- 1Department of Family Medicine, Wan Fang Hospital, Taipei Medical University, Taipei, Taiwan
- 2Department of Public Health, School of Medicine, College of Medicine, Taipei Medical University, Taipei, Taiwan
- 3Department of Medicine, Section of Epidemiology and Population Sciences, Baylor College of Medicine, Houston, TX, United States
- 4Department of Chemistry, Fu Jen Catholic University, New Taipei City, Taiwan
- 5Department of Chinese Medicine, College of Medicine, Chang Gung University, Taoyuan, Taiwan
- 6Division of Nephrology, Department of Internal Medicine, School of Medicine, College of Medicine, Taipei Medical University, Taipei, Taiwan
- 7Division of Nephrology, Department of Internal Medicine, Taipei Medical University Hospital, Taipei, Taiwan
- 8Department of Family Medicine, School of Medicine, College of Medicine, Taipei Medical University, Taipei, Taiwan
- 9Department of Geriatric Medicine, School of Medicine, College of Medicine, Taipei Medical University, Taipei, Taiwan
Introduction: Prior studies indicate that exposure to metals may alter DNA methylation. Evidence also shows that global DNA methylation is associated with chronic kidney disease (CKD). This study aimed to examine the association between CKD and 5-methyl-2-deoxycytidine (5mdC, %), a marker of global DNA methylation, and to evaluate the interaction between metal exposures and 5mdC (%) on CKD. We also explored the mediation effect of 5mdC (%) on the association between metal exposures and renal function (i.e., estimated glomerular filtration rate, eGFR).
Methods: A total of 218 CKD patients and 422 controls were recruited in this case–control study. 5mdC (%), concentrations of blood lead and cadmium, plasma selenium, and total urinary arsenic were measured. CKD cases were clinically defined among patients with eGFR <60 mL/min/1.73 m2 for at least 3 months and without hemodialysis. Odds ratio (OR) and 95% confidence interval (CI) were estimated by logistic regression models to examine the association between metal exposures, 5mdC (%), and CKD, adjusted for confounders. Multivariable linear regression models were used to examine associations between metal exposures, 5mdC (%), and eGFR.
Results and Discussion: CKD cases compared to controls had 6.06-fold (95% CI: 3.11–11.81) higher odds of having high blood cadmium and high 5mdC (%) levels. A positive interaction on an additive scale was identified between blood cadmium and 5mdC (%) on CKD. Cases compared to controls had 4.73-fold (95% CI: 2.65–8.45) higher odds of having low plasma selenium and high 5mdC (%) levels; and a significant multiplicative interaction between plasma selenium and 5mdC (%) on CKD was observed. In addition, we found that blood lead and cadmium concentrations were positively associated, while plasma selenium concentrations were inversely associated, with 5mdC (%). The associations of blood lead and plasma selenium with eGFR were partially mediated by 5mdC (%). Our results suggest that 5mdC (%) may interact with plasma selenium and blood cadmium to influence the risk of CKD. The 5mdC (%) also potentially mediates the associations between exposure to metals and renal function.
1. Introduction
Chronic kidney disease (CKD) is a worldwide public health problem, with an estimated global mean prevalence of 13.4% (1). In Taiwan, the estimated prevalence rate of CKD is as high as 15.46%, and the incidence of CKD is 27.21/1,000 person-years (2). Moreover, end-stage renal disease is widespread in Taiwan, which leads to the highest incidence of renal dialysis therapy in the world (3). Therefore, CKD is a very important issue in Taiwan.
We previously found associations of increasing levels of total urinary arsenic and blood lead and cadmium with reduced estimated glomerular filtration rate (eGFR), while increasing levels of plasma selenium were associated with increasing eGFR (4, 5). Although our studies provided evidence of associations of exposure to arsenic, lead, cadmium, and selenium with CKD (4, 5), the biological mechanism underlying these associations remains unclear. One hypothesis mechanism is that chronic inflammation may cause abnormal DNA methylation and followed by causing glomerular and tubular interstitial lesions (6). DNA methylation is an epigenetic marker that promotes the transfer of methyl groups to cytosine, thereby affecting the expression of CpG rich genes that have an essential role in cell function and maintaining genomic stability (7). Evidence has shown that alteration of DNA methylation in peripheral immune cells has a profound impact on the development of diabetes-or hypertension-related CKD (8). Methylation of DNA cytosine (5-methyl-2-deoxycytidine, 5mdC), a recognized global DNA methylation marker, is the product of the base excision repair and nucleotide excision repair pathways of active DNA methylation (9) and is involved in genome imprinting, X chromosome inactivation, and gene expression regulation (10). Measuring the ratio of 5mdC to 2-deoxyguanine (dG) provides an accurate method for estimating total genomic DNA methylation levels (11, 12). 5mdC (%) is the best-characterized epigenetic modification in mammals (9). Thus, the use of 5mdC (%) may allow exploring whether global DNA methylation is associated with CKD.
Several studies to date have implicated exposure to metals in altering global DNA methylation. Low levels of lead exposure were associated with changes in DNA methylation related to neurodevelopment in a study among Peruvian adults who resided close to mining activities (13). Additionally, a Mexican study found that prenatal lead exposure was associated with global DNA methylation in adolescence (14). Long-term cadmium exposure reduces antioxidant capacity, causes oxidative damage to lipids and DNA, and reduces global DNA methylation levels in juvenile Nile tilapia (15). A review study summarized evidence from in vitro, animal, and human studies, and suggested an inverse association between selenium and global DNA methylation (16); however, another study recently found that selenium was positively associated with DNA methylation (17). Chronic arsenic exposure in parents leads to changes in global DNA methylation and transgenerational genotoxicity, which may be associated with reproductive defects in rats (18). A review paper has summarized environmental exposures, including air pollution, metals, persistent organic compounds, etc., that were associated with DNA methylation in the human population (19). In a recent systematic review encompassing epidemiologic studies and toxicologic animal evidence (20), Elkin et al. concluded that exposure to lead, arsenic, and cadmium was associated with DNA methylation signatures in adults, though replication testing is needed. As epigenetic signatures may have an important biologic effect of metal exposures, continued and expanded studies are needed to understand the role of global DNA methylation in the association with metal exposure-related diseases such as CKD.
The present study aimed to explore the role of global DNA methylation in the association between exposure to metals and CKD. We first evaluated the association of global DNA methylation, using 5mdC (%) as an indicator, with CKD. Secondly, we examined the interaction between exposure to metals and 5mdC (%) on CKD. Given a limited number of prior studies conducted interaction analysis between metal exposures and DNA methylation, both multiplicative and additive scales were conducted in our study to test for the interaction. In addition, we conducted a mediation analysis to explore the mediation effect of 5mdC (%) on the association between exposure to metals and renal function (i.e., eGFR).
