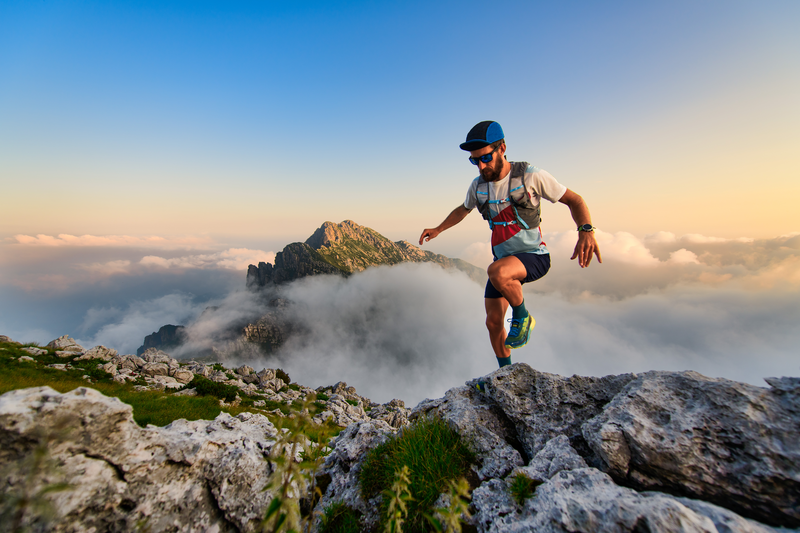
95% of researchers rate our articles as excellent or good
Learn more about the work of our research integrity team to safeguard the quality of each article we publish.
Find out more
ORIGINAL RESEARCH article
Front. Public Health , 13 June 2023
Sec. Infectious Diseases: Epidemiology and Prevention
Volume 11 - 2023 | https://doi.org/10.3389/fpubh.2023.1110903
This article is part of the Research Topic Drivers of Antimicrobial Resistance during and After COVID-19 Pandemic in Low-Middle-Income Countries View all 9 articles
Background: Streptococcus pneumoniae is the most common bacterium that causes community-acquired pneumonia (CAP) in children. The rate of S. pneumoniae resistance to antibiotics is increasing, particularly in patients with severe CAP. Therefore, the level of antibiotic resistance of S. pneumoniae causing severe CAP in Vietnamese children requires regular monitoring.
Methods: This was a cross-sectional descriptive study. Nasopharyngeal aspiration specimens from children were cultured, isolated, and examined for S. pneumoniae. Bacterial strains were assessed for antimicrobial susceptibility, and the minimum inhibitory concentration (MIC) was determined.
Results: Eighty-nine strains of S. pneumoniae were isolated from 239 children with severe CAP. The majority of isolates were completely non-susceptible to penicillin (1.1% intermediate, 98.9% resistant) and highly resistant to erythromycin (96.6%) and clarithromycin (88.8%); the rate of resistance to ceftriaxone was 16.9%, with the proportion of intermediate resistance at 46.0%; 100% of strains were susceptible to vancomycin and linezolid. For most antibiotics, MIC50 and MIC90 were equal to the resistance threshold according to the Clinical and Laboratory Standards Institute 2021; penicillin had an eight-fold increase in MIC90 (64 mg/L) and ceftriaxone had a 1.5-fold increase in MIC90 (6 mg/L).
Conclusion: Streptococcus pneumoniae isolates described in this study were resistant to many antibiotics. Penicillin should not be the first-line antibiotic of choice, and ceftriaxone at an enhanced dose should be used instead.
Streptococcus pneumoniae is the most common bacterium that causes community-acquired pneumonia (CAP) in children (1–3). The burden caused by S. pneumoniae is strongly related to high morbidity and mortality in children under 5 years of age (4). According to the Global Burden of Disease, Injuries, and Risk Factors Study (GBD), in 195 countries in 2016, S. pneumoniae was the cause of more than 341,000 deaths in children under 5 years with lower respiratory tract infections (5).
