- 1Microbial Ecology and Microrganisms Genomics Laboratory, Istituto Zooprofilattico Sperimentale delle Venezie, Viale dell’Università, Legnaro, Italy
- 2Department of Veterinary and Animal Sciences, Faculty of Health and Medical Sciences, University of Copenhagen, Frederiksberg, Denmark
- 3Applied Chemistry Laboratory, Istituto Zooprofilattico Sperimentale delle Venezie, Vicenza, Italy
- 4OIE and National Reference Laboratory for Salmonellosis, Istituto Zooprofilattico Sperimentale delle Venezie, Viale dell’Università, Legnaro, Italy
Introduction: The spread of antimicrobial resistance among zoonotic pathogens such as Salmonella is a serious health threat, and mobile genetic elements (MGEs) carrying antimicrobial resistance genes favor this phenomenon. In this work, phenotypic antimicrobial resistance to commonly used antimicrobials was studied, and the antimicrobial resistance genes (ARGs) and plasmid replicons associated with the resistances were determined.
Methods: Eighty-eight Italian Salmonella enterica strains (n = 88), from human, animal and food sources, isolated between 2009 and 2019, were selected to represent serovars with different frequency of isolation in human cases of salmonellosis. The presence of plasmid replicons was also investigated.
Results and discussion: Resistances to sulphonamides (23.9%), ciprofloxacin (27.3%), ampicillin (29.5%), and tetracycline (32.9%) were the most found phenotypes. ARGs identified in the genomes correlated with the phenotypical results, with blaTEM-1B, sul1, sul2, tetA and tetB genes being frequently identified. Point mutations in gyrA and parC genes were also detected, in addition to many different aminoglycoside-modifying genes, which, however, did not cause phenotypic resistance to aminoglycosides. Many genomes presented plasmid replicons, however, only a limited number of ARGs were predicted to be located on the contigs carrying these replicons. As an expectation of this, multiple ARGs were identified on contigs with IncQ1 plasmid replicon in strains belonging to the monophasic variant of Salmonella Typhimurium. In general, high variability in ARGs and plasmid replicons content was observed among isolates, highlighting a high level of heterogeneity in Salmonella enterica. Irrespective of the serovar., many of the ARGs, especially those associated with critically and highly important antimicrobials for human medicine were located together with plasmid replicons, thus favoring their successful dissemination.
1. Introduction
In Europe, salmonellosis is the second most common zoonosis, with 87,923 confirmed cases in 2019 (1). In spite of more than 2,600 identified Salmonella serovars (2), only three serovars, namely S. enterica serovar Enteritidis (S. enteritidis), S. Typhimurium and the monophasic variant of S. Typhimurium (MVST), accounted for more than 70% of the human cases (1).
Most of the human cases of salmonellosis result in self-limiting gastrointestinal diseases, which does not require treatment with antimicrobials. However, treatment is required, when systemic infections occur, and it is therefore a serious health problem, when strains of important zoonotic pathogens, such as Salmonella, become resistant to commonly used antimicrobials. Antimicrobial overuse and misuse in humans and animals for food production is the main cause for the increase in antimicrobial resistance (AMR) in Salmonella (3), while mobile genetic elements (MGEs) have played a major role in the rapid spread of resistance genes among Salmonella strains (4).
Control strategies against salmonellosis have been implemented in the European countries at primary production level, with the aim of reducing the incidence of target serovars, i.e., serovars which have been designated as ‘particularly relevant for public health’ (Commission Regulation (EU) No 2160/2003, 5). This strategy is however challenged by the fact that serovars that used to be less frequently isolated from human specimens are being detected with increasing frequency (5, 6), also in animal populations in which national control plans to control Salmonella prevalence had been implemented (7). Importantly, Annex III from the Commission Regulation (EU) No 2160/2003 underlined that resistance(s) to relevant therapies for human infections was an important criterion to define which serovars with public health significance should be considered targets for the reduction of Salmonella prevalence in breeders population (8).
While phenotypic susceptibility testing has informed on the current level of resistance in strains of Salmonella, it does not have the power to inform on the underlying mechanisms behind AMR in strains, nor the mechanisms by which AMR spreads in the population. Whole genome sequencing (WGS) has therefore become a valuable support to phenotypic susceptibility testing in surveillance of AMR, allowing detection of the major antimicrobial resistance genes (ARGs) circulating in zoonotic pathogens and the MGEs which contribute to spread of AMR. The current study investigated the correlation between phenotypic and genotypic resistance to antimicrobials in a selection of Salmonella strains, isolated from humans, animals and food in Italy and belonging to serovars associated with different frequency of isolation from human infections. The study aimed to analyze the presence of AMR genes and MGEs in strains with AMR, in order to determine the resistance genes and plasmids, which seemed to contribute to the spread of resistant isolates.
2. Materials and methods
2.1. Dataset description
Eighty-eight Italian Salmonella enterica isolates, belonging to 15 different serovars, were selected. The serovars were chosen to represent serovars which are frequently (F) and rarely (R) isolated from human infections in the European Union (EU) countries (1). The serovars which are frequently isolated from human infections were represented by S. enteritidis, S. Typhimurium and MVST, while rarely isolated serovars from human infections were represented by S. Derby, S. Dublin, S. Hadar, S. infantis, S. Kentucky, S. Livingstone, S. Mbandaka, S. Montevideo, S. Newport, S. Rissen, S. Senftenberg, and S. Thompson. The strains were collected spanning the years 2009–2019, from different Italian regions, and were isolated from animals, food and human sources. The selected strains are part of a broader collection maintained at the National Reference Laboratory for Salmonellosis at the Istituto Zooprofilattico Sperimentale delle Venezie (Legnaro, Italy) and Istituto Superiore di Sanità (Rome, Italy). Two strains were selected for each serovar for each source (human, animal, and food), with the exception of S. Dublin and S. Mbandaka, for which only one human isolate was available (Table 1). The strains were maintained at the Istituto Zooprofilattico Sperimentale delle Venezie (IZSVe), in cryobank tubes at −80°C, with preservative medium (Copan Diagnostics, CA, United States).
2.2. Antimicrobial susceptibility testing
Antimicrobial susceptibility testing was performed as minimum inhibitory concentration (MIC) by broth microdilution method with Sensititre EUVSEC panel (TREK Diagnostics System). Results were interpreted according to European Committee on Antibiotic Susceptibility Testing (EUCAST) epidemiological cut-off values (ECOFFs; http://www.eucast.org). Multidrug-resistant (MDR) strains were defined as resistant to one drug in at least three different antimicrobial classes (9).
2.3. DNA extraction and WGS analysis
The Salmonella isolates were processed for DNA extraction and sequencing as already described in Petrin et al. (10). Briefly, after culturing, genomic DNA (gDNA) was extracted using a commercial column-based kit (QIAamp DNA Mini, QIAGEN, Valencia, CA), and purified gDNA was quantified with a Qubit 3.0 Fluorometer (Life Technologies, Waltham, MA). Libraries for WGS were prepared using the Nextera XT DNA sample preparation kit (Illumina, San Diego, CA) following the manufacturer’s instructions. High-throughput sequencing was performed with MiSeq Reagent kit v3, resulting in 251 bp long paired-end reads, or NextSeq High Output kit v2.5, resulting in 151 bp long paired end reads. Subsequent bioinformatics analyzes on raw reads were performed as previously described in Petrin et al. (11).
2.4. Genomic analyzes
To confirm the serovar., in-silico serotyping was performed using three different tools: MOST 1.0 (12) and SeqSero 1.0 (13) on raw data, and SISTR 1.0.2 (14) on assembled data.
Plasmid replicons were identified using blastn 2.7.1 (15) against PlasmidFinder 1.3 database [downloaded on 05/03/2018 (16)], while acquired antimicrobial resistance genes (ARGs) and chromosomal point mutations against ResFinder 3.0 and PointFinder databases, respectively [downloaded on 05/03/2018 (17)].
E-value thresholds were adjusted for each search depending on database size and were set as follows: 0.001 for plasmid replicons search, and 0.01 for ARGs search, respectively. All hits were required to have a 60% minimum coverage of the reference sequence found in the database, while the minimum required percentage of identity was 90% for plasmid replicons search, and 80% for ARGs search.
To further characterize plasmids that potentially contribute to the spread of antimicrobial resistance genes, only contigs longer that 200 bp were retained from the assemblies. Barrnap v0.9 (18) was used to mask ribosomal sequences on contigs. Contigs on which PlasmidFinder had identified a plasmid replicon were identified and collected to keep track of the incompatibility group(s) for each sample having putative plasmid(s).
A reference database containing plasmids from the taxa Enterobacteriaceae was built as follow:
1. Genebank (.gbk) format files for plasmids identified in taxa Enterobacteriaceae (Taxid 543) were downloaded from the NCBI nucleotide database;
2. only ‘complete sequence’ and ‘circular’ sequences were retained;
3. sequences were clustered using cd-hit v4.8.1 software (19) and setting 100% redundancy;
4. sequences were annotated with Plasmid Finder to search for the incompatibility group.
After building the reference database, blastN (15) was used to identify contigs that matched in the plasmid reference database with 90% identity and 90% coverage: if a contig matched with a plasmid in the reference database having an incompatibility group already identified by PlasmidFinder in that sample, the contig was retained and added to the contig identified by PlasmidFinder. All the contigs from one sample belonging to the same incompatibility group already identified by PlasmidFinder were concatenated by means of 150 bp Ns linkers. BlastN (15) was used to compare the resulting pseudomolecule for each incompatibility group with the plasmid reference database, in order to identify the best match (i.e., the match with the lowest e-value).
