- 1Department of Pathological Sciences, Universidade Estadual de Londrina (UEL), Londrina, Brazil
- 2Department of Biological Sciences, Universidade Estadual do Norte do Paraná (UENP), Jacarezinho, Brazil
- 3Center of Health Sciences, Universidade Estadual do Oeste do Paraná (UNIOESTE), Blumenau, Brazil
- 4Université de Montréal (UdeM), Montreal, QC, Canada
- 5Instituto Nacional de Câncer (INCA), Rio de Janeiro, Brazil
- 6Instituto Federal Catarinense, Blumenau, Brazil
Introduction: Pesticides pose a risk for cancer development and progression. People are continuously exposed to such substances by several routes, including daily intake of contaminated food and water, especially in countries that are highly pesticide consumers and have very permissive legislation about pesticide contamination as Brazil. This work investigated the relationship among pesticides, food contamination, and dietary cancer risk.
Methods: Analyzed two social reports from the Brazilian Government: the Program for Analysis of Residues of Pesticides in Food (PARA) and The National Program for Control of Waste and Contaminants (PNCRC).
Results and discussion: First, we characterized the main pesticide residues detected over the maximum limits allowed by legislation or those prohibited for use in food samples analyzed across the country. Based on this list, we estimated the dietary cancer risks for some of the selected pesticides. Finally, we searched for data about dietary cancer risks and carcinogenic mechanisms of each pesticide. We also provided a critical analysis concerning the pesticide scenario in Brazil, aiming to discuss the food contamination levels observed from a geographical, political, and public health perspective. Exposures to pesticides in Brazil violate a range of human rights when food and water for human consumption are contaminated.
Introduction
Pesticides are a large and heterogeneous group of chemicals used primarily to destroy, repel, or mitigate insects, small animals, weeds, and other undesirable organisms. Chemically these substances are categorized as organochlorines, organophosphates, carbamates, pyrethroids, neonicotinoids, and phenylpyrazoles.
Most of them are considered persistent organic pollutants that can accumulate in the ecosystem and remain in the environment for considerable periods due to their lipophilic characteristic and long half-life (1–3).
Once in the environment, such pesticides can reach the human body through the daily ingestion of contaminated food and drinking water. This exposure may harm humans since these substances are associated with disease development. Neurodegenerative disease (4), respiratory pathologies (5), metabolic disorders (6), reproductive dysfunction (7, 8), and cancer (9, 10) has been linked to pesticides.
In countries whose economy is based on agriculture, this contamination poses a public health issue. In this context, Brazil is at the top of the world's biggest pesticide consumers (11) altogether to China and the United States. Agribusiness is one of the essential activities for the Brazilian economy. Expanding Brazil's export share has been one of the main objectives guiding the Ministry of Agriculture, Livestock, and Supply (MAPA) work (12).
Nonetheless, these active ingredients are not restricted to the production of agricultural commodities. They are commonly found in horticulture and fruit growing, as observed from the reports of the Program for the Analysis of Residues of Pesticides in Food (PARA), coordinated by the National Health Surveillance Agency (ANVISA). This monitoring investigates pesticide residues in food, observing their compliance with the Maximum Residue Limits—MRL allowed and the presence of active ingredients not authorized for a particular crop or banned in the country.
PARA is the most extensive study regarding monitoring the presence of pesticides in foods of plant origin in Brazil, as it has national coverage and all sample analyses are carried out by specialized laboratories. The program is essential, considering that from the results, it is possible to assess the scenario of irregularities and health risks in a country that consumes many pesticides. The activities of PARA began in 2001, and the main goal is to evaluate the levels of pesticide residues that reached the consumer's table. Since then, PARA has coordinated jointly with municipal and state health surveillance agencies and state public health laboratories (13).
Therefore, despite the pivotal role of fruits and vegetables in nutrition and preventing chronic diseases, consuming contaminated food may have critical consequences. As conventional food cultivation uses many pesticides, it poses a chronic risk for cancer development, for example, due to its carcinogenic potential and frequent presence over the maximum residual limits. Studies have developed tools to estimate the dietary cancer index that allows evaluation of the impact of acute and chronic consumption of pesticide-contaminated food on cancer risk (14).
Little information on the cancer risk attributable to food intake is available worldwide, and conflicting results have been reported (15–19). Also, more information is needed concerning the food-derived pesticide-attributable risks for large-scale populations, as in Brazil. In the present study, we investigated literature data about the relationship between food and risk and carcinogenic pathways, considering the main pesticides described in the last Brazilian PARA report. Further, we estimated the Pesticide Residue Index (PRSI) and revised the major mechanisms enrolled regarding its impact on cancer.
Methods
This study aims to comprehend the multiscale relationship between food contamination by pesticides and the cancer risk attributable to its ingestion. Therefore, it comprises three main parts:
1. The analysis of the pesticide food contamination data from the Brazilian PARA Report.
2. The estimative of the dietary cancer risk related to PARA reported food pesticide contamination.
3. A systematic analysis of literature concerning the consequences of this pesticide exposure.
The number of detections of active ingredients reported in PARA and the concentration detected in mg/kg in the vegetable samples were analyzed. From these data, samples that showed some pesticide concentrations were selected, and then the median per crop was applied. The percentage of pesticide residue detection in samples considered satisfactory by the Vegetal PNCRC was consulted in SDA Ordinance No. 448 of November 17, 2021, published in the Official Gazette of the Federal Government (20).
To assess the Pesticide Residue Index (PRSI), which represents the pesticide residues in a single serving, we used the original equation for Theoretical Maximum Daily Intake (TMDI) (Equation 1). Through some minor changes in the TMDI equation, a second equation was generated and applied to food samples to achieve the PRSI. The comparison between both equations showed us the specific foods and pesticides in these samples have higher than recommended pesticide residues.
For the systematic review of literature, data were obtained from studies available in three critical databases (PubMed, Google Scholar, and Web of Science) on pesticide exposure and its correlation with carcinogenesis. We restricted our search to articles published from 2012 to 2022. We used a combination of the following words in the title and abstract: pesticides, cancer, tumor, and carcinogenesis. Four authors reviewed titles, article abstracts to classify eligible articles, and full text if necessary. All of the included pieces were written and published in English. Animal, in vitro, cross-sectional, case-control, cohort, and ecological studies were included.
Results
Results of monitoring pesticides residues in food in Brazil: PARA report analysis
Aiming to understand the picture of food contamination in Brazil, we evaluated the results from the PARA report. The first cycle of the program comprised the period between 2001 and 2007 and analyzed nine types of products. The data showed that foods such as strawberries, tomato, and lettuce had the highest levels of unsatisfactory samples, reaching ~50% of sampling by culture. From 2008 onwards, the amount of food analyzed increased each year, reaching 36 different products in the cycle from 2017 to 2020, although, so far, only the first cycle of 14 varieties has been published (Table 1).
Sampling carried out between 2010 and 2018, on average, showed that 63% of the food samples contained some pesticide residue, indicating that most of the food consumed in Brazil has traces of active ingredients due to the spraying of these products. Of this percentage, 27%, on average, are considered unsatisfactory due to the risk they pose to human health. Furthermore, most samples are deemed inadequate because detected pesticides were unauthorized for the crop, which endangers farmers directly exposed to these products and food consumers (21).
The most recent report in Brazil about PARA (released in 2019) deals with the first phase of the 2017–2018 cycle. This cycle analyzed 4,616 samples and searched up to 270 active ingredients of pesticides. Residues of 122 different active ingredients were detected in the samples analyzed, resulting in a total of 8,270 detections.
The most detected pesticides were the insecticide imidacloprid (713 detections) and the fungicides tebuconazole (570 detections) and carbendazim (526 detections) (Figure 1A). Imidacloprid is among the 10 most commercialized pesticides in Brazil (22) and has been associated with the death of bees (23). For this reason, is prohibited in the European Union (24). Carbendazim has been banned in the United States and the European Union for more than a decade, in association with cancer and fetal malformations.
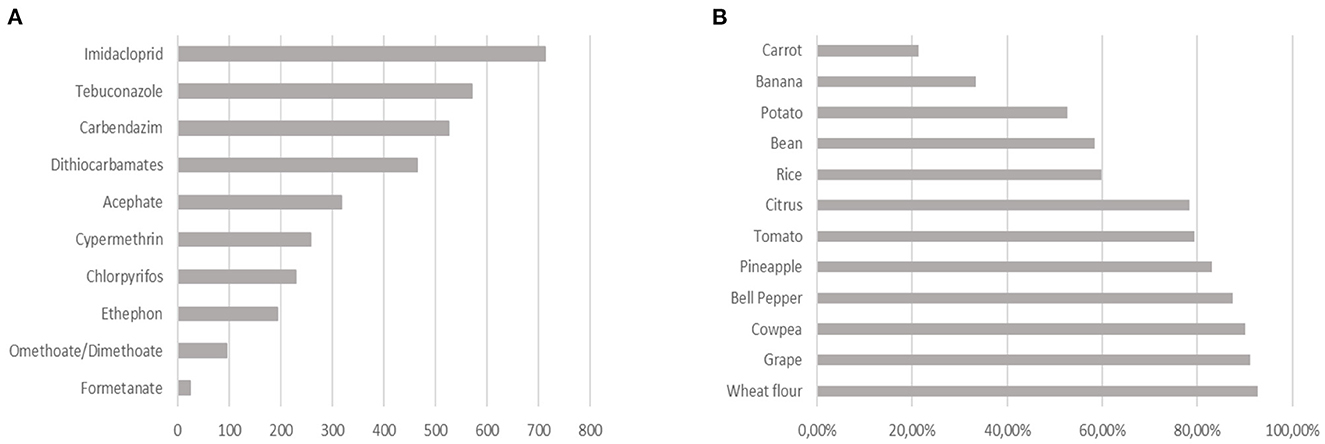
Figure 1. Recognition of pesticide residues according to PARA. (A) Main pesticide residues detected in PARA 2017–2018: total detected by active ingredient. (B) Percentage of pesticide residue detection about the parameters analyzed in the satisfactory samples of the PNCRC Vegetal 2020.
The foods that presented the highest number of unsatisfactory samples were: peppers (81.9%), guava (42.4%), carrots (39.6%), and tomato (34.8%). Of the total monitored, 41 samples from the 2017–2018 cycle (0.89%) had a potential acute health risk; of this amount, 27 were orange (Figure 2). In addition, 2.9% of the samples, corresponding to 134 units, had 10 or more active ingredients in the same food (13).
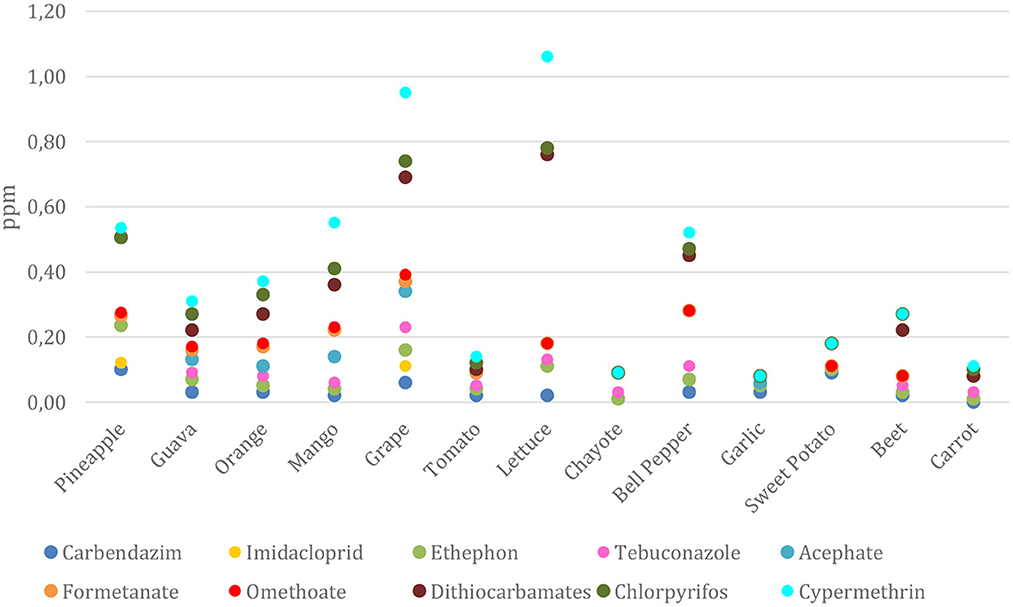
Figure 2. Main pesticide residues detected in PARA 2017–2018: median of values detected per culture (mg/kg).
Despite the advances, the number of samples analyzed in Brazil seems to be less than ideal, given that throughout PARA (2001–2018), 36,069 samples were analyzed, which represents a little more than a third of what was analyzed in the European Union, only in the year 2018. Another point is that Brazil has been much more permissive about the established MRLs and the pesticides that are used in the national territory, which have been banned for years in European Union countries, as is the case of carbendazim, chlorpyrifos, and acephate, which represents a framework of environmental injustice (25).
Another essential element presented in the reports is the multi-exposure; that is, the consumer, when eating, may be ingesting more than one pesticide at a time. This risk of combined action is not yet estimated in Brazil, but methodologies and pilot studies already exist in the European Union and the United States to guarantee consumer safety (26). This, therefore, is a crucial point for the improvement of PARA.
