- 1The Emergency Center, Zhongnan Hospital of Wuhan University, Wuhan, Hubei, China
- 2The Second Clinical School, Wuhan University, Wuhan, Hubei, China
Background: Previous studies have explored the correlation between short-term exposure to air pollution and urinary system diseases, but lack of evidence on the correlation between air pollution and urolithiasis.
Methods: Daily data of emergency department visits (EDVs), concentrations of six air pollutants (SO2, NO2, PM2.5, PM10, CO, and O3) and meteorological variables were collected in Wuhan, China, from 2016 to 2018. And a time-series study was conducted to investigate short-term effects of air pollutants on urolithiasis EDVs. In addition, stratified analyses by season, age and gender were also conducted.
Results: A total of 7,483 urolithiasis EDVs were included during the study period. A 10-μg/m3 increase of SO2, NO2, PM2.5, CO, PM10, and O3 corresponded to 15.02% (95% confidence interval [CI]: 1.69%, 30.11%), 1.96% (95% CI: 0.19%, 3.76%), 1.09% (95% CI:−0.24%, 2.43%), 0.14% (95% CI: 0.02%, 0.26%), 0.72% (95% CI: 0.02%, 1.43%), and 1.17% (95% CI: 0.40%, 1.94%) increases in daily urolithiasis EDVs. Significant positive correlations were observed between SO2, NO2, CO, and O3 and urolithiasis EDVs. The correlations were mainly among females (especially PM2.5 and CO) and younger people (especially SO2, NO2, and PM10) but the effect of CO was more obvious in elders. Furthermore, the effects of SO2 and CO were stronger in warm seasons, while the effects of NO2 were stronger in cool seasons.
Conclusion: Our time-series study indicates that short-term exposure to air pollution (especially SO2, NO2, CO, and O3) was positively correlated with EDVs for urolithiasis in Wuhan, China, and the effects varied by season, age and gender.
1. Introduction
Urolithiasis, a common urinary system disease, including stone in kidney, ureter, bladder, and urethra. Although rarely life-threatening, urolithiasis can often cause intense pain and is associated with increased risks of urinary tract infection, hydronephrosis, and renal function decline (1). In recent decades, urolithiasis's incidence rate and prevalence have continued to rise worldwide and its burden mainly concentrated in Asia and Eastern Europe (41). In the analysis of geographical differences, the prevalence rates of kidney stones were higher in the hot south and southwest regions of China (3). A present study indicated that currently kidney stones affect about one in 17 adults (the adjusted prevalence rate was 5.8%; 6.5% in men and 5.1% in women) in China (3). Renal colic caused by urolithiasis is a common complaint in the emergency department and a significant burden for healthcare systems and emergency departments (4), and it is estimated that this burden is likely to increase over time (39). Therefore, an improved understanding of the risk factors of urolithiasis is of great significance to public health. Some studies have explored the correlation between urolithiasis and diet habits, obesity, diabetes, metabolic syndrome, fluid intake, and environmental factors, such as geographical and meteorological factors (5–7). Among them, the correlations between seasonal changes, ambient temperature and urolithiasis have been extensively studied (7). However, the correlation between air pollutants, the important components of the exposed air environment, and urolithiasis has rarely been explored.
In recent years, air pollution has been considered as one of the crucial factors threatening human health. Exposure to ambient air pollution is associated with an increased risk of global mortality and incidence rate from various diseases, especially in developing countries (8, 9). Several studies have also reported that air pollutants may affect renal function through oxidative stress, inflammatory responses, and other mechanisms, leading to the development of a range of urological diseases, such as chronic kidney disease and renal failure (10, 11, 40). Oxidative stress and inflammation have been suggested as the potential mechanistic pathways affecting stone formation (12, 13). Therefore, we hypothesized that exposure to air pollution might also be correlated with an increased risk of urolithiasis. Our study will be beneficial for hospitals to understand the potential risk of urolithiasis from exposure to ambient air pollution to take precautions and maintain order on particularly heavily polluted days.
Given the above contents, we conducted a time-series study on the correlations between six ambient air pollutants (SO2, NO2, PM2.5, PM10, CO, and O3) and emergency department visits (EDVs) for urolithiasis in Zhongnan Hospital of Wuhan University from January 1, 2016 to December 31, 2018. Season, gender and age differences were considered to examine the adverse health effects of air pollution on different groups. Furthermore, we also drew exposure-response relationship curves between EDVs for urolithiasis and air pollutants and explored the co-effects among six air pollutants.
2. Materials and methods
Wuhan, the capital of Hubei province, is located in central China (latitude 30°35'N and longitude 114°17'E) and consists of seven central districts and six suburban and rural districts (Figure 1). At the end of 2018, Wuhan had a land area of 8,494.41 square kilometers and a population of 11.1 million (http://www.wuhan.gov.cn). As the core city of the Yangtze River Economic Belt, Wuhan is an essential industrial base and transportation hub in China. Vehicle exhaust and industrial emissions are the primary sources of air pollution. Wuhan has a typical subtropical monsoon climate, with hot and humid summers and cold and dry winters. The average temperature is 4.4°C in January and 30.3°C in July.
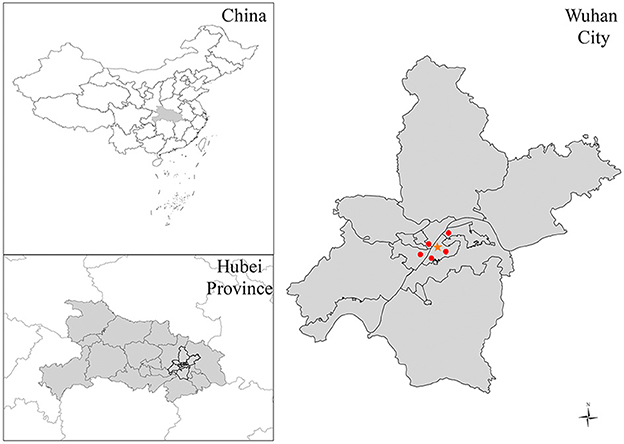
Figure 1. The location of Wuhan, the location of the hospital (the orange star), and monitoring stations (red dots).
