- 1Department of Clinical Laboratory, Key Laboratory of Clinical Laboratory Diagnosis and Translational Research of Zhejiang Province, The First Affiliated Hospital of Wenzhou Medical University, Wenzhou, China
- 2School of Laboratory Medicine and Life Science, Wenzhou Medical University, Wenzhou, China
Klebsiella pneumoniae (K. pneumoniae) is one of the most common causes of bacterial meningitis worldwide. The purpose of this study was to investigate the clinical and microbiological characteristics of K. pneumoniae meningitis, as well as the association of antimicrobial resistance, virulence, and patient prognosis. The clinical data of patients with K. pneumoniae meningitis from 2014 to 2020 in a tertiary teaching hospital were retrospectively evaluated. Antimicrobial susceptibility profiles were performed by the agar dilution method and broth microdilution method. The isolates were detected for virulence-related genes, resistance genes, capsular serotypes, and molecular subtypes. A total of 36 individuals with K. pneumoniae meningitis were included in the study, accounting for 11.3% (36/318) of all cases of bacterial meningitis. Of the 36 available isolates, K1, K47, and K64 were tied for the most frequent serotype (7/36, 19.4%). MLST analysis classified the isolates into 14 distinct STs, with ST11 being the most common (14/36, 38.9%). Carbapenem resistance was found in 44.4% (16/36) of the isolates, while hypervirulent K. pneumoniae (HvKP) was found in 66.7% (24/36) of the isolates. The isolates of hypervirulent carbapenem-resistant K. pneumoniae (Hv-CRKP) were then confirmed to be 36.1% (13/36). Importantly, individuals with meningitis caused by Hv-CRKP had a statistically significant higher mortality than the other patients (92.3%, 12/13 vs. 56.5%, 13/23; P < 0.05). The high percentage and fatality of K. pneumoniae-caused meningitis, particularly in Hv-CRKP strains, should be of significant concern. More effective surveillance and treatment solutions will be required in future to avoid the spread of these life-threatening infections over the world.
Introduction
Bacterial meningitis is a potentially fatal cerebral illness that necessitates lengthy hospital stays and high expenses due to inadequate healthcare delivery (1, 2). The significant mortality and morbidity of bacterial meningitis have prompted widespread and severe concerns all over the world in recent years (2). Bacterial meningitis causes a variety of clinical symptoms, including fever, headache, nausea, vomiting, neck stiffness, or altered mental status, which can lead to herniation, coma, and even death (3, 4).
Klebsiella pneumoniae (K. pneumoniae) is an emerging cause of bacterial meningitis worldwide (5). Previously, K. pneumoniae was an increasingly frequent pathogen in Taiwan, and it was even the first pathogen engaged in adult community-acquired bacterial meningitis (CABM), with a prevalence rate of up to 68.3% among gram-negative pathogenic organisms (6). Fortunately, all third- and fourth-generation cephalosporins were effective against the K. pneumoniae strains, and none of the clinical isolates were shown to be an extended-spectrum β-lactamase-producing pathogen (6). The fast development of antimicrobial resistance bacteria, on the contrary, poses a severe public health risk. One recent research found that K. pneumoniae strains isolated from cerebrospinal fluid (CSF) were resistant to the majority of first-line antibiotics including carbapenems, with the exception of tigecycline and colistin (7). Carbapenem-resistant K. pneumoniae (CRKP) is classified as a “critical” category on the WHO global priority pathogen list (8). KPC-carrying strains are most common in China and are frequently associated with other types of β-lactamases (e.g., ESBLs) (7, 9). Klebsiella pneumoniae has been separated into two essentially nonoverlapping populations over the past three decades: classic K. pneumoniae (cKP) and hypervirulent K. pneumoniae (HvKP), both of which may produce severe invasive and disseminated infections (10, 11). HvKP strains are distinguished from cKP pathogens by genes on a large virulence plasmid or on chromosomal islands. One trustworthy study used the virulence genes peg-344 and iucA to distinguish between HvKP and cKP strains with a sensitivity of 0.94 and specificity of 1.0 (12). Furthermore, K. pneumoniae strains causing meningitis contain distinct resistance genes, capsular serotypes, virulence genes, and related clones, which might eventually lead to plasmid-mediated resistance and virulence transmission, which can have a negative influence on patient prognosis (13). However, few research has been conducted to investigate the characteristics of K. pneumoniae meningitis in depth.
In this study, we conducted a retrospective survey of K. pneumoniae meningitis in a tertiary teaching hospital of southeastern China during a 7-year period, systematically investigating the clinical, microbiological, and molecular epidemiological characteristics of K. pneumoniae meningitis, and comprehensively evaluating the risk factors that affect patients' survival, to provide some data for optimizing treatment of K. pneumoniae meningitis.
Materials and methods
Study patients
A total of 318 patients with K. pneumoniae meningitis were included between 2014 and 2020 in the First Affiliated Hospital of Wenzhou Medical University, which is a large tertiary teaching hospital located in Southeast China with 4,100 beds and more than 160,000 inpatients per year. Inclusion criteria for K. pneumoniae meningitis were as follows: (1) positive K. pneumoniae CSF culture; (2) suggestive symptoms of meningitis including fever, headache, vomiting, unconscious, muscle tone changes, and abnormal clinical laboratory examination findings including white blood cell count, procalcitonin, C-reactive protein, percentage of neutrophils in peripheral blood, red blood cell count, white blood cell count, sugar, chlorine, and protein content in CSF; (3) the case data and follow-up data of patients were complete.
Klebsiella pneumoniae isolation and identification
CSF samples were isolated from meningitis patients and cultured using a BacT/ALERT 3D microbial detection system (bioMerieux, Durham, NC) before being identified as K. pneumoniae in a matrix-assisted laser desorption/ionization time-of-flight mass spectrometry system (MALDI-TOF/MS; bioMérieux, Lyons, France). The isolates were kept at −80°C for further research. Duplicate strains isolated from the same patient were removed from the strain collecting process.