In addition to PARA, another program has been monitoring pesticide residues in plant samples in Brazil since 2008: the National Program for Control of Waste and Contaminants (PNCRC Vegetal), carried out by the Ministry of Agriculture, Livestock, and Supply (MAPA). The PNCRC/Vegetal has the function of monitoring the quality and safety of products of plant origin produced and consumed throughout the national territory concerning the occurrence of pesticide residues and chemical, physical and biological contaminants. Products of plant origin intended for the domestic and export markets are monitored. MAPA has carried out the PNCRC/Vegetal since 2008, and samples are preferably collected at processing establishments and/or packers, wholesalers, and supply centers. MAPA reports show that on average, 56% of the samples analyzed between 2015 and 2020 had some level of residue (27).
The most recent report, from 2020, shows pesticide residues in 67.17% of the total samples, highlighting the high frequency of residue detection for the following foods: cowpea, grapes, peppers, and wheat flour, which had more than 85% of the samples with the presence of pesticides (Figure 1B). Among the most detected active ingredients are carbendazim, chlorpyrifos, and acephate (20).
Dietary cancer risk estimative attributable to PARA reported pesticides residues in food
Based on the residues described in Tables 2, 3, we calculated the dietary cancer risk attributable to food contamination. The equation for the Theoretical Maximum Daily Intake (TMDI) (Equation 1) considers the Maximum Residue Limits (MRL) (28) to establish the highest level of pesticides legally tolerated on food or feed crops. For MRL, we only considered the compounds authorized for use in Brazil since the current guidelines of the National Health Surveillance Agency (Anvisa) do not cover unauthorized products. The daily intake of any particular pesticide residue in a given food is obtained by multiplying the residue level in the food (MRL) by the amount consumed (F).
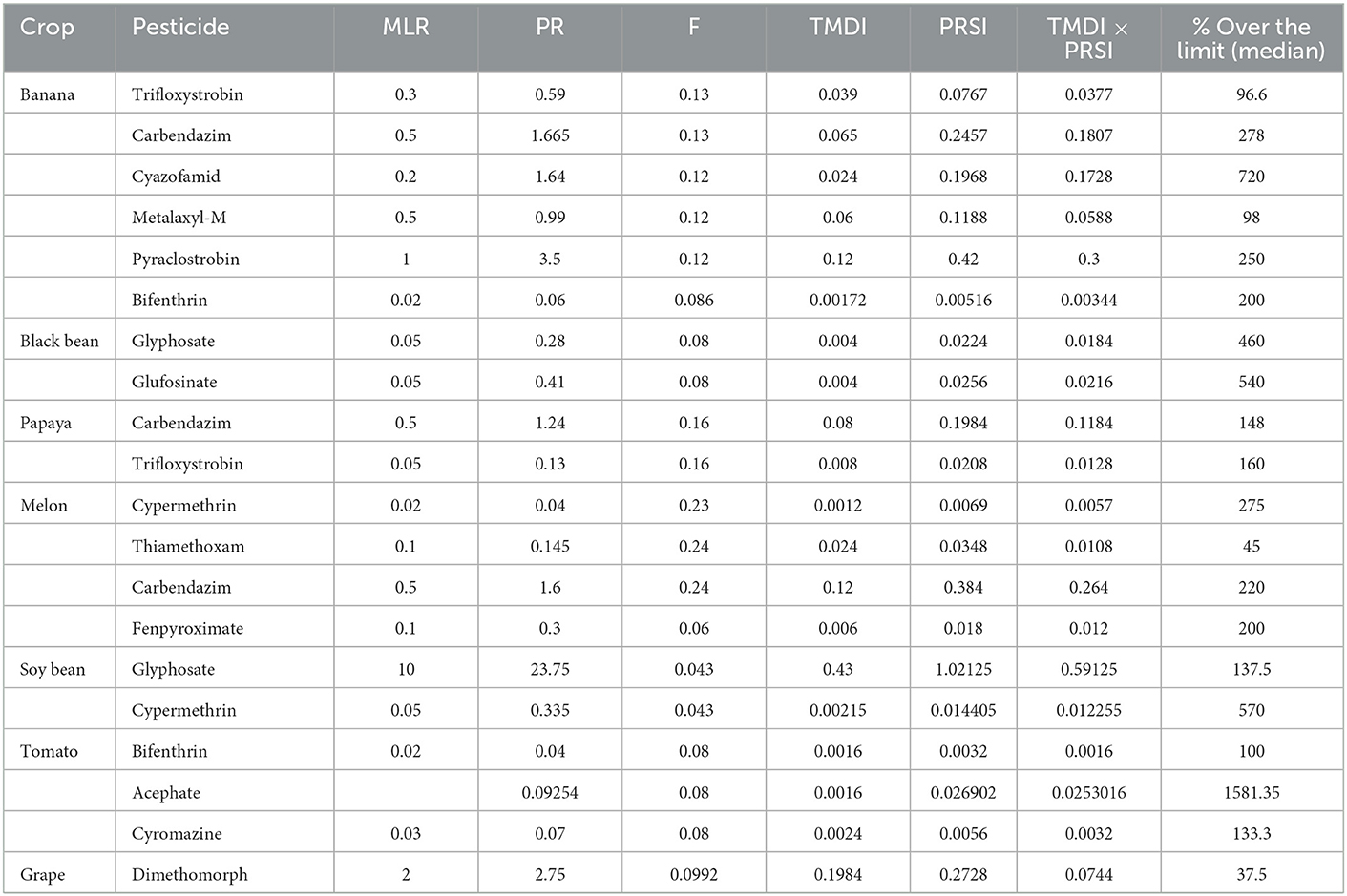
Table 2. Pesticide active substances percentages applied over the limit in agricultural crops according with TMDI and PRSI values in 2019.
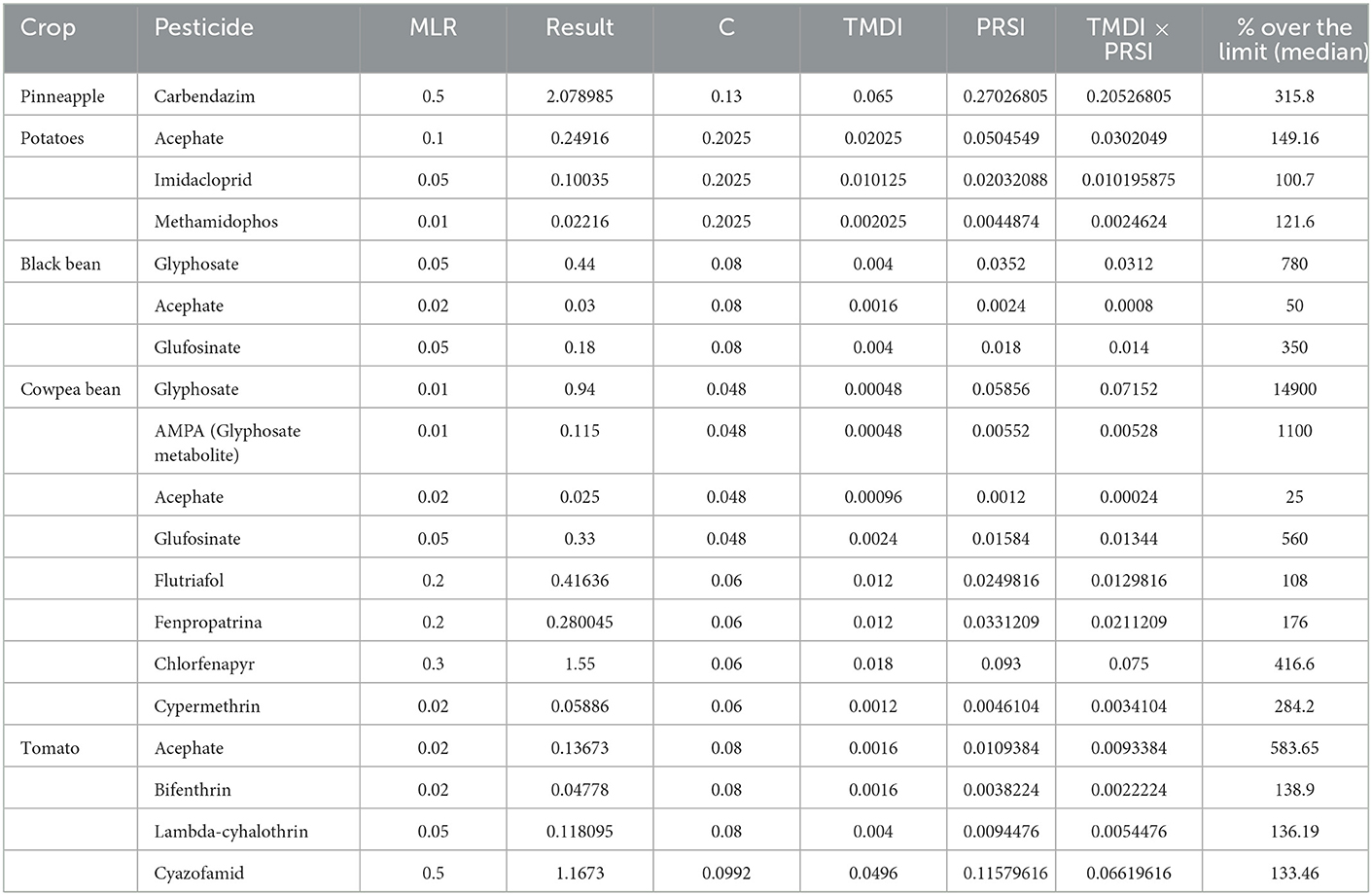
Table 3. Pesticide active substances percentages applied over the limit in agricultural crops according with TMDI and PRSI values in 2020.
Equation 1. Original equation:
TMDI = Theoretical Maximum Daily Intake;
MRL = Maximum Residue Limits (in ppm or mg.kg−1); and
F = Recommended food serving size (in mg).
We evaluated both the TMDI and the Pesticide Residue Sample Index (PRSI) for 44 pesticides applied in crops in 2019 and 33 pesticides used in 2020 (Tables 4, 5). PRSI is an adaptation of the original equation (Equation 1) by replacing MRL with accurate pesticide measurements (PR) from all available crops to identify pesticide contamination (PRSI, Equation 2) in food and/or food crops in different Brazilian regions.
Equation 2. The equation to identify accurate pesticide contamination:
PRSI = Pesticide Residue Sample Index;
PR = Pesticide residues measured in agricultural crops of different Brazilian regions; and
F = Recommended food serving size (in mg), according to the Dietary Guidelines for the Brazilian population (77).
Upon the TMDI equation, we could identify values that were used for comparison with PRSI results in food or food crops potentially consumed by the Brazilian population. To estimate food consumption (F), we used the Dietary Guidelines for the Brazilian people, established by the Brazilian Health Ministry (77), in which food serving portions are recommended. The data on the PR found in the crops were evaluated for each pesticide-active substance.
For comparison purposes, we observed the difference between the TMDI and PRSI from several crops in Brazil in 2019 and 2020. This difference is demonstrated in a percentage higher than the limit tolerated (% higher than the tolerated column, Tables 2, 3). We found that in 2019, all 46 crops analyzed had higher active substance residues than advocated by law, and 38 of these crops displayed 100% more residues than tolerated. The numbers are even worse for 2020 crops, as from 104 crops evaluated, 102 showed 100% more residues than allowed. These findings should be awareness-raising, as several food crops in Brazil were found with increased pesticide residues, often overpassing 1,000%.
It emphasizes the adverse effects that public policies demeaning can have, as Brazil is going against the world and has been increasing the number of legally pesticide-active substances in the country. The draft bill PL 6299/2022 (78)—more widely known as the “poison package,” is an example, already approved by the parliament and waiting to be voted on by the senate (According to the project PL 6299/2002). The demeaning of these public policies not just impacts the overuse of these compounds but also the indiscriminate application of them, as we observe a lot of active substances applied in Brazilian crops are banned for crop use, according to the country's legislation (Supplementary Tables S1, S2).
One example of the importance of sound policies related to pesticides is the latest European Food Safety Authority report, where more than 88.000 food samples produced in 2020 were analyzed, and 94.9% of the samples were within legally permitted levels (79).
Therefore, the dietary risk assessment analysis suggests that the food commodities analyzed are unlikely to concern consumers' health.
The most widely used chemical herbicide is N-(phosphonomethyl) glycine, commonly known as glyphosate (80). In our database, we observed glyphosate as the most used pesticide, applied in eight different regions in 2019 and 43 crops from other areas in 2020, followed by glufosinate. Glyphosate was also the main pesticide used irregularly, i.e., above the maximum allowed residue levels. Economically, this herbicide is popularly sold under the name of Roundup. As a broad-spectrum herbicide, it is used in agriculture and forestry, representing one of the most important chemical compounds in use since its release. Although it is less bioavailable than other herbicides, glyphosate residue levels may represent a risk to consumers depending on several factors, such as the application technique, water quality, and environmental conditions (81).
Glyphosate is considered “safe” because neither its active substance nor its primary degradation product, aminomethylphosphonic acid (AMPA), is associated with any known adverse effect on human health. However, there is a controversy in the literature regarding its carcinogenic potential, as some studies describe its potential to cause endocrine and/or microbiome disruption (80, 82, 83). Besides, glyphosate exposure can also induce epigenetic modulation, such as decreasing global DNA methylation and promoting histone modification, as reviewed elsewhere (84).
