- Parasite and Host Biology Group, ICMR-National Institute of Malaria Research, New Delhi, India
Introduction: In 2021, India contributed for ~79% of malaria cases and ~ 83% of deaths in the South East Asia region. Here, we systematically and critically analyzed data published on malaria in pregnancy (MiP) in India.
Methods: Epidemiological, clinical, parasitological, preventive and therapeutic aspects of MiP and its consequences on both mother and child were reviewed and critically analyzed. Knowledge gaps and solution ways are also presented and discussed. Several electronic databases including Google scholar, Google, PubMed, Scopus, Wiley Online library, the Malaria in Pregnancy Consortium library, the World Malaria Report, The WHO regional websites, and ClinicalTrials.gov were used to identify articles dealing with MiP in India. The archives of local scientific associations/journals and website of national programs were also consulted.
Results: Malaria in pregnancy is mainly due to Plasmodium falciparum (Pf) and P. vivax (Pv), and on rare occasions to P. ovale spp. and P. malariae too. The overall prevalence of MiP is ~0.1–57.7% for peripheral malaria and ~ 0–29.3% for placental malaria. Peripheral Pf infection at antenatal care (ANC) visits decreased from ~13% in 1991 to ~7% in 1995–1996 in Madhya Pradesh, while placental Pf infection at delivery unit slightly decreased from ~1.5% in 2006–2007 to ~1% in 2012–2015 in Jharkhand. In contrast, the prevalence of peripheral Pv infection at ANC increased from ~1% in 2006–2007 to ~5% in 2015 in Jharkhand, and from ~0.5% in 1984–1985 to ~1.5% in 2007–2008 in Chhattisgarh. Clinical presentation of MiP is diverse ranging from asymptomatic carriage of parasites to severe malaria, and associated with comorbidities and concurrent infections such as malnutrition, COVID-19, dengue, and cardiovascular disorders. Severe anemia, cerebral malaria, severe thrombocytopenia, and hypoglycemia are commonly seen in severe MiP, and are strongly associated with tragic consequences such as abortion and stillbirth. Congenital malaria is seen at prevalence of ~0–12.9%. Infected babies are generally small-for-gestational age, premature with low birthweight, and suffer mainly from anemia, thrombocytopenia, leucopenia and clinical jaundice. Main challenges and knowledge gaps to MiP control included diagnosis, relapsing malaria, mixed Plasmodium infection treatment, self-medication, low density infections and utility of artemisinin-based combination therapies.
Conclusion: All taken together, the findings could be immensely helpful to control MiP in malaria endemic areas.
1. Introduction
Globally, an estimated 247 million cases and 619,000 deaths were due to malaria in 2021 (1). This burden has significantly increased compared to previous years, partially due to the current COVID-19 pandemics (2, 3). Malaria is due to five Plasmodium species that are transmitted to human through infecting bites of female Anopheles mosquitoes (4). Plasmodium falciparum (Pf) and Plasmodium vivax (Pv) are the predominant malaria species around the world; Pf is the most severe and dangerous species while Pv is the most geographically spread but can also induce severe clinical attacks (5, 6).
The sub-Saharan Africa (sSA) and South East Asia (SEA) regions are affected by malaria, especially children under 5 years of age and pregnant women (2). Malaria during pregnancy (MiP) poses an important problem to both; the future mother and unborn child. During pregnancy, malaria infection is associated with several maternal, fetal and birth complications including growth restriction, stillbirth, premature delivery, spontaneous abortions, low birth weight (LBW), and even death of mother and/or child (7, 8). The clinical spectrum and outcomes of MiP are various with distinct features depending on factors such as epidemiological situation of the setting, malaria species, gravidity, and coverage of malaria control measures [e.g., intermittent preventive treatment with sulfadoxine + pyrimethamine – IPTp-SP, indoor residual spraying (IRS), and long lasting insecticide-treated nets (LLINs)] (2).
India accounted for ~79% of malaria cases and ~ 83% of deaths seen in SEA in 2021 (2). In the present review, we reviewed the situation of MiP in India with emphasis on its epidemiology, clinical presentation, determinants, outcomes, prevention, and treatment. A brief overview of neonatal and congenital malaria (NCM) characteristics is also presented. Finally, we identify knowledge gaps on MiP research and propose solutions for future directions.
2. Materials and methods
2.1. Search strategy
The strategy used to identify relevant studies was inspired from MiP reviews reported previously (7, 9). Briefly, we used Google scholar, Google, PubMed, Scopus, Wiley Online library, the Malaria in Pregnancy Consortium library, the World Malaria Report, The WHO regional websites, and ClinicalTrials.gov to search for articles dealing with MiP in India published in last 50 years. The archives of local scientific associations/journals (e.g., Indian Journal of Medical Research, Indian Journal of Malariology now known as Journal of Vector Borne Diseases) and websites of national programs were also consulted. We used the search terms “malaria,” “pregnancy,” “pregnant woman,” “burden,” “prevalence,” “epidemiology,” “outcome,” “placental infection,” “congenital malaria,” “neonatal malaria,” “diagnostic,” “prevention,” “control,” “management,” “India,” and Indian regions (Tamil Nadu, Chandigarh, Andaman and Nicobar, Assam, Andhra Pradesh, Bihar, Chhattisgarh, Daman and Diu, Goa, Delhi, Gujarat, Himachal Pradesh, Jammu and Kashmir, Jharkhand, Kerala, Kolkata, Karnataka, Lakshadweep, Maharashtra, Manipur, Mizoram, Madhya Pradesh, Meghalaya, Nagaland, Odisha, Pondicherry, Rajasthan, Sikkim, Tripura, Uttarakhand, Uttar Pradesh, Punjab, Haryana, and West Bengal). Boolean operators including “AND” and “OR” were used in combination with the above mentioned search terms to identify relevant papers through databases such as PubMed. The search strategy was tailored to each of the search databases using search terms and Boolean operators (AND, OR). We also included all publications on neonatal and congenital malaria (NCM). To do so, the same search strategy used for MiP papers was used with some differences. For example search terms for NCM papers were “malaria,” “placental infection,” “congenital malaria,” “neonatal malaria,” “India,” and Indian regions (Tamil Nadu, Chandigarh, Andaman and Nicobar, Assam, Andhra Pradesh, Bihar, Chhattisgarh, Daman and Diu, Goa, Delhi, Gujarat, Himachal Pradesh, Jammu and Kashmir, Jharkhand, Kerala, Kolkata, Karnataka, Lakshadweep, Maharashtra, Manipur, Mizoram, Madhya Pradesh, Meghalaya, Nagaland, Odisha, Pondicherry, Rajasthan, Sikkim, Tripura, Uttarakhand, Uttar Pradesh, Punjab, Haryana, and West Bengal).
2.2. Screening strategy
Titles and abstracts of studies retrieved from databases were independently reviewed by the authors in order to identify those relevant to the study. The full texts were retrieved and scrutinized to extract data of interest. Principal investigators were kindly contacted to request full length paper and/or more details on studies. We also contacted editors-in-chief of national journals to request full length papers in case of a negative reply or no reply at all from principal investigators. Additionally, we reviewed relevant articles cited in references of identified literature and included them as primary sources.
2.3. Eligibility criteria
Only papers published in English and Hindi were included. Publications were considered of interest if they addressed any aspect of MiP and NCM in India including prevalence, clinical presentation, determinants, maternal and fetal/neonatal outcomes, diagnostic, prevention, and treatment. The list of studies is presented in Supplementary Tables 1–5.
2.4. Data extraction
Data of interest were independently extracted from eligible publications, and these consisted of (i) characteristics of studies (first author’ name, year of publication, study design, area, state/union territory, urbanization setting, and year of sample collection); (ii) demographical, obstetrical, and gynecological data (age, timing of screening, parity, trimester of gestation, and route of delivery); (iii) clinical characteristics of MiP and NCM (type of malaria, clinical signs/symptoms, presence of comorbidities, and levels of hemoglobin, blood cells, and biochemical markers); (iv) parasitological information (blood source, parasitological screening method, malaria species, total number of individuals included, total number of malaria infected individuals, number of mono-infections for each malaria species, and number of mixed infections), (v) factors associated with malaria infection in pregnant women and babies; (vi) MiP and NCM outcomes, (vii) malaria preventive methods used, and (viii) efficacy of treatments to control MiP. Setting urbanization was categorized as urban, semi-urban, rural, and not specified. Timing of screening consisted of women screened for malaria parasites in community or at health facility for delivery (DU) and antenatal care visit (ANC). Type of malaria was defined either as asymptomatic malaria, uncomplicated malaria (UM) or severe malaria (SM). In the early 90s, the WHO defined a set of criteria used for diagnosing severe malaria (SM) in children and adults (Table 1) (6, 10, 12–16). The latest WHO guideline lists 12 SM-associated signs/symptoms by malarial species and age group: severe malarial anemia (SMA), severe renal impairment, cerebral malaria (CM; prostration, impaired consciousness/coma, and multiple convulsions), jaundice, hypoglycemia, acidosis/acute respiratory distress syndrome (ARDS), significant bleeding, pulmonary edema, circulatory collapse/shock, and hyperparasitemia (10).
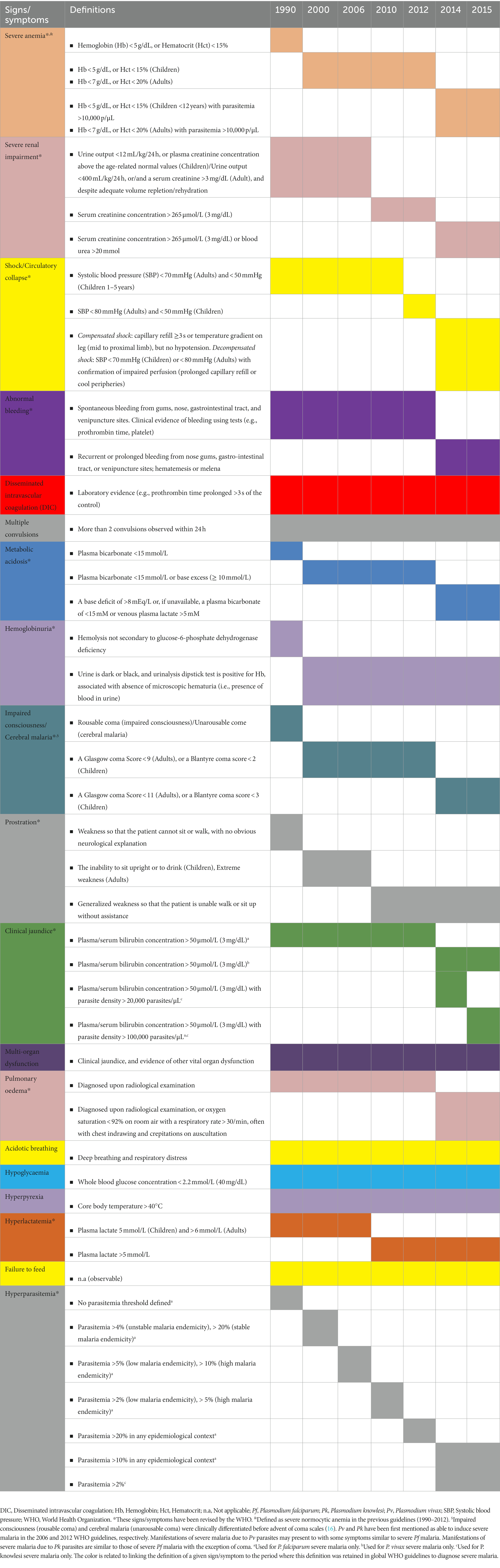
Table 1. Evolution of WHO definitions of severe malaria clinical and laboratory manifestations due to Plasmodium falciparum (1990–2015), Plasmodium vivax (2006–2015), and Plasmodium knowlesi (2012–2015) in children, non-pregnant adults, and pregnant women (10–16).
2.5. Data management
Data were keyed into an Excel spreadsheet (Microsoft Office, United States) by reviewer authors and then coded and verified for consistency, and removed any duplicates. Any discrepancies between the authors were resolved through discussion and consensus. Data were analyzed using StatView 5.0 for Windows (SAS Institute, Inc., Chicago, Illinois, United States) and GraphPad v8.01 for Windows (GraphPad, Inc., California, United States), and summarized as percentages and mean in tables and charts where appropriate. Overall aggregation of data using sophisticated approaches such as meta-analysis was not possible due to the heterogeneity of studies related to study design, diagnostic methods, timing of screening, blood origin, analysis conducted, and effect measures presented. Thus, only summaries of study findings stratified by variables such as diagnostic methods, timing of screening and blood origin are presented in this review. In addition, findings from studies with minimum sample size of 30 were extracted to generate charts (17). We think that this approach of analysis is more appropriate to provide reliable results and avoid misleading conclusions on Indian scenario of MiP and NCM.
3. Results
3.1. Burden of malaria in India
Malaria burden profoundly decreased in last two decades in India, and this is due to diverse strategies implemented and/or scaled up over the country (e.g., LLINs, IRS) (6). The recent national data from National Vector Borne Disease Control Program (NVBDCP) indicated that malaria transmission is low with annual parasite incidence (API) < 1 in most of the areas of India (https://nvbdcp.gov.in; Figure 1A). Malaria control needs to be reinforced in some areas including Bihar, Delhi, Uttarakhand, Orissa, Chhattisgarh, and West Bengal where API is >2 (Figure 1A). The risk of malaria infection is highest in two North-Eastern areas of India namely Tripura and Mizoram with API > 10.
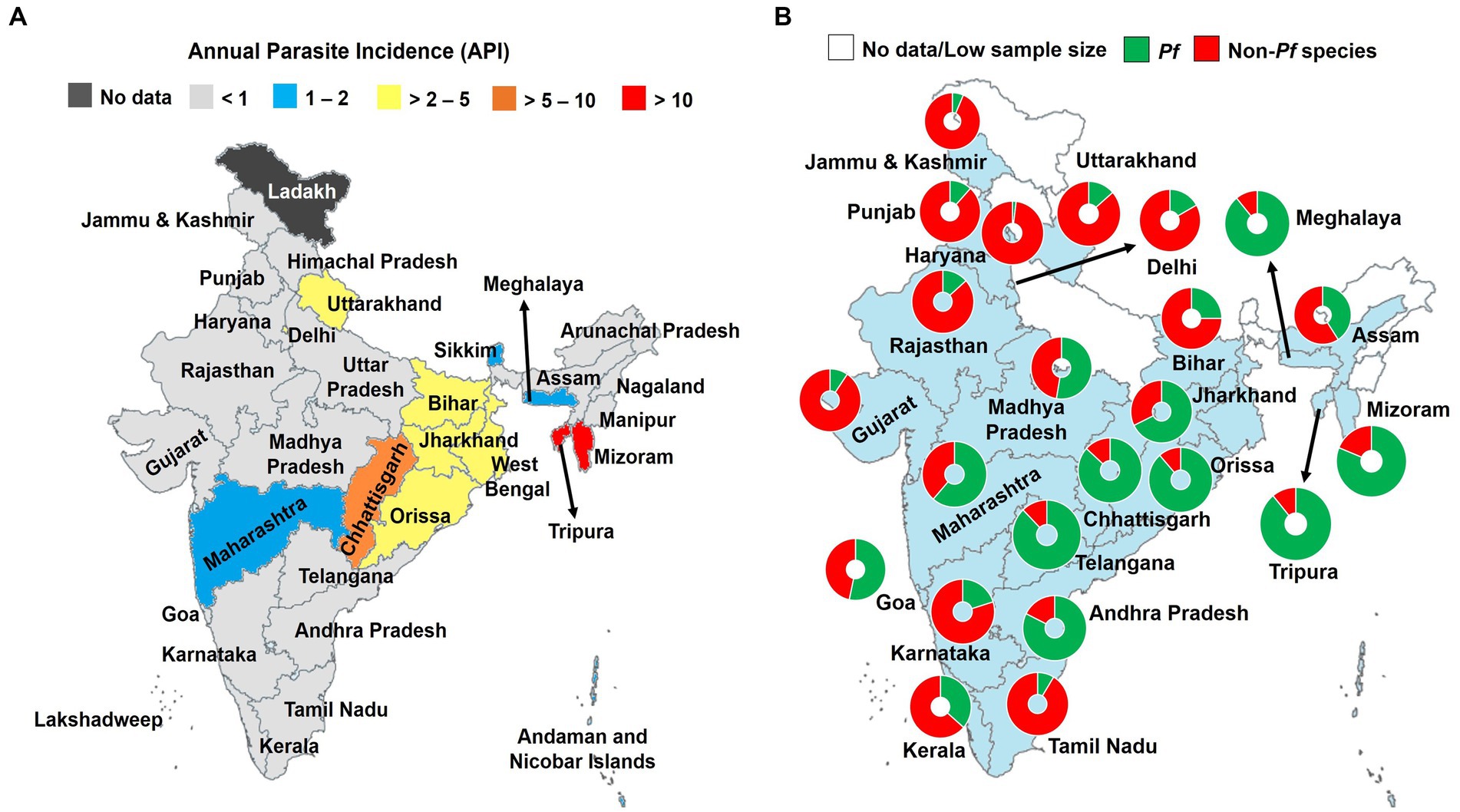
Figure 1. Burden of malaria in India, 2021. (A) Annual parasite incidence of Plasmodium spp. infections, and (B) contribution of Pf and non-Pf species. API, Annual parasite incidence; Pf, Plasmodium falciparum; Pv, P. vivax. Malaria species were identified using light microscopy Non-Pf species are mostly represented by Pv (>90%). The data were retrieved from official website of the National Vector Borne Disease Control Program (https://nvbdcp.gov.in). Pie charts depict the relative contribution of malaria species in total malaria cases. Only sample size of malaria positive slides >30 were retained and presented in (B) (17). The map was retrieved from the official website of the Ministry of External Affairs of Government of India (https://mea.gov.in/india-at-glance.htm).