2.1. Emergency department visits data
The majority of the EDVs of Zhongnan Hospital are from the Wuchang District of Wuhan, where the hospital is located. It is a district that contains 19% of the population of the whole urban area and 12% of the population of Wuhan overall (http://www.wuhan.gov.cn). The daily number of EDVs from January 1, 2016 to December 31, 2018 were obtained from Zhongnan Hospital of Wuhan University in Wuhan, China. The EDV data followed a quasi-Poisson distribution. Outpatient visits diagnosed with urolithiasis (mainly kidney stones) (N = 7,483) were selected as our study population. To ensure the accuracy of the study results, we removed duplicate records and non-Wuhan permanent residents and re-matched the tenth version of the International Classification of Diseases, with codes N20-N23. The study protocol was approved by the Medical Ethics Committee of Zhongnan Hospital (IRB number: 20211018K).
2.2. Air pollution and meteorological data
Daily ambient air pollution data (SO2, NO2, PM2.5, PM10, CO, and O3) from January 2016 to December 2018 was obtained from the Wuhan Ecological Environment Bureau website (http://hbj.wuhan.gov.cn/). The daily average concentrations of air pollutants were measured from the fixed-site monitoring stations. Average daily air pollutant concentrations at the monitoring stations were used as a proxy for the overall exposure of all populations. The maximum 8-h average of O3 and the daily 24-h average of the remaining pollutants were calculated.
The data of two meteorological parameters (daily average temperature [°C] and relative humidity [%]) during the study period were obtained from the Meteorological Data Sharing Service System of the China Meteorological Administration (Beijing, China). Environmental and meteorological data were missing for 32 days (2.92%). Therefore, dates with missing data were excluded from our study.
2.3. Statistical analysis
Generalized additive model (GAM) was used to conduct this time-series study. Because daily emergency visits followed an over-dispersed Poisson distribution, quasi-Poisson regression was used in the GAM. Several covariates were introduced to control for their potential confounding effects. First was a natural cubic smooth function for calendar time, with 7 degrees of freedom (df) per year to exclude long-term and seasonal trends over 2 months (14). Second, natural smooth functions of the two variables were incorporated into the model: mean temperature (6 df) and relative humidity (3 df), to control for the confounding effect of meteorological factors on the correlation between air pollution and urolithiasis EDVs (15). Third, indicators such as public holidays (Holiday) and “day of the week” (DOW) were adjusted as dummy variables in the GAM (15).
The model was as follows:
where E(Yt) is the estimated daily EDVs for kidney stones at day t; β indicates the log-relative rate of urolithiasis correlated with increased air pollutant units; Zt represents the pollutant concentrations at day t; DOW is a dummy variable for the day of the week; ns refers to the natural cubic regression smooth function. The exposure-response (E-R) relationship was drawn between air pollutants and urolithiasis EDVs by adding a 3 df natural spline function to the above model.
To test the stability of the model, three sensitivity analyses were performed. First, due to the uncertainty of the optimal lag days, we further introduced a single-day lag, including lag0, lag1, lag2, lag3, lag4, lag5, lag6, and lag7, and a multi-day moving average exposure, including lag0-1, 0-2, 0-3, 0-4, 0-5, 0-6, and 0-7. To determine the optimal lag structure, model fits were calculated based on three statistics: Akaike Information Criterion (AIC), Generalized Cross Validation (GCV), and Partial Autocorrelation Function (PACF). Second, for the smoothness of the temporal trend, we chose alternative df with 4–10 per year. Third, two-pollutant models were built to assess the stability of the effect estimates after adjusting for co-pollutants and added co-pollutants with a correlation coefficient < 0.7 to the two-pollutant model. In addition, three stratified analyses were conducted respectively according to season (warm: April to September; cool: October to March), age (< 45 years, 45–65 years, and ≥65 years), and gender (females, males). The statistical significance of the differences between the strata effect estimates were further tested by calculating 95% confidence intervals as , where Q1 and Q2 are the estimates for two categories, and SÊ1 and SÊ2 are their respective standard errors. All statistical analyses were performed in R software (version 4.1.0) using the MGCV package. Results were two-sided and p < 0.05 were statistically significant. The results were expressed as the percentage change in urolithiasis EDVs per 10 μg/m3 increase in each pollutant concentrations.
3. Result
Table 1 summarizes the descriptive statistics of EDVs for urolithiasis, air pollutants, and weather data. We collected a total of 7483 cases of urolithiasis admitted to the emergency system of Zhongnan Hospital of Wuhan University from January 1, 2016 to December 31, 2018. The annual mean temperature was 17.87°C and the annual mean humidity was 74.99%. During our study period, an average of seven people presented EDVs for urolithiasis daily. Among all patients, male, female, age < 45 years, age 45–65 years, and age ≥65 years were 72.9, 27.1, 58.6, 33.1, and 8.3% respectively. The EDVs for urolithiasis in warm seasons (57.7%) were higher than those in cool seasons (42.3%). The annual average concentrations of air pollutants were 50.79 μg/m3 for PM2.5, 83.55 μg/m3 for PM10, 47.87 μg/m3 for NO2, 1,005.63 μg/m3 for CO, 9.55 μg/m3 for SO2, and 87.40 μg/m3 for O3. During our study, there were 229 (20.9%) and 382 (24.8%) days respectively where NO2 and PM2.5 levels exceeded Chinese secondary ambient air quality standards. Other air pollutants were within the standard range most of the time. The monthly average variation of pollutants, temperature, relative humidity, and daily emergency department visits for urolithiasis are shown in Figure 2.