2. Materials and methods
2.1. Study subjects
A clinic-based case–control study was conducted among clinically confirmed 218 CKD patients and 422 controls recruited from Taipei Medical University Hospital and Taipei Municipal Wan Fang Hospital (21). Frequency matching was employed in our study to balance the overall distributions of age and sex between cases and controls. Clinical confirmed CKD patients were recruited at the Department of Internal Medicine and Nephrology and diagnosed based on biochemical criteria, including blood urea nitrogen, proteinuria, and serum creatinine. eGFR (mL/min/1.73 m2) was calculated by using the equation from the Modified Diet Formula for Renal Disease: 186.3 × (serum creatinine)-1.154 × (age)-0.203 × 0.742 (if female) (22). According to the clinical practice guidelines from the Kidney Disease Outcomes Quality Initiative (23), patients with eGFR <60 mL/min/1.73 m2 for at least 3 months and without hemodialysis were classified as CKD. Healthy controls with no evidence of CKD were recruited from the Department of Family Medicine. All participants provided written informed consent before being recruited in the study. This study was approved by the Institutional Review Board of Taipei Medical University (N201912103), which was conducted in accordance with the Declaration of Helsinki.
2.2. Specimen collection and covariates
Peripheral blood samples were collected at enrollment in an EDTA-vacuum syringe and separated as plasma, red blood cells, and buffy coat. Urine samples were also collected in sterile polypropylene containers.
All participants completed an interview for the collection of data on sociodemographic characteristics, lifestyle, and disease histories (21). Covariates included sex, age (years), educational level (illiterate/elementary school, junior/senior high school, and college and above), cigarette smoking (non-smoker, former smoker, and current smoker), alcohol, tea, and coffee consumption (never/occasional or frequently), analgesic usage (no, as-needed basis, and routinely), and personal history of hypertension and diabetes (yes/no).
2.3. Metal exposures measurement
As described previously (5), blood lead and cadmium and plasma selenium were quantified by inductively coupled plasma mass spectrometry. Urinary arsenic species including arsenite (AsIII), arsenate (AsV), monomethylarsonic acid (MMAV), and dimethylarsinic acid (DMAV), were measured by high-performance liquid chromatography-hydride generator-atomic absorption spectrometry (21). Concentrations of total urinary arsenic were defined as the sum of the concentrations of AsIII, AsV, MMAV, and DMAV, and then divided by the urinary creatinine concentrations to adjust for hydration status (24). The detection limits, standard reference materials, and reliability of the metal measurements are shown in Supplementary Table S1.
2.4. Global DNA methylation markers: 5mdC measurement
Genomic DNA was extracted by digestion with proteinase K followed by phenol and chloroform. The levels of 5mdC were quantified by high-performance liquid chromatography (Agilent 1260VL, Agilent Technology, United States) equipped with an API 3000™ triple quadrupole mass spectrometer (AB SCIEX, Canada). The data were processed in the multiple reaction monitoring mode through Analyst1.4.2™ software (AB SCIEX). The detailed protocol of the analysis was described previously (11). The levels of 5mdC in the DNA sample were expressed as a percentage of the total cytosine content (methylated and non-methylated) using the following equation: 5mdC (%) = [(5mdC/dG) × 100] (9).
2.5. Statistical analysis
All data were analyzed using SAS 9.4 software (SAS Institute, Cary, NC, United States). A two-sided value of p < 0.05 was considered significant. Characteristics were summarized using the mean ± standard deviation for continuous variables and frequency (percentage) for categorical variables. The Wilcoxon rank-sum test was conducted to compare the metal concentrations and levels of 5mdC (%) between CKD cases and controls. Logistic regression models were used to evaluate the associations between metal exposures, 5mdC (%), and CKD by estimating odds ratio (OR) and 95% confidence interval (CI). The concentrations of metals and levels of 5mdC (%) were categorized into tertiles according to the distribution of the controls, and the lowest tertile was used as the reference group in the logistic regression models. Cochran–Armitage test was used for assessing the linear trend for ORs. All models included in interaction and mediation analyses were adjusted for frequency-matching variables (i.e., age and sex) and confounding. Confounding was informed by prior knowledge and met the criterion that was associated with CKD (value of p < 0.05; i.e., educational level, consumption of alcohol, coffee, and tea, analgesic usage, and personal history of hypertension and diabetes).
2.5.1. Interaction analysis
To explore whether 5mdC (%) was an effect modifier of the association between exposure to metals and CKD, a product term combining the metal exposure and 5mdC (%) was added to the logistic regression models for testing multiplicative interaction. A value of p for interaction term <0.05 is indicative of a multiplicative interaction between metal exposures and 5mdC (%). We also examined the interaction between metal exposures and 5mdC (%) on CKD based on the additive scale. We classified the concentrations of metals and levels of 5mdC (%) based on their median levels according to the distribution of the controls and create a combined exposure variable [e.g., low lead/low 5mdC (%), low lead/high 5mdC (%), high lead/low 5mdC (%), and high lead/high 5mdC (%)]. This combined variable was assessed for its association with CKD in the logistic regression models. The synergy index (SI), relative excess risk due to interaction (RERI), and the attributable proportion (AP) were calculated to quantify additive interactions (25).
2.5.2. Mediation analysis
Our study conducted the mediation analysis using linear regression models, estimated coefficient (β), and standard error (SE), to explore the mediation effect of 5mdC (%) on the association between exposure to metals and renal function (i.e., eGFR). The concentrations of metal, 5mdC (%), and eGFR were assessed as a continuous measure in the models. The test of mediating effect was conducted using the Sobel test (26). The path diagrams were shown to present the total effect [represents the effect of metals on eGFR without controlling for 5mdC (%)], direct effect [represents the effect of metals on eGFR after controlling for 5mdC (%)], and indirect effect [represents the effect of metals on eGFR through 5mdC (%)]. The proportion of mediation was estimated by the ratio of indirect effect to total effect (27).
3. Results
3.1. Participant characteristics
The sociodemographic characteristics, lifestyle, and disease histories between CKD cases and controls are shown in Table 1. The mean and standard deviation of age in the 218 CKD cases and 422 controls were 65.04 ± 13.46 and 64.26 ± 12.63 years, respectively. The CKD cases had a lower educational level compared to controls, with most cases either illiterate or having an elementary school education. No difference in smoking status was observed between cases and controls. More than one-third of controls reported having a habit of consuming alcohol occasionally or frequently compared to the cases. Additionally, over half of the controls reported consuming coffee and tea occasionally or frequently. Of cases, 12.39% reported routinely using analgesics, which was higher than controls. A higher percentage of cases had disease histories of diabetes and hypertension compared to controls.
