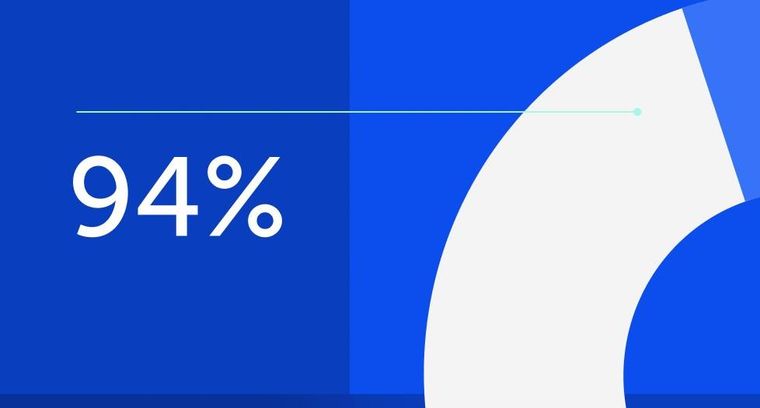
94% of researchers rate our articles as excellent or good
Learn more about the work of our research integrity team to safeguard the quality of each article we publish.
Find out more
ORIGINAL RESEARCH article
Front. Public Health, 10 January 2023
Sec. Infectious Diseases: Epidemiology and Prevention
Volume 10 - 2022 | https://doi.org/10.3389/fpubh.2022.998860
This article is part of the Research TopicAntimicrobial Resistant Pathogens in Sustainable Food Systems: How to Prevent, Detect or Control Contamination?View all 7 articles
The challenges posed by antibiotic-resistant pathogens have continued to increase worldwide, particularly in resource-limited countries. Human-livestock interactions are implicated in the complex AMR causal web. A cross-sectional study was conducted in four districts of Lusaka Province, Zambia to determine the antibiotic resistance patterns, ESBL production of E. coli isolated from stool samples of broiler poultry farm workers, and to assess poultry farmers' antibiotic resistance awareness. Sixty-six human stool samples were collected and processed for E. coli isolation, antibiotic resistance testing, and screened for ESBL production. In addition, 80 farmers were assessed for their level of awareness on antibiotic resistance. A total of 58 single E. coli isolates were obtained which showed high (87.9%) resistance to tetracycline, trimethoprim/sulfamethoxazole (48.3%), and ampicillin (46.8%); followed by nalidixic acid (19.0%), ciprofloxacin (12.1%), cefotaxime (8.6%) and chloramphenicol (5.2%). The prevalence of AMR E. coli was 67.2%, and 29.3% were MDR. Two (3.4%) isolates were identified to be ESBL producers, harboring the CTX-M gene. The study results also showed that broiler farmers were aware and knowledgeable of antibiotic resistance, although knowledge about its impact on human health was low. This study demonstrated the presence of resistant and ESBL producing E. coli among poultry farm workers.
Poultry and poultry products provide a significant and cheap source of protein. As a result, consumer demand has increased worldwide, and the total global production is projected to increase by 3% annually to offset this demand (1, 2). In Zambia, poultry and poultry products are important sources of food and income in most households (3). The poultry industry is the largest contributor to the country's livestock gross domestic product estimated at 48% (4) largely comprising of indigenous, layers and broiler chickens (5). Since 2000, the poultry industry has been growing steadily at a growth rate of between 8 and 10%, attributed to population growth which stands at 2.8% per year (6), increased disposable income, emerging middle class and rapid urbanization which drives the demand for poultry and poultry products due to very competitive prices comparable to other sources of animal proteins such as beef, lamb, pork and fish (5).
Poultry industry in Zambia encompasses both small and commercial scale poultry farmers, with former dominating the industry (4). The specific number of people rearing broiler chickens is unknown (4). Most poultry farmers are mostly settled along the line of rail stretching from Livingstone through Lusaka to Kitwe as well as Chililabombwe on the border with Katanga Region in the Democratic Republic of Congo (4). In 2018, the Ministry of Fisheries and Livestock estimated that there were 6.8 million broiler chickens, 1.7 million layers and 15.4 million village chickens, with Lusaka, Copperbelt, and Central provinces producing nearly 75% of the total broiler birds in Zambia, as of 2018 (5).
For small-scale farmers, poultry is usually kept in the backyard of the farmers' houses under intense conditions such as high population densities, promoting profitability (3). Knowledge about poultry production is usually acquired from friends already in the business, leading to several management dilemmas such as hygiene, feed quality, treatment, and control of infections (7).
Failure in poultry management and biosecurity contribute to the inappropriate use of a wide range of antimicrobials for prophylactic, therapeutic, and growth-promotion purposes over a prolonged period (8). This inappropriate use through overuse and misuse of antimicrobials promotes the selective pressure of antimicrobial-resistant (AMR) microorganisms and may promote the emergence and spread of AMR strains (8–10). Antibiotic use in the Zambian poultry industry is prevalent (5) and studies on broiler chickens have reported AMR strains of microorganisms (11–13). These resistant strains can be transmitted to humans through the environment, food products, and direct contact with food animals (1, 14, 15). Further, resistant strains of commensal microorganisms have a potential to pass resistance genes to human pathogens which in turn can cause health complications in human populations (16). Farm workers are constantly exposed to poultry and are at of risk acquiring AMR microorganisms.
In this study, Escherichia coli (E. coli) was of particular interest because it is a critical specie that can be used as an indicator microorganism for AMR in a particular host or environment, including multidrug resistance (MDR) - resistance to three or more antimicrobial classes (17).
Antimicrobial resistance trends in E. coli are of particular concern given their ubiquity in the environment and human and animal hosts, and the relative ease with which they acquire and transmit genetic determinants that confer resistance to most classes of antibiotics (18).
Transmission of resistant E. coli strains from poultry to humans is of great concern because the acquired resistance can occur in pathogenic bacteria and the endogenous bacterial flora of exposed individuals or populations (19, 20). AMR poses challenges in Diering and Mitchell (16) managing and treating infections in both food animals and humans (21, 22) due to the increase in number of infections, prolonged duration of infection, limited choice of empirical treatment antibiotics, and complications resulting from failed treatment; consequently result in increased cost to society.
E. coli can be resistant to several different classes of antibiotics. However, β-lactamase production is the most critical mediation of resistance to broad-spectrum β-lactams (23). β-lactamases are a broad class of enzymes that are often encoded on plasmids. They confer resistance to penicillins and cephalosporins and are an emerging cause of multidrug resistance in E. coli. Extended-spectrum β-lactamase (ESBL)-producing E. coli has been documented both in humans and poultry, and the prevalence has increased significantly over the past decade (24).