The Pf and Pv species are major species in the country, with ratio close to one at the country level but varied between the different regions (Figure 1B) (18–20). Other species including P. ovale (Po), P. malariae (Pm), and P. knowlesi (Pk) are also found in India but much fewer in extent than that of Pf and Pv (21–24). The Pm species was reported from Madhya Pradesh, Andhra Pradesh, Tamil Nadu, Kerala, Karnataka, and Orissa; while Po spp. was mainly seen in Uttar Pradesh, Assam, and Gujarat states (21, 23). Tyagi and colleagues reported the circulation of Pk in patients living in the Andaman and Nicobar Islands (24). Recently, Pk was reported from Bihar, Uttar Pradesh, and Delhi (22).
3.2. Burden of MiP in India
Data on the epidemiology of MiP in India are largely heterogeneous due to variation in diagnostic methods (LM, PCR, RDT, and histology), timing of screening (ANC, DU, and community), and blood origin (peripheral and placental blood). Again, results are more documented from forested, tribal, and rural areas of three states viz. Madhya Pradesh, Rajasthan, and Chhattisgarh (Table 2; Figure 2). Information on MiP epidemiology is lacking in highly malaria prevalent areas such as Delhi, Bihar, and some North Eastern states (e.g., Arunachal Pradesh and Nagaland). On ANC visits, prevalence of MiP in Madhya Pradesh state ranged from 1.9 to 17.9% using LM for detecting malaria parasites in the peripheral blood (Table 2). In Chhattisgarh, peripheral blood-based MiP prevalence ranged from 20.6 and 29.3% using RDT (42, 49). Combining different diagnostic methods, several authors reported placental malaria prevalence at DU of 2.2 and 29.3% in Madhya Pradesh (35, 40), and 21.9% in Uttar Pradesh (26). In Madhya Pradesh and Chhattisgarh, placental malaria was diagnosed at higher rates using PCR compared to impression smear and LM (44, 47), thereby outlining a high proportion of submicroscopic infections during pregnancy. Fewer studies reported MiP burden in community where peripheral malaria prevalence rates of 55.5% using LM and 0.81% using RDT were reported in Madhya Pradesh and Chhattisgarh, respectively (33, 54).
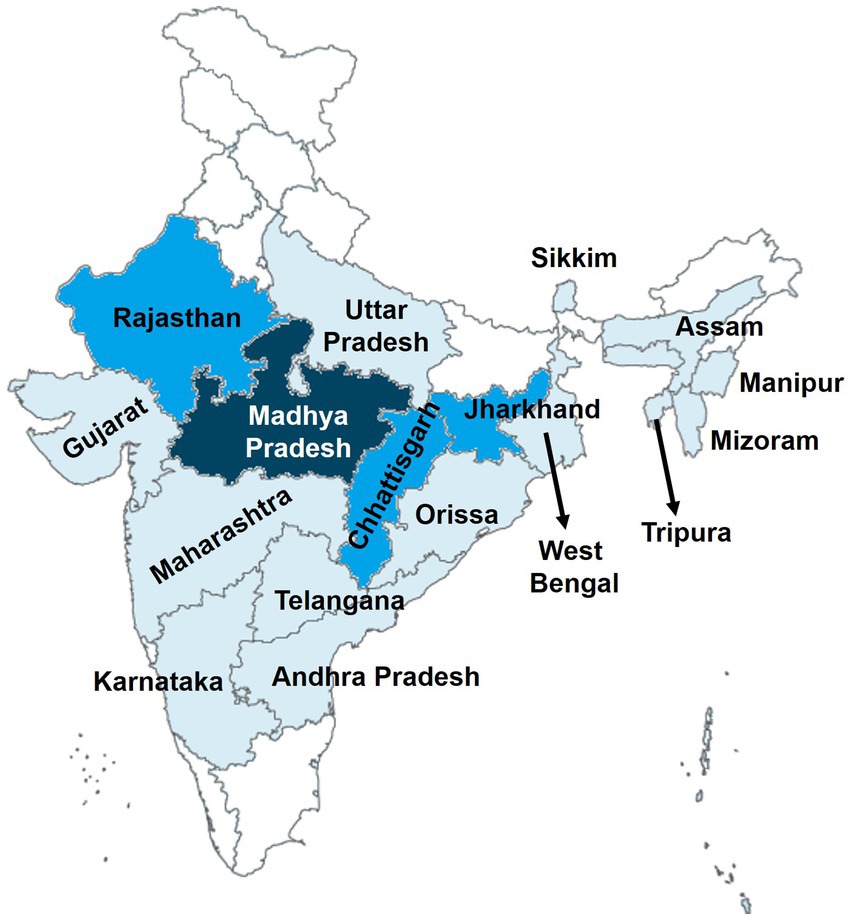
Figure 2. Geographical distribution of studies on MiP in India. The map was retrieved from official website of the Ministry of External Affairs of Government of India (https://mea.gov.in/india-at-glance.htm). Areas colored represent the places where studies on MiP were conducted. The darker the area higher is the number of conducted studies on MiP in those areas.
3.3. Plasmodium species involved in MiP
The information on Plasmodium species-wise MiP proportion is mainly originated from hospital based studies, in a limited number of states such as Madhya Pradesh, Jharkhand, Maharashtra, Rajasthan, and Chhattisgarh (Figure 2). Pf and Pv are predominant species involved in MiP irrespective of diagnostic method and timing of screening. In Madhya Pradesh, Pf was the main MiP-associated malaria species on ANC visits and DU with overall peripheral Pf mono-infections prevalence of 3.4–48.5% based on LM (Figure 3) (28, 30, 31, 33, 36, 43). Likewise, a trend of Pf dominance was also seen in Chhattisgarh with LM-based placental prevalence of Pf mono-infections ranging from 1.2 to 3.2% (Figure 4), even though the contribution of plasmodial species can vary within the same state (41, 47, 57). Singh and colleagues conducted a study in two districts of Chhattisgarh with different malarial endemicity level (i.e., Rajnandgaon and Bastar), and showed that Pv was dominant in Rajnandgaon (low endemic area) both at ANC and DU while Pf was dominant in Bastar (high endemic area; Figure 3) (41). The same team reported Pm as additional cause of MiP in Chhattisgarh using PCR method (47). Similarly, Po was detected as mixed infection with Pf in a multicentric study (58). One study from Rajasthan reported the predominance of Pv species which accounted for 96.2% of all LM-detected peripheral infections among women attending ANC visits (Figure 3) (50).
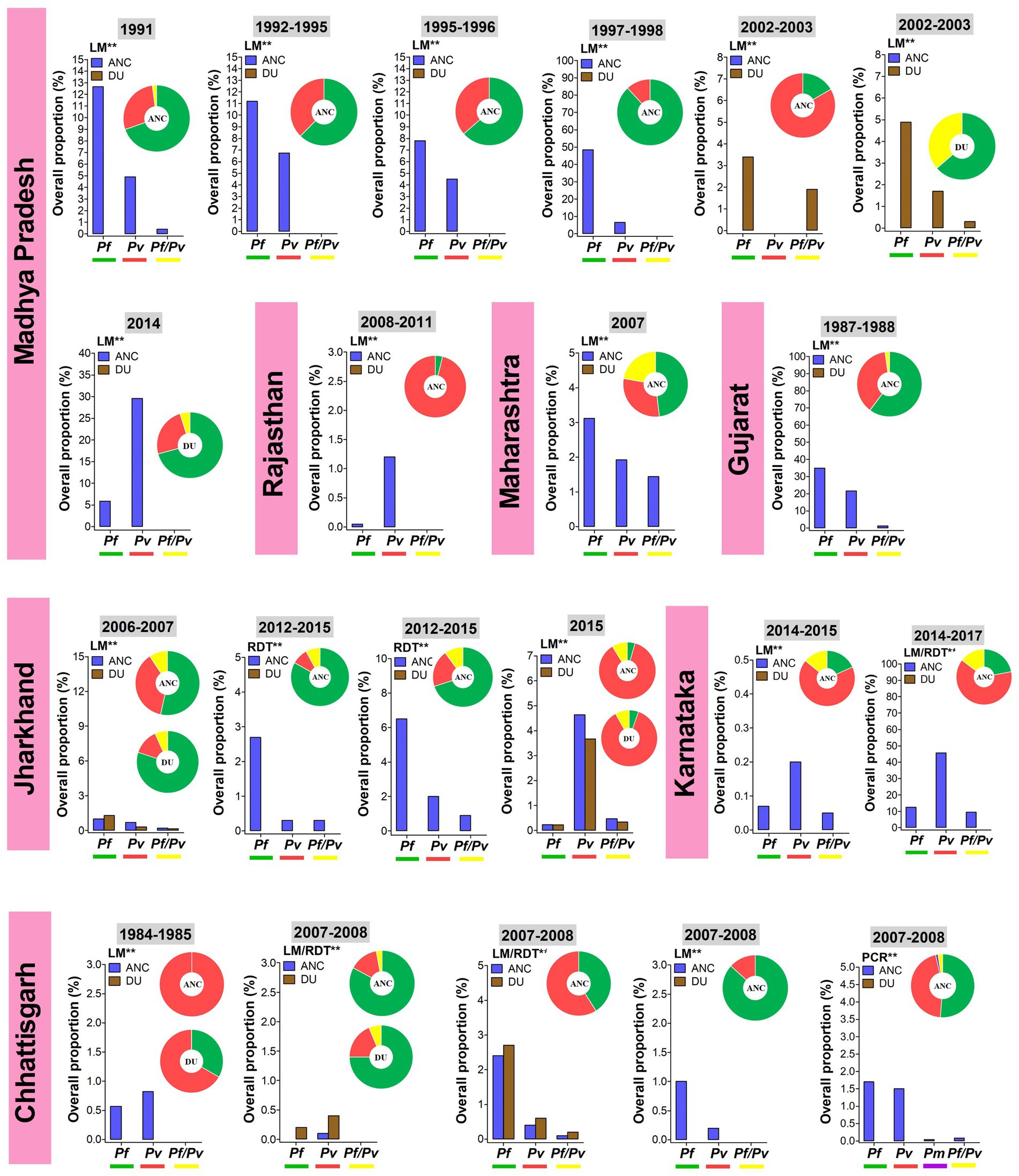
Figure 3. Overall proportion and contribution of Plasmodium species either as peripheral mono- or mixed infections in ANC and DU by states. ANC, Antenatal care visit; DU, Delivery unit; LM, Light microscopy; RDT, Rapid diagnostic test; PCR, Polymerase chain reaction; Pf, Plasmodium falciparum; Pm, Plasmodium malariae; Pv, Plasmodium vivax; Pf/Pv, Mixed infection with P. falciparum and P. vivax. Bars represent overall proportions of Plasmodium infections which are computed as ratio of number of patients with Plasmodium species either mono-infection or mixed infections to total number of patients. Pie charts represent the specific proportion of P. falciparum, P. ovale, P. malariae, and P. vivax species either mono- or mixed infections. These proportions were computed as ratio of total number of patients with either one Plasmodium species either mono- or mixed infections to total number of Plasmodium-infected patients. Findings were stratified by timing of screening (ANC and DU). Pf mono-infections, Pv mono-infections, and Pf/Pv mixed infections are depicted in green, red, and yellow, respectively. **Diagnostic method used for detection Plasmodium infections.
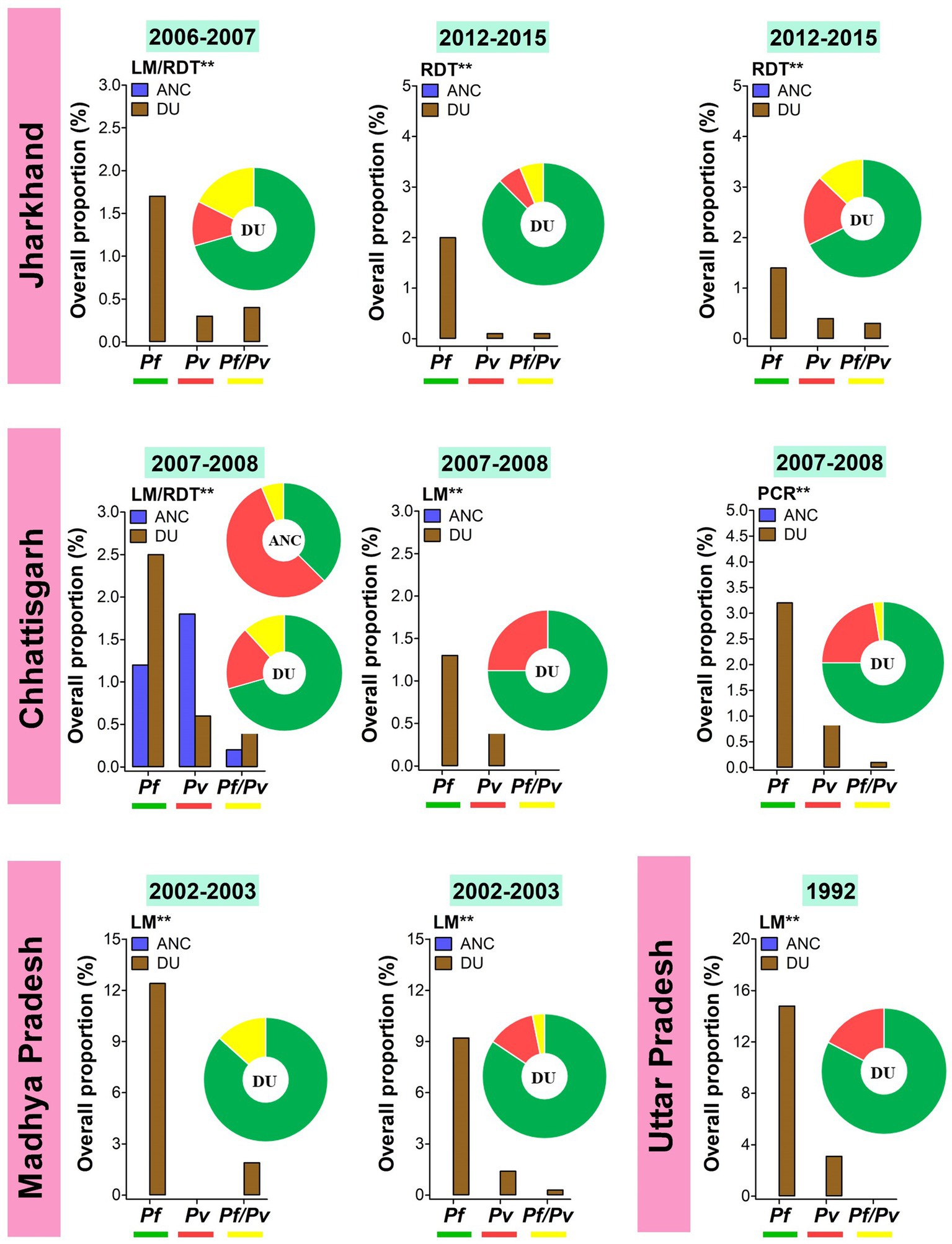
Figure 4. Overall proportion and contribution of Plasmodium species either as placental mono- or mixed infections in ANC and DU by states. ANC, Antenatal care visit; DU, Delivery unit; LM, Light microscopy; RDT, Rapid diagnostic test; PCR, Polymerase chain reaction; Pf, Plasmodium falciparum; Pv, Plasmodium vivax; Pf/Pv, Mixed infection with P. falciparum and P. vivax. Bars represent overall proportions of Plasmodium infections which are computed as ratio of number of patients with Plasmodium species either mono-infection or mixed infections to total number of patients. Pie charts represent the specific proportion of P. falciparum, P. ovale, P. malariae, and P. vivax species either mono- or mixed infections. These proportions were computed as ratio of total number of patients with either one Plasmodium species either mono- or mixed infections to total number of Plasmodium-infected patients. Findings were stratified by timing of screening (ANC and DU). Pf mono-infections, Pv mono-infections, and Pf/Pv mixed infections are depicted in green, red, and yellow, respectively. **Diagnostic method used for detecting Plasmodium infections in the studies.
