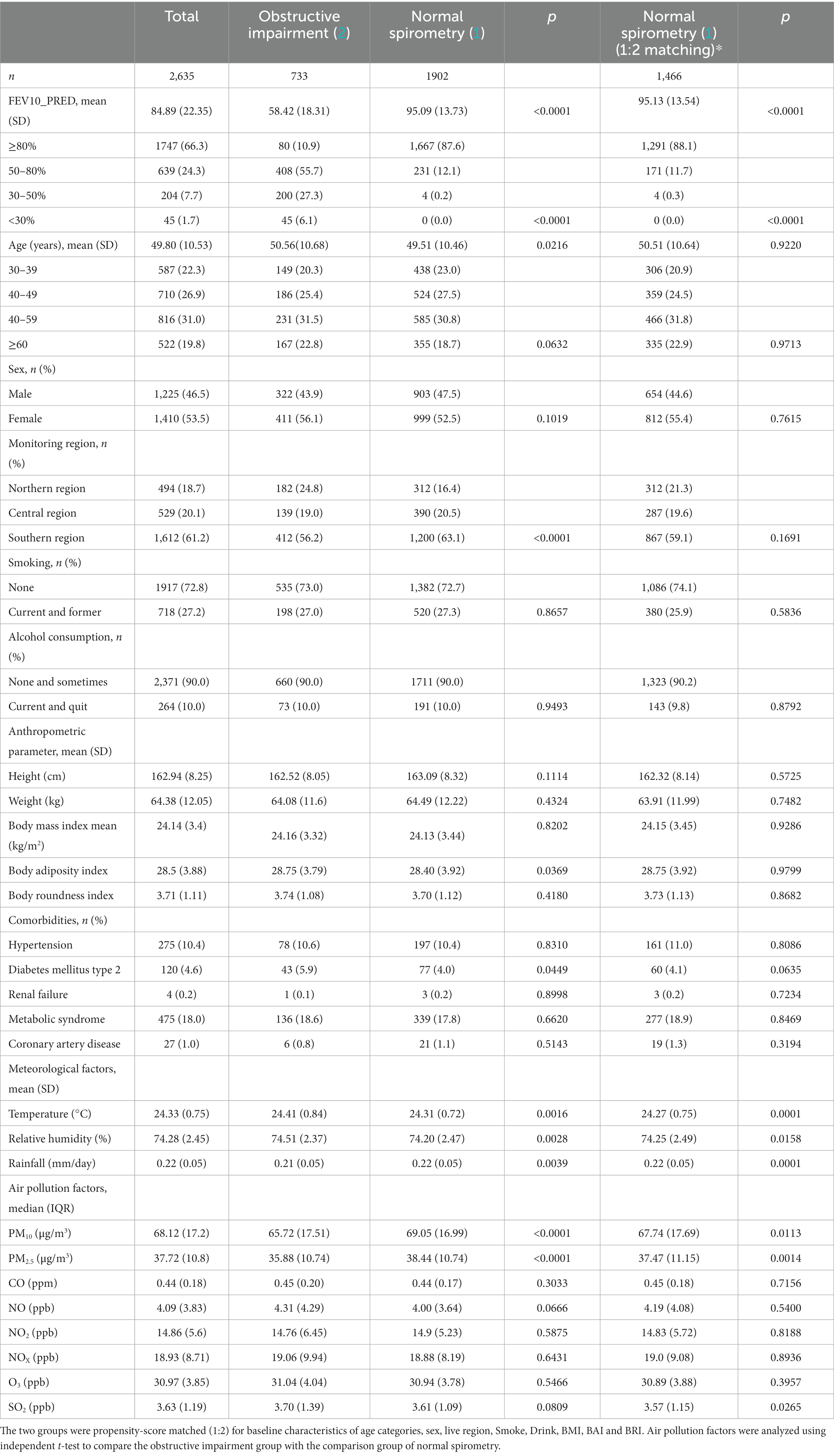
Table 1. Descriptive statistics of the demographic, laboratory, meteorological factors, and air pollutants.
There were no significant differences in age, gender, smoking, alcohol consumption, anthropometric factors and comorbidities, including hypertension, type 2 diabetes mellitus, renal failure, metabolic syndrome, and coronary artery disease between the two groups. Regarding meteorological factors, higher temperature, higher relative humidity, and lower rainfall were risk factors for obstructive lung disease. In addition, we found that exposure to SO2 in the environment increased the impact on patients with obstructive lung disease, whereas PM2.5 and PM10 decreased the impact (Table 1).
3.2. Correlations among meteorological factors and SO2, PM2.5/PM10
We found that SO2 was positively correlated with PM10 and PM2.5, with correlation coefficients of 0.53 (p < 0.0001) and 0.52 (p < 0.0001), respectively (Table 2). In addition, PM10 and PM2.5 were also positively correlated (correlation coefficient = 0.69, p < 0.0001).
