- Department of Expanded Program on Immunization, Hangzhou Center for Disease Control and Prevention, Hangzhou, China
Background: It is necessary to select suitable inactivated poliovirus vaccine(IPV) and live, attenuated oral poliovirus vaccine (OPV) sequential immunization programs and configure the corresponding health resources. An economic evaluation was conducted on the sequential procedures of Sabin strain-based IPV (sIPV) and bivalent OPV (bOPV) with different doses to verify whether a cost-effectiveness target can be achieved. This study aimed to evaluate the cost-effectiveness of different sIPV immunization schedules, which would provide convincing evidence to further change the poliovirus vaccine (PV) immunization strategies in China.
Methods: Five strategies were included in this analysis. Based on Strategy 0(S0), the incremental cost (IC), incremental effect (IE), and incremental cost-effectiveness ratio (ICER) of the four different strategies (S1/S2/S3/S4) were calculated based on the perspective of the society. Seven cost items were included in this study. Results of field investigations and expert consultations were used to calculate these costs.
Results: The ICs of S1/S2/S3/S4 was Chinese Yuan (CNY) 30.77, 68.58, 103.82, and 219.82 million, respectively. The IE of vaccine-associated paralytic poliomyelitis (IEVAPP) cases of S1/S2/S3/S4 were 0.22, 0.22, 0.22, and 0.11, respectively, while the IE of disability-adjusted life-years (IEDALY) of S1/S2/S3/S4 were 8.98, 8.98, 8.98, and 4.49, respectively. The ICERVAPP of S1/S2/S3/S4 gradually increased to CNY 13.99, 31.17, 47.19, and 199.83 million/VAPP, respectively. The ICERDALY of S1/S2/S3/S4 also gradually increased to CNY 0.34, 0.76, 1.16, and 4.90 million/DALY, respectively.
Conclusion: ICERVAPP and ICERDALY were substantially higher for S3 (four-sIPV) and S4 (replacement of self-funded sIPV based on one-sIPV-three-bOPV). Two-sIPV-two-bOPV had a cost-effectiveness advantage, whereas S2/S3/S4 had no cost-effectiveness advantage.
Introduction
Poliovirus can cause limb paralysis, an acute intestinal tract infectious disease commonly known as poliomyelitis. To date, there is no effective treatment for poliomyelitis, and it can only be prevented through vaccination (1). There are three serotypes of poliovirus including serotypes I, II, and III, all of which can cause acute infectious diseases without cross-immunity (2). There are two types of poliovirus vaccines (PV): live, attenuated oral poliovirus vaccine (OPV) and inactivated poliovirus vaccine (IPV). OPV was produced using Sabin virus strains, which were divided into trivalent OPV (tOPV), bivalent OPV (bOPV), and monovalent OPV (mOPV), according to the strains contained in the vaccine. TOPV contains type I, II, and III virus strains, while bOPV contains type I and III virus strains; mOPV is divided into type I-mOPV, type II-mOPV, and type III-mOPV. OPV was first licensed as monovalent OPV (mOPV) in 1961 and trivalent OPV (tOPV) in 1963. IPV was made available in the market and licensed in 1955 (2). OPV is a safe and effective vaccine and has become the vaccine of choice for the Global Polio Eradication Initiative (GPEI) owing to its high immunization efficacy, low cost, and convenient administration. The extremely effective programs implemented by the World Health Organization (WHO) resulted in the declaration of the eradication of wild-type 2 poliovirus in 2016 and wild-type 3 poliovirus in 2019 (3). However, vaccine-associated paralytic poliomyelitis (VAPP) and vaccine-derived poliovirus (VDPV) can occur in an under-immunized population. IPV can be produced using Sabin and Salk strains, both of which are trivalent vaccines that are effective against poliovirus types I, II, and III. IPV can produce an ideal immune response and durable immunity, and inactivation of the vaccine virus does not cause VAPP and VDPV (4). However, owing to its high price, IPVs are mostly used in developed countries (5).