Temporal analysis of burden of MiP was limited in regions such as Madhya Pradesh, Jharkhand, and Chhattisgarh (Figures 3, 4). In Madhya Pradesh, the prevalence of peripheral Pf infection at ANC decreased from ~13% in 1991 to ~7% in 1995–1996, while prevalence of peripheral Pv infection at ANC levels off at ~5% between 1991 and 1997–1998. In Jharkhand, the prevalence of Pv infection at ANC increased from ~1% in 2006–2007 to ~5% in 2015. The same trend was observed for peripheral Pf infection among women attending ANCs in Chhattisgarh where the prevalence of this species increased from ~0.5% in 1984–1985 to ~1.5% in 2007–2008 (Figure 3). In contrast, an increase in prevalence of peripheral Pv infection at DU was noted in Jharkhand (~0.5% in 2006–2007 to ~4% in 2015). Regarding placental infection, the burden of Pf infection slightly decreased from ~1.5% in 2006–2007 to ~1% in 2012–2015 in women living in Jharkhand (Figure 4). There are few studies that analyzed temporal trends of MiP burden in other settings such as sSA and Latin Americas where malaria endemicity patterns are different. One study reported an apparent reduction in Pf MiP burden from ~60 to 5% during years 1994–2019 in different malaria eco-epidemiological regions (coastal savannah zone, middle forest zone, and northern savannah zone) in Ghana (59).
3.4. Clinical and parasitological features of MiP
Clinical presentation of MiP in India is diverse with Plasmodium infections ranging from asymptomatic carriage of the parasites to severe clinical forms.
3.4.1. Asymptomatic malaria
The detection of Plasmodium parasitemia of any density, in the absence of fever or other acute symptoms, in individuals who have not received recent antimalarial treatment is known as asymptomatic malaria (60). All plasmodial species can elicit asymptomatic infections during pregnancy with higher parasitemia in Pf infections compared to non-Pf infections (50). Data on asymptomatic MiP are greatly lacking from India. The burden of asymptomatic malaria varies across Indian areas, and this heterogeneity is related to several factors such as using diagnostic techniques with varying sensitivity and specificity. Using RDT, Corrêa and colleagues reported that ~10% of Plasmodium spp-infected women were asymptomatic in tribal areas of Chhattisgarh, Telangana, and Andhra Pradesh (49). In Jharkhand state, it was reported that >70% of MiP cases were asymptomatic in a randomized trial (53). Another study in the same state reported same results both, at ANC (70.6%) and DU (75.7%) (46). Similarly, a community based study found that asymptomatic cases accounted for 76.7% of all malaria cases in rural areas in Chhattisgarh (54). There is a link between asymptomatic infections and parasite density, with many asymptomatic infections being found at submicroscopic levels and thus can only be detected using molecular tools (61). A recent meta-analysis reported overall prevalence of submicroscopic Plasmodium infections of 0.4–38.4% in general Indian population (62), which is lower estimates as seen in MiP. In Chhattisgarh, Singh and colleagues found significant proportion of both peripheral and placental Plasmodium spp. submiscroscopic infections, for which the extent was species-dependent, among majorly primigravidae/secundigravidae women (47). More than 60% of peripheral and placental infections were submicroscopic with higher rates seen in Pv compared to Pf infections (placental: 66.7 vs. 64.7%, peripheral: 90.5 vs. 50%) (47). Tracking asymptomatic and submicroscopic Plasmodium infections is vital for malaria elimination strategies in endemic areas (63), especially in India where malaria burden significantly decreased in last years.
3.4.2. Uncomplicated malaria
The fraction of asymptomatic infections that will become clinically symptomatic is shaped by a cocktail of factors such as level of transmission, control measure coverage, comorbidities (e.g., human immunodeficiency virus—HIV, malnutrition), host and parasite factors (64, 65). Uncomplicated malaria (UM) encompasses non-pathognomonic and flu-like signs and symptoms which often comprise fever, nausea, rigors, chills, headache, muscle pains, etc. (66). Symptoms usually occur 7–30 days after mosquito bite and last for 6–10 h sequentially three stages: cold, hot, and sweating (67). The same clinical malaria symptomatology is seen in pregnant women living in endemic areas such as India. Most of the Pf and Pv UM-related symptoms reported in Indian pregnant women attending ANC and/or DU included fever, weakness/fatigue, body/joint pain, headache, loss of appetite, diarrhea, dizziness, hepato-splenomegaly, and nausea/vomiting (33, 43, 44, 46, 49, 50, 52, 54).
Malaria is also associated with cell and biochemical changes in multiple organs and tissues. One of the most dominant MiP-associated signs is anemia for which risk is increased in pregnant women and malaria-infected pregnant women (46, 55). The pathophysiological mechanism includes hemolysis of both Plasmodium-infected and uninfected red blood cells (RBCs) and impaired/suppressed hematopoiesis (67, 68). The sequestration pathophysiological phenomenon is known and described well in Pf parasites (69), but not for Pv and Pk though few reports showed ability of Pv- and Pk-infected RBCs to cytoadhere to endothelial cells in placenta, bloodstream vessels and brain even though low cytoadherence of Pk-infected RBCs to cerebral microvascular endothelial cells was found (70–72). In India, malarial anemia during pregnancy is due to Pf and Pv with overall prevalence of 36.6–100% varying across the country (39–43, 46, 47, 50, 58, 73), with mild and moderate forms accounting for >85% of all anemia cases (28, 41, 46, 49). The extent of MiP-associated anemia seems to be higher in primigravidae/secundigravidae and ANC patients. On ANC visits anemia prevalence of ~67–88.3% against ~59.6–83.9% at DU through studies conducted in Jharkhand, Chhattisgarh, and Rajasthan (39, 46, 47, 50).
3.4.3. Severe malaria
Pregnant women are particularly susceptible to Plasmodium infections and its severe forms in malaria endemic regions of India (31, 57, 74). Available data outline that Pf is the principal contributor to severe MiP cases and maternal/fetal outcomes, and few reports about Pv causing SM attacks (75, 76). No severe MiP case due to non-Pf/Pv species has been documented in the world so far. Using systematic review and meta-analysis approach, we recently showed that the overall prevalence of SM in individuals with Pv mono-infection was 29.3% in India, with lowest and highest rates in Karnataka (15.3%) and Uttarakhand (57.8%), respectively (5). In pregnant women, data on SM prevalence are greatly missing in India, and the studies on clinical patterns of severe MiP are focused and/or have evaluated few particular presentations only (e.g., ARDS, CM) (77, 78). One study from Karnataka reported that 32.4% of malaria-infected patients were diagnosed with SM, and Pv was the main contributor of SM cases (56.6%) (52). Most of severe Pf MiP cases occurred in primigravidae as reported by Singh et al. in Madhya Pradesh (29), and Kochar et al. in Rajasthan (79). No severe MiP case with Po, Pm, and Pk have been documented in India till now.
3.4.3.1. Severe malarial anemia
Malaria infection is a risk factor for severe anemia in Indian pregnant women (47, 50). Based on the available data, severe anemia is found at prevalence of 3–15.6% in Plasmodium-infected pregnant women (28, 39, 41, 46, 47, 49, 50, 52, 74). Pf and Pv as mono- and mixed infections are the species responsible for this severe hematological condition, with higher rates seen in Pf-MiP as reported in Karnataka state (52). However, these estimates do not reflect the real burden of MiP related SMA in Indian context for at least four reasons: (i) different thresholds for hemoglobin level were used for diagnosing severe anemia (e.g., Hb < 5 g/dL or < 7 g/dL), (ii) in some studies, moderate and severe anemia were collectively diagnosed with the same Hb threshold (e.g., Hb < 9 g/dL) (41), (iii) very few studies appraised other severe anemia-inducing conditions such as malnutrition (49), and (iv) none of the studies included parasitemia threshold for defining SMA as per WHO guidelines (Tables 2, 3). In this context, it is needed to document the real contribution of SMA in pregnancy in India.
3.4.3.2. Cerebral malaria
Cerebral malaria is a common severe manifestation in Indian pregnant women (38, 77, 84, 87, 88), as high CM prevalence of 60 and 76% reported among malaria-infected individuals from Orissa and Rajasthan states, respectively (79, 82). Routinely, CM is more frequently seen in primigravidae/secundigravidae compared to multigravidae, and is mostly associated with poor maternal and fetal outcomes (28, 31, 77, 88).
3.4.3.3. Hypoglycemia
Hypoglycemia is often reported at low rates in Indian children and non-pregnant adults diagnosed with SM. A systematic review and meta-analysis found a pooled proportion of hypoglycemia of 0.05% due to Pv-related mono-infections in the country (5). In SM pregnant women, prevalence of hypoglycemia is ~3–35% with higher rates found in Pf infected pregnant women compared to their Pv infected counterparts (Table 3). In Rajasthan, Pv induced hypoglycemia in 4% of malaria patients while a prevalence of 35% was reported among Pf-infected women in Uttar Pradesh (Table 3) (48, 74).
3.4.3.4. Acute renal failure
The etiology of acute renal failure (ARF) is multifactorial in pregnancy, and studies outline that malaria contributes toward a small fraction as reported from Gujarat state where ARF proportion is ~0–8% in pregnant women (83). A study from Rajasthan reported a Pf-induced ARF proportion of 20%, but this estimate was obtained on only 45 pregnant women (Table 3) (80).
3.4.3.5. Acute respiratory distress syndrome/pulmonary edema
Acute respiratory distress syndrome is diagnosed in malaria infected pregnant women at lower rates compared to SMA, CM, and ARF, with overall proportion of ~0–4% in states including Rajasthan, Gujarat, and Andhra Pradesh (Table 3). ARDS can pose a veritable diagnostic and therapeutic dilemma as previously reported in a Pv-infected preeclamptic pregnant woman (78). Kochar et al. (81) reported that pulmonary edema occurred in 13.3% of malaria infected pregnant women just after delivery in Rajasthan.
3.4.3.6. Clinical jaundice
Jaundice is the main clinical manifestation seen in Pv SM in Indian patients (5). This condition occurs in malaria infections due to intravascular hemolysis, disseminated intravascular coagulation, and rarely the occurrence of hepatocellular jaundice in Pf malaria infection also known as “malarial hepatitis” (89). Compared to severe Pf infections, the risk of jaundice is lower in severe Pv infections regardless of the living area and age group (5). In Plasmodium spp. infected pregnant women, the proportion of clinical jaundice ranges from ~4–14% with similar rates for Pf and Pv (Table 3).
3.4.3.7. Other severe malaria clinical manifestations
Severe clinical manifestations such as shock, prostration, metabolic acidosis, and abnormal bleeding can also occur in Plasmodium infected pregnant women, but at lower rates (≤ 5%) compared to the above mentioned clinical manifestations (Table 3).
3.4.4. Other severe clinical manifestations: severe thrombocytopenia
Severe thrombocytopenia defined as level of blood platelets below 50,000/μL, is not considered as clinical marker of SM in latest WHO guidelines (10). Previous works in India reported significant fraction of SM patients presenting this hematological disorder (5, 90, 91). Pf and Pv are able to induce severe thrombocytopenia, and available data in India on comparative analysis of these two species outline that the risk of this condition varies with area. In Karnataka, the risk of severe thrombocytopenia is nearly four times higher in Pf-infection compared to Pv-infections. In contrast, this risk is reduced by ~60% in Pf-infections in Rajasthan state (5). In pregnant women, few studies reported disparate findings for severe thrombocytopenia prevalence in Karnataka (6 and 26.8%) for Plasmodium spp. infections while 56 and 82% were found in Rajasthan and Gujarat for Pv mono-infections, respectively (45, 74, 84, 86).
3.5. Comorbidities and concurrent infections seen in MiP
Clinical course and outcomes of MiP are modeled by complex interaction between host, parasite, and environment (48). In addition, external factors such as comorbidities and concurrent infections can modulate interaction between host and Plasmodium parasites, and thus impact the natural history of malarial infection in pregnant women (Figure 5).
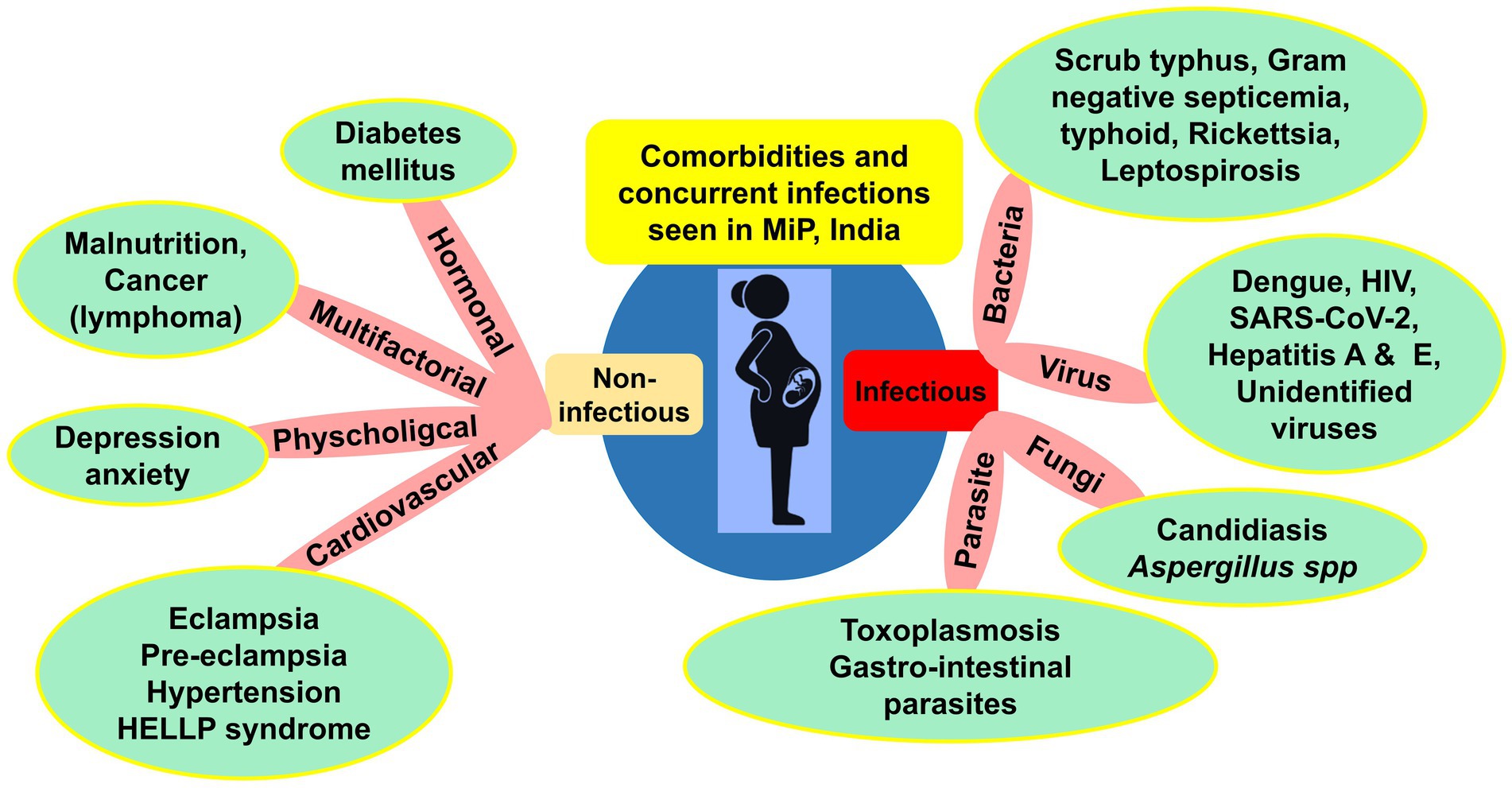
Figure 5. Comorbidities and concurrent infections reported in MiP in India. HELLP, Hemolysis, elevated liver enzymes, low platelet count; HIV, Human immunodeficiency virus infection; MiP, Malaria in pregnancy; SARS-CoV-2, Severe acute respiratory syndrome Coronavirus 2; UTI, Urinary tract infections. Some examples of comorbidities are presented in the green round shapes based on published literature and unpublished reports from health facilities. *Gestational hypertension is included in hypertension cases.
Concurrent infections included diseases caused by bacteria, viruses, parasites, and fungi. In late 80s, Mehta and Mehta reported toxoplasmosis in Plasmodium infected pregnant women from Kolkata, West Bengal (92). In the same period in Chandigarh, septicemia of bacterial origin was reported in one woman diagnosed with CM (57). High diversity of viruses including dengue virus, HIV, and SARS-CoV-2 were concurrently found in malaria infected pregnant women in the country (Figure 5). In Uttar Pradesh, a case report of co-infection with Pf, Pv, and dengue virus was seen in one 6-month pregnancy (93). In Maharashtra, a co-infection case of Pv with SARS-CoV-2 responsible for the current COVID-19 pandemics was described (94).
