- Department of Hepatobiliary Surgery, Third Hospital of Hebei Medical University, Shijiazhuang, Hebei, China
Non-alcoholic fatty liver disease (NAFLD) is the most prevalent liver disorder. With the improvement in human living standards, the prevalence of NAFLD has been increasing in recent years. Endocrine-disrupting chemicals (EDCs) are a class of exogenous chemicals that simulate the effects of hormones in the body. There has been growing evidence regarding the potential effects of EDCs on liver health, especially in NAFLD. This paper aims to summarize the major EDCs that contribute to the growing burden of NAFLD and to raise public awareness regarding the hazards posed by EDCs with the objective of reducing the incidence of NAFLD.
1. Introduction
Non-alcoholic fatty liver disease (NAFLD) is an important public health issue that affects a large portion of the global population. Estimates indicate that the worldwide prevalence of NAFLD ranges from 13% in Africa to 42% in Southeast Asia (1). NAFLD encompasses a spectrum of liver conditions, including simple steatosis or non-alcoholic fatty liver (NAFL), which has a milder course, and non-alcoholic steatohepatitis (NASH), with potential progression to fibrosis or cirrhosis and hepatocellular carcinoma (HCC) (2, 3). The hallmark of NAFLD is the accumulation and deposition of excessive fat in liver cells, which may be related to genetic, dietary, and environmental factors (4). These factors promote the onset of insulin resistance (IR) in adipose tissue, leading to adipocyte dysfunction and increased influx of free fatty acids (FFAs) into the liver (5). These FFAs and their consequent lipotoxic intermediates have been shown to have adverse effects such as abnormal lipids metabolism, oxidative stress, and chronic liver inflammation, all of which contribute to the progression of NAFLD (6, 7).
Environmental endocrine disrupting chemicals (EDCs) are a class of exogenous chemicals that mimic the effects of hormones in the body, causing hormonal dysregulation and mediating various metabolic disorders. The liver, an organ crucial to metabolism and detoxification (8), has been shown to be impacted by EDCs, with studies suggesting exposure to these chemicals can lead to metabolic changes and liver disease (9–12). Because of the difficulty in getting biopsy-confirmed NAFLD histological specimens, the liver injury is typically assessed using serum biomarkers of hepatoxicity of NAFLD. Alanine aminotransferase (ALT), aspartate aminotransferase (AST), alkaline phosphatase (ALP) and γ-glutamyl transferase (GGT) are considered specific biomarkers of liver injury, they are widely used to evaluate the progression of NAFLD. Given the role of EDCs in the worldwide deterioration of metabolic health, it is imperative that the scientific community continues to study and understand their effects. We aimed to provide an overview of EDCs and summarize the effects of EDC exposure on NAFLD.
1.1. Overview of EDCs
Environmental endocrine disruptors (EDCs) are a heterogenous group of chemicals that are widely distributed and easily enriched, present in many forms. In daily life, an increasing number of substances have been identified as EDCs; they enter the body through the digestive tract, respiratory tract, or skin, and produce adverse effects. EDCs can be classified as natural or synthetic, based on their origin. Natural EDC include phytoestrogens and mycotoxins, while synthetic EDCs include chemicals used as industrial solvents and their byproducts (polychlorinated biphenyls, polybrominated biphenyls, and dioxins), plastics (bisphenol A), plasticizers (phthalates), fungicides (vinclozolin), pesticides (methoxychlor and chlorpyrifos), heavy metals (mercury and lead), and pharmaceutical agents present in human and animal foods (11, 13–16).
Studies have shown that EDCs can interfere with various aspects of hormone regulation in the body, including production, release, transport, metabolism, binding, action, and elimination, leading to hormonal dysregulation and contributing to various metabolic disorders (17, 18). It’s also been found that EDCs play a potential role in the regulation of genomic expression, promoting epigenetic modifications that result in the development of pathologies by mediating carcinogenic, neurotoxic, hepatotoxic, and immunotoxic effects (19–21). The challenge in understanding the impact of EDCs is compounded by the fact that humans are exposed, not to a single environmental pollution compound, but to a cocktail of EDCs, making it even more difficult to predict the net effect and evincing the association between a specific EDC and disease (13, 22). Moreover, exposure to persistent EDCs may initiate and promote the pathogenesis of NAFLD (23). EDCs affect the progression of NAFLD through the interaction of nuclear receptors. Activating transcription factors, triggering the imbalances between lipid flow/outflow in the liver, promoting mitochondrial dysfunction, and mediating the hepatic inflammatory are the possible mechanism of NAFLD (17).
2. Methods
In this study, we focused on several key EDCs that are closely related to human health, including per−/polyfluorinated substances, bisphenol A, polychlorinated biphenyls, and phthalates (as outlined in Table 1). Systematic search of PubMed and Embase databases was conducted from January 1, 2010, to December 28, 2022, to identify human studies investigating the relationship between non-alcoholic liver disease and these EDCs. Detailed search strategies are presented in Table 2. After screening the retrieved studies based on their titles and abstracts, we excluded studies without human data, case reports, non-original reports, studies without NAFLD outcomes, and pharmacological or ecological studies.
3. Relationship between NAFLD and EDCs
3.1. Per-/polyfluorinated substance
Per-/polyfluorinated substance (PFAS) is a series of organic compounds containing at least one perfluorinated carbon atom, it is lipophobic and hydrophobic that are useful for manufacturing wide ranges of consumer products (24, 25). PFASs have a stable chemical structure with a half-life of about 2–8 years, allowing them to persist and accumulate in the environment (10, 26). Because of these properties, PFASs are classified as “persistent organic pollutants” (POPs), and their delayed-elimination feature may cause long-term harmful health effects. PFASs have been detected in drinking water, various foods (meat, vegetables, milk, eggs), air, and early life placental or breast milk, and are able to accumulate in biological tissues and organs with high protein content (27–29). Nearly all adults in the U.S. have been found to have accumulated PFAS in their body tissues (10). Current studies have indicated that four congeners account for most human exposure: perfluorooctanesulfonic acid (PFOS), perfluorooctanoic acid (PFOA), perfluorohexanesulfonic acid (PFHxS), and perfluorononanoic acid (PFNA) (10, 30, 31). Compared to the general population, worker in certain occupations (e.g., professional ski-waxers, firefighters, fluorochemical plant workers) experience high PFAS serum concentrations based on their occupation (32). The median concentrations were 24-27 ng/ml (PFOS), 50-57 ng/ml (PFOA), 1.4–1.6 ng/ml (PFHxS) and 12-13 ng/ml (PFNA) in professional ski-waxers (33). And the median concentrations in the general population were 3.59–24.22 ng/mL (PFOS) (34, 35), 0.99–28.0 ng/mL (PFOA) (36, 37), 0.59–1.80 ng/mL (PFHxS) (38, 39), and 0.24–1.60 ng/mL (PFNA) (34, 37), respectively.
The liver is one of the main target organs of PFAS toxicity (40). However, the exact mechanism of PFAS hepatotoxicity remains unclear. PFAS are thought to act as ligands for peroxisome proliferator-activated receptors (PPARs), which promote liver inflammation and triglyceride accumulation through the activation of PPARα and lead to liver injury or NAFLD (41–43). The complementary mechanism also includes activation of the constitutive androstane receptor (CAR) (42, 44), downregulation of nuclear factor erythroid 2-related factor 2 (NRF2) (45, 46), and upregulation of nuclear factor kappa-light-chain-enhancer of activated B cells nuclear factor-kappa B (NF-ĸ B) (47). Animal and epidemiological studies have shown that PFAS can cause an obviously increase in liver lipid volume, induce mitochondrial dysfunction and oxidative stress, and promote inflammatory responses in NAFLD progression (41, 48, 49).