β-lactam antibiotics are recommended as the primary treatment of infections caused by S. pneumoniae. However, since penicillin-non-susceptible pneumococcus was first described in Australia in 1967 (6), the prevalence rate of resistance to β-lactams among S. pneumoniae strains has been increasing and has become one of the most important antimicrobial-resistant threats worldwide. Recent reports have shown that the rate of penicillin-non-susceptible pneumococci in some countries is 46%, 67.4%, or even up to 97.8% (7–9). Similarly, the emergence of multi-drug resistant (MDR) strains of S. pneumoniae has made it difficult to treat diseases caused by this organism (10, 11). Most cases of severe pneumonia fail with initial antibiotic treatment. Data on the role of disease and antibiotic resistance of S. pneumoniae causing pneumonia in Can Tho, a major city in southern Vietnam, is outdated and needs updating. This study aimed to evaluate the role of pathogens and the level of antibiotic resistance by determining the minimum inhibitory concentration (MIC) for S. pneumoniae causing severe CAP in children in Can Tho City and to clarify the appropriate antibiotic treatment decisions.
The study was performed on 239 children with severe CAP admitted to the Department of Respiratory Medicine, General Internal Medicine, Emergency, and Intensive Care Unit of Can Tho Children's Hospital from March 2020 to February 2021. This study was approved by the Institutional Review Board (IRB) of the Ethics Committee of Biomedical Research of Hanoi Medical University, Hanoi, Vietnam (No. 89/GCN-HDDDNCYSH-DHYHN).
Children aged 2 months to 15 years who were diagnosed with severe pneumonia were included in the present study. The diagnostic criteria of severe CAP were the presence of a cough, dyspnoea and tachypnoea, or chest indrawing, with one of the symptoms such as fever (>38.5°C), hypoxia, cyanosis, short period of breathing pause, dehydration, or oxygen desaturation (< 92%); pneumonia was confirmed by standardized chest X-ray; all the study patients were hospitalized within 48 h.
Patients who met one of the following criteria were excluded from the study: patients or their parents refused to be involved in the study, patients who had a hospitalized stay during the 14 previous days, and pneumonia due to a non-infectious cause.
This was a cross-sectional descriptive study. All patients who met the inclusion criteria were enrolled in the study. The patient underwent clinical examination, biological tests, and chest radiography.
Within 48 h, the nasotracheal aspiration (NTA) specimen from each patient was sent to the laboratory for culture and antimicrobial susceptibility testing (12). The quality of the specimens was checked before processing the culture to confirm that the samples originated from the lower respiratory tract. The number of squamous epithelial cells (SECs) and polymorphonuclear cells (PMNs) in the Gram stain smear was counted for each specimen. The presence of < 10 SECs and >25 PMNs per low-power field (magnification, × 100) was considered a high-quality specimen (13).
NTA specimens were cultured on Mueller Hilton blood agar (MHBA) with 5% sheep blood and incubated for 20–24 h at 35°C with 5% CO2. Suspected colonies were identified by α-haemolysis, Gram (+) staining, optochin sensitivity, and bile solubility tests (Figure 1) (14).
Antimicrobial susceptibility testing (AST) was determined by disc diffusion according to the Kirby-Bauer method (Oxoid Ltd., Basingstoke, UK) for trimethoprim/sulfamethoxazole, chloramphenicol, clindamycin, erythromycin, and oxacillin, and the minimum inhibitory concentration (MIC) was defined as the diffusion of antibiotics in agar from E-test (Bio-Mérieux, Marcy l'Etoile, France) for penicillin, ceftriaxone, ciprofloxacin, levofloxacin, vancomycin, clarithromycin, and linezolid. MIC breakpoints or sterile ring diameters were used to determine antibiotic sensitivity or resistance, as recommended by the manufacturer's instructions for the E-test and disc diffusion, as well as by the Clinical and Laboratory Standards Institute (CLSI) in 2021 (14). All isolated organisms were defined as MDR when resistant to ≥3 antibiotic classes (15). MIC50 and MIC90 were defined as the concentrations at which 50 and 90% of the bacterial strains were inhibited, respectively (14).
Qualitative variables were presented as frequencies and percentages (%). All quantitative variables were presented as mean ± standard deviation or median (min–max). A chi-square test with a 95% confidence interval was used to compare the differences between groups. The p-value of < 0.05 was considered significantly different.
