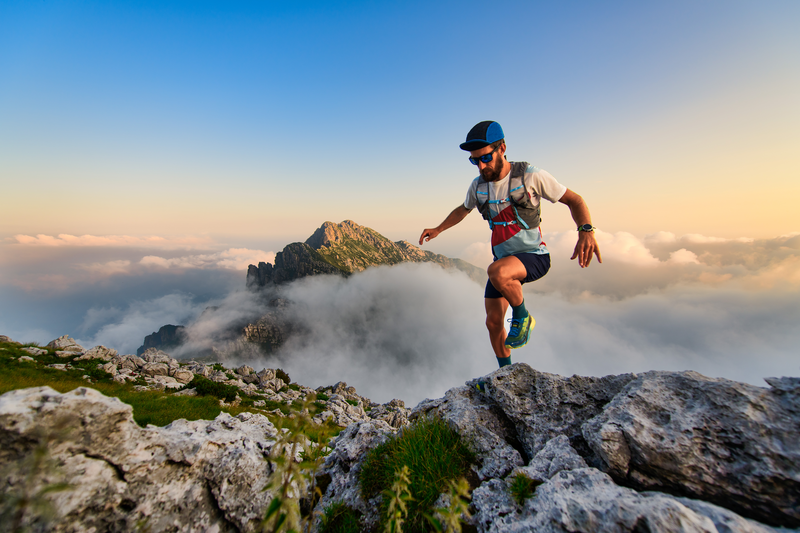
94% of researchers rate our articles as excellent or good
Learn more about the work of our research integrity team to safeguard the quality of each article we publish.
Find out more
SYSTEMATIC REVIEW article
Front. Public Health , 09 June 2023
Sec. Infectious Diseases: Epidemiology and Prevention
Volume 11 - 2023 | https://doi.org/10.3389/fpubh.2023.1195908
This article is part of the Research Topic SARS-CoV-2: Virology, Epidemiology, Diagnosis, Pathogenesis, and Control View all 24 articles
Background: A rapidly growing body was observed of literature evaluating the vaccine effectiveness (VE) against Omicron in test-negative design studies.
Methods: We systematically searched papers that evaluated VE of SARS-CoV-2 vaccines on PubMed, Web of Science, Cochrane Library, Google Scholar, Embase, Scopus, bioRxiv, and medRxiv published from November 26th, 2021, to June 27th, 2022 (full doses and the first booster), and to January 8th, 2023 (the second booster). The pooled VE against Omicron-associated infection and severe events were estimated.
Results: From 2,552 citations identified, 42 articles were included. The first booster provided stronger protection against Omicron than full doses alone, shown by VE estimates of 53.1% (95% CI: 48.0–57.8) vs. 28.6% (95% CI: 18.5–37.4) against infection and 82.5% (95% CI: 77.8–86.2) vs. 57.3% (95% CI: 48.5–64.7) against severe events. The second booster offered strong protection among adults within 60 days of vaccination against infection (VE=53.1%, 95% CI: 48.0–57.8) and severe events (VE=87.3% (95% CI: 75.5–93.4), comparable to the first booster with corresponding VE estimates of 59.9% against infection and 84.8% against severe events. The VE estimates of booster doses against severe events among adults sustained beyond 60 days, 77.6% (95% CI: 69.4–83.6) for first and 85.9% (95% CI: 80.3–89.9) for the second booster. The VE estimates against infection were less sustainable regardless of dose type. Pure mRNA vaccines provided comparable protection to partial mRNA vaccines, but both provided higher protection than non-mRNA vaccines.
Conclusions: One or two SARS-CoV-2 booster doses provide considerable protection against Omicron infection and substantial and sustainable protection against Omicron-induced severe clinical outcomes.
The Omicron variant (B.1.1.529) was first detected in early November 2021 in South Africa and was designated the fifth variant of concern by the World Health Organization (1). In contrast to the original wild-type variant, Omicron accumulated over 50 mutations in the whole genome, including 26–32 in the spike protein. This altered protein receptor-binding efficiency and immunogenicity, increasing infectivity, ability to evade neutralizing antibodies, and risk of reinfection (2). Additional mutations led to multiple Omicron subvariants with increased transmissibility including BA.2, BA.2.12.1, BA.4, BA.4.6, BA.5, BF.7, BQ.1, BQ.1.1, and XBB.1.5, the latter three of which accounted for most infections in the United States as of February 2023 (3). The effective reproduction number (Rt) and basic reproduction number (R0) were estimated to be 3.8 and 2.5 times higher for Omicron than for Delta (4). Compared with the wild-type and Delta variants, Omicron replicates less efficiently in the lung parenchymal tissues and more efficiently in the bronchial tissues, which may contribute to increased transmissibility but decreased disease severity (5–7).
There is a rapidly growing body of literature of real-world vaccine effectiveness (VE) against Omicron. Studies reported that individuals vaccinated with two mRNA doses were less susceptible to Omicron infection, though the level of protection conferred was lower than that of earlier variants, and protection waned over time (8, 9). The emergence of new variants coupled with waning vaccine-induced immunity prompted recommendations for booster doses and second booster doses based on the original Wuhan-Hu-1 strain, which were shown to confer greater protection against Omicron than two mRNA doses (10, 11). Omicron-specific bivalent mRNA booster doses were recently authorized for use in the U.S. by the Food and Drug Administration, and early data demonstrated stronger neutralizing antibody responses against Omicron than the original monovalent mRNA vaccines (12). The BNT162b2 bivalent BA.4/5 COVID-19 vaccine was recently shown to elicit greater neutralizing antibody titers against newer Omicron sublineages (BA.4.6, BA.2.75.2, BQ.1.1 and XBB.1) in adults older than 55 than a fourth dose of the original monovalent BNT162b2 (13). Uptake of the bivalent boosters, however, is low with only 15% of the U.S. adult population vaccinated as of February 2023 (14). Therefore, it is important to quantify the effectiveness of the original vaccines against Omicron.
Two early meta-analyses evaluated VE of a primary vaccine series or single booster dose and demonstrated greater protection for the third dose against symptomatic infection and severe events compared to a two-dose regimen (15, 16). However, they focused on hybrid immunity (immunity developed from SARS-CoV-2 infection and vaccination) (15) and relative vaccine effectiveness of the third dose compared to two doses (16) rather than non-vaccination. Nor did they evaluate VE for a second booster, long-term (>60 days) VE for the first booster, or adult- and child-specific VEs. Herein, we aggregate estimates in the literature to evaluate VE for the initial full doses, first booster dose, and second booster dose against Omicron-related infection and severe events for pure mRNA, partial (mixed) mRNA, and non-mRNA vaccines. We focus our review on test-negative design studies, an increasingly popular epidemiological study design for evaluating VE on infectious pathogens including influenza, rotavirus, pneumococcus, and others (17). In this design, the same clinical definition is used to enroll cases and controls and laboratory testing distinguishes “test positive” cases from “test negative” controls, thereby reducing bias from differential healthcare-seeking behavior between cases and controls (18).