Regarding comorbidities, mostly cardiovascular disorders such as eclampsia and pre-eclampsia were diagnosed in Pv–induced ARDS and CM cases in Delhi and Karnataka were seen (78, 95). The effect of poor nutritional status on malaria risk is still elusive (96). A study from three states (Chhattisgarh, Andhra Pradesh, and Telangana) found malaria infected pregnant women with poor nutritional status (i.e., mid-upper arm circumference < 230 mm) (49). Other comorbidities such as cancer, diabetes and HELLP syndrome have also been reported (Figure 5).
3.6. Factors associated with MiP in India
3.6.1. Parity and gestation trimester
Parity is one of the most important factors associated with both peripheral and placental MiP, with primigravidae much at risk of malaria infection and its deleterious consequences (97). Moreover, studies found that the level of antibodies inhibiting placental sequestration of Pf parasites is increasing over successive pregnancy, thereby supporting a parity-dependent acquired antimalarial immunity (98, 99). In India, many studies reported both higher peripheral and placental malaria prevalence in primigravidae/secundigravidae compared to multigravidae (27, 29, 31, 32, 39, 46, 73, 79). In Jharkhand, a study reported that peripheral infection risk was 4.23 times higher in primigravidae/secundigravidae compared to multigravidae (46). The same team reported that the risk of placental infection was increased by >3 times in primigravidae/secundigravidae, finding that was previously reported in a study conducted in the same state (Figures 6, 7) (39, 46). It has been reported that Plasmodium spp. infection rates are higher during second trimester of pregnancy (65). Data from several states including Madhya Pradesh, Rajasthan, Gujarat also support this observation (27, 31, 33, 80). However, one study conducted in Madhya Pradesh reported highest infection rates during third trimester (30).
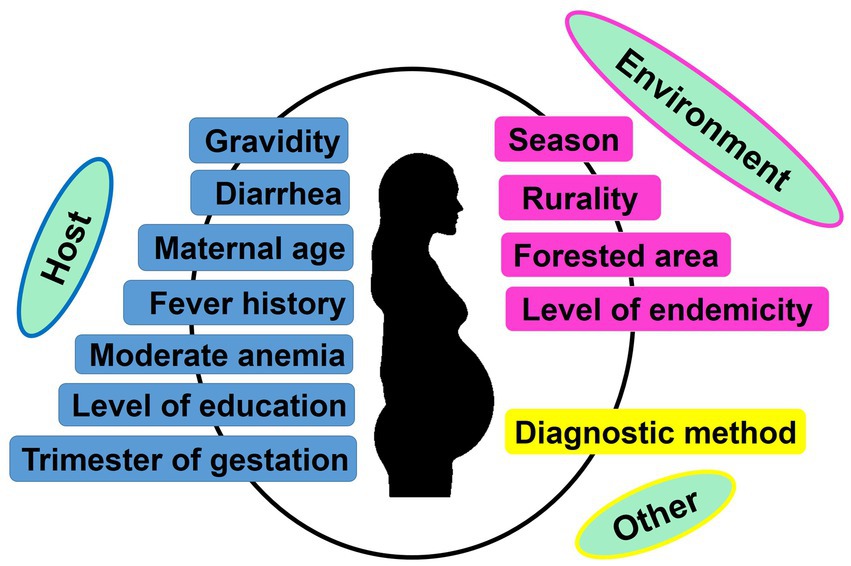
Figure 6. Factors associated with peripheral and/or placental Plasmodium spp. infection during pregnancy. Only factors identified based on multivariate logistic regression as reported in each included study were retained to create the image.
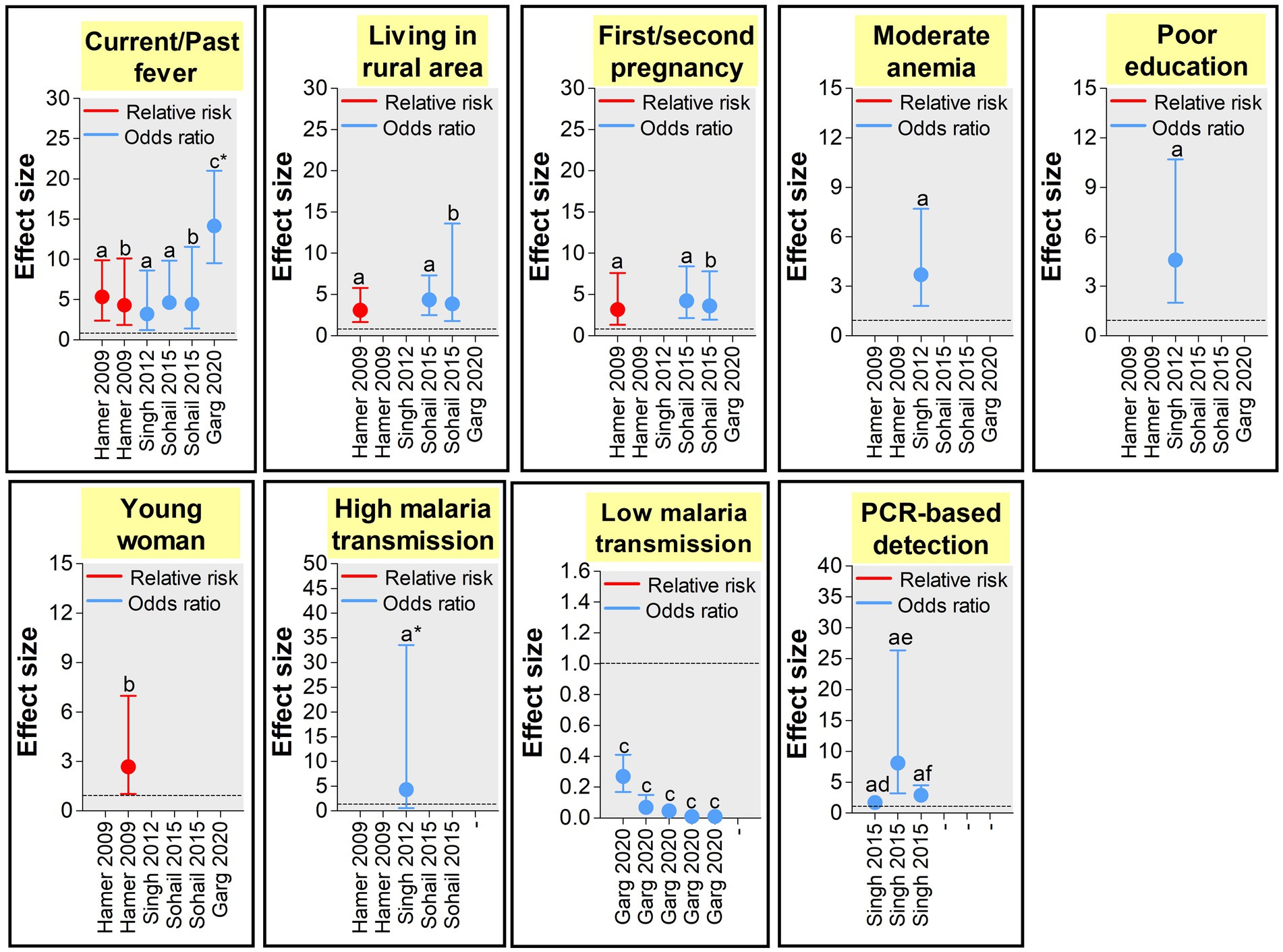
Figure 7. Size effects of peripheral Plasmodium spp. MiP determinants in India (39, 41, 46, 47, 54). Effect size was appraised as odds ratio (OR) and relative risk (RR) as reported in each study used to build the graphs. Only study that reported statistically significant effects sizes were retained. Dashed line represents a OR or RR = 1 (neutral factors). Factors with OR/RR < 1 and > 1 represent protective and risk factors of malaria infection, respectively. aEstimated from pregnant women attending antenatal care visits. bEstimated from pregnant women attending delivery units. cEstimated from pregnant women in community. dEstimated for Plasmodium falciparum infections. eEstimated for Plasmodium vivax infections. fEstimated for Plasmodium spp. infections. *The estimates presented in the graph are 1/10 of real estimates found in the study.
3.6.2. Woman’s demographical, clinical and genetic characteristics
The influence of pregnant woman’s characteristics on malaria infection risk has been reported from studies conducted in India, with role of age, level of education, and clinical symptomatology. In Jharkhand, Hamer et al. (39) found that women aged <20 years attending ANC visits had 2.68 times more risk of peripheral Plasmodium infection compared to their older counterparts (Figures 6, 7). Similarly, an increased risk for peripheral Plasmodium infection was seen in women with lack of formal education in two studies conducted in Chhattisgarh and Jharkhand, respectively (39, 41). Studies reported higher Plasmodium infection in febrile pregnant women with signification association between diarrhea, fever or history of fever, moderate anemia and peripheral/placental infection (41, 46, 54). Fever was a stronger determinant of peripheral and placental Plasmodium infections both at ANC and DU (41, 46, 54).
3.6.3. Residence area
Several aspects of the residence area have been associated with MiP in India and include level of urbanization, forest cover and level of malaria endemicity (39, 41, 46, 54). In Jharkhand, MiP is more prevalent in rural areas compared to urban areas. Indeed, the risk of peripheral Plasmodium infection is ~4–6 times higher in rural women compared to their counterparts from urban areas. Again, the risk of placental Plasmodium infection is increased by ~3–4 times in rural areas compared to urban areas in Jharkhand (Figures 6, 7) (39, 46). Working in two areas of Chhattisgarh differing by malaria endemicity, Singh and colleagues reported peripheral Plasmodium infection risk increased by ~45 times in pregnant women living in Bastar (high endemicity area) compared to those living in Rajnandgaon (low endemicity area) (41).
3.6.4. Diagnostic methods
Given detection sensitivity of malarial diagnosis tools varies, it is expected to have higher chances of detecting low (very low) Plasmodium parasitemia with molecular tools which have higher sensitivity than LM and RDTs (63). Using PCR and LM for detecting peripheral/placental Pf and Pv infections, Singh et al. pointed out that chances of detecting peripheral/placental infections using PCR were increased by ~2.5–2.9 times for Plasmodium spp. infections, ~1.7–2.6 times for Pf infections and ~ 2.5–8.1 times for Pv infections among women attending ANC and DU in Madhya Pradesh (Figures 6, 7) (47).
3.7. Maternal outcomes of MiP
Malaria in pregnancy has a devastating impact on health of mothers and their babies, and is an important cause of maternal and infant mortality in malaria endemic regions. In malaria endemic areas Plasmodium infections are associated with adverse maternal outcomes such as miscarriage, stillbirth, abortion, and mortality. In India, Pf and Pv have been associated with these maternal outcomes which are frequently seen in primigravidae mothers (Figure 8; Supplementary Table 2) (30, 31, 33, 80). CM, pulmonary edema and hypoglycemia were cause of maternal death reported in two studies conducted in Chandigarh and Gujarat (57, 73). The overall prevalence of MiP-related maternal death in malaria-infected individuals is ~0–77.3% in India, with disproportion among different states. Pf is the main contributor to maternal death while few rare death cases associated with Pv infections have also been reported (50, 100). Abortions and stillbirths are reported at prevalence of ~7.2–16.7 and ~ 0–13.3% for Pf, and 0.3–8.4 and ~ 0–8% for Pv, respectively. The highest values of Pf-related abortions and stillbirths were reported in Rajasthan among pregnant women presenting with SM (80, 81).
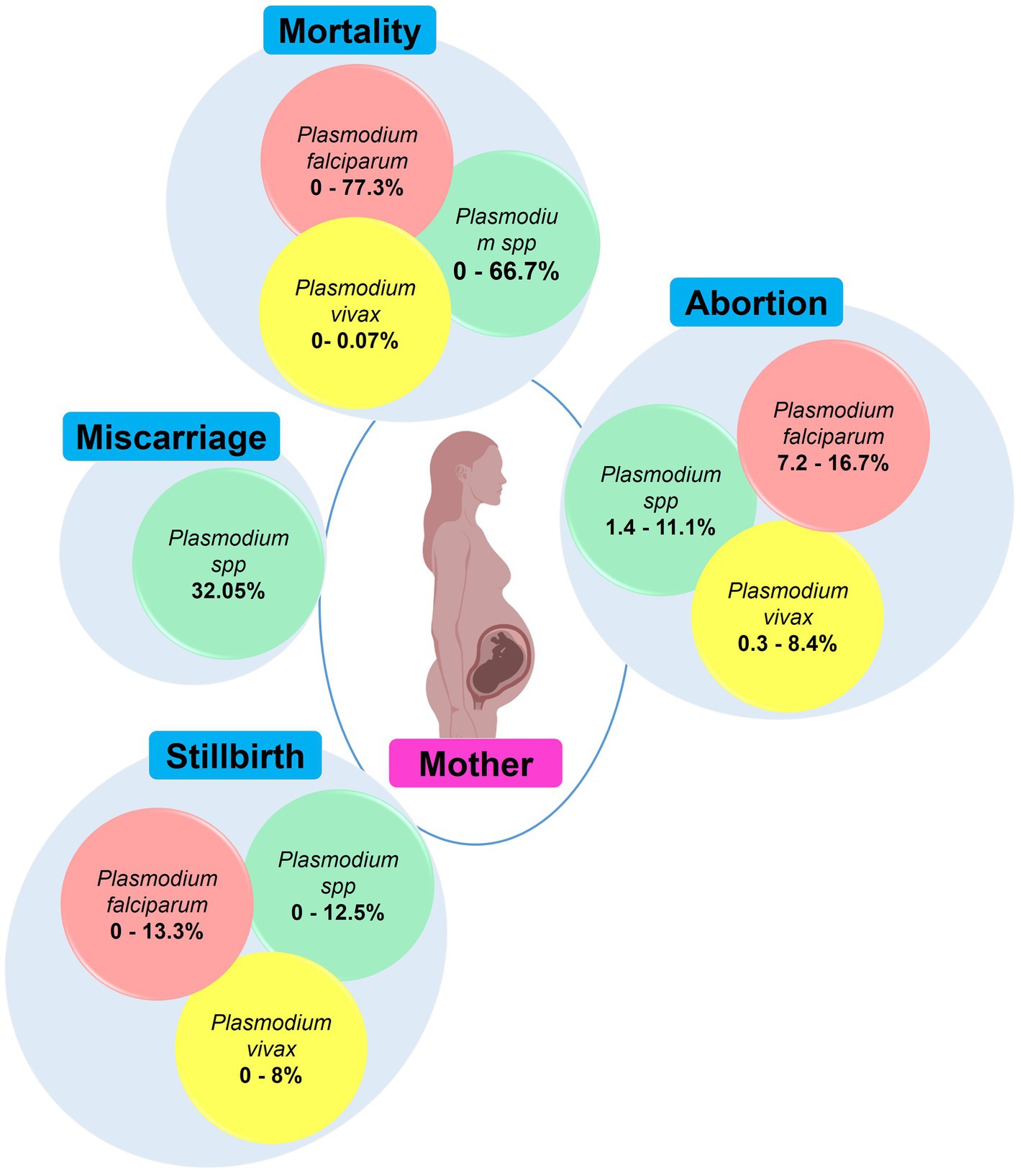
Figure 8. Main maternal outcomes during malaria in pregnancy. The values in round shapes are proportions of each maternal outcome among pregnant women infected with Plasmodium spp. (red), P. falciparum (red), and P. vivax (yellow). The values represent range (minimum and maximum values) for each maternal outcome as reported in each study included in this review. In Plasmodium spp., the malarial species was not specified during association analysis with maternal outcomes in the included studies. Created with Biorender.com.
Only two studies from Chhattisgarh and Rajasthan quantified the risk of maternal outcomes in MiP (47, 50). Using LM, Singh and colleagues found that pregnant women with peripheral Plasmodium spp. infection had 1.8 times higher risk of anemia and 13.7 times higher risk of severe anemia that their uninfected counterparts (Supplementary Table 3). Also, the risk of LBW was nearly six times higher in women with placental Plasmodium spp. infections compared to that with no infection (47). In Rajasthan, clinical Pv infection was associated with five-higher risk of maternal anemia (50). Likewise, the risk of maternal anemia was increased by four times in women with microscopic Pf infection compared to uninfected women. In the same vein, women with placental microscopic Pf infections were 4.28 times more at risk to give birth babies with LBW compared to those with no infection (50) (Supplementary Table 3).
3.8. Fetal/neonatal/infancy outcomes of MiP in India
Placental malaria infections are associated with adverse outcomes on fetus, newborns, and even during infancy. Malaria parasites, especially Pf, have a high tropism for placenta tissue in which the parasites develop and collaterally induce important histological changes (e.g., fibrinoid necrosis, calcification) (44). Such Plasmodium-induced placental changes impair fetal-maternal exchange and lead to disastrous consequences in babies such as low birth weight (LBW), prematurity, intrauterine growth retardation (IUGR), respiratory distress, and deaths in the worst case (Figure 9; Supplementary Table 3). LBW, IUGR and prematurity are the predominant adverse outcomes in India, with Pf and Pv as main contributors.