3.3. Associations among obstructive lung disease, meteorological factors and SO2, PM2.5/PM10
To further determine whether SO2 modified the relationship of PM10 or PM2.5 with the relative risk of obstructive impairment, beta coefficients with standard error [β (SE)] and p-values for interaction were calculated. The results showed that SO2 modified the relationship of both PM10 (β = 0.01, p = 0.0052) and PM2.5 (β = 0.01, p = 0.0155) with the relative risk of obstructive impairment (Table 3). Analysis of per standard deviation (per SD) increase also showed that SO2 modified the relationship of both PM10 (β = 0.11, p = 0.0052) and PM2.5 (β = 0.09, p = 0.0155). Table 3 shows the crude ORs of meteorological factors and SO2, PM2.5/PM10. Compared with the control group, the obstructive impairment group was associated with higher temperature, higher relative humidity, and lower rainfall, and also exposure to a higher level of SO2 and lower levels of PM2.5 and PM10. Interactions were also identified between SO2 and PM2.5/PM10 (Table 3). Model 1 showed that the independent predictive factors were temperature (OR = 1.24; 95% CI = 1.09–1.41; p = 0.0009), relative humidity (OR = 1.05; 95% CI = 1.01–1.10; p = 0.0160), rainfall (OR = 0.08; 95% CI = 0.01–0.68; p = 0.0202), PM10 (OR = 0.99; 95% CI = 0.98–0.99; p < 0.001), and SO2 (OR = 1.25; 95% CI = 1.14–1.36; p < 0.001). Model 2 showed that the independent predictive factors were temperature (OR = 1.31; 95% CI = 1.15–1.49; p < 0.001), relative humidity (OR = 1.04; 95% CI = 1.00–1.09; p = 0.0372), rainfall (OR = 0.08; 95% CI = 0.01–0.67; p = 0.0197), PM2.5 (OR = 0.97; 95% CI = 0.96–0.98; p < 0.001), and SO2 (OR = 1.28; 95% CI = 1.17–1.39; p < 0.001).
3.4. Interactions among obstructive lung disease with SO2 and PM2.5 or PM10
The GAM (Figure 1) showed that obstructive impairment was associated with a quadratic pattern for SO2 (per SD) and PM10 (per SD) in model 1, and a quadratic pattern for SO2 (per SD) but not PM2.5 (per SD) in model 2. We also found that the bivariate thin-plate smoothing spline in models 1 and 2 were significantly associated with obstructive impairment (p < 0.0001) (Table 4). In addition, bivariate smoothing of SO2, PM10 and PM2.5 showed evidence of the risk of obstructive impairment (Figures 2A,B). A semiparametric model was generated using the parametric effects of temperature (°C), relative humidity (%) and rainfall (mm/day) as the linear part of the model.
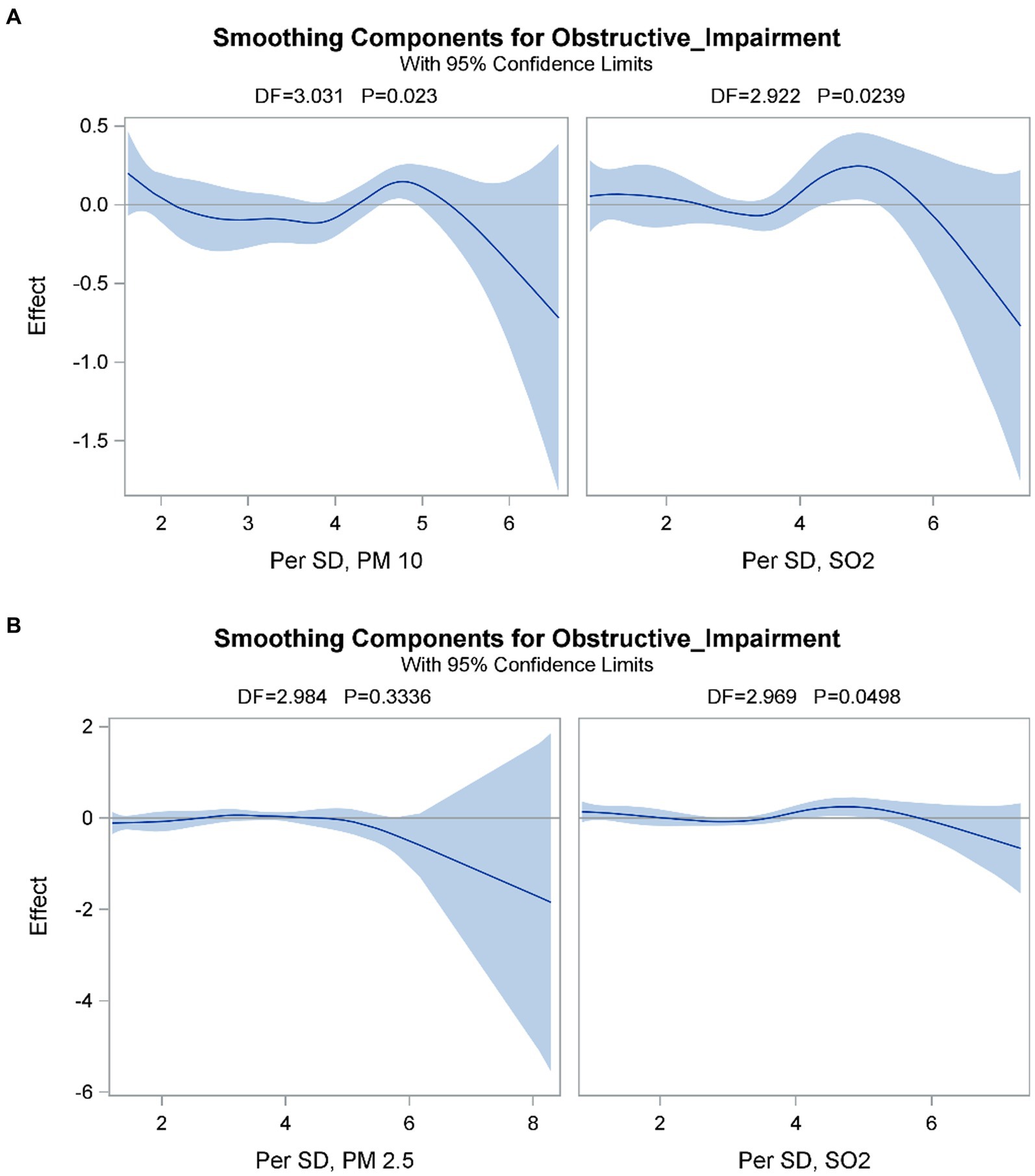
Figure 1. Partial prediction of A) SO2 (Per SD) and PM10 (Per SD) in model 1 and B) SO2 (Per SD) and PM2.5 (Per SD) on the risk of obstructive impairment. A semiparametric model was performed by using the parametric effects of temperature (°C), relative humidity (%) and Rainfall (mm/day) as the linear part of the model. Obstructive impairment was associated with a quadratic pattern for the SO2 (Per SD) and PM10 (Per SD) in model 1 and a quadratic pattern for the SO2 (Per SD) but not PM2.5 (Per SD) in model 2.