Resistance to newer drugs and the emergence of resistance to β-lactam antibiotics have been reported in E. coli and other Enterobacteriaceae from extraintestinal and environmental sources in Zambia (25, 26).
Regardless of this evidence of AMR in Zambia, no such study has been conducted on poultry farmworkers. In addition, knowledge on antibiotic use, and the impact of antibiotic resistance in broiler chickens on human health among broiler farmers is unknown. Improving knowledge about AMR among poultry farmers is particularly important in low-income countries, where diarrhoeal diseases are highest (27). A study by Tebug et al. found that the awareness of AMR and its adverse effects on human health in food animal production, particularly poultry production, ranged from moderate to low among animal health professionals in 20 sub-Saharan African countries (28).
There is a paucity of information on the role of poultry farmworkers in the epidemiology of resistant pathogens from poultry to humans. Therefore, this study aimed to determine the AMR patterns of E. coli isolated from stool samples of farmworkers and assess broiler farmers' awareness of antibiotic resistance and the knowledge about its impact on human health in selected districts in Lusaka Province, Zambia.
This cross-sectional study was conducted in four districts of Lusaka Province (Chilanga, Chongwe, Kafue, and Lusaka) between August 2017 and December 2018, districts in the province with high populations of poultry businesses. Lusaka Province was also selected because it is the home to the capital city and a trading hub for poultry and poultry products.
The sample size was determined using the formula for an unknown population.
The formula used to determine the target sample size was as follows:
Where: N = required minimum sample size; The Z-Standard value (1.96) corresponds to a confidence level at 95%; S = sample standard deviation at 0.5; E = accepted magnitude of error at 0.05.
The minimum target sample size was estimated at 384 participating broiler farmers, adjusted at 6% attrition for non-consent bias and inability to locate the broiler farmers to get 407.
There was no information on the number of farmers who reared broiler chickens. Most broiler farmers were mostly seasonal farmers. According to Phiri et al. a seasonal farmer was defined as the farmer who keeps broiler chicken when the production parameters including the cost of feed, and cost of medicines are favorable and stops when they are not (11). The scaling of the farmers was done according to the National Surveillance Protocol for AMR in broiler chickens currently being applied by Government as follows: small scale (< 500 birds per poultry house); medium scale (500–1,000 birds per poultry house); commercial (>1,000 birds per poultry house). Therefore, broiler poultry farmers were identified and located using the snowball sampling technique. Farmers in production were initially identified with the help of a local veterinary assistant or livestock officer. Such farmers would then lead to other farmers in the season of production.
Firstly, purposive sampling was employed, including only broiler farmers. As the population of broiler farmers was unknown and with the limitation of locating them, the maximum sample of 89 participants (farms located) was attained for this study from all the selected districts. Eighty farmers responded to the questionnaire: Chilanga (n = 21), Chongwe (n = 15), Kafue (n = 20), and Lusaka (n = 24) and 66 participants (farm workers) consented to provide stool samples Chilanga (n = 13), Chongwe (n = 13), Kafue (n = 13), and Lusaka (n = 27).
Farmers, from small farms without farmworkers, who performed the daily work of caring for the broiler chickens responded to the questionnaire and provided the stool sample. We sampled concerting farmers and farmworkers who had routine contacts with chickens.
At least one person from each farm was sampled among those who actively worked daily with broiler chickens in the poultry houses.
Participants were given a sterile stool container into which to place the early morning stool sample. The samples were collected and transported to the University of Zambia, Public Health Laboratory, and analyzed within 7 h of receiving them. In addition, a questionnaire was administered to the poultry farmer or his/her proxy to obtain poultry farm demographic data, poultry farm characteristics, and management, and to assess awareness and knowledge about antibiotic resistance.
Data was collected through face-to-face interviews using a semi-structured questionnaire to assess broiler farmers' awareness and basic knowledge about antibiotic resistance and its impact on human health. The questionnaire included the following sections: farm identification, demographic characteristics, general poultry farm characteristics, antibiotic use, and awareness and knowledge about antibiotic resistance and its impact on human health, as well as the source of information on antibiotic resistance.
Awareness was defined as having an understanding/hearing of antibiotic resistance and respondents were expected to say yes if they knew or had heard about antibiotics resistance. Knowledge about antibiotic resistance was defined as having the correct understanding of or facts on antibiotic resistance. The responses on what antibiotic resistance was, were used to determine basic knowledge about antibiotic resistance.
The questionnaire was pretested among 15 non-participating broiler poultry farmers in Chisamba District, Central Province, with similar characteristics. The findings after pretesting the questionnaire allowed for a modification to ensure the clarity and reliability of the questionnaire.
Upon receipt at the laboratory, samples were pre-enriched in Buffered Peptone Water (BPW) broth (Oxoid Ltd., Basingstoke, Hampshire, UK) by adding 1 g of sample to 9 ml of BPW using a sterile orange stick and incubating overnight at 37°C. A loopful of the sample was then cultured on MacConkey agar (Oxoid Ltd., Basingstoke, Hampshire, UK) using the streaking method and incubated overnight at 37°C. After incubation, small pink colonies were stained using Gram's staining technique and examined under a light microscope. Colonies showing Gram-negative short rods were then sub-cultured onto Eosin Methylene Blue (EMB) agar (Oxoid Ltd, Basingstoke, Hampshire, UK). Colonies showing a metallic green sheen were sub-cultured onto nutrient agar (Oxoid Ltd, Basingstoke, Hampshire, UK) and incubated overnight at 37°C before being subjected to identification tests.
Pure cultures of suspected E. coli isolates on nutrient agar were first subjected to biochemical testing for identification. Biochemical tests performed included Triple Sugar Iron (TSI), Urease, Citrate and Sulfide, Indole, Motility (SIM). Briefly, one to five single pure colonies of bacteria were picked using a sterile inoculating needle and stubbed into the medium and then streaked on the surface the of TSI agar slants. The tubes were then incubated at 37°C for 18–24 h with loosened caps and results recorded. Change in color of the butt and slant from red to yellow was noted, as well as presence or absence of gas and hydrogen sulfide. Materials from the same pure colonies of bacteria used for TSI test were later used for all the other biochemical tests. Sterile inoculating needle was used for each subsequent test. Bacteria was lightly stubbed into the center of the Urea agar slants. The Urea agar slant tubes were then incubated at 37°C for 18–24 h with loosened caps and results recorded. Change in color of the media from yellow to pink/red was noted as a positive result while no change in color of the media was noted as a negative result. Bacteria was lightly stubbed into the Citrate agar slant ~1 cm from the bottom of the tube and made sure not to twist the needle. The tubes were then incubated at 37°C for 18–24 h with loosened caps and results recorded. Change in color of the media from green to blue was noted as a positive result while no change in color of the media was noted as a negative result.