2.5. Conjugation assay, detection of plasmid replicon and antimicrobial resistance genes
In order to confirm the presence of ARGs on plasmids, for convenience reasons, two Salmonella isolates were chosen from those showing at least one ARG and a plasmid replicon on a putative plasmid from the in silico genomic analyzes. The transfer frequencies of tetA and catA1 genes were investigated by conjugation experiments with nalidixic acid resistant E. coli 1816 as recipient strain. Donors and recipient strains were grown in Luria-Bertani (LB) broth for 24 h at 37°C. Then, a 1:50 dilution was prepared for each strain, and bacteria were grown at 37°C to a final OD600 0.4. Five hundred μl of the donor strain was added to 4.5 mL of the recipient strain, and the bacterial suspensions were filtered using 0.22 μm filters (Merck Millipore) on MacConkey plates, pre-heated at 37°C for 1 h. After incubation for 18 h at 37°C, the filters were washed with 10 mL of physiological saline and vortexed to completely resuspend the cells. The cellular suspensions were centrifuged at 5000 rpm for 10 min, and the pellets resuspended in 1 mL of physiological saline after removing the supernatant. Serial dilutions were prepared, and 100 μL were plated on LB plates supplemented with nalidixic acid (50 mg/L) and chloramphenicol (50 mg/L) or nalidixic acid (50 mg/L) and tetracyclin (50 mg/L) to select for transconjugant colonies. The transfer frequencies were calculated as the number of transconjugants obtained per donor. Selected transconjugants colonies were transferred onto MacConkey agar plates to confirm they were E. coli colonies.
Identification of plasmid replicons from transconjugant colonies was performed by PCR-based replicon typing using the PBRT 2.0 kit (Diatheva, Fano, Italy), according to manufacturer’s instructions.
To screen for the presence of chloramphenicol resistance genes in transconjugant colonies, a multiplex PCR targeting catA1, cmlA1 and floR genes was performed according to the protocol described in Guerra et al. (20) using catA1, cmlA1 and floR forward and revers primers. PCR was performed in a final volume of 50 μL using 1X Buffer Taq Gold, 2 mM MgCl2, 400 μM dNTPs, 1 μM each primer, and 2,5 U Taq Gold (Life Technologies). Thermal cycling consisted of 95°C for 5 min, followed by 30 cycles (95°C for 30 s, 55°C for 30 s, 72°C for 40 s) and a final step at 72°C for 5 min. Finally, to screen for the presence of tetracycline resistance genes in transconjugant colonies, a multiplex PCR targeting tet genes was performed according to the protocol described by Ng et al. (21) using tetA, tetB and tetF forward and revers primers. PCR was performed in a final volume of 50 μL using 1X Buffer Taq Gold, 2 mM MgCl2, 200 μM dNTPs, 1 μM each primer, and 2,5 U Taq Gold (Life Technologies). Thermal cycling consisted of 95°C for 5 min, followed by 30 cycles (95°C for 30 s, 55°C for 30 s, 72°C for 30 s) and a final step at 72°C for 5 min. Amplicons were confirmed on a 2% agarose gel (Merck Life Science).
2.6. Statistical analysis
The data were statistically analyzed with RStudio (22) to generate plots, while graphical analysis was performed using the ggplot2 package (23). In order to evaluate the agreement between phenotypic and genotypic resistance, Cohen’s kappa statistics and value of p were calculated in RStudio using the vcd package (24). A kappa value between 0 and 1 is assigned and values ≤0 indicate no agreement; 0.01–0.20 none to slight agreement; 0.21–0.40 fair agreement; 0.41–0.60 moderate agreement; 0.61–0.80 substantial agreement; and values in the range of 0.81–1.00 indicate an almost perfect agreement (25).
3. Results
3.1. Phenotypic resistance to antimicrobials
Antimicrobial susceptibility test, performed with the EUVSEC panel, showed MIC values above the cut-off value to at least one antimicrobial molecule in 48 out of the 88 isolates. Results of MIC tests and definitions of susceptibility testing categories, according to epidemiological cut-off values, are reported in Supplementary Table S1.
Resistances to tetracycline, ampicillin, ciprofloxacin and sulfamethoxazole were common, with more than 20 resistant isolates each (Supplementary Table S1). None of the tested isolates showed resistance to ceftazidime, meropenem and tigecycline, while resistance to azithromycin was identified in one S. Rissen isolate, and resistance to cefotaxime was identified in one S. Derby and one S. infantis isolate.
S. Senftenberg isolates did not show resistance to any tested drugs, while S. Dublin (3 out of 5 isolates) and S. enteritidis (3 out of 6 isolates) showed resistance to colistin only (Figure 1). Only one isolate of S. Mbandaka showed resistance to antimicrobials, and this isolate was resistant to ampicillin and ciprofloxacin (Figure 1). The other tested serovars showed resistance to different antimicrobial molecules (Figure 1). Five out of 6 isolates of S. Hadar showed phenotypic resistance to ciprofloxacin and tetracycline, and three of them to ampicillin and nalidixic acid. S. infantis showed resistance to ciprofloxacin, nalidixic acid and trimethoprim (4 out of 6 isolates), to sulfamethoxazole and tetracycline (3 out of 6 isolates), to ampicillin (2 out of 6 isolates) and one isolate showed resistance to cefotaxime.
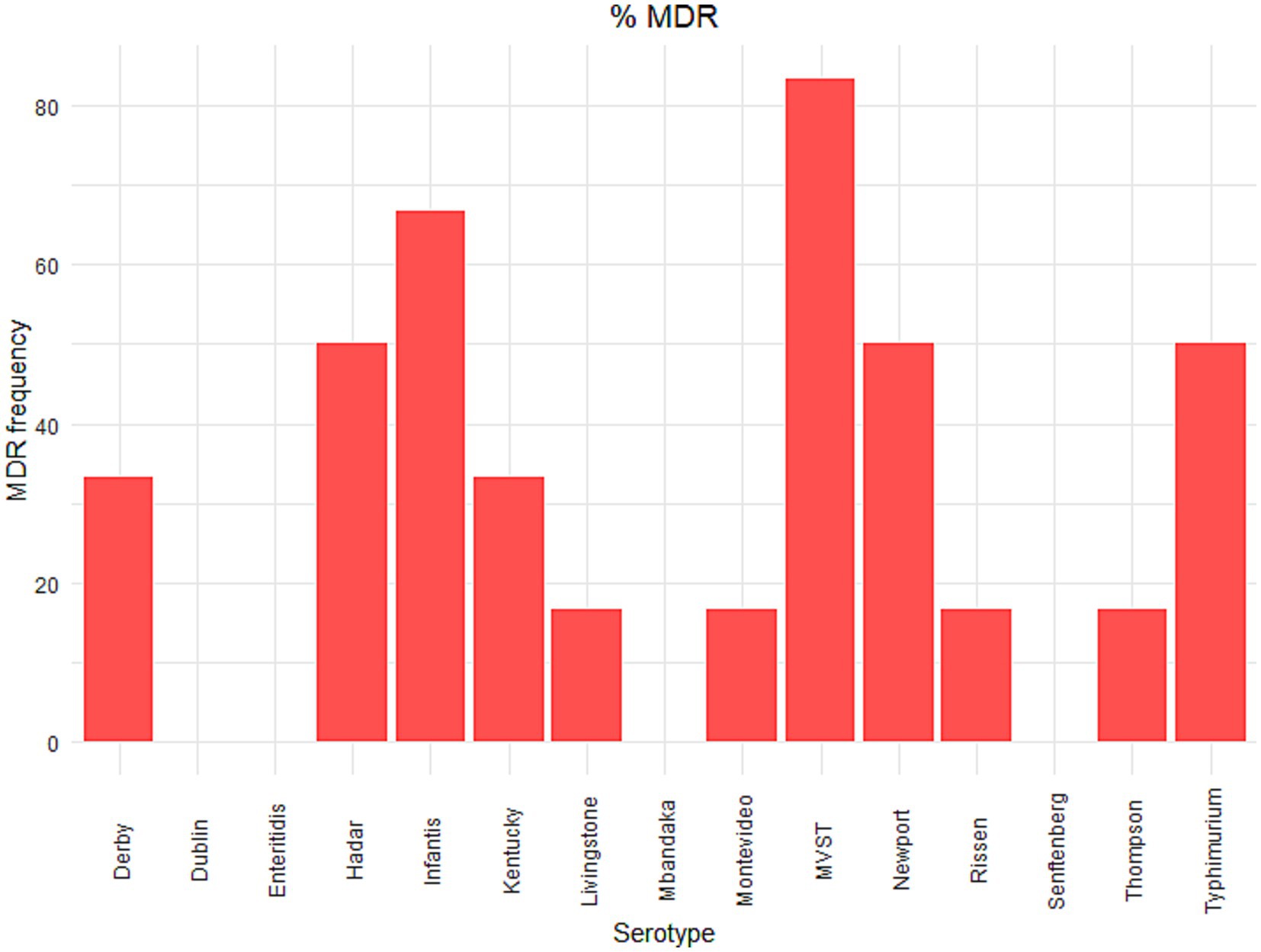
Figure 1. Phenotypic antimicrobial resistance in Salmonella serovars. The number of isolates resistant to antimicrobial molecules according to the European Committee on Antibiotic Susceptibility Testing (EUCAST) epidemiological cut-off values (ECOFFs; http://www.eucast.org). As described in Materials and Methods in the main text, two strains were selected for each serovar for each source (human, animal and food), with the exception of S. Dublin and S. Mbandaka, for which only one human isolate was available. Results of MIC tests and definitions of susceptibility testing categories, according to epidemiological cut-off values, are reported in Supplementary Table S1.
Five out of 6 isolates of S. Kentucky showed phenotypic resistance to ciprofloxacin and nalidixic acid, and two of them, both isolated from human specimens, also resistance to ampicillin, gentamycin, sulfamethoxazole and tetracycline. Resistance to ampicillin and sulfamethoxazole was present in all the tested MVST isolates, and five out of six isolates showed phenotypic resistance also to tetracycline. Finally, four out of six S. Typhimurium isolates showed resistance to ampicillin, three isolates to sulfamethoxazole and tetracycline and two isolates also to chloramphenicol and ciprofloxacin.
Of the isolates, which is a selection from a broader collection maintained at the National Reference Laboratory for Salmonellosis at the Istituto Zooprofilattico Sperimentale delle Venezie (Legnaro, Italy) and Istituto Superiore di Sanità (Rome, Italy), nine isolates from animals, nine isolates from food, and eight isolates from humans (25.9, 30.9, 33.3%, respectively) were multidrug-resistant. The percentage of strains within each serovar showing MDR are reported in Figure 2. The serovars with the highest number of MDR isolates were S. infantis and MVST.
3.2. Antimicrobial resistance genes
The dataset of genomic sequences was searched for the presence of known genes and chromosomal mutations conferring resistance to different classes of antimicrobials, and in total, 221 ARGs and AMR relevant point mutations were found (Table 1).