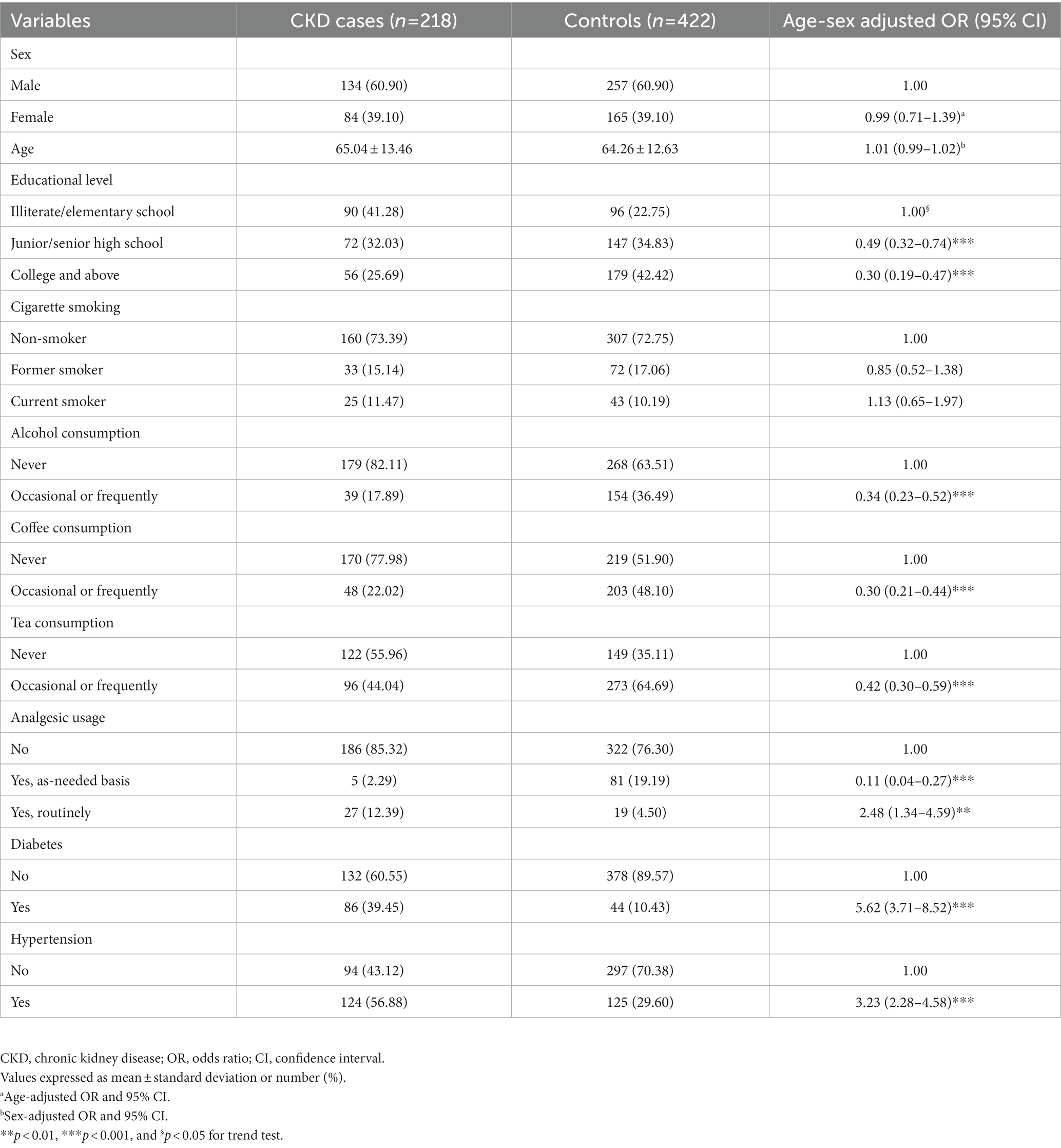
Table 1. Sociodemographic characteristics, lifestyle, and disease histories between the CKD cases and controls.
3.2. Associations between metal exposures, 5mdC (%), and CKD
Table 2 shows the associations between metal exposures, 5mdC (%), and CKD. In multivariable adjusted models, CKD cases compared to controls had 9.00-fold (95% CI: 4.83–16.75) higher odds of having blood lead levels >46.88 μg/dL than blood lead levels ≤28.68 μg/dL. Cases compared to controls had 10.36-fold (95% CI: 5.50–19.54) higher odds of having blood cadmium levels >1.30 μg/L than blood cadmium levels ≤0.82 μg/L. In contrast, cases compared to controls had 0.15-fold (95%CI: 0.08–0.26) lower odds of having plasma selenium levels >243.8 μg/L than plasma selenium levels ≤196.0 μg/L. The 5mdC (%) levels were higher in CKD cases compared to controls (mean ± standard deviation: 5.08 ± 2.51 vs. 4.23 ± 1.77). After adjusting for multivariable in the models, cases compared to controls had 2.14-fold (95%CI: 1.33–3.44) higher odds of having 5mdC levels >4.55% than 5mdC levels ≤3.60%.
3.3. The interaction between metal exposures and 5mdC (%) on CKD
Table 3 shows the combined effects of metal exposures and 5mdC (%) on CKD. After adjusting for multivariable in the models, cases compared to controls had 6.49-fold (95%CI: 3.41–12.36) higher odds of having blood lead levels >37.51 μg/dL and 5mdC levels >4.10% than blood lead levels ≤37.51 μg/dL and 5mdC levels ≤4.10%. Also, the ORs increased gradually from no risk factors [low levels of blood lead and 5mdC (%)], one risk factor [high levels of blood lead or 5mdC (%)], to two risk factors [high levels of blood lead and 5mdC (%)]. Similar associations were also observed in assessing the combined effect of blood cadmium and total urinary arsenic with 5mdC (%) on CKD. A significant positive interaction on an additive scale was identified between blood cadmium and 5mdC (%) on CKD [synergy index = 2.90 (1.13–7.45), RERI = 3.13 (0.62–6.00) and AP = 0.55 (0.29–0.81)], indicating that combined effect was more than the sum of the effects of having blood cadmium levels >1.30 μg/L and 5mdC levels >4.10%. We found that the odds of having plasma selenium levels ≤217.70 μg/L and 5mdC levels >4.10% were higher among CKD cases compared to controls [multivariable adjusted OR (95% CI) = 4.73 (2.65–8.45) relative to the low plasma selenium levels and high 5mdC (%) levels]. We observed a significant multiplicative interaction between plasma selenium and 5mdC (%) on CKD (interaction p < 0.001).
3.4. Mediation effect of 5mdC (%) on the association between metal exposures and eGFR
The associations between exposure to metals, 5mdC (%), and eGFR are displayed in Figure 1. Increases in blood lead and cadmium and total urinary arsenic were each associated with a decrease of eGFR, while an increase of plasma selenium was associated with an increase of eGFR (see arrow of total effect in Figure 1). These associations remained significant after further adjusting 5mdC (%) in the models though the estimated effect was slightly attenuated (see arrow of direct effect in Figure 1). In addition, blood cadmium and lead were associated with increased 5mdC (%), while plasma selenium was associated with reduced 5mdC (%). However, we observed no association between total urinary arsenic and 5mdC (%). Additionally, an increase of 1% in 5mdC (%) was significantly associated with a decrease of 2.475 mL/min/1.73 m2 of eGFR (see arrow of indirect effect in Figure 1). In order to test whether 5mdC (%) may have mediated the associations between exposure to metals and CKD, the Sobel test was conducted among the observed associations of Figure 1. The mediating effect of 5mdC (%) was significant in the associations of blood lead (p < 0.01) and plasma selenium (p < 0.01) with eGFR. However, the test of the mediating effect for blood cadmium (p = 0.05) and total urinary arsenic (p = 0.24) was not significant. The 5mdC (%) mediated 6.7 and 14.9% of the associations of blood lead and plasma selenium, respectively, with eGFR in the mediation analysis.