Nasopharyngeal aspiration specimens were collected from 239 children with severe CAP who had been admitted to Can Tho Children's Hospital. Five samples did not meet the required sample quality with >10 squamous cells and < 25 leukocytes, so the culture was not performed; the remaining 234 samples met the required standard. The rate of bacterial isolation was 157/234 (67.1%), of which the percentage of isolated S. pneumoniae was 89/234 (38.0%; Figure 2).
The median age of the children with severe CAP caused by S. pneumoniae was 17 months; the youngest was 2 months and the oldest was 176 months. The most common age group was under 2 years (64.0%) and rarely occurred in children over 5 years (2.3%). Although severe CAP was more frequent among boys than girls, overall and in episodes caused by S. pneumoniae, the percentage of male patients in the complete patients series was significantly higher than in those with severe S. pneumoniae CAP (64% vs. 55.1%; p = 0.032).
Fever and cough were the two most common symptoms of severe CAP. Most children had physical symptoms in the lungs (90.0% crackles), tachypnoea (88.8%), and chest indrawing (55.1%). Wheezing was less common in children infected with S. pneumoniae than in those infected by other microorganisms (p = 0.037), and 80.9% of the CAP cases caused by S. pneumoniae had peripheral blood oxygen saturation (SpO2) ≤ 96%. The mean WBC count was 14.07 ± 5.94 ( × 103/mm3). WBC increased by >15.000/mm3, accounting for 46.1%; the difference was not statistically significant (p = 0.309). Most (>60%) severe CAP cases showed an increase in CRP of >10 mg/L (Table 1).
All isolates of S. pneumoniae were identified as MDR strains. These strains were completely non-susceptible to penicillin; for ceftriaxone, the majority of strains were intermediate (46.0%), followed by susceptible (37.1%); 100% of the strains were susceptible to vancomycin and linezolid (Table 2).
Table 2. Antibiotic susceptibility patterns of Streptococcus pneumoniae isolates from children (n = 89).
Ceftriaxone-non-susceptible strains were more resistant to ciprofloxacin than ceftriaxone-susceptible strains, and this difference was statistically significant (p = 0.048; Table 3).
Table 3. Susceptibility patterns of ceftriaxone-susceptible Streptococcus pneumoniae (n = 33) and ceftriaxone-non-susceptible S. pneumoniae (n = 56).
Table 4 shows the MIC distribution of the antibiotics, and Table 5 shows the MIC50 and MIC90 values of the antibiotic tested against S. pneumoniae. For most antibiotics, MIC50 coincided with MIC90 and equaled the resistance threshold indicated in CLSI 2021; penicillin had an eight-fold increase in MIC90 (64 mg/L) and ceftriaxone had a 1.5-fold increase in MIC90 (6 mg/L).
Table 5. MIC breakpoints according to CLSI in 2021 and MIC50; MIC90 of antibiotics for isolated Streptococcus pneumoniae.
Bacterial culture is the ‘gold standard' for the identification of pneumonia pathogens (17). In this study, the rate of isolation of bacterial agents of pneumonia was 67.1%, which is in agreement with others (18) and higher than others, with rates of 35% and 42% (19, 20). However, the rate reported here was lower than the one reported by Olwagen et al. (21) of 71%. Obviously, the rate of positive bacterial cultures varies widely by studies and countries and is dependent on sampling techniques, storage, culture, incubation, and temperature used, for which standardized techniques are required when working with such specimens (17). In addition, the present study was conducted with patients with severe pneumonia, which explains why the rate of isolated bacteria was so high.
The present study showed that severe CAP caused by S. pneumoniae (and other microorganisms) was common, mainly in children under 2 years of age, and followed by 2–5 years. This finding is consistent with what is in literature, with many studies recognizing that children under 5 years of age are more susceptible to pneumonia than older children, and tend to suffer a worse prognosis of the disease (18, 22). The proportion of boys with severe CAP due to pneumococci was higher than in girls, a finding which is in agreement with other studies (23, 24). Of the children with severe CAP, 93.3% did not have pneumococcal immunization status and only three (1.3%) were vaccinated. However, all these three were infected with pneumococci, which begs the question of which S. pneumoniae serotypes infected the children in the study group and that matched the serotypes of the vaccine used? This is also a limitation of this study, which is being implemented as a follow-up study in this area. A study in a northern province of Vietnam identified eight serotypes of S. pneumoniae isolated from unvaccinated children under 5 years of age with pneumonia, including 19F, 23F, 19A, 6A/B, 15A, 9V, 11A, and 14 (25).