This analysis followed the Preferred Reporting Items for Systematic Reviews and Meta-analyses (PRISMA) reporting guidelines.
A systematic literature search was conducted of PubMed, Web of Science, Cochrane Library, Google Scholar, Embase, Scopus, and preprint servers (bioRxiv and medRxiv) for papers published from November 26th, 2021, when Omicron was classified as a World Health Organization Variant of Concern (1), to June 27th, 2022 (for full doses and booster), and to January 8th, 2023 (for the second booster). We applied Boolean combinations of the following keywords to identify relevant publications: “SARS-CoV-2”, “COVID-19”, “2019nCoV”, “vaccine”, “booster”, “second booster”, “effectiveness”, “efficacy”, “test-negative case-control”, “test-negative design”, “Omicron”, “infection”, “hospitalization”; the detailed search procedures were presented in the Supplementary material. Publication language was not restricted, and reference lists of selected papers were also screened for additional studies.
The selection of studies followed Participant (P), Intervention (I), Comparator (C), Outcome (O), and Study Type (S), PICOS criteria (19) (Supplementary Table 1). Published studies were eligible for inclusion if they were original analyses with the test-negative design (TND) and reported VE or corresponding odds ratios (OR) of full doses, booster, or second booster against Omicron infection or severe events. We excluded studies that focused on special populations (e.g., patients with kidney disease); did not include circulation period of Omicron variant; combined VE estimates for Omicron with other viral variants such as Delta; reported relative VE between different vaccines, vaccination doses, or variants among vaccinated individuals; did not evaluate VE (e.g., instead, evaluated neutralizing antibodies); or evaluated outcomes other than infection or severe events. All available ages were included. We did not contact authors for additional data.
After removing duplicated results, we first screened studies by titles and abstracts to identify potentially eligible articles. Two pairs of researchers then independently evaluated full texts and selected those meeting the inclusion criteria. Any disagreements were discussed until a consensus was reached. Preprints were checked and updated with their most recent published version if available as of January 10th, 2023. Zotero was used for literature management. Due to the scarcity of published TND studies involving Omicron-specific bivalent booster doses by the time this meta-analysis was conducted, we solely focused on monovalent vaccines and booster doses based on the original Wuhan-Hu-1 strain.
Two pairs of researchers independently extracted the following from the included studies: author names, publication year, study region, study design, dose, vaccine type, test time in reference to vaccination time, adjusted VE point estimate and 95% confidence intervals, and adjustment confounders; if available, the number of vaccinated and unvaccinated individuals in the cases and controls were also recorded.
Study quality and risk of bias were independently assessed by two researchers using the Newcastle-Ottawa Scale (NOS). Studies could earn up to 9 points composed of participant selection (4 points), study comparability (1 point), and outcome of interest (4 points). A score >7 was considered as high quality, 5–6 as medium, and < 5 as low, and studies classified as low were excluded from the meta-analysis. Publication bias was also evaluated by Egger's test, Begg and Mazumdar rank correlation, and funnel plots when at least ten studies were available, with significance set at p < 0.1. If we detected publication bias, we used the Duval and Tweedie trim-and-fill method (20) for adjustment, which consists of imputing missing effect sizes to achieve symmetry.
We categorized full doses and booster VE into short-term, long-term, and overall to evaluate potential waning of VE over time. In the collected studies, there is no uniform definition for short-term vs. long-term VE, but most adopted cut-off points of 60–120 days from last vaccination to lab-testing. Considering the lower and upper bounds of the post vaccination test dates, we used the following guidelines. For initial full doses, a lower bound ≤ 30 days and an upper bound ≤ 180 days constitute short term, and a lower bound ≥90 (except one study used ≥70 days) days and an upper bound that is either ≥200 days or unspecified are considered long term. For booster doses, a lower bound ≤ 30 days and an upper bound ≤ 120 days are considered short term, and a lower bound ≥60 days and an upper bound >120 days or unspecified are considered as long-term. To simplify description, we occasionally use “ < 90 days” and “≥90 days” to represent short-term vs. long-term VEs for the full doses, and use “ < 60 days” and “≥60 days” to represent short-term vs. long-term VEs for the booster doses. If a study reported VEs for finer time intervals than we needed, we used an inverse variance weighted (IVW) averaging approach to combine them.
For each time interval, we further categorized VE by the type of vaccine: pure mRNA vaccines, partial mRNA vaccines, and non-mRNA vaccines. Pure mRNA vaccines comprise of homogenous or heterogeneous BNT162b2 and mRNA-1273, or a population-level mixture of the two if a study does not discriminate between them. Partial mRNA vaccines include either a multi-dose course containing at least one mRNA vaccine dose, or the study indiscriminately reported VEs of a population-level mixture of vaccines including at least one mRNA vaccine. Non-mRNA vaccines refer to the regimens that do not involve mRNA vaccines at all (e.g., Ad26.COV2.S, ChAdOx1).
We evaluated VE against Omicron infection and severe events. Analyses of VE against infection or symptomatic infection combined studies that reported either VE against symptomatic infection or VE against any infection (symptomatic or asymptomatic). Severe events included hospitalizations, noncritical hospitalizations, deaths, emergency department (ED) or urgent care (UC) encounters, ED admissions, intensive care unit (ICU) admissions, and invasive ventilation.
We evaluated VE for the overall vaccine-eligible population as well as for age groups defined as adults (≥18 years) and children/adolescents (5–17 years). If VE was not reported but odds ratios (OR) were provided, we calculated VE as (1 – OR) × 100%. The pooled VE and 95% confidence intervals were calculated via a random effects meta-analysis with restricted maximum likelihood estimation. I2 was used to evaluate between-study heterogeneity with thresholds of 25, 50, and 75% indicating low, moderate, and high heterogeneity, respectively. The metafor package in the R statistical software (version 4.0.5) was used for estimation and visualization in this meta-analysis (21).
For full doses and booster doses, we obtained 1,139 articles from all searched databases (82 from PubMed, 23 from Web of Science, 89 from Embase, 721 from Scopus, 3 from Cochrane Library, 115 from medRxiv, 6 from bioRxiv, and 100 from Google Scholar). After removing duplicates, 952 articles remained, of which 136 were retained for full review following inspection of the title, abstract, and keywords. After full text review of these 136 articles, 33 articles (9, 10, 22–52) with 271 VE estimates were formally included in this meta-analysis (Figure 1A). For the second booster, we obtained 1,413 articles from all databases (56 from PubMed, 22 from Web of Science, 55 from Embase, 1,015 from Scopus, nine from Cochrane Library, 149 from medRxiv, seven from bioRxiv, and 100 from Google Scholar). After removing duplicates, 1,236 articles remained, of which 116 were considered relevant after inspection of the title, abstract, and keywords. These 116 relevant articles were then reviewed in full text for eligibility, and 11 articles (23, 37, 53–61) with 46 VE estimates were finally included in this meta-analysis (Figure 1B).