Antimicrobial susceptibility testing
The minimum inhibitory concentrations (MICs) of ampicillin, aztreonam, ceftriaxone, ceftazidime, cefepime, imipenem, ciprofloxacin, levofloxacin, gentamicin, tobramycin, sulfamethoxazole/trimethoprim, nitrofurantoin, colistin, and tigecycline were detected by the agar dilution method and broth microdilution method. The data were interpreted by the latest guidelines published by the Clinical and Laboratory Standards Institute (CLSI 2021, Pittsburgh, PA, USA). The MIC of colistin was determined with the broth microdilution method and interpreted using the recommendation of the European Committee on Antimicrobial Susceptibility Testing clinical breakpoints (EUCAST, http://www.eucast.org/). Escherichia coli ATCC 25922 was used as the control strain for antimicrobial susceptibility testing.
Detection of capsular serotypes gene, virulence genes, and resistance genes
Genomic DNA of K. pneumoniae strains was extracted using the Biospin Bacterial Genomic DNA Extraction kit (BioFlux, Tokyo, Japan). Subsequently, specific primers (Supplementary Table 1) were used to amplify the capsular serotype gene (wzi), virulence genes (peg-344, iroB, iucA, rmpA, and rmpA2), carbapenem resistance genes (blaKPC − 2, blaNDM, blaIMP, blaVIM, and blaOXA − 48), and ESBLs resistance genes (blaSHV, blaTEM, blaCTX − M−1, blaCTX − M−2, blaCTX − M−9, blaCTX − M−25, and blaCTX − M−65), and membrane porins encode resistance genes (OmpK35, OmpK36, and OmpK37).
Differentiation of HvKP and cKP strains
According to a credible study, we employed two virulence genes, peg-344 and iucA, to separate HvKP and cKP strains with a sensitivity of 0.94 and specificity of 1.0, demonstrating the efficacy of gene markers for accurately identifying HvKP strains (12).
Multilocus sequence typing
MLST analyses of the K. pneumoniae isolates were performed by amplifying seven housekeeping genes (gapA, mdh, phoE, tonB, infB, pgi, and rpoB). Sequence types were allocated by searching the database (https://blast.ncbi.nlm.nih.gov/Blast.cgi?CMD=Web&PAGE_TYPE=BlastHome) using the given methods.
Statistical analysis
The statistical analysis was calculated using SPSS 22.0 software (IBM, Armonk, NY, USA). Continuous data were reported as mean ± standard deviation (mean ± SD) or median [inter-quartile range (IQR)] and analyzed using unpaired Student's t-test (two-tailed), while categorical variables were given as percentages and compared using the chi-square test (two-tailed). Multivariate logistic regression analysis was used to explore the independent risk factors for death from K. pneumoniae meningitis. A P-value of <0.05 was considered as statistically significant.
Ethics approval
This study conformed to the Declaration of Helsinki and was approved by the Ethics Committee of the First Affiliated Hospital of Wenzhou Medical University (Issuing No. 2021R094). Written informed consent for inclusion from each patient was waived by the Ethics Committee of the First Affiliated Hospital of Wenzhou Medical University because this was a retrospective study, and no study-related interventions were included.
Results
Klebsiella pneumoniae strains isolated from cerebrospinal fluid
In this study, a total of 318 cases of bacterial meningitis were detected at the First Affiliated Hospital of Wenzhou Medical University (Wenzhou, China) from 2014 to 2020, and 36 K. pneumoniae meningitis were eventually screened according to the inclusion criteria (Supplementary Figure 1). The percentage of K. pneumoniae isolates ranked fourth (11.3%, 36/318) among all pathogens and second to Acinetobacter baumannii (14.51%, 46/318) among gram-negative pathogens.
Clinical characteristics of the 36 K. pneumoniae meningitis cases
There were 29 male patients and seven female patients among the 36 K. pneumoniae meningitis patients, with an average age of 54 years old (IQR 44, 62), an ICU admission rate of 58.3% (21/36), a mechanical ventilation rate of 75.0% (27/36), and a fatality rate of 69.4% (25/36). About 27.8% (10/36) of the meningitis cases were community-acquired infection, while 72.2% (26/36) were acquired in the hospital. The detailed clinical characteristics of the 36 cases are summarized in Table 1 and Supplementary Table 2. When compared to the survival group, the death group demonstrated statistically significant differences in vomiting (P = 0.015), unconsciousness (P = 0.010), length of stay days (P = 0.016), bacteremia (P = 0.025), ICU admission (P = 0.025), mechanical ventilation (P = 0.012), Glasgow coma scale (GCS) score (P = 0.001), C-reactive protein (P = 0.008), and protein in CSF profiles (P = 0.014; Table 1). When compared to the Hv-CRKP group, the non-Hv-CRKP group showed differences in age (P = 0.034) and diabetes mellitus (P = 0.025, Supplementary Table 3).
Antimicrobial susceptibility profiles
The antimicrobial resistance information and detailed MICs of the 36 isolates are listed in Table 2 and Supplementary Table 4 in the supplemental material. Generally, the isolates showed high resistance rates to ampicillin (AMP), ceftriaxone (CRO), ceftazidime (CAZ), cefepime (FEP), nitrofurantoin (NIT), ciprofloxacin (CIP), levofloxacin (LEV), gentamicin (GEN), and fosfomycin (FOS), and 47.2% (17/36) of the isolates were identified as multi-drug resistant (MDR), which were defined as non-susceptible to three or more different antimicrobial categories. Notably, 41.7% (15/36) of the isolates were resistant to imipenem and meropenem, whereas 44.4% (16/36) were resistant to ertapenem. Colistin and tigecycline were effective against all 36 isolates. There were no significant differences in antimicrobial resistance rates between the surviving and mortality groups (Table 2).