Carbendazim (methyl 2-benzimidazolecarbamate) was also detected above the maximum allowed residual level in different crops in 2019 (pineapple, lettuce, papaya, and pear) and 2020 (pineapple). The Brazilian National Health Surveillance Agency (Anvisa) decided to ban the use of carbendazim in 2022, as it was considered carcinogenic (85). Carbendazim is a systemic fungicide that inhibits microtubule polymerization in cells by acting with β-tubulin (86). This inhibition disrupts the microtubule assembly and leads to impaired segregation of chromosomes during cell division, inducing mitotic arrest (87). Organophosphorous pesticide exposure can cause severe systemic and central nervous system disturbances, primarily associated with inhibiting acetylcholinesterase activity (88). Acephate (O, S-dimethyl-acetyl-phosphoramidothioate) and methamidophos (O,S-dimethyl phosphoramidothioate) are two of the most common and efficient OPs used in agriculture. Acephate is classified as a class II “moderately hazardous” pesticide, and methamidophos is classified as a class Ib “highly hazardous” pesticide (89). Acephate is prohibited in tomato crops but was detected in several samples evaluated. Methamidophos is the toxic metabolite of acephate (90). Despite being banned from Brazil since 2012, we observed contamination above the limits of methamidophos in potato crops in 2020. Both active substances have their use restricted or prohibited in the European Union due to their harmful potential, however, we still found residues of these substances, even under restriction or prohibition by law, in food, water, and crops in Brazil.
Cancer risk evidences for PARA and PNCRC reported pesticides residues
Literature screening
Concerning the literature review, we conducted a literature search using the R software (91) with the bibliometrix package (92) to check the terms “pesticides” and “cancer” and “tumor” and “carcinogenesis” in the PubMed database. The search retrieved 174 articles that met our criteria. These terms are appearing more and more in high-impact journals that are devoted to toxicology or cancer studies (Supplementary Figure S1A). Furthermore, an increase in the number of publications dealing with “cancer and pesticides” is evident in recent years (Supplementary Figure S1B).
Brazil is in the top 10 when we look at the countries with higher article production in the last 10 years; the publication rate is increasing in all countries. The USA and China are the countries with the most significant number of publications. Brazil, in this ranking, occupies ninth place with 43 articles available in the PubMed database (Supplementary Figure S2A). Also, a large number of collaborations between different countries to study the topic in question is striking, thus evidencing the concern with the relation between pesticides and cancer (Supplementary Figure S2B).
The trend topics are diverse, but the terms “exposure,” “cancer,” “human,” “pesticides,” and “carcinogenesis” are highlighted. Besides, there is a direct link among each other, meaning the co-occurrence of those terms. These terms were searched for in the titles of the articles (Supplementary Figure S3).
Selected data are discussed in the following topics.
Human exposure data
Most of the pesticides reported in PARA and PNCRC are classified by IARC as possibly, potentially or proven carcinogenic (Supplementary Table S3), the most used pesticides as active ingredients and basic formula, chemical group, class, agricultural use, classification according to EPA, classification according to IARC, and classification according to WHO (89).
The mechanism of action of pesticides on target-specific pests is well-established in the literature (93–95), but the action of these compounds on human health is a reasonable investigation and needs to be elucidated. The association with human diseases, including cancer, when exposed to pesticides is already well-established. Still, the mechanisms by which these compounds are responsible for human carcinogenesis must be better understood.
The carcinogenic process can occur gradually, taking several years for a single cancer cell to develop and give rise to a tumor. For tumor development, the cell goes through several phases of growth and adaptation, which can be synthesized in three stages: initiation, promotion, and progression (96).
Initiation is the first phase of tumor development. At this stage, the initiator molecules (carcinogenic) meet the cellular microenvironment and lead to DNA damage, which is not adequately repaired, thus establishing mutations. The greater the exposure to these initiator molecules, the greater the risk of tumor development (97). Promotion is the second phase, affecting cells that have already started (mutants). Promoting agents have the role of increasing the proliferative rate, creating a more significant number of mutation-bearing cells. Promoting agents do not directly affect DNA but cell receptors, leading to the alteration of signaling pathways and increased cell proliferation. Promoters can be further divided into two categories: specific promoters, which interact with receptors on target cells, and non-specific promoters, which alter gene expression without the involvement of a known receptor. Promoters do not lead to the formation of tumors alone; they only increase the cellular expansion of cells already initiated, thus leading to the formation of tumors (98).
The third and final phase of carcinogenesis is cell progression. This phase is associated with changes in the cell genotype, an increase in the rate of proliferation, invasive and metastatic capacity, biochemical (glycolytic pathway and oxidative phosphorylation), and morphological changes (99). At this level of development, tumor formation is irreversible.
IARC has been assessing the carcinogenic risk of pesticides to humans and has critically evaluated monographs on individual chemicals, classifying them into risk cancer categories (IARC53). Carcinogenic risk means the probability that an agent will lead to cancer (neoplasm or tumor) in humans exposed to it. Carcinogen denotes an agent or mixture capable of increasing the incidence of malignant neoplasms. Assessment of carcinogenicity is based on evidence from epidemiological studies, depending on variability over time and location of mixtures, processes, occupations, and industries.
Human exposure to these compounds occurs acutely or chronically and can occur through the skin, respiratory and oral routes, food, water, or accidental ingestion (Table 4) (2, 6, 152). In addition, people may be in direct contact with pesticides during the preparation and use of pesticides and/or indirectly through breathing residual concentrations in the air or exposure to residues found on surfaces, food, and dust (100). Children are vulnerable to pesticides because of their physiological and behavioral differences compared to adults, such as hand-to-mouth exposure (101).
Considering the pesticides described in the PARA and PNRCN reports, we bring some information about their cancer-related effects. Parental occupational exposure to pesticides, such as permethrin, acephate, phosmet, and propiconazole, cause changes in their germ cells. It has been related to an increased risk of developing cancer in childhood, such as acute lymphoblastic leukemia, retinoblastoma, central nervous system tumors, and cell tumor testicular germ cells in adolescence (29, 30, 43, 49, 52). A study carried out by Lombardi et al. (40) related dimethoate and propiconazole to an increased risk of developing medulloblastoma in children whose mothers were exposed to the action of these pesticides during pregnancy (40).
The risk intensifies when the mother is exposed during pregnancy and in the first years of the child's life through contamination by air, dust, and clothes used by the parents when applying pesticides, food, and even breast milk. Exposure does not need to be high or extended because their physiological characteristics make them more susceptible to the effects on their body (102). Among these characteristics, the following can be mentioned: absorption through the skin, which is more intense due to the weight/body surface ratio; greater inhalation due to its respiratory rate and ventilation per minute; higher intake of contaminated food and water per body weight compared to adults and incomplete metabolism causing toxicity to the organism (103).
In human studies, aminomethylphosphonic acid (AMPA), chlorpyrifos, and imazalil were positively associated with the risk of breast cancer (18, 32, 37). It is known that some pesticides accumulate in adipose tissue and can act in the body as endocrine disruptors, having estrogenic effects, which is one of the critical factors that contribute to the development of breast cancer. They can influence the synthesis, transport, metabolism, and elimination of estrogen, disrupting the body's normal homeostasis (37).
Organochlorines act as alpha estrogen nuclear receptor agonists, promoting cell proliferation and tumor progression. Other pesticides promote the activation of cytochrome P450 (CYP) member, CYP19 alpha-aromatase enzyme in adipose tissues, indirectly contributing to the increase of estrogen in peripheral tissues and the intratumoral environment (104). Another mechanism would be related to the interaction with aryl hydrocarbon receptors (AhR). This transcription factor regulates enzymes that participate in the metabolism of xenobiotics belonging to the CYP family. This alteration would lead to the accumulation of adducts in the DNA, one of the factors linked to breast carcinogenesis (105).
A positive association was found between chlorpyrifos exposure and lung cancer incidence (38). Other pesticides, such as acetamiprid, clothianidin, and thiamethoxam, were associated with a higher risk of liver cancer (31). In a case-control study carried out with rural workers exposed to pesticides, the tumor biomarkers p53, alpha-fetoprotein, and alpha L-fucosidase were at higher levels when compared to the unexposed control group. Further, the shorter length of the telomeres and decreased telomerase activity were associated with increased DNA damage (106).
There was a link between the increased risk of developing non-Hodgkin's lymphoma and human exposure to glyphosate (44, 45). Several mechanisms triggered by this exposure may contribute to the onset of the disease, such as immunotoxicity, genotoxicity, and hormonal effects (107). Chromosomal aberrations, such as translocations, would be one of the critical effects caused by pesticides in this type of tumor, favoring the overexpression of oncogenes and thus promoting cell proliferation (108).
The use of permethrin has been linked to the occurrence of multiple myeloma (50, 51, 55). Permethrin can act directly on the progression of the disease by having an immunomodulatory effect or, in the same way, lead to monoclonal gammopathy of undetermined significance, which increases the risk of developing multiple myeloma (109). In the study by Shearer et al. (55), a change in myeloid lineage cells was observed, including immature granulocytes and red blood cells. Consequently, the exacerbated presence of immature granulocytes suppressed the antitumor immune response and favored tumor angiogenesis (55).
Individuals exposed to glyphosate, phosmet, and permethrin are more likely to develop leukemia, and the exposure of pregnant women also increases the chances of their children presenting the disease (43, 46, 52). Some pesticides, such as permethrin, can lead to chromosomal rearrangements, which may later inactivate the topoisomerase 2 or cause oxidative stress, promoting breaks in DNA double-strand (49, 110). The presence of polymorphisms in enzymes such as the glutathione S-transferase and CYP450 families alter their normal functioning, compromising the metabolism of xenobiotics, which may also contribute to increased susceptibility to leukemia (111). Chlorpyrifos and carbofuran were associated with an increased risk of prostate cancer in exposed men (34, 36).
Colorectal and renal tumors were associated with a greater chance of developing in workers exposed to the pesticide chlorpyrifos (35, 39). In turn, astrocytoma had a greater chance of occurrence in those exposed to methyl-Kresoxim (40).
Occupational exposure to omethoate demonstrated changes in the length of telomeres (48). The telomeric region of the chromosome is responsible for preventing the degradation of the final portion of chromosomes and end-to-end chromosomal fusion, ensuring genome stability during cell divisions. Changes in this region contribute to several diseases, including cancer, due to oxidative stress and immunotoxicity that generate DNA damage (112).
In vitro and in vivo data
Pesticide-induced effects have been the subject of many published in vitro and in vivo studies aimed at expanding the scientific basis of current risk assessment procedures by allowing a better understanding of the mechanism of chemical-induced toxicity and its safety levels. These experimental studies show that pesticides alter DNA, leading to mutations and chromosomal aberrations that lead to the development of cancers and other diseases (2). The articles in our search that address in vitro and in vivo studies used for this review are listed in Tables 5, 6.
Some pesticides act directly on receptor expression and hormone secretion. The secretion of estrogen is one of the main pathways affected, suggesting a higher risk for women exposed to these pesticides. Imidacloprid and thiamethoxam, pesticides from the neonicotinoid class, increase the expression of the aromatase enzyme cytochrome P450 19 (CYP19), the key to the stimulation of estrogen biosynthesis. This increase is directly related to the increased proliferation of cell lines such as breast cancer lineage Hs578t and adenocarcinoma lineage H295R (72). Cyfluthrin, chlorpyrifos, and glyphosate, the most widely used pesticide globally, act similarly. These increase estradiol (E2) synthesis in adrenocortical carcinoma (H295R) and breast cancer (T47D) cells and increase cell proliferation via the estrogen receptors ERα and ERβ. These results indicate that even low concentrations and environmental levels of pesticides cause increased estrogen levels, which, at high levels, are related to potential risk factors for developing, especially, breast cancer in women (58, 59, 61, 66).
There are indications from studies in animal models that pesticides also act on androgen receptors and on specific factors that stimulate the development of liver neoplasms, such as cyproconazole and propiconazole, from the class of conazoles. These pesticides act as essential mediators for increased hypertrophy and tumor initiation, the constitutive androstane receptor (CAR). Along with these pesticides, imazalil, permethrin, and methidathion act on the liver. These increase hepatocyte proliferation; imazalil, by increasing the expression of transforming growth factor alpha (TGF-α) and genes of the cytochrome p450 family, such as Cyp3a11, a target of the pregnane X receptor (PXR), which has its expression increased in liver carcinogenesis or other adverse events in the organ. In contrast, methidathion and permethrin stimulate liver cell proliferation in a PXR and CAR receptor-independent manner (114, 115, 118, 119, 122, 123, 125, 127).
Other mechanisms may also be responsible for changes in cell proliferation, such as changes in the cell cycle and in the expression of factors linked to tumor progression. Omethoate and cypermethrin alter the cell cycle of hypopharyngeal carcinoma (FaDu) and ovarian cancer (BG-1) lineages. These pesticides activate the Akt/GSK-3β/cyclin D1 signaling pathway and regulate the cyclin D1 gene, which is responsible for the transition between G1-S phases of the cycle; thus, the cell cycle is, in turn, stimulated, resulting in increased cell proliferation (60, 70). Cypermethrin also promotes, in mice, macrophage class switches from M1 (pro-inflammatory) to M2 (anti-inflammatory) that act by inhibiting effector T cells. This modulation can promote lung tumor progression (113).
The pesticide triflumuron, via hypoxia-induced factor 1α (HIF-1α), induces, in hepatocellular carcinoma (HepG2) cells, migration, invasion, and metastasis. Interestingly, this is the first time HIF-α is responsible for promoting these changes in this type of cancer (76). Another factor influenced by pesticide exposure is vascular endothelial growth factor A (VEGF-A). Increased VEGF-A levels in MCF-7 and MDA-MB-231 breast cancer cells increased specific parameters such as angiogenesis, migration, and cell invasion in these cell lines after chlorpyrifos exposure. These findings reinforce the role of angiogenesis in breast cancer progression, and that pesticide exposure contributes to this process (62, 63).