Figure 1. Summary of evidence search and selection. (A) Full doses and first booster (B) second booster.
Among the 33 papers relevant to full doses and booster doses, 14 studies were conducted in the U.S., five in the U.K., four in Canada, three in South Africa, two in Qatar, two in Brazil, and one in each of Belgium, Netherlands and Scotland, respectively. A study could report multiple VEs for different vaccination types and outcomes. In total, there were 271 VE estimates including 124 for full doses and 147 for the first booster doses; 133 for pure mRNA vaccines, 100 for partial mRNA vaccines, and 38 the non-mRNA vaccines; 138 for symptomatic infection, 14 for any infection, and 119 for severe events. For the second booster, out of 11 papers, five studies conducted in the U.S., three in Canada, and three in Thailand. In total, there were 46 VE estimates including 32 for pure mRNA vaccines, 13 for partial mRNA vaccines, and one for non-mRNA vaccines; three for symptomatic infection, 24 for any infections, and 19 for severe events.
The VE estimates for the initial full doses against Omicron symptomatic infection or any infection were summarized in Figure 2A. Pooling all vaccine types and time intervals, the overall VE was estimated to be 28.6% (95% CI: 18.5–37.4%, 25 studies) for all ages and 24.4% (95% CI: 16.2–31.8%, 15 studies) for adults. The overall VE of the pure mRNA vaccines was estimated to be 30.6% (95% CI: 17.1–41.8%, 18 studies) for all ages, 25.4% (95% CI: 11.5–37.1%, 8 studies) for adults, and 54.2% (95% CI: 35.2–67.7%, 5 studies) for children and adolescents. Overall VE estimates for partial mRNA vaccines and non-mRNA vaccines were only available for adults, 28.1% (95% CI: 19.8–35.6%, 5 studies) and 1.5% (95% CI: 0.4–2.7%, 2 studies) respectively. This is also why we do not have a separate overall VE estimate for children and adolescents pooling all vaccine types.
Figure 2. Overall vaccine effectiveness of full doses and first booster against infection or symptomatic infection. (A) Pooled VE of full doses estimated from all 25 studies combined as well as for each vaccine type. (B) Pooled VE of first booster estimated from all 31 studies combined as well as for each vaccine type. Statistics Cochran's Q, I2 and τ2 measure the heterogeneity between studies. End points of the studies are either symptomatic infection (SI) or any infection (AI). Mixed vaccine type indicates the study reported VEs of these vaccines combined without distinguishing between them.
Short-term full-dose VE estimates pooling all vaccine types were 40.7% (95% CI: 34.3–46.5%, 19 studies) for all ages and 37.5% (95% CI: 31.4–43.1%, 10 studies) for adults (Supplementary Figure 1). Short-term VE of pure mRNA vaccines was estimated to be 43.5% (95% CI: 35.4–50.6%, 13 studies) for all ages, 41.3% (95% CI: 40.2–42.4%, 4 studies) for adults, and 45.3% (95% CI: 28.7–58.1%, 6 studies) for children and adolescents. Short-term VE estimate of partial mRNA vaccines was 34.7% (95% CI: 25.4–42.9%, 6 studies) for adults, slightly lower than that of the pure mRNA vaccines.
Long-term full-dose VE estimates against symptomatic or any infection were in general much lower than their short-term counterparts. Pooling all vaccine types, long-term full-dose VE was estimated to be 17.6% (95% CI: 13.2–21.8%, 22 studies) for all ages and 16.6% (95% CI: 10.5–22.3%, 15 studies) for adults (Supplementary Figure 2). Long-term full-dose VE of pure mRNA vaccines was estimated to be 16.4% (95% CI: 13.6–19.1%, 11 studies) for all ages, 13.1% (95% CI: 11.7–14.6%, 4 studies) for adults, and 22.3% (95% CI: 13.6–30.1%, 4 studies) for children and adolescents. Long-term full-dose VE among adults was estimated to be 22.6% (95% CI: 10.8–32.7%, 5 studies) for partial mRNA vaccines and 13.2% (95% CI: 2.6–22.6%, 6 studies) for non-mRNA vaccines.
Compared to unvaccinated controls, the overall VE of the first booster dose against Omicron symptomatic infection or any infection was 53.1% (95% CI: 48.0–57.8%, 31 studies) for all ages and 53.4% (95% CI: 47.7–58.6%, 27 studies) for adults (Figure 2B). No studies included in this analysis reported VE of booster doses for children. When stratified by vaccine type, the overall first-booster VE estimates were 58.0% (95% CI: 51.4–63.6%, 11 studies) for all ages and 61.4% (95% CI: 54.1–67.5%, 7 studies) in adults for pure mRNA vaccination, 56.4% (95% CI: 52.7–59.8%, 15 studies) for adults for partial mRNA vaccines, and 25.2% (95% CI: 2.2–42.8%, 5 studies) for adults for non-mRNA vaccines.
In comparison to its overall VE, the short-term VE estimates of the first booster dose were slightly higher, 59.4% (95% CI: 55.1–63.3%, 33 studies) for all ages and 59.9% (95% CI: 55.1–64.1%, 28 studies) for adults (Supplementary Figure 3). When stratified by vaccine type, the short-term first-booster VE estimates were 63.7% (95% CI: 59.2–67.7%, 15 studies) for all ages and 67.3 % (95% CI: 64.5–69.9%, 10 studies) for adults for pure mRNA vaccination, 62.3% (95% CI: 59.2–65.1%, 12 studies) for adults for partial mRNA vaccines, and 37.2% (95% CI: 19.5–51.0%, 6 studies) for adults for non-mRNA vaccines.
Long-term VE estimates of the first booster dose were moderately lower than their overall counterparts, 34.9% (95% CI: 27.6–41.5%, 22 studies) for all ages and 31.5% (95% CI: 22.7–39.4%, 20 studies) for adults (Supplementary Figure 4). Long-term first-booster VE estimates stratified by vaccine type were 46.6% (95% CI: 36.8–54.8%, 7 studies) for all ages and 50.9% (95% CI: 45.0–56.2%, 5 studies) for adults for pure mRNA vaccination, 34.6% (95% CI: 28.6–40.2%, 11 studies) for adults for partial mRNA vaccines, and 4.6% (95% CI:−9.5–16.9%, 4 studies) for adults for non-mRNA vaccines.
Due to lack of data, we were only able to estimate short-term and long-term VE but not overall VE of the second booster (Figure 3). Furthermore, we were unable to distinguish between vaccine types for the second booster, but the majority of these studies were based on four doses of mRNA vaccines. The short-term second-booster VE against symptomatic infection or any infection for Omicron was 59.6% (95% CI: 52.0–66.1%, 17 studies) in adults, similar to the overall and the short-term first-booster VE estimates in adults. The long-term second-booster VE was 32.7% (95% CI: 15.4–46.4%, 10 studies) in adults, comparable to that of the first booster.