Molecular epidemiological characteristics of the 36 K. pneumoniae strains
High predominance of capsular serotypes was detected in K1 (7/36, 19.4%), K47 (7/36, 19.4%), K64 (7/36, 19.4%), K54 (4/36, 11.1%), and K57 (3/36, 8.3%), whereas K2, K9, K10, K19, K63, and K149 showed relatively low ratios of <10%. There were no statistically significant differences in capsular serotype distribution between strains isolated from patients with poor prognosis and those with good prognosis (Table 3 and Figure 1). MLST classified the 36 K. pneumoniae isolates into 14 distinct sequence types (STs), with ST11 being the most common (14/36, 38.9%), followed by ST23 (5/36, 13.9%), ST29 (4/36, 11.1%), and other STs (Figure 1 and Supplementary Figure 2).
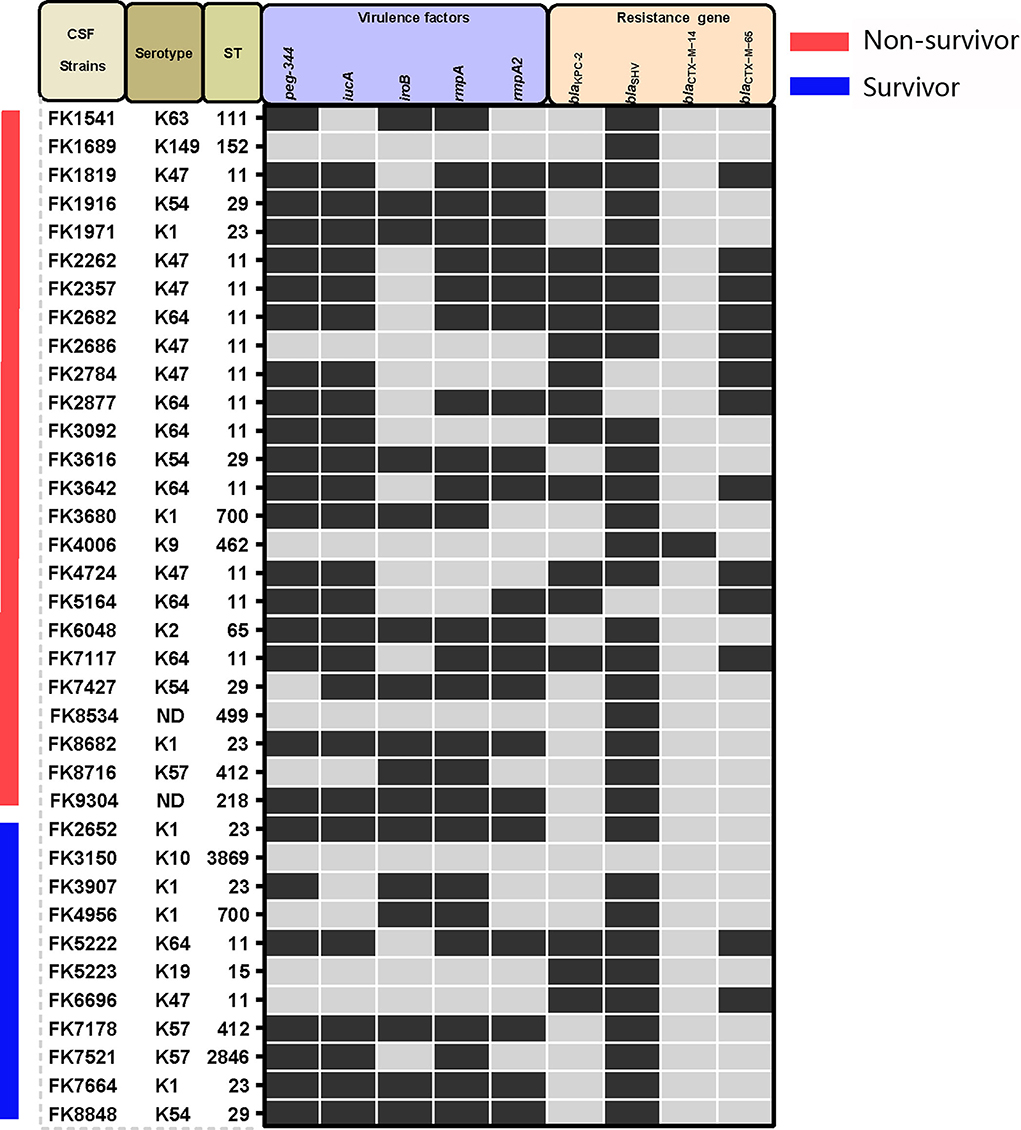
Figure 1. Capsular serotypes, virulence genes, and resistance genes found in the K. pneumoniae strains in this study. Positive is represented by black squares, whereas negative is represented by gray squares.
Distribution of virulence and antimicrobial resistance determinants
Virulence-related genes, including peg-344, iucA, iroB, rmpA, and rmpA2, were found in proportions of 69.4 (25/36), 66.7 (24/36), 44.4 (16/36), 69.4 (25/36), and 55.6% (20/36), respectively, and no significant differences in virulence distribution were found between strains of the two different prognostic groups (Table 3 and Figure 1) Furthermore, the blaKPC − 2 gene was found in 15 out of the 36 isolates, whereas blaCTX−M−14, blaCTX−M−65, and blaSHV were found in one isolate, 13 isolates, and 32 isolates, respectively. The OmpK36 and OmpK37 mutation caused FK3616 to be resistant to carbapenems drugs. Similarly, there was no statistically significant difference in the distribution of antimicrobial resistance genes between the death and survival groups (Table 3 and Figure 1).