A large number of nuclear receptors (NRs) were expressed in liver, making it a critical target for PFAS. In this review, we noticed that a few studies have evaluated the hepatic enzyme abnormalities associated with PFAS exposure. PFOA and PFNA exposure is usually positively associated with higher ALT and GGT levels, suggesting that changes in serum biomarkers are often accompanied by histopathological changes or liver disease (34, 39, 50–53). However, for AST, studies have reported different outcomes in different crowds. Khalil (37) found that there was no relationship between serum PFAS and AST levels in obese children, while PFOA and PFOS were positively correlated with AST in Japanese children (52). In addition, Attanasio et al. reported sex-specific histological effects of PFAS exposure and found positive associations between PFAS and ALT in female adolescents, but conversely in male adolescents, which could be mediated by sex hormones (54). Evidence for sex-specific differences was also found in rats, with ALT increasing more frequently in male rats (55–57). The authors suggest that PFAS exposure may play an important role in the development of NAFLD and carcinoma (Table 3). The PFAS was positively associated with lobular inflammation in adults undergoing bariatric surgery in Northern Europe, however, the reason for this association is unclear. It may be related to changes in lipid and bile acid metabolism (9, 59). In animal models, exposure to subchronic PFOS has been found to enhance hepatic stellate cell (HSC) activation and exacerbate carbon tetrachloride (CCl4)-induced liver fibrosis (60), consistent with the outcomes of Sen’s study, which showed that PFOS was positively associated with hepatic fibrosis in adults (9). In contrast, a study including adults from the C8 Health Project in the USA showed that cumulative PFOA exposure had no effect on all liver diseases, enlarged liver, or cirrhosis (58). Additionally, a study included 1,105 mother–child pairs from the European Human Early-Life Exposome (HELIX) cohort showed that higher exposure to PFAS during pregnancy was associated with higher liver enzyme levels in children (38). PFAS can cross the placenta barrier efficiently and deposit in fetal tissues (61), they altered some amino acid (e.g., valine, leucine, phenylalanine) and lipid (e.g., glycerophospholipid) metabolism that related to NAFLD pathogenesis, which exerted adverse effects in liver (38).
3.2. Polychlorinated biphenyls
Polychlorinated biphenyls (PCBs) are also a type of POP that are manufactured and used commercially as dielectric fluids in transformers (62). They are thermodynamically stable polyhalogenated aromatic hydrocarbons consisting of up to ten chlorine substituents attached to a biphenyl ring (63). Based on their structures, PCB congeners have been subclassified into dioxin-like (DL) and nondioxin-like (NDL). DL PCBs have a coplanar structure, whereas NDL PCBs have a noncoplanar structure, which can be attributed to receptor-based modes of action. PCBs persist in the environment and accumulate in soil, aquatic sediments, and in species that consume these sources (fish, cows, dairy) (64). Despite a ban on their production and emission in 1979, human exposure to PCBs usually occurs through contaminated air, water, or food. As POPs, PCBs accumulate in adipose tissue and are gradually released into the bloodstream (65, 66).
The liver appears to be both a target and an effector organ for PCB-induced endocrine disruption. The occurrence of NAFLD is due to an imbalance between lipid production and elimination, which promotes excessive accumulation of hepatic lipids (17). PCBs have been documented to be related to this phenomenon, as they can induce pathological fat aggregation, both in the DL and NDL groups (67). DLPCBs activate the aryl hydrocarbon receptor (AhR) and peroxisome proliferator-activated receptors alpha and gamma (PPARα/γ) (63, 68), exerting a multimodal effect on lipid accumulation and causing steatosis by disrupting liver lipid metabolism. NDL PCBs, on the other hand, activate the constitutive androstane receptor (CAR) and pregnane X receptor (PXR) (69), which could reduce the protective response of the liver to promote diet induced NAFLD (67).
Studies have shown that PBC exposure can cause NAFLD related metabolic disorders, including insulin resistance, obesity, and lipid metabolic dysfunction. PCBs differentially regulate hepatic lipid metabolism and several related genes. PCB126 exposure increases hepatic lipids and causes toxicant-associated steatosis (mild small-droplet macro vesicular steatosis) (63). Mono-exposure to either PCB126 or Aroclor1260 increased hepatic lipid uptake while decreasing lipid biosynthesis, but these effects were abrogated by exposure to the NDL/DL PCB mixture (63). Pnpla3, a lipase implicated in NAFLD (70), was significantly upregulated by Aroclor1260 exposure, but was suppressed by either PCB126 or Aroclor1260/PCB126 exposure, potentially due to activation of different receptors among NDL or DL PCBs. PCBs have also been associated with the promotion of toxicant-associated steatohepatitis (TASH), which can ultimately lead to secondary liver necrosis, potentially due to a loss of protein phosphorylation and downregulation of the hepatic kinome (71). Positive associations were also observed between PCB concentrations and NAFLD-related biomarkers (Table 4), with most PCB congeners positively correlated with elevated alanine aminotransferase (ALT) levels. A study of 4,582 adults conducted by Cave et al. (76) showed that 10.6% of participants had unexplained ALT elevation, with older age significantly associated with total PCB levels in the highest quartile. Of those participants aged 70 years or older, 71.7% had high PCB levels compared to only 2.2% of those aged under 30 years. A study of 1,108 mother–child pairs from six countries by Midya (72) discovered that prenatal exposure to PCBs is a potential risk factor for pediatric NAFLD, and were further associated with increased CK-18 levels (a novel marker of hepatocyte apoptosis and NAFLD). Notably, researchers have identified potential therapeutic targets for improving PCB-induced NAFLD, including the anti-fibrotic compound recombinant FGF21, which reduced the overexpression of hepatic lipocalin-2 (LCN2), a group of transporters of small lipophilic molecules that are upregulated in several liver diseases, and attenuated NAFLD (62, 77).
3.3. Bisphenol A
Bisphenol A (BPA), which consists of two phenol rings attached by a methyl bridge with two methyl groups (78), is a plasticizer mainly used for polycarbonate plastics and epoxy resins in many consumer products (79). BPA exposure can occur via various sources such as plastic containers, toys, water bottles, food packaging materials, office supplies, and dental sealants (80, 81). BPA has low lipophilicity and degrades rapidly with a half-life of 4–5 h (82). Due to its broad application, BPA is detected in more than 90% of people, and the median urine BPA concentration in adults is 2.24–6.17 ng/mL (83, 84).
The liver is the main organ that metabolizes and transforms BPA into glucuronidation; therefore, it is more susceptible to BPA than other organs (85). BPA increases the risk of NAFLD owing to fat accumulation, obesity, and oxidative stress. Upregulation of lipogenic enzymes and transcription factors, such as sterol regulatory element binding protein-1c (srebp-1c), the carbohydrate responsive element binding protein (ChREBP), and liver X receptor (LXR) (86), promotes de novo lipogenesis (DNL) (87), increasing the risk of lipid accumulation and obesity. In addition, exposure to high doses of BPA decreased the activities of antioxidant indicators, such as superoxide dismutase (SOD) and glutathione (GSH), causing excessive accumulation of free radicals, such as reactive oxygen species (ROS), promoting liver damage and hepatotoxicity (88).
Aminotransferases are the most widely used biomarkers in experiments, and are released into the bloodstream following liver injury (89). Epidemiological studies have shown that BPA exposure could have negative effects on NAFLD-related biomarkers (Table 5). Higher urinary BPA levels usually led to the elevation of ALT (83, 96, 97). A study carried out by Lang et al. reported that higher BPA levels are linked to abnormal GGT (Odds ratio [OR]:1.29, 95% Confidence Interval [CI]:1.14–1.46) and ALP (OR:1.48, 95%CI:1.18–1.85) (98). In addition to liver biomarkers, urinary BPA levels were positively associated with the prevalence of NAFLD in adults and adolescents. In the Korean National Environmental Health Survey (83), which included 3,476 participants with a mean age of 52.96 ± 0.25 years old, the geometric mean concentration of BPA in the NAFLD group was significantly higher than in the non-NAFLD group (2.56 ug/L vs. 2.24 ug/L, p = 0.001). A study analyzing adolescents (12–19 years old) from NHANES in the USA also showed that the risk of suspected NAFLD (ALT≥30 U/L) was increased in participants in higher quartiles of BPA exposure (93). In terms of pathological findings, mice and rats treated with BPA showed liver tissue dilatation of sinusoids, congestion, inflammation, and necrosis in a dose-dependent manner (99). Although BPA can be excreted quickly from the body, people are constantly exposed to it throughout their lives and BPA exposure is associated with metabolic health in offspring. Prenatal BPA exposure has been shown to alter gene expression profiles and result in peripheral insulin resistance and liver lipotoxicity (100, 101). Gestational BPA exposure can promote the development of NAFLD in rodent models through the perturbation of the nuclear transcription factor activity (102). Another study indicated that exposure to BPA may diminish the immune response following hepatitis B vaccination (79).
3.4. Di-(2-ethylhexyl) phthalate
Phthalates are a large group of ubiquitous industrial chemicals that are commonly used in a variety of products such as plasticizers in food wrapping and packaging, coatings, cosmetics, adhesives, medical tubes, and toys (103, 104). Phthalates may enter the human body through the skin, respiratory tract, digestive tract, or even intravenous injection, as they are prone to leaching and transfer to air, soil, or food (105, 106). These chemicals are usually rapidly metabolized and excreted within 24–48 h. Diester phthalates could hydrolyze into monoester phthalates, then excreted as glucuronide conjugates, in the urine (107).