Since 1988, when the WHO began to carry out GPEI (6, 7), the WHO regions of the Americas, Western Pacific, and Europe were officially declared polio free (8), region of South-East Asia was certified in 2014, African region was certified in 2020 (3). China has made significant progress in polio eradication through the use of OPV, strengthening OPV routine immunization (RI) and supplementary immunization activities, and strengthening surveillance of acute flaccid paralysis (AFP). China has been free of indigenous polio cases since September 1994, when the last case of indigenous wild poliovirus (WPV) was reported. In October 2000, the WHO region of Western Pacific, including China's, was declared polio free (9). Polio eradication refers not only to the elimination of WPVs, but also to the elimination of VAPP and VDPV cases. In theory, as long as OPV is used, the above problems are inevitable. Before May 2016, China's PV immunization was implemented as follows: one dose of OPV at 2, 3, and 4 months old and one dose of OPV at 4 years old. The WHO formulated the Polio Eradication and Endgame Strategic Plan 2013–2018 (3) as well as the third Global Action Plan to minimize poliovirus facility-associated risk (GAPIII) (10) requiring all countries to use at least one dose of IPV, replace tOPV with bOPV by the end of April 2016, and finally stop using OPV in 2019–2020. In May 2016, China began to change the PV immunization strategy. Children were vaccinated with one dose of IPV at 2 months old, and one dose of bOPV at 3, 4 months, and 4 years old. On January 14, 2015, China Food and Drug Administration approved the production of the world's first inactivated vaccine (single vaccine) from Sabin polio strain produced by the Institute of Medical Biology, Chinese Academy of Medical Sciences, and the vaccine was officially put on the market in July 2015. The listing of domestic Sabin strain-based IPV (sIPV) provides the possibility for IPV to be included in China's immunization schedule, which plays a crucial role in the complete eradication of poliomyelitis in China (11). Currently, the IPV used in China's immunization planning includes sIPV produced by domestic enterprises and Salk strain-based IPV imported from abroad, and sIPV is primarily used. At present, there is shortage of sIPV supply worldwide (12), and China is one of the few countries that can produce sIPV. In addition, IPV will gradually replace OPV, and Chinese sIPV will play a major role.
Domestic sIPV demonstrated good immunogenicity and safety profile, and the positive conversion rates of neutralizing antibodies against types I, II, and III were 100, 94.9, and 99.0%, respectively, after three doses of basic immunization (13). The sequential procedures of IPV and OPV vary in different countries worldwide, and 1–4 doses of IPV and OPV are used (5). bOPV is a newly developed vaccine in China. Studies have shown that after sequential immunization with Salk strain-based IPV and domestic bOPV at one or two doses, the positive conversion rate of neutralizing antibodies against serum poliovirus types I and III can reach up to >94%, and it is safe (14). According to GAPIII, China will implement the transformation of PV immunization strategies in the future. Hence, it is necessary to select suitable IPV and OPV sequential immunization programs and configure the corresponding health resources. At the same time, the acceptance of different populations to the transformation strategy should be considered prior to the transformation to ensure the smooth implementation of the transformation of the immunization strategy and maintain a polio-free status. Adjustment of the immunization strategy of PV will also greatly affect the allocation of funds and resources for public health immunization programs. An economic evaluation is a comparative analysis of the costs and results of alternative activity processes (15). Therefore, a health economic evaluation must be conducted before adjusting the immunization strategy for PV. An economic evaluation was conducted on the sequential procedures of sIPV and bOPV with different doses to verify whether a cost-effectiveness target can be achieved. The incremental cost-effectiveness ratio (ICER), one of the commonly used evaluation indices, can be used to evaluate the relationship between two or more alternative treatment economies (16) and is suitable for the comparison of different immune programs.
Materials and methods
Study strategy
According to the procedures currently used in Hangzhou and those required by the WHO for conversion, five strategies were included in the analysis. Strategy 0 (S0): one-sIPV-three-bOPV, Strategy 1 (S1): two-sIPV-two-bOPV, Strategy 2 (S2): three-sIPV-one-bOPV, Strategy 3 (S3): four-sIPV, and Strategy 4 (S4): calculation of the replacement of the self-funded sIPV based on S0.