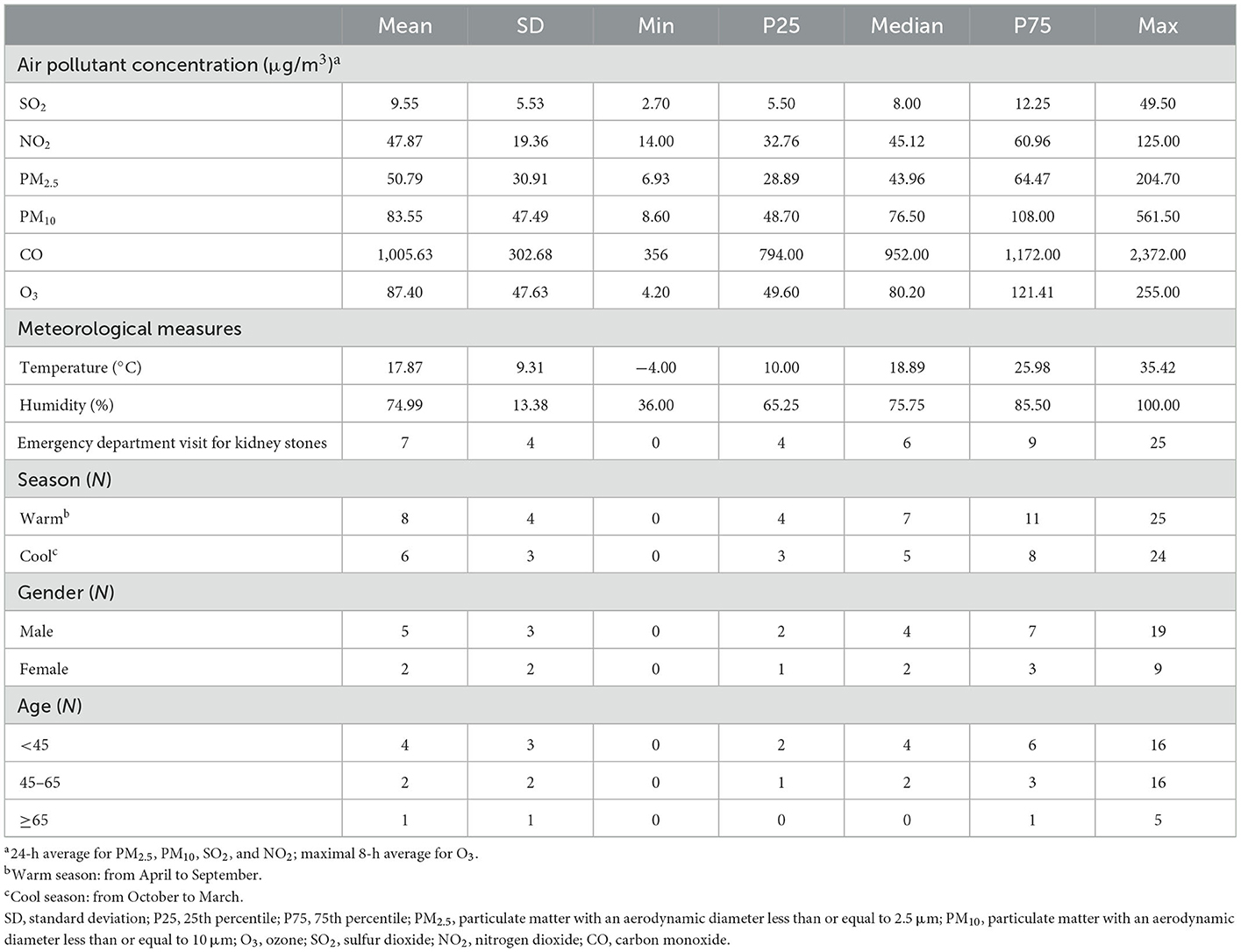
Table 1. The summary of daily air pollutants, weather conditions, and daily emergency department visit for urolithiasis (N = 7,483) during our study period (January 1, 2016–December 31, 2018).
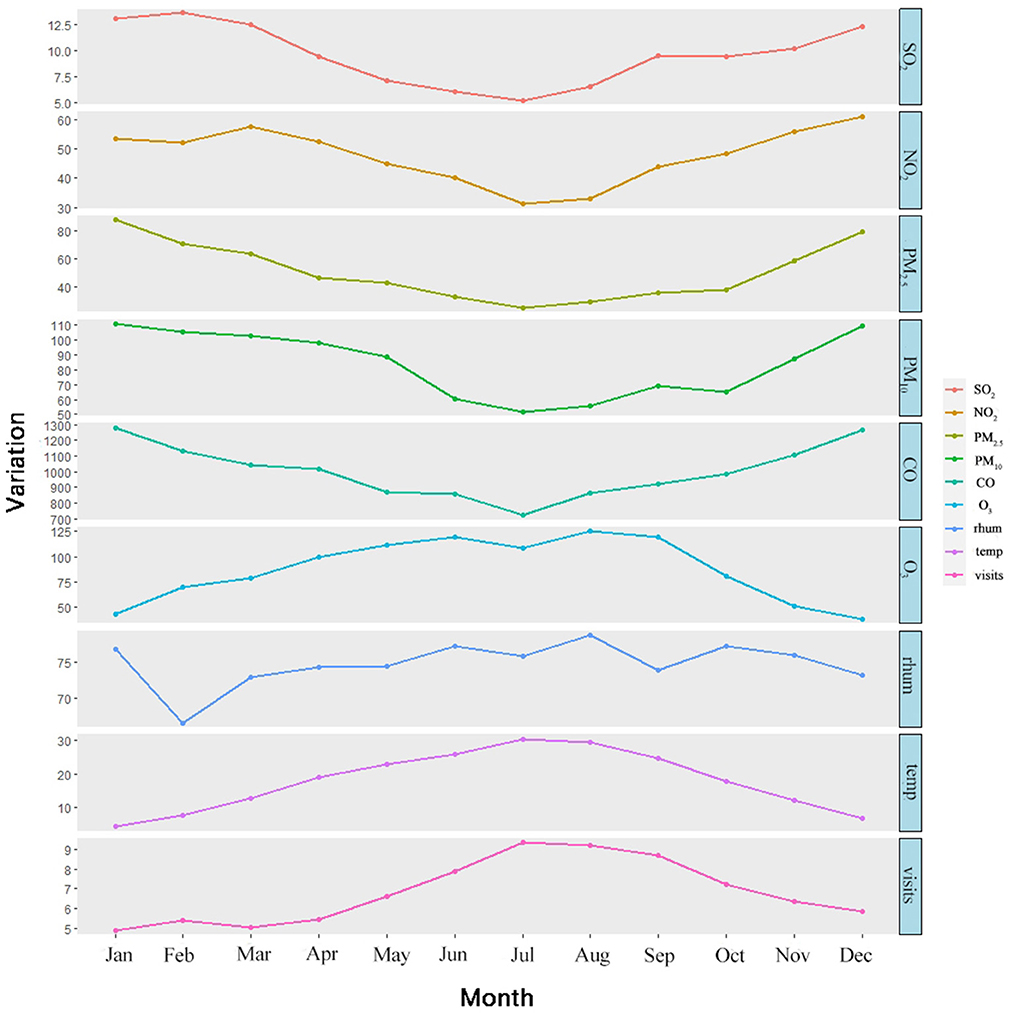
Figure 2. The monthly average variation of pollutants, temperature, relative humidity, and daily emergency department visits for urolithiasis.