The development of NAFLD may be related to the adverse effects of DEHP on lipid metabolism and oxidative stress. DEHP and its active metabolite mono-(2-ethylhexyl) phthalate (MEPH) can affect hepatic accumulation of TGs and exacerbate NAFLD in rodents (108). MEHP also can affect the lipid accumulation in BRL-3A hepatocytes through the inhibition of the Janus kinase 2/Signal transducer and activator of transcription 5 (JAK2/STAT5) pathway, suggesting that the regulation of STAT5 by MEPH plays a critical role in the activation of enzymes involved in fatty acid metabolism (109). Furthermore, DEHP also mediates the deterioration of antioxidant machinery and induces oxidative stress. Higher levels of ROS were observed in MEHP-treated cells, indicating the effect of ROS on pro-inflammatory cytokine production and apoptosis of hepatocytes by inducing NF-κB (110, 111). Other experimental studies in animals have shown that the toxicity of phthalates drives liver fibrosis by oxidative stress pathways (112, 113).
Phthalate exposure is strongly associated with the NAFLD prevalence (114) (Table 6). A study involving 5,800 Korean adults demonstrated that the prevalence of NAFLD defined by the hepatic steatosis index (HSI) was associated with high urinary levels of many types of phthalates, and higher quartiles of MEHHP revealed a significantly higher risk (OR 1.39, 95% CI:1.00–1.92) of NAFLD (119). In addition, NAFLD measured using vibration-controlled transient elastography (VCTE) was also found to be positively associated with MECPP and MEHHP exposure (116). Unlike in adults, DEHP exposure also affects the prevalence of NAFLD in adolescents. Berman et al. (115) studied 387 mother–child pairs in Australia and found that mid-level prenatal exposure to MnBP was associated with a greater incidence of NAFLD at 17 years old. Table 6 also lists epidemiologic studies on the relationship between NAFLD biomarkers. A study involving 102 males aimed to examine the influence of MEP and MEHP on liver function and found that phthalate exposure may be associated with a statistically significant increase in ALT and AST serum levels, while urinary phthalate levels may be correlated with increased serum TG and decreased HDL cholesterol levels (104). A transversal study also demonstrated that serum MEHP levels were correlated with GGT (122). Thus, DEHP may interfere with thyroid function and induce NAFLD. Yang et al. divided 2,308 adults with subclinical hypothyroidism (SCH) into NAFLD and non-NAFLD groups according to the HSI score and found that the levels of phthalate metabolites in urine are positively associated with NAFLD with SCH (118). DEHP possesses a thyroid receptor antagonistic function, while thyroid hormones can activate TH-Receptor β (a potential target in NAFLD therapy) and decrease hepatic steatosis, which may further induce NAFLD (123–125). However, not all studies have discovered an association between phthalates and thyroid hormones, and further studies ought to be conducted to investigate this association.
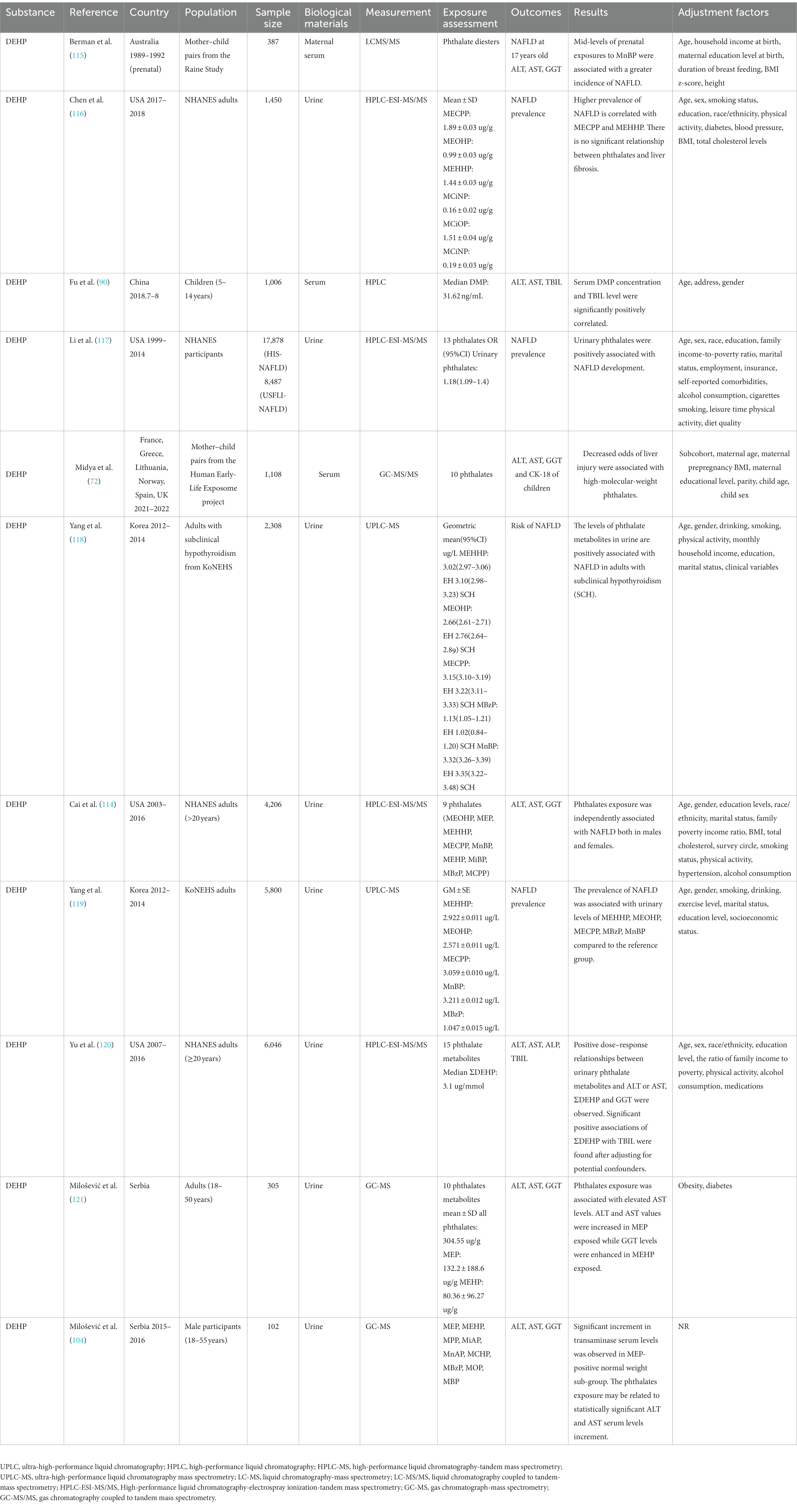
Table 6. Epidemiologic studies on the relationship between phthalates and NAFLD prevalence and biomarkers.
3.5. Sex differences of association between EDCs and NAFLD
Liver expresses androgen and estrogen receptors (126), thus research on sex-specific differences in EDCs has been a hot topic, but no consistent conclusion has been made so far. In the analyses of NHANES 2013–2016, it was observed that an opposite direction of the statistically significant association between PFAS and liver enzyme by sex, elevated AST is associated with increased PFOA in female adolescents, whereas there is an inverse association with increased PFOA, PFNA and PFHxS in males (54). However, Borghese et al. using the data from Canadian Health Measures Survey found that the association between PFOA and AST was twice as strong among men vs. women, this could be because menstruation, pregnancy, and breastfeeding are all prominent excretion pathways for PFAS in women (25). The sex difference was also reported in PCB, Li et al. (127) found that increased mortality from hepatic disease in PCB-exposed, it may be explained by sex-specific effects of estrogenic PCB congeners (73, 128). Relationships between BPA exposure and liver function at puberty were observed, serum AST levels were positively associated with BPA in boys, and the effect sizes were larger for all indicator in boys (94). Due to the differences in sex hormone associated BPA metabolism, women expressed higher levels of the UGT2B1 to catalyze BPA glucuronidation and accelerate the clearance of BPA (129, 130). Trasande et al. (131) identified a near-significant interaction of DEHP metabolites with sex, suggesting a possible role of reduced androgen activity. EDC’s sex difference is complicated, further research is needed to be done.