The present study showed that most children with CAP caused by S. pneumoniae had peripheral blood oxygen saturation (SpO2) ≤ 96%. Some recent studies have shown that there are no signs or symptoms sufficient to confirm pneumonia in children, but diagnostic specificity can be improved when some clinical features, such as tachypnoea, fever, and hypoxia, are present (26, 27). A decline in SpO2 is also a valuable parameter for diagnosing pneumonia. According to Shah et al. (28), the presence of moderate hypoxaemia and increased breathing effort are the most relevant signs of pneumonia. In the present study, wheezing was less common in children infected with S. pneumoniae than in those infected by other microorganisms. A previous study demonstrated that wheezing was more common in atypical or viral pneumonia than in typical bacterial pneumonia (29).
The present study suggests that WBC is not a reliable indicator of the severity of the disease (Table 1), which is consistent with a previous study showing that WBC was a poor indicator of the etiology and severity of pneumonia (24). Esposito et al. (30) highlighted that WBC count had the lowest positive predictive value compared to procalcitonin and CRP. The present study found that up to 65.2% of children with severe CAP due to S. pneumoniae had an increase in CRP levels >10 mg/L. CRP is also considered an acute-phase reactant associated with disease severity in children with bacterial infection (31).
In this study, the majority of S. pneumoniae isolates were identified as MDR, with 98.9% of the isolates completely non-susceptible to penicillin and others. In recent years, the proportion of penicillin non-susceptible pneumococci has increased to over 60% (7–9). Consequently, penicillin is no longer the first-line antibiotic for children with severe CAP in many countries; clinicians are using cefotaxime and ceftriaxone, a third-generation cephalosporin, instead (32, 33). In this study, the rate of S. pneumoniae resistant to ceftriaxone was only 16.9%; however, the percentage of intermediate resistance was 46.0%. This result raises great concern about the likelihood of first-line antibiotic failure. When comparing the sensitivity of antibiotics between the two groups of ceftriaxone-susceptible and ceftriaxone-non-susceptible S. pneumoniae, ceftriaxone-non-susceptible strains were still sensitive to vancomycin (100%), linezolid (100%), and chloramphenicol (93.8%). A previous study on antimicrobial susceptibility testing for isolated S. pneumoniae also showed a low susceptibility rate to all antibiotics except vancomycin (100%), linezolid (100%), and levofloxacin (89.5%) (34). According to the results of a study conducted by Sweden et al., the proportion of MDR S. pneumoniae susceptible to vancomycin and levofloxacin was 100% (16). However, chloramphenicol is rarely used orally and intravenously in Vietnamese children due to its common side effects such as blood dyscrasias, aplastic anemia, and leukemia (35, 36). Therefore, vancomycin and linezoild may be suitable alternative antibiotics for severe MDR pneumococcal pneumonia.
Macrolide antibiotics have become less effective against S. pneumoniae, with high resistance rates reported in the present study for erythromycin (96.6%) and clarithromycin (88.8%). Other studies have found that S. pneumoniae is more resistant to macrolides and does not support the routine combination of β-lactam antibiotics with macrolides (16, 37). However, in contrast, other studies have suggested that macrolide combination therapy is more effective than β-lactam monotherapy, especially in children >5 years of age or in atypical bacterial co-infections with pathogens such as Mycoplasma pneumoniae (38, 39).
Analysis of the distribution of MIC, MIC50, and MIC90 among the studied S. pneumoniae isolates revealed that penicillin had an eight-fold increase in MIC90 (64 mg/L). This finding suggests that ceftriaxone is more suitable than penicillin for the initial treatment of severe CAP caused by S. pneumoniae. However, based on our study findings, an increase in the dose of ceftriaxone is needed, as evidenced by the 1.5-fold increase in MIC90 for this antibiotic in this study. Recommendations for how much to increase the dose depends on the pharmacokinetic/pharmacodynamic (pK/pD) breakpoint, which is another interesting follow-up to this study. In recent years, many recommendations have been made to increase the therapeutic dose of ceftriaxone to 100 mg/kg/day for severe infections or in places with insignificant penicillin resistance (≥25%) (33, 40).
