- 1Animal and Human Health Program, International Livestock Research Institute, Nairobi, Kenya
- 2Department of Veterinary Pathology, Microbiology and Parasitology, Faculty of Veterinary Medicine, University of Nairobi, Nairobi, Kenya
- 3Department of Veterinary and Animal Sciences, University of Copenhagen, Copenhagen, Denmark
- 4Department of Disease Control, London School of Hygiene and Tropical Medicine, London, United Kingdom
Introduction: Campylobacter bacteria is a major cause of foodborne-related bacterial gastroenteritis in humans worldwide. It is known to cause diarrhea in young children which has been shown to directly affect their weight and height as a result of malnutrition. Severe cases of diarrhea can also lead to death. Most of the burden is experienced in resource-limited countries in Africa and Southeast Asia where the disease is linked to poor hygiene and sanitation. The objective of this study was to determine the prevalence of Campylobacter in children aged between 6 and 24 months in Nairobi, Kenya and identify potential risk factors associated with their occurrence.
Methods: A cross-sectional study was carried out between May to December 2021. A total of 585 randomly selected households were visited in two wards (Uthiru/Ruthimitu and Riruta) in Dagoretti South sub-county, Nairobi. A questionnaire regarding how children’s food is handled, the major foods consumed, sanitation and hygiene, and animal ownership was conducted among caregivers to identify associated risk factors. Stool samples were collected from 540/585 children and screened for the presence of Campylobacter using culture-based methods and confirmed through PCR.
Results: Of the 540 children’s stool samples processed, Campylobacter isolates were detected in 4.8% (26/540). Campylobacter jejuni (C. jejuni) was the most common species in 80.8% of positive samples compared to Campylobacter coli (C. coli) in 26.9% of samples. In six samples, both C. jejuni and C. coli were isolated, while in four samples, it was not possible to speciate the Campylobacter. Drinking cow’s milk (OR 4.2, 95% CI 1.4 – 12.6) and the presence of animal feces in the compound (OR 3.4, 95% CI 1.1 – 10.3) were found to be statistically associated with Campylobacter carriage in children.
Discussion: The carriage of Campylobacter in children in this community indicates a need for further investigation on source attribution to understand transmission dynamics and inform where to target interventions. Awareness creation among caregivers on good personal and food hygiene is needed, including boiling milk before consumption. Implementation of biosecurity measures at the household level is highly recommended to reduce contact between animals and humans.
1. Introduction
Campylobacteriosis is a major foodborne zoonotic disease, causing gastroenteritis in humans worldwide (1–3). Campylobacter infection is hyper-endemic in lower and middle income countries (LMIC) (1). It is estimated that Campylobacter organisms are responsible for 100 million foodborne diarrheal cases, and more than 21,000 deaths throughout the world each year (4). The majority (80–90%) of human infections are caused by Campylobacter jejuni, with 10–20% of infections caused by C. coli (1, 5). Other Campylobacter species that have been associated with diarrhea in humans include: C. lari, C. upsaliensis, C. fetus, C. concisus, and C. hyointestinalis (6).
Campylobacter is a leading bacterial cause of diarrhea in children below 2 years of age from high income and LMICs (1, 2, 7). The isolation rate of Campylobacter among children below 5 years with diarrhea, from the LMIC’s is estimated to be 5–20%; with majority of the infection seen in children below 2 years (1, 5, 8). This number may be greatly underestimated because of the fastidious nature of Campylobacter therefore making it difficult to isolate from stool and due to a lack of sensitive and consistent laboratory diagnosis and surveillance for Campylobacter (3). Campylobacter is a major cause of bloody diarrhea in children (2), and affects children’s weight gain and linear growth and is therefore associated with stunting in young children (9).
Campylobacter are considered commensals in food animals, with poultry being the main reservoir (8, 10). Ingestion of contaminated poultry meat and poor handling practices of raw chicken have been shown to be the main cause of foodborne Campylobacter infections in humans (11). Other reported sources of transmission to humans include consumption of contaminated water and raw milk or milk products (8). Presence of animal feces and open garbage in the cooking area, home slaughtering and access to animals in the houses also contribute to the risk of contamination (12).
We conducted a cross sectional study to determine the prevalence of Campylobacter in children aged between 6 and 24 months in a low-income peri-urban setting, and to identify potential sociodemographic, hygiene and sanitation as well as biosecurity factors that could be associated with the occurrence of Campylobacter in children. The importance of this study is the identification of Campylobacter carriage in children 6–24 months. Undetected Campylobacter infections may result in malnutrition and exacerbate deficiencies from food insecurity in this resource limited setting. The identification of risk factors is crucial for developing appropriate interventions to reduce infections in children.
2. Materials and methods
2.1. Study design and study settings
We conducted a cross-sectional study of households with a child aged between 6 to 24 months from May to December 2021. The study took place in a low-income periurban community in Dagoretti South sub-county, Nairobi, Kenya (Figure 1). As per the 2019 census, Dagoretti is termed a peri-urban area, which comprises over 400,000 people (9.9% of Nairobi’s population) and covers 29.1km2 (4.1% of the Nairobi’s surface area) (13). This subcounty has been shown to have poor environmental conditions, a high infectious disease burden, and most dwellers live in low-income settlements (14). The study took place in two wards of the five wards that make up Dagoretti South namely -Uthiru/Ruthimitu and Riruta. Both are classed as low-income settlements (14), with limited water or sanitation infrastructure, poor hygiene, and many residents are livestock keepers or in contact with livestock therefore, at risk of foodborne diseases.