Economic indicators
The IC, incremental effectiveness (IE), and ICER of the four different strategies were calculated based on the perspective of the society. IC refers to the difference between the cost change of the S1/S2/S3/S4 PV immunization strategy and that of S0. The efficacy was measured based on the incidence of VAPP and disability-adjusted life-years (DALY). The DALY is a comprehensive measure of healthy life years lost to disease-related death and disability. To calculate the DALY of VAPP, the years of life lost and years lived with disability were comprehensively calculated. The age relative value (age weight) and time relative value (discounting rate) of life-years were used for weighted adjustment. IE refers to the difference between the effect index of strategies S1/S2/S3/S4 and S0 and the ratio of incremental cost (IC) to IE, namely, the ICER.
The variable costs in this study included seven items: vaccine cost, vaccination cost, storage and transportation cost, medical waste disposal cost, adverse reactions related to PV, VDPV-related cost, and parents' traffic delay cost. The cost of an outbreak was not included in the analysis (17).
Field investigation and expert consultation were used to calculate the above costs.
Incremental cost composition
Basic parameters
Number of newborns
In the Opinions of the Central Committee of the Communist Party of China and the State Council on Adjusting and Improving the Family Planning Policy issued in December 2013, the policy of allowing couples to have two children if one parent was an only child was implemented. According to the total population in the Statistical bulletin of Hangzhou Bureau of Statistics from 2013 to 2018 and the change rule of the number of newborns in Hangzhou Immunization Planning Information System, the average number of newborns from 2013 to 2018 is 123,373 (Supplementary Table 1).
PV immunization coverage rate
The monitoring report of the RI coverage rate can dynamically reflect the overall RI situation in a timely manner. According to a report on the RI coverage rate in Hangzhou from 2016 to 2018, the vaccination coverage rate of four doses of PV in all districts and counties exceeded 99%. To ensure the reliability of the monitoring data, Hangzhou carried out an annual field survey on the vaccination rate of school-age children, which is consistent with the routine monitoring data. The PV coverage rates of the four doses in this study were calculated and analyzed to be 99%.
Self-funded sIPV replacement rate
The self-funded sIPV replacement rate was defined as the proportion of self-funded sIPV (including DTaP/Hib/IPV) as replacement for free sIPV and bOPV routine vaccination. Data on the usage of self-funded sIPV and DTaP/Hib/IPV in Hangzhou from 2016 to 2018 were obtained from the annual report on non-immunization program (NIP) vaccine use in Hangzhou. Data on the PV vaccination dose in Hangzhou from 2016 to 2018 were obtained from the monthly report on RI (Supplementary Table 2). The calculated average replacement rate for self-funded sIPV from 2016 to 2018 was 36.54%. The declining trend in the self-funded sIPV replacement rate from 2016 to 2018 is mainly related to the insufficient supply of DTaP/Hib/IPV. The supply of DTaP/Hib/IPV returned to normal in 2019; therefore, the self-funded sIPV replacement rate in this study was 50%.
Loss coefficient
The loss coefficient of bOPV was 2.50, while that of sIPV was 1.05 based on the regulations for vaccination. According to the monthly report on the Hangzhou immunization planning vaccine, the loss coefficient of bOPV was 1.68, while that of free sIPV was 1.01 from 2016 to 2018. In this study, the bOPV loss coefficient was 1.70, the free sIPV loss coefficient was 1.01, the self-funded sIPV loss coefficient was not considered, and the loss coefficient of syringes was 1.05.
Immunization schedules
In this study, infants aged 2, 3, and 4 months received one dose of PV, while those aged 4 years received one booster dose. The study strategy, S0/S1/S2/S3/S4, was calculated as four doses of PV per child.
Economic indicators
The per capita gross domestic product (GDP) data used in this study were obtained from the official website of the Hangzhou Bureau of Statistics (http://tjj.hangzhou.gov.cn/), and the per capita GDP announced by the Hangzhou Bureau of Statistics in 2018 was Chinese Yuan (CNY) 140,180 per year. The CNY exchange rate data used in this study were obtained from the State Administration of Foreign Exchange (http://www.safe.gov.cn). In 2018, the exchange rate of CNY to USD was 1:6.86. This study did not discount the future, but only described the data from the literature published before 2018.