In the present study, the fluoroquinolones, ciprofloxacin and levofloxacin, had a fairly wide MIC range of 0.18–128 and 0.18–24 mg/L, respectively. These results suggest that it may be difficult to choose a therapeutic dose of ciprofloxacin. Moreover, if a strain of S. pneumoniae was not susceptible to ceftriaxone, it was likely that it would not be susceptible to ciprofloxacin, although the rate of ciprofloxacin susceptibility to S. pneumoniae was 59.6%. Although there have been very few reports of treatment failure with ciprofloxacin, this antibiotic was not considered a “respiratory fluoroquinolone” in the United States (41). Indeed, the CLSI does not provide ciprofloxacin breakpoints for S. pneumoniae (14). Furthermore, ciprofloxacin is not recommended for the treatment of respiratory tract infections in most treatment guidelines (33, 42). In fact, the systemic use of fluoroquinolones in patients under 18 years is not recommended in many countries due to eventual adverse musculoskeletal effects (43). Therefore, extreme caution should be exercised when using ciprofloxacin to treat children with severe CAP.
Our study showed a high prevalence rate of S. pneumoniae strains resistant to many antibiotics, including penicillin-resistant strains with high MICs, among strains from children with severe CAP. Therefore, penicillin should not be the first-line antibiotic of choice, and ceftriaxone at an enhanced dose should be used instead.
The original contributions presented in the study are included in the article/supplementary material, further inquiries can be directed to the corresponding author.
The studies involving human participants were reviewed and approved by Review Board Committee for Ethics Committee in Biomedical Research of Hanoi Medical University, Hanoi, Vietnam (No. 89/GCN-HDDDNCYSH-DHYHN). Written informed consent to participate in this study was provided by the participants' legal guardian/next of kin.
Conceptualization, resources, and investigation: KT-Q, TN-T-D, HT-D, and VP-H. Software: HT-D, BT-X, ML, and SD-Q. Formal analysis: TN-T-D, SD-Q, HT-D, TN-V, BT-X, and ML. Manuscript writing, review, and editing: KT-Q, TN-T-D, HT-D, TN-V, BT-X, ML, and SD-Q. All authors have read, commented upon, and approved the final manuscript.
This work was partially supported by the International Research of the Gene and Immunology Institute, Laboratory of Nam Khoa Biotek Company, Ho Chi Minh City, Vietnam.
The authors would like to thank Can Tho University of Medicine and Pharmacy, Can Tho's Children Hospital, and Mr. Van Hung Pham from the International Research of Gene and Immunology Institute for their technical support.
The authors declare that the research was conducted in the absence of any commercial or financial relationships that could be construed as a potential conflict of interest.
All claims expressed in this article are solely those of the authors and do not necessarily represent those of their affiliated organizations, or those of the publisher, the editors and the reviewers. Any product that may be evaluated in this article, or claim that may be made by its manufacturer, is not guaranteed or endorsed by the publisher.
1. Tramper-Stranders GA. Childhood community-acquired pneumonia: a review of etiology- and antimicrobial treatment studies. Paediatr Respir Rev. (2018) 26:41–8. doi: 10.1016/j.prrv.2017.06.013
2. Mathew JL. Etiology of childhood pneumonia: what we know, and what we need to know! : based on 5th Dr. Ic Verma Excellence Oration Award. Indian J Pediatr. (2018) 85:25–34. doi: 10.1007/s12098-017-2486-y
3. Marangu D, Zar HJ. Childhood pneumonia in low-and-middle-income countries: an update. Paediatr Respir Rev. (2019) 32:3–9. doi: 10.1016/j.prrv.2019.06.001
4. Wahl B, O'Brien KL, Greenbaum A, Majumder A, Liu L, Chu Y, et al. Burden of Streptococcus pneumoniae and Haemophilus influenzae type B disease in children in the era of conjugate vaccines: global, regional, and national estimates for 2000-15. Lancet Glob Health. (2018) 6:e744–57. doi: 10.1016/S2214-109X(18)30247-X
5. GBD 2016 Lower Respiratory Infections Collaborators. GBD 2016 lower respiratory infections collaborators. Estimates of the global, regional, and national morbidity, mortality, and aetiologies of lower respiratory infections in 195 countries, 1990-2016: a systematic analysis for the global burden of disease study 2016. Lancet Infect Dis. (2018) 18:1191–210. doi: 10.1016/S1473-3099(18)30310-4