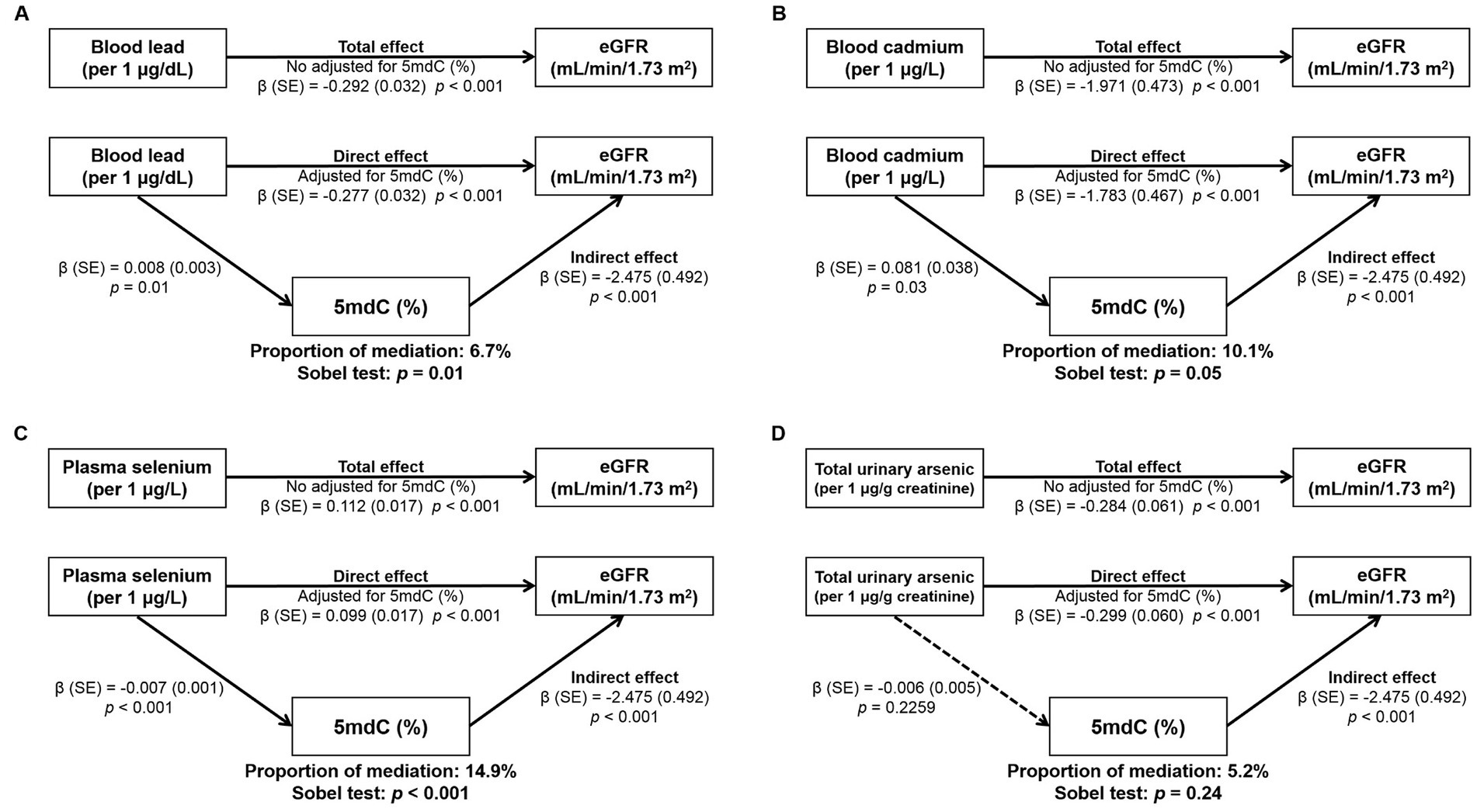
Figure 1. Mediation analysis of the estimated effect of exposure to metals on eGFR through 5mdC: (A) blood lead, (B) blood cadmium, (C) plasma selenium, and (D) total urinary arsenic. Effect estimates were adjusted for age, sex, educational level, consumption of alcohol, coffee, and tea, analgesic usage, and personal history of hypertension and diabetes.
4. Discussion
The present study added to the current knowledge of assessing associations between exposure to metals, 5mdC (%), and CKD. The higher odds of elevated 5mdC (%) were observed among CKD cases compared to controls. We also found a significant synergic effect of blood cadmium and 5mdC (%) on CKD; and a significant multiplicative interacted effect of plasma selenium and 5mdC (%) on CKD. In addition, we observed that blood lead and cadmium concentrations were significantly positively associated, but plasma selenium was significantly negatively associated, with 5mdC (%). Our study is the first to explore the mediating role of 5mdC (%) in the associations between exposure to metals and renal function (i.e., eGFR) and showed that 5mdC (%) might partially mediate the effects of blood lead and plasma selenium on eGFR.
Our study found that the odds of occasional or frequent alcohol, coffee, and tea consumption were lower in CKD cases than in controls, which is consistent with the results from other studies (28–30). These protective effects may be because coffee contains antioxidant and anti-inflammatory components (31), and alcohol and tea contain polyphenols that can reduce reactive oxygen species and anti-inflammatory components (32, 33). We also found that frequent analgesics use, hypertension, and diabetes were significantly associated with CKD, and these results were similar to other studies (34, 35).
Epigenetic modifications, including DNA methylation, are heritable patterns that modulate gene expression without altering DNA sequence (36), and have been implicated in the development of CKD (37). Thus, understanding the role of DNA methylation involved in the etiology of CKD may provide insights into the pathophysiology, severity, and prognosis of kidney disease (38). Evidence from in vitro and in vivo has supported that aberrant DNA methylation was associated with diabetic kidney disease and the progression of renal fibrosis (39, 40). While not focusing on global DNA methylation, studies have identified DNA hypomethylation and hypermethylation at specific loci among CKD patients (41) and found that specific DNA methylation was associated with a reduction in kidney function (42). In the present study, we observed that increased levels of 5mdC (%), a global DNA methylation indicator, were associated with decreased eGFR, and also CKD cases compared to controls had higher odds of having elevated 5mdC levels. This is consistent with a Japanese prospective study that recruited 308 CKD patients (43), which showed that concentrations of 5mdC (measured in urine specimens) were significantly higher among late-stage compared to early-to mid-stages patients, and increased 5mdC levels were associated with low eGFR (<30 mL/min/1.73m2; OR = 2.36, 95%CI: 1.24–4.60) in the multivariable adjusted model. However, in a cross-sectional study of 78 kidney disease patients among stages 2–4 conducted in the Netherlands (44), no differences were found between the levels of global DNA methylation (measured by the ratio of 5-methylcytosine and total cytosine ratio in the blood specimens) and kidney disease stages. Using different indicators of global DNA methylation and study design may be reasons for the inconsistencies observed between our study and the Netherlands’ study. Nevertheless, Ingrosso and Perna reviewed current evidence from in vitro, in vivo, and human studies regarding the role of epigenetic alterations in renal disease and suggested that DNA methylation, either global or gene-specific, may be a prospective biomarker of CKD (45).