4. Conclusion
Exposure to environmental chemicals is ubiquitous and poses a threat to human metabolic health. EDCs affect NAFLD by interacting with nuclear receptors (NRs) and activating transcriptional factors, which promote hepatic lipid accumulation, oxidative stress, and liver dysfunction. Data from epidemiological studies prove an interrelationship between EDCs exposure and NAFLD. However, several challenges remain. For example, EDC mixtures are a complicated issue, and it is difficult to predict the net effect of EDC mixtures at the individual level in humans because it is difficult to perform laboratory detection for individual isomer and every human has a unique exposome (22, 90). In addition, the interpretation of the results on the effects of EDCs has been complicated by using different routes of administration under many experimental conditions such as, difference of doses, absence of dose–response relationships, or small sample sizes (132). In the future, research with larger samples, longer follow-up periods, and a multidisciplinary approach to explore the effect of EDCs in the human body is required. Moreover, the scientific community should help draw public attention to the hazards of EDCs and promote more regulation of industrial pollution.
Author contributions
YC: design, information retrieval, draft writing, and article review and editing. YW: information retrieval, draft writing, and article review and editing. ZC: information retrieval, draft writing, and information visualization. WL: methodology, review, and information visualization. BL: methodology and review. QZ: article review and editing. XZ: article review and editing. JD: methodology, and article review and editing. JC: conceptualization, design, funding acquisition, and article review and editing. All authors contributed to the article and approved the submitted version.
Acknowledgments
We would like to thank Editage (www.editage.cn) for English language editing.
Conflict of interest
The authors declare that the research was conducted in the absence of any commercial or financial relationships that could be construed as a potential conflict of interest.
Publisher’s note
All claims expressed in this article are solely those of the authors and do not necessarily represent those of their affiliated organizations, or those of the publisher, the editors and the reviewers. Any product that may be evaluated in this article, or claim that may be made by its manufacturer, is not guaranteed or endorsed by the publisher.
References
1. Huang, DQ , El-Serag, HB , and Loomba, R . Global epidemiology of NAFLD-related HCC: trends, predictions, risk factors and prevention. Nat Rev Gastroenterol Hepatol. (2021) 18:223–38. doi: 10.1038/s41575-020-00381-6
2. White, DL , Kanwal, F , and el–Serag, HB . Association between nonalcoholic fatty liver disease and risk for hepatocellular cancer, based on systematic review. Clin Gastroenterol Hepatol. (2012) 10:1342–59.e2. doi: 10.1016/j.cgh.2012.10.001
3. Chalasani, N , Younossi, Z , Lavine, JE , Charlton, M , Cusi, K , Rinella, M, et al. The diagnosis and management of nonalcoholic fatty liver disease: practice Guidance from the American Association for the Study of Liver Diseases. Hepatology. (2018) 67:328–57. doi: 10.1002/hep.29367
4. Fang, YL , Chen, H , Wang, CL , and Liang, L . Pathogenesis of non-alcoholic fatty liver disease in children and adolescence: from “two hit theory” to “multiple hit model”. World J Gastroenterol. (2018) 24:2974–83. doi: 10.3748/wjg.v24.i27.2974
5. Carr, RM , Oranu, A , and Khungar, V . Nonalcoholic fatty liver disease: pathophysiology and management. Gastroenterol Clin N Am. (2016) 45:639–52. doi: 10.1016/j.gtc.2016.07.003
6. Petta, S , Gastaldelli, A , Rebelos, E , Bugianesi, E , Messa, P , Miele, L, et al. Pathophysiology of non alcoholic fatty liver disease. Int J Mol Sci. (2016) 17:2082. doi: 10.3390/ijms17122082
7. Masarone, M , Rosato, V , Dallio, M , Gravina, AG , Aglitti, A , Loguercio, C, et al. Role of oxidative stress in pathophysiology of nonalcoholic fatty liver disease. Oxidative Med Cell Longev. (2018) 2018:9547613–4. doi: 10.1155/2018/9547613
8. Beier, JI , and Arteel, GE . Environmental exposure as a risk-modifying factor in liver diseases: knowns and unknowns. Acta Pharm Sin B. (2021) 11:3768–78. doi: 10.1016/j.apsb.2021.09.005
9. Sen, P , Qadri, S , Luukkonen, PK , Ragnarsdottir, O , McGlinchey, A , Jäntti, S, et al. Exposure to environmental contaminants is associated with altered hepatic lipid metabolism in non-alcoholic fatty liver disease. J Hepatol. (2022) 76:283–93. doi: 10.1016/j.jhep.2021.09.039
10. Costello, E , Rock, S , Stratakis, N , Eckel, SP , Walker, DI , Valvi, D, et al. Exposure to per- and polyfluoroalkyl substances and markers of liver injury: a systematic review and meta-analysis. Environ Health Perspect. (2022) 130:46001. doi: 10.1289/ehp10092
11. VoPham, T . Environmental risk factors for liver cancer and nonalcoholic fatty liver disease. Curr Epidemiol Rep. (2019) 6:50–66. doi: 10.1007/s40471-019-0183-2
12. Li, A , Pei, L , Zhao, M , Xu, J , Mei, Y , Li, R, et al. Investigating potential associations between O3 exposure and lipid profiles: a longitudinal study of older adults in Beijing. Environ Int. (2019) 133:105135. doi: 10.1016/j.envint.2019.105135
13. Papalou, O , Kandaraki, EA , Papadakis, G , and Diamanti-Kandarakis, E . Endocrine disrupting chemicals: An occult mediator of metabolic disease. Front Endocrinol. (2019) 10:112. doi: 10.3389/fendo.2019.00112
14. Bergman, A , Heindel, JJ , Kasten, T , Kidd, KA , Jobling, S , Neira, M, et al. The impact of endocrine disruption: a consensus statement on the state of the science. Environ Health Perspect. (2013) 121:A104–6. doi: 10.1289/ehp.1205448
15. Li, A , Zhou, Q , Mei, Y , Zhao, J , Liu, L , Zhao, M, et al. The effect of urinary essential and non-essential elements on serum albumin: evidence from a community-based study of the elderly in Beijing. Front Nutr. (2022) 9:946245. doi: 10.3389/fnut.2022.946245
16. Li, A , Li, Y , Mei, Y , Zhao, J , Zhou, Q , Li, K, et al. Associations of metals and metals mixture with lipid profiles: a repeated-measures study of older adults in Beijing. Chemosphere. (2023) 319:137833. doi: 10.1016/j.chemosphere.2023.137833
17. Cano, R , Pérez, JL , Dávila, LA , Ortega, Á , Gómez, Y , Valero-Cedeño, NJ, et al. Role of endocrine-disrupting Chemicals in the Pathogenesis of non-alcoholic fatty liver disease: a comprehensive review. Int J Mol Sci. (2021) 22:4807. doi: 10.3390/ijms22094807
18. Zhao, M , Yin, G , Xu, J , Ge, X , Li, A , Mei, Y, et al. Independent, combine and interactive effects of heavy metal exposure on dyslipidemia biomarkers: a cross-sectional study in northeastern China. Ecotoxicol Environ Saf. (2023) 250:114494. doi: 10.1016/j.ecoenv.2022.114494
19. Barouki, R . Endocrine disruptors: revisiting concepts and dogma in toxicology. C R Biol. (2017) 340:410–3. doi: 10.1016/j.crvi.2017.07.005
20. Frye, CA , Bo, E , Calamandrei, G , Calzà, L , Dessì-Fulgheri, F , Fernández, M, et al. Endocrine disrupters: a review of some sources, effects, and mechanisms of actions on behaviour and neuroendocrine systems. J Neuroendocrinol. (2012) 24:144–59. doi: 10.1111/j.1365-2826.2011.02229.x