6. Hansman D, Bullen MM. A resistant pneumococcus. Lancet. (1967) 2:264–5. doi: 10.1016/S0140-6736(67)92346-X
7. Lee JK, Yun KW, Choi EH, Kim SJ, Lee SY, Lee HJ. Changes in the serotype distribution among antibiotic resistant carriage Streptococcus pneumoniae isolates in children after the introduction of the extended-valency pneumococcal conjugate vaccine. J Korean Med Sci. (2017) 32:1431–9. doi: 10.3346/jkms.2017.32.9.1431
8. Moore CE, Giess A, Soeng S, Sar P, Kumar V, Nhoung P, et al. Characterisation of invasive Streptococcus pneumoniae isolated from cambodian children between 2007 - 2012. PLoS ONE. (2016) 11:e0159358. doi: 10.1371/journal.pone.0159358
9. Yu YY, Xie XH, Ren L, Deng Y, Gao Y, Zhang Y, et al. Epidemiological characteristics of nasopharyngeal Streptococcus pneumoniae strains among children with pneumonia in Chongqing, China. Sci Rep. (2019) 9:3324. doi: 10.1038/s41598-019-40088-6
10. Beheshti M, Jabalameli F, Feizabadi MM, Hahsemi FB, Beigverdi R, Emaneini M. Molecular characterization, antibiotic resistance pattern and capsular types of invasive Streptococcus pneumoniae isolated from clinical samples in Tehran, Iran. BMC Microbiol. (2020) 20:167. doi: 10.1186/s12866-020-01855-y
11. Liang Z, Fu J, Li L, Yi R, Xu S, Chen J, et al. Molecular epidemiology of Streptococcus pneumoniae isolated from pediatric community-acquired pneumonia in pre-conjugate vaccine era in Western China. Ann Clin Microbiol Antimicrob. (2021) 20:4. doi: 10.1186/s12941-020-00410-x
12. Pham VH. Sputum, Nasotracheal Aspiration, Ronchoalveolar Lavage and Sputum Culture, Specimen Containing Sputum. Clinical Microbiological Testing Technique. Hanoi, Vietnam: Ministry of Health, Medical Publisher (2006), p. 67–77.
13. Murdoch DR, Morpeth SC, Hammitt LL, Driscoll AJ, Watson NL, Baggett HC, et al. Microscopic analysis and quality assessment of induced sputum from children with pneumonia in the Perch Study. Clin Infect Dis. (2017) 64(suppl_3):S271–9. doi: 10.1093/cid/cix083
14. Clinical and Laboratory Standards Institute (CLSI). Performance Standards for Antimicrobial Susceptibility Testing. CLSI Supplement M100, 31st ed. Wayne, PA: Clinical and Laboratory Standards Institute (2021).
15. Sweeney MT, Lubbers BV, Schwarz S, Watts JL. Applying definitions for multidrug resistance, extensive drug resistance and pandrug resistance to clinically significant livestock and companion animal bacterial pathogens. J Antimicrob Chemother. (2018) 73:1460–3. doi: 10.1093/jac/dky043
16. Smith MJ. Macrolides and pediatric community-acquired pneumonia-time for a paradigm shift? JAMA Pediatr. (2017) 171:1147–8. doi: 10.1001/jamapediatrics.2017.3828
17. Charlton CL, Babady E, Ginocchio CC, Hatchette TF, Jerris RC, Li Y, et al. Practical guidance for clinical microbiology laboratories: viruses causing acute respiratory tract infections. Clin Microbiol Rev. (2019) 32:e00042–18. doi: 10.1128/CMR.00042-18
18. Tran Quang K, Tran Do H, Pham Hung V, Nguyen Vu T, Tran Xuan B, Larsson M, et al. Study on the co-infection of children with severe community-acquired pneumonia. Pediatr Int. (2022) 64:e14853. doi: 10.1111/ped.14853
19. Sutcliffe CG, Grant LR, Cloessner E, Klugman KP, Vidal JE, Reid R, et al. Association of laboratory methods, colonization density, and age with detection of Streptococcus pneumoniae in the nasopharynx. Am J Epidemiol. (2019) 188:2110–9. doi: 10.1093/aje/kwz191
20. Jakhar SK, Pandey M, Shah D, Ramachandran VG, Saha R, Gupta N, et al. Etiology and risk factors determining poor outcome of severe pneumonia in under-five children. Indian J Pediatr. (2018) 85:20–4. doi: 10.1007/s12098-017-2514-y
21. Olwagen CP, Adrian PV, Madhi SA. Comparison of traditional culture and molecular qPCR for detection of simultaneous carriage of multiple pneumococcal serotypes in African children. Sci Rep. (2017) 7:4628. doi: 10.1038/s41598-017-04915-y
22. Marcelo CS, Paulo JCM, Renato TS. Pneumonia in children. In:Wilmott RW, , editor. Kendig's Disorders of the Respiratory Tract in Children, 9th ed. Philadelphia: PA: Elsevier (2018), p. 1597–644.