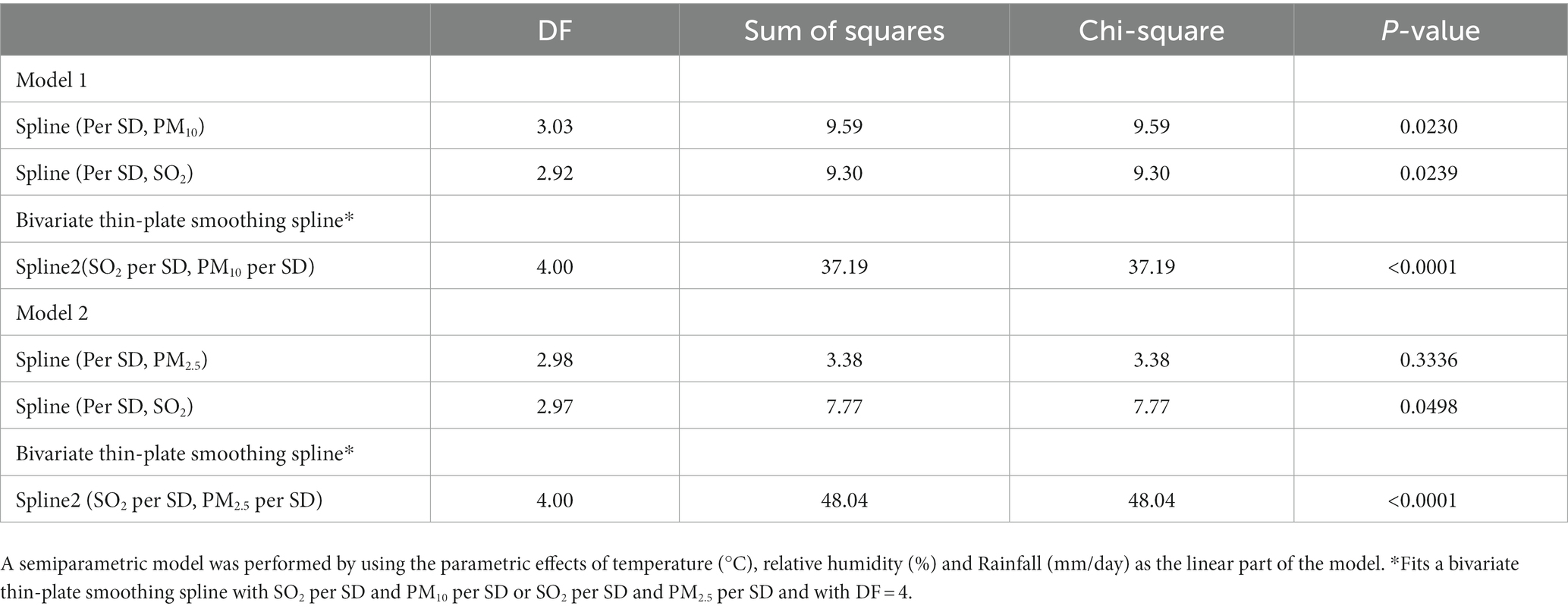
Table 4. Predicted obstructive impairment by generalized additive model, a smoothing spline nonparametric model.
