- 1Department of Public Health and Preventive Medicine, School of Medicine, Jinan University, Guangzhou, Guangdong, China
- 2Guangdong Provincial Engineering and Technology Research Center for Tobacco Breeding and Comprehensive Utilization, Key Laboratory of Crop Genetic Improvement of Guangdong Province, Crops Research Institute, Guangdong Academy of Agricultural Sciences, Guangzhou, Guangdong, China
- 3Reproductive Medicine Center, Guangdong Provincial Key Laboratory of Reproductive Medicine, The First Affiliated Hospital, Sun Yat-sen University, Guangzhou, China
Purpose: Exposure to perfluoroalkyl and polyfluoroalkyl substances causes oxidative stress, which is strongly associated with adverse health effects. Klotho protein plays an anti-aging role via antioxidation activity.
Methods: We investigated the levels of serum α-Klotho and PFAS exposure in adults who participated in the National Health and Nutrition Examination Survey from 2013 to 2016. A nationally representative subsample of 1,499 adults aged 40–79 years was analyzed for the associations of serum α-Klotho levels with serum PFAS exposures by correlation analysis and multiple general linear models. Of note, the potential confounding factors including age and gender were adjusted. Quantile-based g-computation models were used to assess the effects of mixed PFAS exposure on serum α-Klotho levels.
Results: The weighted geometric mean of serum α-Klotho was 791.38 pg/mL for the subjects during 2013–2016. After adjusting for potential confounders, serum Klotho levels showed a statistically significant downward trend with increasing quartiles of PFOA and PFNA. Multivariate adjusted general linear regression analysis showed that increased exposure to PFNA was substantially associated with lower serum levels of α-Klotho, and each 1-unit increase in PFNA concentration was accompanied by a 20.23 pg/mL decrease in α-Klotho level; while no significant association was observed between other PFAS exposures and serum α-Klotho levels. It was negatively correlated between α-Klotho and Q4 for PFNA relative to the lowest quartile (Q1) of exposure (P = 0.025). It was found that the strongest negative correlation between PFNA exposure and serum α-Klotho levels was in the middle-aged (40–59 years) female participants. Furthermore, the mixture of the four PFAS substances showed an overall inverse association with serum α-Klotho concentrations, with PFNA being the major contributor.
Conclusions: Taken together, in a representative sample of the U.S. middle-aged and elderly populations, serum concentrations of PFAS, especially PFNA, have been negatively associated with serum levels of α-Klotho, which is strongly associated with cognition and aging. It was important to note that the majority of associations were limited to middle-aged women. It will be meaningful to clarify the causal relationship and the pathogenic mechanisms of PFAS exposure and α-Klotho levels, which is helpful to aging and aging-related diseases.
1. Introduction
Perfluoroalkyl and polyfluoroalkyl substances (PFAS) are a critical group of persistent contaminants, has been used for decades in industrial and consumer applications mainly including protective coatings for carpets and clothing, water repellents, paper coatings, and surfactants (1). Their widespread use means that they are widely present in nature. PFAS-contaminated water and food, as well as inhalation of PFAS in an indoor setting, were the most common unavoidable exposure ways (2). Despite worldwide efforts were performed to control and reduce the use of PFAS, they are still detected in humans, wild animals, and various environmental matrices due to their ubiquitous application and stable carbon-fluorine bonds. Air pollution and cigarette smoke also contain high concentrations of PFOS. PFAS are environmental endocrine disruptors that can lead to serious human health problems including obesity, liver damage, kidney cancer, hypertension, immunotoxicity, etc. (3, 4). Specifically, it may induce cytotoxic effects through mechanisms such as increased oxidative stress (5–7), induction of apoptosis-related mitochondrial dysfunction (8, 9), and inhibition of gap junction intercellular communication (10). Some PFAS have the possibility of causing oxidative stress in human hepatocytes in terms of DNA damage and the production of reactive oxygen species (ROS), with genotoxic and cytotoxic potential (11). In addition, PFAS exposure is strongly associated with increased renal oxidative stress, and that a key pathway involving oxidative stress might be through disruptive effects on the peroxisome proliferator-activated receptor (PPAR) and its downstream functions (6). Several population studies have shown that serum PFAS concentrations are strongly associated with biomarkers of oxidative and nitrative stress. A general population cohort of Taiwan demonstrated that serum PFAS concentrations increased the levels of 8-nitroguanine and 8-hydroxy-2-deoxyguanosine (8-OHdG) (12). Similarly, 141 senior Koreans in a randomized controlled trial with elevated serum levels of perfluorododecanoic acid (PFDoDA) and PFOS concentrations also had higher levels of malondialdehyde and 8-OHdG in urine (13). In vitro and in vivo experiments, as well as population studies, have suggested that exposure to certain PFAS is associated with increased oxidative stress.
Klotho, initially considered to be a recognized suppressor gene of aging, has aroused great interest and enabled us to better understand the aging process (14). Klotho plays an important role as an anti-aging protein in the pathophysiology of multiple aging-related diseases, including atherosclerosis, diabetes, chronic kidney disease, neurological disorders, and cancers (15, 16). Klotho is a single transmembrane anti-aging protein in the form of α-Klotho, β-Klotho, and γ-Klotho, with different organ-specific expression and functions in vivo (17). Among these isoforms, α-Klotho, a transmembrane co-receptor for fibroblast growth factors 23 (FGF23) and a soluble factor in serum, is predominantly expressed in the kidney and brain, associated with phosphate metabolism, calcium uptake, energy metabolism, and various aging phenotypes (15, 18, 19).
We noticed that α-Klotho can inhibit oxidative stress. The α-Klotho stimulation increases the phosphorylation of forkhead box protein O3a (FOXO3a), inhibiting ROS-related oxidative stress. Transgenic mice overexpressing α-Klotho exhibited higher manganese superoxide dismutase (MnSOD) expression and lower oxidative stress, whereas α-Klotho deficiency significantly increased endogenous ROS production and oxidative stress aggravation (20, 21). Furthermore, overexpression of α-Klotho decreased H2O2-induced apoptosis, mitochondrial DNA breakage, β-galactosidase activity, superoxide anion production, and Bax protein expression (22). Low levels of ROS-associated stress can prolong cellular lifespan (23), and the anti-aging mechanism of α-Klotho may be related to ROS and its downstream signaling pathways.
PFAS may be pro-oxidants and alter oxidative stress levels. A recent study of NHANES data considering the critical role of renal failure first showed a modest association between PFAS exposure and low serum α-Klotho in healthy kidneys (24). In our current study, we again investigated the relationship between serum PFAS and serum α-Klotho levels in adults who participated in NHANES 2013–2016, in which adjustments were also made for potential confounders, and age- and sex-specific analyses were further conducted. Furthermore, we assessed the effects of mixed PFAS exposure on serum α-Klotho levels to understand their combined risk better. The relationship between PFAS and serum α-Klotho may remind us to prevent disorders associated with PFAS and serum α-Klotho, and α-Klotho may be a preventative and therapeutic target for its related diseases after exposure to PFAS.
2. Materials and methods
2.1. Study population
NHANES is conducted by the National Center for Health Statistics (NCHS) of the Centers for Disease Control and Prevention (CDC) as a major program to assess the health and nutritional status of the adults and children in the United States, with a deliberately sampled population intended to be representative of the US population by age, ethnicity, and other demographic factors. The survey is unique, combining interviews and physical examinations, and is publicly available at www.cdc.gov/nchs/nhanes/, with the detailed procedures previously described (25). The NCHS Research Ethics Review Board examined and approved the NHANES protocol.
Participants in this study were drawn from the NHANES cycles for 2013–2014 and 2015–2016. Due to the availability of α-Klotho data, we only included adult participants aged from 40 to 79 years. As shown in Figure 1, the sample size was reduced to 1,499 after eliminating participants with missing data on serum α-Klotho (n = 14,742), PFAS (n = 3,603), and any missing covariates included in the study (n = 302).