23. Jonnalagadda S, Rodríguez O, Estrella B, Sabin LL, Sempértegui F, Hamer DH. Etiology of severe pneumonia in ecuadorian children. PLoS ONE. (2017) 12:e0171687. doi: 10.1371/journal.pone.0171687
24. Williams DJ, Zhu Y, Grijalva CG, Self WH, Harrell FE Jr, Reed C, et al. Predicting severe pneumonia outcomes in children. Pediatrics. (2016) 138:e20161019. doi: 10.1542/peds.2016-1019
25. Son BA, Hai TX, Van Cuong T, Chinh DD, Le TH, Dung NM, et al. Serotype distribution and antibiotic resistance of Streptococcus pneumoniae isolates collected from unvaccinated children with pneumonia at a Province in Central Vietnam. Iran J Microbiol. (2022) 14:653–61. doi: 10.18502/ijm.v14i5.10958
26. Hirsch AW, Monuteaux MC, Neuman MI, Bachur RG. Estimating risk of pneumonia in a prospective emergency department cohort. J Pediatr. (2019) 204:172–6.e1. doi: 10.1016/j.jpeds.2018.08.077
27. Rees CA, Basnet S, Gentile A, Gessner BD, Kartasasmita CB, Lucero M, et al. An analysis of clinical predictive values for radiographic pneumonia in children. BMJ Glob Health. (2020) 5:e2708. doi: 10.1136/bmjgh-2020-002708
28. Shah SN, Bachur RG, Simel DL, Neuman MI. Does this child have pneumonia?: the rational clinical examination systematic review. JAMA. (2017) 318:462–71. doi: 10.1001/jama.2017.9039
29. Guo WL, Wang J, Zhu LY, Hao CL. Differentiation between Mycoplasma and viral community-acquired pneumonia in children with lobe or multi foci infiltration: a retrospective case study. BMJ Open. (2015) 5:e006766. doi: 10.1136/bmjopen-2014-006766
30. Esposito S, Bianchini S, Gambino M, Madini B, Di Pietro G, Umbrello G, et al. Measurement of lipocalin-2 and syndecan-4 levels to differentiate bacterial from viral infection in children with community-acquired pneumonia. BMC Pulm Med. (2016) 16:103. doi: 10.1186/s12890-016-0267-4
31. Jain S, Williams DJ, Arnold SR, Ampofo K, Bramley AM, Reed C, et al. Community-acquired pneumonia requiring hospitalization among US children. N Engl J Med. (2015) 372:835–45. doi: 10.1056/NEJMoa1405870
32. Breuer O, Blich O, Cohen-Cymberknoh M, Averbuch D, Kharasch S, Shoseyov D, et al. Antibiotic treatment for children hospitalized with community-acquired pneumonia after oral therapy. Pediatr Pulmonol. (2015) 50:495–502. doi: 10.1002/ppul.23159
33. Bradley JS, Byington CL, Shah SS, Alverson B, Carter ER, Harrison C, et al. The management of community-acquired pneumonia in infants and children older than 3 months of age: clinical practice guidelines by the pediatric infectious diseases society and the infectious diseases society of America. Clin Infect Dis. (2011) 53:e25–76. doi: 10.1093/cid/cir531
34. El-Kholy A, Badawy M, Gad M, Soliman M. Serotypes and antimicrobial susceptibility of nasopharyngeal isolates of Streptococcus pneumoniae from children less than 5 years old in Egypt. Infect Drug Resist. (2020) 13:3669–77. doi: 10.2147/IDR.S250315
35. Barnhill AE, Brewer MT, Carlson SA. Adverse effects of antimicrobials via predictable or idiosyncratic inhibition of host mitochondrial components. Antimicrob Agents Chemother. (2012) 56:4046–51. doi: 10.1128/AAC.00678-12
36. Fraumeni JF Jr. Bone marrow depression induced by chloramphenicol or phenylbutazone. Leukemia and other sequelae. JAMA. (1967) 201:828–34. doi: 10.1001/jama.201.11.828