A single stab of bacteria was made into the tube of SIM agar ~1 cm from the bottom of the tube and made sure not to twist the needle. The tubes were then incubated at 37°C for 18–24 h with loosened caps and results recorded. Motility and presence or absence of hydrogen sulfide were noted. Three drops of Kovacs reagent were then applied to the surface of the media, and the development of a pink to red color was noted.
Biochemical identification was according to developed standard operating procedures (SOPs) (29, 30), technically supported by the reviewers from the WHO-Advisory Group on Integrated Surveillance of Antimicrobial Resistance (AGISAR).
To confirm the identity of the E. coli isolates after biochemical testing, the isolates were subjected to 16S rRNA sequencing according to the method described by Weisburg et al. (31). Briefly, a loopful of bacterial colonies from the nutrient agar plates were transferred into a sterile Eppendorf tube containing 100 μL of nuclease-free water. The cell suspension was mixed by vortexing and placed on a heating block at 80°C for 10 min to allow cells' thermolysis. The lysate was then centrifuged at 6,000 rpm for 2 min at 4°C, and the supernatant was collected into new sterile Eppendorf tubes. The supernatant samples were then used for PCR amplification and 16S rRNA sequencing to confirm the isolates.
The Kirby-Bauer disk diffusion test was performed on Müeller-Hinton agar (Oxoid Ltd, Basingstoke, Hampshire, UK) according to the Clinical and Laboratory Standards Institute (CLSI) guidelines (32). The drugs tested included amoxicillin-clavulanic acid (30 μg), ampicillin (10 μg), cefotaxime (30 μg), chloramphenicol (30 μg), ciprofloxacin (5 μg), colistin sulfate (10 μg), imipenem (10 μg), nalidixic acid (30 μg), trimethoprim/sulphamethoxazole (25 μg), and tetracycline (30 μg). Plates were prepared as follows: A well-isolated colony was selected from an overnight culture on Nutrient agar (Oxoid Ltd., Basingstoke, Hampshire, UK) with a sterile swab and suspended in 5 ml of sterile normal saline. The turbidity of the suspension was adjusted with sterile saline to obtain a suspension equivalent to 0.5 McFarland standard and evenly spread on the Müeller-Hinton agar (Oxoid Ltd., Basingstoke, Hampshire, UK). An automatic dispenser (Oxoid Ltd., Basingstoke, Hampshire, UK) was then employed to apply the antibiotic discs onto the plates and incubated at 37°C for 16–18 h. Zones of inhibition were measured with a digital vernier caliper, and endpoints were determined based on the areas showing no bacterial growth visible to the naked eye. Results were interpreted according to the CLSI guidelines (32). E. coli control strain ATCC 25922 was used as a control strain for the test. A multidrug-resistant (MDR) strain was defined as resistant to at least three different classes of antimicrobial agents.
The E. coli isolates were also screened for ESBL production using the cephalosporin/clavulanate combination disc method. The isolates were first tested against cefpodoxime and cefotaxime (Mast Diagnostics Ltd., Merseyside, U.K.) as an indicator of cephalosporins and ESBL production was confirmed phenotypically using a combination of discs (cefpodoxime/clavulanic acid cefotaxime/clavulanic acid) (Mast Diagnostics Ltd., Merseyside, U.K.) according to CLSI guidelines (32). The test was considered positive if the inhibition zone diameter was ≥5 mm larger with clavulanic acid than without.
The ESBL-positive isolates were further screened for the presence of common ESBL genes, CTX-M, TEM, and SHV, by PCR using the following primers CTX-M TCTTCCAGAATAAGGAATCCC (Forward), CCGTTTCCGCTATTACAAAC (Reverse), TEM TCCGCTCATGAGACAATAACC (Forward), TTGGTCTGACAGTTACCAATGC (Reverse), and SHV TGGTTATGCGTTATATTCGCC (Forward), GGTTAGCGTTGCCAGTGCT (Reverse).
The data collected through questionnaires was entered in Excel Spreadsheets and cleaned, then exported to SPSS version 22 for analysis. Descriptive statistics were used to analyze all the variables of interest, including age, gender, education level, marital status, awareness, and knowledge about antibiotic resistance, knowledge about the impact of antibiotic resistance on human health and sources of information on antibiotic resistance. Percentages were then generated. The level of confidence was set a 95% (α = 0.05), and Pearson Chi-Square was used to measure the association between categorical variables. Variables included both independent (age, gender, marital status, education, and source of information) and dependent variables (awareness and knowledge about antibiotic resistance).
The proportion of AMR isolates of E. coli was calculated by dividing the number of AMR E. coli over the total isolates. Antimicrobial susceptibility test results were entered into and analyzed in WHONET 2018. Percentage resistance and susceptibility of the E. coli isolates to different antibiotics were calculated. The proportion of ESBL-producing E. coli was determined, and the encoding genes were profiled.
Permission to conduct the study was sought from the Lusaka Provincial Health Office. Before specimen collection, an informed consent statement was obtained from all study participants. Participants were free to accept or decline to participate in the study. No incentive was provided for consenting to participate in the study. To maintain confidentiality, study participants were allocated study-specific codes, and the study data was kept confidential. Ethics clearance was sought from ERES Converge IRB (IRB No. 00005948) Ethics Committee (Study Reference: 2017-Aug-003).
In this study, 89 broiler farmers from four selected districts of Lusaka province were enrolled (Table 1). Out of this, total of 80 broiler farmers (90%) responded to the questionnaire on antibiotic resistance awareness levels and 66 stool samples were collected from farmworkers (74.2%) who were in constant contact with the birds (Table 1).
Most broiler farmers were small-scale (97.6%), of which 56.3% were females, and 41.3% were males, rearing < 500 birds per cycle in backyard poultry houses (72%). Only one female and one male farmer belonged to medium-scale and commercial-scale broiler production categories, respectively (Table 2). The majority of the respondents were in the 21–30 years age category (38.8%), with the 51–60 years age category being the lowest (3.8%) (Table 3). The results also showed that most broiler farmers were married (57.5%) and 36.3% were single. The majority (32.5%) had attained senior secondary school education (Table 3).