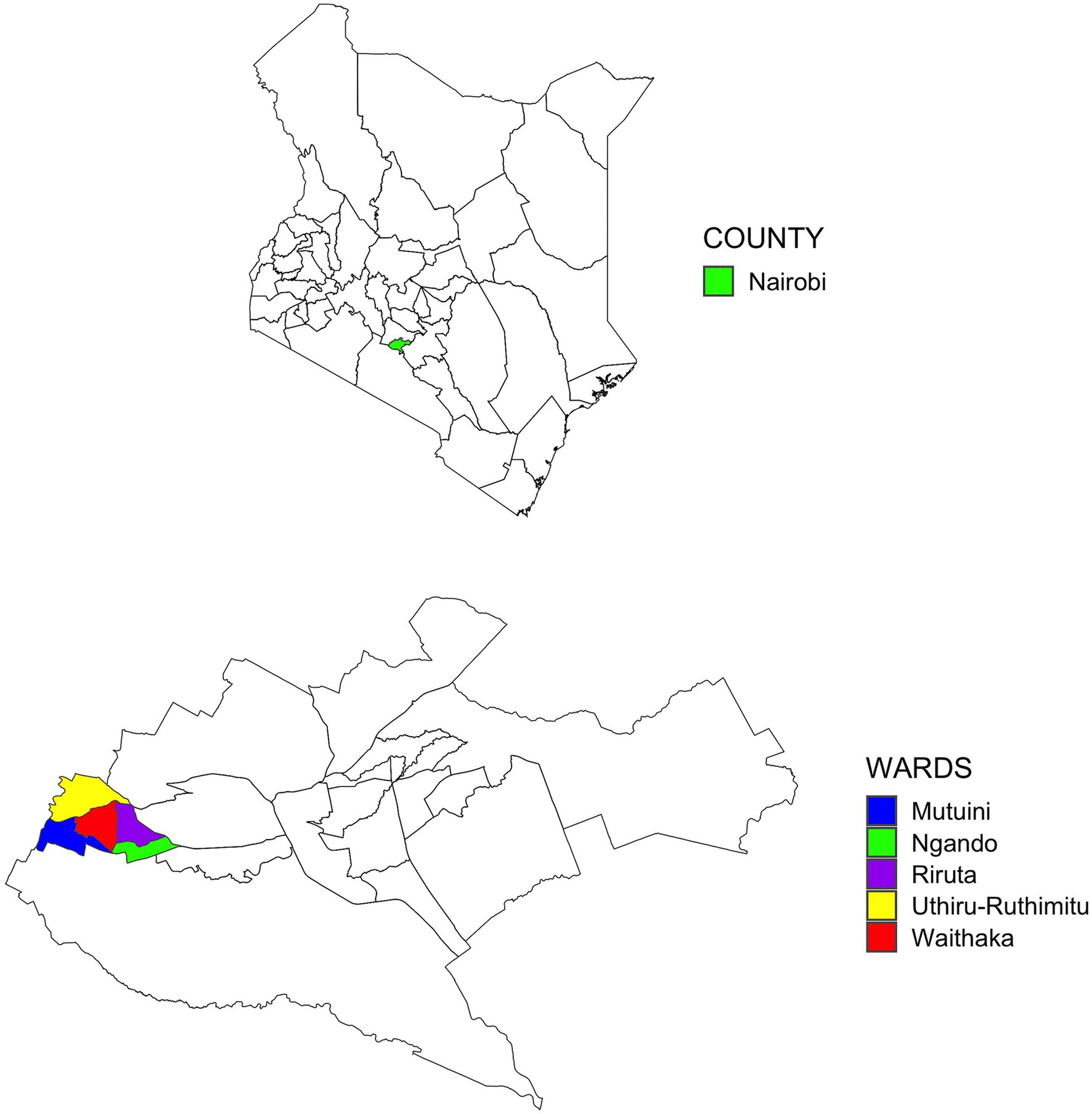
Figure 1. Map of the study area indicating the wards of Dagoretti South Subcounty within Nairobi County (bottom) in Kenya (top).
2.2. Study population and recruitment strategy
The study population was randomly selected from the two wards. Three community health units (CHUs) were identified, one CHU in Riruta and two CHUs in Uthiru/Ruthimitu. These were selected due to availability of a health centre for referrals when needed. All active Community Health Volunteers (CHVs) were identified in the three CHUs (n = 259). Initially, 100 CHVs were randomly selected proportional to the number of CHVs in each community health unit. There were no exclusion criteria. Ninety CHVs were available to participate in the study and provided a list of all eligible households in their catchment area with a child aged between 6 and 24 months. Between five and seven households were randomly selected per CHV to participate in the study, where five was the smallest number of eligible households provided by a CHV. In total, 585 household participated in the study.
2.3. Data and sample collection
Nurses were hired as study enumerators and trained in data and sample collection. They visited each household, obtained informed consent from the primary adult caregiver, and conducted a questionnaire survey using Open Data Kit (ODK) Collect App on a Samsung tablet. The structured questionnaire included demographic information on the household size, income, access to services (electricity, water, and sewerage), age of the caregiver and details regarding the child such as sex and age. Information regarding food types eaten by the child in the previous 24 h and any health events were recorded. Information was also collected on water, sanitation, and hygiene conditions, such as the type of drinking water source, the type of sanitation facility, handwashing, and food hygiene practices. Water sources were classified according to the WHO/UNICEF Joint Monitoring Programme for water supply, sanitation and hygiene (JPM) into: safely managed – drinking water from an improved water source that is accessible on premises, available when needed and free from fecal and priority chemical contamination; basic – drinking water from an improved source, provided collection time is not more than 30 min for a roundtrip including queuing; limited – drinking water from an improved source for which collection time exceeds 30 min for a roundtrip including queuing; unimproved – drinking water from an unprotected dug well or unprotected spring; surface water – drinking water directly from a river, dam, lake, pond, stream, canal or irrigation canal. The JPM classification for sanitation include: safely managed – use of improved facilities that are not shared with other households and where excreta are safely disposed of in situ or removed and treated offsite; basic – use of improved facilities which are not shared with other households; limited – use of improved facilities shared between two or more households; unimproved – use of pit latrines without a slab or platform, hanging latrines or bucket latrines; open defecation – disposal of human feces in fields, forests, bushes, open bodies of water, beaches, and other open spaces or with solid waste (15).
From each recruited child, a stool sample was collected in a disposable diaper (Pampers, Proctor and Gamble, Cincinatti, Ohio, United States). The caregiver was instructed on how to fit the diaper and remove it after the child defecated. A sealable plastic bag labelled with the household ID and date was also supplied and the diaper with the sample was placed inside. Repeated visits were made to caregivers to collect stool up to a week following the original visit. Diapers were collected from the household upon caregiver’s notification to the CHV within 2 h, placed in a cool box, maintained at under 4°C with ice packs and delivered to the International Livestock Research Institute (ILRI) laboratories to be processed within 6 h.