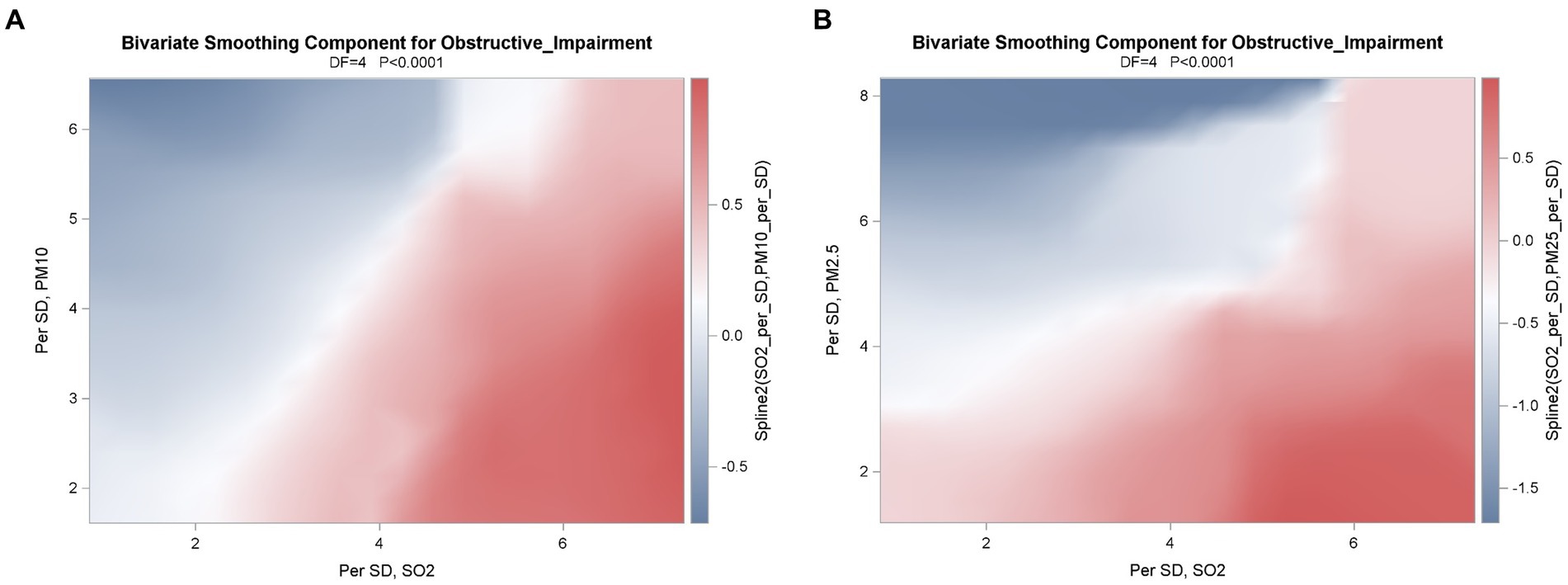
Figure 2. Correlations between A) SO2 (Per SD) and PM10 (Per SD) in model 1 and B) SO2 (Per SD) and PM2.5 (Per SD) in model 2 of obstructive impairment were applied by the use of a generalized additive model (GAM), a smoothing spline nonparametric model. A semiparametric model was performed by using the parametric effects of temperature (°C), relative humidity (%) and Rainfall (mm/day) as the linear part of the model. The graphic suggests that there was an interaction, a diagonal pattern in model 1 and model 2, on the risk of obstructive impairment.
4. Discussion
In this study, we analyzed 2,635 participants in the TWB and found that factors associated with a higher risk of obstructive lung disease included higher temperature, higher relative humidity, and lower rainfall. We also found that SO2 was strongly associated with obstructive lung disease, while PM2.5 and PM10 were not. Further analysis revealed that SO2 synergistically interacted with PM2.5 and PM10 to increase the risk of obstructive lung disease.
Overall, 27.8% of our study population had obstructive impairment. However, a previous study estimated that the prevalence of COPD in Taiwan was around 6.1% (23), with a prevalence of asthma of around 5.1% (24). The higher percentage of obstructive impairment in our study may be due to the presence of higher annual mean concentrations of air pollutants in southern Taiwan than in other areas (25, 26). In Table 1, we present the average air pollution levels based on a total of 2,635 observations, indicating the following values: PM10: 68.12 μg/m3, PM2.5: 37.72 μg/m3, SO2: 3.63 ppb, CO: 0.44 ppm, NO: 4.09 ppb, NO2: 14.86 ppb, NOx: 18.93 ppb. Furthermore, around 1,612 individuals, which accounts for 61.2% of the total, were from southern Taiwan. The findings align with those of our prior study (21). Fine particles play an essential role in the development of obstructive lung disease (25), and thus people exposed to higher concentrations of air pollution may have a higher prevalence of lung impairment.
We also found that people living in areas with a higher temperature, higher relative humidity In a previous study in Taiwan, Wu et al. reported a V/U shaped relationship between temperature and air pollutants (12), and a temperature between 24.3–24.9°C was associated with exposure to the lowest concentration of air pollutants. Thus, a higher or lower temperature may result in higher exposure to air pollution, which may then affect the development of obstructive lung disease. A study in New York City found that the risk of hospitalization due to respiratory diseases increased by 2.7% per °C above the threshold of 28.9°C on the same day (27). Another study in London revealed that the risk of respiratory diseases was related to admission when the temperature increased by 5.44% per °C above a threshold (23°C) with a lag of 0–2 days (28). Thus, a higher temperature appears to increase the risk of developing obstructive lung disease. When considering temperature and relative humidity, previous research has revealed a 0.7% decrease in FVC when there is a 5°C increase in the 3-day moving average temperature, and a 0.2% decrease in FVC when there is a 5% increase in the 7-day moving average relative humidity (14). Thermoregulation involves increasing cardiac output, cutaneous blood flow, and breathing rate. However, in conditions of high relative humidity evaporation by perspiration is limited, which creates physiological stress leading to dysfunction in respiratory function (29), especially in older people (30, 31). High temperature with high humidity has also been shown to affect thermoregulation and trigger bronchoconstriction (32). Thus, the risk of developing obstructive lung disease would increase under these conditions.