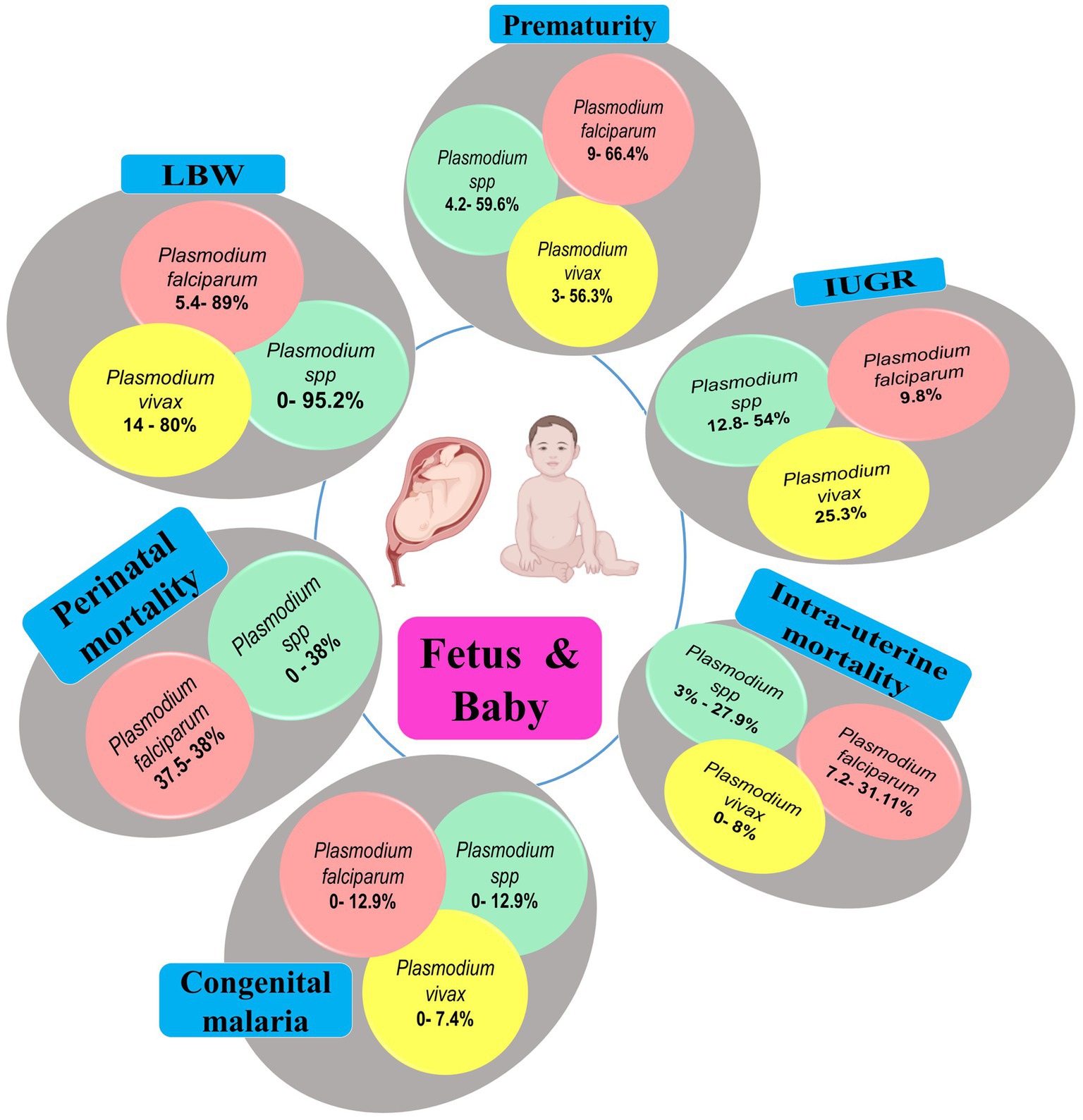
Figure 9. Main fetal/neonatal outcomes during MiP, India. IUGR, Intra-uterine growth retardation; LBW, Low birthweight; MiP, Malaria in pregnancy. The values in round shapes are proportions of each fetal/neonatal outcome among babies infected with Plasmodium spp. (red), P. falciparum (red), and P. vivax (yellow). The values represent range (minimum and maximum values) for each fetal/neonatal outcome as reported in each study included in this review. In Plasmodium spp., the malarial species was not specified during association analysis with fetal/neonatal outcomes in the included studies. Created with Biorender.com.
Studies outlines that ~5.4–89 and ~ 14–80% of Pf- and Pv-infected women gave birth babies with LBW, respectively. In Madhya Pradesh, it was reported that almost all babies (95.2%), born to malaria infected women, had LBW (33, 36). Similarly, the prevalence of prematurity can often surpass 50% for both Pf and Pv in Indian women. It was reported that severe maternal anemia was risk factor for both LBW and prematurity in Jharkhand (101). Growth restricted babies are also frequently seen during MiP with prevalence of ~12.8–54, ~0–9.8, and ~ 0–25.3% for Plasmodium spp., Pf, and Pv, respectively (Figure 9; Supplementary Table 4). It is now recognized that Pv can also cause poor birth outcomes including perinatal and intrauterine mortality, but at lower extent than its Pf counterpart. In Indian context, the prevalence of Pv-induced intrauterine death during MiP ranges from 0 to 8% while that of Pf is estimated at ~7.2–31.1% (Figure 9; Supplementary Table 4).
Malaria in pregnancy and its birth consequences are consistently correlated with increased malaria risk during infancy (102, 103). A study found an increased 2-fold risk for malaria infection and clinical malaria in small-for-gestational age babies born to Beninese women (104). Such data are scarce in India, but in one study conducted in Madhya Pradesh, mothers and their infants were followed up for 1 year. The authors reported increase in malaria prevalence, intensity and frequency during the follow up, and three of all Pf-infected infants died before their first birthday (33).
3.9. Neonatal and congenital malaria
In clinical practice, congenital malaria is defined as presence of Plasmodium asexual stages in cord blood and peripheral blood of the baby during first week of life (105). In neonatal malaria, Plasmodium asexual stages are found in neonates aged ≤28 days (105). A recent meta-analysis estimated global NCM prevalence at 40.4 and 12%, due to several variable factors including area and detection methods (106).
The prevalence of congenital malaria in India ranges from 0 to 12.9% (Figure 9). Prevalence data on neonatal malaria are absent in India, but some case reports outlined its occurrence in the country (Supplementary Table 5). NCM cases have been reported throughout India especially in states such as West Bengal, Madhya Pradesh, Rajasthan and Uttar Pradesh (Supplementary Tables 3–5). In India, NCM cases are mostly born to primigravidae women, have LBW and are aged 26 days on average with male predominance. On admission, babies present at hospital with mosaic of signs/symptoms mostly including pallor, hepatosplenomegaly, fever, jaundice/icterus, and irritability. Anemia, thrombocytopenia, leucopenia and clinical jaundice are frequently seen in newborns infected with malaria parasites having parasitemia in ranges from 0.1 to 25% and Pv mono-infections account for ~60% of all LM/RDT-detected NCM cases (Figure 10; Supplementary Table 5).
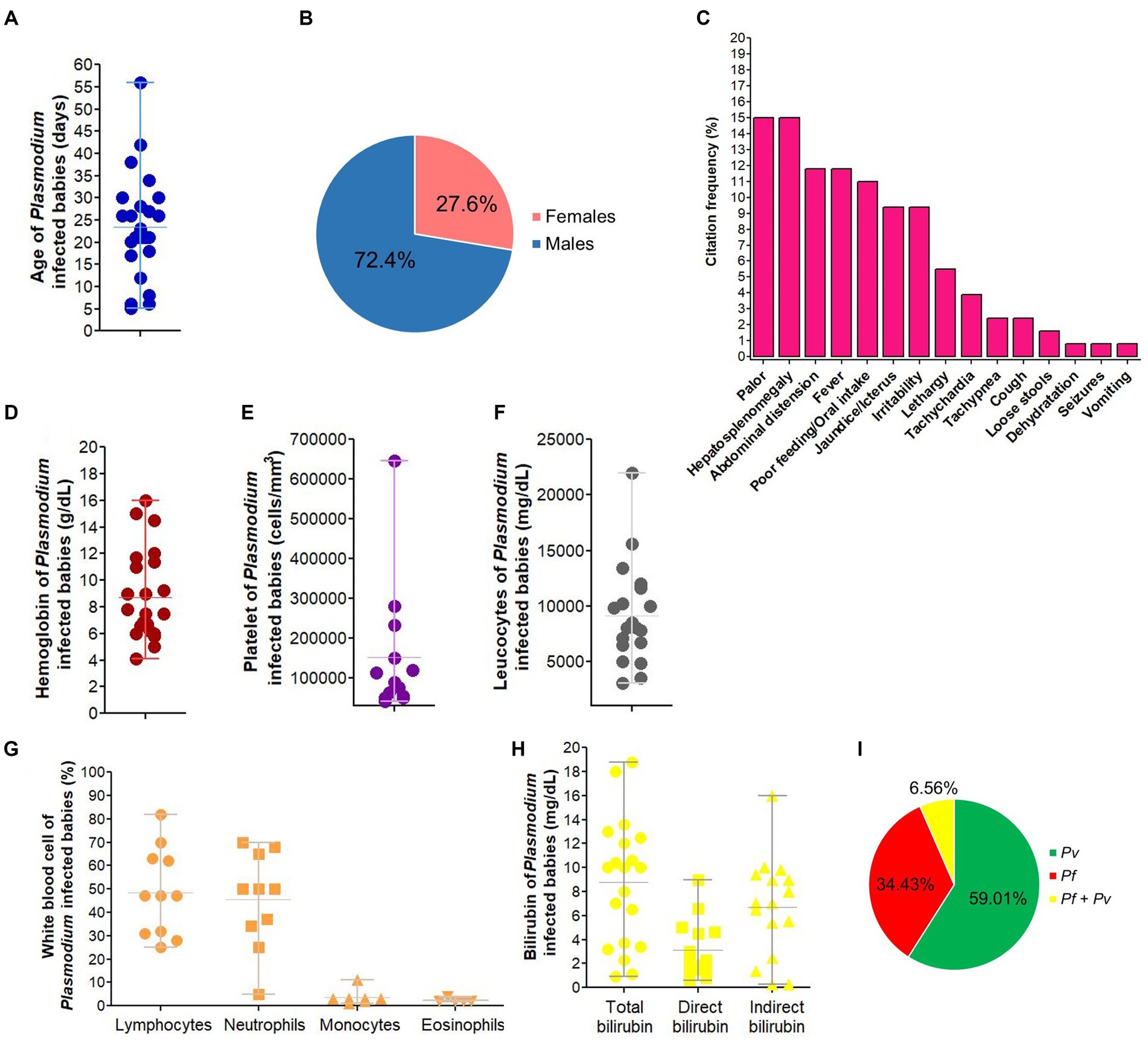
Figure 10. Characteristics of neonatal malaria cases in India. Pf, Plasmodium falciparum, Pv, Plasmodium vivax. The image depicts characteristics of Plasmodium infected babies for age (A), gender-distribution (B), signs/symptoms presented on admission/birth (C), hemoglobin level (D), platelet count (E), total leucocytes (F), white blood cell populations (G), total, direct and indirect bilirubin level (H), and malaria infection (I). We excluded data from congenital malaria due to very low number of cases (n = 3). In panel (C), percentage of each symptom on admission were computed by dividing the number of times that symptom was cited to the total number of citations for all symptoms. In panel (I), malaria species were identified using LM and/or RDT.
3.10. Prevention of MiP
In last 2 decades, India greatly scaled up large number of malaria prevention methods throughout the territory to achieve elimination objectives by 2030 (107, 108). Malaria prevention in India relies essentially on free provision of ITNs/LLINs and IRS (109). LLINs are recommended in high malaria risk areas even though no formal system exist for their distribution during ANC visits (110). Complementary control strategies such as chemoprophylaxis, sensitization campaigns for behavior changes, reduction of breeding sites for mosquito vectors and early case detection and prompt treatment are also encouraged by the Government of India. Ownership and use rates of LLINs vary greatly within and between states, and even when LLINs are present in household they are not adequately used by Indian population (111, 112). A large scale household based study reported LLINs use rates of 89 and 91% among pregnant women in the Odisha state (113). Similar rate (88.6%) was reported in pregnant women in another study conducted in the same state (114). In contrast, available data from health facility-based studies suggest that ITNs/LLINs use rates are lower than those seen in community studies. The proportion of pregnant women using ITNs/LLINs most of nights is ~18.3–82.8% and that of women sleeping under ITNs/LLINs the last night is ~0.05–82.5% (39, 41, 44, 47, 50, 52, 115). Also, the utilization of untreated ITNs/LLINs by pregnant women was common in few areas (39, 46). IRS is generally less used in households by pregnant women compared to ITNs/LLINs, with use rates of ~0–58.5% (39, 41, 44, 47, 50). Taking malaria chemoprophylaxis is very uncommon in pregnant women as per the studies conducted in forested and tribal areas of Jharkhand, Chhattisgarh, Rajasthan, and Madhya Pradesh (39, 41, 44, 46, 47, 50).
3.11. Therapeutic approaches for MiP control and drug resistance in India
Prevention of MiP with IPTp-SP is not implemented in India, and control of the disease in pregnancy relies on passive case detection during ANC visits. Until 2010, the Indian national guidelines recommended the utilization of quinine for Pf malaria and CQ for Pv malaria regardless the trimester of gestation (116). Since then, this MiP treatment policy changed with regard to Indian state, malaria species, severity of the infection and trimester of gestation. For uncomplicated Pv infections, CQ is administered in all trimesters. The utilization of primaquine (PQ) for radical cure of Pv infection is not recommended to pregnant women and her fetus. The treatment of uncomplicated Pf MiP is CQ for first trimester women while ACTs artemether + lumefantrine (AL) or artesunate + sulfadoxine/pyrimethamine (AS + SP) are used for second and third trimesters. Due to high level of SP resistance in Northeastern states, AL is recommended for treatment while AS + SP is used in other states. Parenteral quinine, artesunate, or artemether are given for SM (6, 116, 117).
Drug efficacy studies are limited in MiP in the country, but existing data outline both high efficacy and safety of AS + SP and AS + MEF (118). CQ and SP resistance are both well established, and studies reported low frequency Pfk13 mutations associated with artemisinin resistance (i.e., 446I, 539 T, and 561H) in Arunachal Pradesh and West Bengal (119–121). Several mutations in the Pfk13 gene—i.e., F446I, N458Y, C469Y, M476I, Y493H, R539T, I543T, P553L, R561H, P574L, C580Y, R622I, and A675V—are strongly associated with resistance of Pf parasites to ACTs, the current antimalarial drugs recommended for treatment of uncomplicated Pf malaria (1, 119). There is a dearth of data on antimalarial drug resistance status in MiP. Using in vitro and in vivo studies, some authors found that 100 and 31.4% of Pf isolates collected from Madhya Pradesh and Uttar Pradesh states were resistant to CQ (34, 48). Although not purposely designed for appraising drug resistance, other studies reported adequate clinical and parasitological response of ~19.7–79% in Pf-infected pregnant women treated with CQ at health facilities (28, 30, 58).
3.12. Challenges and future directions on MiP in India
In this quest for achieving malaria elimination objectives, MiP should also be taken into account and a certain number of challenges ranging from prevention to treatment should be investigated in future by Indian researchers. Missing links on MiP research and proposed solutions are presented below and summarized in Figure 11.