Most (98.8%) of broiler farmers reared broiler chickens for sale and home consumption. Majority of farmers (68.8%) used antibiotics to treat sick birds. Commonly used antibiotics were oxytetracycline, doxycycline, sulfamethoxazole, and gentamycin (Table 2). Thirty-five percent (35%) used antibiotics for the growth promotion of their birds. The results also showed that most farmers (52.5%) sold their chickens both live and dressed, with 47.5% of them selling only live chickens (Table 2).
The results showed no significant (p > 0.05) association between awareness of antibiotic resistance and socio-demographic characteristics (Table 3). However, results showed a considerable difference in age, gender, and marital status regarding awareness.
With regard to marital status, the results show that the widowed (100%) were the most aware of the antibiotic resistance, while the divorced were least. In terms of education levels, farmers who had reached tertiary education (62.5%) were the most aware of antibiotic resistance. In contrast, the least aware (16.7%) were those who only attained primary education. In terms of gender, women (59.1%) were relatively more aware of antibiotic resistance than their male counterparts (47%).
The results also showed that the majority (67%) of broiler farmers had a basic understanding of antibiotic resistance (Table 4). However, majority (74%) of the broiler farmers were unaware of the impact of antibiotic resistance on human health (Table 4). Some of the correct responses from the interviews on antibiotic resistance included “overuse of antibiotics that make them stop working thus cannot treat infections,” “bacteria don't respond to drugs administered,” and “disease does not go away after taking antibiotics.” The incorrect responses included “something about chlorine,” “drug reactions,” “cholera prevention,” and “can't explain.”
The primary sources of information on antibiotic resistance among the broiler farmers were the suppliers of broiler chicks (70.1%) and peers/friends (16.1%). Others were television (5.8%), hospital (4.6%), and social media (3.5%).
A total of 66 sample stools were collected and 58 E. coli were isolated. Thirty-nine (67.2%) E. coli isolates showed resistance to at least one antibiotic and 17 (29.3%) to at least three antibiotics (Table 1).
Five E. coli isolates were found to be ESBL-producers using the phenotypic testing approach, but only two of the isolates had a detectable ESBL gene (CTX-M). No TEM or SHV gene was detected in any of the isolates tested (Table 1).
E. coli isolates exhibited the highest resistance to tetracycline (61.7%, n = 36), sulfamethoxazole/trimethoprim (48.3%, n = 28), and ampicillin (46.8%, n = 27) (Table 5). One hundred percent (100%) susceptibility was observed to colistin and imipenem (Table 5).
This study aimed to assess poultry farmer awareness and basic understanding of antibiotic resistance and determine the AMR patterns of E. coli isolated from stool samples of poultry farm workers.
Lusaka district had the highest number of broiler farmers investigated. These results are consistent with the findings of Lungu which showed that most of the broiler production in Zambian occurred within Lusaka Province (4). Lusaka district is a densely populated area with a high broiler consumption rate. Therefore, many people engage in broiler poultry production as a source of income and to meet the demand for chicken meat for the city's growing population.
Majority of the broiler farmers were small-scale (97.6%). This high number of small-scale farmers could be attributed to the high cost and inadequate capital required to operate at commercial production (33).
This study also found that for most farmers in this study, the birds were reared in backyard poultry houses usually with high bird stocking density and poor ventilation. Backyard poultry production is often associated with low levels of biosecurity (32), which increases the risk of disease occurrence and consequent use of antibiotics (34). Moreover, backyard poultry production contributes to the emergence of AMR due to the increased need and extended use of antibiotics for growth promotion, disease prevention, and infection treatment (35, 36). Hedman et al. and Hoelzer et al. who reviewed antimicrobial drug use and antimicrobial resistance in poultry production found that in low-resource settings backyard poultry production contributes to the emergence of AMR due to the shortcomings in biosafety management that is commonly addressed with increased use of antimicrobial agents (35, 36).
Most broiler chickens are sold both live and dressed, reflecting consumers' preference when buying broiler chickens. Joshua Olorunwa's study also showed that most broiler farmers reared broiler chickens for meat and home consumption, with only a few rearing broilers for sale (37). Broiler chickens traded on the market were either processed at a recognized slaughter facility or at the home of the farmer, particularly done by back yard farmers. Chickens processed at home posed a relatively higher risk of carrying AMR pathogens than those processed in a slaughter facility because of increased likelihood of cross-contamination resulting from unhygienic processing conditions (11, 12, 34). The cross contamination and spread of AMR microorganisms to the environment and the public, in general, could also be due to the marketing of live birds at local open markets and roadside stalls, with limited sanitation, where humans and food animals interface (38–41). With these different activities during broiler poultry production, there could be a transmission of resistant microorganisms from poultry to the farmworkers and vice versa due to frequent interactions.
This study showed no association between awareness of antibiotic resistance and socio-demographic characteristics such as age, marital status, education, and gender which could be attributed to the small sample size. Perhaps other factors such as broiler farming experience may influence awareness of antibiotic resistance among the study participants.
Even though statistical results showed no association between the characteristics mentioned earlier and antibiotic resistance awareness, results showed a considerable difference in age, gender, and marital status regarding awareness. For instance, most farmers were aware of antibiotic resistance, particularly from the 41–50 age category. This is probably because most of these, had adequate levels of experience to understand these effects. Unsurprisingly the age group of 15–20 years was utterly unaware of antibiotic resistance. Most individuals in this category were beginning their broiler chicken business and did not have much experience. A study by Pham-Duc and Sriparamananthan found females to have better knowledge of antibiotics and AMR in comparison to males (42). This was attributed to females being more likely to seek help and visit healthcare facilities, thus receiving more information on antibiotics (42). Concerning marital status, the widowed were more aware, which could be attributed to age and adequate levels of experience and knowledge in poultry production. In addition, broiler production could be their main or only source of income, thus putting effort to acquire knowledge in production.
Knowledge about the impact of antibiotic resistance on human health was low. This lack of knowledge could be partly attributed to inadequate formal sensitization of antibiotic resistance's impact on human health. These results agree with Katakweba et al. (42, 43) in Tanzania, who also observed an extreme lack of awareness of health risks associated with AMR among food animal producers, posing a serious human health risk. Insufficient knowledge about the impact of AMR on human health is of great concern, as it can adversely affect human health, especially if special care is not taken into account to eradicate or minimize antibiotic resistance. Most broiler farmers obtained information from the chick sellers they regularly contacted. Other sources of information included hospitals, peers, social media, and television. There is a need to identify effective channels of communication to increase the dissemination of AMR information and its possible risks to human health.