Composition of IC
Vaccine IC
Vaccine IC for S1/S2/S3/S4 = vaccine cost for S1/S2/S3/S4 – vaccine cost for S0.
Vaccine cost = number of newborns × PV coverage rate × (sIPV loss coefficient × sIPV dose × sIPV vaccine price + bOPV loss coefficient × bOPV dose × bOPV vaccine price).
According to the price of vaccines purchased through bidding in Zhejiang Province, free sIPV was CNY 35 per dose, bOPV was CNY 2.72 per dose, self-funded sIPV was CNY 168 per dose, and DTaP/Hib/IPV was CNY 599 per dose.
The vaccine cost of S0 was CNY 6011,939, while the vaccine ICs of S1/S2/S3/S4 were CNY 3,752,851, 7,505,703, 11,258,554, and 29,931,938, respectively. The costs of S1-S4 increased gradually.
Vaccination IC
Vaccination IC of S1/S2/S3/S4 = vaccination cost of S1/S2/S3/S4 – vaccination cost of S0.
Vaccination cost = number of newborns × vaccination rate of PV × [sIPV dose times × (syringe price × syringe loss coefficient + sIPV vaccination subsidy + sIPV vaccination working time cost) + bOPV dose times × (bOPV vaccination subsidy + bOPV vaccination working time cost)].
The price of the syringe in this study refers to the price of a 2-ml syringe in Zhejiang province, which is set at CNY 0.22 per syringe.
According to the principle of “Notice on Strengthening Vaccination Work” issued by the General Office of the Ministry of Health in 2012, each dose of planned immunization vaccine should not be less than CNY 5 of the subsidy. The service fee for non-immunization vaccination is CNY 28 per dose, according to the price standard of Zhejiang Province. In this study, the subsidy for free vaccine injection was CNY 5 per dose, while the subsidy for self-funded vaccine was CNY 28 per dose.
Due to time constraints, this study in the process of estimating incremental work time cost, combined with routine vaccination outpatient service supervision and assessment through the monitoring of timely sIPV and bOPV vaccination differences in work time and the vaccination that the doctors know, confirm that the differences in the influence of two vaccines' work time are not obvious, and the incremental work time cost is zero.
The IC inoculated in S1/S2/S3/S4 was CNY 28,214, 56,428, 84,642, and 4,213,804, respectively, and the cost of inoculation in S1-S4 gradually increased.
Storage and transportation of IC
Storage and transportation IC = Δvaccine cold chain cost + Δtransportation cost = ΔChange in vaccine volume × (cold chain cost per unit volume + transportation cost per unit volume).
In this study, the change ratio of the total volume of NIP vaccine for each child after the introduction of sIPV was calculated. If the change range was ±20%, the change in storage and transportation costs was considered to be 0; the judgment basis was as follows: (1) The cold chain volume matching degree (MVC) refers to the ratio of the vaccine-cold chain capacity needs of a region to the capacity of cold chain equipment required to store vaccines when providing vaccination services for the population in the region within a certain period of time. 0.8 ≤ MVC ≤ 1.2 means that it can operate normally without adding cold-chain equipment (18). (2) The Hangzhou Center for Disease Control and Prevention (CDC) entrusted the storage and transportation of vaccines in the immunization program to qualified third-party vaccine distribution units for distribution; of the 15 district and county CDCs in Hangzhou, 13 were entrusted with the storage and transportation of all immunization and non-immunization vaccines to two qualified third-party vaccine distribution units; therefore, the introduction of sIPV has little impact on the volume of vaccine storage and transportation, does not require the increase in the cost of cold chain equipment and transportation, and does not affect the price of vaccine storage and transportation services.