Hypervirulent carbapenem-resistant K. pneumoniae was associated with high patient mortality
We identified the peg-344 and iucA carrying strains as hypervirulence according to one reliable preliminary research with highest efficiency of a sensitivity of 0.94 and specificity of 1.0 (12). Twenty-four isolates were eventually classified as hypervirulent K. pneumoniae (HvKP), and 12 isolates were classified as classic K. pneumoniae (cKP, Supplementary Table 4). However, only nine strains were identified as hypermucoviscosity phenotype using the traditional string test, and the consistency between hypervirulence and hypermucoviscosity phenotype was only 20.8% (5/24). Furthermore, 16 isolates were identified as carbapenem-resistant K. pneumoniae (CRKP) based on antimicrobial susceptibility profiles and 20 isolates as carbapenem-susceptible K. pneumoniae (CSKP, Table 2 and Supplementary Table 4). Thus, by overlapping the 24 HvKP strains and 16 CRKP strains, 13 hypervirulent carbapenem-resistant K. pneumoniae (Hv-CRKP) isolates were identified, while the other 23 isolates were designated as non-Hv-CRKP strains. Time distributions of the HvKP, CRKP, and Hv-CRKP isolates from 2014 to 2020 are shown in Figure 2A. There were no statistically significant differences in patient mortality between the CRKP and CSKP groups (Figure 2B), nor between HvKP and cKP groups (Figure 2C). However, the Hv-CRKP group had a much greater death rate (92.3%, 12/13) than the non-Hv-CRKP group (56.5%, 13/23, Figure 2D).
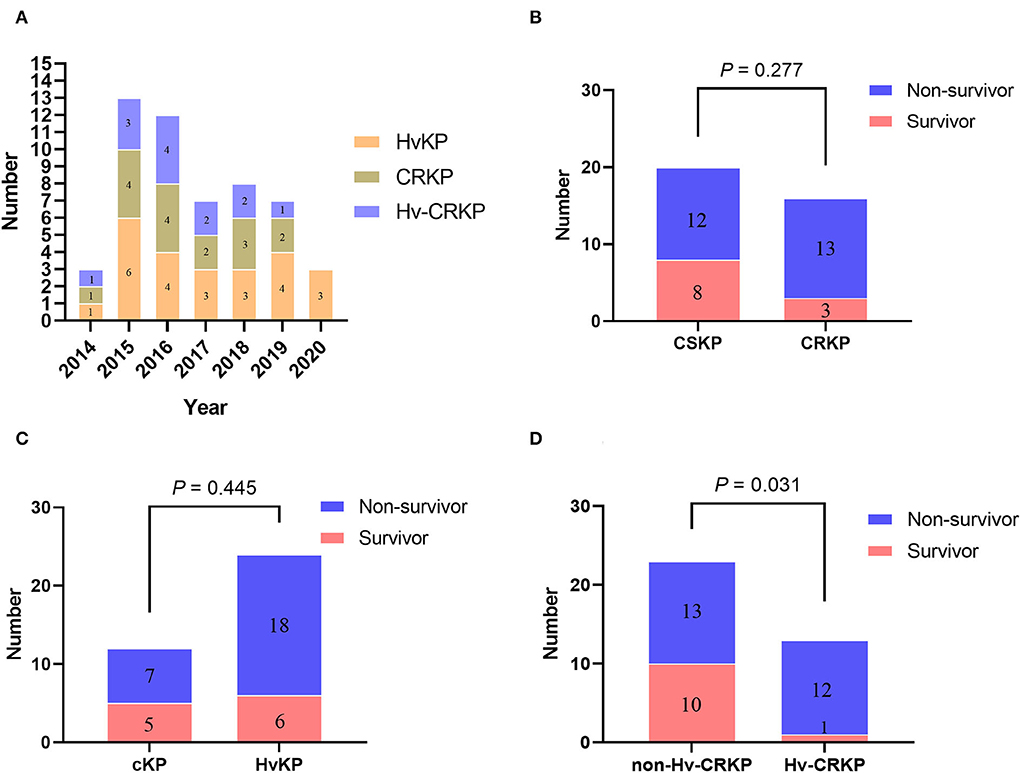
Figure 2. Time distribution and survival rate characteristics of the strains. (A) The number of specific strains detected in each year; (B) Comparison of mortality between patients infected with CSKP and CRKP; (C) Comparison of mortality between patients infected with cKP and HvKP; (D) Comparison of mortality between patients infected with non-Hv-CRKP and Hv-CRKP.
Discussion
Bacterial meningitis, which has a high morbidity and fatality rate, has emerged as a critical public health hazard in recent years (5, 14). This study comprehensively explored the clinical, microbiological, and molecular epidemiological characteristics of K. pneumoniae-induced meningitis in a tertiary teaching hospital in China from 2014 to 2020.
In our current study, we discovered a relatively high prevalence of K. pneumoniae (11.3%, 36/318) in bacterial meningitis, which is consistent with the epidemiological data from some specific geographic areas (i.e., the Netherlands and Taiwan), where K. pneumoniae is commonly described as the primary and predominant meningitis-related pathogens (15, 16). Unfortunately, the fatality rate for the 36 K. pneumoniae meningitis patients reached up to 69.4% (25/36). Male gender, advanced age, hypertension, diabetes mellitus, history of surgery, head trauma, and intracerebral hemorrhage were all common among the bacterial meningitis patients, as reported by Lien et al. (17). Only the death group had clinical signs of vomiting and headache, which might be attributed to central nervous system impairment. When compared to the survival group, the death group had a significantly higher frequency of mechanical ventilation and peripheral deep vein catheter operation, an ICU admission rate, and lower GCS score. The death group also had a higher C-reactive protein (CRP) level of blood and protein level of CSF profiles, which was consistent with previous research (18, 19). One study found that Hv-CRKP can be killed by neutrophil extracellular traps (NETs) in diabetics because neutrophils isolated from patients with type 2 diabetes mellitus exhibited enhanced NET formation (20), which may explain why the Hv-CRKP strain is rare in diabetics. In this study, we found that bacteremia is closely related to meningitis patients with poor prognosis, 92.9% (13/14) of the patients with bacteremia and meningitis died, and bacteremia followed by meningitis was identified in 64.3% (9/14) of patients, indicating that bacteremia is an important cause of subsequent meningitis. Our other unpublished findings indicated that outer membrane protein A (OmpA) may help K. pneumoniae cross the blood–brain barrier and cause meningitis in bacteremia patients. Because interactions between hosts and pathogens lead to a different clinical outcomes during bacterial infections, clinicians should pay special attention to patients who exhibit the features listed above.