Oxidative stress is one of the mechanisms involved in the process of carcinogenesis already established, according to the literature, in several types of cancer, including childhood leukemias. In this sense, studies have been carried out to understand if there is any influence on pesticide exposure and the generation of oxidative stress. Thus, an investigation conducted with lung cell line A549 observed that the pesticide chlorpyrifos could generate oxidative stress in these cells by activating the NRF2 pathway, a transcription factor. Although NRF2 plays a role in decreasing oxidative stress and inflammation, it has been shown that in some cancers, this factor enables malignant cells to undergo metabolic changes leading to rapid proliferation and, therefore, tumor growth, and this is a possible survival mechanism for tumor cells (64, 129, 130).
Another study involving the pesticide Triflumuron was conducted experimentally in animals and HCT 116 cells. This work aimed to evaluate the genotoxicity of this chemical in the models chosen for the experiment. They observed that triflumuron induced the generation of reactive oxygen species, followed by lipid peroxidation, due to increased levels of malondialdehyde, a pro-oxidative parameter, and activating the antioxidant enzymes, catalase, and superoxide dismutase, in human colon tumor cells (HCT 116). These studies suggest that exposure to these substances, even at low concentrations, can induce oxidative stress, a well-established carcinogenic factor in cancer pathophysiology, including a marker of therapeutic response (75, 129).
Other pesticides have shown pro-carcinogenic effects in animal models. For example, in the zebrafish model, the pesticide pyraclostrobin affected apoptosis-related pathways, cancer, and membrane components, leading to mitochondrial dysfunction and cell apoptosis. It is because it induced the production of reactive oxygen species (ROS) and increased the activity of antioxidant enzymes such as catalase (CAT) and superoxide dismutase (SOD). These findings portend the need for further research into pesticide toxicity in aquatic models (120).
It was also observed once the increase of oxidative parameters, such as MDA, in rats exposed to the insecticide pyraclostrobin and the decrease of antioxidant defenses, DNA damage, and histopathological analysis was also observed in the kidneys and liver of these animals (121).
Among pesticides, glyphosate is a widely used herbicide worldwide. Many researchers aim to understand the relationship of this herbicide with cancer because the product is cytotoxic, even at low concentrations and a short duration of exposure. Stur et al. studying a cell line treated with roundup (composed of glyphosate and surfactants) observed that this compound can induce the production of reactive oxygen species by altering cellular metabolism and mitochondrial oxygen consumption, leading to a sequence of events that culminates in cell death. Oxidative stress is one of the pathways altered by glyphosate, but other pathways suffer interference and are also the target of studies (67, 131).
Exposure to pesticides can also induce the expression of genes involved in carcinogenesis. However, it remains unclear which genes and the mechanism responsible for their triggering, so to elucidate which pathways are stimulated, both in vitro and in vivo studies are carried out (132).
For example, studies carried out with glyphosate demonstrated through animal and in vitro experiments the pathways related to the development of the investigated cancer. In the case of exposure to small doses of glyphosate (0.05%) in vitro in the breast cancer cell line MCF-7 and MDA-MB-231, dysregulation of 11 canonical gene pathways was observed. The most essential included cell cycle and DNA damage repair and accumulating mutations, once again demonstrating the role of pesticides in mutagenicity by generating stress and cell cycle dysregulation (67).
While an experiment was carried out in an animal model, in this case with female Sprague-Dawley rats, a change in the expression of liver genes was observed, reflecting the activation of the TP53 gene due to the damage caused to the DNA. Furthermore, there was a decrease in the expression of miR-30 and an increase in the expression of miR-10. Dysregulation in the expression of microRNAs can alter the expression of target genes and disrupt cellular pathways. DNA base methylation is another modification capable of influencing gene expression, and this mechanism was also changed by glyphosate methylation (69). The work carried out by Wang et al. verified that MYC mice treated with glyphosate showed benign monoclonal gammopathy, anemia, and increased plasma cells in the bone marrow and spleen. Such findings place pesticides as a potential risk factor for developing multiple myeloma and non-Hodgkin lymphoma (116).
In research carried out in vitro to analyze the consequences of exposure to permethrin em ETV6-RUNX1 and IGH-BCL2 genes in K562 cells (chronic myeloid leukemia cells), induction was found of breakage and fusion of the damaging genes associated with lymphoma development (71). Furthermore, permethrin exposure induced numerical aberrations frequently observed in the metaphase phase (49).
Other results from in vitro and in vivo exposure to pesticides evaluated in the present review are shown in Tables 5, 6.
Perspectives and conclusions
Some considerations need to be pointed out about PARA reliability and data validity. Among the positive points of PARA, it should be noted that since its implementation in 2001, the program has been expanded in four dimensions: the number of participating states, number of samples analyzed, types of food analyzed and number of active ingredients researched (21). Although there was no standardization in the presentation of results from the beginning, the reports proved to be more detailed and complete. In the case of the Vegetal PNCRC, there have also been advances, especially from 2019 onwards, when the Ministry of Agriculture, Supply, and Livestock, through inspection actions, began to fine irregularities (133). However, the reports are still strictly technical, issued through ordinances, and not very accessible to the general population.
Considering that Brazil is among the three countries that use pesticides in the world, as well as the significant increase in the number of concessions for registration of pesticides in the country from 2016 onwards (134), official surveillance institutions should pay greater attention to the problem, especially in which refers to the contamination of food by these agrochemicals. The PARA and PNCRC Vegetal methodologies still need to be improved to ensure transparency and transmit greater security to the consumer.
In this sense, in the case of PARA, the number of samples is still low compared to other countries, such as the European Union. Recently, it has involved only 1.38% of Brazilian municipalities, 77 out of 5,568 (13). Another point to be highlighted is that Brazil's two most commercialized active ingredients (glyphosate and 2,4-D), widely used in the production of monocultures, only entered the analysis from 2016 onwards. On the other hand, glyphosate is one of the most detected pesticides in the Vegetal PNCRC, mainly in bean samples (20).
Brazilian researchers have also questioned the fact that the multi-exposure risk assessment is not adopted (135–137) since the reports by PARA and PNCRC Vegetal indicate samples contaminated by more than one active ingredient. Thus, the effects that add up and potentiate should be considered in methodologies for analyzing pesticide residues in food.
It is noteworthy that the publication and dissemination of results could be more problematic in the reports. The focus is on the absence of danger, disregarding that more than half of the total samples have some pesticide residue. Thus, if the sample is considered “satisfactory” for the Brazilian MRLs (which are highly permissive), the impression is that Brazilians are purchasing foods that are perfectly suitable for consumption and are also healthy. It is also not usually publicized that not all active ingredients approved for use in Brazil are monitored. In addition, in 2020, the PARA was suspended due to the COVID-19 pandemic, and no results were released after the 2017–2018 cycle.
It is very important to point out that the exposure to pesticides in Brazil is continuous. It occurs directly for farmers who frequently (138) handle these products and indirectly, through the drift of active ingredients to neighboring areas, as well as the contact of farmers' wives and children with different amounts of pesticides, by having contact with clothing used for work, and even pesticide packaging (139, 140). Children are vulnerable to pesticides because of their physiological and behavioral differences compared to adults, such as hand-to-mouth exposure (101, 141). Urban dwellers are also affected, as the urban water supply and many commercialized foods are already contaminated with pesticides (142).
There is no provision in the Brazilian legislation about the review process of the registration of authorized pesticides, and even today, products banned in other countries are used. Decree No. 4.074/2002 (143) recommends that this review could, in theory, occur at any time, guided by international alerts, new scientific studies, or complaints made by reference institutions under its subsection VI, art. 2. It is also noticed that, even in cases of international alerts, the limited resources available in the agencies or the lawsuits filed by corporations linked to agribusiness, not rarely end up hindering and delaying such reviews, worsening the exposure of the population to pesticides (144).
The MRL is defined as the maximum amount of pesticide residue officially accepted in food as a result of proper application at a specific stage, from its production to consumption, expressed in parts (by weight) of the pesticide or its residues per million parts of food (by weight) (ppm or mg/kg) (145). As for the levels of residues contained in food, they must be below the MRLs, established as references after conducting the necessary toxicological studies. In this context, the issue of maximum residue levels (MRLs) is one of the most relevant for food safety in trade negotiations between countries and companies.
When analyzing the PARA reports, one point that draws attention is that there is a category in which the samples are considered satisfactory when they present pesticide residues within a maximum residue limit pre-established through federal government regulations and the Codex Alimentarius. In general, 30–40% of the samples analyzed in each report fall into this classification. However, setting these limits ends up disregarding essential factors such as the joint action of several chemical compounds acting simultaneously in the human body (146), differences in susceptibility according to age and genetic factors, and the effects of chronic exposure (33).
Pesticide exposures in Brazil violate many human rights of the population. The right to life is potentially violated when pesticides contaminate food and water for human consumption. Bodies become ill (147), and the biodiversity of ecosystems is also threatened.
The Brazilian Constitution provides in its article 225 (148) that everyone has the right to an ecologically balanced environment, an asset for shared use by the people and essential to a healthy quality of life, imposing on the government and the community the duty to defend and preserve it for present and future generations. However, Brazil has adopted a position contrary to several countries that start from the precautionary principle concerning pesticides, such as those belonging to the European Union.
Approximately 80% of the pesticides authorized in Brazil are not permitted for use in at least three countries of the Organization for Economic Cooperation and Development (OECD), including countries with agriculture as an essential economic activity. Australia has 40% of its agricultural territory, a similar condition to Brazil, and no records of 114 active ingredients of pesticides allowed in the Brazilian territory were found. Although Brazil and India have relatively close soil and climate conditions, more than 50% of the pesticides that are registered in the first country are not allowed in the second, and the list of active ingredients of pesticides authorized in Brazil includes examples with recognized toxicity on human health and the environment. It extends to the 279 active chemical ingredients for agricultural use registered in Brazil with their regulatory status in the European Union, the United States, Canada, and Japan, which exposes massive differences. While in the European Union, 136 substances registered in Brazil are approved (143 are not approved), in the United States and Canada, 218 substances are approved. In Japan, 205 active ingredients registered in Brazil are approved (149).
Among the most used pesticides in Brazil, glyphosate stands out. In Brazil's regulations, glyphosate has a maximum residue limit of 1 mg/kg in coffee and sugar cane and 10 mg/kg in soy, corresponding to 10, 20, and 200 times the values allowed in the European Union for the same foods. In the human body, glyphosate is detected in blood, breast milk, and urine, with urinary levels in the general population of 0.16–7.6 μg/L, while in the occupationally exposed population, it is 0.26–73.5 μg/L (150, 151).
The European legislation establishes rules for the use and limits of pesticide residues and practices to be incorporated in the member countries of the European Union to gradually reduce the use of pesticides, as well as the use of alternatives that replace the use of chemicals, aiming to protect human and animal health and the environment. These limits are also extended to countries that intend to export to the European Union.
Currently, Bill 6.299/2002 (78) is being processed in the National Congress, already approved in the House of Representatives, which aims to further relax the legislation on pesticides (152) by facilitating the registration of active ingredients known to be prohibited in other countries, among other serious proposals that favor the indiscriminate use. Among the proposed changes is removing the registration prohibition criteria for potentially carcinogenic agents, toxic to the reproductive system, endocrine disruptors, and teratogenic agents, which are currently similar to the requirements adopted in Europe. With the changes, the use of substances associated with these effects may be permitted, subject to risk assessment. In Europe, there is also pressure on this provision. Still, studies have shown that the supposed economic losses would not be more significant than the health costs, loss of individual quality of life, deaths, and reduced productivity due to absenteeism, among others (153). In addition to its various effects, endocrine disruption indicates prohibition in the European Community. However, this device meets resistance to being fully implemented due to the controversies and doubts produced by the economic sectors to define the criteria for this classification, common strategies regarding the regulation, and use of toxic substances (154).
It is important to note that this bill is being processed even after opposing manifestations of Brazilian official technical bodies (155) such as the Brazilian Institute of Environment and Renewable Natural Resources, the National Health Surveillance Agency, the National Cancer Institute, and the Ministry of Labor.
The observations and recommendations of entities linked mainly to health and the environment were ignored by 2/3 (two-thirds) of the parliamentarians of the House of Representatives, who voted in favor of the continuity of the bill's passage (according to project PL 4166/12). It shows that economic interests, high productivity, and profitability are prioritized to the detriment of the population's quality of life.
Regarding food contamination, there is no direct association in the literature between exposure to pesticides and cancer development. However, pesticides such as glyphosate can cause disruptions in several biological pathways that may be linked to carcinogenesis. Glyphosate was our evaluation's most widely applied active ingredient on crops during 2019 and 2020.
Contact with glyphosate can occur via the oral, respiratory (pulmonary), or dermal route (156). The dermal route is the complaint of workers exposed to glyphosate by the absorption route of this element (157). Its accumulation in the body is found mainly in the liver, kidneys, colon, and small intestine, and its excretion happens through about 90% in the feces and within 48h in the urine (156). Importantly, even with many studies already confirmed and still being investigated, the ubiquitous cause of glyphosate and its health safety is of great concern (158).