21. Gore, AC , Chappell, VA , Fenton, SE , Flaws, JA , Nadal, A , Prins, GS, et al. Executive summary to EDC-2: the Endocrine Society's second scientific statement on endocrine-disrupting chemicals. Endocr Rev. (2015) 36:593–602. doi: 10.1210/er.2015-1093
22. Ribeiro, E , Ladeira, C , and Viegas, S . EDCs mixtures: a stealthy hazard for human health? Toxics. (2017) 5:5. doi: 10.3390/toxics5010005
23. Deierlein, AL , Rock, S , and Park, S . Persistent endocrine-disrupting chemicals and fatty liver disease. Curr. Environ. Health Rep. (2017) 4:439–49. doi: 10.1007/s40572-017-0166-8
24. Cao, L , Guo, Y , Chen, Y , Hong, J , Wu, J , and Hangbiao, J . Per-/Polyfluoroalkyl substance concentrations in human serum and their associations with liver cancer. Chemosphere. (2022) 296:134083. doi: 10.1016/j.chemosphere.2022.134083
25. Borghese, MM , Liang, CL , Owen, J , and Fisher, M . Individual and mixture associations of perfluoroalkyl substances on liver function biomarkers in the Canadian Health Measures Survey. Environ. Health. (2022) 21:85. doi: 10.1186/s12940-022-00892-6
26. Olsen, GW , Burris, JM , Ehresman, DJ , Froehlich, JW , Seacat, AM , Butenhoff, JL, et al. Half-life of serum elimination of perfluorooctanesulfonate, perfluorohexanesulfonate, and perfluorooctanoate in retired fluorochemical production workers. Environ Health Perspect. (2007) 115:1298–305. doi: 10.1289/ehp.10009
27. Jiang, JJ , Okvitasari, AR , Huang, FY , and Tsai, CS . Characteristics, pollution patterns and risks of perfluoroalkyl substances in drinking water sources of Taiwan. Chemosphere. (2021) 264:128579. doi: 10.1016/j.chemosphere.2020.128579
28. Zhou, J , Baumann, K , Mead, RN , Skrabal, SA , Kieber, RJ , Avery, GB, et al. PFOS dominates PFAS composition in ambient fine particulate matter (PM2.5) collected across North Carolina nearly 20 years after the end of its US production. Environ Sci Process Impacts. (2021) 23:580–7. doi: 10.1039/d0em00497a
29. Zhang, T , Sun, HW , Wu, Q , Zhang, XZ , Yun, SH , and Kannan, K . Perfluorochemicals in meat, eggs and indoor dust in China: assessment of sources and pathways of human exposure to perfluorochemicals. Environ Sci Technol. (2010) 44:3572–9. doi: 10.1021/es1000159
30. Hu, XC , Andrews, DQ , Lindstrom, AB , Bruton, TA , Schaider, LA , Grandjean, P, et al. Detection of poly- and perfluoroalkyl substances (PFASs) in U.S. drinking water linked to industrial sites, military fire training areas, and wastewater treatment plants. Environ Sci Technol Lett. (2016) 3:344–50. doi: 10.1021/acs.estlett.6b00260
31. Kannan, K , Corsolini, S , Falandysz, J , Fillmann, G , Kumar, KS , Loganathan, BG, et al. Perfluorooctanesulfonate and related fluorochemicals in human blood from several countries. Environ Sci Technol. (2004) 38:4489–95. doi: 10.1021/es0493446
32. Lucas, K , Gaines, LGT , Paris-Davila, T , and Nylander-French, LA . Occupational exposure and serum levels of per- and polyfluoroalkyl substances (PFAS): a review. Am J Ind Med. (2022) 1–14. doi: 10.1002/ajim.23454
33. Freberg, BI , Haug, LS , Olsen, R , Daae, HL , Hersson, M , Thomsen, C, et al. Occupational exposure to airborne perfluorinated compounds during professional ski waxing. Environ Sci Technol. (2010) 44:7723–8. doi: 10.1021/es102033k
34. Nian, M , Li, QQ , Bloom, M , Qian, ZM , Syberg, KM , Vaughn, MG, et al. Liver function biomarkers disorder is associated with exposure to perfluoroalkyl acids in adults: isomers of C8 health project in China. Environ Res. (2019) 172:81–8. doi: 10.1016/j.envres.2019.02.013
35. Jin, R , McConnell, R , Catherine, C , Xu, S , Walker, DI , Stratakis, N, et al. Perfluoroalkyl substances and severity of nonalcoholic fatty liver in children: An untargeted metabolomics approach. Environ Int. (2020) 134:105220. doi: 10.1016/j.envint.2019.105220
36. Gallo, V , Leonardi, G , Genser, B , Lopez-Espinosa, MJ , Frisbee, SJ , Karlsson, L, et al. Serum perfluorooctanoate (PFOA) and perfluorooctane sulfonate (PFOS) concentrations and liver function biomarkers in a population with elevated PFOA exposure. Environ Health Perspect. (2012) 120:655–60. doi: 10.1289/ehp.1104436
37. Khalil, N , Ebert, JR , Honda, M , Lee, M , Nahhas, RW , Koskela, A, et al. Perfluoroalkyl substances, bone density, and cardio-metabolic risk factors in obese 8–12 year old children: a pilot study. Environ Res. (2018) 160:314–21. doi: 10.1016/j.envres.2017.10.014
38. Stratakis, N , V. Conti, D , Jin, R , Margetaki, K , Valvi, D , Siskos, AP, et al. Prenatal exposure to perfluoroalkyl substances associated with increased susceptibility to liver injury in children. Hepatology. (2020) 72:1758–70. doi: 10.1002/hep.31483
39. Gleason, JA , Post, GB , and Fagliano, JA . Associations of perfluorinated chemical serum concentrations and biomarkers of liver function and uric acid in the us population (NHANES), 2007–2010. Environ Res. (2015) 136:8–14. doi: 10.1016/j.envres.2014.10.004
40. Xing, J , Wang, G , Zhao, J , Wang, E , Yin, B , Fang, D, et al. Toxicity assessment of perfluorooctane sulfonate using acute and subchronic male C57bl/6J mouse models. Environ Pollut. (2016) 210:388–96. doi: 10.1016/j.envpol.2015.12.008
41. das, KP , Wood, CR , Lin, MT , Starkov, AA , Lau, C , Wallace, KB, et al. Perfluoroalkyl acids-induced liver steatosis: effects on genes controlling lipid homeostasis. Toxicology. (2017) 378:37–52. doi: 10.1016/j.tox.2016.12.007
42. Schlezinger, JJ , Puckett, H , Oliver, J , Nielsen, G , Heiger-Bernays, W , and Webster, TF . Perfluorooctanoic acid activates multiple nuclear receptor pathways and skews expression of genes regulating cholesterol homeostasis in liver of humanized PPARα mice fed an American diet. Toxicol Appl Pharmacol. (2020) 405:115204. doi: 10.1016/j.taap.2020.115204
43. Behr, AC , Plinsch, C , Braeuning, A , and Buhrke, T . Activation of human nuclear receptors by perfluoroalkylated substances (PFAS). Toxicol. In Vitro. (2020) 62:104700. doi: 10.1016/j.tiv.2019.104700
44. Zhang, Y , Zhang, Y , Klaassen, CD , and Cheng, X . Alteration of bile acid and cholesterol biosynthesis and transport by perfluorononanoic acid (PFNA) in mice. Toxicol. Sci. (2018) 162:225–33. doi: 10.1093/toxsci/kfx237
45. Wan, C , Han, R , Liu, L , Zhang, F , Li, F , Xiang, M, et al. Role of Mir-155 in fluorooctane sulfonate-induced oxidative hepatic damage via the Nrf2-dependent pathway. Toxicol Appl Pharmacol. (2016) 295:85–93. doi: 10.1016/j.taap.2016.01.023
46. Lv, Z , Wu, W , Ge, S , Jia, R , Lin, T , Yuan, Y, et al. Naringin protects against perfluorooctane sulfonate-induced liver injury by modulating NRF2 and NF-κB in mice. Int Immunopharmacol. (2018) 65:140–7. doi: 10.1016/j.intimp.2018.09.019
47. Fang, X , Zou, S , Zhao, Y , Cui, R , Zhang, W , Hu, J, et al. Kupffer cells suppress perfluorononanoic acid-induced hepatic peroxisome proliferator-activated receptor α expression by releasing cytokines. Arch Toxicol. (2012) 86:1515–25. doi: 10.1007/s00204-012-0877-4
48. Han, R , Hu, M , Zhong, Q , Wan, C , Liu, L , Li, F, et al. Perfluorooctane sulphonate induces oxidative hepatic damage via mitochondria-dependent and NF-κB/TNF-α-mediated pathway. Chemosphere. (2018) 191:1056–64. doi: 10.1016/j.chemosphere.2017.08.070