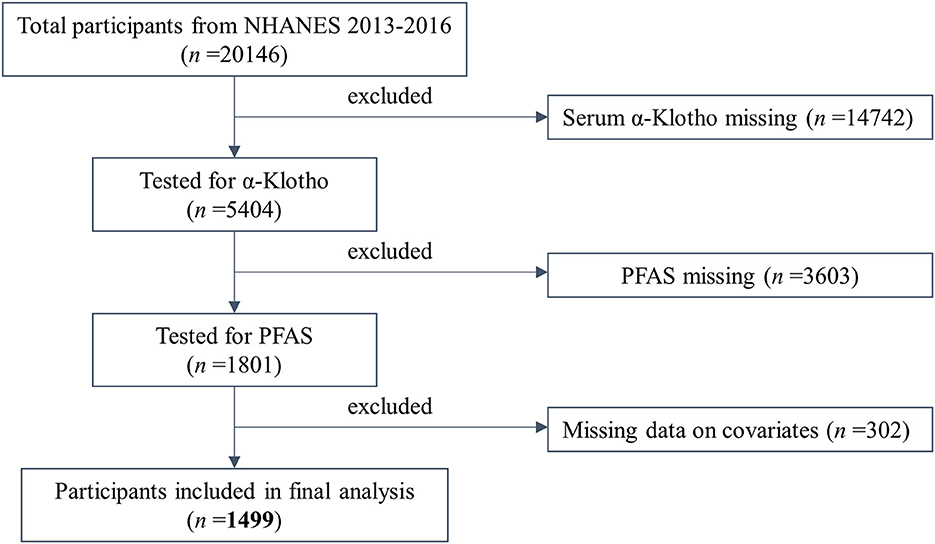
Figure 1. Flow chart of the population included in the final analysis of this study. n = 1,499, NHANES, USA, 2013–2016.
2.2. Measurement of serum α-Klotho level
Fresh frozen serum samples, stored at −80°C, were used for α-Klotho concentration measurements by a CDC certified laboratory using a commercially available ELISA kit (IBL International, Japan). The IBL ELISA method was extensively validated to measure Klotho concentrations in human samples prior to formal analysis. The sensitivity of the assay was 6.15 pg/mL. For quality assurance, the average of two replicate analyses was used, and the detailed description of the analytical method was included as previous document (26). All measurements were completed by the U.S. Centers for Disease Control and Prevention for the ongoing NHANES.
2.3. Measurements of PFAS
One third of the population aged 12 years and older was randomly selected in each survey cycle and examined for the presence of PFAS in their serum by using online solid-phase extraction-high performance liquid chromatography-turbine ionization-tandem mass spectrometry measurements. A total of 12 PFAS were detected from 2013 to 2016. Details of the analytical method could be found on the NHANES website (27–29).
This study focused on those PFAS compounds with detection rates higher than 80% among survey participants from 2013 to 2016, including PFOA, PFOS, PFNA, and PFHxS. The concentrations of linear perfluorooctanoate (n-PFOA) and branched isomers of perfluorooctanoate (Sb-PFOA) were summed to total PFOA. The concentrations of linear perfluorooctane sulfonate (n-PFOS) and monomethyl branched isomers of PFOS (Sm-PFOS) were calculated as total PFOS. The values below the lower limit of detection (LLOD) were imputed by the LLOD divided by the square root of 2 (30).
2.4. Covariates
Based on clinical significance and univariate analysis, covariates were specifically selected from questionnaire data on demographics, socioeconomic status, health behaviors, and health status (31). We included age, gender, race, household income, education, physical activity, body mass index (BMI), smoking, alcohol consumption, diabetes, hypertension, urine albumin/urine creatinine (uACR), and glomerular filtration rate (eGFR) as covariates.
Sociodemographic variables included age (40–59 and 60–79), gender (male and female), race (non-Hispanic white, non-Hispanic black, Mexican American, and other race), family income to poverty ratio (<1 and ≥1), and education (less than high school, high school or equivalent, and more than high school). Health-related behavior information included physical activity (yes and no), BMI (<25 kg/m2, 25 to < 30 kg/m2, and ≥30 kg/m2), smoking status (never smoker, past smoker, and current smoker), and alcohol consumption (yes and no). Health status included a history of hypertension (yes and no), and a history of diabetes (yes and no). Of these, physical activity was classified as moderate or vigorous physical recreational activity vs. no activity. BMI was calculated as weight in kilograms divided by height in meters squared. For smoking status, past smokers and never smokers were categorized according to having smoked at least 100 cigarettes in their lifetime. Those who smoked more than 100 cigarettes during their lifetime and smoked some days or every day were defined as current smokers. Alcohol consumers and non-alcohol consumers were separated based on having consumed at least 12 alcoholic beverages in their lifetime.
Estimated glomerular filtration rate (eGFR) was calculated according to the CKD-EPI-Scr creatinine for participants aged ≤70 years (32), and the BIS1-Scr creatinine equation for participants aged >70 years (33). Urinary albumin and creatinine levels were gold-scaled and calibrated according to the NHANES's recommended standard method, where uACR = urinary albumin/urine creatinine.
2.5. Statistical analyses
In this study, we took into account the complex survey design and data clustering, following the NHANES procedure of using subsample population weights to obtain estimates typical of the U.S. population. Since the data for the four serum PFAS and α-Klotho concentrations were significantly right-skewed, the data were log-transformed to obtain a near-normal distribution in part of our analysis (34, 35). Weighted geometric means and 95% CIs of serum α-Klotho levels and the four serum PFAS were calculated for the entire study population as well as for covariates based on the characteristics of the study population. To determine the statistical significance of the mean differences, a t-test was used for two-group comparisons, and a one-way ANOVA was conducted for comparisons of multiple groups.
PFAS levels were analyzed as continuous variables and quartiles, respectively. A Pearson correlation analysis on the log-transformed serum α-Klotho levels and these four log-transformed PFAS concentrations was performed. The adjusted geometric mean of serum α-Klotho was calculated by quartiles of serum PFAS levels. The median value of the quartiles was applied to the PFAS exposure variable, as a continuous variable in a linear trend test. The relationship between serum α-Klotho (dependent variable) and serum PFAS (independent variable) was evaluated using linear regression, and the corresponding beta coefficients and 95% CIs were calculated. Beta coefficients and 95% CIs for serum α-Klotho levels among individuals with higher levels of serum PFAS (quartiles 2 through 4) compared with those with the lowest level of serum PFAS (quartile 1) were also calculated. We further performed regression analyses stratified by age and sex to ascertain an age- or gender-specific association between PFAS concentrations and serum α-Klotho levels. The above analysis was performed using the package “nhanesR” version 0.9.4.0. Using the R package “qgcomp,” the quantile-based g-computation models were used to assess the relationship between combined exposures to PFAS and serum α-Klotho levels. All statistical analyses were performed using R version 4.2.1 and Microsoft Excel 2016. P < 0.05 was considered statistically significant.
3. Results
3.1. General characteristics of the study participants
The weighted population characteristics of the study participants were presented in Table 1. The weighted mean age was 56.72 years, in the range of 40–79 years, with more females (53.50%). The constituent ratio of the study population was 39.76% for non-Hispanic white, 20.81% for non-Hispanic black, 15.01% for Mexican American, and 24.42% for other races. Most of the participants had a family income above the poverty level (79.99%) and had more than a high school diploma (54.90%). The weighted mean BMI was 29.71 kg/m2 with a high number of overweight and obese individuals (33.62 and 43.63%, respectively). The majority of participants engaged in physical activities (71.58%), never smoked (53.17%), drank alcohol (85.46%), did not have diabetes (71.65%), and had hypertension (54.57%).
Weighted geometric means (GM) and 95% CIs of serum α-Klotho and serum PFOA, PFOS, PFNA, and PFHxS levels were calculated for the entire study population as well as for covariates based on the characteristics of the study population separately in Table 2. For the entire study population, the geometric mean (95% CI) α-Klotho level was 791.38 (776.17, 806.88) pg/mL. Serum α-Klotho levels varied significantly by age, sex, race, education, BMI, smoking status, alcohol consumption, and hypertension history. As expected, the geometric mean serum α-Klotho level was significantly higher in middle adults aged from 40 to 59 years (814.93 pg/mL) than that in older participants aged from 60 to 79 years (756.82 pg/mL). The higher α-Klotho levels were also found in female (male vs. female, 773.81 vs. 808.30 pg/mL), black (non-Hispanic white, non-Hispanic black, Mexican American, and other race; 784.26, 833.33, 783.78, and 807.59 pg/mL), and highly educated (less than high school, high school or equivalent, and more than high school; 774.98, 753.20, and 807.68 pg/mL) subjects. Furthermore, α-Klotho serum levels were significantly lower in overweight (777.00 pg/mL) and obese (781.65 pg/mL) people than in normal and low weight people (830.01 pg/mL). For health-related factors, those with higher serum α-Klotho levels never smoked (current smoker, past smoker, and never smoker; 719.62, 797.65, and 815.98 pg/mL), did not drink alcohol (drinker vs. non-drinker; 783.50 vs. 875.76 pg/mL), and were never diagnosed with hypertension (yes vs. no; 772.09 vs. 810.22 pg/mL).