Figure 3. Overall vaccine effectiveness of second booster dose against infection or symptomatic infection and against severe events. Pooled VE estimates are stratified by short-term (< 60 days) vs. long-term (≥60 days). Statistics Cochran's Q, I2 and τ2 measure the heterogeneity between studies. For infection, possible end points of the studies are symptomatic infection (SI) or any infection (AI). For severe events, possible end points are hospitalization (H), death (D), severe outcomes (SO) or invasive procedures (INV). Mixed vaccine type indicates the study reported VEs of these vaccines combined without distinguishing between them.
The overall VE of the full doses against Omicron-associated severe events was estimated to be 57.3% (95% CI: 48.5–64.7%, 24 studies) for all ages and 57.9% (95% CI: 51.5%-63.4%, 16 studies) for adults (Figure 4A). The overall VE estimates of pure mRNA vaccines were 60.9% (95% CI: 50.7–68.9%, 18 studies) for all ages, 60.1% (95% CI: 53.1–66.0%, 10 studies) for adults, and 59.9% (95% CI: 24.7–78.6%, 6 studies) for children and adolescents. The overall VE of partial mRNA vaccines for adults was slightly lower than that of pure mRNA vaccines, 54.5% (95% CI: 41.1–64.8%, 6 studies). We did not find studies estimating the overall VE of non-mRNA vaccines against Omicron-related severe events.
Figure 4. Overall vaccine effectiveness of full doses and first booster against severe events. (A) Pooled VE of full doses estimated from all 24 studies combined as well as for each vaccine type. (B) Pooled VE of first booster estimated from all 28 studies combined as well as for each vaccine type. Statistics Cochran's Q, I2 and τ2 measure the heterogeneity between studies. Possible end points of the studies are hospitalization (H), hospitalization or death (H/D), emergency department or urgent care encounter (ED/UC), or hospital admissions from emergency care (EC → H). Mixed vaccine type indicates the study reported VEs of these vaccines combined without distinguishing between them.
The short-term VE of the full doses against Omicron-associated severe events was estimated to be 66.9% (95% CI: 58.3–73.8%, 16 studies) for all ages and 69.9% (95% CI: 62.8–75.6%, 10 studies) for adults (Supplementary Figure 5). Stratified by vaccine type, the short-term VE estimates were 64.0% (95% CI: 50.2–74.0%, 9 studies) for all ages, 70.5% (95% CI: 64.9–75.2%, 3 studies) for adults, 60.7% (95% CI: 36.6–75.6%, 6 studies) for children and adolescents for pure mRNA vaccines and 70.7% (95% CI: 59.2%-78.9%, 7 studies) for adults for partial mRNA vaccines.
Long-term VE estimates of the full doses against Omicron-associated severe events were comparable to the overall VE estimates, 58.3% (95% CI: 45.5–68.1%, 18 studies) for all ages and 59.0% (95% CI: 49.0–67.1%, 13 studies) for adults (Supplementary Figure 6). Stratified by vaccine type, the long-term VE estimates were 62.4% (95% CI: 38.9–76.8%, 9 studies) for all ages, 67.7% (95% CI: 56.3–76.1%, 4 studies) for adults, and 56.4% (95% CI:−3.6–81.7%, 5 studies) for children and adolescents for pure mRNA vaccines, 50.7% (95% CI: 29.9–65.2%, 6 studies) for adults for partial mRNA vaccines, and 60.1% (95% CI: 39.7–73.6%, 3 studies) for adults for non-mRNA vaccines.
First booster doses generally showed higher VEs against Omicron-associated severe disease than full doses. The pooled overall VE of the first booster dose was estimated to be 82.5% (95% CI: 77.8%-86.2%, 28 studies) for all ages and 82.0% (95% CI: 77.0%-86.0%, 25 studies) for adults (Figure 4B). Pure mRNA vaccines and partial mRNA vaccines showed similar overall VEs against severe events, 83.6% (95% CI: 77.0–88.2%, 11 studies) for all ages, 82.5% (95% CI: 74.7–88.0%, 8 studies) for adults for the former, and 84.6% (95% CI: 77.6–89.5%, 12 studies) for adults for the latter. The overall VE was moderately lower for non-mRNA vaccines, 71.4% (95% CI: 52.1–82.9%, 5 studies) for adults.
Short-term and long-term VEs of the booster dose against Omicron-associated severe events were only available for adults (Supplementary Figure 7). We estimated the short-term VE to be 84.8% (95% CI: 80.4–88.1%, 17 studies) and the long-term VE to be 77.6% (95% CI: 69.4–83.6%, 16 studies) for all vaccine types combined. Short-term vs. long-term booster VE estimates were 85.3% (95% CI: 79.8–89.3%, 6 studies) vs. 80.1% (95% CI: 64.6–88.8%, 5 studies) for pure mRNA vaccines, 88.1% (95% CI: 83.4–91.4%, 7 studies) vs. 78.0% (95% CI: 64.3–86.4%, 8 studies) for partial mRNA vaccines, and 73.0% (95% CI: 53.7–84.3%, 4 studies) vs. 70.5% (95% CI: 47.3–83.5%, 3 studies) for non-mRNA vaccines.
Pooled short-term and long-term VE estimates for the second booster against Omicron-associated severe events among adults were 87.3% (95% CI: 75.5–93.4%, 14 studies), and 85.9% (95% CI: 80.3–89.9%, 5 studies) respectively (Figure 3), both of which are comparable to those of the first booster, though the long-term VE of the second booster appears to decay at a slower rate.
Publication bias was detected in the pooled estimates of overall VE of the full doses against severe events (Egger's test p = 0.073, Begg's test p = 0.208), long-term VE of the full doses against severe events (Egger's test p = 0.027, Begg's test p = 0.369), short-term VE of the first booster dose against severe events (Egger's test p = 0.098, Begg's test p = 0.49), and short-term VE of the second booster dose against severe events (Egger's test p = 0.001, Begg's test p = 0.747), as shown in Supplementary Figures 8–11. Additionally, publication bias was found in four subgroups defined by age group and vaccine type (Supplementary Figure 12). Results were corrected for these biases using the trim-and-fill method.
In this systematic review and meta-analysis of 42 studies, we found that one or two booster doses in addition to the initial full COVID-19 vaccine series provided substantial protection against Omicron infection with VE ≥ 50% and severe events with VE ≥ 80%, compared to no vaccination. In general, pure and partial mRNA vaccines provided comparable protection levels against infection or severe disease, and both were more effective than non-mRNA vaccines, though the difference was less dramatic in terms of protection against severe disease. The VEs of the full doses and the booster doses against severe disease only wane slightly after 3 months, but the VEs against infection wane more quickly.
Both the first and second booster doses provided considerably higher VE against infection and severe events compared to completion of the initial full series only. Studies have reported higher anti-receptor binding domain specific memory B cells and anti-spike antibodies after booster doses compared to full series only (23, 62). Similarly, T cell immunity against Omicron is provided by booster doses though at a reduced level compared to ancestral variants (63). While the initial full doses provided inadequate protection against infection (Figure 2A), they did render practically meaningful (≥50%) VE against severe disease (Figure 4A).