In details, 13 genes conferring resistance to aminoglycosides were identified and 6 genes conferring resistance to β-lactams. Among these, the most frequently identified ARGs to aminoglycosides were aph(3″)-Ib (strA) and aph (5)-Id (strB) identified in 19 genomic sequences, while the most common ARG to β-lactams was blaTEM-1B, found in 16 genomic sequences. The ARGs sul1 and sul2, conferring resistance to sulphonamides, were found in 11 and 13 genomic sequences, respectively. The ARGs tetA, tetB, tetG and tetD, conferring resistance to tetracyclines, were found in 18, 11, 2, and 2 genomic sequences, respectively. None of the selected samples presented mcr genes or mutation(s) in the chromosomal pmr genes, conferring resistance to colistin. When present, reduced susceptibility or resistance to (fluoro)quinolones was mainly caused by point mutations in gyrA (S83Y, D87N, D87Y, D87G, S83F) and parC (T57S) genes, while qnr genes were less commonly seen. Seven genomes were characterized by a point mutation in gyrA gene and a second mutation in gyrA, gyrB, or parC gene, while four genomes presented a point mutation in gyrA gene and qnr genes. This latter combination only confers reduced susceptibility to quinolones. Finally, genes conferring resistance to phenicol, trimethoprim, lincosamides and macrolides, and fosfomycin were present but in a limited number of genomic sequences (Table 2).
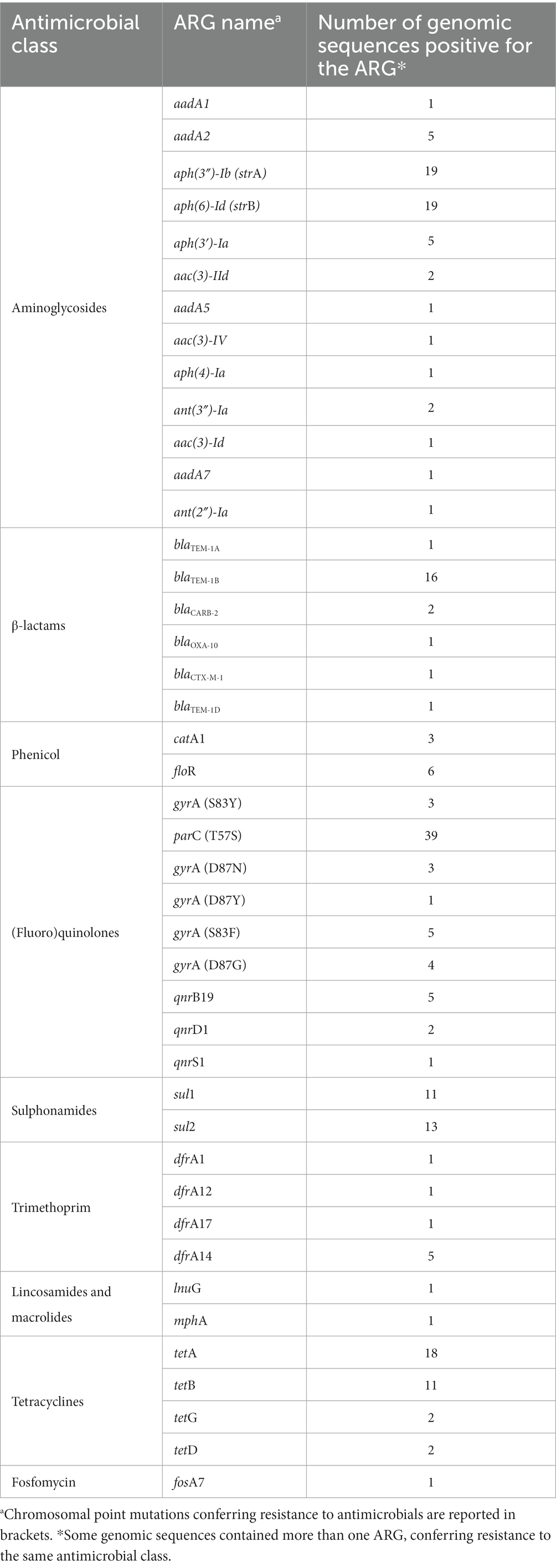
Table 2. Number of genomic sequences positive for ARGs and AMR relevant point mutations, divided by antimicrobial classes.
A substantial agreement with statistical significance between genotypic predictions and phenotypic resistance to ampicillin (k = 0.79, p–value <0.01), azithromycin (k = 0.66, p = 0.03), chloramphenicol (k = 0.78, p–value <0.01), sulfamethoxazole (k = 0.78 p–value <0.01) and tetracycline (k = 0.64, p–value <0.01) was observed. Only fair agreement was found for ciprofloxacin (k = 0.35, p–value <0.01), nalidixic acid (k = 0.37, p–value <0.01) and trimethoprim (k = 0.40, p–value <0.01) resistance, while no to slight agreement was observed for cefotaxime (k = 0.04, p–value = 0.498) and gentamicin (k = 0.15, p–value = 0.06) resistance (Table 3).
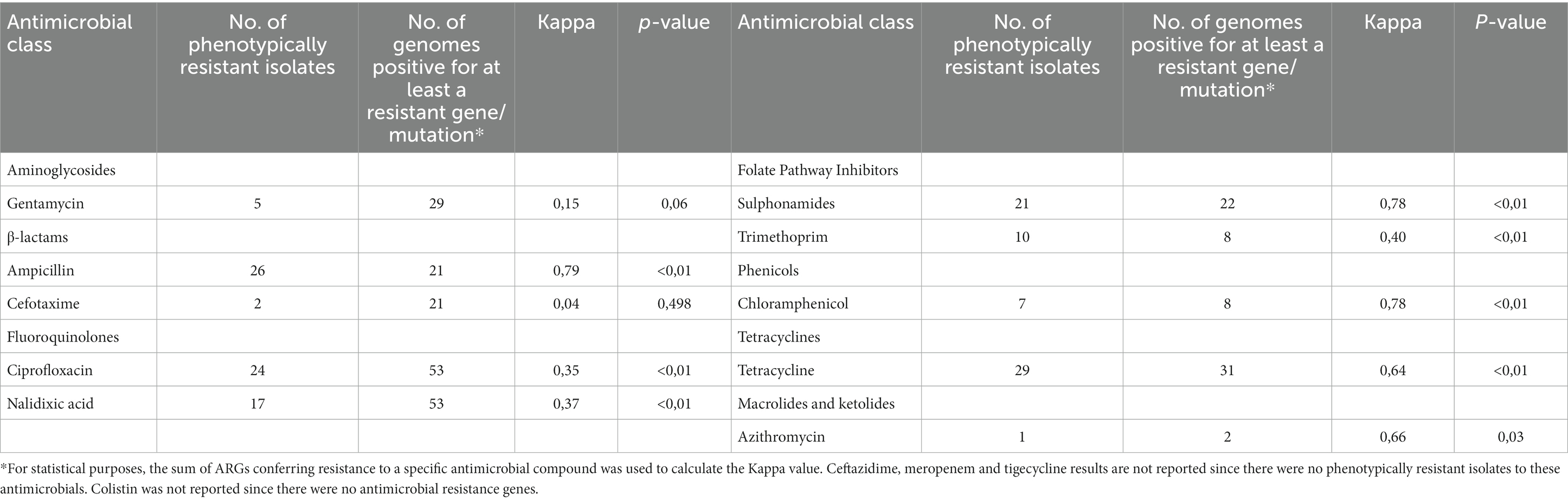
Table 3. Comparison of phenotypic and genotypic characterization of 88 Salmonella isolates and results of the Cohen’s kappa statistics and value of p used to evaluate the agreement between phenotypic and genotypic resistance.
The distribution of ARGs per serovar showed that strains of S. Dublin and S. enteritidis, in agreement with their lack of phenotypic resistances which are not associated with point mutations, were without antimicrobial resistance genes (Supplementary Figures S1–S15). The distribution of ARGs per source revealed similar profiles in animal, food and human strains. The most frequently identified ARGs in the three sources were sul1 and sul2, tetA and tetB, aph(3″)-lb (strA) and aph (5)-Id (strB), and the chromosomal point mutation in parC (T57S), potentially conferring reduced susceptibility to (fluoro)quinolons (Supplementary Figures S16–S18).
3.3. Plasmid replicons and co-location of plasmid replicons with ARGs
Among the 88 strains, 61 contained DNA sequences, which matched at least with one plasmid replicon (Supplementary Table S2). In total, 22 different plasmid replicons were detected, with Col(pHAD28), IncQ1 and IncFII(S) as the most frequently found (n = 20, n = 12 and n = 10 strains, respectively). In 20 sequences, at least two different plasmid replicons were detected and 5/20 contained IncX1 plasmid replicon. The frequency of detection of the plasmid replicon is reported in Table 4.