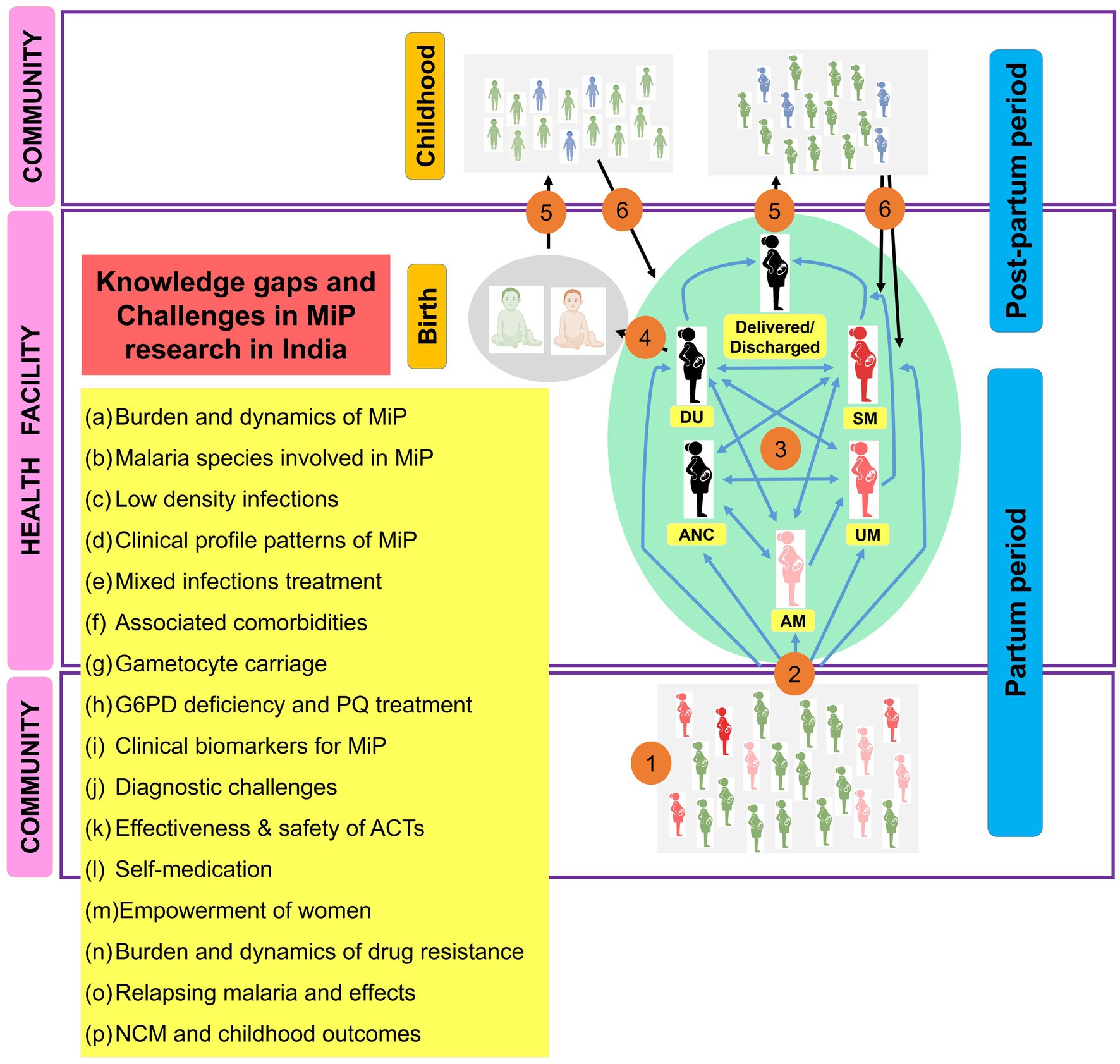
Figure 11. Main research gaps and challenges to be addressed on MiP in India. ACTs, Artemisinin based combination therapies; AM, Asymptomatic malaria; ANC, Antenatal care; DU, Delivery unit; G6PD, Glucos-6-phosphate dehydrogenase; MiP, Malaria in pregnancy; NCM, Neonatal and congenital malaria; PQ, Primaquine; UM, Uncomplicated malaria; SM, Severe malaria. The image presents the main knowledge gaps and challenges on MiP research in India. (1) At community, pregnant women are exposed to infecting Anopheles bites and some of them will be infected (red). The plasmodial infection may exist as asymptomatic and/or symptomatic. (2) Both asymptomatic and symptomatic (UM and SM), women can attend health facilities for diverse reasons (e.g., ANC, DU, and care seeking). (3) Women attending ANC/DU can present symptoms or not, and will be managed as per national health guidelines if malaria infection is diagnosed. (4) Women came for delivery can give birth to uninfected babies (gray) or babies diagnosed with congenital malaria (red), and these will be treated as per national guidelines. (5) Women and babies discharged after successful antimalarial treatment will go back home. (6) Women and babies are treated with CQ if Pv infection was diagnosed, but using PQ is prohibited for them. Thus, some of them (blue) can develop recurrent malaria episodes during post-partum period and childhood due to reactivation of hypnozoites. During this itinerary for women and babies, several challenges and knowledge gaps (yellow box) have been identified and should be taken into account in future researches in India.
Indian researches on epidemiology of MiP have carried out in few states such as Madhya Pradesh and Chhattisgarh, and there is a dearth of cross-sectional/longitudinal studies from other areas including Northeastern states (e.g., Mizoram, Meghalaya, and Tripura), Bihar and Uttarakhand where malaria endemicity is still high. Also, more studies on MiP and NCM in tribal and forested settings are also greatly needed (122, 123).
Minor species including Po and Pm are also associated with MiP in India (22). LM is the standard and reports pinpointed that Po and Pm infections are misdiagnosed as Pv and Pf infections using LM in the country (124, 125). It is worth determining the extent and characterizing these species in MiP as previous studies showed their ability to elicit SM and noted pathophysiological differences between P. ovale curtisi and P. ovale wallikeri (4, 126–129).
In general, MiP studies are conducted in health facility settings either during ANC or DU periods. It would be helpful to more document MiP in community setting especially the extent of asymptomatic and/or submicroscopic parasitemia and their impact on clinical course of MiP. In addition, non-Pf low density infections (LDI) especially Pv parasitemia are problematic given efficient transmission of Pv LDI to Anopheles vectors (130, 131).
Clinical presentation of MiP in India encompasses asymptomatic, uncomplicated and severe malaria. In the latter clinical form, SMA is predominantly seen during pregnancy in India. However, other factors such as malnutrition and helminthiasis may also cause SMA, but these are rarely concomitantly addressed in MiP studies. Macronutrient undernutrition is a big contributor to severe adverse outcomes during pregnancy (132), but such studies are lacking in India.
As above discussed national guidelines for treatment of MiP are different for Pf and Pv species. However, studies report high rates of mixed infections on field that can hinder control of malaria in India (133, 134). Also, treatment of malaria in presence of comorbidities such as HIV may also be tricky and impact pregnancy outcome (e.g., drug–drug interactions). It was reported that severity and mortality are increased in patients co-infected with Plasmodium parasites and HIV (135, 136).
Pregnant women are also reservoir for gametocytes, the transmission development stage of Plasmodium parasites. Singh and coworkers reported gametocyte carriage rates of 54 and 73.8% in Pf-infected pregnant women from Madhya Pradesh (28, 30). This research area is understudied in MiP and should be investigated in future.
Plasmodium vivax parasites produce dormant stages called hypnozoites and responsible for malaria relapses, which are associated with transmittable gametocytaemia and delayed mortality (137, 138). PQ is currently recommended for preventing Pv relapses but its utilization is associated with risk of severe hemolysis in persons diagnosed with glucose-6-phosphate dehydrogenase (G6PD) deficiency (139). In India, G6PD testing is rarely performed at health facilities coupled with poor adherence to PQ-based 14-day regimen treatment and high prevalence of G6PD deficiency (e.g., Odisha) (5, 140, 141). This limits researches on evaluation of real burden of Pv relapses, effectiveness, and development of new hypnozoiticidal drugs.
Immunity against Pv is more rapidly acquired than that against Pf, that results in high proportion of Pv asymptomatic infections (142–144), which are often associated with high carriage of hypnozoites and undetected by LM and RDT (142, 144–146). Development of point-of-care tests based on biomarkers could be promising approach. We recently proposed a simple theoretical framework for identifying, evaluating and validating diagnostic, therapeutic prognostic and predictive biomarkers for malaria, and these could be translated to MiP (147). Longley et al. (148) proposed an interesting approach based on serological markers to detect recent Pv infection.
Another cause of lower sensitivity of RDTs in pregnant women could be likely deletions in the histidine rich protein 2 gene (pfhrp2) which encodes a Pf-specific protein antigen (149). No studies have evaluated pfhrp2 deletions in pregnant women in India (150, 151), and it would be interesting to appraise the prevalence profile of such deletions at ANC, DU, and community settings.
No studies on SP and artemisinin resistance in MiP in India have been carried out. Also, data on incidence of CQ resistance in MiP, which are probably may parallel CQ resistance in normal Pf infections occurring in the same region, are still needed. It is required to conduct more studies especially longitudinal studies to detect temporal variation of the drug resistance gene profile in pregnancy and its association with maternal outcomes.
Even though ACTs are recommended by National guidelines for treating MiP, there is a lack of studies on their effectiveness and safety in India (118). Systematic reviews and meta-analyses of prospective studies and clinical trials conducted in African and Asian settings have showed the efficacy and safety of ACTs during MiP (152, 153). In this context, further investigations are required to determine pharmacological aspects, effectiveness and inocuity profile of ACTs in MiP all around the country.
Self-medication both with traditional medicines and antimalarial drugs should also be addressed in pregnancy. Few studies reported pregnant woman were self-medicating, and this expose them to adverse effects of drugs, often of poor quality as reported in several endemic sSA and SEA areas including India (154, 155).
Some studies reported poor awareness of pregnant women toward malaria, its treatment and prevention due to several causes including social barriers. Thus, there is need to empower pregnant and childbearing women toward malaria and preventive methods and this could be achieved through sensitization during community campaigns and ANC visits.
4. Conclusion
Malaria in pregnancy is still a serious public health concern in India. Its epidemiological burden is high in Indian pregnant women, with Pv and Pf as main causative agents while minor species (Pm, Po) are also involved. The epidemiology of these species in MiP is greatly varied with important role of mother’s demographic and obstetrical characteristics, geography, and ecoclimatic features of the area. Plasmodium infections in pregnant women, often associated with comorbidities and concurrent infections, may progress from asymptomatic carriage of parasites to SM, which is mostly represented by SMA, CM, and hypoglycemia, and more frequently seen in Pf infections. MiP has deleterious effects on mother and her child that can often end with death. This review provided a comprehensive overview on epidemiological situation and identified important missing links in MiP and NCM to inform population, clinicians, and researchers. There is urgent need for further studies on the different above mentioned points addressed in the present review. If adequately addressed, the future findings could be greatly helpful for efficiently controlling MiP in India through development, implementation, and scale up of control strategies and policy makers, and thus achieve malaria control and elimination objectives in the country.
Author contributions
LPKF and VS conceptualized the paper. LPKF conducted literature review and extracted and analyzed data from papers, conceived the figures and maps, performed extraction and analysis data, and drafted the first version of the final manuscript. VS revised the manuscript for important intellectual content and supervised the work at all stages. All authors contributed to the article and approved the submitted version.
Funding
LPKF is supported by the Department of Biotechnology, New Delhi, India, and The World Academy of Sciences, Trieste, Italy, in the framework of a prestigious fellowship (DBT-TWAS Postgraduate Fellowship Grant N° 3240300010).
Acknowledgments
The authors are grateful to the National Vector Borne Disease Control Program for website updated data on malaria epidemiology in India. We acknowledge the support of colleagues Monika Matlani (Department of Microbiology, Vardhman Mahavir Medical College & Safdarjung Hospital, New Delhi, India) and Shyam Sundar Meena (Department of Pediatrics, Vardhman Mahavir Medical College & Safdarjung Hospital, New Delhi, India) for their inputs in generating Figure 5, and Rashid Perwez (Assistant Librarian & Information Officer, ICMR-NIMR, New Delhi, India) for sharing full-texts of some relevant papers included in this review. We thank ICMR-NIMR for infrastructure support toward providing a working environment.
Conflict of interest
The authors declare that the research was conducted in the absence of any commercial or financial relationships that could be construed as a potential conflict of interest.
Publisher’s note
All claims expressed in this article are solely those of the authors and do not necessarily represent those of their affiliated organizations, or those of the publisher, the editors and the reviewers. Any product that may be evaluated in this article, or claim that may be made by its manufacturer, is not guaranteed or endorsed by the publisher.
Supplementary material
The Supplementary material for this article can be found online at: https://www.frontiersin.org/articles/10.3389/fpubh.2023.1150466/full#supplementary-material
References
1. WHO (2022). World malaria report 2022, World Health Organization. World Health Organisation, Geneva.
4. Kojom Foko, LP, Kumar, A, Hawadak, J, and Singh, V. Plasmodium cynomolgi in humans: current knowledge and future directions of an emerging zoonotic malaria parasite. Infection. (2023) 51:623–40. doi: 10.1007/s15010-022-01952-2
5. Kojom Foko, LP, Arya, A, Sharma, A, and Singh, V. Epidemiology and clinical outcomes of severe plasmodium vivax malaria in India. J Inf Secur. (2021a) 82:231–46. doi: 10.1016/j.jinf.2021.03.028
6. NIMR and NVBDCP. Guidelines on Diagnosis and Treatment of Malaria in India, 2014. National Center for Vector Borne Disease Control Program. 3rd ed (2014).
7. Desai, M, ter Kuile, FO, Nosten, F, McGready, R, Asamoa, K, Brabin, B, et al. Epidemiology and burden of malaria in pregancy. Lancet Infect Dis. (2007) 7:93–104. doi: 10.21776/ub.jitode.2017.005.01.02
8. Ngai, M, Weckman, AM, Erice, C, McDonald, CR, Cahill, LS, Sled, JG, et al. Malaria in pregnancy and adverse birth outcomes: new mechanisms and therapeutic opportunities. Trends Parasitol. (2020) 36:127–37. doi: 10.1016/j.pt.2019.12.005
9. Rijken, MJ, McGready, R, Boel, ME, Poespoprodjo, R, Singh, N, Syafruddin, D, et al. Malaria in pregnancy in the Asia-Pacific region. Lancet Infect Dis. (2012) 12:75–88. doi: 10.1016/S1473-3099(11)70315-2
15. WHO. Severe falciparum malaria. Trans R Soc Trop Med Hyg. (2000) 94:S1–S90. doi: 10.1016/S0035-9203(02)90099-4
17. Kar, SS, and Ramalingam, A. Is 30 the magic number? Issues in sample size. Nat J Commun Med. (2013) 4:175–9.
18. Anvikar, AR, Shah, N, Dhariwal, AC, Sonal, GS, Pradhan, MM, Ghosh, SK, et al. Epidemiology of plasmodium vivax malaria in India. Am J Trop Med Hyg. (2016) 95:108–20. doi: 10.4269/ajtmh.16-0163
19. Das, A, Anvikar, AR, Cator, LJ, Dhiman, RC, Eapen, A, Mishra, N, et al. Malaria in India: the center for the study of complex malaria in India. Acta Trop. (2013) 121:267–73. doi: 10.1016/j.actatropica.2011.11.008
20. Siwal, N, Singh, US, Dash, M, Kar, S, Rani, S, Rawal, C, et al. Malaria diagnosis by PCR revealed differential distribution of mono and mixed species infections by Plasmodium falciparum and P. vivax in India. PLoS One. (2018) 13:e0193046. doi: 10.1371/journal.pone.0193046
21. Bharti, PK, Chand, SK, Singh, MP, Mishra, S, Shukla, MM, and Singh, R. Emergence of a new focus of plasmodium malariae in forest villages of district Balaghat, Central India: implications for the diagnosis of malaria and its control. Tropical Med Int Health. (2013) 18:12–7. doi: 10.1111/tmi.12005
22. Chaturvedi, R, Deora, N, Bhandari, D, Parvez, S, Sinha, A, and Sharma, A. Trends of neglected plasmodium species infection in humans over the past century in India. One Health. (2021b) 11:100190. doi: 10.1016/j.onehlt.2020.100190
23. Haanshuus, CG, Chandy, S, Manoharan, A, and Vivek, R. A high malaria prevalence identified by PCR among patients with acute undifferentiated fever in India. PLoS One. (2016) 11:e0158816. doi: 10.1371/journal.pone.0158816
24. Tyagi, RK, Das, MK, Singh, SS, and Sharma, YD. Discordance in drug resistance-associated mutation patterns in marker genes of Plasmodium falciparum and Plasmodium knowlesi during coinfections. J Antimicrob Chemother. (2013) 68:1081–8. doi: 10.1093/jac/dks508
25. Sholapurkar, S, Mahajan, R, Gupta, A, and Prasad, R. Malarial parasite density in infected pregnant women from northern India. Indian J Med Res. (1988b) 88:228–30.
26. Kaushik, A, Sharma, VK, Sadhna Kumar, R, and Mitra, R. Malarial placental infection and low birth weight babies. Materia Medica Polona. Pol J Med Pharm. (1992) 24:109–10.
27. Nair, LS, and Nair, AS. Effects of malaria infection on pregnancy. Indian J Malariol. (1993) 30:207–14.
28. Singh, N, Shukla, MM, Srivastava, R, and Sharma, V. Prevalence of malaria among pregnant and non-pregnant women of district Jabalpur, Madhya Pradesh. Indian J Malariol. (1995) 32:6–13.
29. Singh, N, Shukla, MM, and Valecha, N. Malaria parasite density in pregnant women of district Jabalpur, Madhya Pradesh. Indian J Malariol. (1996) 33:41–7.
30. Singh, N, Saxena, A, Chand, S, Valecha, N, and Sharma, V. Studies on malaria during pregnancy in a tribal area of Central India (Madhya Pradesh). Indian J Malariol. (1998) 29:10–7.
31. Singh, N, Shukla, MM, and Sharma, V. Epidemiology of malaria in pregnancy in Central India. Bull World Health Organ. (1999) 77:567–72.