There are at least 78 capsular serotypes of K. pneumoniae strains, with capsule types K1 and K2 being particularly pathogenic (21). The prevalence of K1 and K2 in our study was 19.4 (7/36) and 2.8% (1/36), respectively, which was essentially consistent with the previous report (7). ST11 was determined to be the most common type in the MLST study, with a rate of 38.9% (14/36), which was similar to a previous report (22). ST11 is the most dominant blaKPC − 2-bearing K. pneumoniae clone in China, with more numerous genes that allow for tremendous adaptation and transmission throughout the hospital and community (23, 24). Among the 13 strains of Hv-CRKP in this study, there were 12 strains of ST11 with serotype K47/K64. It is necessary to pay attention to KPC-2-producing ST11-K47/K64 Hv-CRKP as they act as a serious clinical threat (25). Furthermore, 13.9% (5/36) of the strains belonged to ST23, which has been identified as the most prevalent MLST type of capsule serotype K1 K. pneumoniae isolates (26), and our study revealed that all ST23 strains were K1 capsule serotype. In summary, the dispersed STs in this study confirmed the genetic and characteristic variety of the K. pneumoniae isolates, indicating that these strains did not exhibit clonal and cluster transmission features.
Antimicrobial resistance and virulence were thought to be important factors in disease development. In this study, most K. pneumoniae isolates were extremely resistant to a wide range of antimicrobial agents, including β-lactamase inhibitors, third generation cephalosporins, and carbapenems, with a resistance rate that was greater than that reported from Taiwan and Beijing (6, 27). Fortunately, all of the 36 K. pneumoniae isolates were susceptible to polymyxins and tigecycline. WHO classified carbapenem-resistant K. pneumoniae (CRKP) as a critical priority tier of pathogens and the highest priority in novel antimicrobial development in 2017 (8). In China, KPC is the most prevalent genetic mechanism of carbapenem resistance in K. pneumoniae species, and it is frequently coupled with other forms of β-lactamases (e.g., ESBLs) (7, 9, 28, 29). In this study, we discovered the co-existence of KPC gene and ESBLs genes. Furthermore, distinct carbapenemase genes in K. pneumoniae are frequently coupled with mobile structures, including insertion sequences (IS), plasmids, and transposons, which can accelerate resistance transmission (30), so it should be closely monitored in the medical environment.
Traditionally, the HvKP was characterized by a positive string test or a substantial contributor marker to virulence (31), but this is not totally right. In this study, we identified nine strains as hypermucoviscosity phenotype, and the consistency between hypervirulence and hypermucoviscosity phenotype was only 20.8% (5/24), indicating that the string test performed suboptimally to define HvKP, and will result in substantially more erroneous classifications than with virulence genes detection, which is consistent with other studies (12, 32). Recently, genetic research revealed elevated levels of capsule and siderophore production (predominantly aerobactin) as virulence features, and the combination of peg-344 and iucA effectively raised HvKP definition accuracy to 0.98 (sensitivity 0.94 and specificity 1.0) (12, 33). According to one study, the virulence genes, peg-344, iroB, iucA, rmpA, and rmpA2, all had diagnostic accuracy more than 0.95 for identifying HvKP strains (7, 12). Among these virulence genes, rmpA and rmpA2 act as mucoid phenotype regulators through enhanced capsule production (33, 34) and were found in 69.4 (25/36) and 55.6% (20/36) of the 36 isolates in this study, respectively. Some rmpA and rmpA2-positive strains, however, did not exhibit hypermucoviscosity phenotype (data not shown), indicating that alternative regulatory mechanisms for hypermucoviscosity expression may exist. Peg-344 functions as an inner membrane transporter and is identified on the HvKP virulence plasmid, which can improve the potential of HvKP to spread (35). In our study, 69.4% (25/36) of the isolates tested positive for the peg-344 gene; salmochelin biosynthetic gene, iroB, synthesizing salmonella siderophores, being associated with K. pneumoniae virulence (12, 36), was found in 44.4% (16/36) of the 36 isolates in this study. Aerobactin is essential for K. pneumoniae growth and virulence in the host via regulation of iron supply, which could serve as a potential anti-virulence target (34, 37), and our study found that up to 66.7% (24/36) of the isolates were aerobactin-positive, as confirmed by iucA, one of the genes in iuc operon encoding for aerobactin.
We then studied the antimicrobial resistance and virulence characteristics that were associated with diverse prognosis. There was no statistically significant difference in patient mortality between CRKP and CSKP groups, and nor between HvKP and cKP groups. However, as compared to the other cases (non-Hv-CRKP group, 56.5 %, 13/23), patients with K. pneumoniae meningitis in the Hv-CRKP group had a significantly greater mortality rate (92.3 %, 12/13), indicating a synergistic deadly impact of carbapenem resistance and hypervirulence. Similarly, one study previously demonstrated a 100% fatal outbreak of carbapenem-resistant hypervirulent K. pneumoniae isolated from five patients with pneumonia in China (38). To make matters worse, it has been demonstrated that K. pneumoniae strains causing meningitis can cause plasmid-mediated resistance and virulence transmission, plasmids encoding resistance to kanamycin, tobramycin, and ampicillin could be conjugated to Escherichia coli (39), and that plasmids harboring various virulence genes including rmpA, rmpA2, aerobactin, and salmochelin could disseminate to other strains and sublineages (40). Furthermore, plasmids containing blaKPC − 2 in carbapenem-resistant K. pneumoniae (CRKP) of sequence type 11 (ST11) can be horizontally transferred between other species via conjugation (41). If this condition continues to deteriorate, Hv-CRKP might become a true global “superbug” posing a major threat to public health (42). As a result, early and precise diagnosis and treatment of Hv-CRKP is extremely beneficial in lowering patient mortality.