In 2015, the International Agency for Research on Cancer (IARC), part of the World Health Organization, published its carcinogenicity assessment of glyphosate, concluding that this pesticide would likely be carcinogenic to humans (group 2A) based on limited epidemiological evidence in humans, primarily for non-Hodgkin's lymphoma, and significant evidence of carcinogenicity in animals (159, 160), operating through two critical pathways of known human carcinogens, specifically genotoxicity and oxidative stress induction.
Much research verifies glyphosate use and cancer incidence (46). The IARC evaluation of glyphosate resulted in intense opposition from the pesticide industry and led to many industry-sponsored articles and analyses on this subject (161–169). Notably, two of these studies were conducted in communities that had contact with this herbicide through aerial spraying, and caused DNA damage (170) and micronuclei (171).
Subsequently, the European Food Safety Authority (162) and the US Environmental Protection Agency (172) also reviewed this issue. They found that glyphosate is probably not carcinogenic in humans. Most pesticide regulatory agencies in other countries have followed their lead, suggesting that data sets and methodological differences partially explain these divergent views. However, this topic is complex and beyond the scope of this article.
Thus, it is imperative to have strict policies regarding these chemicals, following each crop's recommendations in class and the number of chemicals used.
Data availability statement
The original contributions presented in the study are included in the article/Supplementary material, further inquiries can be directed to the corresponding author.
Author contributions
Conceptualization: JB, TF, MO, PL, GB, MC, BG, JS, TS, SG, LZ, and JM. Methodology: GB, MC, TS, and SG. Validation: LZ and JM. Data curation: MO and PL. Writing—original draft preparation: JB, TF, MO, PL, GB, MC, BG, JS, TS, SG, LZ, JM, FR, and CP. Writing—review and editing: JB, TF, FR, and CP. Supervision: FR and CP. Project administration: CP. All authors have read and agreed to the published version of the manuscript.
Funding
CP was granted by Conselho Nacional de Desenvolvimento Científico e Tecnológico (grants 402364/2021-0 and 305335/2021-9) and Fundação Araucária Call 09/2021.
Conflict of interest
The authors declare that the research was conducted in the absence of any commercial or financial relationships that could be construed as a potential conflict of interest.
Publisher's note
All claims expressed in this article are solely those of the authors and do not necessarily represent those of their affiliated organizations, or those of the publisher, the editors and the reviewers. Any product that may be evaluated in this article, or claim that may be made by its manufacturer, is not guaranteed or endorsed by the publisher.
Supplementary material
The Supplementary Material for this article can be found online at: https://www.frontiersin.org/articles/10.3389/fpubh.2023.1130893/full#supplementary-material
References
1. Lee YM, Kim SA, Choi GS, Park SY, Jeon SW, Lee HS. Association of colorectal polyps and cancer with low-dose persistent organic pollutants: a case-control study. PLoS ONE. (2018) 13:e0208546. doi: 10.1371/journal.pone.0208546
2. Medeiros JF, Acayaba RD, Montagner CC. A química na avaliação do impacto à saúde humana diante da exposição aos pesticidas. Química Nova. (2021) 44:584–98. doi: 10.21577/0100-4042.20170699
3. Flores AV, Ribeiro JN, Neves AA, Queiroz ELR. Organoclorados: um problema de saúde pública. Artigos Ambient Soc. (2004) 7. doi: 10.1590/S1414-753X2004000200007
4. Vellingiri B, Chandrasekhar M, Sri Sabari S, Gopalakrishnan AV, Narayanasamy A, Venkatesan D, et al. Neurotoxicity of pesticides - a link to neurodegeneration. Ecotoxicol Environ Saf. (2022) 243:113972. doi: 10.1016/j.ecoenv.2022.113972
5. Tarmure S, Alexescu TG, Orasan O, Negrean V, Sitar-Taut AV, Coste SC, et al. Influence of pesticides on respiratory pathology – a literature review. Ann Agric Environ Med. (2020) 27:194–200. doi: 10.26444/aaem/121899
6. Leemans M, Couderq S, Demeneix B, Fini JB. Pesticides with potential thyroid hormone-disrupting effects: A review of recent data. Front Endocrinol (Lausana). (2019) 10:743. doi: 10.3389/fendo.2019.00743
7. Fucic A, Duca RC, Galea KS, Maric T, Garcia K, Bloom MS, et al. Reproductive health risks associated with occupational and environmental exposure to pesticides. Int J Environ Res Public Health. (2021) 18:6576. doi: 10.3390/ijerph18126576
8. Landrigan PJ. Pesticides and Human Reproduction. JAMA Intern Med. (2018) 178:26–27. doi: 10.1001/jamainternmed.2017.5092
9. Pedroso TMA, Benvindo-Souza M, De Araújo Nascimento F, Woch J, Dos Reis FG, de Melo E Silva D. Cancer and occupational exposure to pesticides: a bibliometric study of the past 10 years. Environ Sci Pollut Res. (2022) 29:17464–75. doi: 10.1007/s11356-021-17031-2
10. Koual M, Tomkiewicz C, Cano-Sancho G, Antignac JP, Bats AS, Coumoul X. Environmental chemicals, breast cancer progression and drug resistance. Environ Health. (2020) 19:117. doi: 10.1186/s12940-020-00670-2
11. FAOSTAT FAO. Pesticide Use. Food Agric Organ (2019). Available online at: https://www.fao.org/faostat/en/#data/RP/visualize7
12. IBGE. Census of Agriculture. Brazil Inst Geogr Stat (2017). Available online at: https://sidra.ibge.gov.br/pesquisa/censo-agropecuario/censo-agropecuario-2017/resultados-definitivos (accessed February 07, 2023).
13. Agência Nacional De Vigilância Sanitária (ANVISA),. Relatório PARA 2017/2018. (2019). Available online at: https://www.gov.br/anvisa/pt-br/assuntos/agrotoxicos/programa-de-analise-de-residuos-em-alimentos/arquivos/3770json-file-1 (accessed February 07, 2023).
14. El-Sheikh E, Ramadan MM, El-Sobki AE, Shalaby AA, Mccoy MR, Hamed IA, et al. Pesticide residues in vegetables and fruits from farmer markets and associated dietary risks. Molecules. (2022) 27:8072. doi: 10.3390/molecules27228072
15. Cote DJ, Bever AM, Chiu YH, Sandoval-Insausti H, Smith-Warner SA, Chavarro JE, et al. Pesticide residue intake from fruit and vegetable consumption and risk of glioma. Am J Epidemiol. (2022) 191:825–33. doi: 10.1093/aje/kwac007
16. Mahdavi V, Gordan H, Peivasteh-Roudsari L, Thai VN, Fakhri Y. Carcinogenic and non-carcinogenic risk assessment induced by pesticide residues in commercially available ready-to-eat raisins of Iran based on Monte Carlo Simulation. Environ Res. (2021) 206:112253. doi: 10.1016/j.envres.2021.112253
17. Parween M, Ramanathan AL, Raju NJ. Assessment of toxicity and potential health risk from persistent pesticides and heavy metals along the Delhi stretch of river Yamuna. Environ Res. (2021) 202:111780. doi: 10.1016/j.envres.2021.111780
18. Rebouillat P, Vidal R, Cravedi JP, Taupier-Letage B, Debrauwer L, Gamet-Payrastre L, et al. Prospective association between dietary pesticide exposure profiles and postmenopausal breast-cancer risk in the NutriNet-Santé cohort. Int J Epidemiol. (2021) 50:1184–98. doi: 10.1093/ije/dyab015
19. Odewale GO, Sosan MB, Oyekunle JAO, Adeleye AO. Human health risk assessment of dichlorodiphenyltrichloroethane (DDT) and hexachlorocyclohexane (HCH) pesticide residues in fruits and vegetables in Nigeria. Environ Sci Pollut Res Int. (2021) 28:33133–45. doi: 10.1007/s11356-021-12747-7
20. Brasil. Portaria DAS n° 448, de 17 de novembro de 2021. (2021). Available online at: https://www.gov.br/agricultura/pt-br/assuntos/inspecao/produtos-vegetal/pncrc-vegetal/arquivos/23-portaria-sda-mapa-448-2021-resultados2019e2020.pdf
21. Agência Nacional De Vigilância Sanitária (ANVISA),. Programa de Análise de Resíduos de Agrotóxicos em Alimentos (PARA). Brasília: ANVISA (2020). Available online at: https://www.gov.br/anvisa/pt-br/assuntos/agrotoxicos/programa-de-analise-de-residuos-em-alimentos (accessed February 07, 2023).
22. IBAMA. Relatórios de Comercialização de Agrotóxicos. Brazil Inst Environ Renew Nat Resour (2019). Available online at: http://www.ibama.gov.br/agrotoxicos/relatorios-de-comercializacao-de-agrotoxicos (accessed February 07, 2023).
23. Catae AF, Roat TC, Prataviera M, Pratavieira M, Palma MS, Malaspina O. Exposure to a sublethal concentration of imidacloprid and the side effects on target and nontarget organs of Apis mellifera (Hymenoptera, Apidae). Ecotoxicology. (2018) 27:109–21. doi: 10.1007/s10646-017-1874-4
24. European Food Safety Authority (EFSA). Peer review of the pesticide risk assessment for bees for the active substance imidacloprid considering the uses as seed treatments and granules. EFSA J. (2018) 16:e05178. doi: 10.2903/j.efsa.2018.5178
25. Gaboardi SC. Resíduos de agrotóxicos em alimentos no Brasil: considerações acerca do monitoramento do PARA (2001-2018): pesticides residues in food products in Brazil: Considerations about PARA monitoring (2001-2018). Ambientes Rev Geogr Ecol Polít. (2022) 4:160–200. doi: 10.48075/amb.v4i1.28294
26. Boobis AR, Ossendorp BC, Banasiak U, Hamey PY, Sebestyen I, Moretto A. Cumulative risk assessment of pesticide residues in food. Toxicol Lett. (2008) 180:137–50. doi: 10.1016/j.toxlet.2008.06.004
27. Moradi S, Issah A, Mohammadi H, Mirzaei K. Associações entre índice inflamatório dietético e incidência de câncer de mama e próstata: uma revisão sistemática e meta-análise. Nutrição. (2018) 55–6:168–78 doi: 10.1016/j.nut.2018.04.018
28. Marques and Silva. Estimativa de ingestão crônica de resíduos de agrotóxicos por meio da dieta. Rev Saúde Publica. (2021) 55:36. doi: 10.11606/s1518-8787.2021055002197
29. Thompson S, Ritz B, Cockburn., Heck JE. Prenatal ambient pesticide exposure and childhood retinoblastoma. Int J Hyg Environ Health. (2022) 245:114025. doi: 10.1016/j.ijheh.2022.114025
30. Swartz SJ, Morimoto LM, Whitehead TP, Derouen MC, Ma X, Wang R, et al. Proximity to endocrine-disrupting pesticides and risk of testicular germ cell tumors (TGCT) among adolescents: a population-based case-control study in california. Int J Hyg Environ Health. (2022) 239:113881. doi: 10.1016/j.ijheh.2021.113881
31. Zhang H, Zhang R, Zeng X, Wang X, Wang D, Jia H, et al. Exposure to neonicotinoid insecticides and their characteristic metabolites: association with human liver cancer. Environ Res. (2022) 208:112703. doi: 10.1016/j.envres.2022.112703
32. Franke AA, Li X, Shvetsov YB, Lai JF. Pilot study on the urinary excretion of the glyphosate metabolite aminomethyphosphonic acid and breast cancer risk: the multiethnic cohort study. Environ Pollut. (2021) 277:116848. doi: 10.1016/j.envpol.2021.116848
33. Lopes CVA, Albuquerque GSC. Agrotóxicos e seus impactos na saúde humana e ambiental: uma revisão sistemática. Saúde em Debate. (2018) 42:518–34. doi: 10.1590/0103-1104201811714
34. Barry KH, Koutros S, Andreotti G, Sandler DP, Burdette LA, Yeager M, et al. Genetic variation in nucleotide excision repair pathway genes, pesticide exposure and prostate cancer risk. Carcinogenesis. (2012) 33:331–7. doi: 10.1093/carcin/bgr258
35. Matich EK, Laryea J, Seely KA, Shelbie S, Su J, Hsu P-C. Association between pesticide exposure and colorectal cancer risk and incidence: a systematic review. Ecotoxicol Environ Saf . (2021) 219:112327. doi: 10.1016/j.ecoenv.2021.112327
36. Abhishek A, Ansari NG, Singh V, Sinha RJ, Mishra P, Mishra A. Genetic susceptibility of CYP1A1 gene and risk of pesticide exposure in prostate cancer. Cancer Biomark. (2020) 29:429–40. doi: 10.3233/CBM-190636
37. Tayour C, Ritz B, Langholz B, Mills PK, Wu A, Wilson JP, et al. A case–control study of breast cancer risk and ambient exposure to pesticides. Environ Epidemiol. (2019) 3:e070. doi: 10.1097/EE9.0000000000000070
38. Lee WJ, Blair A, Hoppin JA, Lubin JH, Rusiecki JA, Sandler DP, et al. Cancer incidence among pesticide applicators exposed to chlorpyrifos in the Agricultural Health Study. J Natl Cancer Inst. (2004) 96:1781–9. doi: 10.1093/jnci/djh324