Our study also found that lower rainfall increased the risk of obstructive lung disease. A study conducted in Korea reported that the concentrations of air pollutants, including PM10 and NO2 were lower during rainfall compared to dry conditions (33). Another study in Korea revealed that pollutant (PM10, SO2, NO2, and CO) concentrations and rainfall intensity were significantly negatively correlated due to precipitation scavenging. Among those pollutants, PM10 was the most effectively scavenged by rain (34). In addition, a study in Spain reported a washout effect, with a 20% reduction in the number of particles during rainfall with an intensity of over 3.2 ± 1.5 mm/h (35). Thus, concentrations of air pollutants decrease due to a washout effect during rainfall, and consequently lower rainfall may be associated with a higher risk of obstructive lung disease.
Another finding of this study is that exposure to a higher level of SO2 and lower levels of PM2.5 and PM10 increased the risk of obstructive lung disease. SO2 is produced from volcanoes gas, burning fuel and industrial production processes (36–38). Exposure to SO2 has been shown to affect the respiratory tract and cause oxidative stress and DNA damage, which would further damage the lungs (39). Several studies have revealed a relationship between SO2 exposure and respiratory diseases (40–42). Goudarzi et al. concluded that a higher SO2 concentration was associated with an increased relative risk of hospital admission for respiratory diseases (43).
Particulate matter can be generated from soil dust, road traffic, industry, and fuel combustion, and it is a crucial indicator of the health effects of air pollution (44, 45). Several studies have discussed the relationship between PM and lung function change and respiratory diseases (12, 46, 47). Penttinen et al. reported a decrease in average evening peak expiratory flow by 1.14 L/min when the average concentration of PM2.5 increased by one interquartile (1.3 μg/m3) in a 5-day average (48). In addition, Downs et al. found significant negative associations between a lower concentration of PM10 and worsening lung function. They found that the annual decline in lung function with regards to FEV1 and FEF25–75 decreased by 9 and 16%, respectively, with a 10 μg/m3 reduction in PM10 over an 11-year period (49). Thus, higher concentrations of SO2 and PM appear to increase the risk of worsening lung function and developing obstructive lung disease. In our study, lower levels of PM2.5 and PM10 increased the risk of developing obstructive lung disease, which is contrast to most of previous studies. That is because, we found that there was a synergistic effect between SO2 and PM2.5/PM10. Yun et al. found that synergistic injury in terms of cell survival and apoptosis occurred under low concentrations of PM10 and SO2 (15). The proposed mechanism was that PM10 and SO2 synergistic induced cytotoxicity of radical oxygen species production and nuclear factor kappa B (NF-κB) activation (15, 50). Thus, the synergistic effect could increase the risk of respiratory diseases, even with low concentrations of the air pollutants. The synergistic effect could also explain our finding that a higher level of SO2 and lower levels of PM2.5 and PM10 increased the risk of obstructive lung disease. Furthermore, our results also showed that high SO2 exposure could affect lower concentrations of PM2.5 and PM10 with similar patterns (Figures 1, 2). These interesting findings indicate that SO2 could trigger PM2.5 and PM10, and that the interaction between SO2 and PM2.5/PM10 may play a vital role in developing obstructive lung disease.
Although our study is the first to comprehensively investigate the associations among obstructive lung disease (classified by lung function), air pollution, and meteorological factors, several limitations should be acknowledged. First, the design of this study was cross-sectional. Determining the progression of lung function and obstructive lung disease over time is complex, and further prospective studies are needed to elucidate the causal effects. Second, lung function assessments were used to identify chronic lung disease, and follow-up checkups are required to further evaluate the progression of the disease. Third, the TWB does not contain information regarding occupational exposure to toxic substances. Some poisonous substances may influence lung function, however we could not analyze this. Finally, because the participant’s residential address was used as the air pollutant exposure point, we did not include all factors affecting lung function, such as personal exposure, travel exposure, and indoor air quality. This may have led to underestimation of the risk of lung function impairment and the association with obstructive lung disease.
5. Conclusion
Compared with the normal spirometry group, we found that factors associated with a higher risk of obstructive lung disease included a higher temperature, higher relative humidity, and lower rainfall. Furthermore, we identified interactions and synergistic effects among SO2 and PM2.5/PM10. These findings could explain why a higher level of SO2 and lower levels of PM2.5/PM10 were associated with a higher risk of obstructive lung disease. Our findings also highlight the importance of interactions between air pollutants. We suggest that the synergistic effects of air pollutants should be considered when investigating the actual impact on developing obstructive lung disease.
Data availability statement
The original contributions presented in the study are included in the article/supplementary material, further inquiries can be directed to the corresponding author.
Ethics statement
The studies involving humans were approved by Institutional Review Board-1, Kaohsiung Medical University Chung-Ho-Memorial Hospital [KMUHIRB-E(I)-20180242]. The studies were conducted in accordance with the local legislation and institutional requirements. Written informed consent for participation was not required from the participants or the participants’ legal guardians/next of kin because this cross-sectional study obtained data from the Taiwan Biobank and Taiwan Air Quality Monitoring Database.
Author contributions
P-SC and S-CC: conceptualization and supervision. T-YC and D-WW: writing original draft and formal analysis. H-PT: methodology and supervision. C-WW: investigation and formal analysis. C-HH and C-HL: investigation and supervision. C-SH: writing review and editing. C-HK: supervision. All authors have read and agreed to the published version of the manuscript.
Funding
This work was supported partially by the Research Center for Precision Environmental Medicine, Kaohsiung Medical University, Kaohsiung, Taiwan from The Featured Areas Research Center Program within the framework of the Higher Education Sprout Project by the Ministry of Education (MOE) in Taiwan and by Kaohsiung Medical University Research Center Grants (KMU-TC112A01) and Kaohsiung Municipal Siaogang Hospital (S-110-05).