2.4. Isolation and identification of Campylobacter
Stool samples were prepared for enrichment and cultured according to the protocol by Global Salm-Surv (16). Initially, 0.2 grams of feces was enriched in Preston broth and incubated at 38° C overnight under microaerophilic conditions generated using CampyGen Gas Generation Sachets (Oxoid, Thermo Fischer Scientific, Basingstoke, Hampshire, United Kingdom). After 24 h, 10 μL of each of the incubated samples was streaked onto modified Charcoal Cefoperazone Deoxycholate agar (mCCDA) (Oxoid, Thermo Fischer Scientific, Basingstoke, Hampshire, United Kingdom) plates and incubated at 38° C for 48 h under microaerophilic conditions. Plates were examined for presence of presumptive Campylobacter colonies by morphological characteristics (17). A suspect colony was Gram-stained and viewed under a light microscope at x100 magnification lens under oil emersion (16). Gram negative curved rods, some forming s-shapes, were sub-cultured onto a Columbia blood agar plate and incubated under micro-aerophilic conditions for 24 h at 38° C. Further characterization of the isolates was done by catalase and oxidase tests (16). Differentiation between C. jejuni and C. coli was done using the hippurate hydrolysis test.
DNA was extracted from suspected Campylobacter colonies grown on 5% sheep blood agar for 24 to 48 h at 38°C under microaerophilic conditions by boiling (18). Suspect isolates were confirmed to be of Campylobacter genus and C. jejuni or C. coli species by PCR (18, 19). The primer sequences and the respective PCR conditions are outlined in Supplementary Table S1.
2.5. Ethical approvals and participant consent
Before data collection, ethical approvals were sought from the London School of Hygiene and Tropical Medicine (LSHTM) Observational / Interventions Research Ethics Committee Reference 17,188 and the International Livestock Research Institute—Institutional Research Ethics Committee (ILRI-IREC) with project reference ILRI-IREC2019-26. ILRI-IREC is accredited by the National Commission for Science, Technology and Innovation (NACOSTI) in Kenya. Project and individual student approval was also acquired from NACOSTI. The study was conducted in accordance with the Declaration of Helsinki and each caregiver gave signed informed consent. Samples were collected according to the institutional guidelines and in accordance with the approved protocol.
2.6. Data cleaning
The data was assessed visually and programmatically to identify any quality and tidiness issues. Variables with too many categories were regrouped for ease of analyses. Missing values were assessed and addressed. Using R version 4.2.1, each household metadata was linked to corresponding samples and laboratory results inconsistences were discussed between the field and laboratory team to clean the dataset.
2.7. Data analysis
Descriptive summary statistics were calculated to describe prevalence and general household characteristics. A two-step statistical logistic regression analysis, using univariable and a backward stepwise multivariable approach, was performed to identify risk factors associated with the occurrence of Campylobacter in children. The lme4 package in R statistical software (version 4.2.2) was used to build multilevel logistic regression models (20). The CHV was used as a random effect to account for clustering in the model regarding risk factors for Campylobacter in children. For univariable analysis, all variables with a p-value lower than 0.2 were retained for assessment in the multivariable analyses. For multivariable analysis, a backward stepwise approach was utilized to select the final logistic regression model using the variables identified from the univariable analysis to identify risk factors responsible for the occurrence of Campylobacter in children. The CHU was included as a fixed effect to account for any variation between units. Odds ratio (OR) and 95% confidence intervals (CI) were calculated and p-values less than 0.05 were considered statistically significant. Two-way interactions between predictors were assessed using a likelihood ratio test and considered significant if p ≤ 0.05. To evaluate for collinearity effect between predictors, the level of association between risk factors identified during the univariable analysis were assessed using a Fisher’s test, and risk factors with more than two-fold changes in the logistic regression coefficients were also checked during the selection process.
The final model was considered valid if the residuals plot versus fitted values plot for each fixed effect showed no clear clustering patterns nor outliers, and deviations from the empirical and expected quantile distribution were not significant (p-value > 0.05) using the simulateResiduals function from the DHARMa package (21). A Q-Q plot was visualised to detect deviations from the expected distribution which included goodness-of-fit tests such as tests for correct distribution, overdispersion and outliers. Variance Inflation Factors (VIFS) were calculated to check for collinearity. VIFS >4 were considered a problem and the variable removed from the model.
3. Results
3.1. Descriptive statistics of the household characteristics
We recruited 585 households into the study from May to October 2021. The mean household size was four people (range 2–14). Most people lived in a rented house (463/585, 79.1%), used electricity as a source of lighting (583/585, 99.6%), and the houses had cemented floors (564/585, 96.4%). The preparation of child food was predominantly done inside the house (578/585, 98.8%) and preparation surface was always cleaned beforehand. More than half of the households did not have a hand washing facility (321/585, 54.9%). Majority of the recruited households sourced their drinking (513/585, 87.7%) and cooking water (505/585, 86.3%) from basic sources according to WHO/UNICEF Joint Monitoring programme (JPM). Only half of the households indicated treating drinking water (277/585, 47.4%) before use. Treatment of cooking water was not a common practice in the households (77/585, 13.2%). Sanitation facilities were mainly basic (354/585, 60.5%), limited (117/585, 20%) and unimproved (74/585, 12.6%).
Almost all caregivers had at least primary school education (581/585, 99.3%) and most had secondary education (428/585, 73.3%). Household income was mostly non-permanent with casual labour (270/585, 46.2%) or petty trading (164/585, 28.4%) being the most common sources of income. Of the recruited households, 167/585 (28.5%) indicated they owned animals and 436/585 (74.5%) indicated to be in contact with animals in the compound.
We recruited 585 children aged 6–24 months into the study. The children were equally divided between male (n = 293) and female (n = 292). The number of participants in each age category was also evenly distributed (6–11 months n = 187; 12–17 months n = 204; 18–23 months n = 194). Almost one fifth of children were reported to have had diarrhea in the past week (including the day of sampling prior to the interview) (113/585, 19.3%).