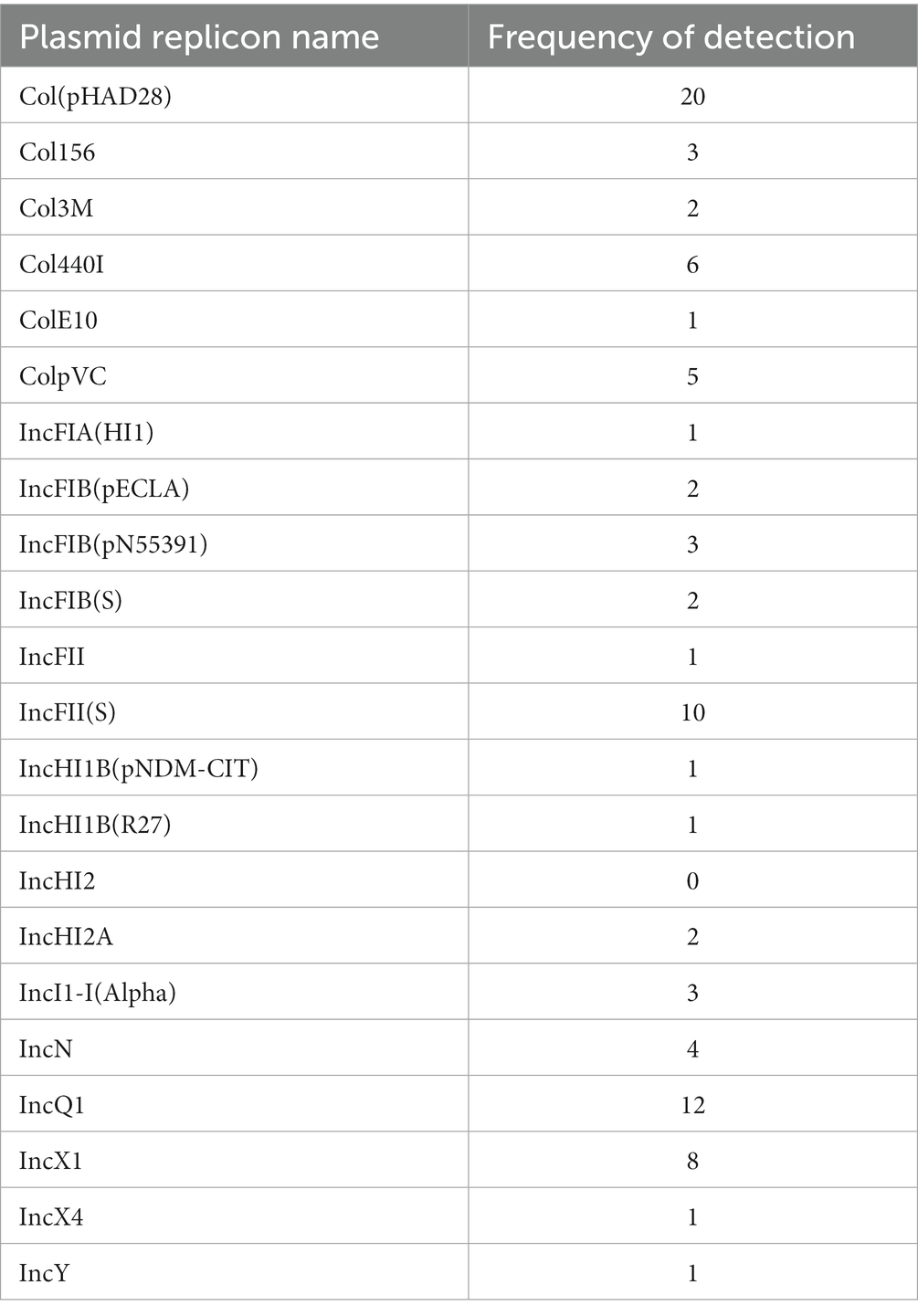
Table 4. Frequency of detection of plasmid replicons in strains of Salmonella, based on PlasmidFinder results.
Col(pHAD28) replicon plasmid was mainly found in S. Hadar (3/6) and S. Rissen (4/6) genomes, while IncFIB(pN55391) was only identified on S. infantis (3/6) genomes. IncX1 was predominantly found in S. Dublin (5/5) genomes, IncQ1 in MVST (6/6) genomes and IncFII(S) in S. Dublin (2/5), S. enteritidis (5/6) and S. Typhimurium (3/6) genomes (Supplementary Table S2).
In 14 genomic sequences, ARGs and at least one plasmid replicon were found to be located on the same contig, and only 3 strains carried plasmids with just one resistance gene [tetB (n = 1), floR (n = 2)]. Detailed information about the co-occurrence of plasmid replicons and ARGs on contigs scored as plasmid contigs is reported in Supplementary Table S3.
3.4. Conjugation assay and confirmation of transconjugants determinants
Conjugation experiments using E. coli 1816 as recipient strain were successful and frequencies of conjugation were calculated as being 3.45 transconjugants per donor for a S. Newport strain resistant to tetracycline, and 1.48E-06 transconjugants per donor for a S. Livingstone strain resistant to chloramphenicol.
Twelve E. coli transconjugant colonies selected from LB plates supplemented with nalidixic acid and chloramphenicol showed the catA1 gene amplicon and tetA gene was successfully amplified from sixteen E. coli transconjugant colonies selected from LB plates supplemented with nalidixic acid and tetracycline (Supplementary Figures S19, S20). The presence of IncN and IncHI2 plasmid replicons were confirmed in transconjugants from S. Newport and S. Livingstone strains, respectively.
4. Discussion
Antimicrobial resistance (AMR) occurs in microorganisms that become resistant to molecules intended to limit or prevent their growth, and it is considered a major threat to human and animal health (26, 27). In recent years, MDR has emerged as one of the most important threats to human health (28) and the spread of AMR is of particular concern in bacteria that represent common causes of infections in the human population, such as Salmonella spp. (29, 30).
Resistance levels in Salmonella vary by country, but on average 29,0%, 25,8% and 25,6% Salmonella isolates from human infections were reported to be resistant to sulphonamides, ampicillin and tetracyclines (30). The ability of Salmonella to acquire resistance genes from other bacteria is well described (4) and multidrug resistant (MDR) strains could cause infections that are more serious compared to those caused by pan-susceptible stains (28).
In this study, we characterized the phenotypic and genotypic antimicrobial resistance in a selection of Italian Salmonella isolates from human, food and animal sources. Moreover, WGS data were used to verify the co-occurrence of resistance gene and plasmids. Conjugation experiments confirming the plasmidic nature of ARGs were successfully carried out in two strains, where only one ARG and one replicon type were present, and transfer of resistance could firmly be linked to this MGE.
Only 48 strains among the 88 strains subjected to phenotypic testing were resistant to at least one antimicrobial, and among these isolates, 26 displayed MDR phenotype, with serovars S. infantis and MVST being the most MDR serovars. This level of MDR is similar to what has been reported at the EU level (1). In accordance with the reported data for the EU as a whole, MDR among Italian isolates was high among strains of MVST and S. infantis. Conversely, MDR is very high among isolates of S. Kentucky at the EU level [73.7% (31)], while the proportion was much lower in the studied samples, where only 33.3% of S. Kentucky isolates were MDR, probably reflecting the low number of strains analyzed in the current study.
Interestingly, the proportion of MDR isolates was found to be higher in isolates from food and human sources than from animals. This result opens to two possible scenarios: a) the ARGs stabilize in bacterial communities isolated from food handling environment, eventually reaching the final products. Indeed it is commonly recognized that meat from animals never treated with antibiotics could harbor antibiotic resistant bacteria, and b) other sources, for example, meat handlers or meat processing surfaces hosting resistant bacteria, could contribute to the ARGs stabilization in the bacterial communities in food processing environments (32). An alternative explanation could be the ban of antimicrobial as growth promoter in veterinary settings (33).
The proportion (33.3%), coherently with the Italian scenario, was slightly higher than what has been reported from the EU as a whole (31), and it was comparable to the proportion of MDR in food isolates (30.9%).
The degree of concordance observed between predictions of ARGs and resistance to a specific class of antimicrobial varied from no agreement, as in the case of colistin to substantial agreement, as in the case of ampicillin, chloramphenicol and sulfamethoxazole. Similar variability in agreement has been reported in other studies (34, 35). For those classes where agreement is high, surveillance for resistance by WGS of strains is a possibility, while care should be taken to base surveillance on this methodology for the classes with low agreement.
One possible explanation for low agreement between some resistance genes with the related phenotypes is a biological explanation. Indeed, ARGs could not be expressed due to the presence of weak or distant promoter or due to mutations in the promoter regions (36, 37). Alternatively, a technical explanation can be given: when the epidemiological cut-off values used to define whether an isolate is resistant or susceptible are higher than the resistance imparted by the resistance genes, isolates are classified as susceptible, as already described for aadA genes and streptomycin resistance (38).
Resistance to ampicillin was found in almost 30% of the isolates, and in 60% of these isolates, this was sustained by the presence of blaTEM-1B gene. Most of the isolates bearing the blaTEM-1B gene were of serovar MVST, S. Hadar, and S. Newport. Other bla genes were identified in genomes of isolates resistant to ampicillin. The TEM β-lactamase genes are usually carried by transposons (39) and found in plasmids (40), which increases the spread of this mechanism of resistance, posing a great concern for human health. Ampicillin is indeed classified as a critically important antimicrobial (CIA) by WHO (3), and the presence of blaTEM gene characterized pandemic clones such as the multiresistant MVST circulating in the European countries since 2006 (41).
Only two strains showed phenotypical resistance to cefotaxime, a third-generation cephalosporin, classified as highest priority CIA, and interestingly both were of human origin. For just one of them, an S. infantis isolate, it was possible to identify a blaCTX-M-1 gene responsible for the resistance. Also in this case, it is interesting to report that clonal lineages of ESBL-producing MDR S. infantis emerged recently in Italy and other European countries, causing human infections (42–44). Other identified genes conferring resistance to β-lactam antimicrobials (blaOXA and blaCARB) were only detected in few genomic sequences. Nonetheless, given they usually are carried by plasmids and other MGE (45), they could potentially be transferred to other enteric bacteria and limit the therapeutic treatments in case of severe human infections. On the genomic sequences from S. Derby, the second isolate phenotypically resistant to cefotaxime, we could not identify any resistance gene that confers resistance to cefotaxime (46). We could therefore hypothesize the expression of efflux pumps that contribute to resistance to cefotaxime in this case (47, 48).
Resistance to sulfamethoxazole was found in 24% of the tested isolates, most of which carried sul1 or sul2 resistance genes. These genes are indeed the most common in the analyzed genomic sequences, especially in serovars S. infantis, S. Typhimurium and its monophasic variant. It is interesting to note that nine strains showing sul2 gene located on plasmid contig were not phenotypically resistant to sulfamethoxazole. Further studies are needed to elucidate this finding.
Only five isolates (5.7%) were phenotypically resistant to gentamicin, however, ResFinder identified resistance genes [aadA2, aadA5, aadA7, aac(3)-Id, aac(3)-IId, aph(3′)-Ia, aph(3″)-Ib (strA), aph(6)-Id (strB), and ant(2″)-Ia] in only four of them. Multiple genes were found in the same isolate, as already reported by other studies (49, 50). For the isolate genomic sequence where we could not identify any resistance gene, the presence and expression of efflux pump, such as AcrD, can contribute to gentamicin resistance (51, 52). The agreement between genotypic and phenotypic resistance was indeed only slight. Moreover, there were different isolates in which resistance genes to aminoglycosides and streptomycin were identified (53), but which did not show phenotypic resistance to antimicrobials. It is possible that these isolates lack other components necessary to transfer an acetyl group that is required for the resistance mechanism of kanamycin, and further studies are needed to understand the lack of kanamycin resistance in these strains, as suggested by other authors (35). The most common detected resistance genes to aminoglycosides were aph(3″)-Ib (strA) and aph(6)-Id (strB) especially in genomes of S. Hadar and MVST serovars, while the resistance gene aadA2 was mainly identified in isolated from S. Derby and S. Typhimurium.