Conflict of interest
The authors declare that the research was conducted in the absence of any commercial or financial relationships that could be construed as a potential conflict of interest.
Publisher’s note
All claims expressed in this article are solely those of the authors and do not necessarily represent those of their affiliated organizations, or those of the publisher, the editors and the reviewers. Any product that may be evaluated in this article, or claim that may be made by its manufacturer, is not guaranteed or endorsed by the publisher.
References
1. Soriano, JB, Abajobir, AA, Abate, KH, Abera, SF, Agrawal, A, Ahmed, MB, et al. GBD 2015 Chronic Respiratory Disease Collaborators. Global, regional, and national deaths, prevalence, disability-adjusted life years, and years lived with disability for chronic obstructive pulmonary disease and asthma, 1990-2015: a systematic analysis for the global burden of disease study 2015. Lancet Respir Med. (2017) 5:691–706. doi: 10.1016/s2213-2600(17)30293-x
2. Anees ur Rehman,, Ahmad Hassali, MA, Muhammad, SA, Shah, S, Abbas, S, Hyder Ali, IAB, et al. The economic burden of chronic obstructive pulmonary disease (COPD) in the USA, Europe, and Asia: results from a systematic review of the literature. Expert Rev Pharmacoecon Outcomes Res. (2020) 20:661–72. doi: 10.1080/14737167.2020.1678385
3. Guarascio, AJ, Ray, SM, Finch, CK, and Self, TH. The clinical and economic burden of chronic obstructive pulmonary disease in the USA. Clinicoecon Outcomes Res. (2013) 5:235–45. doi: 10.2147/ceor.S34321
4. Anderson, HR, Spix, C, Medina, S, Schouten, JP, Castellsague, J, Rossi, G, et al. Air pollution and daily admissions for chronic obstructive pulmonary disease in 6 European cities: results from the APHEA project. Eur Respir J. (1997) 10:1064–71. doi: 10.1183/09031936.97.10051064
5. Sanyal, S, Rochereau, T, Maesano, CN, Com-Ruelle, L, and Annesi-Maesano, I. Long-term effect of outdoor air pollution on mortality and morbidity: a 12-year follow-up study for metropolitan France. Int J Environ Res Public Health. (2018) 15:2487. doi: 10.3390/ijerph15112487
6. Ko, FW, and Hui, DS. Air pollution and chronic obstructive pulmonary disease. Respirology. (2012) 17:395–401. doi: 10.1111/j.1440-1843.2011.02112.x
7. Isaifan, R. The dramatic impact of coronavirus outbreak on air quality: has it saved as much as it has killed so far? Global J Environ Sci Manage. (2020) 6:275–88. doi: 10.22034/gjesm.2020.03.01
8. WHO, Mortality and burden of disease from ambient air pollution. (2020). Available at: https://www.who.int/gho/phe/outdoor_air_pollution/burden
9. Franklin, BA, Brook, R, and Arden Pope, C. Air pollution and cardiovascular disease. Curr Probl Cardiol. (2015) 40:207–38. doi: 10.1016/j.cpcardiol.2015.01.003
10. Miller, MR, and Newby, DE. Air pollution and cardiovascular disease: car sick. Cardiovasc Res. (2020) 116:279–94. doi: 10.1093/cvr/cvz228
11. Block, ML, and Calderón-Garcidueñas, L. Air pollution: mechanisms of neuroinflammation and CNS disease. Trends Neurosci. (2009) 32:506–16. doi: 10.1016/j.tins.2009.05.009
12. Wu, DW, Chen, SC, Tu, HP, Wang, CW, Hung, CH, Chen, HC, et al. The impact of the synergistic effect of temperature and air pollutants on chronic lung diseases in subtropical Taiwan. J Personal Med. (2021) 11:819. doi: 10.3390/jpm11080819
13. Lee, W, Choi, HM, Kim, D, Honda, Y, Leon Guo, YL, and Kim, H. Synergic effect between high temperature and air pollution on mortality in Northeast Asia. Environ Res. (2019) 178:108735. doi: 10.1016/j.envres.2019.108735
14. Lepeule, J, Litonjua, AA, Gasparrini, A, Koutrakis, P, Sparrow, D, Vokonas, PS, et al. Lung function association with outdoor temperature and relative humidity and its interaction with air pollution in the elderly. Environ Res. (2018) 165:110–7. doi: 10.1016/j.envres.2018.03.039
15. Yun, Y, Gao, R, Yue, H, Li, G, Zhu, N, and Sang, N. Synergistic effects of particulate matter (PM10) and SO2 on human non-small cell lung cancer A549 via ROS-mediated NF-κB activation. J Environ Sci. (2015) 31:146–53. doi: 10.1016/j.jes.2014.09.041
16. Ku, T, Chen, M, Li, B, Yun, Y, Li, G, and Sang, N. Synergistic effects of particulate matter (PM2.5) and sulfur dioxide (SO2) on neurodegeneration via the microRNA-mediated regulation of tau phosphorylation. Toxicol Res (Camb). (2017) 6:7–16. doi: 10.1039/c6tx000314a
17. Huang, YC, Rappold, AG, Graff, DW, Ghio, AJ, and Devlin, RB. Synergistic effects of exposure to concentrated ambient fine pollution particles and nitrogen dioxide in humans. Inhal Toxicol. (2012) 24:790–7. doi: 10.3109/08958378.2012.718809
18. Brook, RD, Brook, JR, Urch, B, Vincent, R, Rajagopalan, S, and Silverman, F. Inhalation of fine particulate air pollution and ozone causes acute arterial vasoconstriction in healthy adults. Circulation. (2002) 105:1534–6. doi: 10.1161/01.cir.0000013838.94747.64