39. Andreotti G, Freeman LEB, Shearer JJ, Lerro CC, Koutros S, Parks CG, et al. Occupational Pesticide Use and Risk of Renal Cell Carcinoma in the Agricultural Health Study. Environ Health Perspect. (2020) 128:67011. doi: 10.1289/EHP6334
40. Lombardi C, Thompson S, Ritz B, Cockburn M, Heck JE. Residential proximity to pesticide application as a risk factor for childhood central nervous system tumors. Environ Res. (2021) 197:111078. doi: 10.1016/j.envres.2021.111078
41. Pardo LA, Freeman LEB, Lerro CC, Andreotti G, Hofmann JN, Parks CG, et al. Pesticide exposure and risk of aggressive prostate cancer among private pesticide applicators. Environ Health. (2020) 19:30. doi: 10.1186/s12940-020-00583-0
42. Kumar P, Sharma S, Sundriyal D, Navria SC, Sehrawat A. an institution-based demographic study of urinary bladder cancer from North India. Indian J Surg Oncol. (2022) 13:432–4. doi: 10.1007/s13193-022-01508-8
43. Park AS, Ritz B, Yu F, Cockburn M, Heck JE. Prenatal pesticide exposure and childhood leukemia- a california statewide case-control study. Int J Hyg Environ Health. (2020) 226:113486. doi: 10.1016/j.ijheh.2020.113486
44. Cocco P, Satta G, Dubois S, Pili C, Pilleri M, Zucca M, et al. Lymphoma risk and occupational exposure to pesticides: results of the Epilymph study. Occup Environ Med. (2013) 70:91–8. doi: 10.1136/oemed-2012-100845
45. Zhang L, RANA I, Shaffer RM, Taioli E, Sheppard L. Exposure to glyphosate-based herbicides and risk for non-Hodgkin lymphoma: a meta-analysis and supporting evidence. Mutat Res Rev Mutat Res. (2019) 781:186–206. doi: 10.1016/j.mrrev.2019.02.001
46. Andreotti G, Koutros S, Hofmann JN, Sandler DP, Lubin JH, Lynch CF, et al. Uso de glifosato e incidência de câncer no estudo de saúde agrícola. J Natl Cancer Inst. (2018) 110:509–16. doi: 10.1093/jnci/djx233
47. Schinasi L, Leon ME. Non-Hodgkin lymphoma and occupational exposure to agricultural pesticide chemical groups and active ingredients: a systematic review and meta-analysis. Int J Environ Res Public Health. (2014) 11:4449–527. doi: 10.3390/ijerph110404449
48. Duan X, Yang Y, Wang S, Feng X, Wang T, Wang P, et al. Changes in the expression of genes involved in cell cycle regulation and the relative telomere length in the process of canceration induced by omethoate. Tumour Biol. (2017) 39:1010428317719782. doi: 10.1177/1010428317719782
49. Navarrete-Meneses MP, Pedraza-Meléndez AI, Salas-Labadía C, Moreno-Lorenzana D, Pérez-Vera P. Low concentrations of permethrin and malathion induce numerical and structural abnormalities in KMT2A and IGH genes in vitro. J Appl Toxicol. (2018) 38:1262–70. doi: 10.1002/jat.3638
50. Hofmann JN, Beane Freeman LE, Murata K, Andreotti G, Shearer JJ, Thoren K, et al. Lifetime pesticide use and monoclonal gammopathy of undetermined significance in a prospective cohort of male farmers. Environ Health Perspect. (2021) 129:17003. doi: 10.1289/EHP6960
51. Alavanja MCR, Hofmann JN, Lynch CF, Hines CJ, Barry KH, Barker J, et al. Non-hodgkin lymphoma risk and insecticide, fungicide and fumigant use in the agricultural health study. PLoS ONE. (2014) 9:e109332. doi: 10.1371/journal.pone.0109332
52. Ferreira JD, Couto AC, Pombo-De-Oliveira MS, Koifman S, Brazilian Collaborative Study Group of Infant Acute Leukemia. In utero pesticide exposure and leukemia in Brazilian children < 2 years of age. Environ Health Perspect. (2012) 121:269–75. doi: 10.1289/ehp.1103942
53. Hou L, Andreotti G, Baccarelli AA, Savage S, Hoppin JA, Sandler DP, et al. Lifetime pesticide use and telomere shortening among male pesticide applicators in the Agricultural Health Study. Environ Health Perspect. (2013) 121:919–24. doi: 10.1289/ehp.1206432
54. Madrigal JM, Jones RR, Gunier RB, Whitehead TP, Reynolds P, Metayer C, et al. Residential exposure to carbamate, organophosphate, and pyrethroid insecticides in house dust and risk of childhood acute lymphoblastic leukemia. Environ Res. (2021). 201:111501. doi: 10.1016/j.envres.2021.111501
55. Shearer J, Beane Freeman LE, Liu D, Andreotti G, Hamilton J, Happel J, et al. Longitudinal investigation of hematological alterations among permethrin-exposed pesticide applicators in the Biomarkers of Exposure and Effect in Agriculture study. Occup Environ Med. (2019) 76:467–70. doi: 10.1136/oemed-2018-105559
56. De Roos AJ, Schinasi LH, Miligi L, Cerhan JR, Bhatti PT, Baris D, et al. Occupational insecticide exposure and risk of non-hodgkin lymphoma: a pooled case-control study from the interlymph consortium. Int J Cancer. (2021) 149:1768–86. doi: 10.1002/ijc.33740
57. Schwingl PJ, Lunn RM, Mehta SS. A tiered approach to prioritizing registered pesticides for potential cancer hazard evaluations: implications for decision making. Environ Health. (2021) 20:13. doi: 10.1186/s12940-021-00696-0
58. Li X, He S, Xiao H, He TT, Zhang JD, LUO ZR. Neonicotinoid insecticides promote breast cancer progression via G protein-coupled estrogen receptor: in vivo, in vitro and in silico studies. Environ Int. (2022) 170:107568. doi: 10.1016/j.envint.2022.107568
59. Cardona B, Rudel RA. Application of an in vitro assay to identify chemicals that increase estradiol and progesterone synthesis and are potential breast cancer risk factors. Environ Health Perspect. (2021) 129:77003. doi: 10.1289/EHP8608
60. Kim CW, Go RE, Choi KC. Treatment of BG-1 ovarian cancer cells expressing estrogen receptors with lambda-cyhalothrin and cypermethrin caused a partial estrogenicity via an estrogen receptor-dependent pathway. Toxicol Res. (2015) 31:331–7. doi: 10.5487/TR.2015.31.4.331
61. Moyano P, García J, García JM, Pelayo A, Muñoz-Calero P, Frejo MT, et al. Chlorpyrifos-induced cell proliferation in human breast cancer cell lines differentially mediated by estrogen and aryl hydrocarbon receptors and KIAA1363 enzyme after 24 h and 14 days exposure. Chemosphere. (2020) 251:126426. doi: 10.1016/j.chemosphere.2020.126426
62. Zárate LV, Pontillo CA, Español A, Miret NV, Chiappini F, Cocca C, et al. Angiogenesis signaling in breast cancer models is induced by hexachlorobenzene and chlorpyrifos, pesticide ligands of the aryl hydrocarbon receptor. Toxicol Appl Pharmacol. (2020) 401:115093. doi: 10.1016/j.taap.2020.115093
63. Lasagna M, Ventura C, Hielpos MS, Mardirosian MN, Martín G, Miret N, et al. Endocrine disruptor chlorpyrifos promotes migration, invasion, and stemness phenotype in 3D cultures of breast cancer cells and induces a wide range of pathways involved in cancer progression. Environ Res. (2022) 204(Pt A):111989. doi: 10.1016/j.envres.2021.111989
64. Thakur S, Sarkar B, Dhiman M, Mantha AK. Organophosphate-pesticides induced survival mechanisms and APE1-mediated Nrf2 regulation in non-small-cell lung cancer cells. J Biochem Mol Toxicol. (2020) 35:e22640. doi: 10.1002/jbt.22640
65. Hirano T, Minagawa S, Furusawa Y, Yunoki T, Ikenaka Y, Yokoyama T, et al. Growth and neurite stimulating effects of the neonicotinoid pesticide clothianidin on human neuroblastoma SH-SY5Y cells. Toxicol Appl Pharmacol. (2019) 383:114777. doi: 10.1016/j.taap.2019.114777
66. Thongprakaisang S, Thiantanawat A, Rangkadilok N, Suriyo T, Satayavivad J. Glyphosate induces human breast cancer cells growth via estrogen receptors. Food Chem Toxicol. (2013) 59:129–36. doi: 10.1016/j.fct.2013.05.057
67. Stur E, Aristizabal-Pachon AF, Peronni KC, Agostini LP, Waigel S, Chariker J, et al. Glyphosate-based herbicides at low doses affect canonical pathways in estrogen positive and negative breast cancer cell lines. PLoS ONE. (2019) 14:e0219610. doi: 10.1371/journal.pone.0219610
68. Caron-Beaudoin É, Viau R, Sanderson JT. Effects of neonicotinoid pesticides on promoter-specific aromatase (CYP19) expression in Hs578t breast cancer cells and the role of the VEGF pathway. Environ Health Perspect. (2018) 126:047014. doi: 10.1289/EHP2698
69. Mesnage R, Ibragim M, Mandrioli D, Falcioni L, Tibaldi E, Belpoggi F, et al. Comparative toxicogenomics of glyphosate and roundup herbicides by mammalian stem cell-based genotoxicity assays and molecular profiling in sprague-dawley rats. Toxicol Sci. (2022) 186:83–101. doi: 10.1093/toxsci/kfab143
70. Huo D, Jiang S, Qin Z, Feng Y, Yang R, Lv L, et al. Omethoate induces pharyngeal cancer cell proliferation and G1/S cell cycle progression by activation of Akt/GSK-3β/cyclin D1 signaling pathway. Toxicology. (2019) 427:152298. doi: 10.1016/j.tox.2019.152298
71. Navarrete-Meneses MP, Salas-Labadía C, Sanabrais-Jiménez M, Santana-Hernández J, Serrano-Cuevas A, Juárez-Velázquez R, et al. “Exposure to the insecticides permethrin and malathion induces leukemia and lymphoma-associated gene aberrations in vitro”. Toxicol In Vitro. (2017) 44:17–26. doi: 10.1016/j.tiv.2017.06.013
72. Caron-Beaudoin É, Denison MS, Sanderson JT. Effects of neonicotinoids on promoter-specific expression and activity of aromatase (CYP19) in human adrenocortical carcinoma (H295R) and primary umbilical vein endothelial (HUVEC) cells. Toxicol Sci. (2022) 149:134–44. doi: 10.1093/toxsci/kfv220
73. Caron-Beaudoin E, Viau R, Hudon-Thibeault AA, Vaillancourt C, Sanderson JT. The use of a unique co-culture model of fetoplacental steroidogenesis as a screening tool for endocrine disruptors: the effects of neonicotinoids on aromatase activity and hormone production. Toxicol Appl Pharmacol. (2017) 332:15–24. doi: 10.1016/j.taap.2017.07.018
74. Nguyen K, Sanchez CL, Brammer-Robbins E, Pena-Delgado C, Kroyter N, El Ahmadie N, et al. Neurotoxicity assessment of QoI strobilurin fungicides azoxystrobin and trifloxystrobin in human SH-SY5Y neuroblastoma cells: insights from lipidomics and mitochondrial bioenergetics. Neurotoxicology. (2022) 91:290–304. doi: 10.1016/j.neuro.2022.06.002
75. Timoumi R, Amara I, Ayed Y, Ben Salem I, Abid-Essefi S. Triflumuron induces genotoxicity in both mice bone marrow cells and human Colon cancer cell line. Toxicol Mech Methods. (2020) 30:438–49. doi: 10.1080/15376516.2020.1758981
76. Ning X, Wang Ym Yan W, Li G, Sang N. Chitin synthesis inhibitors promote liver cancer cell metastasis via interfering with hypoxia-inducible factor 1α. Chemosphere. (2018) 206:231–7. doi: 10.1016/j.chemosphere.2018.05.014
77. Ministério Da Saúde,. Guia Alimentar para a População Brasileira, 2nd ed. (2006). Available online at: https://www.gov.br/saude/pt-br/assuntos/saude-brasil/publicacoes-para-promocao-a-saude/guia_alimentar_populacao_brasileira_2ed.pdf/view (accessed February 07, 2023).
78. Bill (PL 6299/2002). Available online at: https://www.camara.leg.br/proposicoesWeb/fichadetramitacao?idProposicao=46249 (accessed February 07, 2023).
79. European Food Safety Authority (EFESA),. Pesticides in food: latest report published. (2022). Available online at: https://www.efsa.europa.eu/en/news/pesticides-food-latest-report-published (accessed February 07, 2023).
80. Muñoz JP, Bleak TC, Calaf GM. Glyphosate and the key characteristics of an endocrine disruptor: a review. Chemosphere. (2021) 270:128619. doi: 10.1016/j.chemosphere.2020.128619
81. Kogan M, Alister C. Glyphosate use in forest plantations. Chilean J Agric Res. (2010) 70:652–66. doi: 10.4067/S0718-58392010000400017
82. Davoren MJ, Schiestl RH. Glyphosate-based herbicides and cancer risk: a post-IARC decision review of potential mechanisms, policy and avenues of research. Carcinogenesis. (2018) 39:1207–15. doi: 10.1093/carcin/bgy105
83. Araújo-Ramosa T, Passoni MT, Romano MA, Martino-Andrade AJ. Controversies on endocrine and reproductive effects of glyphosate and glyphosate-based herbicides: a mini-review. Front Endocrinol. (2021) 12:627210. doi: 10.3389/fendo.2021.627210
84. Rossetti MF, Canesini G, Lorenz V, Milesi MM, Varayoud J, Ramos GJ. Epigenetic changes associated with exposure to glyphosate-based herbicides in mammales. Front Endocrinol. (2021) 12:671991. doi: 10.3389/fendo.2021.671991
85. Agência Nacional De Vigilância Sanitária (ANVISA),. Carbendazim. (2022). Available online at: https://www.gov.br/anvisa/pt-br/assuntos/noticias-anvisa/2022/carbendazim-anvisa-concluiu-processo-de-reavaliacao-e-mantem-o-banimento/CarbendazimDICOL.pdf (accessed February 07, 2023).