Aminoglycoside resistance genes are enlisted as current threats for human health, since they are commonly associated to ESKAPE pathogens (54). Finding these genes in Salmonella isolates highlights the need for an active surveillance of emerging resistances also in community associated bacteria.
Phenotypic resistance to ciprofloxacin and nalidixic acid was expressed by 27 and 19% of the isolates, respectively, while only a limited number of strains carried qnr resistance genes, a transferable resistance mechanisms responsible for reduced susceptibility to quinolones (55). Susceptibility to nalidixic acid co-occurring with resistance to ciprofloxacin was observed, corroborating the possible occurrence of plasmid mediated quinolone resistance mechanisms (30). One S. Mbandaka isolate carried a qnrS1 resistance gene, 2 S. Montevideo isolates carried a qnrD1 gene and five isolates a qnrB19 gene (S. Thompson (N = 1), S. Newport (N = 2) and S. Hadar (N = 2)). Interestingly, only one of the isolates carrying a qnr gene was of human origin and none of them belonged to S. enteritidis, which showed an increased proportion of resistant isolates in 2016 (56), nor to S. infantis or S. Kentucky serovars, in which resistance to (fluoro)quinolones is widespread (57). High level of ciprofloxacin resistance is observed in isolates with both qnr genes and chromosomal mutations, such as double substitutions in gyrA and a single substitution in parC genes, such in the case of the dominant clone of S. Kentucky ST198 in Europe (57, 58). Indeed, point mutations in DNA gyrase A (gyrA gene, position 83 or 87) and topoisomerase C (parC gene, at position 57) were present in different Italian isolates and also in the Salmonella genomes from different European countries, especially S. infantis and S. Kentucky sequences (58, 59). Of interest, a high number of genomes showed a point mutation at position 57 of parC gene (T57S, n = 39). Previous report showed that parC T57S is a spontaneous compensatory mutation, resulting in resistance to nalidixic acid but sensitivity to ciprofloxacin in Salmonella isolates (60, 61). However, accumulation of mutations in gyrA or parC genes, together with parC T57S, resulted in complete resistance to ciprofloxacin in different Salmonella serovars (62, 63). The resistance to quinolones has been widely reported in Salmonella serovars, especially in serovars frequently isolated from poultry sources such as S. infantis and S. Kentucky, probably due to the selective pressure exerted on the microbial communities of poultry farming where the use of quinolones as therapeutic are still present (64). As for aminoglycoside resistance genes, also the presence of mobile genes such as qnr (S and B) are ranked among the current threads having the potential to contribute to MDR in pathogens (54).
Resistance to trimethoprim, encoded by dfrA1, dfrA12, dfrA17, dfrA14 genes, was identified in 11,4% of the Italian isolates and this level of resistance has been confirmed at the European level, also in successful epidemic clones (65, 66). Interestingly, two isolates, which showed phenotypic resistance to trimethoprim, lacked resistance genes to the molecule. Further investigations are needed to explore the possibility of efflux pumps or other mechanisms that could explain this phenomenon. The resistance to trimethoprim was particularly common among isolates of S. infantis, as already demonstrated (66). This high level of strains carrying trimethoprim resistance genes is quite alarming as these genes are enlisted among the Rank I AMR genes contributing to MDR in human pathogens (54). This is of particular relevance in Salmonella as the serovar mainly displaying these genes is S. infantis, a serovar with high potential of causing severe infections in humans and well known to carry SGI and large plasmids harboring MRG cassettes (58, 67).
Resistance to chloramphenicol was sustained by catA1 (n = 3) and floR (n = 6) genes in the Italian isolates, while at the European level also cmlA1 gene was widespread. Chloramphenicol is not used for treatment of humans due to toxicity risks, however, this drug class is classified as highly important antimicrobial for human health (3). Epidemic clones of chloramphenicol-resistant Salmonella, such as S. Typhimurium ST313 in Africa (68), S. Typhimurium DT104 (69), and even S. typhi, have emerged and chloramphenicol resistance genes are often carried in plasmids, together with other genes conferring resistance to streptomycin, sulfonamides, and tetracyclines (70).
Tetracycline resistance was confirmed in 33% of the Italian isolates, where tetA and tetB genes were identified. While tetA was identified in different serovars, such as S. Hadar, S. infantis, S. Newport, S. Rissen, tetB was predominantly identified in S. Typhimurium and its monophasic variant. The reasons for this different occurrence are not known, however, multiple studies showed the presence of tetB in clinically relevant clone of S. Typhimurium and its monophasic variant (71–74). In the current study, tetA was shown to be present on a conjugative plasmid in a tetracycline resistant strain of S. Newport, and this plasmid transferred resistance with high frequency to a strain of E. coli, suggesting that such plasmid confer high ability of spread of resistance.
Interestingly, resistance to colistin was identified only in eight isolates, most of which were of serovars S. Dublin and S. enteritidis. None of the isolates showed relevant chromosomal mutations or acquired mcr genes. These serovars belong to group D Salmonella, which are characterized by a decreased susceptibility to colistin, due to the presence of abequose, the dideoxyhexose characterizing O-antigen epitope of this group (75, 76). Despite the increasing number of Salmonella isolates carrying mcr genes conferring resistance to colistin (77–81), and the diverse variants of mcr genes (82), we did not identify any mcr variant in the studied genomes.
Many of the resistance genes identified in the studied genomes are usually located on plasmids, that play a major role in evolution and horizontal gene transfer of bacterial antimicrobial resistance (83). Plasmid replicons were indeed detected in 69% of the genomic sequences and belonging to all the selected serovars. Of note, plasmid replicons (Inc groups) were in most of the cases associated with only one serovar., with the exception of IncFIB(S) and IncFII(S), that were identified in both S. enteritidis and S. Typhimurium and IncX1, that was identified in both S. Dublin and S. Kentucky. Previous reports demonstrated that certain serovars presented specific incompatible plasmids (83–86). We developed a workflow to map the plasmid replicons against known Salmonella plasmids, and all the identified replicon carrying contigs showed similarity to published plasmid sequences Salmonella enterica strains.
The resistance genes located on the same contig(s) as plasmid replicons were encoded on IncQ1 plasmid type, mainly harbored by genomes of the MVST. Interestingly, multiple resistance genes (sul2, aph(3″)-I and aph (6)-Ic) were identified on such IncQ1 contigs. IncQ1 plasmids are present in 4–12 copies/cell and have a size range from 8 to 14 kb (83) and were reported to carry bla genes (87) or the sul2-strA-strB cluster (88, 89). Of note, bla genes were not located on contigs carrying plasmid replicons, such as IncX plasmids, which usually carry resistance genes to β-lactams and quinolones (83). Similar IncX1 plasmids were already identified in Salmonella and E. coli strains. Interestingly, tet genes, usually found on plasmids, were not detected on IncQ1 plasmids. Recently, Oliva et al. (90) reported a novel IncQ1 plasmid carrying tet genes and postulated that recombination between a recipient IncQ1 plasmid and the tetR-tetA gene cluster had occurred. We did not search for recombination events nor genetic elements that could favor recombination, however it is worth noting that plasticity in bacteria genomes could likely mobilize such regions and contribute to the spread of plasmids with multiple resistance genes. IncQ1 contigs were found to match with plasmids already identified in E. coli and K. pneumoniae (91, 92).
Surprisingly, only a limited number of replicon containing contigs, with the exception of contigs found in MVST and S. Typhimurium genomes, were predicted to have ARGs. This however can be explained by the multiple mechanism by which antimicrobial resistance could arise in Salmonella. Besides horizontal transfer, also translocation from plasmids to chromosome has been described, creating clusters or antimicrobial resistance islands that are now regarded as an efficient means of resistance genes dissemination (67, 93, 94). Moreover, MGEs together with integrons, transposons and insertion sequences, favoring genetic recombination mechanisms, facilitate the accumulation on resistance islands (70, 95, 96). Another explanation could be that multiple resistance genes are carried on very large plasmids, as in the case of the pESI megaplasmid in S. infantis (42, 97). Such mega plasmids, with sizes ranging from 280 to 320 kb, unlikely would be completely assembled from short-read sequencing technology, such as used in the current study. It is therefore possible that plasmid replicon and antimicrobial resistance gene(s) would be identified in different contigs, hampering the association between plasmid and resistances (98).
The advent of WGS has enabled the prediction of AMR and antimicrobial resistance surveillance from genomic data alone (99), demonstrating high concordance between the presence of known ARGs or mutations and MIC of several antimicrobials (100). Despite the need to harmonize and standardize pipelines and databases, one of the most important advantage of WGS for AMR surveillance is the unprecedented level of detail in one assay, that made it possible also to define multidrug-resistance with great precision compared to phenotypic tests, allowed the description of current and emerging trends in AMR and allowed to trace specific allele profiles, rather than just phenotypic patterns by drug class (100).
5. Conclusion
Salmonella enterica represents an extremely heterogeneous species, and diseases caused by non-typhoidal Salmonella serovars vary considerably, with some serovars being significantly more prone to cause infections in humans. The reasons behind this are not completely understood, even if virulence mechanisms and genetic differences are believed to contribute to its success (101). In this paper, we described the variability in resistance genes and potential plasmids that characterize a set of Italian Salmonella isolates. Many of the identified genes, especially those that confer resistance to critically and highly important antimicrobials for human medicine were located together with plasmid replicons on contigs, which mapped to known plasmid sequences, and such plasmids can potentially favor in the spread and dissemination of ARGs. Indeed, genome plasticity, even more if associated to multidrug resistance, seems to be an important characteristic of successful Salmonella clones, regardless of the serovar.
Data availability statement
The datasets presented in this study can be found in online repositories. The names of the repository/repositories and accession number(s) can be found at: [https://www.ncbi.nlm.nih.gov/bioproject/ and PRJNA817603].
Author contributions
SP conceived the work, performed the experiments and wrote the manuscript. MO and AM performed the analyzes. JO, CL, and LB critically reviewed the manuscript. CL and LB concepted the work. All authors approved the final version of the manuscript.
Funding
This research was supported by EU funding within the NextGenerationEU-MUR PNRR Extended Partnership initiative on Emerging Infectious Diseases (Project no. PE00000007, INF-ACT).