3.2. Campylobacter prevalence
Stool samples were collected from 540/585 children. Caregivers of the 45 children who did not give a sample either refused or were unable to provide a sample. Campylobacter was detected in 26/540 stool samples giving a prevalence of 4.8% (95% CI 3.3 – 7.0%). C. jejuni (n = 21) was the most frequently isolated and accounted for 80.8% of positive samples compared to C. coli which was detected in 7 (26.9%) of positive samples. Six children had co-carriage with C. jejuni and C. coli at a prevalence of 1.1% (95% CI 0.5 – 2.4%) or 6/26 (23.1%) positive samples. There were 4 isolates 0.7% (95% CI 0.3 – 1.9%) that were Campylobacter species, but were not identified as belonging to either C. jejuni or C. coli, which accounted for 15.4% of positive samples (Table 1).

Table 1. Prevalence of Campylobacter species detected in children aged 6–24 months in Dagoretti South Subcounty, Nairobi.
3.3. Risk factor analysis for Campylobacter carriage in children
The univariable analysis for risk factors for Campylobacter occurrence in children included age, gender, livestock ownership, contact with livestock, food consumed, and source of water (Table 2). Chicken ownership (OR 3.0, 95% CI 1.3 – 7.1), goat ownership (OR 5.4, 95%, 1.2 – 24.3), drinking cow’s milk (OR 3.9, 95% CI 1.3 – 11.8) and presence of animal feces in the compound (OR 4.4, 95% CI 1.4 – 14.3) were significantly associated with Campylobacter carriage in children. There was also a significant difference in Campylobacter in children between CHUs, with children from Ruthimitu more likely to be positive (OR 7.1 95% CI 1.6 – 30.8). There was no association between Campylobacter prevalence and a recent episode of diarrhea (within the previous 7 days including the day of sampling) (OR 0.8, 95% CI 0.3 – 2.5) (Supplementary Table S2).
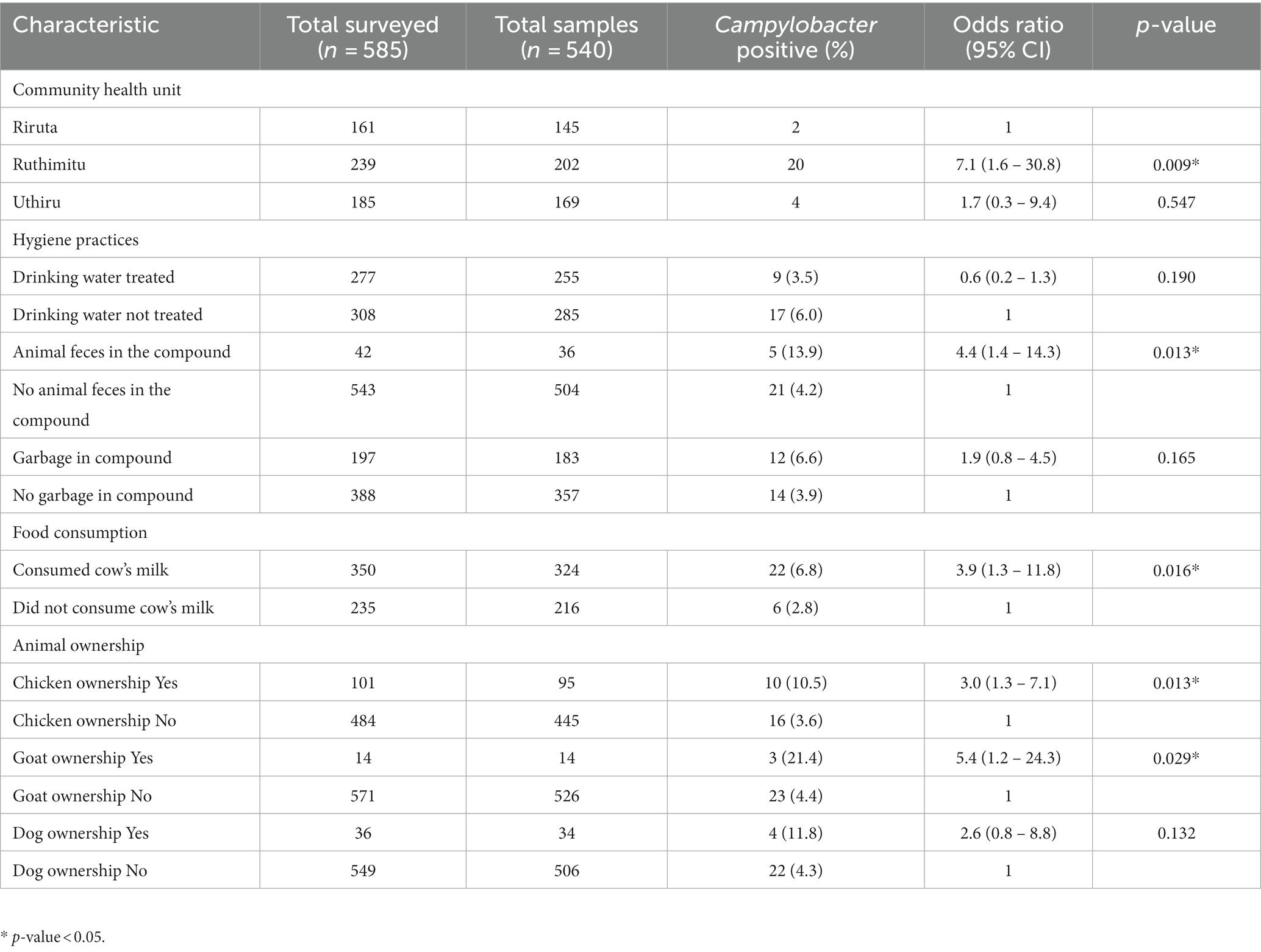
Table 2. Results of univariable analysis of variables measured for association with Campylobacter positivity in children from Dagoretti South Subcounty, Nairobi and considered for inclusion in the multivariable model.
3.4. Multivariable mixed effects logistic regression model for Campylobacter carriage
The results of the final multivariable mixed effect logistic regression model are presented in Table 3 with the backwards stepwise approach presented in Table 4. In the final model, we included two effects (drinking milk, and animal feces in the compound), CHU was included as a fixed effect to account for any variation between units, and a random effect (CHV) was incorporated for the dependency among observations.

Table 3. Results of multivariable analysis for Campylobacter carriage in children from Dagoretti South Subcounty, Nairobi.