Pure and partial mRNA vaccines offered comparable protection levels against infection, 25.4% vs. 28.1% for the full doses and 61.4% vs. 56.4% for the first booster among adults, and both were much more effective than the non-mRNA vaccines (1.5% for the full doses and 25.2% for the first booster). Studies included in this analysis reported lower binding activities between anti-spike and anti-receptor among Ad26.COV2 recipients compared to mRNA recipients (23). Similar trends were observed against severe events, though the gap between mRNA and non-mRNA vaccines was much narrower. In particular, full-dose non-mRNA vaccines provided a similar level of sustained protection against severe disease (VE = 60%) compared to full-dose mRNA vaccines (Supplementary Figure 6), suggesting that the initial full doses of non-mRNA vaccines should be encouraged among unvaccinated individuals in regions where mRNA vaccine supply is insufficient.
The VEs of the initial full doses and the first booster dose against Omicron infection waned substantially over time, from 40.7% within 3 months of boosting to 17.6% for full doses and 59.4 to 34.9% for the first booster. The VEs against Omicron-associated severe disease waned at a slower pace, from 66.9% to 58.3% for the full doses and from 84.8% to 77.6% (in adults) for the first booster dose. Our findings are consistent with other studies reporting waning immunity of COVID-19 vaccines for earlier variants (19, 58) as well as for Omicron regardless of age, immunocompromised status, and vaccine product (55). One study reported that VE against symptomatic infection waned more rapidly among older adults (64), which was also reflected in this meta-analysis, e.g., the full-dose VE of pure mRNA vaccines against infection declined from 45.3 to 22.3% among children and from 41.3 to 13.1% among adults (Supplementary Figures 1, 2). These age differences in decay rates were not observed for the VEs against severe disease (Supplementary Figures 5, 6).
The second booster of pure or partial mRNA vaccines protected adults from Omicron infection with a VE of 59.6% which is slightly lower than the short-term VE of the first booster for pure mRNA (67.3%) or partial mRNA vaccines (62.3%) among adults. A similar gap was seen for the long-term VE among adults as well, 32.7% for the second booster vs. 50.9% for pure mRNA and 34.6% for partial mRNA first boosters. This seemingly unexpected gap (not statistically significant) may result from the fact that the dominant Omicron subvariants were mostly BA.1 and BA.2 for the first booster studies but BA.4 and BA.5 were taking over for the second booster studies. BA.4 and BA.5 are known to be associated with high immune escape and transmissibility compared to BA.1 and BA.2, e.g., the effective reproductive number was estimated to be 5.11 and 5.22 for BA.4 and BA.5 compared to 3.22 and 5.04 for BA.1 and BA.2 (65).
In terms of protection against severe disease among adults, we observed comparable VE estimates between the second booster and the first booster doses for both short term (87.3% for the second booster vs. 85.3% and 88.1% for pure and partial mRNA first boosters) and long term (85.9% for second booster vs. 80.1% and 78.0 for pure and partial mRNA first boosters). The second booster appears to wane to a lesser extent over time. However, a caveat is that nearly all data used to estimate the long-term VE of the second booster against severe disease came from the same study among elderly residents of long-term care facilities in Ontario, Canada (60). In addition, this long-term VE is against BA.1 and BA.2, the dominant subvariants during the study period of 31 December 2021 to 27 April 2022, according to the Ontario Ministry of Health.
Our study had several limitations. First, in several test-negative studies, we included, the same control group for multiple vaccine groups, which introduces dependence among the VE estimates. However, such dependence was not accounted for in our analysis due to lack of covariance estimates. Second, there was significant heterogeneity in VE estimates, which may be attributable to differences between studies in terms of a whole host of characteristics, including study design, follow-up duration, definitions of VE, time since vaccination, dosing intervals, confounders adjusted for, and others. Finally, as most studies did not provide subvariant-specific VE estimates and there is ambiguity in which Omicron subvariants were dominant for many studies, we were not able to stratify the meta-analysis by subvariant.
Our findings demonstrate that completion of a full COVID-19 vaccine series plus one or two booster doses provides considerable VE against Omicron infection and strong VE against severe events compared to non-vaccination. Although VEs generally wane after 2–3 months, the second booster clearly generates more sustainable protection. As the Omicron family continues to evolve with more genetic and antigenic variation, e.g., the XBB* and BQ.1* sublineages, lower VEs and faster waning of protection of the Wuhan-Hu-1-based boosters should be expected. Meanwhile, the level and longevity of efficacies of Omicron-specific bivalent vaccines should be closely monitored using meta-analytic approaches. To facilitate comparison and synthesis of VE estimates across studies, we recommend the following improvements to future vaccine studies: (i) longer follow-up to better understand long-term VE; (ii) stratification of VE by age group, vaccine type and variant whenever possible; and (iii) when multiple VE estimates are reported, providing covariance or correlation among the estimates via, e.g., resampling the data.
Publicly available datasets were analyzed in this study. The raw summary-level data were derived from publicly available papers cited in the reference. Extracted data and programming codes will be made available upon request by email to the corresponding authors.
YY and IL conceived the study. SS, ZM, and ML collected the data. SS, ZM, ML, and YY reviewed the data. SS analyzed data under the supervision of YY, ZM, and IL. SS, ZM, and YY drafted the manuscript. All authors contributed to the article and approved the submitted version.
This study was partially supported by the US CDC grant U01 CK000670 (YY, IL, and SS) and the US NIH grant R01 AI139761 (YY, IL, and ZM). The funders had no role in study design, data collection, data analysis, data interpretation, or the writing of the report.
The authors declare that the research was conducted in the absence of any commercial or financial relationships that could be construed as a potential conflict of interest.
All claims expressed in this article are solely those of the authors and do not necessarily represent those of their affiliated organizations, or those of the publisher, the editors and the reviewers. Any product that may be evaluated in this article, or claim that may be made by its manufacturer, is not guaranteed or endorsed by the publisher.
The Supplementary Material for this article can be found online at: https://www.frontiersin.org/articles/10.3389/fpubh.2023.1195908/full#supplementary-material
1. World Health Organization. Tracking SARS-CoV-2 Variants. (2022) Available online at: https://www.who.int/activities/tracking-SARS-CoV-2-variants. (accessed February 08, 2023).
2. Tian D, Sun Y, Xu H, Ye Q. The emergence and epidemic characteristics of the highly mutated SARS-CoV-2 Omicron variant. J Med Virol. (2022) 94:2376–83. doi: 10.1002/jmv.27643
3. Centers for Disease Control Prevention. Monitoring Variant Proportions. (2022) Available online at: https://covid.cdc.gov/covid-data-tracker/#variant-proportions. (accessed February 08, 2023).