49. Han, R , Zhang, F , Wan, C , Liu, L , Zhong, Q , and Ding, W . Effect of perfluorooctane sulphonate-induced kupffer cell activation on hepatocyte proliferation through the NF-κB/TNF-Α/Il-6-dependent pathway. Chemosphere. (2018) 200:283–94. doi: 10.1016/j.chemosphere.2018.02.137
50. Jain, RB , and Ducatman, A . Selective associations of recent low concentrations of perfluoroalkyl substances with liver function biomarkers: NHANES 2011 to 2014 data on us adults aged ≥20 years. J Occup Environ Med. (2019) 61:293–302. doi: 10.1097/jom.0000000000001532
51. Salihovic, S , Stubleski, J , Kärrman, A , Larsson, A , Fall, T , Lind, L, et al. Changes in markers of liver function in relation to changes in perfluoroalkyl substances – a longitudinal study. Environ Int. (2018) 117:196–203. doi: 10.1016/j.envint.2018.04.052
52. Yamaguchi, M , Arisawa, K , Uemura, H , Katsuura-Kamano, S , Takami, H , Sawachika, F, et al. Consumption of seafood, serum liver enzymes, and blood levels of PFOS and PFOA in the Japanese population. J Occup Health. (2013) 55:184–94. doi: 10.1539/joh.12-0264-oa
53. Lin, CY , Lin, LY , Chiang, CK , Wang, WJ , Su, YN , Hung, KY, et al. Investigation of the associations between low-dose serum perfluorinated chemicals and liver enzymes in us adults. Am J Gastroenterol. (2010) 105:1354–63. doi: 10.1038/ajg.2009.707
54. Attanasio, R . Sex differences in the association between Perfluoroalkyl acids and liver function in us adolescents: analyses of NHANES 2013–2016. Environ Pollut. (2019) 254:113061. doi: 10.1016/j.envpol.2019.113061
55. Butenhoff, JL , Olsen, GW , and Chang, S . Toxicological response of Sprague Dawley rats from inhalation exposure to perfluorooctane sulfonyl fluoride (POSF). Toxicol Lett. (2017) 271:38–49. doi: 10.1016/j.toxlet.2017.02.017
56. Butenhoff, JL , Chang, SC , Olsen, GW , and Thomford, PJ . Chronic dietary toxicity and carcinogenicity study with potassium perfluorooctanesulfonate in Sprague Dawley rats. Toxicology. (2012) 293:1–15. doi: 10.1016/j.tox.2012.01.003
57. Bagley, BD , Chang, SC , Ehresman, DJ , Eveland, A , Zitzow, JD , Parker, GA, et al. Perfluorooctane sulfonate-induced hepatic steatosis in male Sprague Dawley rats is not attenuated by dietary choline supplementation. Toxicol Sci. (2017) 160:284–98. doi: 10.1093/toxsci/kfx185
58. Darrow, LA , Groth, AC , Winquist, A , Shin, HM , Bartell, SM , and Steenland, K . Modeled perfluorooctanoic acid (PFOA) exposure and liver function in a mid-Ohio Valley Community. Environ Health Perspect. (2016) 124:1227–33. doi: 10.1289/ehp.1510391
59. Rantakokko, P , Männistö, V , Airaksinen, R , Koponen, J , Viluksela, M , Kiviranta, H, et al. Persistent organic pollutants and non-alcoholic fatty liver disease in morbidly obese patients: a cohort study. Environ. Health. (2015) 14:79. doi: 10.1186/s12940-015-0066-z
60. Wan, C , Gu, T , Ling, J , Qin, Y , Luo, J , Sun, L, et al. Perfluorooctane sulfonate aggravates CCl4-induced hepatic fibrosis via HMGB1/TLR4/Smad signaling. Environ Toxicol. (2022) 37:983–94. doi: 10.1002/tox.23458
61. Mamsen, LS , Björvang, RD , Mucs, D , Vinnars, MT , Papadogiannakis, N , Lindh, CH, et al. Concentrations of perfluoroalkyl substances (PFASS) in human embryonic and fetal organs from first, second, and third trimester pregnancies. Environ Int. (2019) 124:482–92. doi: 10.1016/j.envint.2019.01.010
62. Kim, HY , and Yoo, YH . Recombinant FGF21 attenuates polychlorinated biphenyl-induced NAFLD/NASH by modulating hepatic lipocalin-2 expression. Int J Mol Sci. (2022) 23:8899. doi: 10.3390/ijms23168899
63. Shi, H , Jan, J , Hardesty, JE , Falkner, KC , Prough, RA , Balamurugan, AN, et al. Polychlorinated biphenyl exposures differentially regulate hepatic metabolism and pancreatic function: implications for nonalcoholic steatohepatitis and diabetes. Toxicol Appl Pharmacol. (2019) 363:22–33. doi: 10.1016/j.taap.2018.10.011
64. Shipley, HJ , Sokoly, D , and Johnson, DW . Historical data review and source analysis of PCBs/Arochlors in the Lower Leon Creek Watershed. Environ Monit Assess. (2017) 189:75. doi: 10.1007/s10661-016-5720-6
65. Serdar, B , LeBlanc, WG , Norris, JM , and Dickinson, LM . Potential effects of polychlorinated biphenyls (PCBs) and selected organochlorine pesticides (OCPs) on immune cells and blood biochemistry measures: a cross-sectional assessment of the NHANES 2003–2004 data. Environ Health. (2014) 13:114. doi: 10.1186/1476-069x-13-114
66. Shin, MY , Shin, C , Choi, JW , Lee, J , Lee, S , and Kim, S . Pharmacokinetic profile of propyl paraben in humans after oral administration. Environ Int. (2019) 130:104917. doi: 10.1016/j.envint.2019.104917
67. Wahlang, B , Hardesty, JE , Jin, J , Falkner, KC , and Cave, MC . Polychlorinated biphenyls and nonalcoholic fatty liver disease. Curr. Opin. Toxicol. (2019) 14:21–8. doi: 10.1016/j.cotox.2019.06.001
68. Larigot, L , Juricek, L , Dairou, J , and Coumoul, X . AhR signaling pathways and regulatory functions. Biochim. Open. (2018) 7:1–9. doi: 10.1016/j.biopen.2018.05.001
69. Wahlang, B , Prough, RA , Falkner, KC , Hardesty, JE , Song, M , Clair, HB, et al. Polychlorinated biphenyl-xenobiotic nuclear receptor interactions regulate energy metabolism, behavior, and inflammation in non-alcoholic-steatohepatitis. Toxicol. Sci. (2016) 149:396–410. doi: 10.1093/toxsci/kfv250
70. Speliotes, EK , Butler, JL , Palmer, CD , Voight, BF , and Hirschhorn, JN . PNPLA3 variants specifically confer increased risk for histologic nonalcoholic fatty liver disease but not metabolic disease. Hepatology. (2010) 52:904–12. doi: 10.1002/hep.23768
71. Hardesty, JE , Wahlang, B , Falkner, KC , Shi, H , Jin, J , Wilkey, D, et al. Hepatic signalling disruption by pollutant polychlorinated biphenyls in steatohepatitis. Cell Signal. (2019) 53:132–9. doi: 10.1016/j.cellsig.2018.10.004
72. Midya, V , Colicino, E , Conti, DV , Berhane, K , Garcia, E , Stratakis, N, et al. Association of prenatal exposure to endocrine-disrupting chemicals with liver injury in children. JAMA Netw Open. (2022) 5:e2220176. doi: 10.1001/jamanetworkopen.2022.20176
73. Clair, HB , Pinkston, CM , Rai, SN , Pavuk, M , Dutton, ND , Brock, GN, et al. Liver disease in a residential cohort with elevated polychlorinated biphenyl exposures. Toxicol. Sci. (2018) 164:39–49. doi: 10.1093/toxsci/kfy076
74. Kumar, J , Lind, L , Salihovic, S , van Bavel, B , Ingelsson, E , and Lind, PM . Persistent organic pollutants and liver dysfunction biomarkers in a population-based human sample of men and women. Environ Res. (2014) 134:251–6. doi: 10.1016/j.envres.2014.07.023
75. Yorita Christensen, KL , Carrico, CK , Sanyal, AJ , and Gennings, C . Multiple classes of environmental chemicals are associated with liver disease: NHANES 2003–2004. Int J Hyg Environ Health. (2013) 216:703–9. doi: 10.1016/j.ijheh.2013.01.005
76. Cave, M , Appana, S , Patel, M , Falkner, KC , McClain, CJ , and Brock, G . Polychlorinated biphenyls, lead, and mercury are associated with liver disease in American adults: NHANES 2003–2004. Environ Health Perspect. (2010) 118:1735–42. doi: 10.1289/ehp.1002720
77. Xiao, X , Yeoh, BS , and Vijay-Kumar, M . Lipocalin 2: An emerging player in iron homeostasis and inflammation. Annu Rev Nutr. (2017) 37:103–30. doi: 10.1146/annurev-nutr-071816-064559
78. Shelby, MD . NTP-CERHR monograph on the potential human reproductive and developmental effects of bisphenol A. NTP CERHR MON. (2008) 22:v, vii–ix, 1–64 passim.