Previous in vitro and in vivo studies have implicated that exposure to metals may alter DNA methylation. A study of cell-line experiments found that cadmium exposure initially induced DNA hypomethylation, but prolonged exposure resulted in DNA hypermethylation (46). In an animal study, exposure to cadmium-and lead-contaminated soils caused tissue metal accumulation and epigenetic alterations (increased global DNA methylation levels) in rats (47). An inverse association between selenium and global DNA methylation was also shown in vitro, animal, and human studies (16). In the present study, we observed that increased blood lead or cadmium levels were associated with increasing 5mdC (%), while increased plasma selenium levels were associated with decreasing 5mdC (%). Our results were consistent with a Mexican birth cohort study (n = 144) which observed prenatal exposure to lead (measured from maternal blood specimens) was associated with elevated levels of lead exposure-related gene-specific DNA methylation among their children ages 11–18 (14). In a Taiwanese cross-sectional study that enrolled 738 participants of ages 12–30, Lin et al. observed that increased urinary concentrations of lead and cadmium were associated with 5mdC (%) when adjusting for covariates related to cardiovascular disease (48). In contrast to our observed association, in a Norwegian prospective birth cohort study (631 mother–child pair), Weyde et al. found that maternal blood selenium concentrations during pregnancy were positively associated with global DNA methylation (measured by 5-methylcytosine in percentage) both in mothers and newborns (49). Additionally, in a study that consisted of 202 Argentinian women with low levels of environmental cadmium exposure (median blood cadmium concentration: 0.36 μg/L), Hossian et al. found that urinary cadmium levels were inversely associated with global DNA methylation (measured by long interspersed nuclear element-1) (50). Previous studies in Bangladesh have supported that arsenic exposure is associated with global DNA methylation and epigenetic alterations can serve as biomarkers of arsenic toxicity (51, 52), inconsistent with our finding of a lack of association between total urinary arsenic and 5mdC (%). The urinary concentrations of arsenic found in both Bangladeshi studies (median urinary arsenic concentrations range from 137.3 to 201.5 μg/g creatinine for three cohorts) (51, 52) were extremely higher than those found in our study population, which may be one reason for the inconsistent findings. Differences in study designs and populations, exposure matrices, and indicators of global DNA methylation may lead to discrepant results when assessing the association between metal exposures and global DNA methylation. Further studies are needed to gain a better understanding of the association between exposure to metals and DNA methylation.
Our study showed that 5mdC (%) partially mediates the associations of blood lead or plasma selenium with eGFR. The observed mediated result may be reasonable because exposure to environmental toxic metals (e.g., lead) may induce inflammation through altered DNA methylation and, thus, reduce eGFR (53, 54). One study noted that specific DNA methylation levels in urine (SMTNL2 and G6PC), reflecting the proportion of exfoliated proximal tubule cells due to injury, were significantly associated with decreased eGFR (55). Additionally, in an epigenomic association study of kidney function and blood DNA methylation levels of 69 CpGs associated with declines in eGFR were identified, and most eGFR-related CpGs were also significantly associated with CKD and microalbuminuria (56). While not focusing on renal function, in a Taiwanese cross-sectional study of 738 young participants aged 12–30 (48), Lin et al. found that 5mdC (%) may mediate the association between urinary lead and carotid intima-media thickness, a marker of subclinical atherosclerosis. By reducing oxidative stress, it is possible that the association between selenium and increased eGFR may by decreasing global DNA methylation (57). A Chinese Kashin–Beck disease study found that selenium treatment decreased the methylation of O6-methylguanine-DNA methyltransferase, a DNA damage-repair gene that reduces chondrocyte damage by T-2 toxin (58). Also, selenium can significantly prevent tert-butyl hydroperoxide-induced chondrocyte apoptosis, mediated by reducing glutathione peroxidase 3 DNA methylation and increasing glutathione peroxidase 3 gene expression (59).
In this study, we found a significant synergic effect of blood cadmium and 5mdC (%) on CKD, possibly because exposure to cadmium may increase the production of reactive oxygen species and thereby cause oxidative stress. Excessive cellular reactive oxygen species can lead to damage to proteins, nucleic acids, lipids, membranes, and organelles, which are associated with various diseases, including CKD (60). In addition, DNA hypermethylation of specific genes that increase oxidative stress (57) and lead to renal fibrosis (61), together with high blood cadmium, increase the risk of CKD and this needs to be further explored. Our study also found that a significant multiplicative interaction effect of plasma selenium and 5mdC (%) on CKD. The reason may be that a high selenium state reduces inflammation and oxidative stress (62), followed by reducing DNA methylation lightening inflammation, and improving kidney function (54). Additionally, the present study identified a mediating role of global DNA methylation on the association between selenium and renal function based on the classical mediation approach that assumes linear association and no interaction among the variables. As our study also found suggestive evidence of interaction between selenium and 5mdC (%) on CKD, future prospective research with the more complex setting of exposure-mediator interactions (63) is warranted to accommodate in order to elucidate the relationship between metal exposures, global DNA methylation, and the risk of CKD.
Our study had several limitations. The case–control study findings should be interpreted cautiously in describing the temporal relationship between metal exposures, global DNA methylation, and eGFR, especially when interpreting the results of mediation analysis. Additionally, we calculated the interaction effects in the log-odds scale due to the case–control design. The OR estimates might be slightly biased as the high prevalence of CKD in the Taiwanese population (2). However, our results may inform future studies with a prospective design to enhance understanding of the underlying mechanism of DNA methylation in the etiology of exposure to metals and CKD. Second, plasma selenium, blood cadmium and lead, and total urinary arsenic concentrations were only assessed in urine and blood samples that were collected once, and so a possible misclassification error cannot be ruled out. However, these measured values may be reliable if all subjects maintained their usual lifestyle. Last, the statistical power may be limited because the number of samples in this study was relatively small; therefore, further work with a large sample size is warranted to provide adequate power.
5. Conclusion
In conclusion, our study found joint impacts of global DNA methylation marker, using 5mdC (%) as an indicator, with blood cadmium or plasma selenium on CKD, which supports the hypothesis of the adverse impacts of exposure to metals on CKD being modified by global DNA methylation. In addition, the present study provides suggestive evidence that global DNA methylation may partially mediate the association between exposure to metals (i.e., lead and selenium) and renal function measured as eGFR. This provides insights into the mechanisms of global DNA methylation for the metal exposures related to CKD.
Data availability statement
The datasets presented in this article are not readily available because of privacy and ethical restrictions. Requests to access the datasets should be directed to the corresponding author.
Ethics statement
The studies involving human participants were reviewed and approved by Institutional Review Board of Taipei Medical University (N201912103). The patients/participants provided their written informed consent to participate in this study.