The geometric mean (95% CI) of serum PFOA, PFOS, PFNA, and PFHxS for the total study population were 2.00 (1.87–2.14) ng/mL, 5.93 (5.50–6.39) ng/mL, 0.71 (0.66–0.77) ng/mL, and 1.41 (1.29–1.54) ng/mL, respectively. Serum concentrations of these four PFAS were higher in older adults (60–79 years) than those in the middle (40–59 years), with higher blood concentrations in males than those in females. Four PFAS levels were found to be lower in the Mexican American population significantly. Participants with high family income had higher concentrations of PFOA and PFNA in their blood with significance. PFNA and PFHxS concentrations were higher in the blood of overweight participants and PFOS in the blood of hypertensive participants. Interestingly, blood PFOS concentrations were higher in participants who were past smokers or never smokers compared with those in current smokers.
3.2. Measurements and correlations of serum PFAS and the relationships with α-Klotho
The PFAS concentrations in the study population were shown in Supplementary Table 1. All four PFAS were detectable in more than 98% of the population. PFOS had the highest detection rate as 99.40%, followed by PFOA as 99.33%, PFNA as 98.87% and PFHxS as 98.73%. Notably, PFOS had the highest concentration with GM 5.93 ng/mL, while PFNA had the lowest with GM 0.71 ng/mL.
From Figure 2, it showed the correlation matrix of log-transformed α-Klotho and serum PFAS concentrations. Serum α-Klotho levels presented a negative correlation with blood PFHxS, PFNA, and PFOA levels separately. The correlation coefficients ranged from −0.09 to −0.06, with greater correlation coefficients for PFHxS and PFNA levels compared with PFOA. However, serum PFOS levels had no significant correlation with serum α-Klotho. Furthermore, the correlation coefficients among the four PFAS were significance (P < 0.001). The strongest correlation was found between PFOS and PFNA (r = 0.71), followed by the correlation between PFOS and PFHxS (r = 0.67), while the weakest correlation was between PFNA and PFHxS (r = 0.51).
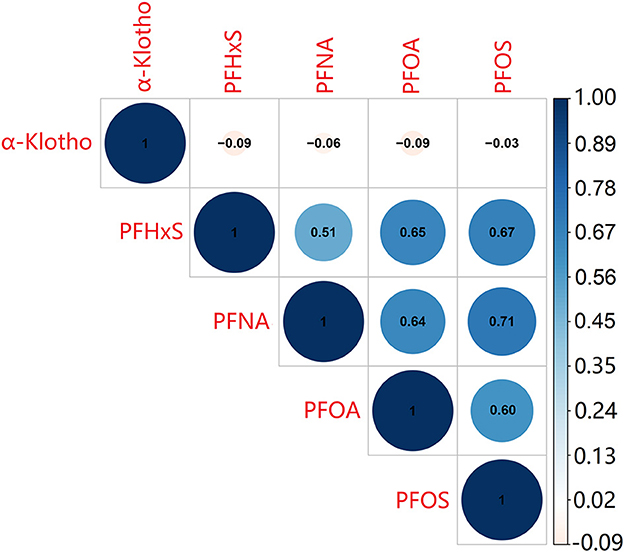
Figure 2. The correlation between the four PFAS and α-Klotho levels. The test was Pearson's correlation coefficients among log-transformed indices.
3.3. Mean serum α-Klotho levels by quartiles of PFAS
As shown from Figure 3 and Table 3, the geometric mean serum α-Klotho levels revealed a statistically significant declining trend with rising serum PFOA (P = 0.007), PFNA (P = 0.005) and PFHxS (P = 0.007). The adjusted geometric means of serum α-Klotho showed a statistically significant downward trend with serum PFOA (P = 0.047) and PFNA (P = 0.010), after adjusting for all covariables.
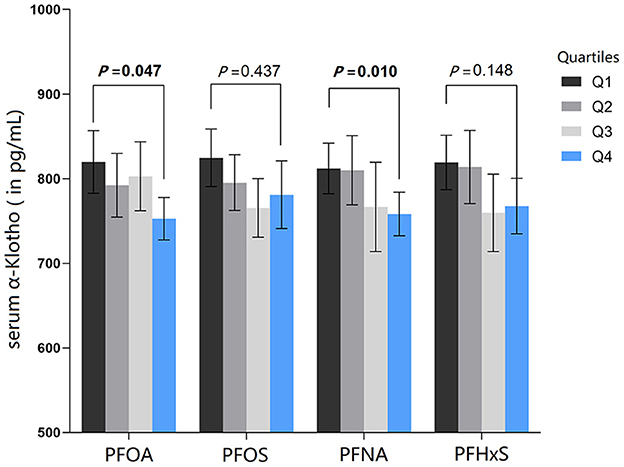
Figure 3. All-adjusted geometric means (and 95% CIs) of serum α-Klotho by quartiles of serum PFAS. The all-adjusted model for serum PFAS were adjusted for age, gender, race, family income to poverty ratio, education, physical activity, BMI, smoking, alcohol consumption, diabetes, hypertension, uACR, and eGFR.
3.4. Linear relationships between serum α-Klotho and exposure to PFAS
Multiple general linear models were then performed to investigate the association between blood PFAS concentrations and serum α-Klotho levels in the total population. Associations between continuous and quartiles of PFAS and α-Klotho measurement were reported in Table 4. In most cases, continuous PFAS concentrations and α-Klotho measurements were negatively correlated, but only the association between PFNA and α-Klotho was statistically significant in both unadjusted and adjusted models. After adjusting for all covariates, α-Klotho levels were decreased by 20.23 pg/mL for each 1-unit increase in PFNA concentration (P = 0.036). In quartile analysis, there was a significant negative association between α-Klotho and Q4 of PFOA [unadjusted β (95% CI) = −79.41 (−138.20, −20.63), P = 0.010], Q3 of PFOS [unadjusted β (95% CI) = −66.64 (−125.70, −7.59), P = 0.028], Q4 of PFNA [unadjusted β (95% CI) = −57.83 (−104.03, −11.64), P = 0.016], and Q4 of PFHxS [unadjusted β (95% CI) = −60.55 (−111.34, −9.75), P = 0.021] in the unadjusted model relative to the lowest quartile (Q1) of each exposure. However, in the adjusted model, only a significant negative correlation was found between α-Klotho and Q4 of PFNA [adjusted β (95% CI) = −61.36 (−113.26, −9.45), P = 0.025] relative to Q1.
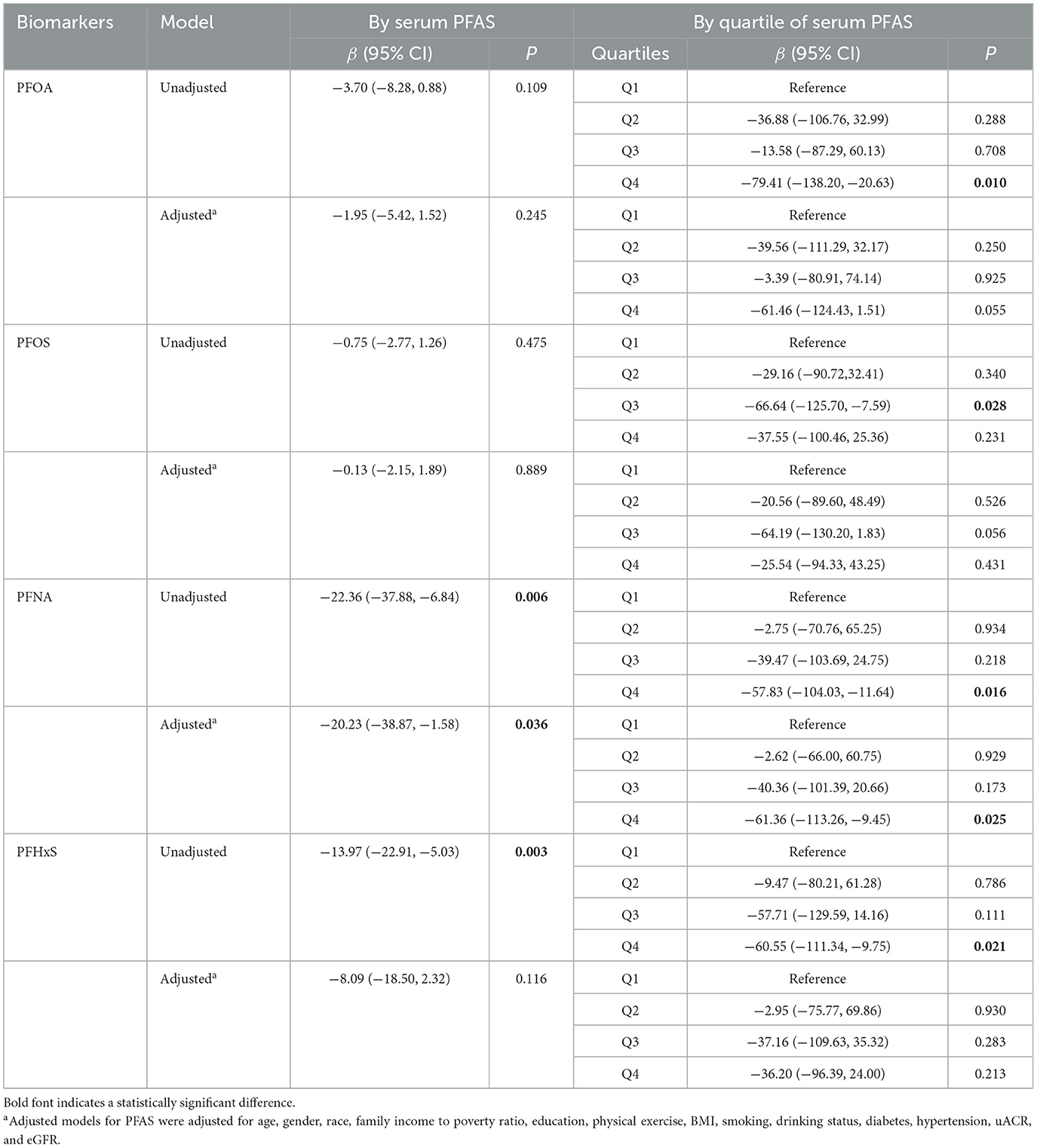
Table 4. Linear regression analysis of serum α-Klotho levels and serum PFAS concentrations among study participants.