79. Uhm, JY , and Kim, HR . Cross-sectional association of urinary bisphenol A and vaccine-induced immunity against hepatitis B virus: data from the 2003–2014 national health and nutrition examination survey. Int J Environ Res Public Health. 19:1103. doi: 10.3390/ijerph19031103
80. Kim, Y , Park, M , Nam, DJ , Yang, EH , and Ryoo, JH . Relationship between seafood consumption and bisphenol A exposure: the Second Korean National Environmental Health Survey (KoNEHS 2012–2014). Ann Occupat Environ Med. (2020) 32:e10. doi: 10.35371/aoem.2020.32.e10
81. Vandenberg, LN , Hauser, R , Marcus, M , Olea, N , and Welshons, WV . Human exposure to bisphenol A (BPA). Reproduct Toxicol. (2007) 24:139–77. doi: 10.1016/j.reprotox.2007.07.010
82. Jalal, N , Surendranath, AR , Pathak, JL , Yu, S , and Chung, CY . Bisphenol A (BPA) the mighty and the mutagenic. Toxicol Rep. (2018) 5:76–84. doi: 10.1016/j.toxrep.2017.12.013
83. An, SJ , Yang, EJ , Oh, S , Park, KJ , Kim, T , Hong, YP, et al. The association between urinary bisphenol a levels and nonalcoholic fatty liver disease in Korean adults: Korean National Environmental Health Survey (KoNEHS) 2015–2017. Environ Health Prev Med. (2021) 26:91. doi: 10.1186/s12199-021-01010-7
84. Dallio, M , Masarone, M , Errico, S , Gravina, AG , Nicolucci, C , di Sarno, R, et al. Role of bisphenol a as environmental factor in the promotion of non-alcoholic fatty liver disease: in vitro and clinical study. Aliment Pharmacol Ther. (2018) 47:826–37. doi: 10.1111/apt.14499
85. Vahdati Hassani, F , Abnous, K , Mehri, S , Jafarian, A , Birner-Gruenberger, R , Yazdian Robati, R, et al. Proteomics and phosphoproteomics analysis of liver in male rats exposed to bisphenol A: mechanism of hepatotoxicity and biomarker discovery. Food Chem. Toxicol. (2018) 112:26–38. doi: 10.1016/j.fct.2017.12.021
86. Marmugi, A , Ducheix, S , Lasserre, F , Polizzi, A , Paris, A , Priymenko, N, et al. Low doses of bisphenol a induce gene expression related to lipid synthesis and trigger triglyceride accumulation in adult mouse liver. Hepatology. (2012) 55:395–407. doi: 10.1002/hep.24685
87. Dallio, M , Diano, N , Masarone, M , Gravina, AG , Patanè, V , Romeo, M, et al. Chemical effect of bisphenol a on non-alcoholic fatty liver disease. Int J Environ Res Public Health. (2019) 16:3134. doi: 10.3390/ijerph16173134
88. Hassan, ZK , Elobeid, MA , Virk, P , Omer, SA , ElAmin, M , Daghestani, MH, et al. Bisphenol a induces hepatotoxicity through oxidative stress in rat model. Oxidative Med Cell Longev. (2012) 2012:1–6. doi: 10.1155/2012/194829
89. Zhao, M , Ge, X , Xu, J , Li, A , Mei, Y , Yin, G, et al. Association between urine metals and liver function biomarkers in Northeast China: a cross-sectional study. Ecotoxicol Environ Saf. (2022) 231:113163. doi: 10.1016/j.ecoenv.2022.113163
90. Fu, X , He, J , Zheng, D , Yang, X , Wang, P , Tuo, F, et al. Association of endocrine disrupting chemicals levels in serum, environmental risk factors, and hepatic function among 5- to 14-year-old children. Toxicology. (2022) 465:153011. doi: 10.1016/j.tox.2021.153011
91. Federico, A , Dallio, M , Gravina, AG , Diano, N , Errico, S , Masarone, M, et al. The bisphenol a induced oxidative stress in non-alcoholic fatty liver disease male patients: a clinical strategy to antagonize the progression of the disease. Int J Environ Res Public Health. (2020) 17:3369. doi: 10.3390/ijerph17103369
92. Kim, D , Yoo, ER , Li, AA , Cholankeril, G , Tighe, SP , Kim, W, et al. Elevated urinary bisphenol a levels are associated with non-alcoholic fatty liver disease among adults in the United States. Liver Int. (2019) 39:1335–42. doi: 10.1111/liv.14110
93. Verstraete, SG , Wojcicki, JM , Perito, ER , and Rosenthal, P . Bisphenol a increases risk for presumed non-alcoholic fatty liver disease in hispanic adolescents in NHANES 2003–2010. Environ Health. (2018) 17:12. doi: 10.1186/s12940-018-0356-3
94. Lee, S , Lee, HA , Park, B , Han, H , Park, BH , Oh, SY, et al. A prospective cohort study of the association between bisphenol a exposure and the serum levels of liver enzymes in children. Environ Res. (2018) 161:195–201. doi: 10.1016/j.envres.2017.11.007
95. Albeldawi, M , Lopez, R , and Zein, NN . Is there an association between bisphenol a and liver disease? Results from NHANES 2005–2006. Gastroenterology. (2011) 140:S–728. doi: 10.1016/S0016-5085(11)63025-4
96. Arsik, I , Frediani, JK , Frezza, D , Chen, W , Ayer, T , Keskinocak, P, et al. Alanine aminotransferase as a monitoring biomarker in children with nonalcoholic fatty liver disease: a secondary analysis using tonic trial data. Children. (2018) 5:64. doi: 10.3390/children5060064
97. Martin-Rodriguez, JL , Gonzalez-Cantero, J , Gonzalez-Cantero, A , Arrebola, JP , and Gonzalez-Calvin, JL . Diagnostic accuracy of serum alanine aminotransferase as biomarker for nonalcoholic fatty liver disease and insulin resistance in healthy subjects, using 3t MR spectroscopy. Medicine. (2017) 96:e6770. doi: 10.1097/md.0000000000006770
98. Lang, IA , Galloway, TS , Scarlett, A , Henley, WE , Depledge, M , Wallace, RB, et al. Association of Urinary Bisphenol A concentration with medical disorders and laboratory abnormalities in adults. JAMA. (2008) 300:1303–10. doi: 10.1001/jama.300.11.1303
99. Liu, R , Liu, B , Tian, L , Jiang, X , Li, X , Cai, D, et al. Exposure to bisphenol A caused hepatoxicity and intestinal flora disorder in rats. Int J Mol Sci. (2022) 23:8042. doi: 10.3390/ijms23148042
100. Puttabyatappa, M , Martin, JD , Andriessen, V , Stevenson, M , Zeng, L , Pennathur, S, et al. Developmental programming: changes in mediators of insulin sensitivity in prenatal bisphenol a-treated female sheep. Reprod Toxicol. (2019) 85:110–22. doi: 10.1016/j.reprotox.2019.03.002
101. Puttabyatappa, M , Saadat, N , Elangovan, VR , Dou, J , Bakulski, K , and Padmanabhan, V . Developmental programming: impact of prenatal bisphenol-a exposure on liver and muscle transcriptome of female sheep. Toxicol Appl Pharmacol. (2022) 451:116161. doi: 10.1016/j.taap.2022.116161
102. Long, Z , Fan, J , Wu, G , Liu, X , Wu, H , Liu, J, et al. Gestational bisphenol a exposure induces fatty liver development in male offspring mice through the inhibition of HNF1b and upregulation of PPARγ. Cell Biol Toxicol. (2021) 37:65–84. doi: 10.1007/s10565-020-09535-3
103. Chen, Y , Li, C , Song, P , Yan, B , Yang, X , Wu, Y, et al. Hepatic and renal tissue damage in Balb/C mice exposed to diisodecyl phthalate: the role of oxidative stress pathways. Food Chem Toxicol. (2019) 132:110600. doi: 10.1016/j.fct.2019.110600
104. Milošević, N , Milić, N , Živanović Bosić, D , Bajkin, I , Perčić, I , Abenavoli, L, et al. Potential influence of the phthalates on normal liver function and cardiometabolic risk in males. Environ Monit Assess. (2017) 190:17. doi: 10.1007/s10661-017-6398-0
105. Schettler, T . Human exposure to phthalates via consumer products. Int J Androl. (2006) 29:134–9; discussion 81-5. doi: 10.1111/j.1365-2605.2005.00567.x
106. Wormuth, M , Scheringer, M , Vollenweider, M , and Hungerbühler, K . What are the sources of exposure to eight frequently used phthalic acid esters in Europeans? Risk Anal. (2006) 26:803–24. doi: 10.1111/j.1539-6924.2006.00770.x