The prevalence of AMR and MDR E. coli was found to be 67.2 and 29.3%, respectively. This prevalence was higher compared to 39.7% observed by Aworh et al. (44) who carried out a similar study in Abuja, Nigeria (44). Resistance to tetracycline (61.7%), sulfamethoxazole/trimethoprim (48.3%), and ampicillin (46.8) was highest. Aworh et al. also obtained similar results of resistance; 83.3, 79.2, and 77.1% to tetracycline, sulphamethoxazole/trimethoprim, and ampicillin, respectively. A recent study on AMR in young children in Lusaka and Ndola districts of Zambia showed that E. coli had highest resistance against ampicillin (78.0%), trimethoprim-sulfamethoxazole (70.4%), and tetracycline (62.8%) (45). A review by Tadesse et al. on AMR in humans found that a high level of drug resistance existed to commonly prescribed antibiotics in the African continent (46). E. coli was commonly isolated from patients with bloodstream infections (17/87, 19.5%), urinary tract infections (17/87, 19.5%), and wound infections (16/87, 18.4%) (46). Resistance to amoxicillin, ampicillin and trimethoprim/sulfamethoxazole was high. Median resistance of Escherichia coli to amoxicillin, ampicillin trimethoprim and gentamicin was 88.1, 86.7, and 80.7%, respectively (46). Resistance to either ceftriaxone or cefotaxime, which is suggestive of extended–spectrum beta lactamase (ESBL) production was reported in 20.0% of E. coli (46).
This study also found tetracycline and sulfamethoxazole/trimethoprim, to be among the most commonly used antibiotics for treatment, among the poultry farmers interviewed, during the production process. However, ampicillin was not among the commonly used antibiotics during the production process. A study from Tanzania further, indicated that oxytetracycline and sulphadimidines were the most used antibiotics and that few broiler farmers used antibiotics for growth promotion (43). Supplements given to poultry during production contain antibiotics such as oxytetracycline, doxycycline, and sulfamethoxazole, to which the birds were constantly exposed; while other antibiotics like amoxicillin, oxytetracycline, and ceftriaxone were excessively used for the treatment of infections during the production process (44). Over-the-counter-sale of some antibiotics without prescriptions (47) and inadequate AMR surveillance in food animals could exacerbate the problem of AMR.
Studies conducted in Zambia, including Lusaka Province on AMR in broiler chickens, showed E. coli resistance to tetracycline, ampicillin, and sulfamethoxazole/trimethoprim (11, 12). Phiri et al. observed the prevalence of AMR to be 94.6% and resistance to tetracycline (81.4%), sulfamethoxazole/trimethoprim (65.4%), and ampicillin (72.9%) among E. coli isolates from broilers at abattoirs and open markets (11). Similarly, Muligisa-Muonga et al. found prevalence of AMR to be 88% and resistance to tetracycline (79.4%), sulfamethoxazole/trimethoprim (49.7%), and ampicillin (51.9%) of E. coli isolates from retail broiler carcasses (12).
Generally, an increase in the prevalence of ESBL-producing E. coli in Africa and around the world has been observed (48, 49).
In terms of genetic determinants of AMR, TEM and SHV genes have been frequently reported. However, recently, CTX-M has been the most implicated in both humans and broilers (40, 41).
Of the targeted ESBL-producing resistance genes for this study, CTX-M was the only gene detected, similar to what was found by Dahms et al. (50). The other two targeted genes, TEM and SHV, were not detected despite the phenotypic data showing ESBL production. This disparity could be due to differences in the sequences of the target genes. The detection of CTX-M could be attributed to the fact that CTX-M producing ESBLs have become more prevalent in the recent past (51, 52) as reported in the review done by Kawamura et al. (53).
The detection of ESBL-producing strains of E. coli is of great concern to the human, food animal populations and their environments, as these microorganisms can easily be transferred or acquired through excessive bacterial growth and cross-contamination as a result of poor food handling, consumption of contaminated food or poor sanitation. A study by Hedman et al. revealed high CTX resistance (66.1%) in farmed broiler chickens, an increase in CTX resistance over time in backyard chickens not fed antibiotics (2.3–17.9%), and identical blaCTX−M sequences from human and chicken bacteria, suggesting a spillover event (35). Another study conducted in the Netherlands showed a genetic similarity among the ESBL producing E. coli strains found in retail chicken meat and those in healthy but infected humans (54). AMR in humans is interlinked with AMR in other populations, especially farm animals and in the wider environment (38).
AMR leads to early empirical treatment failure, limitations in the choice of antibiotics, and possible complications resulting from the failed treatment (27, 28). Zambia has experienced the negative effects of resistant pathogenic microorganisms as evident in a study by Songe et al. (25) who demonstrated resistance to some foodborne pathogens (non-typhoidal Salmonellae and Shigella flexneri and S. dysenteries) in patients with HIV-related persistent diarrhea. In addition, a review by Mshana et al. (55) showed an increasing trend in the incidence of antibiotic resistance in Zambia, the Democratic Republic of the Congo, Mozambique, and Tanzania. Of significant concern has been the increase in multidrug-resistant Escherichia coli, Klebsiella pneumoniae, Staphylococcus aureus, Vibrio cholerae, non-typhoid Salmonella, and other pathogens responsible for nosocomial infections.
Zambia has a challenge of high poverty levels (>60%) (56). Poverty and malnutrition are endemic in rural areas (56). One of the major causes is the country's high disease burden (57) due to food safety issues among others. The sick and caregivers do not contribute effectively to the country's economy, and this worsens poverty. It is, therefore, a cause for concern wherever antimicrobials are in use.
Most of the broiler farmers were aware of antibiotic resistance though they were not aware of the impact of antibiotic resistance on human health. Through the “One Health” approach, the government should increase awareness on AMR in humans, food animal production, the environment and its possible public health threat. Awareness among food animal farmers and local consumers is important considering their strategic position in the food chain.
The prevalence of AMR E. coli was relatively high, with the highest resistance to tetracycline, sulfamethoxazole/trimethoprim, and ampicillin which could be attributed to the use of antibiotics in humans for treatment of infections, food animal production and the spread of AMR microorganisms. More research is needed to identify the subgroups of E. coli and further molecular research to determine the genes that confer this resistance against antibiotics. In addition, more studies should also be conducted to identify the sources and administration of antibiotics particularly among small scale broiler farmers.