Author contributions
Y-MH, Y-CL, and H-SS partly contributed to the conception and design of the work. H-HC, Y-CL, and W-JC recruited the study subjects. H-LL has done the experiment. Y-MH, W-JC, and Y-LH contributed to the statistical analysis and analyzed the data. Y-MH wrote the original manuscript. W-JC, Y-LH, and S-LH reviewed and edited the manuscript. Y-CL performed the study design and executed the whole research plan. All authors contributed to the article and approved the submitted version.
Funding
This study was supported by grants from the Ministry of Science and Technology of Taiwan (MOST 106-2314-B-038-066, MOST 107-2320-B-039-010, MOST 106-2314-B-002-235-MY3, MOST 107-2314-B-038-073, MOST 108-2314-B-038 -089, MOST 109-2314-B-038-081, MOST 109-2314-B-038-067, and MOST 110-2314-B-038-054). This study received no specific grant from any funding agency in the public, commercial, or not-for-profit sectors.
Conflict of interest
The authors declare that the research was conducted in the absence of any commercial or financial relationships that could be construed as a potential conflict of interest.
Publisher’s note
All claims expressed in this article are solely those of the authors and do not necessarily represent those of their affiliated organizations, or those of the publisher, the editors and the reviewers. Any product that may be evaluated in this article, or claim that may be made by its manufacturer, is not guaranteed or endorsed by the publisher.
Supplementary material
The Supplementary material for this article can be found online at: https://www.frontiersin.org/articles/10.3389/fpubh.2023.1104692/full#supplementary-material
References
1. Hill, NR, Fatoba, ST, Oke, JL, Hirst, JA, O'Callaghan, CA, Lasserson, DS, et al. Global prevalence of chronic kidney disease—a systematic review and Meta-analysis. PLoS One. (2016) 11:e0158765. doi: 10.1371/journal.pone.0158765
2. Tsai, MH, Hsu, CY, Lin, MY, Yen, MF, Chen, HH, Chiu, YH, et al. Incidence, prevalence, and duration of chronic kidney disease in Taiwan: results from a community-based screening program of 106, 094 individuals. Nephron. (2018) 140:175–84. doi: 10.1159/000491708
3. Lin, YC, Hsu, CY, Kao, CC, Chen, TW, Chen, HH, Hsu, CC, et al. Incidence and prevalence of ESRD in Taiwan renal registry data system (TWRDS): 2005-2012. Acta Nephrologica. (2014) 28:65–6. doi: 10.6221/AN.2014011
4. Hsueh, YM, Chung, CJ, Shiue, HS, Chen, JB, Chiang, SS, Yang, MH, et al. Urinary arsenic species and CKD in a Taiwanese population: a case-control study. Am J Kidney Dis. (2009) 54:859–70. doi: 10.1053/j.ajkd.2009.06.016
5. Wu, CY, Wong, CS, Chung, CJ, Wu, MY, Huang, YL, Ao, PL, et al. The association between plasma selenium and chronic kidney disease related to lead, cadmium and arsenic exposure in a Taiwanese population. J Hazard Mater. (2019) 375:224–32. doi: 10.1016/j.jhazmat.2019.04.082
6. Akchurin, OM, and Kaskel, F. Update on inflammation in chronic kidney disease. Blood Purif. (2015) 39:84–92. doi: 10.1159/000368940
7. Greenberg, MVC, and Bourc'his, D. The diverse roles of DNA methylation in mammalian development and disease. Nat Rev Mol Cell Biol. (2019) 20:590–607. doi: 10.1038/s41580-019-0159-6
8. Chen, XJ, Zhang, H, Yang, F, Liu, Y, and Chen, G. DNA methylation sustains "inflamed" memory of peripheral immune cells aggravating kidney inflammatory response in chronic kidney disease. Front Physiol. (2021) 12:637480. doi: 10.3389/fphys.2021.637480
9. Song, L, James, SR, Kazim, L, and Karpf, AR. Specific method for the determination of genomic DNA methylation by liquid chromatography-electrospray ionization tandem mass spectrometry. Anal Chem. (2005) 77:504–10. doi: 10.1021/ac0489420
10. Chen, Y, Hong, T, Wang, S, Mo, J, Tian, T, and Zhou, X. Epigenetic modification of nucleic acids: from basic studies to medical applications. Chem Soc Rev. (2017) 46:2844–72. doi: 10.1039/C6CS00599C
11. Chung, CJ, Chang, CH, Liou, SH, Liu, CS, Liu, HJ, Hsu, LC, et al. Relationships among DNA hypomethylation, cd, and Pb exposure and risk of cigarette smoking-related urothelial carcinoma. Toxicol Appl Pharmacol. (2017) 316:107–13. doi: 10.1016/j.taap.2016.12.016
12. Liou, SH, Wu, WT, Liao, HY, Chen, CY, Tsai, CY, Jung, WT, et al. Global DNA methylation and oxidative stress biomarkers in workers exposed to metal oxide nanoparticles. J Hazard Mater. (2017) 331:329–35. doi: 10.1016/j.jhazmat.2017.02.042
13. Childebayeva, A, Goodrich, JM, Chesterman, N, Leon-Velarde, F, Rivera-Ch, M, Kiyamu, M, et al. Blood lead levels in Peruvian adults are associated with proximity to mining and DNA methylation. Environ Int. (2021) 155:106587. doi: 10.1016/j.envint.2021.106587
14. Rygiel, CA, Goodrich, JM, Solano-Gonzalez, M, Mercado-Garcia, A, Hu, H, Tellez-Rojo, MM, et al. Prenatal Lead (Pb) exposure and peripheral blood DNA methylation (5mC) and Hydroxymethylation (5hmC) in Mexican adolescents from the ELEMENT birth cohort. Environ Health Perspect. (2021) 129:067002. doi: 10.1289/EHP8507
15. Hu, F, Yin, L, Dong, F, Zheng, M, Zhao, Y, Fu, S, et al. Effects of long-term cadmium exposure on growth, antioxidant defense and DNA methylation in juvenile Nile tilapia (Oreochromis niloticus). Aquat Toxicol. (2021) 241:106014. doi: 10.1016/j.aquatox.2021.106014
16. Speckmann, B, and Grune, T. Epigenetic effects of selenium and their implications for health. Epigenetics. (2015) 10:179–90. doi: 10.1080/15592294.2015.1013792
17. Zhang, Q, Zheng, S, Wang, S, Jiang, Z, and Xu, S. The effects of low selenium on DNA methylation in the tissues of chickens. Biol Trace Elem Res. (2019) 191:474–84. doi: 10.1007/s12011-019-1630-0
18. Nava-Rivera, LE, Betancourt-Martinez, ND, Lozoya-Martinez, R, Carranza-Rosales, P, Guzman-Delgado, NE, Carranza-Torres, IE, et al. Transgenerational effects in DNA methylation, genotoxicity and reproductive phenotype by chronic arsenic exposure. Sci Rep. (2021) 11:8276. doi: 10.1038/s41598-021-87677-y
19. Martin, EM, and Fry, RC. Environmental influences on the epigenome: exposure-associated DNA methylation in human populations. Annu Rev Public Health. (2018) 39:309–33. doi: 10.1146/annurev-publhealth-040617-014629
20. Elkin, ER, Higgins, C, Aung, MT, and Bakulski, KM. Metals exposures and DNA methylation: current evidence and future directions. Curr Environ Health Rep. (2022) 9:673–96. doi: 10.1007/s40572-022-00382-4
21. Chen, WJ, Huang, YL, Shiue, HS, Chen, TW, Lin, YF, Huang, CY, et al. Renin-angiotensin-aldosterone system related gene polymorphisms and urinary total arsenic is related to chronic kidney disease. Toxicol Appl Pharmacol. (2014) 279:95–102. doi: 10.1016/j.taap.2014.05.011
22. Levey, AS, Bosch, JP, Lewis, JB, Greene, T, Rogers, N, and Roth, D. A more accurate method to estimate glomerular filtration rate from serum creatinine: a new prediction equation. Modification of diet in renal disease study group. Ann Intern Med. (1999) 130:461–70. doi: 10.7326/0003-4819-130-6-199903160-00002