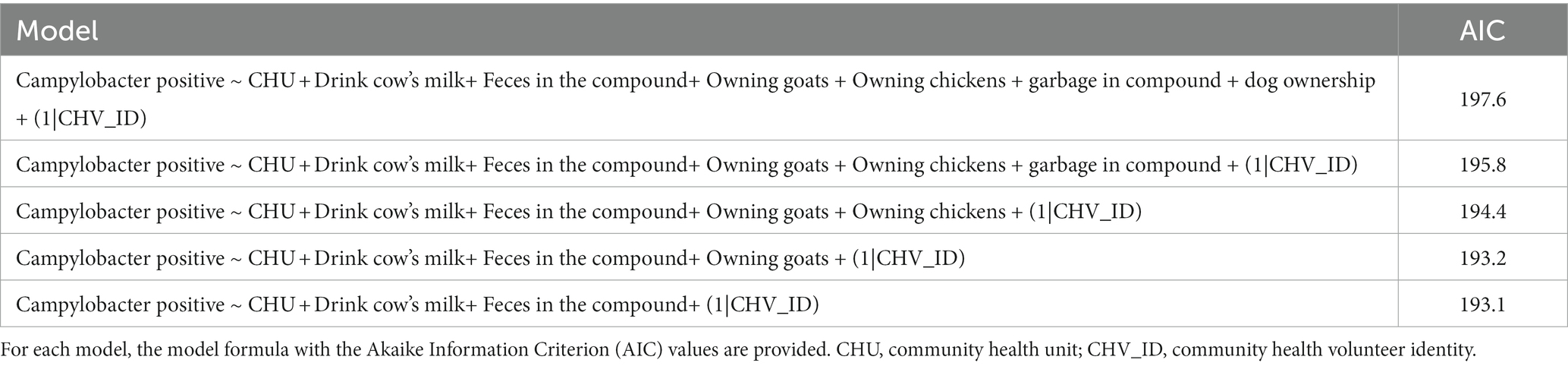
Table 4. Comparison of mixed-effects logistic regression risk factor models for Campylobacter carriage in children.
The final model had the lowest Akaike Information Criterion (AIC) of 193.1. Model validation showed no obvious clustering patterns of simulated residuals nor over dispersion, zero-inflation and outliers tests were not significant (p-value > 0.05).
Risk factors identified in the final model to be significantly associated with Campylobacter carriage in children included: drinking cows milk (OR 4.2, 95% CI 1.4–12.6), and presence of animal feces in the compound (OR 3.4, 95% CI 1.1 – 10.3) (Table 3).
4. Discussion
This study determined the prevalence of Campylobacter in children and identified risk factors associated with Campylobacter carriage in children in Dagoretti South, Kenya. The prevalence of Campylobacter carriage was 4.8%. The prevalence among these children is similar to what has been reported in other African countries. In children below 5 years old, Campylobacter prevalence has been shown to range between 2% in Sudan and 21% in South Africa (22). The majority of Campylobacter positive children were asymptomatic (76.9%). This is consistent with a recent case control study in children under 5 years in Malawi which showed no difference in Campylobacter prevalence in children with or without diarrhea (23). In contrast, a study conducted in Morogoro, Tanzania, indicated a higher prevalence of Campylobacter in symptomatic (12.9%) compared to asymptomatic individuals (6.7%) (24). In South Africa, a similar finding was reported where the prevalence of Campylobacter was higher among diarrheal stool samples (20.4%) compared to non-diarrheal stool samples (12.4%) (5). Asymptomatic occurrence of Campylobacter could be a consequence of a resolved infection since the bacteria may be excreted in the feces up to 12 to 40 weeks after infection (25). Immunity may develop from continued exposure to low doses due to poor hygienic/sanitary conditions and contact with reservoir animals such as chickens (25).
C. jejuni was the most common species (80.8%) followed by C. coli (26.9%) in our study. Previous studies have identified C. jejuni as the leading bacterial cause of food-borne diseases in both high income and lower and middle income countries, causing human bacterial gastroenteritis (5, 24). Our results are similar to another study conducted in Western Kenya, where C. jejuni in children was identified in 89.2% and C. coli in 10.8% of isolates (8), and consistent with a recent global review which showed similar findings with respect to C. jejuni and C. coli prevalence (26).
Our study detected co-carriage of C. jejuni and C. coli in 23.1% of the positive samples. Coinfections were mainly detected among samples which showed swarming growth characteristics during culture, and it was therefore difficult to pick a single colony for subculturing. Co-infections have been described in other studies (27). Co-occurrence of four Campylobacter species in stool from asymptomatic children aged below 2 years was also recorded in children from Ethiopia (28). The co-occurrence suggests that Campylobacter colonization of children occurred through multiple reservoirs or from a reservoir in which several Campylobacter species co-inhabited.
Other uncharacterized species of Campylobacter were identified in 15.4% of isolates. Occurrence of other Campylobacter species including: C. upsaliensis, C. hyointestinalis, C. lari, and C. fetus have been identified from children and animal stool (28, 29). A study involving asymptomatic school going children in Kibera, Kenya, also identified C. lari in 1.9% of children (30).
Campylobacter organisms were initially identified using culture methods and then confirmed using genus and species level primers (18). This process was laborious and due to the nature of the testing algorithm mixed infections with other unidentified Campylobacter species might not have been detected. This was particularly true for samples that were positive for C. jejuni and C. coli as these were not further screened for other species, and this may have underestimated the carriage of additional Campylobacter organisms.
Direct PCR on fecal material without enrichment has been described with comparable sensitivity and specificity to conventional culture techniques (31). However, this earlier work was done on clinical samples where the number of organisms was likely higher, and this approach may not have been effective in our population which was mostly asymptomatic (32). In future the use of multiplex PCR methods may reduce the time required to screen samples for multiple pathogens without impacting sensitivity or specificity (33, 34).
Risk factors identified in the multivariable model to be significantly associated with Campylobacter carriage in children included: drinking cow’s milk (OR 4.2, 95% CI 1.4–12.6), and presence of animal feces in the compound (OR 3.4, 95% CI 1.1-10.3). In previous studies, consumption of raw milk was linked with Campylobacter outbreaks (8, 35). All milk types available in Kenya, unpackaged, fresh pasteurized, and ultra-high temperature (UHT), may be contaminated and bacterial contamination of milk can occur from the point of purchase to consumption (36). Interventions for improving food hygiene and to reduce contamination of complementary foods for weaning children may target caregiver practices such as boiling milk, treating water, handwashing with soap, and food storage practices (37). However these practices are likely to be determined by the social and economic status of the caregiver so recommendations need to be context specific (37–39).