Generally, there were moderate to strong correlation coefficients among PM2.5, PM10, SO2, NO2, and CO (Spearman's correlation coefficient ranged from 0.55 to 0.87), and they all were weakly correlated with O3 (−0.27 to 0.09). All pollutants were negatively correlated with temperature except for O3 (0.65) and with relative humidity except for CO (0.02) (Figure 3).
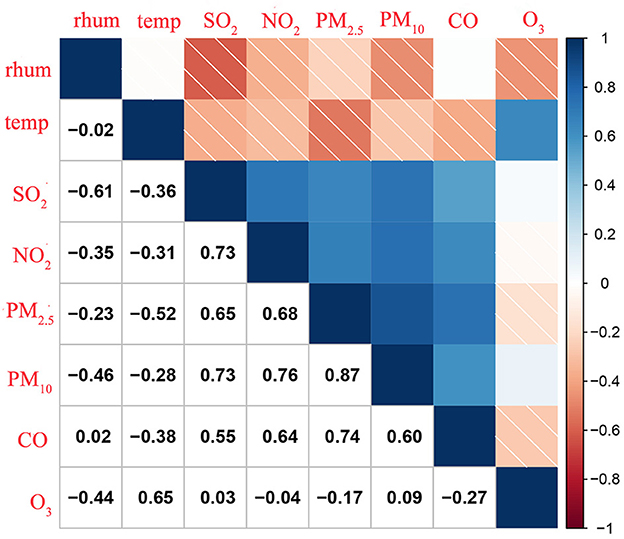
Figure 3. Spearman correlations among exposure variables in Wuhan, China (2016–2018). temp, Temperature; rhum, Relative Humidity.
Figure 4 exhibits the percentage change (mean and 95% CI) in urolithiasis EDVs associated with a 10 μg/m3 increase in the concentration of six pollutants when using different lag structures—single lag day (lag1-lag7) and moving average lag models (lag01-lag07). Notably, we found statistically significant correlations between emergency hospital admissions for urolithiasis and SO2, NO2, CO, and O3. According to the model fitting statistics, we selected lag06 for SO2, lag3 for NO2, lag6 for PM2.5 and CO, and lag2 for PM10 and O3 as the best lag structures because they can produce the smallest AIC/GCV/PACF values. A total of 10 μg/m3 increases of SO2, NO2, PM2.5, CO, PM10, and O3 respectively corresponded to increases in daily EDVs for urolithiasis of 15.02% (95% CI: 1.69%, 30.11%), 1.96% (95% CI: 0.19%, 3.76%), 1.09% (95% CI: −0.24%, 2.43%), 0.14% (95% CI: 0.02%, 0.26%), 0.72% (95% CI: 0.02%, 1.43%), and 1.17% (95% CI: 0.40%, 1.94%) (Table 2). The associations of all pollutants with EDVs for urolithiasis were generally positive, but in all lag structures we examined, PM2.5 and PM10 were statistically insignificant.
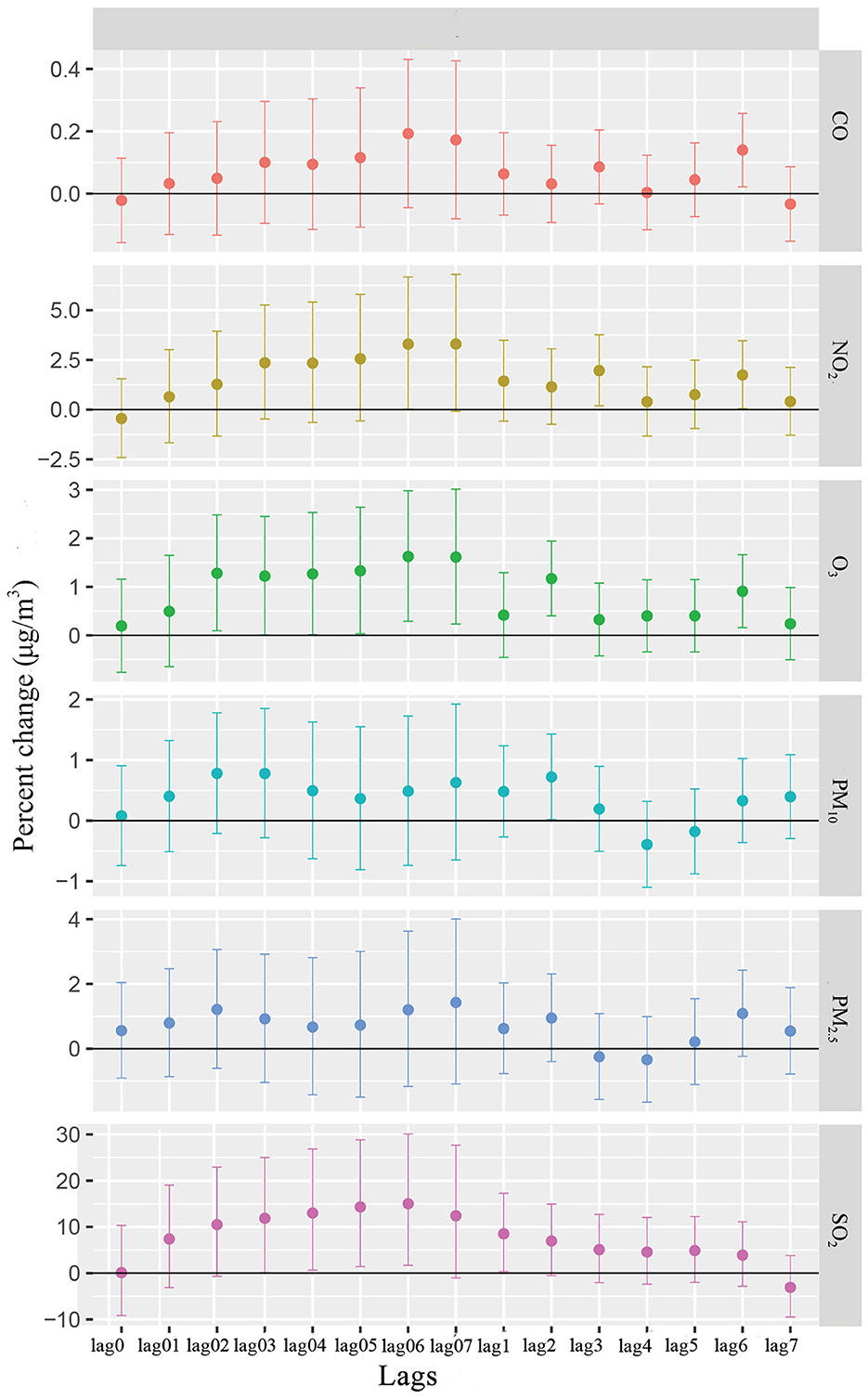
Figure 4. Percentage change (%) of EDVs for urolithiasis (mean and 95%CI) associated with a 10 μg/m3 increase in various air pollutant concentrations using different lag structures.