19. Siddika, N, Rantala, AK, Antikainen, H, Balogun, H, Amegah, AK, Ryti, NRI, et al. Synergistic effects of prenatal exposure to fine particulate matter (PM2.5) and ozone (O3) on the risk of preterm birth: a population-based cohort study. Environ Res. (2019) 176:108549. doi: 10.1016/j.envres.2019.108549
20. Gong, H Jr, Linn, WS, Clark, KW, Anderson, KR, Geller, MD, and Sioutas, C. Respiratory responses to exposures with fine particulates and nitrogen dioxide in the elderly with and without COPD. Inhal Toxicol. (2005) 17:123–32. doi: 10.1080/08958370590904481
21. Wu, DW, Cheng, YC, Wang, CW, Hung, CH, Chen, PS, Chu-Sung Hu, S, et al. Impact of the synergistic effect of pneumonia and air pollutants on newly diagnosed pulmonary tuberculosis in southern Taiwan. Environ Res. (2022) 212:113215. doi: 10.1016/j.envres.2022.113215
22. Hsieh, SW, Wu, DW, Wang, CW, Chen, SC, Hung, CH, and Kuo, CH. Poor cognitive function is associated with obstructive lung diseases in Taiwanese adults. Int J Environ Res Public Health. (2021) 18:2344. doi: 10.3390/ijerph18052344
23. Yu, CJ, Cheng, SL, Chan, MC, Wang, CC, Lin, CH, Wang, HC, et al. COPD in Taiwan: a National Epidemiology Survey. Int J Chron Obstruct Pulmon Dis. (2015) 10:2459–67. doi: 10.2147/copd.S89672
24. Hsieh, CJ, Yu, PY, Tai, CJ, Jan, RH, Wen, TH, Lin, SW, et al. Association between the first occurrence of asthma and residential greenness in children and teenagers in Taiwan. Int J Environ Res Public Health. (2019) 16:2076. doi: 10.3390/ijerph16122076
25. Hwang, SL, Guo, SE, Chi, MC, Chou, CT, Lin, YC, Lin, CM, et al. Association between atmospheric fine particulate matter and hospital admissions for chronic obstructive pulmonary disease in southwestern Taiwan: a population-based study. Int J Environ Res Public Health. (2016) 13:366. doi: 10.3390/ijerph13040366
26. Cheng, MH, Chiu, HF, and Yang, CY. Coarse particulate air pollution associated with increased risk of hospital admissions for respiratory diseases in a Tropical City, Kaohsiung, Taiwan. Int J Environ Res Public Health. (2015) 12:13053–68. doi: 10.3390/ijerph121013053
27. Lin, S, Luo, M, Walker, RJ, Liu, X, Hwang, SA, and Chinery, R. Extreme high temperatures and hospital admissions for respiratory and cardiovascular diseases. Epidemiology. (2009) 20:738–46. doi: 10.1097/EDE.0b013e3181ad5522
28. Hayes, D Jr, Collins, PB, Khosravi, M, Lin, RL, and Lee, LY. Bronchoconstriction triggered by breathing hot humid air in patients with asthma: role of cholinergic reflex. Am J Respir Crit Care Med. (2012) 185:1190–6. doi: 10.1164/rccm.201201-0088OC
29. Foster, KG, Ellis, FP, Doré, C, Exton-Smith, AN, and Weiner, JS. Sweat responses in the aged. Age Ageing. (1976) 5:91–101. doi: 10.1093/ageing/5.2.91
30. Cheung, SS. Responses of the hands and feet to cold exposure. Temperature (Austin). (2015) 2:105–20. doi: 10.1080/23328940.2015.1008890
31. Lin, RL, Hayes, D Jr, and Lee, LY. Bronchoconstriction induced by hyperventilation with humidified hot air: role of TRPV1-expressing airway afferents. J Appl Physiol. (2009) 106:1917–24. doi: 10.1152/japplphysiol.00065.2009
32. Kim, S, Hong, KH, Jun, H, Park, YJ, Park, M, and Sunwoo, Y. Effect of precipitation on air pollutant concentration in Seoul. Korea Asian J Atmospheric Environ. (2014) 8:202–11. doi: 10.5572/ajae.2014.8.4.202
33. Yoo, JM, Lee, YR, Kim, D, Jeong, MJ, Stockwell, WR, Kundu, PK, et al. New indices for wet scavenging of air pollutants (O3, CO, NO2, SO2, and PM10) by summertime rain. Atmos Environ. (2014) 82:226–37. doi: 10.1016/j.atmosenv.2013.10.022
34. Castro, A, Alonso-Blanco, E, González-Colino, M, Calvo, AI, Fernández-Raga, M, and Fraile, R. Aerosol size distribution in precipitation events in León, Spain. Atmos Res. (2010) 96:421–35. doi: 10.1016/j.atmosres.2010.01.014
35. Iwasawa, S, Kikuchi, Y, Nishiwaki, Y, Nakano, M, Michikawa, T, Tsuboi, T, et al. Effects of SO2 on respiratory system of adult Miyakejima resident 2 years after returning to the island. J Occup Health. (2009) 51:38–47. doi: 10.1539/joh.L8075
36. Burtraw, D, Evans, DA, Krupnick, A, Palmer, K, and Toth, R. Economics of pollution trading for SO2 and NOx. Annu Rev Environ Resour. (2005) 30:253–89. doi: 10.1146/annurev.energy.30.081804.121028
37. Luvsan, ME, Shie, RH, Purevdorj, T, Badarch, L, Baldorj, B, and Chan, CC. The influence of emission sources and meteorological conditions on SO2 pollution in Mongolia. Atmos Environ. (2012) 61:542–9. doi: 10.1016/j.atmosenv.2012.07.044
38. Meng, Z, Qin, G, Zhang, B, Geng, H, Bai, Q, Bai, W, et al. Oxidative damage of sulfur dioxide inhalation on lungs and hearts of mice. Environ Res. (2003) 93:285–92. doi: 10.1016/s0013-9351(03)00045-8
39. French, JG, Lowrimore, G, Nelson, WC, Finklea, JF, English, T, and Hertz, M. The effect of sulfur dioxide and suspended sulfates on acute respiratory disease. Archives Environ Health. (1973) 27:129–33. doi: 10.1080/00039896.1973.10666340
40. Charan, NB, Myers, CG, Lakshminarayan, S, and Spencer, TM. Pulmonary injuries associated with acute sulfur dioxide inhalation. Am Rev Respir Dis. (1979) 119:555–60.