There are economic, nutritional and sociocultural reasons for keeping animals in peri-urban areas and animals are commonly kept within the living areas (40). In the households that were visited, animals were housed in structures attached to the main house and were free to roam during the day and only housed during the night. The free-range farming, especially of chickens, coupled with low biosecurity and poor hygiene contributed to the presence of animal feces in the compound. Children were noted to be playing in the same compound therefore increasing contact with the feces. Animal ownership and animal waste in the compound is associated with contamination of household drinking water (41). Campylobacter organisms are frequently isolated from animal feces, especially livestock (poultry, cattle, sheep, goat, and swine) and pets (3). Shedding of Campylobacter organisms by livestock into water sources, environment, and food can cause infection in children through poor personal hygiene practices such as not washing hands regularly, unhygienic food handling practices and poor water hygiene practices (35). Interventions to reduce zoonotic disease transmission in periurban areas should focus on sanitation and biosecurity measures, free-range farming should be discouraged, animals should not be in the same house as humans, and manure should not be used as fuel.
5. Conclusion
This study provides evidence of asymptomatic and symptomatic Campylobacter infections in children, with C. jejuni being the most common species. In future studies, it would be important to understand the incidence and maintenance of Campylobacter infection in this age group and to understand the impact on malnutrition particularly in settings with food and nutritional insecurity. Future work may also consider direct molecular approaches for detection of pathogens to reduce time and resources spent in the laboratory and generate results in real-time.
Drinking cow’s milk and presence of animal fecal material within the compound of the household were associated with carriage of Campylobacter in children. As well as the potential transmission of Campylobacter from animals to children, the occurrence of Campylobacter in asymptomatic children may pose a threat to public health as the organisms are continuously shed in feces which can promote spread of the disease to other humans and or animals. Further research work using genotypic methods for determination of relatedness of the isolates circulating in animals, humans and the environment is required for source attribution of the isolates in order to target intervention measures to the most incriminated source.
A “One Health” approach is recommended where multisectoral collaborative efforts from veterinary, human medicine, and environmental managers is required to design efficient and effective strategies for prevention and control of infections both in human and animal populations and reduce contamination of food and the environment.
Data availability statement
The raw data supporting the conclusions of this article will be made available by the authors, without undue reservation.
Ethics statement
The studies involving humans were approved by the London School of Hygiene and Tropical Medicine (LSHTM) Observational/Interventions Research Ethics Committee Reference 17188 and the International Livestock Research Institute—Institutional Research Ethics Committee (ILRI- IREC) with project reference ILRI-IREC2019-26. ILRI-IREC is accredited by the National Commission for Science, Technology and Innovation (NACOSTI) in Kenya. The studies were conducted in accordance with the local legislation and institutional requirements. Written informed consent for participation in this study was provided by the participants’ legal guardians/next of kin.
Author contributions
AK and EC: concept, methodology, data collection, analysis, and manuscript preparation. LB and GG: supervision. LO and MA: methodology and analysis. NO: data collection and analysis. CM and JW: analysis. SM: data collection. AM-T: supervision and funding. JW: concept and methodology. OC: concept, methodology, and funding. All authors contributed to the article and approved the submitted version.
Funding
The project Development of a comprehensive intervention to address foodborne enteric disease risks among young children living in low income informal neighbourhoods of Maputo and Nairobi was supported by the Bill & Melinda Gates Foundation (BMGF) and the Foreign, Commonwealth and Development Office (FCDO) of the UK Government (INV-008449). This research was also conducted as part of the CGIAR Initiative on One Health (Protecting human health through a One Health approach) and is supported by contributors to the CGIAR Trust Fund (https://www.cgiar.org/funders).
Acknowledgments
Thank you to the participants for their time, the county officials for their support, community health workers, and nurse enumerators. Particularly we would like to thank Catherine Mugo, Esther Gichache, Charity Sayo, Nicholas Ireri, John Ng’ang’a, Collins Mbuvi, Jean Amour, Mark Irumva, Immaculate Nyakabi, Hellen Omina, and Cynthia Mukanda.
Conflict of interest
The author EAJC declared that they were an editorial board member of Frontiers, at the time of submission. This had no impact on the peer review process and the final decision.
The remaining authors declare that the research was conducted in the absence of any commercial or financial relationships that could be construed as a potential conflict of interest.
Publisher’s note
All claims expressed in this article are solely those of the authors and do not necessarily represent those of their affiliated organizations, or those of the publisher, the editors and the reviewers. Any product that may be evaluated in this article, or claim that may be made by its manufacturer, is not guaranteed or endorsed by the publisher.