4. Liu Y, Rocklöv J. The effective reproductive number of the Omicron variant of SARS-CoV-2 is several times relative to Delta. J Travel Med. (2022) 29: taac037. doi: 10.1093/jtm/taac037
5. Hui KP, Ng K-C, Ho JC, et al. Replication of SARS-CoV-2 Omicron BA. 2 variant in ex vivo cultures of the human upper and lower respiratory tract. EBioMedicine. (2022) 83:104232. doi: 10.1016/j.ebiom.2022.104232
6. Wu Y, Kang L, Guo Z, Liu J, Liu M, Liang W. Incubation Period of COVID-19 caused by unique SARS-CoV-2 strains: a systematic review and meta-analysis. JAMA Network Open. (2022) 5:e2228008–e2228008. doi: 10.1001/jamanetworkopen.2022.28008
7. Nyberg T, Ferguson NM, Nash SG, Webster HH, Flaxman S, Andrews N, et al. Comparative analysis of the risks of hospitalisation and death associated with SARS-CoV-2 omicron (B. 11 529) and delta (B 1617 2) variants in England: a cohort study. The Lancet. (2022) 399:1303–12. doi: 10.1016/S0140-6736(22)00462-7
8. Madewell ZJ, Yang Y, Longini IM, Halloran ME, Dean NE. Household secondary attack rates of SARS-CoV-2 by variant and vaccination status: an updated systematic review and meta-analysis. JAMA Network Open. (2022) 5:e229317–e229317. doi: 10.1001/jamanetworkopen.2022.9317
9. Ferdinands JM, Rao S, Dixon BE, Mitchell PK, DeSilva MB, Irving SA, et al. Waning 2-dose and 3-Dose Effectiveness of mRNA Vaccines Against COVID-19–Associated emergency department and urgent care encounters and hospitalizations among adults during periods of delta and omicron variant predominance — vision network, 10 states, August 2021–January 2022. MMWR Morb Mortal Wkly Rep. (2022) 71:255–63. doi: 10.15585/mmwr.mm7107e2
10. Tenforde MW, Self WH, Gaglani M, Ginde AA, Douin DJ, Talbot HK, et al. Effectiveness of mRNA vaccination in preventing COVID-19–associated invasive mechanical ventilation and death — United States, March 2021–January 2022. MMWR Morb Mortal Wkly Rep. (2022) 71:459–65. doi: 10.15585/mmwr.mm7112e1
11. Arashiro T, Arima Y, Muraoka H, Sato A, Oba K, Uehara Y, et al. Coronavirus Disease 19 (COVID-19) vaccine effectiveness against symptomatic severe acute respiratory syndrome coronavirus 2 (SARS-CoV-2) infection during delta-dominant and omicron-dominant periods in japan: a multicenter prospective case-control study (factors associated with sars-cov-2 infection and the effectiveness of COVID-19 Vaccines Study). Clin Infect Dis. (2023) 76:e108–15. doi: 10.1093/cid/ciac635
12. Chalkias S, Harper C, Vrbicky K, et al. A bivalent Omicron-containing booster vaccine against COVID-19. N Engl J Med. (2022) 387:1279–91. doi: 10.1056/NEJMoa2208343
13. Fang Z, Monteiro VS, Hahn AM, Grubaugh ND, Lucas C. Chen S. Bivalent mRNA vaccine booster induces robust antibody immunity against Omicron lineages BA2, BA2121, BA275 and BA5. Cell Discov. (2022) 8:1–4. doi: 10.1101/2022.07.19.500616
14. CDC. COVID Data Tracker. Centers for Disease Control and Prevention. (2020) Available online at: https://covid.cdc.gov/covid-data-tracker (accessed February 6, 2023).
15. Bobrovitz N, Ware H, Ma X, Li Z, Hosseini R, Cao C, et al. Protective effectiveness of previous SARS-CoV-2 infection and hybrid immunity against the omicron variant and severe disease: a systematic review and meta-regression. Lancet Infect Dis. (2023) 18:5. doi: 10.1016/S1473-3099(22)00801-5
16. Pratama NR, Wafa IA, Budi DS, Sutanto H, Asmarawati TP, Effendi GB, et al. Effectiveness of COVID-19 vaccines against SARS-CoV-2 omicron variant (B11529): a systematic review with meta-analysis and meta-regression. Vaccines. (2022) 10:2180. doi: 10.3390/vaccines10122180
17. Chua H, Feng S, Lewnard JA, Sullivan SG, Blyth CC, Lipsitch M, et al. The use of test-negative controls to monitor vaccine effectiveness: a systematic review of methodology. Epidemiology. (2020) 31:43. doi: 10.1097/EDE.0000000000001116
18. Sullivan SG, Tchetgen Tchetgen EJ, Cowling BJ. Theoretical basis of the test-negative study design for assessment of influenza vaccine effectiveness. Am J Epidemiol. (2016) 184:345–53. doi: 10.1093/aje/kww064
19. Methley AM, Campbell S, Chew-Graham C, McNally R, Cheraghi-Sohi S. PICOS and SPIDER: a comparison study of specificity and sensitivity in three search tools for qualitative systematic reviews. BMC Health Serv Res. (2014) 14:579. doi: 10.1186/s12913-014-0579-0
20. Duval S, Tweedie R. Trim and fill: A simple funnel-plot-based method of testing and adjusting for publication bias in meta-analysis. Biometrics. (2000) 56:455–63. doi: 10.1111/j.0006-341X.2000.00455.x
21. Viechtbauer W. Conducting meta-analyses in R with the metafor package. J Stat Softw. (2010) 36:1–48. doi: 10.18637/jss.v036.i03
22. Accorsi EK, Britton A, Fleming-Dutra KE, Smith ZR, Shang N, Derado G, et al. Association between 3 doses of mRNA COVID-19 vaccine and symptomatic infection caused by the SARS-CoV-2 omicron and delta variants. JAMA. (2022) 327:639–51. doi: 10.1001/jama.2022.0470
23. Adams K, Rhoads JP, Surie D, Gaglani M, Ginde AA, McNeal T, et al. Vaccine effectiveness of primary series and booster doses against covid-19 associated hospital admissions in the United States: living test negative design study. BMJ. (2022) 379:e072065. doi: 10.1136/bmj-2022-072065
24. Altarawneh HN, Chemaitelly H, Ayoub HH, Tang P, Hasan MR, Yassine HM, et al. Effects of previous infection and vaccination on symptomatic omicron infections. N Engl J Med. (2022) 387:21–34. doi: 10.1056/NEJMoa2203965
25. Andeweg SP, de Gier B, Eggink D, van den Ende C, van Maarseveen N, Ali L, et al. Protection of COVID-19 vaccination and previous infection against Omicron BA1, BA2 and Delta SARS-CoV-2 infections. Nat Commun. (2022) 13:4738. doi: 10.1038/s41467-022-31838-8
26. Andrews N, Stowe J, Kirsebom F. Covid-19 vaccine effectiveness against the omicron (B11529) variant. N Engl J Med. (2022) 386:1532–46. doi: 10.1056/NEJMoa2119451