Acknowledgments
Laura Villa and Ida Luzzi (Istituto Superiore di Sanità, Rome, Italy) are warmly thanked for providing the human isolates. Eleonora Mastrorilli (EMBL, Heidelberg, Germany) is greatly acknowledged for her help with the bioinformatics analyzes.
Conflict of interest
The authors declare that the research was conducted in the absence of any commercial or financial relationships that could be construed as a potential conflict of interest.
Publisher’s note
All claims expressed in this article are solely those of the authors and do not necessarily represent those of their affiliated organizations, or those of the publisher, the editors and the reviewers. Any product that may be evaluated in this article, or claim that may be made by its manufacturer, is not guaranteed or endorsed by the publisher.
Supplementary material
The Supplementary material for this article can be found online at: https://www.frontiersin.org/articles/10.3389/fpubh.2023.1221351/full#supplementary-material
References
1. EFSA and ECDC. The European Union one health 2019 zoonoses report. EFSA J. (2021) 19:6406. doi: 10.2903/j.efsa.2021.6406
2. Grimont, PAD, and Weill, F-X. Antigenic Formulae of the Salmonella Serovars. 9th Edn. Paris: Institut Pasteur, pp. 1–166. (2007).
3. World Health Organization. Critically important antimicrobials for human medicine. Geneva: World Health Organization (2019).
4. Partridge, SR, Kwong, SM, Firth, N, and Jensen, SO. Mobile genetic elements associated with antimicrobial resistance. Clin. Microbiol. Rev. (2018) 31:e00088. doi: 10.1128/CMR.00088-17
5. Graziani, C, Luzzi, I, Owczarek, S, Dionisi, AM, and Busani, L. Salmonella enterica serovar Napoli infection in Italy from 2000 to 2013: spatial and spatio-temporal analysis of cases distribution and the effect of human and animal density on the risk of infection. PLoS One. (2015) 10:e0142419. doi: 10.1371/journal.pone.0142419
6. Sabbatucci, M, Dionisi, AM, Pezzotti, P, Lucarelli, C, Barco, L, Mancin, M, et al. Molecular and epidemiologic analysis of reemergent Salmonella enterica serovar Napoli, Italy, 2011–2015. Emerg Infect Dis. (2018) 24:562–5. doi: 10.3201/eid2403.171178
7. Leati, M, Zaccherini, A, Ruocco, L, D’Amato, S, Busani, L, Villa, L, et al. The challenging task to select Salmonella target serovars in poultry: the Italian point of view. Epidemiol Infect. (2021) 149:e160. doi: 10.1017/S0950268821001230
8. European Parliament. Regulation (EC) No 2160/2003 of the European Parliament and of the Council of 17 November 2003 on the control of salmonella and other specified food-borne zoonotic agents. (2003).
9. Magiorakos, AP, Srinivasan, A, Carey, RB, Carmeli, Y, Falagas, ME, Giske, CG, et al. Multidrug-resistant, extensively drug-resistant and pandrug-resistant bacteria: an international expert proposal for interim standard definitions for acquired resistance. Clin Microbiol Infect. (2012) 18:268–81. doi: 10.1111/j.1469-0691.2011.03570.x
10. Petrin, S, Mancin, M, Losasso, C, Deotto, S, Olsen, JE, and Barco, L. Effect of pH and salinity on the ability of Salmonella serotypes to form biofilm. Front Microbiol. (2022) 13:821679. doi: 10.3389/fmicb.2022.821679
11. Petrin, S, Wijnands, L, Benincà, E, Mughini-Gras, L, Delfgou-van Asch, EHM, Villa, L, et al. Assessing phenotypic virulence of Salmonella enterica across serovars and sources. Front Microbiol. (2023) 14:1184387. doi: 10.3389/fmicb.2023.1184387
12. Tewolde, R, Dallman, TJ, Schaefer, U, Sheppard, CL, Ashton, PM, Pichon, B, et al. MOST: A modified MLST typing tool based on short read sequencing. PeerJ. (2016) 4:e2308. doi: 10.7717/peerj.2308
13. Zhang, S, Yin, Y, Jones, MB, Zhang, Z, Kaiser, BLD, Dinsmore, BA, et al. Salmonella serotype determination utilizing high-throughput genome sequencing data. J Clin Microbiol. (2015) 53:1685–92. doi: 10.1128/JCM.00323-15
14. Wen, SCH, and Best, E. Non-typhoidal Salmonella infections in children: review of literature and recommendations for management. J Paediatr Child Health. (2017) 53:936–41. doi: 10.1111/jpc.13585
15. Altschul, SF, Gish, W, Miller, W, Myers, EW, and Lipman, DJ. Basic local alignment search tool. J Mol Biol. (1990) 215:403–10. doi: 10.1016/S0022-2836(05)80360-2
16. Carattoli, A, Zankari, E, Garciá-Fernández, A, Larsen, MV, Lund, O, Villa, L, et al. In silico detection and typing of plasmids using plasmidfinder and plasmid multilocus sequence typing. Antimicrob Agents Chemother. (2014) 58:3895–903. doi: 10.1128/AAC.02412-14
17. Zankari, E, Hasman, H, Cosentino, S, Vestergaard, M, Rasmussen, S, Lund, O, et al. Identification of acquired antimicrobial resistance genes. J Antimicrob Chemother. (2012) 67:2640–4. doi: 10.1093/jac/dks261
19. Fu, L, Niu, B, Zhu, Z, Wu, S, and Li, W. CD-HIT: accelerated for clustering the next-generation sequencing data. Bioinformatics. (2012) 28:3150–2. doi: 10.1093/bioinformatics/bts565
20. Guerra, B, Soto, SM, Argüelles, JM, and Mendoza, MC. Multidrug resistance is mediated by large plasmids carrying a class 1 Integron in the Emergent Salmonella enterica serotype [4,5,12:i:−]. Antimicrob Agents Chemother. (2001) 45:1305–8. doi: 10.1128/AAC.45.4.1305-1308.2001
21. Ng, L-K, Mulvey, MR, Martin, I, Peters, GA, and Johnson, W. Genetic characterization of antimicrobial resistance in Canadian isolates of Salmonella Serovar Typhimurium DT104. Antimicrob Agents Chemother. (1999) 43:3018–21. doi: 10.1128/AAC.43.12.3018
22. RStudio Team. RStudio: integrated development environment for R. Boston, MA: RStudio, PBC (2021).
25. McHugh, ML. Interrater reliability: the kappa statistic. Biochem Med. (2012) 22:276–82. doi: 10.11613/BM.2012.031
26. Baker, S, Thomson, NR, Weill, FX, and Holt, KE. Genomic insights into the emergence and spread of antimicrobial-resistant bacterial pathogens. Science. (2018) 360:733–8. doi: 10.1126/science.aar3777
27. Michael, CA, Dominey-Howes, D, and Labbate, M. The antimicrobial resistance crisis: causes, consequences, and management. Front Public Health. (2014) 2:145. doi: 10.3389/fpubh.2014.00145
28. Parisi, A, Crump, JA, Glass, K, Howden, BP, Furuya-Kanamori, L, Vilkins, S, et al. Health outcomes from multidrug-resistant Salmonella infections in high-income countries: a systematic review and Meta-analysis. Foodborne Pathog Dis. (2018) 15:428–36. doi: 10.1089/fpd.2017.2403
29. Huedo, P, Gori, M, Amato, E, Bianchi, R, Valerio, E, Magnoli, L, et al. A multischool outbreak due to Salmonella enterica serovar napoli associated with elevated rates of hospitalizations and bacteremia, Milan, Italy, 2014. Foodborne Pathog Dis. (2016) 13:417–22. doi: 10.1089/fpd.2015.2091
30. EFSA and ECDC. The European Union summary report on antimicrobial resistance in zoonotic and indicator bacteria from humans, animals and food in 2017/2018. EFSA J. (2020) 18:e06007. doi: 10.2903/j.efsa.2020.6007
31. EFSA and ECDC. The European Union summary report on antimicrobial resistance in zoonotic and indicator bacteria from humans, animals and food in 2018/2019. EFSA J. (2021) 19:6490. doi: 10.2903/j.efsa.2021.6490
32. Salerno, B, Furlan, M, Sabatino, R, Di Cesare, A, Leati, M, Volanti, M, et al. Antibiotic resistance genes load in an antibiotic free organic broiler farm. Poult Sci. (2022) 101:101675. doi: 10.1016/j.psj.2021.101675
33. European Parliament. Regulation (EC) No 1831/2003 of the European Parliament and of the Council of 22 September 2003 on additives for use in animal nutrition. (2003).