32. Das, L. Malaria during pregnancy and its effects on foetus in a tribal area of Koraput district, Orissa. Indian J Malariol. (2000) 37:11–7.
33. Singh, N, Mehra, R, and Srivastava, N. Malaria during pregnancy and infancy, in an area of intense malaria transmission in Central India. Ann Trop Med Parasitol. (2001a) 95:19–29. doi: 10.1080/00034983.2001.11813611
34. Singh, N, Saxena, A, and Sharma, V. Status of chloroquine efficacy against plasmodium falciparum in pregnant women in a tribal area of Central India. Curr Sci. (2001b) 80:618–20.
35. Singh, N, Saxena, A, and Shrivastava, R. Placental Plasmodium vivax infection and congenital malaria in Central India. Ann Trop Med Parasitol. (2003) 97:875–8. doi: 10.1179/000349803225002688
36. Singh, N, Saxena, A, Awadhia, SB, Shrivastava, R, and Singh, MP. Evaluation of a rapid diagnostic test for assessing the burden of malaria at delivery in India. Am J Trop Med Hyg. (2005) 73:855–8. doi: 10.4269/ajtmh.2005.73.855
37. Ahmed, R, Singh, N, ter Kuile, FO, Udhayakumar, V, Desai, M, Dash, A, et al. Rapid assessment of the burden of malaria in pregnancy in Madhya Pradesh. India Trop Med Int Health. (2007) 12:46.
38. Chawla, S, and Manu, V. Malaria in pregnancy. Med J Armed Forces India. (2007) 63:147–8. doi: 10.1016/S0377-1237(07)80060-8
39. Hamer, DH, Singh, MP, Wylie, BJ, Yeboah-Antwi, K, Tuchman, J, Desai, M, et al. Burden of malaria in pregnancy in Jharkhand state, India. Malar J. (2009) 8:210. doi: 10.1186/1475-2875-8-210
40. Guin, G, Shaw, K, and Khare, S. Placental malaria prevalence of infestation amongst febrile pregnant women in Central India: maternal and perinatal outcome. J Obstetr Gynecol India. (2012) 62:25–31. doi: 10.1007/s13224-012-0153-9
41. Singh, N, Singh, MP, Wylie, BJ, Hussain, M, Kojo, YA, Shekhar, C, et al. Malaria prevalence among pregnant women in two districts with differing endemicity in Chhattisgarh, India. Malar J. (2012) 11:274. doi: 10.1186/1475-2875-11-274
42. Qureshi, IA, Arlappa, N, and Qureshi, MA. Prevalence of malaria and anemia among pregnant women residing in malaria-endemic forest villages in India. Int J Gynecol Obstet. (2014) 127:93. doi: 10.1016/j.ijgo.2014.06.009
43. Singh, J, Soni, D, Mishra, D, Singh, HP, and Bijesh, S. Placental and neonatal outcome in maternal malaria. Indian Pediatr. (2014) 51:285–8. doi: 10.1007/s13312-014-0402-3
44. Ahmed, R, Singh, N, Ter Kuile, FO, Bharti, PK, Singh, PP, Desai, M, et al. Placental infections with histologically confirmed plasmodium falciparum are associated with adverse birth outcomes in India: a cross-sectional study. Malar J. (2014) 13:1–12. doi: 10.1186/1475-2875-13-232
45. Palem, G, and Pal, SJ. Maternal and fetal outcome of malaria in pregnancy. Int J Reprod Contracept Obstet Gynecol. (2019) 8:4040–4. doi: 10.18203/2320-1770.ijrcog20194377
46. Sohail, M, Shakeel, S, Kumari, S, Bharti, A, Zahid, F, Anwar, S, et al. Prevalence of malaria infection and risk factors associated with anaemia among pregnant women in semiurban community of Hazaribag, Jharkhand, India. Biomed Res Int. (2015) 2015:740512. doi: 10.1155/2015/740512
47. Singh, N, Bharti, PK, Singh, MP, Singh, R, Yeboah-Antwi, K, Desai, M, et al. What is the burden of submicroscopic malaria in pregnancy in Central India? Pathog Glob Health. (2015) 109:30–8. doi: 10.1179/2047773215Y.0000000002
48. Khan, HM, Kirmani, S, Khan, PA, and İslamss, U. Prevalence of chloroquine resistant plasmodium falciparum malaria in pregnant females attending north Indian hospital. East J Med. (2016) 21:64–8. doi: 10.5505/ejm.2016.93377
49. Corrêa, G, Das, M, Kovelamudi, R, Jaladi, N, Pignon, C, Vysyaraju, K, et al. High burden of malaria and anemia among tribal pregnant women in a chronic conflict corridor in India. Confl Heal. (2017) 11:10. doi: 10.1186/s13031-017-0113-1
50. Bardají, A, Martínez-Espinosa, FE, Arévalo-Herrera, M, Padilla, N, Kochar, S, Ome-Kaius, M, et al. Burden and impact of plasmodium vivax in pregnancy: a multi-Centre prospective observational study. PLoS Negl Trop Dis. (2017) 11:e0005606. doi: 10.1371/journal.pntd.0005606
51. Singh, KP, Shakeel, S, Naskar, N, Bharti, A, Kaul, A, Anwar, S, et al. Role of IL-1β IL-6 and TNF-α cytokines and TNF-α promoter variability in plasmodium vivax infection during pregnancy in endemic population of Jharkhand, India. Mol Immunol. (2018) 97:82–93. doi: 10.1016/j.molimm.2018.03.019
52. Chandrashekar, VN, Punnath, K, Dayanand, KK, Achur, RN, Kakkilaya, SB, Jayadev, P, et al. Malarial anemia among pregnant women in the south-western coastal city of Mangaluru in India. Inform Med Unlock. (2019) 15:100159. doi: 10.1016/j.imu.2019.02.003
53. Kuepfer, I, Mishra, N, Bruce, J, Mishra, V, Anvikar, AR, Satpathi, S, et al. Effectiveness of intermittent screening and treatment for the control of malaria in pregnancy: a cluster randomised trial in India. BMJ Glob Health. (2019) 4:1–8. doi: 10.1136/bmjgh-2019-001399
54. Garg, S, Dewangan, M, and Barman, O. Malaria prevalence in symptomatic and asymptomatic pregnant women in a high malaria-burden state in India. Trop Med Health. (2020) 48:71. doi: 10.1186/s41182-020-00259-y
55. Singh, PP, Bhandari, S, Sharma, RK, Singh, N, and Bharti, PK. Association of angiopoietin dysregulation in placental malaria with adverse birth outcomes. Dis Markers. (2020) 2020:6163487. doi: 10.1155/2020/6163487
56. Chandrashekar, VN, Dayanand Kiran, K, Punnath, K, Achur Rajeshwara, N, Kumari Suchetha, N, and Channe Gowda, D. Epidemiology of malaria during pregnancy in Mangaluru city in the southwestern coastal region of India. Res J Biotechnol. (2021) 16:103–8.
57. Sholapurkar, S, Gupta, A, and Mahajan, R. Clinical course of malaria in pregnancy—a prospective controlled study from India. Trans R Soc Trop Med Hyg. (1988a) 82:376–9. doi: 10.1016/0035-9203(88)90124-1
59. Osarfo, J, Ampofo, GD, and Tagbor, H. Trends of malaria infection in pregnancy in Ghana over the past two decades: a review. Malar J. (2022) 21:3. doi: 10.1186/s12936-021-04031-3
60. Chen, I, Clarke, SE, Gosling, R, Hamainza, B, Killeen, G, Magill, A, et al. “Asymptomatic” malaria: a chronic and debilitating infection that should be treated. PLoS Med. (2016) 13:e1001942. doi: 10.1371/journal.pmed.1001942
61. Lin, JT, Saunders, DL, and Meshnick, SR. The role of submicroscopic malaria in malaria transmission: what is the evidence? Trends Parasitol. (2014) 30:183–90. doi: 10.1016/j.pt.2014.02.004
62. Deora, N, Yadav, C, Pande, V, and Sinha, A. A systematic review and meta-analysis on sub-microscopic Plasmodium infections in India: different perspectives and global challenges. Lancet Reg Health. (2022) 2:100012. doi: 10.1016/j.lansea.2022.05.001
63. Wu, L., Hoogen, L.L.Van, Den, Slater H., Walker, P.G.T., Ghani, A.C., Drakeley, C.J., et al. (2015). Comparison of diagnostics for the detection of asymptomatic Plasmodium falciparum infections to inform control and elimination strategies. Nature 7580, S86–S93. doi: 10.1038/nature16039
64. Cowman, AF, Healer, J, Marapana, D, and Marsh, K. Malaria: biology and disease. Cells. (2016) 167:610–24. doi: 10.1016/j.cell.2016.07.055
65. Schantz-Dunn, J, and Nour, NM. Malaria and pregnancy: a global health perspective. Rev Obstet Gynecol. (2009) 2:186–92. doi: 10.3909/riog0091
66. White, NJ, Pukrittayakamee, S, Tinh Hien, T, Abul Faiz, M, Mokuolu, OA, and Dondorp, AM. Malaria. Lancet. (2014) 391:1608–21. doi: 10.1016/S0140-6736(18)30324-6
67. Lagerberg, RE. Malaria in pregnancy: a literature review. J Midwifery Womens Health. (2008) 53:209–15. doi: 10.1016/j.jmwh.2008.02.012
68. Haldar, K, and Mohandas, N. Malaria, erythrocytic infection, and anemia. Hematology. (2009):87–93. doi: 10.1182/asheducation-2009.1.87
69. Ho, M, and White, NJ. Molecular mechanisms of cytoadherence in malaria. Am J Phys Cell Phys. (1999) 276:C1231–42. doi: 10.1177/175114370400500315
70. Carvalho, BO, Lopes, SCP, Nogueira, PA, Orlandi, PP, Bargieri, DY, Blanco, YC, et al. On the cytoadhesion of Plasmodium vivax-infected erythrocytes. J Infect Dis. (2010) 202:638–47. doi: 10.1086/654815
71. Chotivanich, K, Udomsangpetch, R, Suwanarusk, R, Pukrittayakamee, S, Wilairatana, P, Beeson, JG, et al. Plasmodium vivax adherence to placental glycosaminoglycans. PLoS One. (2012) 7:e34509. doi: 10.1371/journal.pone.0034509
72. Lee, W-C, Shahari, S, Nguee, SYT, Lau, Y-L, and Rénia, L. Cytoadherence properties of plasmodium knowlesi-infected erythrocytes. Front Microbiol. (2022) 12:804417. doi: 10.3389/fmicb.2021.804417
73. Maitra, N, Joshi, M, and Hazra, M. Maternal manifestations of malaria in pregnancy: a review. Ind J Matern Child Health. (1993) 4:98–101.
74. Nayak, KC, Khatri, MP, Gupta, BK, Sirohi, P, Choudhary, V, Verma, SK, et al. Spectrum of vivax malaria in pregnancy and its outcome: a hospital-based study. J Vector Borne Dis. (2009) 46:299–302.
75. Matlani, M, Kojom, LP, Mishra, N, Dogra, V, and Singh, V. Severe vivax malaria trends in the last two years: a study from a tertiary care Centre, Delhi, India. Ann Clin Microbiol Antimicrob. (2020) 19:49. doi: 10.1186/s12941-020-00393-9
76. Rahimi, BA, Thakkinstian, A, White, NJ, Sirivichayakul, C, Dondorp, AM, and Chokejindachai, W. Severe vivax malaria: a systematic review and meta-analysis of clinical studies since 1900. Malar J. (2014) 13:481. doi: 10.1186/1475-2875-13-481
77. Arya, T, Prasad, R, and Virk, K. Cerebral malaria in pregnancy. J Assoc Physicians India. (1989) 37:592–3.
78. Sharma, R, Suneja, A, Yadav, A, and Guleria, K. Plasmodium vivax induced acute respiratory distress syndrome—a diagnostic and therapeutic dilemma in preeclampsia. J Clin Diagn Res. (2017) 11:QD03–QD04. doi: 10.7860/JCDR/2017/25529.9710
79. Kochar, DK, Thanvi, I, Joshi, A, Shubhakaran Agarwal, N, and Jain, N. Mortality trends in falciparum malaria—effect of gender difference and pregnancy. J Assoc Physicians India. (1999) 47:774–8.
80. Kochar, DK, Thanvi, I, Joshi, A, Subhakaran Aseri, S, and Kumawat, BL. Falciparum malaria and pregnancy. Indian J Malariol. (1998) 35:123–30.
81. Kochar, DK, Kumawat, BL, Karan, S, Kochar, SK, and Argawal, RP. Severe and complicated malarial in Bikaner (Rajasthan), Western India. Southeast Asian J Trop Med Public Health. (1997) 28:259–67.
82. Mishra, S, Mohanty, S, Satpathy, S, and Patnaik, J. Complicated falciparum malaria during pregnancy. J Obstet Gynaecol. (1998) 48:31–4.
83. Goplani, K, Shah, P, Gera, D, Gumber, M, Dabhi, M, Feroz, A, et al. Pregnancy-related acute renal failure: a single-center experience. Ind J Nephrol. (2008) 18:17–27. doi: 10.4103/0971-4065.41283
84. Hirani, M, and Vadhvana, SK. Study of clinical profile of P. vivax malaria in pregnancy. J Res Med Dental Sci. (2015) 3:3076311. doi: 10.5455/jrmds.20153414
85. Datta, M, Biswas, J, Dasgupta, S, Banerjee, K, Choudhury, S, Sengupta, SK, et al. Comparative study on antenatal and perinatal outcome of vivax and falciparum malaria in a tertiary care hospital of Kolkata, India. J Clin Diagn Res. (2017) 11:QC01–QC04. doi: 10.7860/JCDR/2017/23051.9195
86. Rao Bhaskara, VJ, Kumar, AV, Deepthi, V, and Thotakura, TM. Comparative study of plasmodium falciparum and plasmodium vivax malaria in pregnant and non-pregnant women in Visakhapatnam, Andhra Pradesh, India. Int J Health Clin Res. (2021) 4:404–7.
87. Mathur, SL, Hakim, A, Mathur, S, and Choudhary, P. Cerebral malaria in pregnancy. J Assoc Physicians India. (1991) 39:583–4.
88. Seal, SL, Mukhopadhay, S, and Ganguly, RP. Malaria in pregnancy. J Indian Med Assoc. (2010) 108:487–90.
89. Anand, AC, and Puri, P. Jaundice in malaria. J Gastroenterol Hepatol. (2005) 20:1322–32. doi: 10.1111/j.1440-1746.2005.03884.x
90. Gupta, P, Sharma, R, Chandra, J, Kumar, V, Singh, R, Pande, V, et al. Clinical manifestations and molecular mechanisms in the changing paradigm of vivax malaria in India. Infect Genet Evol. (2016) 39:317–24. doi: 10.1016/j.meegid.2016.02.014
91. Kumar, A, and Shashirekha,. Thrombocytopenia—an indicator of acute vivax malaria. Indian J Pathol Microbiol. (2006) 49:505–8.
92. Mehta, AA, and Mehta, AC. Clinical presentation of toxoplasmosis in pregnant women. J Obstet Gynaecol. (1989) 5:165–70.