There is a need to regulate the use of and access to antibiotics through strict legislation for pharmacies, pharmaceutical companies as well as agro-vet shops. Stewardship programs are essential and need to be developed and supported to ensure that human and veterinary antibiotics are used properly.
The government should develop and maintain registers of food animal farmers such as poultry farmers. There is also a need for surveillance of AMR; in human, poultry and other livestock and the environment, for assessing the spread of AMR and inform policies.
The study was limited in scope and studies to look at poultry meat, live birds, and the environment are needed along with human data.
Statistically significant findings that are really present in a study population are hard to detect with small sample size. The small number of samples against the computed number was that we could only manage to locate a small number of farmers that were in production. The lack of a database contributed to the challenge of locating the broiler farmers to include in our study and hence failed to meet the target sample size. Additionally, some broiler farmers reared their chickens in spare rooms within their main houses, making it difficult to be identified in the neighborhood. Further, the use of a semi-structured questionnaire had potential limitations such as recall bias and interpretation issues; however, it still gave some insight into the AMR problem among the broiler poultry farmers in Lusaka Province.
The data presented in the study were deposited in National Center for Biotechnology Information (NCBI) accession numbers are as follows: SUB12488218 ks13 OQ121818, SUB12488218 LS28 OQ121819, SUB12488218 LS281 OQ121820, SUB12488218 LS171 OQ121821, SUB12488218 CS02 OQ121822, SUB12488218 CS13 OQ121823.
The studies involving human participants were reviewed and approved by ERES Converge Ethics Committee (IBR No. 00005948). Affiliation is non-governmental. The patients/participants provided their written informed consent to participate in this study.
JM, GM, MMu, MMw, and GK conceived and designed the study idea. MMu, MMw, EM, and IS were involved in data collection. MMw, GK, NP, EM-M, and KY analyzed the laboratory samples. MMu, MMw, EM, GK, and JM were involved in the interpretation of the results. MMu and JM drafted the manuscript. GM, GK, and FB reviewed and edited the manuscript. JM coordinated the study. All authors contributed to the article and approved the submitted version.
This work was supported by AGISAR grant number/Reference ID: 204954.
We would like to thank the WHO-funded Advisory Group for Integrated Surveillance of Antimicrobial Resistance (AGISAR) Project at the University of Zambia for the provision of funds and research materials required for laboratory sample analysis and the survey. The authors wish to thank further Dr. Charles Mubita, Mr. Joseph Ndebe, Mr. Patrick Katemangwe, Mr. Penjani Kapila, and all the laboratory staff at The University of Zambia, Public Health Laboratory for the professional assistance rendered during the laboratory work.
The authors declare that the research was conducted in the absence of any commercial or financial relationships that could be construed as a potential conflict of interest.
All claims expressed in this article are solely those of the authors and do not necessarily represent those of their affiliated organizations, or those of the publisher, the editors and the reviewers. Any product that may be evaluated in this article, or claim that may be made by its manufacturer, is not guaranteed or endorsed by the publisher.
1. Van Boeckel TP, Brower C, Gilbert M, Grenfell BT, Levin SA. Global trends in antimicrobial use in food animals. Proc Natl Acad Sci USA. (2015) 112:5649–54. doi: 10.1073/pnas.1503141112
2. Agunos A, Pierson FW, Lungu B, Dunn PA, Tablante N. Review of nonfoodborne zoonotic and potentially zoonotic poultry diseases. Avian Dis. (2016) 60:553–75. doi: 10.1637/11413-032416-Review.1
3. Andersson C. Broiler Production in Zambia - Management, Growth, Diseases and Welfare. Zambia: The Embassy of The Kingdom of The Netherlands in Zimbabwe in Conjunction with AgriProFocus Zambia and Poultry Association of Zambia (2014).
4. Bronkhorst B, Chongo RM. Market Study – Poultry Investment Opportunities in the Zambian Poultry Sector (and in the Katanga Region of the DR Congo). Zambia:The Embassy of The Kingdom of The Netherlands in Zimbabwe in Conjunction with AgriProFocus Zambia and Poultry Association of Zambia (2015). p. 1–46.
5. Embassy of the Kingdom of the Netherlands in Harare in conjunction with AgriProFocus Zambia and the Poultry Association of Zambia. January 2020. Curr Hist. (2020) 119:120. doi: 10.1525/curh.2020.119.815.120
6. Zambia Statistical Agency. 2010 Census of Population Summary Report. Repub Zambia Cent Stat Off (2012). Available online at: https://www.zamstats.gov.zm/index.php/publications/category/14-2010 (accessed November 2, 2022).
7. Caudell MA, Dorado-Garcia A, Eckford S, Creese C, Byarugaba DK, Afakye K, et al. Towards a bottom-up understanding of antimicrobial use and resistance on the farm: a knowledge, attitudes, and practices survey across livestock systems in five African countries. PLoS ONE. (2020) 15:1–26. doi: 10.1371/journal.pone.0220274
8. Ramirez AA. Use of antibiotics in broiler production: global impacts and alternatives. Anim Nutr. (2018) 4:170–8. doi: 10.1016/j.aninu.2018.03.002
9. Bok E, Mazurek J, Stosik M, Wojciech M. Prevalence of virulence determinants and antimicrobial resistance among commensal Escherichia coli derived from dairy and beef cattle. Int J Environ Res Public Health. (2015) 12:970–85. doi: 10.3390/ijerph120100970
10. Coli E. A survey of antimicrobial agents usage in poultry farms and an -tibiotic resistance in Escherichia coli and staphylococci isolates from the poultry in ile-ife. Nigeria. (2018) 4:4–11. doi: 10.23937/2474-3658/1510047
11. Phiri N, Mainda G, Mukuma M, Sinyangwe NN, Banda LJ, Kwenda G G, et al. Antibiotic-resistant Salmonella species and Escherichia coli in broiler chickens from farms, abattoirs, and open markets in selected districts of Zambia. J Epidemiol Res. (2020) 6:13–21. doi: 10.5430/jer.v6n1p13
12. Muligisa-Muonga E, Mainda G, Mukuma M, Kwenda G, Hang'ombe B, Flavien BN. Antimicrobial resistance of Escherichiacoli and Salmonella isolated from retail broiler chicken carcasses in Zambia. J Epidemiol Res. (2021) 6:35. doi: 10.5430/jer.v6n1p35
13. Mtonga S, Nyirenda SS, Mulemba SS, Ziba MW, Muuka GM, Fandamu P. Epidemiology and antimicrobial resistance of pathogenic E. coli in chickens from selected poultry farms in Zambia. J Zoonotic Dis. (2020) 2021:18–28.