23. KDIGO Clinical practice guideline for the evaluation and management of chronic kidney disease. (2012) 2012.
24. Barr, DB, Wilder, LC, Caudill, SP, Gonzalez, AJ, Needham, LL, and Pirkle, JL. Urinary creatinine concentrations in the U.S. population: implications for urinary biologic monitoring measurements. Environ Health Perspect. (2005) 113:192–200. doi: 10.1289/ehp.7337
25. Vander Weele, TJ, and Knol, MJ. A tutorial on interaction. Epidemiol Methods. (2014) 3:33–72. doi: 10.1097/EDE.0000000000000121
26. Sobel, ME. Asymptotic confidence intervals for indirect effects in structural equation models. Sociol Methodol. (1982) 13:290–312. doi: 10.2307/270723
27. Ditlevsen, S, Christensen, U, Lynch, J, Damsgaard, MT, and Keiding, N. The mediation proportion: a structural equation approach for estimating the proportion of exposure effect on outcome explained by an intermediate variable. Epidemiology. (2005) 16:114–20. doi: 10.1097/01.ede.0000147107.76079.07
28. Li, Y, Zhu, B, Song, N, Shi, Y, Fang, Y, and Ding, X. Alcohol consumption and its association with chronic kidney disease: evidence from a 12-year China health and nutrition survey. Nutr Metab Cardiovasc Dis. (2022) 32:1392–401. doi: 10.1016/j.numecd.2022.02.012
29. Tang, L, Yang, L, Chen, W, Li, C, Zeng, Y, Yang, H, et al. Sex-specific association between coffee consumption and incident chronic kidney disease: a population-based analysis of 359, 906 participants from the UK biobank. Chin Med J. (2022) 135:1414–24. doi: 10.1097/CM9.0000000000002234
30. Zhang, Y, Xiong, Y, Shen, S, Yang, J, Wang, W, Wu, T, et al. Causal association between tea consumption and kidney function: a Mendelian randomization study. Front Nutr. (2022) 9:801591. doi: 10.3389/fnut.2022.801591
31. van Dam, RM, Hu, FB, and Willett, WC. Coffee, caffeine, and health. N Engl J Med. (2020) 383:369–78. doi: 10.1056/NEJMra1816604
32. Hsu, SP, Wu, MS, Yang, CC, Huang, KC, Liou, SY, Hsu, SM, et al. Chronic green tea extract supplementation reduces hemodialysis-enhanced production of hydrogen peroxide and hypochlorous acid, atherosclerotic factors, and proinflammatory cytokines. Am J Clin Nutr. (2007) 86:1539–47. doi: 10.1093/ajcn/86.5.1539
33. Rodrigo, R, and Bosco, C. Oxidative stress and protective effects of polyphenols: comparative studies in human and rodent kidney. A review. Comp Biochem Physiol C Toxicol Pharmacol. (2006) 142:317–27. doi: 10.1016/j.cbpc.2005.11.002
34. Akokuwebe, ME, and Idemudia, ES. Knowledge and risk perceptions of chronic kidney disease risk factors among women of childbearing age in Lagos state, Nigeria: from a health demography approach. Int J Nephrol. (2022) 2022:5511555. doi: 10.1155/2022/5511555
35. Gansevoort, RT, Correa-Rotter, R, Hemmelgarn, BR, Jafar, TH, Heerspink, HJ, Mann, JF, et al. Chronic kidney disease and cardiovascular risk: epidemiology, mechanisms, and prevention. Lancet. (2013) 382:339–52. doi: 10.1016/S0140-6736(13)60595-4
36. Wing, MR, Ramezani, A, Gill, HS, Devaney, JM, and Raj, DS. Epigenetics of progression of chronic kidney disease: fact or fantasy? Semin Nephrol. (2013) 33:363–74. doi: 10.1016/j.semnephrol.2013.05.008
37. Tain, YL, and Hsu, CN. Developmental origins of chronic kidney disease: should we focus on early life? Int J Mol Sci. (2017) 18:381. doi: 10.3390/ijms18020381
38. Larkin, BP, Glastras, SJ, Chen, H, Pollock, CA, and Saad, S. DNA methylation and the potential role of demethylating agents in prevention of progressive chronic kidney disease. FASEB J. (2018) 32:5215–26. doi: 10.1096/fj.201800205R
39. Reddy, MA, and Natarajan, R. Epigenetics in diabetic kidney disease. J Am Soc Nephrol. (2011) 22:2182–5. doi: 10.1681/ASN.2011060629
40. Larkin, BP, Saad, S, Glastras, SJ, Nguyen, LT, Hou, M, Chen, H, et al. Low-dose hydralazine during gestation reduces renal fibrosis in rodent offspring exposed to maternal high fat diet. PLoSOne. (2021) 16:e0248854. doi: 10.1371/journal.pone.0248854
41. Smyth, LJ, McKay, GJ, Maxwell, AP, and McKnight, AJ. DNA hypermethylation and DNA hypomethylation is present at different loci in chronic kidney disease. Epigenetics. (2014) 9:366–76. doi: 10.4161/epi.27161
42. Wing, MR, Devaney, JM, Joffe, MM, Xie, D, Feldman, HI, Dominic, EA, et al. DNA methylation profile associated with rapid decline in kidney function: findings from the CRIC study. Nephrol Dial Transplant. (2014) 29:864–72. doi: 10.1093/ndt/gft537
43. Onishi, A, Sugiyama, H, Kitagawa, M, Yamanari, T, Tanaka, K, Ogawa-Akiyama, A, et al. Urine 5MedC, a marker of DNA methylation, in the progression of chronic kidney disease. Dis Markers. (2019) 2019:1–10. doi: 10.1155/2019/5432453
44. Nanayakkara, PW, Kiefte-de Jong, JC, Stehouwer, CD, van Ittersum, FJ, Olthof, MR, Kok, RM, et al. Association between global leukocyte DNA methylation, renal function, carotid intima-media thickness and plasma homocysteine in patients with stage 2-4 chronic kidney disease. Nephrol Dial Transplant. (2008) 23:2586–92. doi: 10.1093/ndt/gfn040
45. Ingrosso, D, and Perna, AF. DNA methylation dysfunction in chronic kidney disease. Genes (Basel). (2020) 11:811. doi: 10.3390/genes11070811
46. Takiguchi, M, Achanzar, WE, Qu, W, Li, G, and Waalkes, MP. Effects of cadmium on DNA-(Cytosine-5) methyltransferase activity and DNA methylation status during cadmium-induced cellular transformation. Exp Cell Res. (2003) 286:355–65. doi: 10.1016/S0014-4827(03)00062-4