Supplementary material
The Supplementary material for this article can be found online at: https://www.frontiersin.org/articles/10.3389/fpubh.2023.1147180/full#supplementary-material
References
1. Coker, AO, Isokpehi, RD, Thomas, BN, Amisu, KO, and Obi, CL. Human campylobacteriosis in developing countries. Emerg Infect Dis. (2002) 8:237–43. doi: 10.3201/eid0803.010233
2. Platts-Mills, JA, and Kosek, M. Update on the burden of campylobacter in developing countries. Curr Opin Infect Dis. (2014) 27:444–50. doi: 10.1097/QCO.0000000000000091
3. Gahamanyi, N, Mboera, LEGG, Matee, MI, Mutangana, D, and Komba, EVGG. Prevalence, risk factors, and antimicrobial resistance profiles of Thermophilic campylobacter species in humans and animals in sub-Saharan Africa: a systematic review. Int J Microbiol. (2020) 2020:1–12. doi: 10.1155/2020/2092478
4. Kirk, MD, Pires, SM, Black, RE, Caipo, M, Crump, JA, Devleesschauwer, B, et al. World health organization estimates of the global and regional disease burden of 22 foodborne bacterial, protozoal, and viral diseases, 2010: a data synthesis. PLoS Med. (2015) 12:e1001940. doi: 10.1371/journal.pmed.1001940
5. Samie, A, Moropeng, RC, Tanih, NF, Dillingham, R, Guerrant, R, and Bessong, PO. Epidemiology of campylobacter infections among children of 0–24 months of age in South Africa. Arch Public Health. (2022) 80:107. doi: 10.1186/s13690-022-00850-1
6. Igwaran, A, and Okoh, AI. Human campylobacteriosis: a public health concern of global importance. Heliyon. (2019) 5:e02814. doi: 10.1016/j.heliyon.2019.e02814
7. Liu, J, Platts-Mills, JA, Juma, J, Kabir, F, Nkeze, J, Okoi, C, et al. Use of quantitative molecular diagnostic methods to identify causes of diarrhoea in children: a reanalysis of the GEMS case-control study. Lancet. (2016) 388:1291–301. doi: 10.1016/S0140-6736(16)31529-X
8. Ouko, TT, Nyerere, AK, Njeru, JM, Fèvre, EM, and Kariuki, S. Prevalence and risk factors associated with campylobacter infection in diarrheal patients in Busia County, Kenya. Adv Microbiol. (2021) 11:657–80. doi: 10.4236/aim.2021.1111048
9. Amour, C, Gratz, J, Mduma, ER, Svensen, E, Rogawski, ET, McGrath, M, et al. Epidemiology and impact of campylobacter infection in children in 8 low-resource settings: results from the MAL-ED study. Clin Infect Dis. (2016) 63:1171–9. doi: 10.1093/cid/ciw542
10. Mageto, L. Prevalence and risk factors for campylobacter infection of chicken in peri-urban areas of Nairobi, Kenya. J Dairy Vet Anim Res. (2018) 7:22–7. doi: 10.15406/jdvar.2018.07.00184
11. Altekruse, SF, Stern, NJ, Fields, PI, and Swerdlow, DL. Campylobacter jejuni – an emerging foodborne pathogen. Emerg Infect Dis. (1999) 5:28–35. doi: 10.3201/eid0501.990104
12. Osbjer, K, Boqvist, S, Sokerya, S, Chheng, K, San, S, Davun, H, et al. Risk factors associated with campylobacter campylobacter by PCR in humans and animals in rural Cambodia. Epidemiol Infect. (2016) 144:2979–88. doi: 10.1017/S095026881600114X
13. KNBS. 2019 Kenya population and housing census. Volume I population by county and subcounty. Nairobi: (2019) Available at: https://www.knbs.or.ke/publications/.
14. Dominguez-Salas, P, Alarcón, P, Häsler, B, Dohoo, IR, Colverson, K, Kimani-Murage, EW, et al. Nutritional characterisation of low-income households of Nairobi: socioeconomic, livestock and gender considerations and predictors of malnutrition from a cross-sectional survey. BMC Nutr. (2016) 2:1–20. doi: 10.1186/s40795-016-0086-2
15. UNICEF, WHO. (2022). Progress on drinking water, sanitation and hygiene in schools: 2000–2021 data update. New York. Available at: https://data.unicef.org/resources/jmp-wash-in-schools-2022/#:~:text=TheWHO%2FUNICEF Joint Monitoring, SDG) targets related to WASH.
16. Hendriksen, RS, Wagenaar, J, and van Bergen, M. (2003). Laboratory protocols level 2 training course isolation of thermotolerant campylobacter from faeces. Global Salm-Surv. Available at: chrome-extension://efaidnbmnnnibpcajpcglclefindmkaj/https://www.uu.nl/sites/default/files/Campylobacter isolation from faeces.pdf (Accessed Dec 7, 2022).
17. Hong, J, Woo, KJ, Jun, MK, So, HK, Hye, CK, Ser, J, et al. Quantification and differentiation of campylobacter jejuni and Campylobacter coli in raw chicken meats using a real-time PCR method. J Food Prot. (2007) 70:2015–22. doi: 10.4315/0362-028X-70.9.2015
18. Adiguzel, MC, Sigirci, BD, Celik, B, Kahraman, BB, Metiner, K, Ikiz, S, et al. Phenotypic and genotypic examination of antimicrobial resistance in Thermophilic campylobacter species isolated from poultry in Turkey. J Vet Res. (2018) 62:463–8. doi: 10.2478/jvetres-2018-0071
19. Müller, W, Böhland, C, and Methner, U. Detection and genotypic differentiation of campylobacter jejuni and Campylobacter coli strains from laying hens by multiplex PCR and fla-typing. Res Vet Sci. (2011) 91:e48–52. doi: 10.1016/j.rvsc.2011.01.028
20. Bates, D, Maechler, M, Bolher, B, and Walker, S. (2015). lme4: Linear mixed-effects models using Eigen and S4. R package version 1.1-10.
21. Hartig, F. (2022) DHARMa: Residual diagnostics for hierarchical (multi-level/mixed) regression models. Available at: https://cran.r-project.org/package=DHARMa (Accessed Dec 1, 2022).