86. Yenjerla M, Cox C, Wilson L, Jordan MA. Carbendazim inhibits cancer cell proliferation by suppressing microtubule dynamics. J Pharmacol Exp Ther. (2008) 328:390–8. doi: 10.1124/jpet.108.143537
87. Rama EM, Bortolan S, Vieira ML, Gerardin DCC, Moreira EG. Reproductive and possible hormonal effects of carbendazim. Regul Toxicol Pharmacol. (2014) 69:476–86. doi: 10.1016/j.yrtph.0.2014.05.016
88. Starks SE, Gerr F, Kamel F, Lynch CF, Jones MP, Alavanja MC, et al. Neurobehavioral function and organophosphate insecticide use among pesticide applicators in the Agricultural Health Study. Neurotoxicol Teratol. (2012) 34:168–76. doi: 10.1016/j.ntt.2011.08.014
89. World Health Organization (WHO) (2019). Available online at: https://apps.who.int/iris/bitstream/handle/10665/332193/9789240005662-eng.pdf (accessed February 07, 2023).
90. Kong Z, Dong F, Xu J, Liu X, Li J, Li Y, et al. Degradation of acephate and its metabolite methamidophos in rice during processing and storage. Food Control. (2012) 23:149–53. doi: 10.1016/j.foodcont.2011.07.001
91. R Core Team,. R: A language environment for statistical computing. Vienna: R Foundation for Statistical Computing (2021). Avaialble online at: https://www.R-project.org/ (accessed February 07, 2023).
92. Aria M, Cuccurullo C. Bibliometrix: an R-tool for comprehensive science mapping analysis. J Informetr. (2017) 11:959–75. doi: 10.1016/j.joi.2017.08.007
93. Abolhassani M, Asadikaram G, Paydar P. Organochlorine and organophosphorous pesticides may induce colorectal cancer; a case-control study. Ecotoxicol Environ Saf. (2019) 178:168–77. doi: 10.1016/j.ecoenv.2019.04.030
94. Braga IA, Valle D. Aedes aegypti: inseticidas, mecanismos de ação e resistência. Epidemiol Serv Saúde Brasíl. (2007) 16:179–293. doi: 10.5123/S1679-49742007000400006
95. Ihara M, Matsuda K. Neonicotinoids: molecular mechanisms of action, insights into resistance and impact on pollinators. Curr Opin Insect Sci. (2018) 30:86–92. doi: 10.1016/j.cois.2018.09.009
96. Liu Y, Yin T, Feng Y, Cona MM, Huang G, Liu J, et al. Mammalian models of chemically induced primary malignancies exploitable for imaging-based preclinical theragnostic research. Quant Imaging Med Surg. (2015) 5:708–29. doi: 10.3978/j.issn.2223-4292.2015.06.01
97. Irigaray P, Belpomme D. Basic properties and molecular mechanisms of exogenous chemical carcinogens. Carcinogenesis. (2010) 31:135–48. doi: 10.1093/carcin/bgp252
98. Klaunig JE, Kamendulis LM, XU Y. Epigenetic mechanisms of chemical carcinogenesis. Hum Exp Toxicol. (2000) 543–55. doi: 10.1191/096032700701546442
99. Oliveira PA, Colaço A, Chaves R, Guedes-Pinto H, De-La-Cruz LFP, Lopes C. Chemical carcinogenesis. Proc Braz Acad Sci. (2007) 79:593–616. doi: 10.1590/S0001-37652007000400004
100. Ataei M, Abdollahi M. A systematic review of mechanistic studies on the relationship between pesticide exposure and cancer induction. Toxicol Appl Pharmacol. (2022) 456:116280. doi: 10.1016/j.taap.2022.116280
101. Van Maele-Fabry G, Gamet-Payrastre L, Lison D. Residential exposure to pesticides as risk factor for childhood and young adult brain tumors: a systematic review and meta-analysis. Environ Int. (2017) 106:69–90. doi: 10.1016/j.envint.2017.05.018
102. Coste A, Bailey HD, Kartal-Kaess M, Renella R, Berthet A, Spycher BD. Parental occupational exposure to pesticides and risk of childhood cancer in Switzerland: a census-based cohort study. BMC Cancer. (2020) 20:819. doi: 10.1186/s12885-020-07319-w
103. Pascale A, Laborde A. Impact of pesticide exposure in childhood. Rev Environ Health. (2020) 35:221–7. doi: 10.1515/reveh-2020-0011
104. Landau-Ossondo M, Rabia N, Jos-Pelage J, Marquet LM, Isidore Y, Saint-Aimé C, et al. Why pesticides could be a common cause of prostate and breast cancers in the French Caribbean Island, Martinique. An overview on key mechanisms of pesticide-induced cancer. Biomed Pharmacother. (2009) 63:383–95. doi: 10.1016/j.biopha.2009.04.043
105. Fenga C. Occupational exposure and risk of breast cancer (review). Biomedical Reports. (2015) 4:282–92. doi: 10.3892/br.2016.575
106. Saad-Hussein A, Beshir S, Taha MM, Shahy EM, Shaheen W, Abdel-Shafy EA, et al. Early prediction of liver carcinogenicity due to occupational exposure to pesticides. Mut Res Genet Toxicol Environ Mutagen. (2018) 838:46–53. doi: 10.1016/j.mrgentox.2018.12.004
107. Hu L, Luo G, Zhou T, Tao Y, Feng J, Mei S. The association between non-Hodgkin lymphoma and organophosphate pesticides exposure: a meta-analysis. Environ Pollut. (2017) 231:319–28. doi: 10.1016/j.envpol.2017.08.028
108. Chiu B, Blai A. Pesticides, chromosomal aberrations, and non-Hodgkin's lymphoma. Agromedicine. (2009) 14:250–5. doi: 10.1080/10599240902773140
109. Rusiecki J, Patel R, Koutros S, Beane-Freeman L, Landgren O, Bonner MR, et al. Cancer incidence among pesticide applicators exposed to permethrin in the Agricultural Health Study. Environ Health Perspect. (2009) 117. doi: 10.1289/ehp.11318
110. Hernandez A, Menendez P. Linking pesticide exposure with pediatric leukemia: potential underlying mechanisms. Int J Mol Sci. (2016) 17:461. doi: 10.3390/ijms17040461
111. Maryam Z, Sajad A, Maral N, Zahra L, Sima N, Zeinab A, et al. Relationship between exposure to pesticides and occurrence of acute leukemia in Iran. Asian Pac J Cancer Prev. (2015) 16:239–44. doi: 10.7314/APJCP.2015.16.1.239
112. Andreotti G, Hoppin JA, Hou L, Koutros S, Gadalla SM, Savage SA, et al. (2015). Pesticide use and relative leukocyte telomere length in the agricultural health study. PLoS ONE. 10:e0133382. doi: 10.1371/journal.pone.0133382
113. Huang F, Chen Z, Chen H, Lu W, Xie S, Meng QH, et al. Cypermethrin promotes lung cancer metastasis via modulation of macrophage polarization by targeting microRNA-155/Bcl6. Toxicol Sci. (2018) 163:454–65. doi: 10.1093/toxsci/kfy039
114. Hester S, Moore T, Padgett WT, Murphy L, Wood CE, Nesnow S. The hepatocarcinogenic conazoles: cyproconazole, epoxiconazole, and propiconazole induce a common set of toxicological and transcriptional responses. Toxicol Sci. (2012) 127:54–65. doi: 10.1093/toxsci/kfs086
115. Tamura K, Inoue K, Takahashi M, Matsuo S, Irie K, Kodama Y, et al. Involvement of constitutive androstane receptor in liver hypertrophy and liver tumor development induced by triazole fungicides. Food Chem Toxicol. (2015) 78:86–95. doi: 10.1016/j.fct.2015.01.021
116. Wang L, Deng Q, Hu H, Liu M, Gong Z, Zhang S, et al. Glyphosate induces benign monoclonal gammopathy and promotes multiple myeloma progression in mice. J Hematol Oncol. (2019) 12:70. doi: 10.1186/s13045-019-0767-9
117. Yoshimaru S, Shizu R, Tsuruta S, Amaike Y, Kano M, Hosaka T, et al. Acceleration of murine hepatocyte proliferation by imazalil through the activation of nuclear receptor PXR. J Toxicol Sci. (2018) 43:443–50. doi: 10.2131/jts.43.443
118. Rooney J, Wehmas LC, Ryan N, Chorley BN, Hester SD, Kenyon EM, et al. Genomic comparisons between hepatocarcinogenic and non-hepatocarcinogenic organophosphate insecticides in the mouse liver. Toxicology. (2022) 465:153046. doi: 10.1016/j.tox.2021.153046
119. Quist EM, Boorman GA, Cullen JM, Maronpot RR, Remick AK, Swenberg JA, et al. Reevaluation of hepatocellular neoplasms in CD-1 Mice from a 2-year oral carcinogenicity study with permethrin. Toxicol Pathol. (2019) 47:11–7. doi: 10.1177/0192623318809304
120. Jiang J, Wu S, Lv L, Liu X, Chen L, Zhao X, et al. Mitochondrial dysfunction, apoptosis and transcriptomic alterations induced by four strobilurins in zebrafish (Danio rerio) early life stages. Environ Pollut. (2019) 253:722–30. doi: 10.1016/j.envpol.2019.07.081
121. Navruz FZ. Resveratrol alleviates pyraclostrobin induced-lipidperoxidation, oxidative stress, and DNA damage in rats. Environ Sci Pollut Res. (2022) 3:6414–23. doi: 10.21203/rs.3.rs-920465/v2
122. Nesnow S. Mini-review Integration of toxicological approaches with “‘omic”' and related technologies to elucidate mechanisms of carcinogenic action: propiconazole, an example. Cancer Lett. (2013) 334:20–7. doi: 10.1016/j.canlet.2012.11.003
123. Currie RA, Peffer RC, Goetz AK, Omiecinski CJ, Goodman Richard C JI. Phenobarbital and propiconazole toxicogenomic profiles in mice show major similarities consistent with the key role that constitutive androstane receptor (CAR) activation plays in their mode of action NIH Public Access. Toxicology. (2014) 321:80–8. doi: 10.1016/j.tox.2014.03.003
124. Tu TY, Hong CY, Sasado T, Kashiwada S, Chen PJ. Early life exposure to a rodent carcinogen propiconazole fungicide induces oxidative stress and hepatocarcinogenesis in medaka fish. Aquatic Toxicol. (2016) 170:52–61. doi: 10.1016/j.aquatox.2015.11.014
125. Bhat VS, Hester SD, Nesnow S, Eastmond DA. Concordance of transcriptional and apical benchmark dose levels for conazole-induced liver effects in mice. Toxicol Sci. (2013) 136:205–15. doi: 10.1093/toxsci/kft182
126. Roos JF, Leavitt SA, Schmid JE, Nelson GB. Quantitative changes in endogenous DNA adducts correlate with conazole in vivo mutagenicity and tumorigenicity. Mutagenesis. (2012) 27:541–9. doi: 10.1093/mutage/ges017
127. Oshida K, Vasani N, JONES C, Moore T, Hester S, nesnow S, et al. Identification of chemical modulators of the constitutive activated receptor (CAR) in a gene expression compendium. Nucl Recept Signal. (2015) 13:2. doi: 10.1621/nrs.13002
128. De Morais CR, Carvalho SM, Carvalho Naves MP, Araujo G, De Rezende AAA, Bonetti AM, et al. Potencial mutagênico, recombinogênico e carcinogênico do inseticida tiametoxam e produto formulado em células somáticas de Drosophila melanogaster. Quimiosfera. (2017) 187:163–72. doi: 10.1016/j.chemosphere.2017.08.108
129. Broto GE, Silva PRB, Trigo FC, Victorino VJ, Bonifácio KL, Pavanelli WR, et al. Impact of the induction phase chemotherapy on cytokines and oxidative markers in peripheral and bone marrow plasma of children with acute lymphocytic leukemia. Curr Res Immunol. (2021) 2:163–8. doi: 10.1016/j.crimmu.2021.09.002
130. He F, Antonucci L, Karin M. NRF2 as a regulator of cell metabolism and inflammation in cancer. Carcinogenesis. (2020) 41:405–16. doi: 10.1093/carcin/bgaa039
131. Chaufan G, Coalova I, Ríos De Molina Mdel C. Glyphosate commercial formulation causes cytotoxicity, oxidative effects, and apoptosis on human cells: differences with its active ingredient. Int J Toxicol. (2014) 33:29–38. doi: 10.1177/1091581813517906
132. Anjitha R, Antony A, Shilpa O, Anupama K, Mallikarjunaiah S, Gurushankara H. Malathion induced cancer-linked gene expression in human lymphocytes. Environ Res. (2020) 182. doi: 10.1016/j.envres.2020.109131
133. Ministério Da Agricultura Pecuária e Abastecimento,. MAPA faz monitoramento de resíduos de agrotóxicos na safra de maçã em Santa Catarina e no Rio Grande do Sul. (2022). Available online at: https://www.gov.br/agricultura/pt-br/assuntos/noticias/mapa-faz-monitoramento-de-residuos-de-agrotoxicos-na-safra-de-maca-em-santa-catarina-e-no-rio-grande-do-sul (accessed February 07, 2023).