37. Williams DJ, Edwards KM, Self WH, Zhu Y, Arnold SR, McCullers JA, et al. Effectiveness of β-lactam monotherapy vs macrolide combination therapy for children hospitalized with pneumonia. JAMA Pediatr. (2017) 171:1184–91. doi: 10.1001/jamapediatrics.2017.3225
38. Al Saeedy D, Gillani SW, Al-Salloum J, Moosvi A, Eissa M, Gulam SM. Comparative efficacy of beta-lactams and macrolides in the treatment of pediatric pneumonia: a systematic review. Curr Pediatr Rev. (2020) 16:307–13. doi: 10.2174/1573396316666200907115800
39. Ambroggio L, Taylor JA, Tabb LP, Newschaffer CJ, Evans AA, Shah SS. Comparative effectiveness of empiric β-lactam monotherapy and β-lactam-macrolide combination therapy in children hospitalized with community-acquired pneumonia. J Pediatr. (2012) 161:1097–103. doi: 10.1016/j.jpeds.2012.06.067
40. Harris M, Clark J, Coote N, Fletcher P, Harnden A, McKean M, et al. British thoracic society guidelines for the management of community acquired pneumonia in children: update 2011. Thorax. (2011) 66(Suppl 2):ii1–23. doi: 10.1136/thoraxjnl-2011-200598
41. Rapp RP. Fluoroquinolone positioning in hospital antimicrobial stewardship programs. US Pharm. (2007) 32:10–7.
42. Vardakas KZ, Siempos II, Grammatikos A, Athanassa Z, Korbila IP, Falagas ME. Respiratory fluoroquinolones for the treatment of community-acquired pneumonia: a meta-analysis of randomized controlled trials. CMAJ. (2008) 179:1269–77. doi: 10.1503/cmaj.080358
Keywords: Streptococcus pneumoniae, antibiotics resistance, community-acquired pneumonia, children, Vietnam
Citation: Tran-Quang K, Nguyen-Thi-Dieu T, Tran-Do H, Pham-Hung V, Nguyen-Vu T, Tran-Xuan B, Larsson M and Duong-Quy S (2023) Antibiotic resistance of Streptococcus pneumoniae in Vietnamese children with severe pneumonia: a cross-sectional study. Front. Public Health 11:1110903. doi: 10.3389/fpubh.2023.1110903
Received: 15 December 2022; Accepted: 25 May 2023;
Published: 13 June 2023.
Edited by:
Tauqeer Hussain Mallhi, Jouf University, Saudi ArabiaReviewed by:
Mar Olga Pérez-Moreno, Hospital de Tortosa Verge de la Cinta, SpainCopyright © 2023 Tran-Quang, Nguyen-Thi-Dieu, Tran-Do, Pham-Hung, Nguyen-Vu, Tran-Xuan, Larsson and Duong-Quy. This is an open-access article distributed under the terms of the Creative Commons Attribution License (CC BY). The use, distribution or reproduction in other forums is permitted, provided the original author(s) and the copyright owner(s) are credited and that the original publication in this journal is cited, in accordance with accepted academic practice. No use, distribution or reproduction is permitted which does not comply with these terms.
*Correspondence: Sy Duong-Quy, sduongquy.jfvp@gmail.com
†These authors share first authorship
Disclaimer: All claims expressed in this article are solely those of the authors and do not necessarily represent those of their affiliated organizations, or those of the publisher, the editors and the reviewers. Any product that may be evaluated in this article or claim that may be made by its manufacturer is not guaranteed or endorsed by the publisher.
Research integrity at Frontiers
Learn more about the work of our research integrity team to safeguard the quality of each article we publish.