Our study elaborated the clinical, microbiological, and molecular epidemiological characteristics of K. pneumoniae isolated from 36 meningitis cases, although it has several limitations. First, it was single-center research with a small number of patients included retrospectively enrolled limited cases, which might restrict epidemiological scope and raise selection and information biases, and it even influenced us to investigate the independent risk factors for death from K. pneumoniae meningitis. Second, because there are no reference standards for HvKP identification, additional reliable in vivo tests (such as mice models and neutrophil phagocytosis assay) may be required to define HvKP strains.
Conclusion
Our findings showed that antimicrobial resistance and hypervirulence are common in K. pneumoniae strains recovered from bacterial meningitis patients, as well as the increased mortality caused by Hv-CRKP strains. Greater innovation and investment in developing several novel techniques to tackle these life-threatening infections will be necessary in future.
Data availability statement
The original contributions presented in the study are included in the article/Supplementary material, further inquiries can be directed to the corresponding authors.
Ethics statement
The studies involving human participants were reviewed and approved by the Ethics Committee of the First Affiliated Hospital of Wenzhou Medical University. Written informed consent from the participants' legal guardian/next of kin was not required to participate in this study in accordance with the national legislation and the institutional requirements.
Author contributions
NH, HJ, and BZ conceived of the presented idea. CZ, JC, and WL collected the data. NH, SL, and JY drafted the manuscript. LW, LiqC, and LijC were responsible for the statistical evaluation. NH, TZ, and JY contributed to the interpretation of the results. All authors have approved the final manuscript.
Funding
This study was supported by the research grants from the Health Department of Zhejiang Province of the People's Republic of China (no. 2019KY098), the National Natural Science Foundation of China (nos. 81971986 and 82072347), and Key Laboratory of Clinical Laboratory Diagnosis and Translational Research of Zhejiang Province (no. 2022E10022).
Conflict of interest
The authors declare that the research was conducted in the absence of any commercial or financial relationships that could be construed as a potential conflict of interest.
Publisher's note
All claims expressed in this article are solely those of the authors and do not necessarily represent those of their affiliated organizations, or those of the publisher, the editors and the reviewers. Any product that may be evaluated in this article, or claim that may be made by its manufacturer, is not guaranteed or endorsed by the publisher.
Supplementary material
The Supplementary Material for this article can be found online at: https://www.frontiersin.org/articles/10.3389/fpubh.2022.991306/full#supplementary-material
References
1. Figueiredo AHA, Brouwer MC, van de Beek D. Acute community-acquired bacterial meningitis. Neurol Clin. (2018) 36:809–20. doi: 10.1016/j.ncl.2018.06.007
2. Wall EC, Chan JM, Gil E, Heyderman RS. Acute bacterial meningitis. Curr Opin Neurol. (2021) 34:386–95. doi: 10.1097/WCO.0000000000000934
3. van de Beek D, de Gans J, Spanjaard L, Weisfelt M, Reitsma JB, Vermeulen M. Clinical features and prognostic factors in adults with bacterial meningitis. N Engl J Med. (2004) 351:1849–59. doi: 10.1056/NEJMoa040845
4. Putz K, Hayani K, Zar FA. Meningitis. Prim Care. (2013) 40:707–26. doi: 10.1016/j.pop.2013.06.001
5. Piednoir P, Clarac U, Rolle A, Bastian S, Gruel G, Martino F, et al. Spontaneous community-acquired bacterial meningitis in adults admitted to the intensive care units in the Caribbean French West Indies: unusual prevalence of K. pneumonia. Int J Infect Dis. (2020) 100:473–5. doi: 10.1016/j.ijid.2020.09.1420
6. Lee PY, Chang WN, Lu CH, Lin MW, Cheng BC, Chien CC, et al. Clinical features and in vitro antimicrobial susceptibilities of community-acquired K. pneumoniae meningitis in Taiwan. J Antimicrob Chemother. (2003) 51:957–62. doi: 10.1093/jac/dkg158
7. Xu M, Fu Y, Fang Y, Xu H, Kong H, Liu Y, et al. High prevalence of KPC-2-producing hypervirulent K. pneumoniae causing meningitis in Eastern China. Infect Drug Resist. (2019) 12:641–53. doi: 10.2147/IDR.S191892
8. Tacconelli E, Carrara E, Savoldi A, Harbarth S, Mendelson M, Monnet DL, et al. Discovery, research, and development of new antibiotics: the WHO priority list of antibiotic-resistant bacteria and tuberculosis. Lancet Infect Dis. (2018) 18:318–27. doi: 10.1016/S1473-3099(17)30753-3