134. Ministério Da Agricultura Pecuária e Abastecimento,. Informações Técnicas. (2022). Available online at: https://www.gov.br/agricultura/pt-br/assuntos/insumos-agropecuarios/insumos-agricolas/agrotoxicos/informacoes-tecnicas (accessed February 07, 2023).
135. Costa VIB, Mello MSC, Friedrich K. Exposição ambiental e ocupacional a agrotóxicos e o linfoma não Hodgkin. Saúde em Debate. (2017) 41:49–62. doi: 10.1590/0103-1104201711205
136. Melgarejo L, Gurgel AM. Agrotóxicos, seus mitos e implicações. In: Gurgel AM, Santos MOS, Gurgel IGD, editors. Saúde do Campo e Agrotóxicos: vulnerabilidades socioambientais, político-institucionais e teórico-metodológicas Recife: Ed UFPE. Ciênc. saúde coletiva (2019). p. 39–75.
137. Friedrich K, do Monte Gurgel A, Sarpa M, Bedor CNG, de Siqueira MT, Gurgel IGD, et al. Toxicologia crítica aplicada aos agrotóxicos – perspectivas em defesa da vida. Saúde em Debate. (2022) 46:293–315. doi: 10.1590/0103-11042022e220
138. Marcelino AF, Wachtel CC, Ghisi NDC. Are our farm workers in danger? Genetic damage in farmers exposed to pesticides. Int J Environ Res Public Health. (2019) 16:358. doi: 10.3390/ijerph16030358
139. Sabarwal A, Kumar K, Singh RP. Hazardous effects of chemical pesticides on human health-Cancer and other associated disorders. Environ Toxicol Pharmacol. (2018) 63:103–14. doi: 10.1016/j.etap.2018.08.018
140. Patel DM, Jones RR, Booth BJ, Olsson AC, Kromhout H, Straif K, et al. Parental occupational exposure to pesticides, animals and organic dust and risk of childhood leukemia and central nervous system tumors: findings from the International Childhood Cancer Cohort Consortium (I4C). Int J Cancer. (2020) 146:943–52. doi: 10.1002/ijc.32388
141. Candiotto LZP, Ferreira MO, Ferreira IN, Teixeira GTT, Carla Da Silva J, Tedesco EH, et al. Diagnostic evaluation of the presence of residues of glyphosate-AMPA and 2.4D pesticides in urine samples from people living in a rural Brazilian Community. medRxiv. (2021). doi: 10.1101/2021.08.16.21259798
142. Panis C, Candiotto LZP, Gaboardi SC, Gurzenda S, Cruz J, Castro M, et al. Widespread pesticide contamination of drinking water and impact on cancer risk in Brazil. Environ Int. (2022) 165:107321. doi: 10.1016/j.envint.2022.107321
143. Brasil. Decreto n° 4.074, de 4 de janeiro de 2002. Regulamenta a Lei n o 7.802, de 11 de julho de 1989, que dispõe sobre a pesquisa, a experimentação, a produção, a embalagem e rotulagem, o transporte, o armazenamento, a comercialização, a propaganda comercial, a utilização, a importação, a exportação, o destino final dos resíduos e embalagens, o registro, a classificação, o controle, a inspeção e a fiscalização de agrotóxicos, seus componentes e afins, e dá outras providências. Diário Oficial da União (2002).
144. Franco CR, Pelaez V. Constructing the politi - cal agenda of control over pesticides in Brazil. Ambiente Sociedade. (2016) 1:215–32. doi: 10.1590/1809-4422ASOC143673V1932016
145. Food Agriculture Organization (FAO). Limite máximo de resíduos. (2005). Available online at: https://www.fao.org/pesticide-registration-toolkit/information-sources/maximum-residue-limits/en/ (accessed February 07, 2023).
146. Carneiro FF, Augusto LGS, Rigotto RM, Friedrich K, Búrgio AC. Dossiê ABRASCO: um alerta sobre os impactos dos agrotóxicos na saúde. Rio de Janeiro: EPSJV Available online at: https://www.abrasco.org.br/dossieagrotoxicos/wpcontent/uploads/2013/10/DossieAbrasco_2015_web.pdf (accessed February 07, 2023).
147. Panis C, Kawassaki ACB, Crestani APJ, Pascotto CR, Bortoloti DS, Vicentini GE, et al. Evidence on human exposure to pesticides and the occurrence of health hazards in the Brazilian population: a systematic review. Front Public Health. (2022) 9:787438. doi: 10.3389/fpubh.2021.787438
148. Brasil. Constituição da República Federativa do Brasil de 1988. Brasília, DF: Presidência da República (1988). Avaialble online at: http://www.planalto.gov.br/ccivil_03/constituicao/constituicao.htm (accessed February 07, 2023).
149. Friedrich K, Silveira GR, Amazonas JC, Gurgel AM, Almeida VES, Sarpa M. International regulatory situation of pesticides authorized for use in Brazil: potential for damage to health and environmental impacts. Cad Saúde Pública São Paulo. (2021) 4:1–18. doi: 10.1590/0102-311X00061820
150. Acquavella J, Alexander B, Mandel J, Gustin C, Baker B, Chapman P, et al. Biomonitoramento de glifosato para agricultores e suas famílias: resultados do estudo de exposição familiar agrícola. Perspect Saúde Ambiental. (2004) 112:321–6. doi: 10.1289/ehp.6667
151. Gillezeau C, Vangerwen M, Shaffer R, Rana I, Zhang L, Sheppard L, et al. A evidência da exposição humana ao glifosato: uma revisão. Saúde Ambiental. (2019) 18: 2. doi: 10.1186/s12940-018-0435-5
152. Panis C, Pessôa candi Otto LZ, Gaboardi SC. Permissiveness of Brazilian legislation, widespread contamination by pesticides in food and water, and risks to the population's Health. Front Environ Sci. (2022) 10:926434. doi: 10.3389/fenvs.2022.926434
153. Policy Department Economic Scientific Policy European Parliament. The benefits of strict cut-off criteria on human health in re - lation to the proposal for a Regulation con - cerning plant protection products. (2008). Available online at: http://www.europarl.europa.eu/RegData/etudes/etudes/join/2008/408559/IPOL-JOIN_ET(2008)-408559_EN.pdf (accessed February 07, 2023).
154. Michaels D. Doubt is Their Product: How Industry's Assault on Science Threatens Your Health. New York, NY: Oxford University Press (2008).
155. Gaboardi SC. O uso de agrotóxicos no Sudoeste do Paraná a partir de uma perspectiva geográfica multiescalar. Tese [Doutorado em Geografia]. Universidade Estadual do Oeste do Paraná. Francisco Beltrão/PR/Brasil (2021).
156. Williams G, Kroes R, Munro I. Safety evaluation and risk assessment of the herbicide Roundup and its active ingredient, glyphosate, for humans. Regul Toxicol Pharmacol. (2000) 31:117–65. doi: 10.1006/rtph.1999.1371
157. Connolly A, Coggins M, Galea K, Jones K, Kenny L, Mcgowan P, et al. Avaliando as rotas de exposição ao glifosato e sua contribuição para a carga corporal total: um estudo entre horticultores de amenidades. Ann Work Expo Saúde. (2019) 63:133–47. doi: 10.1093/annweh/wxy104
158. Peillex C, Pelletier M. O impacto e a toxicidade do glifosato e de herbicidas à base de glifosato na saúde e na imunidade. J Imunotoxicol. (2020) 17:163–74. doi: 10.1080/1547691X.2020.1804492
159. Pearce N, Blair A, Vineis P, et al. IARC monographs: 40 years of evaluating carcinogenic hazards to humans. Environ Health Perspect. (2015) 123:507–14. doi: 10.1289/ehp.1409149
160. IARC. Working Group on the Evaluation of Carcinogenic Risks to Humans. Some organophosphate insecticides and herbicides. Lyon, France: International Agency for Research on Cancer (IARC Monographs on the Evaluation of Carcinogenic Risks to Humans, No. 112.) (2017). Available online at: https://www.ncbi.nlm.nih.gov/books/NBK436774/ (accessed February 07, 2023).
161. Guyton KZ, Loomis D, Grosse Y, El Ghissassi F, Benbrahim-Tallaa L, Guha N, et al. Carcinogenicity of tetrachlorvinphos, parathion, malathion, diazinon, and glyphosate. Lancet Oncol. (2015) 16:490–1. doi: 10.1016/S1470-2045(15)70134-8
162. European Food Safety Authority (EFSA). Conclusion on the peer review of the pesticide risk assessment of the active substance glyphosate. EFSA J. (2015) 13:1–107 doi: 10.2903/j.efsa.2015.4302
163. Acquavella J, Garabrant D, Marsh G, Sorahan T, Weed DL. Glyphosate epidemiology expert panel review: a weight of evidence systematic review of the relationship between glyphosate exposure and non-Hodgkin's lymphoma or multiple myeloma. Crit Rev Toxicol. (2016) 46:28–43. doi: 10.1080/10408444.2016.1214681
164. Brusick D, Aardema M, Kier L, Kirkland D, Williams G. Genotoxicity Expert Panel review: weight of evidence evaluation of the genotoxicity of glyphosate, glyphosate-based formulations, and aminomethylphosphonic acid. Crit Rev Toxicol. (2016) 46:56–74. doi: 10.1080/10408444.2016.1214680
165. Chang ET, Delzell E. Systematic review and meta-analysis of glyphosate exposure and risk of lymphohematopoietic cancers. J Environ Sci Health B. (2016) 51:402–34. doi: 10.1080/03601234.2016.1142748
166. Solomon KR. Glyphosate in the general population and in applicators: a critical review of studies on exposures. Crit Rev Toxicol. (2016) 46:21–7. doi: 10.1080/10408444.2016.1214678
167. Williams GM, Aardema M, Acquavella J, Berry SC, Brusick D, Burns MM, et al. A review of the carcinogenic potential of glyphosate by four independent expert panels and comparison to the IARC assessment. Crit Rev Toxicol. (2016) 46:3–20. doi: 10.1080/10408444.2016.1214677
168. Williams GM, Berry C, Burns M, De Camargo JLV, Greim H. Glyphosate rodent carcinogenicity bioassay expert panel review. Crit Rev Toxicol. (2016) 46:44–55. doi: 10.1080/10408444.2016.1214679
169. Tarone RE. On the International Agency for Research on Cancer classification of glyphosate as a probable human carcinogen. Eur J Cancer Prev. (2018) 27:82–7 doi: 10.1097/CEJ.0000000000000289
170. Paz-Y-Miño C, Sánchez M, Arévalo M, Muño MJ, Witte T, De-la-Carrera GO, et al. Avaliação de dano ao DNA em uma população equatoriana exposta ao glifosato. Genet Mol Biol. (2007) 30:456–60. doi: 10.1590/S1415-47572007000300026
171. Bolognesi C, Carrasquilla G, Volpi S, Solomon KR, Marshall EJP. Biomonitoramento do risco genotóxico em trabalhadores agrícolas de cinco regiões colombianas: associação à exposição ocupacional ao glifosato. J Toxicol Environ Health A. (2009) 72:986–97. doi: 10.1080/15287390902929741
172. Environmental Protection Agency (EPA),. Glyphosate issue paper: evaluation of carcinogenic potential. (2016). Available online at: https://www.epa.gov/sites/production/files/2016-09/documents/glyphosate_issue_paper_evaluation_of_carcincogenic_potential.pdf (accessed February 07, 2023).
Keywords: pesticide, food intake, cancer risk, environmental exposure, Brazil
Citation: Bitencourt de Morais Valentim JM, Fagundes TR, Okamoto Ferreira M, Lonardoni Micheletti P, Broto Oliveira GE, Cremer Souza M, Geovana Leite Vacario B, Silva JCd, Scandolara TB, Gaboardi SC, Zanetti Pessoa Candiotto L, Mara Serpeloni J, Rodrigues Ferreira Seiva F and Panis C (2023) Monitoring residues of pesticides in food in Brazil: A multiscale analysis of the main contaminants, dietary cancer risk estimative and mechanisms associated. Front. Public Health 11:1130893. doi: 10.3389/fpubh.2023.1130893
Received: 23 December 2022; Accepted: 27 January 2023;
Published: 22 February 2023.
Edited by:
Qun Xu, Chinese Academy of Medical Sciences and Peking Union Medical College, ChinaReviewed by:
Octavio Jiménez-Garza, University of Guanajuato, MexicoYangchang Zhang, Capital Medical University, China
Copyright © 2023 Bitencourt de Morais Valentim, Fagundes, Okamoto Ferreira, Lonardoni Micheletti, Broto Oliveira, Cremer Souza, Geovana Leite Vacario, Silva, Scandolara, Gaboardi, Zanetti Pessoa Candiotto, Mara Serpeloni, Rodrigues Ferreira Seiva and Panis. This is an open-access article distributed under the terms of the Creative Commons Attribution License (CC BY). The use, distribution or reproduction in other forums is permitted, provided the original author(s) and the copyright owner(s) are credited and that the original publication in this journal is cited, in accordance with accepted academic practice. No use, distribution or reproduction is permitted which does not comply with these terms.
*Correspondence: Carolina Panis, Y2Fyb2xwYW5pc0Bob3RtYWlsLmNvbQ==