3.5. Stratified analysis by age and gender
Then the relationships between serum α-Klotho and PFAS concentrations were stratified by age and gender as shown in Table 5. After stratified by age, only a significantly negative association between PFNA concentration and α-Klotho was observed in middle adults (40–59 years) in the adjusted model with a β of −50.98 (P = 0.017). In sex-stratified analyses, a significantly negative association between the concentration of PFNA and α-Klotho was observed only in the females in the adjusted model with a β of −37.01 (P = 0.047). Additionally, when stratified by age and sex, significant negative associations of PFOA, PFNA, and PFHxS with serum α-Klotho were found in the unadjusted model in middle (40–59 years) female participants. A negative correlation between PFNA and serum α-Klotho was also found in older (60–79 years) females. However, in the adjusted model, we only found a negative association of PFNA with serum α-Klotho in middle-aged (40–59 years) female participants, with an adjusted β of −109.66 (P < 0.001).
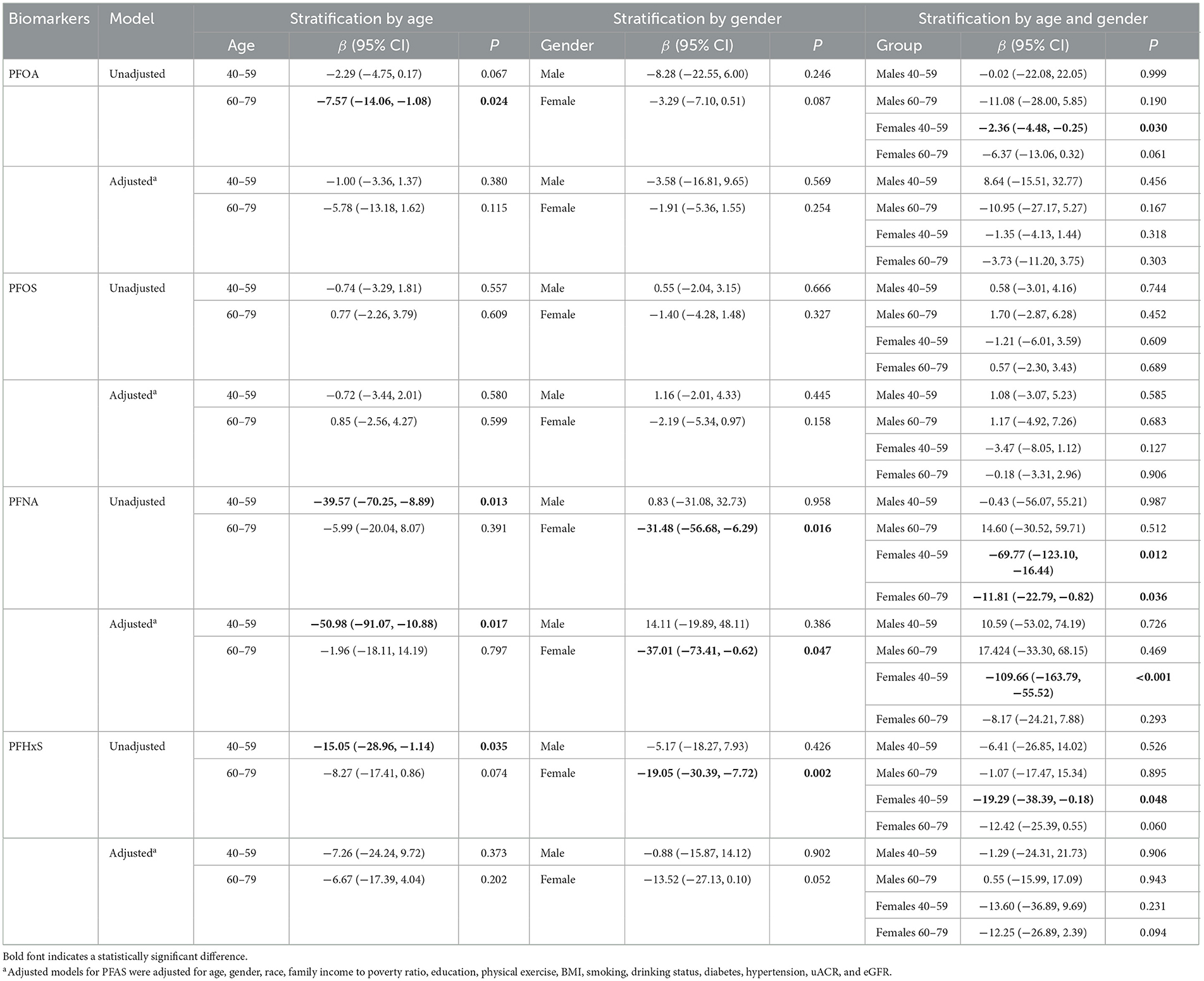
Table 5. Linear regression analysis of serum α-Klotho levels and serum PFAS concentrations among study participants stratified by age and gender.
3.6. Quantile-based g-computation (Q-gcomp) models
After adjusting for all covariates, the mixture of the four PFAS showed a significant inverse relationship with α-Klotho concentration in the Q-gcomp analysis, PFNA was considered the major contributor and assigned the largest negative weight from Figure 4A. Furthermore, the estimated α-Klotho decreased linearly by quartile of PFAS mixture concentrations in Figure 4B.