41. Geravandi, S, Goudarzi, G, Mohammadi, MJ, Taghavirad, SS, and Salmanzadeh, S. Sulfur and nitrogen dioxide exposure and the incidence of health endpoints in Ahvaz, Iran. Health Scope. (2015) 4:e24318. doi: 10.17795/jhealthscope-24318
42. Goudarzi, G, Geravandi, S, Idani, E, Hosseini, SA, Baneshi, MM, Yari, AR, et al. An evaluation of hospital admission respiratory disease attributed to sulfur dioxide ambient concentration in Ahvaz from 2011 through 2013. Environ Sci Pollut Res. (2016) 23:22001–7. doi: 10.1007/s11356-016-7447-x
43. Karagulian, F, Belis, CA, Dora, CFC, Prüss-Ustün, AM, Bonjour, S, Adair-Rohani, H, et al. Contributions to cities' ambient particulate matter (PM): a systematic review of local source contributions at global level. Atmos Environ. (2015) 120:475–83. doi: 10.1016/j.atmosenv.2015.08.087
44. Adams, K, Greenbaum, DS, Shaikh, R, van Erp, AM, and Russell, AG. Particulate matter components, sources, and health: systematic approaches to testing effects. J Air Waste Manage Assoc. (2015) 65:544–58. doi: 10.1080/10962247.2014.1001884
45. Hansel, NN, McCormack, MC, and Kim, V. The effects of air pollution and temperature on COPD. COPD: J Chron Obstruct Pulmon Dis. (2016) 13:372–9. doi: 10.3109/15412555.2015.1089846
46. Xia, T, Kovochich, M, and Nel, AE. Impairment of mitochondrial function by particulate matter (PM) and their toxic components: implications for PM-induced cardiovascular and lung disease. Front Biosci. (2007) 12:1238–46. doi: 10.2741/2142
47. Penttinen, P, Vallius, M, Tiittanen, P, Ruuskanen, J, and Pekkanen, J. Source-specific fine particles in urban air and respiratory function among adult asthmatics. Inhal Toxicol. (2006) 18:191–8. doi: 10.1080/08958370500434230
48. Downs, SH, Schindler, C, Liu, LJS, Keidel, D, Bayer-Oglesby, L, Brutsche, MH, et al. Reduced exposure to PM10 and attenuated age-related decline in lung function. N Engl J Med. (2007) 357:2338–47. doi: 10.1056/NEJMoa073625
49. Li, R, Zhao, L, Tong, J, Yan, Y, and Xu, C. Fine particulate matter and sulfur dioxide Coexposures induce rat lung pathological injury and inflammatory responses via TLR4/p38/NF-κB pathway. Int J Toxicol. (2017) 36:165–73. doi: 10.1177/1091581816682225
Keywords: synergistic effect, air pollutants, climate factors, obstructive lung disease, generalized additive model
Citation: Chen T-Y, Chen S-C, Wang C-W, Tu H-P, Chen P-S, Hu SC-S, Li C-H, Wu D-W, Hung C-H and Kuo C-H (2023) The impact of the synergistic effect of SO2 and PM2.5/PM10 on obstructive lung disease in subtropical Taiwan. Front. Public Health. 11:1229820. doi: 10.3389/fpubh.2023.1229820
Edited by:
Liubin Huang, Shandong University, ChinaReviewed by:
Zhijing Lin, Anhui Medical University, ChinaSasan Faridi, Tehran University of Medical Sciences, Iran
Copyright © 2023 Chen, Chen, Wang, Tu, Chen, Hu, Li, Wu, Hung and Kuo. This is an open-access article distributed under the terms of the Creative Commons Attribution License (CC BY). The use, distribution or reproduction in other forums is permitted, provided the original author(s) and the copyright owner(s) are credited and that the original publication in this journal is cited, in accordance with accepted academic practice. No use, distribution or reproduction is permitted which does not comply with these terms.
*Correspondence: Da-Wei Wu, dTg5MDAwMzBAeWFob28uY29tLnR3