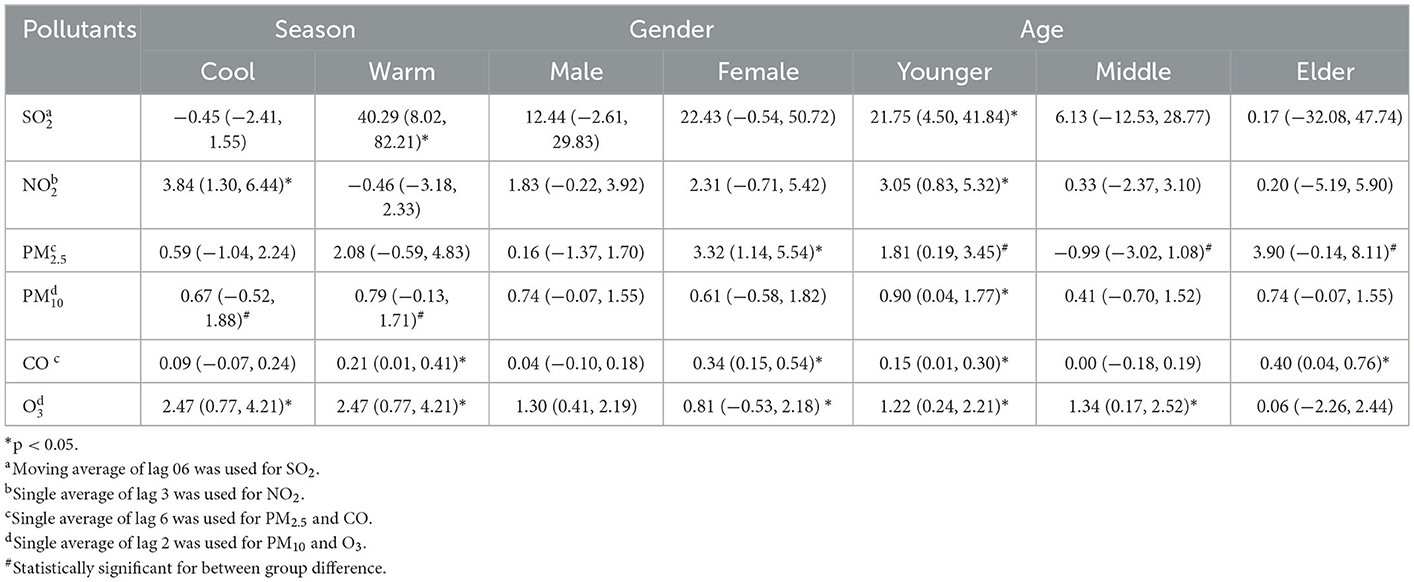
Table 2. Percent change (95% CI) in urolithiasis EDVs with a 10 μg/m3 increase in air pollutant concentrations by season, gender and age in Wuhan, China.
The exposure-response (E-R) curves of air pollutants and urolithiasis EDVs are shown in Figure 5. The E-R relationships of CO and O3 were nearly linear, indicating no thresholds for their associations with EDVs for urolithiasis. The curve for NO2 showed a steep slope at concentrations < 40 μg/m3 and became a J-shape at concentrations > 40μg/m3. For the curve of PM2.5, a steep slope was observed at concentrations < 50 μg/m3, a relatively flat slope at concentrations between 50 μg/m3 and 100 μg/m3, and became steep again at concentrations > 100 μg/m3. The E-R curves of PM10 and SO2 rose sharply when the concentrations were < 75 μg/m3 and < 10 μg/m3 and gradually flattened after the concentrations were higher than the critical values.
Table 2 displays how different effect estimates of ambient air pollution on urolithiasis EDVs varied by season, gender, and age. In season-specific analysis, the results suggested that the correlations between urolithiasis EDVs and SO2 and CO were generally stronger in warm seasons than in cool seasons, while correlations with NO2 were stronger in cool seasons. In gender-specific analysis, stronger correlations between PM2.5 and CO and urolithiasis EDVs were observed in females than in males. Among female patients, PM2.5, CO, and O3 were significantly correlated with urolithiasis EDVs, whereas the results were positive but not significant in male patients. In age-specific analysis, the effects of SO2, NO2, and PM10 tended to be greater for people aged < 45 years, while CO was more apparent in people aged ≥ 65 years, and the correlation of O3 was more pronounced in people aged 465 years.
The estimated effects were not significantly affected by adjusting for temporal smoothness using an alternative df from 4 to 10 per year (Supplementary Figure 1). Table 3 shows the correlations between urolithiasis EDVs and air pollution after adding co-pollutants with spearman's correlation coefficients < 0.7 to the two-pollutants model for adjustment. When adjusting for all other pollutants, we found that the risk estimates of CO and O3 on urolithiasis EDVs remained statistically significant. The correlations of PM10, SO2, and urolithiasis EDVs were statistically significant only when controlling for CO. For PM2.5, the estimated effects were insignificant regardless of which pollutant was adjusted.
4. Discussion
According to statistics, the prevalence of urolithiasis is gradually rising (16). Due to the acute nature of presentation, urolithiasis generates a large number of emergency department visits and hospital admissions (17). In recent years, numerous epidemiological studies have reported the positive correlations between air pollution and the development of urological diseases. However, few studies have explored the correlations between air pollutants and urolithiasis. Our research demonstrated a significant correlation between short-term exposure to air pollutants, including SO2, NO2, CO, and O3 and increased risks of urolithiasis. The correlations between CO, O3 and urolithiasis EDVs were robust with co-pollutants adjustment. The correlations were found mainly in females (especially from PM2.5 and CO) and in younger people (especially from SO2, NO2, and PM10). In contrast, the correlation between O3 and urolithiasis EDVs was more pronounced in males and middle-aged people, and the correlation of CO was more evident in elders. The effects of SO2 and CO were stronger in warm seasons than in cool seasons, while the effect of NO2 was more substantial in cool seasons. Our findings have added to the limited evidence that air pollution may be a potential risk factors for the incidence of urolithiasis.