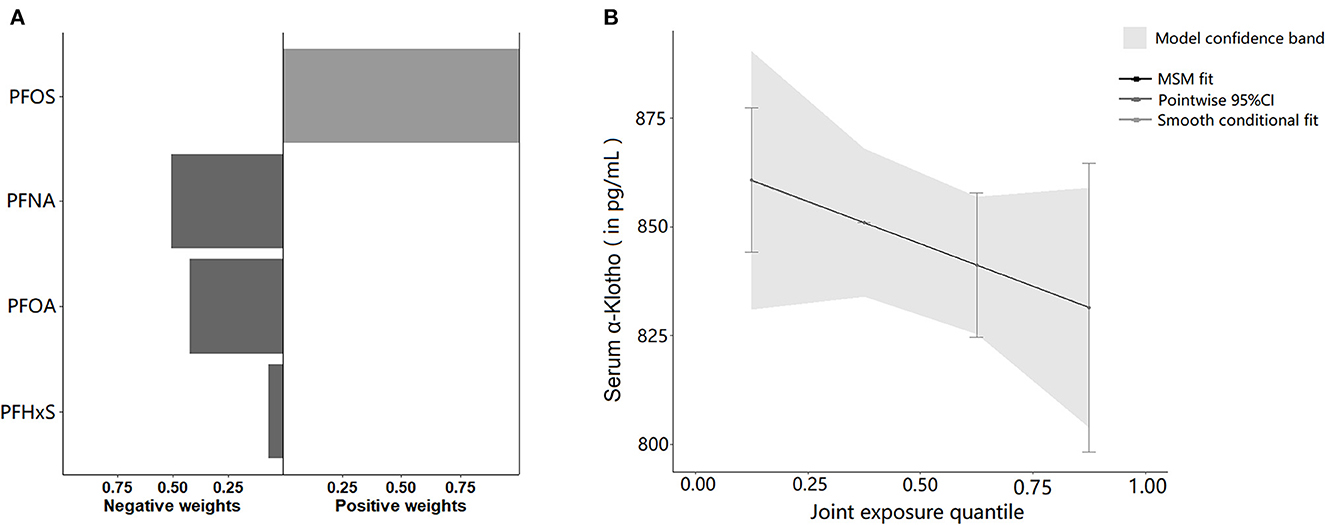
Figure 4. (A) The directions and magnitude of the assigned weights for each PFAS in relation to serum α-Klotho in quantile g-computation. (B) Mixed effects of four serum PFAS concentrations on serum α-Klotho. The model was adjusted for age, gender, race, family income to poverty ratio, education, physical activity, BMI, smoking, alcohol consumption, diabetes, hypertension, uACR, and eGFR.
4. Discussion
Serum soluble α-Klotho, as an endocrine, plays an important role in regulating oxidative stress and the aging process. In this study, we investigated the association between four serum PFAS and serum α-Klotho levels in adults aged 40–79 years, using samples from the US. The main results were included in Figure 5. Interestingly, we found that PFAS exposure levels, specifically PFNA, were inversely associated with soluble α-Klotho serum contents in US adult participants after correcting potential confounding factors. The α-Klotho serum levels were decreased with increasing PFAS exposure levels, and this association was stronger in females aged from 40 to 59, providing new insights into the potential role of PFAS in oxidative stress and aging processes.
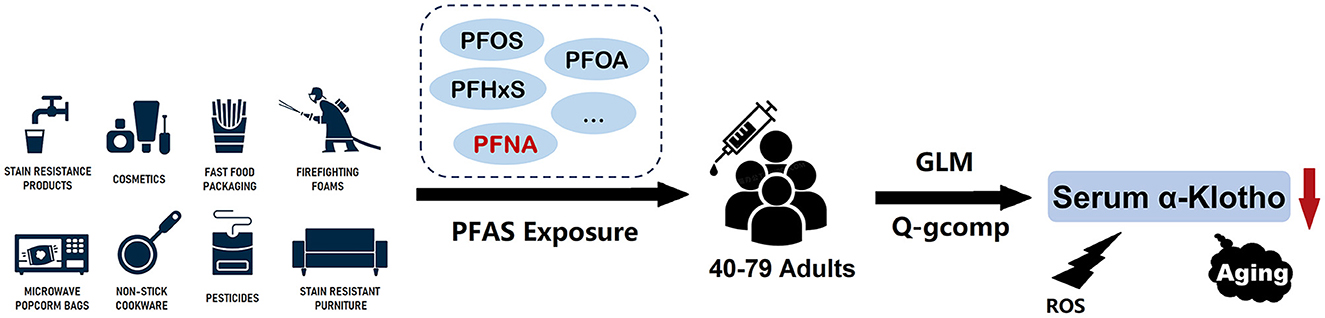
Figure 5. Overview of the results of the association between PFAS exposure and serum α-Klotho levels in middle-old aged participants.
The variations in α-Klotho levels may be connected to the pathophysiology of the chronic kidney injury and neurotoxic effects associated with PFAS exposure according to previous studies (6, 36–38). Increasing studies have connected PFAS exposure to a rise in oxidative stress in the kidney, including DNA damage, increased production of mitochondrial transport chain proteins (39), decreased cell proliferation, and apoptosis (40). PFOS exposure might cause renal damage via multiple pathways, including dysregulation of PPARα and PPARγ, two key nuclear receptor hormones, highly expressed in the proximal renal tubule and involved in lipid metabolism, lipogenesis, glucose homeostasis, and cell growth and differentiation (41, 42). The renal tubular epithelial (KTE) cells exposed to PFOS showed a dramatic increase in apoptosis and fibrosis through Sirt1-mediated PPARγ deacetylation in vitro (43). An experimental study showed that maternal exposure to PFOS and PFNA resulted in reduced nephron counts and early hypertension in rat offspring (44). Accumulation of PFAS in the brain may have toxic effects on the central nervous system, including PFAS-induced behavioral and cognitive deficits, related with the effects of PFAS on calcium balance and alterations in neurotransmitters of neurons (45). Additionally, a-Klotho protein is a co-receptor of FGF23 and highly expressed in the kidney and brain. The kidney has the highest level of Klotho as a major source of soluble Klotho (46). Chronic kidney disease (CKD) has been a condition of Klotho deficiency, and animal models of CKD showed the reduced levels of total Klotho gene expression and in kidney tissue, with low soluble Klotho in circulation. In numerous experimental models, upregulating the soluble Klotho or the endogenous Klotho protected the kidney from injury, and inhibited renal fibrosis in CKD (47–49). In brain, Klotho is mainly produced by the choroid plexus and expressed at lower levels in specific brain regions, particularly in the hippocampus and pituitary (50). Behavioral studies on Klotho-knockout-mice showed deficits in memory retention compared with those of wild-type mice, possibly due to the increased oxidative stress in brain (51). In addition, Klotho may activate an antioxidant enzyme system, protecting hippocampus neurons against amyloid and glutamate toxicity, as a neuroprotective protein (52). Thus, decreased α-Klotho levels by PFAS exposure could explain some of the potential chronic kidney injury and neurotoxic effects from PFAS. However, further studies are needed to fully understand the regulatory role of α-Klotho on chronic kidney injury and neurotoxic effects after PFAS exposure.
It is still unclear how PFAS exposure regulates soluble α-Klotho levels in humans. The Klotho gene has been regulated by epigenetic and non-epigenetic mechanisms in renal disease (53). Exposure to higher levels of environmental pollutants (e.g., lead and cadmium) is associated with elevated levels of methylation of the Klotho gene, which may alter Klotho mRNA synthesis, leading to decreased protein levels of Klotho (54). Some PFAS, such as PFOA, PFNA, PFOS, and PFHxS had the potentials to cause oxidative stress in terms of ROS generation and DNA damage and were linked to indicators of inflammation and oxidative stress (11, 55). On the other hand, several studies on animals have revealed that higher Klotho was connected to lower oxidative stress (51, 56). PFAS exposure may affect human serum Klotho levels through multiple pathways. Future researches are currently needed to elucidate the specific biological processes or toxicological pathways by which PFAS exposure correlates inversely with human serum α-Klotho levels.
We performed multiple comparisons and observed age- and sex-specific associations in this study. Our study revealed higher serum concentrations of four PFAS in males than in females, consistent with the results of previous studies, and the possible reasons for these differences were mainly related to menstruation, pregnancy and placental transfer, breastfeeding, and other subtle causes, such as differences in serum albumin (57). The male data needed to be analyzed separately from the female data to obtain additional effects. The negative correlation between PFAS exposure and serum α-Klotho levels was the strongest in middle-aged females, suggesting that PFAS might have effects on endocrine and metabolic function and that women may be more sensitive to the deleterious effects of PFAS. The similar observations were consistent with other exposure studies in both animal and human studies (58, 59). A very comprehensive review of the relationship between PFAS exposure and liver injury by Costello et al. (60), showed that evidences from rodents consistently supported the results of human studies and suggested that PFAS exposure might lead to liver injury with elevated liver enzymes, steatosis, and histopathological changes. Female mice exhibited more lobular and portal inflammation compared to male mice exposed to PFAS (58). Analysis of population data also revealed gender differences between serum PFAS levels and liver function biomarkers, with PFOA and PFNA associated with clinically elevated alanine aminotransferase in female adolescents (59). Previous studies have found higher levels of soluble α-Klotho in patients with cirrhosis, suggesting that α-Klotho was also associated with impaired liver function (61). The age and sex-specific correlations of PFAS exposure with serum α-Klotho levels were observed in this study, as well as the hepatotoxic effects of PFAS in animal experiments and human studies, providing evidences for further investigations of the hepatotoxic toxic effects from PFAS exposure on age and sex in humans and the potential mediating role of α-Klotho.