9. Sawa T, Kooguchi K, Moriyama K. Molecular diversity of extended-spectrum beta-lactamases and carbapenemases, and antimicrobial resistance. J Intensive Care. (2020) 8:13. doi: 10.1186/s40560-020-0429-6
10. Tang Y, Liu H, Zhao J, Yi M, Yuan Y, Xia Y. Clinical and microbiological prognostic factors of in-hospital mortality caused by hypervirulent K. pneumoniae infections: a retrospective study in a tertiary hospital in Southwestern China. Infect Drug Resist. (2020) 13:3739–49. doi: 10.2147/IDR.S276642
11. Lin ZW, Zheng JX, Bai B, Xu GJ, Lin FJ, Chen Z, et al. Characteristics of hypervirulent K. pneumoniae: does low expression of rmpA contribute to the absence of hypervirulence? Front Microbiol. (2020) 11:436. doi: 10.3389/fmicb.2020.00436
12. Russo TA, Olson R, Fang CT, Stoesser N, Miller M, MacDonald U, et al. Identification of biomarkers for differentiation of hypervirulent K. pneumoniae from classical K. pneumoniae. J Clin Microbiol. (2018) 56:e00776–18. doi: 10.1128/JCM.00776-18
13. Ku YH, Chuang YC, Chen CC, Lee MF, Yang YC, Tang HJ, et al. Klebsiella pneumoniae isolates from meningitis: epidemiology, virulence and antibiotic resistance. Sci Rep. (2017) 7:6634. doi: 10.1038/s41598-017-06878-6
14. Brouwer MC, Tunkel AR, van de Beek D. Epidemiology, diagnosis, and antimicrobial treatment of acute bacterial meningitis. Clin Microbiol Rev. (2010) 23:467–92. doi: 10.1128/CMR.00070-09
15. Bijlsma MW, Brouwer MC, Kasanmoentalib ES, Kloek AT, Lucas MJ, Tanck MW, et al. Community-acquired bacterial meningitis in adults in the Netherlands, 2006–2014: a prospective cohort study. Lancet Infect Dis. (2016) 16:339–47. doi: 10.1016/S1473-3099(15)00430-2
16. Lien CY, Huang CR, Tsai WC, Hsu CW, Tsai NW, Chang CC, et al. Epidemiologic trend of adult bacterial meningitis in southern Taiwan (2006–2015). J Clin Neurosci. (2017) 42:59–65. doi: 10.1016/j.jocn.2017.03.017
17. Lien CY, Lee JJ, Tsai WC, Chen SY, Huang CR, Chien CC, et al. The clinical characteristics of spontaneous gram-negative bacterial meningitis in adults: a hospital-based study. J Clin Neurosci. (2019) 64:101–5. doi: 10.1016/j.jocn.2019.03.047
18. Long F, Chen QL, Wu SY, Liu Y, Zhang WL, Liao QF, et al. Etiology, prognosis and risk factors of 181 adult community-acquired acute bacterial meningitis. Sichuan Da Xue Xue Bao Yi Xue Ban. (2018) 49:808–11. doi: 10.13464/j.scuxbyxb.2018.05.025
19. Khan FY, Abukhattab M, AbuKamar M, Anand D. Adult K. pneumoniae meningitis in Qatar: clinical pattern of ten cases. Asian Pac J Trop Biomed. (2014) 4:669–72. doi: 10.12980/APJTB.4.201414B100
20. Jin L, Liu Y, Jing C, Wang R, Wang Q, Wang H. Neutrophil extracellular traps (NETs)-mediated killing of carbapenem-resistant hypervirulent K. pneumoniae (CR-hvKP) are impaired in patients with diabetes mellitus. Virulence. (2020) 11:1122–30. doi: 10.1080/21505594.2020.1809325
21. Podschun R, Ullmann U. Klebsiella spp. as nosocomial pathogens: epidemiology, taxonomy, typing methods, and pathogenicity factors. Clin Microbiol Rev. (1998) 11:589–603. doi: 10.1128/CMR.11.4.589
22. Hu Y, Liu C, Shen Z, Zhou H, Cao J, Chen S, et al. Prevalence, risk factors and molecular epidemiology of carbapenem-resistant K. pneumoniae in patients from Zhejiang, China, 2008–2018. Emerg Microbes Infect. (2020) 9:1771–9. doi: 10.1080/22221751.2020.1799721
23. Li DX, Zhai Y, Zhang Z, Guo YT, Wang ZW, He ZL, et al. Genetic factors related to the widespread dissemination of ST11 extensively drug-resistant carbapenemase-producing K. pneumoniae strains within hospital. Chin Med J. (2020) 133:2573–85. doi: 10.1097/CM9.0000000000001101
24. Liu C, Du P, Zhao J, Li B, Wang C, Sun L, et al. Phenotypic and genomic characterization of virulence heterogeneity in multidrug-resistant ST11 K. pneumoniae during inter-host transmission and evolution. Infect Drug Resist. (2020) 13:1713–21. doi: 10.2147/IDR.S243836
25. Wei T, Zou C, Qin J, Tao J, Yan L, Wang J, et al. Emergence of hypervirulent ST11-K64 K. pneumoniae poses a serious clinical threat in older patients. Front Public Health. (2022) 10:765624. doi: 10.3389/fpubh.2022.765624
26. Siu LK, Fung CP, Chang FY, Lee N, Yeh KM, Koh TH, et al. Molecular typing and virulence analysis of serotype K1 K. pneumoniae strains isolated from liver abscess patients and stool samples from noninfectious subjects in Hong Kong, Singapore, and Taiwan. J Clin Microbiol. (2011) 49:3761–5. doi: 10.1128/JCM.00977-11
27. Chang JB, Wu H, Wang H, Ma BT, Wang RZ, Wei JJ. Prevalence and antibiotic resistance of bacteria isolated from the cerebrospinal fluid of neurosurgical patients at Peking Union Medical College Hospital. Antimicrob Resist Infect Control. (2018) 7:41. doi: 10.1186/s13756-018-0323-3