During our study period, the annual average concentrations of NO2 (58.89 μg/m3) and PM2.5 (47.87 μg/m3), and PM10 (83.55 μg/m3) in Wuhan exceeded China's National Ambient Air Quality standards (40 μg/m3, 40 μg/m3 and 15 μg/m3). Since Wuhan is the largest city in central China, the expansion of cities, the development of industry, the increase of motor vehicles, and the growth of population may all be contributors to the severe air pollution.
Our study observed positive correlations between air pollutants (including SO2, NO2, PM2.5, PM10, CO, and O3) and the EDVs for urolithiasis, especially SO2, NO2, CO and O3, which is partially consistent with previous studies. Oxidative stress may be a primary mechanism of the positive correlations (18). Studies have shown that air pollutants, including O3, SO2, CO, NO2, and PM, are sources of several ROS and other byproducts of oxidative stress (19). In kidneys, they can cause damage to renal tubular epithelial cells and participate in the modification of crystalloid regulators (13, 20, 21), further affecting the formation of calcium oxalate crystals and urinary stones (22–25). Another potential mechanism is that air pollutants can affect urinary tract metabolites (26, 38), and specific urinary tract metabolites such as dimethyl-L-arginine and 2-oxo-arginine are thought to be associated with the formation of kidney stones (27). In addition, immune damage caused by gaseous pollutants may also play an important role (28). Macrophage-associated immune damage is a major change in the immune response observed in renal stone disease (2) and may promote the formation of Randall's plaque (calcified spots appearing in the renal papillae before the formation of kidney stones) and calcium oxalate stones (29). However, the exact biological mechanism remains unknown and warrants further study.
Our findings are partially consistent with those of previous studies. For example, a time series study conducted in Korea mentioned that PM2.5 and CO might be new potential risk factors for urolithiasis (18). A nationwide time-series study in China observed consistent associations between same-day PM2.5 and several genitourinary diseases, including nephritis, nephrosis, and renal sclerosis; chronic renal failure; and calculus of urinary tract (30). However, PM2.5 did not demonstrate a significant correlation with urolithiasis in our study, which may due to different study designs, study populations, confounding factors, such as outdoor activities and dietary habits on disease, and the characteristics of different urban air pollution mixtures (31).
Our study further demonstrated the association between short-term exposure to air pollutants and daily EDVs for urolithiasis varied according to season, age and gender. It is observed that the effects of SO2 and CO on urolithiasis EDVs were stronger in warmer seasons. This may be attributed to the physiological impact of temperature itself. Mechanisms of this correlation include insufficient fluid intake, increased sweating, and water evaporation, which lead to concentrated urine and further promote calcium, oxalic acid, uric acid, phosphate crystallization, and stone formation (32, 33). It is supposed the difference that NO2 showing higher effects in cool seasons might be related to varying concentrations, sources and composition of air pollution in different seasons (34).
In age stratification, our study indicates that SO2, NO2 and PM10 tended to have more significant effects in people aged < 45 years, which is contrary to the epidemiological evidence of a high incidence rate of urolithiasis in the elderly (35). This may be because young people usually have more outdoor activities, increasing their exposure to air pollutants. However, O3 and CO would significantly increase the risk of urolithiasis in middle-aged and older adults. In gender stratification, it is found that females are more vulnerable to PM2.5 and CO, while males are more susceptible to O3. We cannot find sufficient evidence in the literature for the phenomenon that different individual pollutants have a maximum impact on people of different ages and genders. Since individual pollutants are rarely produced in isolation, so sorting out the effects of individual pollutants is severely limited by potential confounding factors. Observational studies based on populations should consider correlations between them (36).
Interpreting trends in exposure-response relationships is critical to assessing public health. In the present research, threshold concentrations were not observed above which NO2 and PM2.5 were not correlated with urolithiasis EDVs. It is worth noting that the E-R curve for SO2 flattens out at high concentrations. This may result from a “harvest effect”, where sensitive populations might have already been affected and gone to the hospital before air pollutant concentrations reached significant levels (37). Multiple factors such as location, population sensitivity, air pollution mixtures, and climate characteristics may impact the E-R relationship (31). Therefore, more research is still needed in the future to explore the characteristics of the E-R relationship.
Our study has some noted limitations. First, average concentrations of air pollutants measured by stationary site monitoring were used to represent individual exposures, leading to misclassification of exposures and ignoring the spatial effect of air pollution on urolithiasis. Secondly, some factors were not taken into account that may affect the formation of urolithiasis and impair a person's tolerance to air pollutants, such as dietary habits, lifestyle, and metabolic syndrome. As a result, combined estimates may skew the effects of air pollutants in specific populations. Third, the data we collected was only from a highly polluted city; thus, the general study application of study may be limited. Therefore, further studies are needed to confirm our results, and molecular biology or animal experiments are necessary to explore the exact mechanisms between air pollutants and urinary stones formation.
5. Conclusion
Our time-series study found that ambient air pollution (especially SO2, NO2, CO, and O3) positively correlated with the EDVs for urolithiasis in Wuhan, China. These effects were found to vary by season, gender, and age. Our study adds limited evidence to how air pollutants affect urolithiasis and we hope this study can support hospitals and clinical staff in taking effective preventive measures to maintain emergency department order, particularly on heavily polluted days.
Data availability statement
The original contributions presented in the study are included in the article/Supplementary material, further inquiries can be directed to the corresponding author.
Ethics statement
The studies involving human participants were reviewed and approved by the Medical Ethics Committee of Zhongnan Hospital (IRB number: 20211018K). Written informed consent for participation was not required for this study in accordance with the national legislation and the institutional requirements.
Author contributions
HX: methodology, software, visualization, and writing—original draft. YL: investigation, writing—reviewing, and editing. JW: illustration production. XJ: supervision, project administration, and funding acquisition. All authors commented on previous versions of the manuscript and read and approved the final manuscript.
Funding
This work was supported by Zhongnan Hospital of Wuhan University Science, Technology and Innovation Seed Fund, Project znpy2018064.
Acknowledgments
The authors thank AiMi Academic Services (www.aimieditor.com) for the English language editing and review services. And we would like to appreciate all the participants who made this study possible.