14. Klous G, Huss A, Heederik DJJ, Coutinho RA. Human-livestock contacts and their relationship to transmission of zoonotic pathogens, a systematic review of literature. One Heal. (2016) 2:65–76. doi: 10.1016/j.onehlt.2016.03.001
15. Spellberg B, Hansen GR, Kar A, Cordova CD, Price LB, Johnson JR. Antibiotic resistance in humans and animals. NAM Perspect. (2016) 6:3903–10. doi: 10.31478/201606d
16. Diering M, Mitchell F. HHS public access. Physiol Behav. (2018) 176:139–48. doi: 10.1007/s00248-017-0985-z
17. WHO Antibiotic Resistance. Available online at: https://www.who.int/news-room/fact-sheets/detail/antibiotic-resistance (accessed July 31, 2020).
18. Leski TA, Taitt CR, Bangura U, Stockelman MG, Ansumana R, Cooper WH, et al. High prevalence of multidrug resistant Enterobacteriaceae isolated from outpatient urine samples but not the hospital environment in Bo, Sierra Leone. BMC Infect Dis. (2016) 1–9. doi: 10.1186/s12879-016-1495-1
19. Poolman JT. Escherichia coli. Int Encycl Public Heal. (2017) 585–93. doi: 10.1016/B978-0-12-803678-5.00504-X
20. Gbaguidi-Haore H, Dumartin C, L'Hériteau F, Péfau M, Hocquet D, Rogues A-M, et al. Antibiotics involved in the occurrence of antibiotic-resistant bacteria: a nationwide multilevel study suggests differences within antibiotic classes. J Antimicrob Chemother. (2013) 68:461–70. doi: 10.1093/jac/dks406
21. Dadgostar P. Antimicrobial resistance: implications and costs. Infect Drug Resist. (2019) 12:3903–10. doi: 10.2147/IDR.S234610
22. Khanal S, Khadka U, Dhungel L. Antimicrobial resistance: scoping review article. Int J Med Biomed Sci. (2017) 2:1–4. doi: 10.55530/ijmbiosnepal.v2i4.31
23. Allocati N, Masulli M, Alexeyev MF, Di Ilio C. Escherichia coli in Europe: an overview. Int J Environ Res Public Health. (2013) 10:6235–54. doi: 10.3390/ijerph10126235
24. Olsen RH, Bisgaard M, Löhren U, Robineau B, Christensen H. Extended-spectrum β-lactamase-producing Escherichia coli isolated from poultry: a review of current problems, illustrated with some laboratory findings. Avian Pathol. (2014) 43:199–208. doi: 10.1080/03079457.2014.907866
25. Songe MM, Hang BM, Knight-jones TJD, Grace D. Antimicrobial resistant enteropathogenic Escherichia coli and Salmonella spp. in houseflies infesting fish in food markets in Zambia. Int J Environ Res Public Health. (2016) 14:21. doi: 10.3390/ijerph14010021
26. Mumbula EM, Kwenda G, Samutela MT, Kalonda A, Mwansa JCL, Mwenya D, et al. Extended spectrum β-lactamases producing klebsiella pneumoniae from the neonatal intensive care unit at the university teaching hospital in Lusaka, Zambia. J Med Sci Technol. (2015) 4:85–91.
27. Randrianirina F, Ratsima EH, Ramparany L, Randremanana R, Rakotonirina HC, Andriamanantena T, et al. Antimicrobial resistance of bacterial enteropathogens isolated from stools in Madagascar. BMC Infect Dis. (2014) 14:1–7. doi: 10.1186/1471-2334-14-104
28. Tebug SF, Mouiche MMM, Abia WA, Teno G, Tiambo CK, Moffo F, et al. Antimicrobial use and practices by animal health professionals in 20 sub-Saharan African countries. Prev Vet Med. (2021) 186:105212. doi: 10.1016/j.prevetmed.2020.105212
29. Jackson GJ, Merker RI, Bandler R, Andrews WH, Hammack TS, Bryce JR. Bacteriological Analytical Manual. (2001).
30. Vandepitte J, Verhaegen, Engbaek K, Rohner P, Piot P, Heuck CC. Laboratory procedures. Nutrients. (2003) 188.
31. Weisburg WG, Barns SM, Pelletier DA, Lane DJ. 16S ribosomal DNA amplification for phylogenetic study. J Bacteriol. (1991) 173:697–703. doi: 10.1128/jb.173.2.697-703.1991
32. Standards P, Testing AS. M100 Performance Standards for Antimicrobial. Clinical Laboratory Standards Institute (2018).