Another interesting finding was that plasma levels of PFOS were lower in active smokers than those in non-smokers. Similar finding was made in a prior Danish cohort research of 1,076 pregnant women, which discovered that daily smoking during pregnancy was linked to lower maternal PFOS plasma levels than never smoking (62). A Danish cohort study of 652 men also reported finding higher plasma PFOS levels in non-smokers than in current smokers (63). These findings should reflect lifestyle differences between non-smokers and smokers related to PFOS exposure sources, or may reflect the increased elimination of PFOS by smokers. However, studies from three different geographic regions of Korea found the higher PFAS levels in smokers than in non-smokers (64), and several studies showed no significant association between serum PFOA levels and smoking (65, 66). Therefore, no consistent trends regarding smoking and PFAS exposure were observed in the studies.
Briefly, our results demonstrated a substantial correlation between low serum α-Klotho levels and blood PFAS, particularly PFNA. Numerous evidences suggested that PFAS was associated with ROS production and DNA methylation (11), while serum α-Klotho levels were regulated to some extent by ROS and DNA methylation (51). The causal pathway might be that PFAS in blood led to increased Klotho gene methylation or ROS production between PFAS exposure and serum α-Klotho levels, with decreased Klotho gene levels. Further studies are needed to confirm the related mechanisms.
Jain et al. firstly analyzed the association between α-Klotho concentrations and selected PFAS in US adults using NHANES data (24), and they found two important confounding factors, glomerular filtration rate status and albuminuria, and a modest correlation between PFAS exposure and low serum α-Klotho in healthy kidneys. Our study used correlation analysis and multiple general linear models to analyze the association between serum α-Klotho levels and four PFAS exposures with a detection rate higher than 98% in US adults, and also confirmed a negative association between PFAS exposure and serum α-Klotho levels, based on the NHANES database. In contrast to previous studies, we performed the subgroup analyses by age and sex further and used a mixed-methods approach to estimate the association between individual PFAS and mixtures of four PFAS with serum α-Klotho. This study again validated the negative association between PFAS exposure and serum Klotho, complementing the association characteristics of gender and age, and found that the mixture of the four PFAS also showed a significant inverse relationship with α-Klotho concentration, with PFNA as the main contributor. At the same time, we recognized that changes in urinary protein and renal function were gradual. So, we used the urine protein-creatinine ratio and eGFR for correction rather than the presence or absence of urine protein or SKD staging in order to better reflect the relationships.
Although the cross-sectional research design was unable to establish a causal connection, reverse causality was implausible given the long half-lives (several years) of the PFAS chemicals studied in this study. This investigation focused on the four most studied persistent PFAS chemicals, and further risk evaluation of other newer short-chain and alternative PFAS chemicals are needed to do urgently. Although we have included most of the potential confounders in this study, there are residual confounders. For instance, humans may be contemporaneously exposed to several environmental contaminants, such as heavy metals and p-dichlorobenzene (54, 67), possibly associated with serum α-Klotho levels.
5. Conclusion
In all, this study demonstrated that serum levels of PFAS, of which PFNA was a major contributor, may be inversely related to serum levels of α-Klotho in the US adult population. And the relationship was particularly strong in middle-aged females. This study may also explain the earlier reported chronic renal injury and neurotoxic effects associated with PFAS exposure, as circulating α-Klotho plays an important role in aging-related diseases such as renal system disorders and neurological diseases. Future researches are necessary to confirm the causal relationship between serum PFAS and α-Klotho levels and elucidate the possible intrinsic mechanisms.
Data availability statement
Publicly available datasets were analyzed in this study. This data can be found at: https://www.cdc.gov/nchs/nhanes/index.htm.
Ethics statement
Ethical review and approval was not required for the study on human participants in accordance with the local legislation and institutional requirements. Written informed consent for participation was not required for this study in accordance with the national legislation and the institutional requirements.
Author contributions
ML and YM analyzed data and writing—original draft. WC, LZ, and CZ analyzed data and revised manuscript. WenjiZ and WenjuZ conceived the project, analyzed data, wrote the manuscript, provided supervision, and project administration. All authors were responsible for revising the manuscript, approved the final version, and read and agreed to the published version of the manuscript.
Funding
This work was supported by Guangdong Basic and Applied Basic Research Foundation (2021A1515011220 and 2023A1515010353), the Special Fund for Scientific Innovation Strategy-Construction of High Level Academy of Agriculture Science (grant numbers: R2018YJ-YB3002 and R2022PY-QY004), and Foundation of Director of Crops Research Institute, Guangdong Academy of Agricultural Sciences (202205).
Conflict of interest
The authors declare that the research was conducted in the absence of any commercial or financial relationships that could be construed as a potential conflict of interest.
Publisher's note
All claims expressed in this article are solely those of the authors and do not necessarily represent those of their affiliated organizations, or those of the publisher, the editors and the reviewers. Any product that may be evaluated in this article, or claim that may be made by its manufacturer, is not guaranteed or endorsed by the publisher.
Supplementary material
The Supplementary Material for this article can be found online at: https://www.frontiersin.org/articles/10.3389/fpubh.2023.1136454/full#supplementary-material
References
1. Podder A, Sadmani A, Reinhart D, Chang NB, Goel R. Per and poly-fluoroalkyl substances (PFAS) as a contaminant of emerging concern in surface water: A transboundary review of their occurrences and toxicity effects. J Hazard Mater. (2021) 419:126361. doi: 10.1016/j.jhazmat.2021.126361
2. Sunderland EM, Hu XC, Dassuncao C, Tokranov AK, Wagner CC, Allen JG, et al. Review of the pathways of human exposure to poly- and perfluoroalkyl substances (PFASs) and present understanding of health effects. J Expo Sci Environ Epidemiol. (2019) 29:131–47. doi: 10.1038/s41370-018-0094-1
3. Jha G, Kankarla V, McLennon E, Pal S, Sihi D, Dari B, et al. Per- and polyfluoroalkyl substances (PFAS) in integrated crop-livestock systems: Environmental exposure and human health risks. Int J Environ Res Public Health. (2021) 18:2312550. doi: 10.3390/ijerph182312550
4. Evich MG, Davis MJB, McCord JP, Acrey B, Awkerman JA, Knappe DRU, et al. Per- and polyfluoroalkyl substances in the environment. Science. (2022) 375:eabg9065. doi: 10.1126/science.abg9065
5. Zhang Z, Sarkar D, Biswas JK, Datta R. Biodegradation of per- and polyfluoroalkyl substances (PFAS): A review. Bioresour Technol. (2022) 344:126223. doi: 10.1016/j.biortech.2021.126223