47. Nakayama, SMM, Nakata, H, Ikenaka, Y, Yabe, J, Oroszlany, B, Yohannes, YB, et al. One year exposure to cd-and Pb-contaminated soil causes metal accumulation and alteration of global DNA methylation in rats. Environ Pollut. (2019) 252:1267–76. doi: 10.1016/j.envpol.2019.05.038
48. Lin, CY, Lee, HL, Hwang, YT, Huang, PC, Wang, C, Sung, FC, et al. Urinary heavy metals, DNA methylation, and subclinical atherosclerosis. Ecotoxicol Environ Saf. (2020) 204:111039. doi: 10.1016/j.ecoenv.2020.111039
49. Weyde, KVF, Olsen, AK, Duale, N, Kamstra, JH, Skogheim, TS, Caspersen, IH, et al. Gestational blood levels of toxic metal and essential element mixtures and associations with global DNA methylation in pregnant women and their infants. Sci Total Environ. (2021) 787:147621. doi: 10.1016/j.scitotenv.2021.147621
50. Hossain, MB, Vahter, M, Concha, G, and Broberg, K. Low-level environmental cadmium exposure is associated with DNA hypomethylation in Argentinean women. Environ Health Perspect. (2012) 120:879–84. doi: 10.1289/ehp.1104600
51. Niedzwiecki, MM, Hall, MN, Liu, X, Oka, J, Harper, KN, Slavkovich, V, et al. A dose-response study of arsenic exposure and global methylation of peripheral blood mononuclear cell DNA in Bangladeshi adults. Environ Health Perspect. (2013) 121:1306–12. doi: 10.1289/ehp.1206421
52. Demanelis, K, Argos, M, Tong, L, Shinkle, J, Sabarinathan, M, Rakibuz-Zaman, M, et al. Association of Arsenic Exposure with whole blood DNA methylation: an epigenome-wide study of Bangladeshi adults. Environ Health Perspect. (2019) 127:057011. doi: 10.1289/EHP3849
53. Ray, PD, Yosim, A, and Fry, RC. Incorporating epigenetic data into the risk assessment process for the toxic metals arsenic, cadmium, chromium, lead, and mercury: strategies and challenges. Front Genet. (2014) 5:201. doi: 10.3389/fgene.2014.00201
54. Stenvinkel, P, Karimi, M, Johansson, S, Axelsson, J, Suliman, M, Lindholm, B, et al. Impact of inflammation on epigenetic DNA methylation—a novel risk factor for cardiovascular disease? J Intern Med. (2007) 261:488–99. doi: 10.1111/j.1365-2796.2007.01777.x
55. Marumo, T, Hoshino, J, Kawarazaki, W, Nishimoto, M, Ayuzawa, N, Hirohama, D, et al. Methylation pattern of urinary DNA as a marker of kidney function decline in diabetes. BMJ Open Diabetes Res Care. (2020) 8:e001501. doi: 10.1136/bmjdrc-2020-001501
56. Schlosser, P, Tin, A, Matias-Garcia, PR, Thio, CHL, Joehanes, R, Liu, H, et al. Meta-analyses identify DNA methylation associated with kidney function and damage. Nat Commun. (2021) 12:7174. doi: 10.1038/s41467-021-27234-3
57. Jia, HH, Xu, YT, Yin, ZP, Wu, XM, Qing, M, Fan, YJ, et al. Transcriptomes and DNA methylomes in apomictic cells delineate nucellar embryogenesis initiation in citrus. DNA Res. (2021) 28:1–16. doi: 10.1093/dnares/dsab014
58. Zhang, D, Zhang, D, Wang, C, Zhang, R, Li, Q, and Xiong, Y. Mechanism of DNA methylation-mediated downregulation of O6-Methylguanine-DNA methyltransferase in cartilage injury of Kashin-Beck disease. Rheumatology (Oxford). (2021) 61:3471–80. doi: 10.1093/rheumatology/keab913
59. Han, L, Yang, X, Sun, W, Li, Z, Ren, H, Li, B, et al. The study of GPX3 methylation in patients with Kashin-Beck disease and its mechanism in chondrocyte apoptosis. Bone. (2018) 117:15–22. doi: 10.1016/j.bone.2018.08.017
60. Unsal, V, Dalkiran, T, Cicek, M, and Kolukcu, E. The role of natural antioxidants against reactive oxygen species produced by cadmium toxicity: a review. Adv Pharm Bull. (2020) 10:184–202. doi: 10.34172/apb.2020.023
61. Yu, Y, Guan, X, Nie, L, Liu, Y, He, T, Xiong, J, et al. DNA hypermethylation of sFRP5 contributes to indoxyl sulfate-induced renal fibrosis. J Mol Med. (2017) 95:601–13. doi: 10.1007/s00109-017-1538-0
62. Alehagen, U, Aaseth, J, Alexander, J, Brismar, K, and Larsson, A. Selenium and coenzyme Q10 supplementation improves renal function in elderly deficient in selenium: observational results and results from a subgroup analysis of a prospective randomised double-blind placebo-controlled trial. Nutrients. (2020) 12:3780. doi: 10.3390/nu12123780
Keywords: 5-methyl-2-deoxycytidine, arsenic, cadmium, lead, selenium, chronic kidney disease
Citation: Hsueh Y-M, Chen W-J, Lee H-L, Huang Y-L, Shiue H-S, Hsu S-L, Chen H-H and Lin Y-C (2023) Global DNA methylation and the association between metal exposure and chronic kidney disease. Front. Public Health. 11:1104692. doi: 10.3389/fpubh.2023.1104692
Edited by:
Renata Sisto, National Institute for Insurance against Accidents at Work (INAIL), ItalyReviewed by:
Folarin Owagboriaye, Olabisi Onabanjo University, NigeriaCesar Higgins, University of Michigan, United States
Copyright © 2023 Hsueh, Chen, Lee, Huang, Shiue, Hsu, Chen and Lin. This is an open-access article distributed under the terms of the Creative Commons Attribution License (CC BY). The use, distribution or reproduction in other forums is permitted, provided the original author(s) and the copyright owner(s) are credited and that the original publication in this journal is cited, in accordance with accepted academic practice. No use, distribution or reproduction is permitted which does not comply with these terms.
*Correspondence: Ying-Chin Lin, Z3JlZW4xOTkwQHRtdS5lZHUudHc=