27. Braeye T, Loenhout J van, Brondeel R. COVID-19 Vaccine effectiveness against symptomatic infection and hospitalization in Belgium, July 2021-APRIL 2022. medRxiv [Preprint]. (2022). Available online at: https://www.medrxiv.org/content/10.1101/2022.05.09.22274623v1
28. Buchan SA, Chung H, Brown KA, Austin PC, Fell DB, Gubbay JB, et al. Estimated effectiveness of COVID-19 vaccines against omicron or delta symptomatic infection and severe outcomes. JAMA Network Open. (2022) 5:e2232760. doi: 10.1001/jamanetworkopen.2022.32760
29. Buchan SA, Nguyen L, Wilson SE, Kitchen SA, Kwong JC. Vaccine effectiveness of BNT162b2 against delta and omicron variants in adolescents. Pediatrics. (2022) 150:e2022057634. doi: 10.1542/peds.2022-057634
30. Carazo S, Skowronski DM, Brisson M. Protection against omicron (B11529) BA2 reinfection conferred by primary omicron BA1 or pre-omicron SARS-CoV-2 infection among health-care workers with and without mRNA vaccination: a test-negative case-control study. Lancet Infect Dis. (2022) 23:45–55. doi: 10.1016/S1473-3099(22)00578-3
31. Chemaitelly H, Ayoub HH, AlMukdad S, Coyle P, Tang P, Yassine HM, et al. Duration of mRNA vaccine protection against SARS-CoV-2 Omicron BA1 and BA2 subvariants in Qatar. Nat Commun. (2022) 13:3082. doi: 10.1038/s41467-022-30895-3
32. Chin ET, Leidner D, Lamson L, Lucas K, Studdert DM, Goldhaber-Fiebert JD, et al. Protection against Omicron from vaccination and previous infection in a prison system. N Engl J Med. (2022) 387:1770–82. doi: 10.1056/NEJMoa2207082
33. Collie S, Champion J, Moultrie H, Bekker L-G, Gray G. Effectiveness of BNT162b2 vaccine against omicron variant in South Africa. N Engl J Med. (2022) 386:494–6. doi: 10.1056/NEJMc2119270
34. Fleming-Dutra KE, Britton A, Shang N, Derado G, Link-Gelles R, Accorsi EK, et al. Association of Prior BNT162b2 COVID-19 vaccination with symptomatic SARS-CoV-2 infection in children and adolescents during omicron predominance. JAMA. (2022) 327:2210–9. doi: 10.1001/jama.2022.7493
35. Florentino PTV, Millington T, Cerqueira-Silva T, Robertson C, Oliveira V, Júnior JBS, et al. Vaccine effectiveness of two-dose BNT162b2 against symptomatic and severe COVID-19 among adolescents in Brazil and Scotland over time: a test-negative case-control study. Lancet Infect Dis. (2022) 22:1577–86. doi: 10.1016/S1473-3099(22)00451-0
36. Gray G, Collie S, Goga A. Effectiveness of Ad26COV2S and BNT162b2 vaccines against omicron variant in South Africa. N Engl J Med. (2022) 386:2243–5. doi: 10.1056/NEJMc2202061
37. Grewal R, Kitchen SA, Nguyen L, Buchan SA, Wilson SE, Costa AP, et al. Effectiveness of a fourth dose of covid-19 mRNA vaccine against the omicron variant among long term care residents in Ontario, Canada: test negative design study. BMJ. (2022) 378:e071502. doi: 10.1136/bmj-2022-071502
38. Kim SS, Chung JR, Talbot HK, Grijalva CG, Wernli KJ, Kiniry E, et al. Effectiveness of two and three mRNA COVID-19 vaccine doses against omicron- and delta-related outpatient illness among adults, October 2021–February 2022. Influenza Other Respir Viruses. (2022) 16:975–85. doi: 10.1111/irv.13029
39. Kirsebom FCM, Andrews N, Stowe J. COVID-19 vaccine effectiveness against the omicron (BA2) variant in England. Lancet Infect Dis. (2022) 22:931–3. doi: 10.1016/S1473-3099(22)00309-7
40. Kirsebom FCM, Andrews N, Sachdeva R, Stowe J, Ramsay M, Lopez Bernal J. Effectiveness of ChAdOx1-S COVID-19 booster vaccination against the Omicron and Delta variants in England. Nat Commun. (2022) 13:7688. doi: 10.1038/s41467-022-35168-7
41. Klein NP, Stockwell MS, Demarco M, Gaglani M, Kharbanda AB, Irving SA, et al. Effectiveness of COVID-19 Pfizer-BioNTech BNT162b2 mRNA vaccination in preventing COVID-19–associated emergency department and urgent care encounters and hospitalizations among non-immunocompromised children and adolescents aged 5–17 Years — VISION Network, 10 States, April 2021–January 2022. MMWR Morb Mortal Wkly Rep. (2022) 71:352–8. doi: 10.15585/mmwr.mm7109e3
42. Lauring AS, Tenforde MW, Chappell JD, Gaglani M, Ginde AA, McNeal T, et al. Clinical severity of, and effectiveness of mRNA vaccines against, covid-19 from omicron, delta, and alpha SARS-CoV-2 variants in the United States: prospective observational study. BMJ. (2022) 376:e069761. doi: 10.1136/bmj-2021-069761
43. Lind ML, Robertson AJ, Silva J. Effectiveness of Primary and Booster Covid-19 MRNA vaccination against omicron variant sars-cov-2 Infection in People with a Prior SARS-CoV-2 Infection. Infection. (2022) 25:4056. doi: 10.1101/2022.04.19.22274056
44. Natarajan K, Prasad N, Dascomb K, Irving SA, Yang D-H, Gaglani M, et al. Effectiveness of homologous and heterologous COVID-19 booster doses following 1 ad.26.cov2.s (Janssen [Johnson & Johnson]) vaccine dose against COVID-19–associated emergency department and urgent care encounters and hospitalizations among adults — vision network, 10 States, December 2021–March 2022. MMWR Morb Mortal Wkly Rep. (2022) 71:495–502. doi: 10.15585/mmwr.mm7113e2
45. Nunes MC, Mbotwe-Sibanda S, Baillie VL, et al. SARS-CoV-2 omicron symptomatic infections in previously infected or vaccinated South African healthcare workers. Vaccines (Basel). (2022) 10:459. doi: 10.3390/vaccines10030459
46. Powell AA, Kirsebom F, Stowe J, McOwat K, Saliba V, Ramsay ME, et al. Effectiveness of BNT162b2 against COVID-19 in adolescents. Lancet Infect Dis. (2022) 22:581–3. doi: 10.1016/S1473-3099(22)00177-3
47. Zeng G. BNT162b2 Protection against the omicron variant in children and adolescents. N Engl J Med. (2022) 386:1899–909. doi: 10.1056/NEJMc2205107
48. Ranzani OT, Hitchings MDT, de Melo RL, de França GVA., Fernandes CdR, Lind ML, et al. Effectiveness of an inactivated Covid-19 vaccine with homologous and heterologous boosters against Omicron in Brazil. Nat Commun. (2022) 13:5536. doi: 10.1038/s41467-022-33169-0
49. Stowe J, Andrews N, Kirsebom F, Ramsay M, Bernal JL. Effectiveness of COVID-19 vaccines against Omicron and Delta hospitalisation, a test negative case-control study. Nat Commun. (2022) 13:5736. doi: 10.1038/s41467-022-33378-7