Conflict of interest
The authors declare that the research was conducted in the absence of any commercial or financial relationships that could be construed as a potential conflict of interest.
Publisher's note
All claims expressed in this article are solely those of the authors and do not necessarily represent those of their affiliated organizations, or those of the publisher, the editors and the reviewers. Any product that may be evaluated in this article, or claim that may be made by its manufacturer, is not guaranteed or endorsed by the publisher.
Supplementary material
The Supplementary Material for this article can be found online at: https://www.frontiersin.org/articles/10.3389/fpubh.2023.1091672/full#supplementary-material
Supplementary Figure 1. Percentage change (%) of EDVs for urolithiasis (mean and 95%CI) associated with a 10 μg/m3 increase in various air pollutant concentrations at their peak lag day using different degrees of freedom per day.
Supplementary Table 1. Percent change (mean and 95% CI) in EDVs for urolithiasis associated with a 10 μg/m3 increase in concentrations of six pollutants using different lag structures.
References
1. Bartoletti R, Cai T, Mondaini N, Melone F, Travaglini F, Carini M, et al. Epidemiology and risk factors in urolithiasis. Urol Int. (2007) 79:3–7. doi: 10.1159/000104434
2. Wang Z, Zhang Y, Zhang J, Deng Q, Liang H. Recent advances on the mechanisms of kidney stone formation (Review). Int J Mol Med. (2021) 48:2. doi: 10.3892/ijmm.2021.4982
3. Zeng G, Mai Z, Xia S, Wang Z, Zhang K, Wang L, et al. Prevalence of kidney stones in China: an ultrasonography based cross-sectional study. BJU Int. (2017) 120:109–16. doi: 10.1111/bju.13828
4. Leveridge M, D'Arcy FT, O'Kane D, Ischia JJ, Webb DR, Bolton DM, et al. Renal colic: current protocols for emergency presentations. Eur J Emerg Med. (2016) 23:2–7. doi: 10.1097/MEJ.0000000000000324
5. Ping H, Lu N, Wang M, Lu J, Liu Y, Qiao L, et al. New-onset metabolic risk factors and the incidence of kidney stones: a prospective cohort study. BJU Int. (2019) 124:1028–33. doi: 10.1111/bju.14805
6. Zhuo D, Li M, Cheng L, Zhang J, Huang H, Yao Y, et al. Study of diet and lifestyle and the risk of urolithiasis in 1,519 patients in Southern China. Med Sci Monit. (2019) 25:4217–24. doi: 10.12659/MSM.916703
7. Zhang Y, Long G, Ding B, Sun G, Ouyang W, Liu M, et al. The impact of ambient temperature on the incidence of urolithiasis: a systematic review and meta-analysis. Scand J Work Environ Health. (2020) 46:117–26. doi: 10.5271/sjweh.3866
8. Cohen AJ, Brauer M, Burnett R, Anderson HR, Frostad J, Estep K, et al. Estimates and 25-year trends of the global burden of disease attributable to ambient air pollution: an analysis of data from the Global Burden of Diseases Study 2015. Lancet. (2017) 389:1907–18. doi: 10.1016/S0140-6736(17)30505-6
9. Manisalidis I, Stavropoulou E, Stavropoulos A, Bezirtzoglou E. Environmental and health impacts of air pollution: a review. Front Public Health. (2020) 8:14. doi: 10.3389/fpubh.2020.00014
10. Chan TC, Zhang Z, Lin BC, Lin C, Deng HB, Chuang YC, et al. Long-term exposure to ambient fine particulate matter and chronic kidney disease: a cohort study. Environ Health Perspect. (2018) 126:107002. doi: 10.1289/EHP3304
11. Kuzma Ł Małyszko J Bachórzewska-Gajewska H Kralisz P Dobrzycki S Exposure to air pollution and renal function. Sci Rep. (2021) 11:11419. doi: 10.1038/s41598-021-91000-0
12. Zecher M, Guichard C, Velásquez MJ, Figueroa G, Rodrigo R. Implications of oxidative stress in the pathophysiology of obstructive uropathy. Urol Res. (2009) 37:19–26. doi: 10.1007/s00240-008-0163-3
13. Khan SR. Reactive oxygen species as the molecular modulators of calcium oxalate kidney stone formation: evidence from clinical and experimental investigations. J Urol. (2013) 189:803–11. doi: 10.1016/j.juro.2012.05.078
14. Peng RD, Dominici F, Louis AT. Model choice in time series studies of air pollution and mortality. J Royal Statistical Soc. (2006) 169 179–203. doi: 10.1111/j.1467-985X.2006.00410.x
15. Yang C, Chen A, Chen R, Qi Y, Ye J, Li S, et al. Acute effect of ambient air pollution on heart failure in Guangzhou, China. Int J Cardiol. (2014) 177:436–41. doi: 10.1016/j.ijcard.2014.09.003
16. Sorokin I, Mamoulakis C, Miyazawa K, Rodgers A, Talati J, Lotan Y. Epidemiology of stone disease across the world. World J Urol. (2017) 35:1301–20. doi: 10.1007/s00345-017-2008-6
17. Corbo J, Wang J. Kidney and ureteral stones. Emerg Med Clin North Am. (2019) 37:637–48. doi: 10.1016/j.emc.2019.07.004
18. Noh TI, Hong J, Kang SH, Jung J. Association of meteorological factors and ambient air pollution on medical care utilization for urolithiasis: a population-based time-series study. BMC Nephrol. (2021) 22:402. doi: 10.1186/s12882-021-02614-5
19. Yang W, Omaye ST. Air pollutants, oxidative stress and human health. Mutat Res. (2009) 674:45–54. doi: 10.1016/j.mrgentox.2008.10.005
20. Nemmar A, Karaca T, Beegam S, Yuvaraju P, Yasin J, Hamadi NK, et al. Prolonged pulmonary exposure to diesel exhaust particles exacerbates renal oxidative stress, inflammation and DNA damage in mice with adenine-induced chronic renal failure. Cell Physiol Biochem. (2016) 38:1703–13. doi: 10.1159/000443109
21. Ratliff BB, Abdulmahdi W, Pawar R, Wolin SM. Oxidant mechanisms in renal injury and disease. Antioxid Redox Signal. (2016) 25:119–46. doi: 10.1089/ars.2016.6665