28. Zhao Y, Zhang S, Fang R, Wu Q, Li J, Zhang Y, et al. Dynamic epidemiology and virulence characteristics of carbapenem-resistant K. pneumoniae in Wenzhou, China from 2003 to 2016. Infect Drug Resist. (2020) 13:931-940. doi: 10.2147/IDR.S243032
29. Zhang X, Li F, Cui S, Mao L, Li X, Awan F, et al. Prevalence and distribution characteristics of blaKPC-2 and blaNDM-1 genes in K. pneumoniae. Infect Drug Resist. (2020) 13:2901–10. doi: 10.2147/IDR.S253631
30. Cerdeira LT, Lam MMC, Wyres KL, Wick RR, Judd LM, Lopes R, et al. Small IncQ1 and col-like plasmids harboring bla KPC-2 and non-Tn4401 elements (NTEKPC-IId) in high-risk lineages of K. pneumoniae CG258. Antimicrob Agents Chemother. (2019) 63:e02140–18. doi: 10.1128/AAC.02140-18
31. Shon AS, Bajwa RP, Russo TA. Hypervirulent (hypermucoviscous) K. pneumoniae: a new and dangerous breed. Virulence. (2013) 4:107–18. doi: 10.4161/viru.22718
32. Lin YC, Lu MC, Tang HL, Liu HC, Chen CH, Liu KS, et al. Assessment of hypermucoviscosity as a virulence factor for experimental K. pneumoniae infections: comparative virulence analysis with hypermucoviscosity-negative strain. BMC Microbiol. (2011) 11:50. doi: 10.1186/1471-2180-11-50
33. Cheng HY, Chen YS, Wu CY, Chang HY, Lai YC, Peng HL. RmpA regulation of capsular polysaccharide biosynthesis in K. pneumoniae CG43. J Bacteriol. (2010) 192:3144–58. doi: 10.1128/JB.00031-10
34. Russo TA, Olson R, MacDonald U, Beanan J, Davidson BA. Aerobactin, but not yersiniabactin, salmochelin, or enterobactin, enables the growth/survival of hypervirulent (hypermucoviscous) K. pneumoniae ex vivo and in vivo. Infect Immun. (2015) 83:3325–33. doi: 10.1128/IAI.00430-15
35. Bulger J, MacDonald U, Olson R, Beanan J, Russo TA. Metabolite transporter PEG344 is required for full virulence of hypervirulent K. pneumoniae strain hvKP1 after pulmonary but not subcutaneous challenge. Infect Immun. (2017) 85:e00093–17. doi: 10.1128/IAI.00093-17
36. Yan Q, Zhou M, Zou M, Liu WE. Hypervirulent K. pneumoniae induced ventilator-associated pneumonia in mechanically ventilated patients in China. Eur J Clin Microbiol Infect Dis. (2016) 35:387–96. doi: 10.1007/s10096-015-2551-2
37. Russo TA, Olson R, Macdonald U, Metzger D, Maltese LM, Drake EJ, et al. Aerobactin mediates virulence and accounts for increased siderophore production under iron-limiting conditions by hypervirulent (hypermucoviscous) K. pneumoniae. Infect Immun. (2014) 82:2356–67. doi: 10.1128/IAI.01667-13
38. Gu D, Dong N, Zheng Z, Lin D, Huang M, Wang L, et al. A fatal outbreak of ST11 carbapenem-resistant hypervirulent K. pneumoniae in a Chinese hospital: a molecular epidemiological study. Lancet Infect Dis. (2018) 18:37–46. doi: 10.1016/S1473-3099(17)30489-9
39. Woloj M, Tolmasky ME, Roberts MC, Crosa JH. Plasmid-encoded amikacin resistance in multiresistant strains of K. pneumoniae isolated from neonates with meningitis. Antimicrob Agents Chemother. (1986) 29:315–9. doi: 10.1128/AAC.29.2.315
40. Rodrigues C. d'Humieres C, Papin G, Passet V, Ruppe E, Brisse S. Community-acquired infection caused by the uncommon hypervirulent K. pneumoniae ST66-K2 lineage. Microb Genom. (2020) 6:7641421. doi: 10.1099/mgen.0.000419
41. Zeng L, Zhang J, Hu K, Li J, Wang J, Yang C, et al. Microbial characteristics and genomic analysis of an ST11 carbapenem-resistant K. pneumoniae strain carrying bla KPC-2 conjugative drug-resistant plasmid. Front Public Health. (2021) 9:809753. doi: 10.3389/fpubh.2021.809753
Keywords: Klebsiella pneumoniae, meningitis, hypervirulence, carbapenem-resistant, infection
Citation: Huang N, Jia H, Zhou B, Zhou C, Cao J, Liao W, Liu S, Wang L, Chen L, Chen L, Zhou T and Ye J (2022) Hypervirulent carbapenem-resistant Klebsiella pneumoniae causing highly fatal meningitis in southeastern China. Front. Public Health 10:991306. doi: 10.3389/fpubh.2022.991306
Received: 11 July 2022; Accepted: 26 September 2022;
Published: 17 October 2022.
Edited by:
Eduardo Rodriguez-Noriega, Civil Hospital of Guadalajara, MexicoReviewed by:
Kasturi Banerjee, Stony Brook University, United StatesNatália Silva Da Costa Granato, Federal University of Rio de Janeiro, Brazil
Jianmin Wang, Chongqing Medical University, China
Luigi Principe, Azienda Sanitaria Provinciale di Crotone, Italy
Copyright © 2022 Huang, Jia, Zhou, Zhou, Cao, Liao, Liu, Wang, Chen, Chen, Zhou and Ye. This is an open-access article distributed under the terms of the Creative Commons Attribution License (CC BY). The use, distribution or reproduction in other forums is permitted, provided the original author(s) and the copyright owner(s) are credited and that the original publication in this journal is cited, in accordance with accepted academic practice. No use, distribution or reproduction is permitted which does not comply with these terms.
*Correspondence: Jianzhong Ye, anp5ZTg5JiN4MDAwNDA7MTYzLmNvbQ==; Tieli Zhou, d3l6dGxpJiN4MDAwNDA7MTYzLmNvbQ==