50. Tartof SY, Slezak JM, Puzniak L, Hong V, Xie F, Ackerson BK, et al. Durability of BNT162b2 vaccine against hospital and emergency department admissions due to the omicron and delta variants in a large health system in the USA: a test-negative case–control study. The Lancet Respiratory Medicine. (2022) 10:689–99. doi: 10.1016/S2213-2600(22)00101-1
51. Tseng HF, Ackerson BK, Luo Y, Sy LS, Talarico CA, Tian Y, et al. Effectiveness of mRNA-1273 against SARS-CoV-2 omicron and delta variants. Nat Med. (2022) 28:1063–71. doi: 10.1038/s41591-022-01753-y
52. Young-Xu Y, Zwain GM, Izurieta HS, Korves C, Powell EI, Smith J, et al. Effectiveness of mRNA COVID-19 vaccines against Omicron and Delta variants in a matched test-negative case–control study among US veterans. BMJ Open. (2022) 12:e063935. doi: 10.1136/bmjopen-2022-063935
53. Intawong K, Chariyalertsak S, Chalom K, Wonghirundecha T, Kowatcharakul W, Thongprachum A, et al. Effectiveness of heterologous 3rd and 4th dose COVID-19 vaccine schedules for SARS-CoV-2 infection during delta and omicron predominance in Thailand. Res. Sq. (2022) 28:1–4. doi: 10.21203/rs.3.rs-1792139/v1
54. Link-Gelles R, Levy ME, Gaglani M, Irving SA, Stockwell M, Dascomb K, et al. Effectiveness of 2, 3, and 4 COVID-19 mRNA vaccine doses among immunocompetent adults during periods when SARS-CoV-2 Omicron BA.1 and BA.2/BA.2.12.1 sublineages predominated — VISION network, 10 States, December 2021–June 2022. MMWR Morb Mortal Wkly Rep. (2022) 71:931. doi: 10.15585/mmwr.mm7129e1
55. Ferdinands JM, Rao S, Dixon BE, Mitchell PK, DeSilva MB, Irving SA, et al. Warning of vaccine effectiveness against moderate and severe covid-19 among adults in the US from the VISION network: test negative, case-control study. BMJ. (2022) 379:e072141. doi: 10.1136/bmj-2022-072141
56. Tseng HF, Ackerson BK, Bruxvoort KJ, et al. Effectiveness of mRNA-1273 against infection and COVID-19 hospitalization with SARS-CoV-2 Omicron subvariants: BA.1. BA2, BA2121, BA4, and BA5 Nat Commun. (2022) 14:1–10. doi: 10.1101/2022.09.30.22280573
57. Tartof SY, Slezak JM, Puzniak L, Hong V, Frankland TB, Ackerson BK, et al. BNT162b2 vaccine effectiveness against SARS-CoV-2 omicron BA4 and BA5. Lancet Infect Dis. (2022) 22:1663–5. doi: 10.1016/S1473-3099(22)00692-2
58. Grewal R, Nguyen L, Buchan SA, Wilson SE, Costa AP, Kwong JC. Effectiveness and duration of protection of a fourth dose of coronavirus disease 2019 messenger RNA vaccine among long-term care residents in Ontario, Canada. J Inf Dis. (2022) 3:468. doi: 10.1093/infdis/jiac468
59. Intawong K, Chariyalertsak S, Chalom K, Wonghirundecha T, Kowatcharakul W, Thongprachum A, et al. Effectiveness of heterologous third and fourth dose COVID-19 vaccine schedules for SARS-CoV-2 infection during delta and omicron predominance in Thailand: a test-negative, case-control study. The Lancet Regional Health-Southeast Asia. (2022) 3:121. doi: 10.1016/j.lansea.2022.100121
60. Grewal R, Nguyen L, Buchan SA, Wilson SE, Nasreen S, Austin PC, et al. Effectiveness of mRNA COVID-19 vaccine booster doses against Omicron severe outcomes. medRxiv. (2022) 1:766. doi: 10.1101/2022.10.31.22281766
61. Nittayasoot N, Suphanchaimat R, Thammawijaya P, et al. Real-World Effectiveness of COVID-19 vaccines against severe outcomes during the period of omicron predominance in Thailand: a test-negative nationwide case–control study. Vaccines. (2022) 10:2123. doi: 10.3390/vaccines10122123
62. Gilboa M, Regev-Yochay G, Mandelboim M, Indenbaum V, Asraf K, Fluss R, et al. Durability of immune response after COVID-19 booster vaccination and association with COVID-19 omicron infection. JAMA network open. (2022) 5:e2231778–e2231778. doi: 10.1001/jamanetworkopen.2022.31778
63. Jacobsen H, Jimenez VC, Sitaras I, et al. Post-vaccination T cell immunity to omicron. Front Immunol. (2022) 13:944713. doi: 10.3389/fimmu.2022.944713
64. Cerqueira-Silva T., Oliveira Vd, Paixão ES, Júnior JB, Penna GO, Werneck GL, et al. Duration of protection of CoronaVac plus heterologous BNT162b2 booster in the Omicron period in Brazil. Nat Commun. (2022) 13:1–6. doi: 10.1038/s41467-022-31839-7
Keywords: Omicron, vaccine effectiveness, meta-analysis, test negative, booster dose
Citation: Song S, Madewell ZJ, Liu M, Longini IM and Yang Y (2023) Effectiveness of SARS-CoV-2 vaccines against Omicron infection and severe events: a systematic review and meta-analysis of test-negative design studies. Front. Public Health 11:1195908. doi: 10.3389/fpubh.2023.1195908
Received: 29 March 2023; Accepted: 18 May 2023;
Published: 09 June 2023.
Edited by:
Severino Jefferson Ribeiro da Silva, University of Toronto, CanadaReviewed by:
George William Carnell, University of Cambridge, United KingdomCopyright © 2023 Song, Madewell, Liu, Longini and Yang. This is an open-access article distributed under the terms of the Creative Commons Attribution License (CC BY). The use, distribution or reproduction in other forums is permitted, provided the original author(s) and the copyright owner(s) are credited and that the original publication in this journal is cited, in accordance with accepted academic practice. No use, distribution or reproduction is permitted which does not comply with these terms.
*Correspondence: Yang Yang, eWFuZy55YW5nNEB1Z2EuZWR1
†These authors share first authorship
‡These authors share senior authorship
Disclaimer: All claims expressed in this article are solely those of the authors and do not necessarily represent those of their affiliated organizations, or those of the publisher, the editors and the reviewers. Any product that may be evaluated in this article or claim that may be made by its manufacturer is not guaranteed or endorsed by the publisher.
Research integrity at Frontiers
Learn more about the work of our research integrity team to safeguard the quality of each article we publish.