6. Stanifer JW, Stapleton HM, Souma T, Wittmer A, Zhao X, Boulware LE. Perfluorinated chemicals as emerging environmental threats to kidney health: A scoping review. Clin J Am Soc Nephrol. (2018) 13:1479–92. doi: 10.2215/CJN.04670418
7. Kim S, Thapar I, Brooks BW. Epigenetic changes by per- and polyfluoroalkyl substances (PFAS). Environ Pollut. (2021) 279:116929. doi: 10.1016/j.envpol.2021.116929
8. López-Arellano P, López-Arellano K, Luna J, Flores D, Jiménez-Salazar J, Gavia G, et al. Perfluorooctanoic acid disrupts gap junction intercellular communication and induces reactive oxygen species formation and apoptosis in mouse ovaries. Environ Toxicol. (2019) 34:92–8. doi: 10.1002/tox.22661
9. Choi EM, Suh KS, Rhee SY, Oh S, Woo JT, Kim SW, et al. Perfluorooctanoic acid induces mitochondrial dysfunction in MC3T3-E1 osteoblast cells. J Environ Sci Health A Tox Hazard Subst Environ Eng. (2017) 52:281–9. doi: 10.1080/10934529.2016.1253402
10. Zhou Y, Li H, Lin C, Mao Y, Rao J, Lou Y, et al. Perfluorooctanoic acid (PFOA) inhibits the gap junction intercellular communication and induces apoptosis in human ovarian granulosa cells. Reprod Toxicol. (2020) 98:125–33. doi: 10.1016/j.reprotox.2020.09.005
11. Wielsøe M, Long M, Ghisari M, Bonefeld-Jørgensen EC. Perfluoroalkylated substances (PFAS) affect oxidative stress biomarkers in vitro. Chemosphere. (2015) 129:239–45. doi: 10.1016/j.chemosphere.2014.10.014
12. Lin CY, Lee HL, Hwang YT, Su TC. The association between total serum isomers of per- and polyfluoroalkyl substances, lipid profiles, and the DNA oxidative/nitrative stress biomarkers in middle-aged Taiwanese adults. Environ Res. (2020) 182:109064. doi: 10.1016/j.envres.2019.109064
13. Kim JH, Park HY, Jeon JD, Kho Y, Kim SK, Park MS, et al. The modifying effect of vitamin C on the association between perfluorinated compounds and insulin resistance in the Korean elderly: A double-blind, randomized, placebo-controlled crossover trial. Eur J Nutr. (2016) 55:1011–20. doi: 10.1007/s00394-015-0915-0
14. Xu Y, Sun Z. Molecular basis of Klotho: From gene to function in aging. Endocr Rev. (2015) 36:174–93. doi: 10.1210/er.2013-1079
15. Zhao Y, Zeng CY, Li XH, Yang TT, Kuang X, Du JR. Klotho overexpression improves amyloid-β clearance and cognition in the APP/PS1 mouse model of Alzheimer's disease. Aging Cell. (2020) 19:e13239. doi: 10.1111/acel.13239
16. Mencke R, Olauson H, Hillebrands JL. Effects of Klotho on fibrosis and cancer: A renal focus on mechanisms and therapeutic strategies. Adv Drug Deliv Rev. (2017) 121:85–100. doi: 10.1016/j.addr.2017.07.009
17. Kale A, Sankrityayan H, Anders HJ, Gaikwad AB. Klotho in kidney diseases: A crosstalk between the renin-angiotensin system and endoplasmic reticulum stress. Nephrol Dial Transplant. (2021) 2021:gfab340. doi: 10.1093/ndt/gfab340
18. Razzaque MS. The role of Klotho in energy metabolism. Nat Rev Endocrinol. (2012) 8:579–87. doi: 10.1038/nrendo.2012.75
19. Kuro-o M. Klotho, phosphate and FGF-23 in ageing and disturbed mineral metabolism. Nat Rev Nephrol. (2013) 9:650–60. doi: 10.1038/nrneph.2013.111
20. Emerling BM, Weinberg F, Liu JL, Mak TW, Chandel NS, PTEN. regulates p300-dependent hypoxia-inducible factor 1 transcriptional activity through Forkhead transcription factor 3a (FOXO3a). Proc Natl Acad Sci USA. (2008) 105:2622–7. doi: 10.1073/pnas.0706790105
21. Hsieh CC, Kuro-o M, Rosenblatt KP, Brobey R, Papaconstantinou J. The ASK1-Signalosome regulates p38 MAPK activity in response to levels of endogenous oxidative stress in the Klotho mouse models of aging. Aging. (2010) 2:597–611. doi: 10.18632/aging.100194
22. Wang Y, Kuro-o M, Sun Z. Klotho gene delivery suppresses Nox2 expression and attenuates oxidative stress in rat aortic smooth muscle cells via the cAMP-PKA pathway. Aging Cell. (2012) 11:410–7. doi: 10.1111/j.1474-9726.2012.00796.x
23. Sena LA, Chandel NS. Physiological roles of mitochondrial reactive oxygen species. Mol Cell. (2012) 48:158–67. doi: 10.1016/j.molcel.2012.09.025
24. Jain RB, Ducatman A. Associations between the concentrations of α-klotho and selected perfluoroalkyl substances in the presence of eGFR based kidney function and albuminuria: Data for US adults aged 40-79 years. Sci Total Environ. (2022) 838:155994. doi: 10.1016/j.scitotenv.2022.155994
25. Calafat AM, Kuklenyik Z, Reidy JA, Caudill SP, Tully JS, Needham LL. Serum concentrations of 11 polyfluoroalkyl compounds in the us population: Data from the national health and nutrition examination survey (NHANES). Environ Sci Technol. (2007) 41:2237–42. doi: 10.1021/es062686m
26. CDC. National Health and Nutrition Examination Survey: 2015−2016 Data Documentation, Codebook, and Frequencies (SSKL_I). (2016). Available online at: https://wwwn.cdc.gov/Nchs/Nhanes/2015-2016/SSKL_I.htm (accessed February 23, 2023).
27. CDC. 2016 Data Documentation, Codebook, and Frequencies Perfluoroalkyl and Polyfluoroalkyl (PFAS_I) Centers for Disease Control and Prevention. (2018). Available online at: https://wwwn.cdc.gov/Nchs/Nhanes/2015-2016/PFAS_I.htm#LBDBFOAL (accessed February 23, 2023).
28. CDC. Perfluoroalkyl and Polyfluoroalkyl Substances - Linear and Branched PFOS and PFOA Isomers (Surplus) (SSPFAS_H). (2013). Available online at: https://wwwn.cdc.gov/Nchs/Nhanes/2013-2014/SSPFAS_H.htm (accessed February 23, 2023).
29. CDC. Perfluoroalkyl and Polyfluoroalkyl Substances (SSPFSU_H). (2013). Available online at: https://wwwn.cdc.gov/Nchs/Nhanes/2013-2014/SSPFSU_H.htm (accessed February 23, 2023).
30. Hornung RW, Reed LD. Estimation of average concentration in the presence of nondetectable values. Appl Occup Environ Hyg. (1990) 5:46–51. doi: 10.1080/1047322X.1990.10389587
31. Chowdhury MZI, Turin TC. Variable selection strategies and its importance in clinical prediction modelling. Fam Med Community Health. (2020) 8:e000262. doi: 10.1136/fmch-2019-000262
32. Levey AS, Stevens LA, Schmid CH, Zhang YL, Castro AF 3rd, Feldman HI, et al. A new equation to estimate glomerular filtration rate. Ann Intern Med. (2009) 150:604–12. doi: 10.7326/0003-4819-150-9-200905050-00006
33. Schaeffner ES, Ebert N, Delanaye P, Frei U, Gaedeke J, Jakob O, et al. Two novel equations to estimate kidney function in persons aged 70 years or older. Ann Intern Med. (2012) 157:471–81. doi: 10.7326/0003-4819-157-7-201210020-00003
34. Akimoto T, Yoshizawa H, Watanabe Y, Numata A, Yamazaki T, Takeshima E, et al. Characteristics of urinary and serum soluble Klotho protein in patients with different degrees of chronic kidney disease. BMC Nephrol. (2012) 13:155. doi: 10.1186/1471-2369-13-155
35. Wang Y, Aimuzi R, Nian M, Zhang Y, Luo K, Zhang J. Perfluoroalkyl substances and sex hormones in postmenopausal women: NHANES 2013-2016. Environ Int. (2021) 149:106408. doi: 10.1016/j.envint.2021.106408
36. Lu Y, Gao K, Li X, Tang Z, Xiang L, Zhao H, et al. Mass spectrometry-based metabolomics reveals occupational exposure to per- and polyfluoroalkyl substances relates to oxidative stress, fatty acid β-oxidation disorder, and kidney injury in a manufactory in China. Environ Sci Technol. (2019) 53:9800–9. doi: 10.1021/acs.est.9b01608
37. Di Nisio A, Pannella M, Vogiatzis S, Sut S, Dall'Acqua S, Rocca MS, et al. Impairment of human dopaminergic neurons at different developmental stages by perfluoro-octanoic acid (PFOA) and differential human brain areas accumulation of perfluoroalkyl chemicals. Environ Int. (2022) 158:106982. doi: 10.1016/j.envint.2021.106982
38. Eggers Pedersen K, Basu N, Letcher R, Greaves AK, Sonne C, Dietz R, et al. Brain region-specific perfluoroalkylated sulfonate (PFSA) and carboxylic acid (PFCA) accumulation and neurochemical biomarker responses in east Greenland polar bears (Ursus maritimus). Environ Res. (2015) 138:22–31. doi: 10.1016/j.envres.2015.01.015