107. Frederiksen, H , Skakkebaek, NE , and Andersson, AM . Metabolism of phthalates in humans. Mol Nutr Food Res. (2007) 51:899–911. doi: 10.1002/mnfr.200600243
108. Chen, H , Zhang, W , Rui, BB , Yang, SM , Xu, WP , and Wei, W . Di(2-Ethylhexyl) phthalate exacerbates non-alcoholic fatty liver in rats and its potential mechanisms. Environ Toxicol Pharmacol. (2016) 42:38–44. doi: 10.1016/j.etap.2015.12.016
109. Zhang, Y , Wang, S , Zhao, T , Yang, L , Guo, S , Shi, Y, et al. Mono-2-ethylhexyl phthalate (MEHP) promoted lipid accumulation via JAK2/STAT5 and aggravated oxidative stress in BRL-3A cells. Ecotoxicol Environ Saf. (2019) 184:109611. doi: 10.1016/j.ecoenv.2019.109611
110. Park, CG , Sung, B , Ryu, CS , and Kim, YJ . Mono-(2-ethylhexyl) phthalate induces oxidative stress and lipid accumulation in zebrafish liver cells. Comparat Biochem Physiol Toxicol Pharmacol. (2020) 230:108704. doi: 10.1016/j.cbpc.2020.108704
111. Peverill, W , Powell, LW , and Skoien, R . Evolving concepts in the pathogenesis of NASH: beyond steatosis and inflammation. Int J Mol Sci. (2014) 15:8591–638. doi: 10.3390/ijms15058591
112. Zhang, Y , Hui, J , Xu, Y , Ma, Y , Sun, Z , Zhang, M, et al. Mehp promotes liver fibrosis by down-regulating STAT5A in BRL-3A hepatocytes. Chemosphere. (2022) 295:133925. doi: 10.1016/j.chemosphere.2022.133925
113. Zhao, ZB , Ji, K , Shen, XY , Zhang, WW , Wang, R , Xu, WP, et al. Di(2-Ethylhexyl) phthalate promotes hepatic fibrosis by regulation of oxidative stress and inflammation responses in rats. Environ Toxicol Pharmacol. (2019) 68:109–19. doi: 10.1016/j.etap.2019.03.008
114. Cai, S , Fan, J , Ye, J , Rao, X , and Li, Y . Phthalates exposure is associated with non-alcoholic fatty liver disease among us adults. Ecotoxicol Environ Saf. (2021) 224:112665. doi: 10.1016/j.ecoenv.2021.112665
115. Berman, YE , Doherty, DA , Mori, TA , Beilin, LJ , Ayonrinde, OT , Adams, LA, et al. Associations between prenatal exposure to phthalates and features of the metabolic syndrome in males from childhood into adulthood. Int J Environ Res Public Health. (2022) 19:15244. doi: 10.3390/ijerph192215244
116. Chen, X , Tian, F , Wu, J , Liu, L , Li, Y , Yu, G, et al. Associations of phthalates with NAFLD and liver fibrosis: a nationally representative cross-sectional study from NHANES 2017–2018. Front Nutr. (2022) 9:1059675. doi: 10.3389/fnut.2022.1059675
117. Li, W , Xiao, H , Wu, H , Pan, C , Deng, K , Xu, X, et al. Analysis of environmental chemical mixtures and nonalcoholic fatty liver disease: NHANES 1999–2014. Environ Pollut. (2022) 311:119915. doi: 10.1016/j.envpol.2022.119915
118. Yang, EJ , Choi, BS , and Yang, YJ . Risk of nonalcoholic fatty liver disease is associated with urinary phthalate metabolites levels in adults with subclinical hypothyroidism: Korean National Environmental Health Survey (KoNEHS) 2012–2014. Int J Environ Res Public Health. (2022) 19:3267. doi: 10.3390/ijerph19063267
119. Yang, YJ , Kim, T , and Hong, YP . Urinary phthalate levels associated with the risk of nonalcoholic fatty liver disease in adults: the Korean National Environmental Health Survey (KoNEHS) 2012–2014. Int J Environ Res Public Health. (2021) 18:6035. doi: 10.3390/ijerph18116035
120. Yu, L , Yang, M , Cheng, M , Fan, L , Wang, X , Xu, T, et al. Associations between urinary phthalate metabolite concentrations and markers of liver injury in the us adult population. Environ Int. (2021) 155:106608. doi: 10.1016/j.envint.2021.106608
121. Milošević, N , Milanović, M , Sudji, J , Bosić Živanović, D , Stojanoski, S , Vuković, B, et al. Could phthalates exposure contribute to the development of metabolic syndrome and liver disease in humans? Environ Sci Pollut Res Int. (2020) 27:772–84. doi: 10.1007/s11356-019-06831-2
122. Medic-Stojanoska, M , Milosevic, N , Milanovic, M , Stojanoski, S , and Milic, N . Can phthalates impair liver function? Endocrine abstracts (2019) 63 GP232. doi: 10.1530/endoabs.63.GP232
123. Zhou, J , Tripathi, M , Ho, JP , Widjaja, AA , Shekeran, SG , Camat, MD, et al. Thyroid hormone decreases hepatic steatosis, inflammation, and fibrosis in a dietary mouse model of nonalcoholic steatohepatitis. Thyroid. (2022) 32:725–38. doi: 10.1089/thy.2021.0621
124. Liu, L , Yu, Y , Zhao, M , Zheng, D , Zhang, X , Guan, Q, et al. Benefits of levothyroxine replacement therapy on nonalcoholic fatty liver disease in subclinical hypothyroidism patients. Int J Endocrinol. (2017) 2017:5753039–10. doi: 10.1155/2017/5753039
125. Shen, O , du, G , Sun, H , Wu, W , Jiang, Y , Song, L, et al. Comparison of in vitro hormone activities of selected phthalates using reporter gene assays. Toxicol Lett. (2009) 191:9–14. doi: 10.1016/j.toxlet.2009.07.019
126. Buzzetti, E , Parikh, PM , Gerussi, A , and Tsochatzis, E . Gender differences in liver disease and the drug-dose gender gap. Society. (2017) 120:97–108. doi: 10.1016/j.phrs.2017.03.014
127. Li, MC , Chen, PC , Tsai, PC , Furue, M , Onozuka, D , Hagihara, A, et al. Mortality after exposure to polychlorinated biphenyls and polychlorinated dibenzofurans: a meta-analysis of two highly exposed cohorts. Int J Cancer. (2015) 137:1427–32. doi: 10.1002/ijc.29504
128. Pan, JJ , and Fallon, MB . Gender and racial differences in nonalcoholic fatty liver disease. World J Hepatol. (2014) 6:274–83. doi: 10.4254/wjh.v6.i5.274
129. Takeuchi, T , Tsutsumi, O , Nakamura, N , Ikezuki, Y , Takai, Y , Yano, T, et al. Gender difference in serum bisphenol a levels may be caused by liver UDP-glucuronosyltransferase activity in rats. Biochem Biophys Res Commun. (2004) 325:549–54. doi: 10.1016/j.bbrc.2004.10.073
130. Takeuchi, T , and Tsutsumi, O . Serum bisphenol a concentrations showed gender differences, possibly linked to androgen levels. Biochem Biophys Res Commun. (2002) 291:76–8. doi: 10.1006/bbrc.2002.6407
131. Trasande, L , Sathyanarayana, S , Spanier, AJ , Trachtman, H , Attina, TM , and Urbina, EM . Urinary phthalates are associated with higher blood pressure in childhood. J Pediatr. (2013) 163:747–53.e1. doi: 10.1016/j.jpeds.2013.03.072
Keywords: non-alcoholic fatty liver disease, endocrine-disrupting chemicals, per-/polyfluorinated substance, bisphenol A, polychlorinated biphenyls, phthalates
Citation: Chen Y, Wang Y, Cui Z, Liu W, Liu B, Zeng Q, Zhao X, Dou J and Cao J (2023) Endocrine disrupting chemicals: A promoter of non-alcoholic fatty liver disease. Front. Public Health. 11:1154837. doi: 10.3389/fpubh.2023.1154837
Edited by:
Qun Xu, Chinese Academy of Medical Sciences and Peking Union Medical College, ChinaReviewed by:
Weitao Su, Fujian Medical University, ChinaBingqing Zhao, Stanford University, United States
Copyright © 2023 Chen, Wang, Cui, Liu, Liu, Zeng, Zhao, Dou and Cao. This is an open-access article distributed under the terms of the Creative Commons Attribution License (CC BY). The use, distribution or reproduction in other forums is permitted, provided the original author(s) and the copyright owner(s) are credited and that the original publication in this journal is cited, in accordance with accepted academic practice. No use, distribution or reproduction is permitted which does not comply with these terms.
*Correspondence: Jinglin Cao, 380176833@qq.com