22. Khan SR. Role of renal epithelial cells in the initiation of calcium oxalate stones. Nephron Exp Nephrol. (2004) 98:e55–60. doi: 10.1159/000080257
23. Khan SR, Kok DJ. Modulators of urinary stone formation. Front Biosci. (2004) 9:1450–82. doi: 10.2741/1347
24. Hirose M, Yasui T, Okada A, Hamamoto S, Shimizu H, Itoh Y, et al. Renal tubular epithelial cell injury and oxidative stress induce calcium oxalate crystal formation in mouse kidney. Int J Urol. (2010) 17:83–92. doi: 10.1111/j.1442-2042.2009.02410.x
25. Yasui T, Okada A, Hamamoto S, Ando R, Taguchi K, Tozawa K, et al. Pathophysiology-based treatment of urolithiasis. Int J Urol. (2017) 24:32–8. doi: 10.1111/iju.13187
26. Chen C, Li H, Niu Y, Liu C, Lin Z, Cai J, et al. Impact of short-term exposure to fine particulate matter air pollution on urinary metabolome: a randomized, double-blind, crossover trial. Environ Int. (2019) 130:104878. doi: 10.1016/j.envint.2019.05.072
27. Agudelo J, Fedrigon D, Faris A, Wilkins L, Monga M, Miller WA. Delineating the role of the urinary metabolome in the lithogenesis of calcium-based kidney stones. Urology. (2022) 167:49–55. doi: 10.1016/j.urology.2022.06.004
28. Turcanu V, Dhouib M, Gendrault JL, Poindron P. Carbon monoxide induces murine thymocyte apoptosis by a free radical-mediated mechanism. Cell Biol Toxicol. (1998) 14:47–54. doi: 10.1023/A:1007416505088
29. Khan SR, Canales BK, Dominguez-Gutierrez RP. Randall's plaque and calcium oxalate stone formation: role for immunity and inflammation. Nat Rev Nephrol. (2021) 17:417–33. doi: 10.1038/s41581-020-00392-1
30. Gu J, Shi Y, Zhu Y, Chen N, Wang H, Zhang Z, et al. Ambient air pollution and cause-specific risk of hospital admission in China: a nationwide time-series study. PLoS Med. (2020) 17:e1003188. doi: 10.1371/journal.pmed.1003188
31. Chen C, Liu C, Chen R, Wang W, Li W, Kan H, et al. Ambient air pollution and daily hospital admissions for mental disorders in Shanghai, China. Sci Total Environ. (2018) 613–614:324–30. doi: 10.1016/j.scitotenv.2017.09.098
32. Lo SS, Johnston R, Al Sameraaii A, Metcalf PA, Rice ML, Masters GJ. Seasonal variation in the acute presentation of urinary calculi over 8 years in Auckland, New Zealand. BJU Int. (2010) 106:96–101. doi: 10.1111/j.1464-410X.2009.09012.x
33. Eisner BH, Sheth S, Herrick B, Pais VM, Sawyer M, Miller N, et al. The effects of ambient temperature, humidity and season of year on urine composition in patients with nephrolithiasis. BJU Int. (2012) 110:E1014–1017. doi: 10.1111/j.1464-410X.2012.11186.x
34. Song J, Lu M, Lu J, Chao L, An Z, Liu Y, et al. Acute effect of ambient air pollution on hospitalization in patients with hypertension: a time-series study in Shijiazhuang, China. Ecotoxicol Environ Saf. (2019) 170:286–92. doi: 10.1016/j.ecoenv.2018.11.125
35. Wang W, Fan J, Huang G, Li J, Zhu X, Tian Y, et al. Prevalence of kidney stones in mainland China: a systematic review. Sci Rep. (2017) 7:41630. doi: 10.1038/srep41630
36. Chen TM, Gokhale J, Shofer S, Kuschner GW. Outdoor air pollution: nitrogen dioxide, sulfur dioxide, and carbon monoxide health effects. Am J Med Sci. (2007) 333:249–56. doi: 10.1097/MAJ.0b013e31803b900f
37. Chen R, Yin P, Meng X, Liu C, Wang L, Xu X, et al. Fine particulate air pollution and daily mortality. A nationwide analysis in 272 Chinese cities. Am J Respir Crit Care Med. (2017) 196:73–81. doi: 10.1164/rccm.201609-1862OC
38. Li GX, Duan YY, Wang Y, Bian LJ, Xiong MR, Song WP, et al. Potential urinary biomarkers in young adults with short-term exposure to particulate matter and bioaerosols identified using an unbiased metabolomic approach. Environ Pollut. (2022) 305:119308. doi: 10.1016/j.envpol.2022.119308
39. Li S, Huang X, Liu J, Yue S, Hou X, Hu L, et al. Trends in the incidence and DALYs of Urolithiasis from 1990 to 2019: results from the global burden of disease study 2019. Front Public Health. (2022) 10:825541. doi: 10.3389/fpubh.2022.825541
40. Wang W, Wu C, Mu Z, Gu Y, Zheng Y, Ren L, et al. Effect of ambient air pollution exposure on renal dysfunction among hospitalized patients in Shanghai, China. Public Health. (2020) 181:196–201. doi: 10.1016/j.puhe.2020.01.001
Keywords: air pollution, urolithiasis, emergency department visits, time-series study, public health
Citation: Xu H, Liu Y, Wang J and Jin X (2023) Short-term effects of ambient air pollution on emergency department visits for urolithiasis: A time-series study in Wuhan, China. Front. Public Health 11:1091672. doi: 10.3389/fpubh.2023.1091672
Received: 07 November 2022; Accepted: 13 January 2023;
Published: 30 January 2023.
Edited by:
Ang Li, Chinese Academy of Medical Sciences and Peking Union Medical College, ChinaReviewed by:
Yibing Yang, Chinese Center for Disease Control and Prevention, ChinaMostafa Yuness Abdelfatah Mostafa, Minia University, Egypt
Copyright © 2023 Xu, Liu, Wang and Jin. This is an open-access article distributed under the terms of the Creative Commons Attribution License (CC BY). The use, distribution or reproduction in other forums is permitted, provided the original author(s) and the copyright owner(s) are credited and that the original publication in this journal is cited, in accordance with accepted academic practice. No use, distribution or reproduction is permitted which does not comply with these terms.
*Correspondence: Xiaoqing Jin, cmVkamluQHdodS5lZHUuY24=
†These authors have contributed equally to this work and share first authorship