39. Diaz MJ, Chinje E, Kentish P, Jarnot B, George M, Gibson G. Induction of cytochrome P4504A by the peroxisome proliferator perfluoro-n-octanoic acid. Toxicology. (1994) 86:109–22. doi: 10.1016/0300-483X(94)90056-6
40. Gorrochategui E, Lacorte S, Tauler R, Martin FL. Perfluoroalkylated substance effects in Xenopus laevis A6 kidney epithelial cells determined by ATR-FTIR spectroscopy and chemometric analysis. Chem Res Toxicol. (2016) 29:924–32. doi: 10.1021/acs.chemrestox.6b00076
41. Abbott BD, Wood CR, Watkins AM, Tatum-Gibbs K, Das KP, Lau C. Effects of perfluorooctanoic acid (PFOA) on expression of peroxisome proliferator-activated receptors (PPAR) and nuclear receptor-regulated genes in fetal and postnatal CD-1 mouse tissues. Reprod Toxicol. (2012) 33:491–505. doi: 10.1016/j.reprotox.2011.11.005
42. Arukwe A, Mortensen AS. Lipid peroxidation and oxidative stress responses of salmon fed a diet containing perfluorooctane sulfonic- or perfluorooctane carboxylic acids. Comp Biochem Physiol C Toxicol Pharmacol. (2011) 154:288–95. doi: 10.1016/j.cbpc.2011.06.012
43. Wen LL, Lin CY, Chou HC, Chang CC, Lo HY, Juan SH. Perfluorooctanesulfonate mediates renal tubular cell apoptosis through PPARgamma inactivation. PLoS ONE. (2016) 11:e0155190. doi: 10.1371/journal.pone.0155190
44. Rogers JM, Ellis-Hutchings RG, Grey BE, Zucker RM, Norwood J Jr, Grace CE, et al. Elevated blood pressure in offspring of rats exposed to diverse chemicals during pregnancy. Toxicol Sci. (2014) 137:436–46. doi: 10.1093/toxsci/kft248
45. Cao Y, Ng C. Absorption, distribution, and toxicity of per- and polyfluoroalkyl substances (PFAS) in the brain: A review. Environ Sci Process Impacts. (2021) 23:1623–40. doi: 10.1039/D1EM00228G
46. Lindberg K, Amin R, Moe OW, Hu MC, Erben RG, Östman Wernerson A, et al. The kidney is the principal organ mediating klotho effects. J Am Soc Nephrol. (2014) 25:2169–75. doi: 10.1681/ASN.2013111209
47. Haruna Y, Kashihara N, Satoh M, Tomita N, Namikoshi T, Sasaki T, et al. Amelioration of progressive renal injury by genetic manipulation of Klotho gene. Proc Natl Acad Sci USA. (2007) 104:2331–6. doi: 10.1073/pnas.0611079104
48. Mitani H, Ishizaka N, Aizawa T, Ohno M, Usui S, Suzuki T, et al. In vivo klotho gene transfer ameliorates angiotensin II-induced renal damage. Hypertension. (2002) 39:838–43. doi: 10.1161/01.HYP.0000013734.33441.EA
49. Hu MC, Moe OW. Klotho as a potential biomarker and therapy for acute kidney injury. Nat Rev Nephrol. (2012) 8:423–9. doi: 10.1038/nrneph.2012.92
50. German DC, Khobahy I, Pastor J, Kuro OM, Liu X. Nuclear localization of Klotho in brain: An anti-aging protein. Neurobiol Aging. (2012) 33:1483.e25–30. doi: 10.1016/j.neurobiolaging.2011.12.018
51. Nagai T, Yamada K, Kim HC, Kim YS, Noda Y, Imura A, et al. Cognition impairment in the genetic model of aging klotho gene mutant mice: A role of oxidative stress. FASEB J. (2003) 17:50–2. doi: 10.1096/fj.02-0448fje
52. Abraham CR, Mullen PC, Tucker-Zhou T, Chen CD, Zeldich E. Klotho is a neuroprotective and cognition-enhancing protein. Vitam Horm. (2016) 101:215–38. doi: 10.1016/bs.vh.2016.02.004
53. Kale A, Sankrityayan H, Anders HJ, Gaikwad AB. Epigenetic and non-epigenetic regulation of Klotho in kidney disease. Life Sci. (2021) 264:118644. doi: 10.1016/j.lfs.2020.118644
54. Kim D, Lee S, Choi JY, Lee J, Lee HJ, Min JY, et al. Association of α-klotho and lead and cadmium: A cross-sectional study. Sci Total Environ. (2022) 843:156938. doi: 10.1016/j.scitotenv.2022.156938
55. Omoike OE, Pack RP, Mamudu HM, Liu Y, Strasser S, Zheng S, et al. Association between per and polyfluoroalkyl substances and markers of inflammation and oxidative stress. Environ Res. (2021) 196:110361. doi: 10.1016/j.envres.2020.110361
56. Yu D, Zhang L, Yu G, Nong C, Lei M, Tang J, et al. Association of liver and kidney functions with Klotho gene methylation in a population environment exposed to cadmium in China. Int J Environ Health Res. (2020) 30:38–48. doi: 10.1080/09603123.2019.1572106
57. Jain RB, Ducatman A. Serum concentrations of selected perfluoroalkyl substances for US females compared to males as they age. Sci Total Environ. (2022) 842:156891. doi: 10.1016/j.scitotenv.2022.156891
58. Roth K, Yang Z, Agarwal M, Liu W, Peng Z, Long Z, et al. Exposure to a mixture of legacy, alternative, and replacement per- and polyfluoroalkyl substances (PFAS) results in sex-dependent modulation of cholesterol metabolism and liver injury. Environ Int. (2021) 157:106843. doi: 10.1016/j.envint.2021.106843
59. Attanasio R. Sex differences in the association between perfluoroalkyl acids and liver function in US adolescents: Analyses of NHANES 2013-2016. Environ Pollut. (2019) 254:113061. doi: 10.1016/j.envpol.2019.113061
60. Costello E, Rock S, Stratakis N, Eckel SP, Walker DI, Valvi D, et al. Exposure to per- and polyfluoroalkyl substances and markers of liver injury: A systematic review and meta-analysis. Environ Health Perspect. (2022) 130:46001. doi: 10.1289/EHP10092
61. Quintero-Platt G, González-Reimers E, Rodríguez-Gaspar M, Martín-González C, Pérez-Hernández O, Romero-Acevedo L, et al. Alpha Klotho and fibroblast growth factor-23 among alcoholics. Alcohol Alcohol. (2017) 52:542–9. doi: 10.1093/alcalc/agx041
62. Halldorsson TI, Fei C, Olsen J, Lipworth L, McLaughlin JK, Olsen SF. Dietary predictors of perfluorinated chemicals: A study from the Danish National Birth Cohort. Environ Sci Technol. (2008) 42:8971–7. doi: 10.1021/es801907r
63. Eriksen KT, Sørensen M, McLaughlin JK, Tjønneland A, Overvad K, Raaschou-Nielsen O. Determinants of plasma PFOA and PFOS levels among 652 Danish men. Environ Sci Technol. (2011) 45:8137–43. doi: 10.1021/es100626h
64. Cho CR, Lam NH, Cho BM, Kannan K, Cho HS. Concentration and correlations of perfluoroalkyl substances in whole blood among subjects from three different geographical areas in Korea. Sci Total Environ. (2015) 512–3:397–405. doi: 10.1016/j.scitotenv.2015.01.070
65. Harada K, Saito N, Inoue K, Yoshinaga T, Watanabe T, Sasaki S, et al. The influence of time, sex and geographic factors on levels of perfluorooctane sulfonate and perfluorooctanoate in human serum over the last 25 years. J Occup Health. (2004) 46:141–7. doi: 10.1539/joh.46.141
66. Emmett EA, Shofer FS, Zhang H, Freeman D, Desai C, Shaw LM. Community exposure to perfluorooctanoate: relationships between serum concentrations and exposure sources. J Occup Environ Med. (2006) 48:759–70. doi: 10.1097/01.jom.0000232486.07658.74
Keywords: perfluoroalkyl and polyfluoroalkyl substances (PFAS), α-Klotho, NHANES, perfluorononanoic acid (PFNA), middle-aged women
Citation: Li M, Ma Y, Cheng W, Zhang L, Zhou C, Zhang W and Zhang W (2023) Association between perfluoroalkyl and polyfluoroalkyl internal exposure and serum α-Klotho levels in middle-old aged participants. Front. Public Health 11:1136454. doi: 10.3389/fpubh.2023.1136454
Received: 03 January 2023; Accepted: 11 April 2023;
Published: 04 May 2023.
Edited by:
Zhiwei Xu, The University of Queensland, AustraliaReviewed by:
Roberta Andreoli, University of Parma, ItalyAlan Ducatman, West Virginia University, United States
Copyright © 2023 Li, Ma, Cheng, Zhang, Zhou, Zhang and Zhang. This is an open-access article distributed under the terms of the Creative Commons Attribution License (CC BY). The use, distribution or reproduction in other forums is permitted, provided the original author(s) and the copyright owner(s) are credited and that the original publication in this journal is cited, in accordance with accepted academic practice. No use, distribution or reproduction is permitted which does not comply with these terms.
*Correspondence: Wenji Zhang, zhangwenji@gdaas.cn; Wenjuan Zhang, zwj2080@126.com
†These authors have contributed equally to this work and share first authorship