34. Viana, C, Grossi, JL, Sereno, MJ, Yamatogi, RS, Bersot, L, Dos, S, et al. Phenotypic and genotypic characterization of non-typhoidal Salmonella isolated from a Brazilian pork production chain. Food Res Int. (2020) 137:109406. doi: 10.1016/j.foodres.2020.109406
35. Jibril, AH, Okeke, IN, Dalsgaard, A, Menéndez, VG, and Olsen, JE. Genomic analysis of antimicrobial resistance and resistance plasmids in Salmonella Serovars from poultry in Nigeria. Antibiotics. (2021) 10:99. doi: 10.3390/antibiotics10020099
36. Enne, VI, Delsol, AA, Roe, JM, and Bennett, PM. Evidence of antibiotic resistance gene silencing in Escherichia coli. Antimicrob Agents Chemother. (2006) 50:3003–10. doi: 10.1128/AAC.00137-06
37. Rosengren, LB, Waldner, CL, and Reid-Smith, RJ. Associations between antimicrobial resistance phenotypes, antimicrobial resistance genes, and virulence genes of fecal Escherichia coli isolates from healthy grow-finish pigs. Appl Environ Microbiol. (2009) 75:1373–80. doi: 10.1128/AEM.01253-08
38. Sunde, M, and Norström, M. The genetic background for streptomycin resistance in Escherichia coli influences the distribution of MICs. J Antimicrob Chemother. (2005) 56:87–90. doi: 10.1093/jac/dki150
39. Bailey, JK, Pinyon, JL, Anantham, S, and Hall, RM. Distribution of the blaTEM gene and blaTEM-containing transposons in commensal Escherichia coli. J Antimicrob Chemother. (2011) 66:745–51. doi: 10.1093/jac/dkq529
40. Poirel, L, Bonnin, RA, and Nordmann, P. Genetic support and diversity of acquired extended-spectrum β-lactamases in gram-negative rods. Infect Genet Evol. (2012) 12:883–93. doi: 10.1016/j.meegid.2012.02.008
41. Hopkins, KL, Kirchner, M, Guerra, B, Granier, SA, Lucarelli, C, Porrero, MC, et al. Multiresistant Salmonella enterica serovar 4,[5],12:i:- in Europe: A new pandemic strain? Eur Secur. (2010) 15:19580. doi: 10.2807/ese.15.22.19580-en
42. Franco, A, Leekitcharoenphon, P, Feltrin, F, Alba, P, Cordaro, G, Iurescia, M, et al. Emergence of a clonal lineage of multidrug-resistant ESBL-producing Salmonella Infantis transmitted from broilers and broiler meat to humans in Italy between 2011 and 2014. PLoS One. (2015) 10:e0144802. doi: 10.1371/journal.pone.0144802
43. Hindermann, D, Gopinath, G, Chase, H, Negrete, F, Althaus, D, Zurfluh, K, et al. Salmonella enterica serovar infantis from food and human infections, Switzerland, 2010-2015: poultry-related multidrug resistant clones and an emerging ESBL producing clonal lineage. Front Microbiol. (2017) 8:1322. doi: 10.3389/fmicb.2017.01322
44. Bogomazova, AN, Gordeeva, VD, Krylova, EV, Soltynskaya, IV, Davydova, EE, Ivanova, OE, et al. Mega-plasmid found worldwide confers multiple antimicrobial resistance in Salmonella Infantis of broiler origin in Russia. Int J Food Microbiol. (2020) 319:108497. doi: 10.1016/j.ijfoodmicro.2019.108497
45. Carattoli, A. Plasmids in gram negatives: molecular typing of resistance plasmids. Int J Med Microbiol. (2011) 301:654–8. doi: 10.1016/j.ijmm.2011.09.003
46. Hussain, HI, Aqib, AI, Seleem, MN, Shabbir, MA, Hao, H, Iqbal, Z, et al. Genetic basis of molecular mechanisms in β-lactam resistant gram-negative Bacteria. Microb Pathog. (2021) 158:105040. doi: 10.1016/j.micpath.2021.105040
47. Lou, H, Chen, M, Black, SS, Bushell, SR, Ceccarelli, M, Mach, T, et al. Altered antibiotic transport in OmpC mutants isolated from a series of clinical strains of multi-drug Resistant E. coli. PLoS One. (2011) 6:e25825. doi: 10.1371/journal.pone.0025825
48. Padilla, E, Llobet, E, Doménech-Sánchez, A, Martínez-Martínez, L, Bengoechea, JA, and Albertí, S. Klebsiella pneumoniae AcrAB efflux pump contributes to antimicrobial resistance and virulence. Antimicrob Agents Chemother. (2012) 54:177–83. doi: 10.1128/AAC.00715-09
49. Mastrorilli, E, Pietrucci, D, Barco, L, Ammendola, S, Petrin, S, Longo, A, et al. A comparative genomic analysis provides novel insights into the ecological success of the monophasic Salmonella Serovar 4,[5],12:i. Front Microbiol. (2018) 9:715. doi: 10.3389/fmicb.2018.00715
50. McMillan, EA, Gupta, SK, Williams, LE, Jové, T, Hiott, LM, Woodley, TA, et al. Antimicrobial resistance genes, cassettes, and plasmids present in Salmonella enterica associated with United States food animals. Front Microbiol. (2019) 10:832. doi: 10.3389/fmicb.2019.00832
51. Rosenberg, EY, Ma, D, and Nikaido, H. AcrD of Escherichia coli is an aminoglycoside efflux pump. J Bacteriol. (2000) 182:1754–6. doi: 10.1128/JB.182.6.1754-1756.2000
52. Eaves, DJ, Ricci, V, and Piddock, LJV. Expression of acrB, acrF, acrD, marA, and soxS in Salmonella enterica Serovar Typhimurium: role in multiple antibiotic resistance. Antimicrob Agents Chemother. (2004) 48:1145–50. doi: 10.1128/AAC.48.4.1145-1150.2004
53. Ramirez, MS, and Tolmasky, ME. Aminoglycoside modifying enzymes. Drug Resist Updat. (2010) 13:151–71. doi: 10.1016/j.drup.2010.08.003
54. Zhang, A-N, Gaston, JM, Dai, CL, Zhao, S, Poyet, M, Groussin, M, et al. An omics-based framework for assessing the health risk of antimicrobial resistance genes. Nat Commun. (2021) 12:25096. doi: 10.1038/s41467-021-25096-3
55. Veldman, K, Cavaco, LM, Mevius, DJ, Battisti, A, Franco, A, Botteldoorn, N, et al. International collaborative study on the occurrence of plasmid-mediated quinolone resistance in Salmonella enterica and Escherichia coli isolated from animals, humans, food and the environment in 13 European countries. J Antimicrob Chemother. (2011) 66:1278–86. doi: 10.1093/jac/dkr084
56. EFSA and ECDC. The European Union summary report on trends and sources of zoonoses, zoonotic agents and food-borne outbreaks in 2017. EFSA J. (2018) 16:e05500. doi: 10.2903/j.efsa.2018.5500
57. EFSA and ECDC. The European Union summary report on antimicrobial resistance in zoonotic and indicator bacteria from humans, animals and food in 2017. EFSA J. (2019) 17:e05598. doi: 10.2903/j.efsa.2019.5598
58. Le Hello, S, Bekhit, A, Granier, SA, Barua, H, Beutlich, J, Zajaṃc, M, et al. The global establishment of a highly-fluoroquinolone resistant Salmonella enterica serotype Kentucky ST198 strain. Front Microbiol. (2013) 4:395. doi: 10.3389/fmicb.2013.00395
59. Ceyssens, P-J, Mattheus, W, Vanhoof, R, and Bertrand, S. Trends in serotype distribution and antimicrobial susceptibility in salmonella enterica isolates from humans in Belgium, 2009 to 2013. Antimicrob Agents Chemother. (2015) 59:544–52. doi: 10.1128/AAC.04203-14
60. Smith, AM, Govender, N, and Keddy, KH. Quinolone-resistant Salmonella Typhi in South Africa, 2003-2007. Epidemiol Infect. (2010) 138:86–90. doi: 10.1017/S0950268809990331
61. Eaves, DJ, Randall, LP, Gray, DT, Buckley, AM, Woodward, MJ, White, AP, et al. Prevalence of mutations within the quinolone resistance-determining region of gyrA, gyrB, parC, and parE and association with antibiotic resistance in quinolone-resistant Salmonella enterica. Antimicrob Agents Chemother. (2004) 48:4012–5. doi: 10.1128/AAC.48.10.4012-4015.2004
62. Qian, H, Cheng, S, Liu, G, Tan, Z, Dong, C, Bao, J, et al. Discovery of seven novel mutations of gyrB, parC and parE in Salmonella Typhi and Paratyphi strains from Jiangsu Province of China. Sci Rep. (2020) 10:1–8. doi: 10.1038/s41598-020-64346-0
63. Campos, MJ, Palomo, G, Hormeño, L, Herrera-León, S, Domínguez, L, Vadillo, S, et al. Prevalence of quinolone resistance determinants in non-typhoidal Salmonella isolates from human origin in Extremadura, Spain. Diagn Microbiol Infect Dis. (2014) 79:64–9. doi: 10.1016/j.diagmicrobio.2014.01.010
64. Gouvêa, R, Dos Santos, FF, De Aquino, M, and de Pereira, VL. Fluoroquinolones in industrial poultry production, bacterial resistance and food residues: a review. Brazilian. J Poult Sci. (2015) 17:1–10. doi: 10.1590/1516-635x17011-10
65. Cadel-Six, S, Cherchame, E, Douarre, PE, Tang, Y, Felten, A, Barbet, P, et al. The spatiotemporal dynamics and microevolution events that favored the success of the highly clonal multidrug-resistant monophasic Salmonella Typhimurium circulating in Europe. Front Microbiol. (2021) 12:12. doi: 10.3389/fmicb.2021.651124
66. Mughini-Gras, L, van Hoek, AHAM, Cuperus, T, Dam-Deisz, C, van Overbeek, W, van den Beld, M, et al. Prevalence, risk factors and genetic traits of Salmonella Infantis in Dutch broiler flocks. Vet Microbiol. (2021) 258:109120. doi: 10.1016/j.vetmic.2021.109120
67. Mulvey, MR, Boyd, DA, Olson, AB, Doublet, B, and Cloeckaert, A. The genetics of Salmonella genomic island 1. Microbes Infect. (2006) 8:1915–22. doi: 10.1016/j.micinf.2005.12.028
68. Okoro, CK, Kingsley, RA, Connor, TR, Harris, SR, Parry, CM, Al-Mashhadani, MN, et al. Intracontinental spread of human invasive Salmonella Typhimurium pathovariants in sub-Saharan Africa. Nat Genet. (2012) 44:1215–21. doi: 10.1038/ng.2423
69. Threlfall, EJ. Epidemic Salmonella Typhimurium DT 104–a truly international multiresistant clone. J Antimicrob Chemother. (2000) 46:7–10. doi: 10.1093/jac/46.1.7
70. Crump, JA, Sjölund-Karlsson, M, Gordon, MA, and Parry, CM. Epidemiology, clinical presentation, laboratory diagnosis, antimicrobial resistance, and antimicrobial management of invasive Salmonella infections. Clin Microbiol Rev. (2015) 28:901–37. doi: 10.1128/CMR.00002-15