33. Mbuza F, Manishimwe R, Mahoro J, Simbankabo T, Nishimwe K. Characterization of broiler poultry production system in Rwanda. Trop Anim Health Prod. (2017) 49:71–7. doi: 10.1007/s11250-016-1160-0
34. Conan A, Ponsich A, Goutard FL, Khiev R, Tarantola A, Sorn S, et al. A community-based education trial to improve backyard poultry biosecurity in rural Cambodia. Acta Trop. (2013) 125:294–302. doi: 10.1016/j.actatropica.2012.12.006
35. Hedman HD, Vasco KA, Zhang L. A review of antimicrobial resistance in poultry farming within low-resource settings. Animals. (2020) 10:1–39. doi: 10.3390/ani10081264
36. Hoelzer K, Wong N, Thomas J, Talkington K, Jungman E, Coukell A. Antimicrobial drug use in food-producing animals and associated human health risks: what, and how strong, is the evidence? BMC Vet Res. (2017) 13:211. doi: 10.1186/s12917-017-1131-3
37. Joshua Olorunwa O. Economic analysis of broiler production in Lagos State Poultry Estate, Nigeria. J Invest Manag. (2018) 7:35. doi: 10.11648/j.jim.20180701.15
38. Woolhouse M, Ward M, Van Bunnik B, Farrar J. Antimicrobial resistance in humans, livestock and the wider environment. Philos Trans R Soc B Biol Sci. (2015) 370:83. doi: 10.1098/rstb.2014.0083
40. Falgenhauer L, Imirzalioglu C, Oppong K, Akenten CW, Hogan B, Krumkamp R, et al. Detection and characterization of ESBL-producing Escherichia coli from humans and poultry in Ghana. Front Microbiol. (2019) 10:1–8. doi: 10.3389/fmicb.2018.03358
41. Castanheira M, Simner PJ, Bradford PA. Extended-spectrum β -lactamases: an update on their characteristics, epidemiology and detection. JAC Antimicrobial Resist. (2021) 3. doi: 10.1093/jacamr/dlab092
42. Pham-Duc P, Sriparamananthan K. Exploring gender differences in knowledge and practices related to antibiotic use in Southeast Asia: a scoping review. PLoS ONE. (2021) 16:1–18. doi: 10.1371/journal.pone.0259069
43. Sonola VS, Katakweba AS, Misinzo G, Matee MIN. Occurrence of multi-drug-resistant Escherichia coli in. Antibiotics. (2021) 10:1–12. doi: 10.3390/antibiotics10091137
44. Aworh MK, Kwaga J, Okolocha E, Mba N, Thakur S. Prevalence and risk factors for multi-drug resistant Escherichia coli among poultry workers in the Federal Capital Territory, Abuja, Nigeria. PLoS ONE. (2019) 14:1–15. doi: 10.1371/journal.pone.0225379
45. Bumbangi FN, Llarena A-K, Skjerve E, Hang'ombe BM, Mpundu P, Mudenda S, et al. Evidence of community-wide spread of multi-drug resistant Escherichia coli in young children in Lusaka and Ndola Districts, Zambia. Microorganisms. (2022) 10. doi: 10.3390/microorganisms10081684
46. Tadesse BT, Ashley EA, Ongarello S, Havumaki J, Wijegoonewardena M, González IJ. Antimicrobial resistance in Africa: a systematic review. BMC Infect Dis. (2017) 17:1–17. doi: 10.1186/s12879-017-2713-1
47. Kalungia AC, Burger J, Godman B, Costa J de O, Simuwelu C. Non-prescription sale and dispensing of antibiotics in community pharmacies in Zambia. Expert Rev Anti Infect Ther. (2016) 14:1215–23. doi: 10.1080/14787210.2016.1227702
48. Muthupandian S, Balajee R, Barabadi H. The prevalence and drug resistance pattern of extended spectrum β-lactamases (ESBLs) producing Enterobacteriaceae in Africa. Microb Pathog. (2018) 114:180–92. doi: 10.1016/j.micpath.2017.11.061
49. Wu C, Wang Y, Shi X, Wang S, Ren H, Shen Z, et al. Rapid rise of the ESBL and mcr-1 genes in Escherichia coli of chicken origin in China, 2008-2014. Emerg Microbes Infect. (2018) 7:2008–14. doi: 10.1038/s41426-018-0033-1
50. Dahms C, Hubner NO, Kossow A, Mellmann A, Dittmann K, Kramer A. Occurrence of ESBL-producing Escherichia coli in livestock and farm workers in Mecklenburg-Western Pomerania, Germany. PLoS One. (2015) 10:1–13. doi: 10.1371/journal.pone.0143326
51. Chishimba K, Hang'ombe BM, Muzandu K, Mshana SE, Matee MI, Nakajima C, et al. Detection of extended-spectrum beta-lactamase-producing Escherichia coli in market-ready Chickens in Zambia. Int J Microbiol. (2016) 2016:5275724. doi: 10.1155/2016/5275724
52. Norizuki C, Wachino J-I, Suzuki M, Kawamura K, Nagano N, Kimura K, et al. Specific blaCTX-M-8/IncI1 plasmid transfer among genetically diverse Escherichia coli isolates between humans and chickens. Antimicrob Agents Chemother. (2017) 61:6–12. doi: 10.1128/AAC.00663-17
53. Kawamura K, Sugawara T, Matsuo N, Hayashi K, Tamai K, Norizuki C, et al. Spread of CTX-type extended-spectrum β-lactamase-producing escherichia coli isolates of epidemic clone B2-O25-ST131 among dogs and cats in Japan. Microb Drug Resist. (2017) 23:1059–66. doi: 10.1089/mdr.2016.0246
54. Overdevest I, Willemsen I, Rijnsburger M, Eustace A, Xu L, Hawkey P, et al. Extended-spectrum β-lactamase genes of Escherichia coli in chicken meat and humans, the Netherlands. Emerg Infect Dis. (2011) 17:1216–22. doi: 10.3201/eid1707.110209
55. Mshana SE, Matee M, Rweyemam M. Antimicrobial resistance in human and animal pathogens in Zambia. Ann Clin Microbiol Antimicrob. (2013) 12:1–10. Available online at: http://www.ann-clinmicrob.com/content/12/1/28#B22
56. Chapoto A, Sitko NJ. Agriculture in Zambia Past, Present and Future. Zambia: The Embassy of The Kingdom of The Netherlands in Zimbabwe in Conjunction with AgriProFocus Zambia and Poultry Association of Zambia
Keywords: antimicrobial resistance, poultry, ESBL E. coli, poultry farmworker, Zambia
Citation: Mwansa M, Mukuma M, Mulilo E, Kwenda G, Mainda G, Yamba K, Bumbangi FN, Muligisa-Muonga E, Phiri N, Silwamba I and Muma JB (2023) Determination of antimicrobial resistance patterns of Escherichia coli isolates from farm workers in broiler poultry production and assessment of antibiotic resistance awareness levels among poultry farmers in Lusaka, Zambia. Front. Public Health 10:998860. doi: 10.3389/fpubh.2022.998860
Received: 20 July 2022; Accepted: 09 December 2022;
Published: 10 January 2023.
Edited by:
Joseph D. Eifert, Virginia Tech, United StatesReviewed by:
Jacob Kwada Kwaga, Ahmadu Bello University, NigeriaCopyright © 2023 Mwansa, Mukuma, Mulilo, Kwenda, Mainda, Yamba, Bumbangi, Muligisa-Muonga, Phiri, Silwamba and Muma. This is an open-access article distributed under the terms of the Creative Commons Attribution License (CC BY). The use, distribution or reproduction in other forums is permitted, provided the original author(s) and the copyright owner(s) are credited and that the original publication in this journal is cited, in accordance with accepted academic practice. No use, distribution or reproduction is permitted which does not comply with these terms.
*Correspondence: Mercy Mukuma, bWVyY3kubXVrdW1hQHVuemEuem0=
Disclaimer: All claims expressed in this article are solely those of the authors and do not necessarily represent those of their affiliated organizations, or those of the publisher, the editors and the reviewers. Any product that may be evaluated in this article or claim that may be made by its manufacturer is not guaranteed or endorsed by the publisher.
Research integrity at Frontiers
Learn more about the work of our research integrity team to safeguard the quality of each article we publish.