22. Asuming-Bediako, N, Kunadu, APH, Abraham, S, and Habib, I. Campylobacter at the human–food interface: the African perspective. Pathogens. (2019) 8:87. doi: 10.3390/pathogens8020087
23. Iturriza-Gómara, M, Jere, KC, Hungerford, D, Bar-Zeev, N, Shioda, K, Kanjerwa, O, et al. Etiology of diarrhea among hospitalized children in Blantyre, Malawi, following rotavirus vaccine introduction: a case-control study. J Infect Dis. (2019) 220:213–8. doi: 10.1093/infdis/jiz084
24. Komba, EVG, Mdegela, RH, Msoffe, PLM, Nielsen, LN, and Ingmer, H. Prevalence, antimicrobial resistance risk factors for Thermophilic campylobacter infections in symptomatic asymptomatic humans in Tanzania. Zoonoses Public Health. (2015) 62:557–68. doi: 10.1111/zph.12185
25. Hlashwayo, DF, Sigaúque, B, Noormahomed, EV, Afonso, SMS, Mandomando, IM, and Bila, CG. A systematic review and meta-analysis reveal that campylobacter spp. and antibiotic resistance are widespread in humans in sub-Saharan Africa. PLoS One. (2021) 16:e0245951. doi: 10.1371/journal.pone.0245951
26. Liu, F, Lee, SA, Xue, J, Riordan, SM, and Zhang, L. Global epidemiology of campylobacteriosis and the impact of COVID-19. Front Cell Infect Microbiol. (2022) 12:979055. doi: 10.3389/fcimb.2022.979055
27. Abbasi, E, and Ghaznavi-Rad, E. A report of two clinical coinfections with campylobacter jejuni and Campylobacter coli in infantile dysentery. Arch Pediatr Infect Dis. (2019) 7:e80116. doi: 10.5812/pedinfect.80116
28. Terefe, Y, Deblais, L, Ghanem, M, Helmy, YA, Mummed, B, Chen, D, et al. Co-occurrence of campylobacter species in children from eastern Ethiopia, and their association with environmental enteric dysfunction, diarrhea, and host microbiome. Front Public Health. (2020) 8:99. doi: 10.3389/fpubh.2020.00099
29. Chala, G, Eguale, T, Abunna, F, Asrat, D, and Stringer, A. Identification and characterization of campylobacter species in livestock, humans, and water in livestock owning households of peri-urban Addis Ababa, Ethiopia: a one health approach. Front Public Health. (2021) 9:750551. doi: 10.3389/fpubh.2021.750551
30. Gitahi, N, Gathura, P, Gicheru, M, Wandia, B, and Nordin, A. Multidrug-resistant Campylobacter jejuni, campylobacter coli and Campylobacter lari isolated from asymptomatic school-going children in Kibera slum, Kenya. F1000Res. (2020) 9:92. doi: 10.12688/f1000research.21299.2
31. Lawson, AJ, Shafi, MS, Pathak, K, and Stanley, J. Detection of campylobacter in gastroenteritis: comparison of direct PCR assay of faecal samples with selective culture. Epidemiol Infect. (1998) 121:547–53. doi: 10.1017/S0950268898001630
32. Barletta, F, Mercado, EH, Lluque, A, Ruiz, J, Cleary, TG, and Ochoa, TJ. Multiplex real-time PCR for detection of campylobacter, salmonella, and Shigella. J Clin Microbiol. (2013) 51:2822–9. doi: 10.1128/JCM.01397-13
33. Al Amri, A, Senok, AC, Ismaeel, AY, Al-Mahmeed, AE, and Botta, GA. Multiplex PCR for direct identification of campylobacter spp. in human and chicken stools. J Med Microbiol. (2007) 56:1350–5. doi: 10.1099/jmm.0.47220-0
34. Wiemer, D, Loderstaedt, U, von Wulffen, H, Priesnitz, S, Fischer, M, Tannich, E, et al. Real-time multiplex PCR for simultaneous detection of Campylobacter jejuni, salmonella, Shigella and Yersinia species in fecal samples. Int J Med Microbiol. (2011) 301:577–84. doi: 10.1016/j.ijmm.2011.06.001
35. Behailu, Y, Hussen, S, Alemayehu, T, Mengistu, M, and Fenta, DA. Prevalence, determinants, and antimicrobial susceptibility patterns of campylobacter infection among under-five children with diarrhea at governmental hospitals in Hawassa city, Sidama, Ethiopia. A cross-sectional study. PLoS One. (2022) 17:1–19. doi: 10.1371/journal.pone.0266976
36. Hoffmann, V, Simiyu, S, Sewell, DK, Tsai, K, Cumming, O, Mumma, J, et al. Milk product safety and household food hygiene influence bacterial contamination of infant food in peri-urban Kenya. Front Public Health. (2022) 9:772892. doi: 10.3389/fpubh.2021.772892
37. Simiyu, S, Czerniewska, A, Aseyo, ER, Baker, KK, Cumming, O, Mumma, JAO, et al. Designing a food hygiene intervention in low-income, peri-urban context of Kisumu, Kenya: application of the trials of improved practices methodology. Am J Trop Med Hyg. (2020) 102:1116–23. doi: 10.4269/ajtmh.19-0629
38. Mumma, JAO, Cumming, O, Simiyu, S, Czerniewska, A, Aseyo, RE, Muganda, DN, et al. Infant food hygiene and childcare practices in context: findings from an urban informal settlement in Kenya. Am J Trop Med Hyg. (2020) 102:220–2. doi: 10.4269/ajtmh.19-0279
39. Simiyu, S, Aseyo, E, Anderson, J, Cumming, O, Baker, KK, Dreibelbis, R, et al. A mixed methods process evaluation of a food hygiene intervention in low-income informal neighbourhoods of Kisumu, Kenya. Matern Child Health J. (2022) 27:824–36. doi: 10.1007/s10995-022-03548-6
40. Barnes, AN, Mumma, J, and Cumming, O. Role, ownership and presence of domestic animals in peri-urban households of Kisumu, Kenya. Zoonoses Public Health. (2018) 65:202–14. doi: 10.1111/zph.12429
Keywords: children, infant, Campylobacter, diarrhea, LMIC, zoonoses, food safety
Citation: Kiarie A, Bebora L, Gitao G, Ochien’g L, Okumu N, Mutisya C, Wasonga J, Masudi SP, Moodley A, Amon-Tanoh MA, Watson J, Cumming O and Cook EAJ (2023) Prevalence and risk factors associated with the occurrence of Campylobacter sp. in children aged 6–24 months in peri-urban Nairobi, Kenya. Front. Public Health. 11:1147180. doi: 10.3389/fpubh.2023.1147180
Edited by:
Teresa Estrada-Garcia, National Polytechnic Institute of Mexico (CINVESTAV), MexicoReviewed by:
Maribel Denise Riveros, Universidad Peruana Cayetano Heredia, PeruFang Liu, University of New South Wales, Australia
Copyright © 2023 Kiarie, Bebora, Gitao, Ochien’g, Okumu, Mutisya, Wasonga, Masudi, Moodley, Amon-Tanoh, Watson, Cumming and Cook. This is an open-access article distributed under the terms of the Creative Commons Attribution License (CC BY). The use, distribution or reproduction in other forums is permitted, provided the original author(s) and the copyright owner(s) are credited and that the original publication in this journal is cited, in accordance with accepted academic practice. No use, distribution or reproduction is permitted which does not comply with these terms.
*Correspondence: Alice Kiarie, njokikiarie1@gmail.com; Elizabeth A. J. Cook, E.cook@cgiar.org