71. Lucarelli, C, Dionisi, AM, Torpdahl, M, Villa, L, Graziani, C, Hopkins, KL, et al. Evidence for a second genomic island conferring multidrug resistance in a clonal group of strains of Salmonella enterica serovar Typhimurium and its monophasic variant circulating in Italy, Denmark, and the United Kingdom. J Clin Microbiol. (2010) 48:2103–9. doi: 10.1128/JCM.01371-09
72. Biswas, S, Li, Y, Elbediwi, M, and Yue, M. Emergence and dissemination of mcr-carrying clinically relevant Salmonella Typhimurium Monophasic clone st34. Microorganisms. (2019) 7:298. doi: 10.3390/microorganisms7090298
73. Mourão, J, Machado, J, Novais, C, Antunes, P, and Peixe, L. Characterization of the emerging clinically-relevant multidrug-resistant Salmonella enterica serotype 4,[5],12:i:- (monophasic variant of S. Typhimurium) clones. Eur J Clin Microbiol Infect Dis. (2014) 33:2249–57. doi: 10.1007/s10096-014-2180-1
74. García, P, Hopkins, KL, García, V, Beutlich, J, Mendoza, MC, Threlfall, EJ, et al. Diversity of plasmids encoding virulence and resistance functions in Salmonella enterica subsp. enterica Serovar Typhimurium monophasic variant 4,[5],12:i:- strains circulating in Europe. PLoS One. (2014) 9:e89635. doi: 10.1371/journal.pone.0089635
75. Ricci, V, Zhang, D, Teale, C, and Piddock, LJV. The o-antigen epitope governs susceptibility to colistin in Salmonella enterica. MBio. (2020) 11:19. doi: 10.1128/mBio.02831-19
76. Agersø, Y, Torpdahl, M, Zachariasen, C, Seyfarth, A, Hammerum, AM, and Nielsen, EM. Tentative colistin epidemiological cut-off value for Salmonella spp. Foodborne Pathog Dis. (2012) 9:367–9. doi: 10.1089/fpd.2011.1015
77. Carnevali, C, Morganti, M, Scaltriti, E, Bolzoni, L, Pongolini, S, and Casadei, G. Occurrence ofmcr-1in Colistin-resistant Salmonella enterica isolates recovered from humans and animals in Italy, 2012 to 2015. Antimicrob Agents Chemother Am Soc Microbiol. (2016) 60:7532–4. doi: 10.1128/AAC.01803-16
78. Litrup, E, Kiil, K, Hammerum, AM, Roer, L, Nielsen, EM, and Torpdahl, M. Plasmid-borne colistin resistance gene mcr-3 in Salmonella isolates from human infections, Denmark, 2009-17. Eur Secur. (2017) 22:30587. doi: 10.2807/1560-7917.ES.2017.22.31.30587
79. Anjum, MF, Duggett, NA, AbuOun, M, Randall, LP, Nunez-Garcia, J, Ellis, RJ, et al. Colistin resistance in Salmonella and Escherichia coli isolates from a pig farm in Great Britain. J Antimicrob Chemother. (2016) 71:2306–13. doi: 10.1093/jac/dkw149
80. Carattoli, A, Villa, L, Feudi, C, Curcio, L, Orsini, S, Luppi, A, et al. Novel plasmid-mediated colistin resistance mcr-4 gene in Salmonella and Escherichia coli, Italy 2013, Spain and Belgium, 2015 to 2016. Eur Secur. (2017) 22:30589. doi: 10.2807/1560-7917.ES.2017.22.31.30589
81. Carroll, LM, Gaballa, A, Guldimann, C, Sullivan, G, Henderson, LO, and Wiedmann, M. Identification of novel mobilized colistin resistance gene mcr-9 in a multidrug-resistant, colistin-susceptible Salmonella enterica serotype Typhimurium isolate. MBio. (2019) 10:e00853. doi: 10.1128/mBio.00853-19
82. Hussein, NH, Al-Kadmy, IMS, Taha, BM, and Hussein, JD. Mobilized colistin resistance (mcr) genes from 1 to 10: a comprehensive review. Mol Biol Rep. (2021) 48:2897–907. doi: 10.1007/s11033-021-06307-y
83. Rozwandowicz, M, Brouwer, MSM, Fischer, J, Wagenaar, JA, Gonzalez-Zorn, B, Guerra, B, et al. Plasmids carrying antimicrobial resistance genes in Enterobacteriaceae. J Antimicrob Chemother. (2018) 73:1121–37. doi: 10.1093/jac/dkx488
84. Kudirkiene, E, Andoh, LA, Ahmed, S, Herrero-Fresno, A, Dalsgaard, A, Obiri-Danso, K, et al. The use of a combined bioinformatics approach to locate antibiotic resistance genes on plasmids from whole genome sequences of Salmonella enterica serovars from humans in Ghana. Front Microbiol. (2018) 9:1010. doi: 10.3389/fmicb.2018.01010
85. Villa, L, Garciá-Fernández, A, Fortini, D, and Carattoli, A. Replicon sequence typing of IncF plasmids carrying virulence and resistance determinants. J Antimicrob Chemother. (2010) 65:2518–29. doi: 10.1093/jac/dkq347
86. Allard, MW, Luo, Y, Strain, E, Pettengill, J, Timme, RE, Wang, C, et al. On the evolutionary history, population genetics and diversity among isolates of Salmonella Enteritidis PFGE pattern JEGX01.0004. PLoS One. (2013) 8:e55254. doi: 10.1371/journal.pone.0055254
87. Kotsakis, SD, Tzouvelekis, LS, Lebessi, E, Doudoulakakis, A, Bouli, T, Tzelepi, E, et al. Characterization of a mobilizable IncQ plasmid encoding cephalosporinase CMY-4 in Escherichia coli. Antimicrob Agents Chemother. (2015) 59:2964–6. doi: 10.1128/AAC.05017-14
88. Yau, S, Liu, X, Djordjevic, SP, and Hall, RM. RSF1010-like plasmids in Australian Salmonella enterica serovar Typhimurium and origin of their sul2-strA-strB antibiotic resistance gene cluster. Microb Drug Resist. (2010) 16:249–52. doi: 10.1089/mdr.2010.0033
89. Poirel, L, Carattoli, A, Bernabeu, S, Bruderer, T, Frei, R, and Nordmann, P. A novel IncQ plasmid type harbouring a class 3 integron from Escherichia coli. J Antimicrob Chemother. (2010) 65:1594–8. doi: 10.1093/jac/dkq166
90. Oliva, M, Monno, R, D’Addabbo, P, Pesole, G, Dionisi, AM, Scrascia, M, et al. A novel group of IncQ1 plasmids conferring multidrug resistance. Plasmid. (2017) 89:22–6. doi: 10.1016/j.plasmid.2016.11.005
91. Ogura, Y, Ooka, T, Iguchi, A, Toh, H, Asadulghani, M, Oshima, K, et al. Comparative genomics reveal the mechanism of the parallel evolution of O157 and non-O157 enterohemorrhagic Escherichia coli. Proc Natl Acad Sci U S A. (2009) 106:17939–44. doi: 10.1073/pnas.0903585106
92. Nicoletti, AG, Marcondes, MFM, Martins, WMBS, Almeida, LGP, Nicolás, MF, Vasconcelos, ATR, et al. Characterization of BKC-1 class A carbapenemase from Klebsiella pneumoniae clinical isolates in Brazil. Antimicrob Agents Chemother. (2015) 59:5159–64. doi: 10.1128/AAC.00158-15
93. Miriagou, V, Carattoli, A, and Fanning, S. Antimicrobial resistance islands: Resistance gene clusters in Salmonella chromosome and plasmids. Microb Infect. (2006) 8:1923–30. doi: 10.1016/j.micinf.2005.12.027
94. Levings, RS, Partridge, SR, Djordjevic, SP, and Hall, RM. SGI1-K, a variant of the SGI1 genomic island carrying a mercury resistance region, in Salmonella enterica serovar Kentucky. Antimicrob Agents Chemother. (2007) 51:317–23. doi: 10.1128/AAC.01229-06
95. Poirel, L, Decousser, JW, and Nordmann, P. Insertion sequence ISEcp1B is involved in expression and mobilization of a blaCTX-M β-lactamase gene. Antimicrob Agents Chemother. (2003) 47:2938–45. doi: 10.1128/AAC.47.9.2938-2945.2003
96. Petrin, S, Orsini, M, Mastrorilli, E, Longo, A, Cozza, D, Olsen, JE, et al. Identification and characterization of a spreadable IncI1 plasmid harbouring a blaCTX-M-15 gene in an Italian human isolate of Salmonella serovar Napoli. Plasmid. (2021) 114:102566. doi: 10.1016/j.plasmid.2021.102566
97. Aviv, G, Tsyba, K, Steck, N, Salmon-Divon, M, Cornelius, A, Rahav, G, et al. A unique megaplasmid contributes to stress tolerance and pathogenicity of an emergent Salmonella enterica serovar Infantis strain. Environ Microbiol. (2014) 16:977–94. doi: 10.1111/1462-2920.12351
98. Arredondo-Alonso, S, Willems, RJ, van Schaik, W, and Schürch, AC. On the (im)possibility of reconstructing plasmids from whole-genome short-read sequencing data. Microb. Genomics. (2017) 3:e000128. doi: 10.1099/mgen.0.000128
99. Hendriksen, RS, Bortolaia, V, Tate, H, Tyson, GH, Aarestrup, FM, and McDermott, PF. Using genomics to track global antimicrobial resistance. Front Public Health. (2019) 7:242. doi: 10.3389/fpubh.2019.00242
100. Zankari, E, Hasman, H, Kaas, RS, Seyfarth, AM, Agersø, Y, Lund, O, et al. Genotyping using whole-genome sequencing is a realistic alternative to surveillance based on phenotypic antimicrobial susceptibility testing. J Antimicrob Chemother. (2013) 68:771–7. doi: 10.1093/jac/dks496
Keywords: Salmonella, whole genome sequencing, antimicrobial resistance, MIC, multidrug resistance, plasmids
Citation: Petrin S, Orsini M, Massaro A, Olsen JE, Barco L and Losasso C (2023) Phenotypic and genotypic antimicrobial resistance correlation and plasmid characterization in Salmonella spp. isolates from Italy reveal high heterogeneity among serovars. Front. Public Health. 11:1221351. doi: 10.3389/fpubh.2023.1221351
Edited by:
Vera Manageiro, Instituto Nacional de Saúde Doutor Ricardo Jorge (INSA), PortugalReviewed by:
Sultana Solaiman, United States Food and Drug Administration, United StatesSanjana Mukherjee, Georgetown University, United States
Copyright © 2023 Petrin, Orsini, Massaro, Olsen, Barco and Losasso. This is an open-access article distributed under the terms of the Creative Commons Attribution License (CC BY). The use, distribution or reproduction in other forums is permitted, provided the original author(s) and the copyright owner(s) are credited and that the original publication in this journal is cited, in accordance with accepted academic practice. No use, distribution or reproduction is permitted which does not comply with these terms.
*Correspondence: Carmen Losasso, Y2xvc2Fzc29AaXpzdmVuZXppZS5pdA==