93. Pande, A, and Guharoy, D. A case report of Plasmodium vivax, Plasmodium falciparum and dengue co-infection in a 6 months pregnancy. Ann Med Health Sci Res. (2013) 3:S16–7. doi: 10.4103/2141-9248.121211
94. Mahajan, NN, Kesarwani, SN, Shinde, SS, Nayak, A, Modi, DN, Mahale, SD, et al. Co-infection of malaria and dengue in pregnant women with SARS-CoV-2. Int J Gynecol Obstet. (2020) 151:459–62. doi: 10.1002/ijgo.13415
95. Jaju, PB, and Bhavi, SB. Convulsions: eclampsia or malaria or both! J South Asian Feder Obstetr Gynaecol. (2015) 7:245–6. doi: 10.5005/jp-journals-10006-1373
96. Das, D, Grais, R, Okiro, E, Stepniewska, K, Mansoor, R, van der Kam, S, et al. BMC Med. (2018) 16:186. doi: 10.1186/s12916-018-1177-5
97. Rogerson, SJ, Hviid, L, Duffy, PE, Leke, RF, and Taylor, DW. Malaria in pregnancy: pathogenesis and immunity. Lancet Infect Dis. (2007) 7:105–17. doi: 10.1016/S1473-3099(07)70022-1
98. Duffy, PE, and Fried, M. Antibodies that inhibit plasmodium falciparum adhesion to chondroitin sulfate a are associated with increased birth weight and the gestational age of newborns. Infect Immun. (2003) 71:6620–3. doi: 10.1128/IAI.71.11.6620
99. O’Neil-Dunne, I, Achur, RN, Agbor-Enoh, ST, Valiyaveettil, M, Naik, RS, Ockenhouse, CF, et al. Gravidity-dependent production of antibodies that inhibit binding of plasmodium falciparum-infected erythrocytes to placental chondroitin sulfate proteoglycan during pregnancy. Infect Immun. (2001) 69:7487–92. doi: 10.1128/IAI.69.12.7487-7492.2001
100. Singh, N, Nagpal, AC, Saxena, A, and Singh, MP. Changing scenario of malaria in Central India, the replacement of Plasmodium vivax by Plasmodium falciparum (1986-2000). Tropical Med Int Health. (2004) 9:364–71. doi: 10.1046/j.1365-3156.2003.01181.x
101. Kumari, S, Garg, N, Kumar, A, Guru, PKI, Ansari, S, Anwar, S, et al. Maternal and severe anaemia in delivering women is associated with risk of preterm and low birth weight: a cross sectional study from Jharkhand, India. One Health. (2019) 8:100098. doi: 10.1016/j.onehlt.2019.100098
102. Moya-Alvarez, V, Abellana, R, and Cot, M. Pregnancy-associated malaria and malaria in infants: an old problem with present consequences. Malar J. (2014) 13:271. doi: 10.1186/1475-2875-13-271
103. Park, S, Nixon, CE, Miller, O, Choi, NK, Kurtis, JD, Friedman, JF, et al. Impact of malaria in pregnancy on risk of malaria in young children: systematic review and meta-analyses. J Infect Dis. (2020) 222:538–50. doi: 10.1093/infdis/jiaa139
104. Agbota, G, Accrombessi, M, Cottrell, G, Martin-Prevel, Y, Milet, J, Ouedraogo, S, et al. Increased risk of malaria during the first year of life in small-for-gestational-age infants: a longitudinal study in Benin. J Infect Dis. (2019) 219:1642–51. doi: 10.1093/infdis/jiy699
105. Olupot-Olupot, P, Eregu, EIE, Naizuli, K, Ikiror, J, Acom, L, and Burgoine, K. Neonatal and congenital malaria: a case series in malaria endemic eastern Uganda. Malar J. (2018) 17:171. doi: 10.1186/s12936-018-2327-0
106. Danwang, C, Bigna, JJ, Nzalie, RNT, and Robert, A. Epidemiology of clinical congenital and neonatal malaria in endemic settings: a systematic review and meta-analysis. Malar J. (2020) 19:312. doi: 10.1186/s12936-020-03373-8
107. Ghosh, SK, and Rahi, M. Malaria elimination in India—the way forward. J Vector Borne Dis. (2019) 56:32–40. doi: 10.4103/0972-9062.257771
108. Ministry of Health & Family Welfare (2016). National framework for malaria elimination, 2016–2030.
109. Rahi, M, and Sharma, A. Malaria control initiatives that have the potential to be gamechangers in India’s quest for malaria elimination. Lancet Reg Health\. (2022) 2:100009. doi: 10.1016/j.lansea.2022.04.005
110. Webster, J, Mishra, VK, Anvikar, AR, Kuepfer, I, Hoyt, J, Bruce, J, et al. Evaluation of implementation of intermittent screening and treatment for control of malaria in pregnancy in Jharkhand, India. Am J Trop Med Hyg. (2020) 102:1343–50. doi: 10.4269/ajtmh.19-0514
111. Raghavendra, K, Chourasia, MK, Swain, DK, Bhatt, RM, Uragayala, S, Dutta, GDP, et al. Monitoring of long-lasting insecticidal nets (LLINs) coverage versus utilization: a community-based survey in malaria endemic villages of Central India. Malar J. (2017) 16:647. doi: 10.1186/s12936-017-2117-0
112. Sundararajan, R, Kalkonde, Y, Gokhale, C, Greenough, PG, and Bang, A. Barriers to malaria control among marginalized tribal communities: a qualitative study. PLoS One. (2013) 8:e81966. doi: 10.1371/journal.pone.0081966
113. Department of He alth & Family Welfare (2012). Evaluation of use of long lasting insecticidal net (LLIN) by pregnant women under ‘Mo Mashari’ scheme and effectiveness of behavior change communication (BCC) messages. Odisha.
114. Jambulingam, P, Gunasekaran, K, Sahu, S, and Vijayakumar, T. Insecticide treated mosquito nets for malaria control in India-experience from a tribal area on operational feasibility and uptake. Mem Institut Oswaldo Cruz, Rio Jan. (2008) 103:165–71. doi: 10.1590/s0074-02762008005000009
115. Wylie, BJ, Hashmi, AH, Singh, N, Singh, MP, Tuchman, J, Hussain, M, et al. Availability and utilization of malaria prevention strategies in pregnancy in eastern India. BMC Public Health. (2010) 10:557. doi: 10.1186/1471-2458-10-557
116. Anvikar, AR, Arora, U, Sonal, G, Mishra, N, Shahi, B, Savargaonkar, D, et al. Antimalarial drug policy in India: past, present & future. Indian J Med Res. (2014) 139:205–15.
117. Pandey, M, Rahi, M, and Sharma, A. The Indian burden of malaria in pregnancy needs assessment. Medicine. (2021) 2:464–9. doi: 10.1016/j.medj.2021.04.017
118. Anvikar, AR, Kuepfer, I, Mishra, V, Bruce, J, Arya, T, Mishra, DR, et al. Efficacy of two artemisinin-based combinations for the treatment of malaria in pregnancy in India: a randomized controlled trial. Malar J. (2018) 17:246. doi: 10.1186/s12936-018-2393-3
119. Arya, A, Kojom Foko, LP, Chaudhry, S, Sharma, A, and Singh, V. Artemisinin-based combination therapy (ACT) and drug resistance molecular markers: a systematic review of clinical studies from two malaria endemic regions – India and sub-Saharan Africa. Int J Parasitol Drugs Drug Resist. (2021) 15:43–56. doi: 10.1016/j.ijpddr.2020.11.006
120. Chaturvedi, R, Chhibber-Goel, J, Verma, I, Gopinathan, S, Parvez, S, and Sharma, A. Geographical spread and structural basis of sulfadoxine-pyrimethamine drug-resistant malaria parasites. Int J Parasitol. (2021a) 51:505–25. doi: 10.1016/j.ijpara.2020.12.011
121. Chhibber-Goel, J, and Sharma, A. Profiles of Kelch mutations in plasmodium falciparum across South Asia and their implications for tracking drug resistance. Int J Parasitol Drugs Drug Resist. (2019) 11:49–58. doi: 10.1016/j.ijpddr.2019.10.001
122. Ranjha, R, and Sharma, A. Forest malaria: the prevailing obstacle for malaria control and elimination in India. BMJ Glob Health. (2021) 6:1–7. doi: 10.1136/bmjgh-2021-005391
123. Sharma, RK, Thakor, HG, Saha, KB, Sonal, GS, Dhariwal, AC, and Singh, N. Malaria situation in India with special reference to tribal areas. Indian J Med Res. (2015) 142:537–45. doi: 10.4103/0971-5916.159510
124. Kotepui, M, Masangkay, FR, Kotepui, KU, and De Jesus Milanez, G. Misidentification of plasmodium ovale as plasmodium vivax malaria by a microscopic method: a meta-analysis of confirmed P. ovale cases. Sci Rep. (2020c) 10:21807. doi: 10.1038/s41598-020-78691-7
125. Savargaonkar, D, Shah, N, das, M, Srivastava, B, and Valecha, N. Plasmodium malariae infection: a case of missed diagnosis. J Vector Borne Dis. (2014) 51:149–51.
126. Groger, M, Veletzky, L, Lalremruata, A, Cattaneo, C, Mischlinger, J, Zoleko, RM, et al. Prospective clinical and molecular evaluation of potential Plasmodium ovale curtisi and wallikeri relapses in a high-transmission setting. Clin Infect Dis. (2019) 69:2119–26. doi: 10.1093/cid/ciz131
127. Kotepui, M, Kotepui, KU, Milanez, GD, and Masangkay, FR. Global prevalence and mortality of severe Plasmodium malariae infection: a systematic review and meta-analysis. Malar J. (2020a) 19:274. doi: 10.1186/s12936-020-03344-z
128. Kotepui, M, Kotepui, KU, Milanez, GD, and Masangkay, FR. Severity and mortality of severe plasmodium ovale infection: a systematic review and meta-analysis. PLoS One. (2020b) 15:e0235014. doi: 10.1371/journal.pone.0235014
129. Mahittikorn, A, Masangkay, FR, Kotepui, KU, Milanez, GDJ, and Kotepui, M. Comparison of plasmodium ovale curtisi and plasmodium ovale wallikeri infections by a meta-analysis approach. Sci Rep. (2021) 11:6409. doi: 10.1038/s41598-021-85398-w
130. Bousema, T, and Drakeley, C. Epidemiology and infectivity of plasmodium falciparum and plasmodium vivax gametocytes in relation to malaria control and elimination. Clin Microbiol Rev. (2011) 24:377–410. doi: 10.1128/CMR.00051-10
131. Sattabongkot, J, Tsuboi, T, Zollner, GE, Sirichaisinthop, J, and Cui, L. Plasmodium vivax transmission: chances for control? Trends Parasitol. (2004) 20:192–8. doi: 10.1016/j.pt.2004.02.001
132. Unger, HW, Ashorn, P, Cates, JE, Dewey, KG, and Rogerson, SJ. Undernutrition and malaria in pregnancy—a dangerous dyad? BMC Med. (2016) 14:142. doi: 10.1186/s12916-016-0695-2
133. Nema, S, Singh, A, Krishna, S, Poriya, R, Dubey, S, Ali, NA, et al. Unreported mixed plasmodium species infection may increase vivax malaria in India: a challenge for malaria elimination. Trans R Soc Trop Med Hyg. (2022) 116:2022. doi: 10.1093/trstmh/trac007
134. Singh, US, Siwal, N, Pande, V, and Das, A. Can mixed parasite infections thwart targeted malaria elimination program in India? Biomed Res Int. (2017) 2017:2847548. doi: 10.1155/2017/2847548
135. Alemu, A, Shiferaw, Y, Addis, Z, Mathewos, B, and Birhan, W. Effect of malaria on HIV/AIDS transmission and progression. Parasit Vectors. (2013) 6:18. doi: 10.1186/1756-3305-6-18
136. Kwenti, TE. Malaria and HIV coinfection in sub-Saharan Africa: prevalence, impact, and treatment strategies. Res Rep Trop Med. (2018) 9:123–36. doi: 10.2147/rrtm.s154501
137. Dini, S, Douglas, NM, Poespoprodjo, JR, Kenangalem, E, Sugiarto, P, Plumb, ID, et al. The risk of morbidity and mortality following recurrent malaria in Papua, Indonesia: a retrospective cohort study. BMC Med. (2020) 18:28. doi: 10.1186/s12916-020-1497-0
138. Price, RN, Commons, RJ, Battle, KE, Thriemer, K, and Mendis, K. Plasmodium vivax in the era of the shrinking P. Falciparum map. Trends Parasitol. (2020) 36:560–70. doi: 10.1016/j.pt.2020.03.009
139. Luzzatto, L, Nannelli, C, and Notaro, R. Glucose-6-phosphate dehydrogenase deficiency. Hematol Oncol Clin North Am. (2016) 30:373–93. doi: 10.1016/j.hoc.2015.11.006
140. Anvikar, AR, Sahu, P, Pradhan, MM, Sharma, S, Ahmed, N, Yadav, CP, et al. Active pharmacovigilance for primaquine radical cure of plasmodium vivax malaria in Odisha, India. Am J Trop Med Hyg. (2022) 106:831–40. doi: 10.4269/ajtmh.21-0816
141. Saravu, K, Kumar, R, Ashok, H, Kundapura, P, Kamath, V, Kamath, A, et al. Therapeutic assessment of chloroquine-primaquine combined regimen in adult cohort of Plasmodium vivax malaria from primary care centres in southwestern India. PLoS One. (2016) 11:e0157666. doi: 10.1371/journal.pone.0157666
142. Antonelli, LR, Junqueira, C, Vinetz, JM, Golenbock, DT, Ferreira, MU, and Gazzinelli, RT. The immunology of Plasmodium vivax malaria. Immunol Rev. (2020) 293:163–89. doi: 10.1111/imr.12816
143. Lin, E, Kiniboro, B, Gray, L, Dobbie, S, Robinson, L, Laumaea, A, et al. Differential patterns of infection and disease with P. falciparum and P. vivax in young Papua new Guinean children. PLoS One. (2010) 5:e9047. doi: 10.1371/journal.pone.0009047
144. Michon, P, Cole-Tobian, JL, Dabod, E, Schoepflin, S, Igu, J, Susapu, M, et al. The risk of malarial infections and disease in Papua new Guinean children. Am J Trop Med Hyg. (2007) 76:997–1008. doi: 10.4269/ajtmh.2007.76.997
145. Kojom Foko, LP, Pande, V, and Singh, V. Field performances of rapid diagnostic tests detecting human plasmodium species: a systematic review and meta-analysis in India, 1990-2020. Diagnostics. (2021b) 11:590. doi: 10.3390/diagnostics11040590
146. White, NJ. Determinants of relapse periodicity in Plasmodium vivax malaria. Malar J. (2011) 10:297. doi: 10.1186/1475-2875-10-297
147. Kojom Foko, LP, Narang, G, Tamang, S, Hawadak, J, Jakhan, J, Sharma, A, et al. The spectrum of clinical biomarkers in severe malaria and new avenues for exploration. Virulence. (2022) 13:634–54. doi: 10.1080/21505594.2022.2056966
148. Longley, RJ, White, MT, Takashima, E, Brewster, J, Morita, M, Harbers, M, et al. Development and validation of serological markers for detecting recent Plasmodium vivax infection. Nat Med. (2020) 26:741–9. doi: 10.1038/s41591-020-0841-4
149. Poti, KE, Sullivan, DJ, Dondorp, AM, and Woodrow, CJ. HRP2: transforming malaria diagnosis, but with caveats. Trends Parasitol. (2020) 36:112–26. doi: 10.1016/j.pt.2019.12.004
150. Kojom, LP, and Singh, V. Prevalence of plasmodium falciparum field isolates with deletions in histidine-rich protein 2 and 3 genes in context with sub-Saharan Africa and India: a systematic review and meta-analysis. Malar J. (2020) 19:46. doi: 10.1186/s12936-019-3090-6
151. Singh, V, and Kojom, LP. Deletions in the plasmodium falciparum histidine-rich protein 2 gene: an emerging threat to the elimination of malaria in India. J Vector Borne Dis. (2019) 56:85–6. doi: 10.1128/CMR.15.1.66-78.2002
152. Saito, M, McGready, R, Tinto, H, Rouamba, T, Mosha, D, Rulisa, S, et al. Pregnancy outcomes after first-trimester treatment with artemisinin derivatives versus non-artemisinin antimalarials: a systematic review and individual patient data meta-analysis. Lancet. (2023) 401:118–30. doi: 10.1016/S0140-6736(22)01881-5
153. Shibeshi, W, Baye, AM, Alemkere, G, and Engidawork, E. Efficacy and safety of artemisinin-based combination therapy for the treatment of uncomplicated malaria in pregnant women: a systematic review and meta-analysis. Ther Clin Risk Manag. (2021) 17:1353–70. doi: 10.2147/TCRM.S336771
154. Kojom Foko, L, Ntoumba, A, Nyabeyeu Nyabeyeu, H, Wepnje, GB, Tonga, C, and Lehman, LG. Prevalence, patterns and predictors of self-medication with anti-malarial drugs among Cameroonian mothers during a recent illness episode. J Med Biomed Sci. (2018) 7:29–39. doi: 10.4314/jmbs.v7i1.4
155. Nayyar, GML, Breman, JG, Newton, PN, and Herrington, J. Poor-quality antimalarial drugs in Southeast Asia and sub-Saharan Africa. Lancet Infect Dis. (2012) 12:488–96. doi: 10.1016/S1473-3099(12)70064-6
Glossary
Keywords: malaria, pregnancy, epidemiology, outcomes, prevention, treatment, India
Citation: Kojom Foko LP and Singh V (2023) Malaria in pregnancy in India: a 50-year bird’s eye. Front. Public Health. 11:1150466. doi: 10.3389/fpubh.2023.1150466
Edited by:
Miguel Angel Garcia Bereguiain, University of the Americas, EcuadorReviewed by:
Gerhard Wunderlich, University of São Paulo, BrazilStephen Rogerson, The University of Melbourne, Australia
Copyright © 2023 Kojom Foko and Singh. This is an open-access article distributed under the terms of the Creative Commons Attribution License (CC BY). The use, distribution or reproduction in other forums is permitted, provided the original author(s) and the copyright owner(s) are credited and that the original publication in this journal is cited, in accordance with accepted academic practice. No use, distribution or reproduction is permitted which does not comply with these terms.
*Correspondence: Vineeta Singh, dmluZWV0YXNfMjAwMEB5YWhvby5jb20=
‡ORCID: Loick Pradel Kojom Foko orcid.org/0000-0002-4286-147X
Vineeta Singh orcid.org/0000-0003-0113-7394