S1/S2/S3/S4 vaccine volume changes were calculated using the WHO-recommended vaccine volume calculation tool (19). The total volume of NIP vaccines/children was calculated according to the vaccine package volume of China's immunization program in Supplementary Table 3, the total volume of NIP vaccine/children was calculated, and the volume change rate of the PV strategy was compared. The adjustment of S1/S2/S3/S4 was 3.04–9.12% of the total volume change rate of the vaccine (Table 1). From the perspective of the society as a whole, this study considered that the overall storage and transportation cost of sIPV introduced by NIP remained unchanged; ΔStorage and transportation cost = 0.
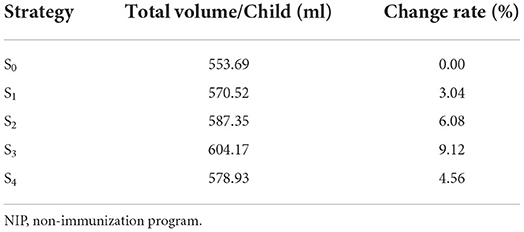
Table 1. Vaccine volume calculation tool to calculate the volume change of NIP vaccine for each child.
Medical waste treatment IC
The medical waste treatment IC of S1/S2/S3/S4 = medical waste treatment cost of S1/S2/S3/S4-medical waste treatment cost of S0.
Cost of medical waste disposal = number of newborns × PV vaccination rate × weight of medical waste in each child × unit price of medical waste disposal.
Medical waste refers to the use of disposable syringes during vaccination, cotton swabs, Schering bottles, ampule bottles, etc., and the medical waste disposal management team understands the fees related to the disposal of this type of wastes. For hospitals without beds, CNY 1,000 is usually charged per month; however, as every collector uses one box only, a preferential price of 800 per month is offered, which is paid annually. Vaccination clinics were also informed that the medical waste disposal management cost for PV using different strategies would not increase. Therefore, the IC of medical waste treatment in S1/S2/S3/S4 was 0.
IC for adverse reactions to PV
IC for S1/S2/S3/S4 associated with the adverse reactions to PV = S1/S2/S3/S4 associated with the adverse reactions to PV cost–S0 associated with the adverse reactions to PV cost.
The WHO official position paper mentioned that sIPV is one of the safest vaccines in RI, whether used alone or in combination (20). There were no confirmed adverse events other than mild local erythema (0.5–1%), induration (3–11%), and tenderness (14–29%) (20). No serious adverse reactions were observed, and the cost of sIPV was set at 0 after discussion with the experts.
OPV is a safe vaccine; however, adverse events can occur in rare cases. In this study, cost referred to the cost of treating VAPP caused by OPV.
Cost of VAPP = cost of VAPP disabling + cost of VAPP dying = Number of OPV first-dose vaccinations × incidence rate of VAPP × (1-VAPP fatality rate) × cost of VAPP disability/cases + number of OPV first-dose vaccinations × incidence rate of VAPP × mortality rate of VAPP × cost of VAPP death/cases.
The incidence of VAPP varies greatly from country to country owing to the sensitivity of the monitoring systems. Platt et al. evaluated the incidence of VAPP caused by OPV use in 4.70 per million neonates worldwide (range: 2.40–9.70 per million neonates) (21). The AFP monitoring system in Hangzhou is highly sensitive, and the incidence of VAPP was 8.82/million neonates from 2010 to 2014 (22), which was higher than that reported by Platt et al., but within the credible range; therefore, the incidence of VAPP caused by OPV was set at 8.82/million neonates in this study.
No VAPP-related deaths were reported in Hangzhou from 2010 to 2014, which may be due to the low number of VAPP cases (five cases). Foreign studies conducted by Griffiths et al. set the fatality rate of VAPP at 5% (23). According to the data from the China CDC, the fatality rate of VAPP is estimated to be 2.36% (24). Combined with the local monitoring situation, the fatality rate of VAPP caused by OPV was 2.36% in this study. Ulla et al. set the VAPP failure coefficient to 0.37. Based on the research data from Li Renpeng in China, the VAPP failure coefficient was set to 0.48.
According to the monitoring results of the immune success rate in this study, the positive conversion rates of poliovirus type I antibody and poliovirus type III antibody in the one-sIPV-two-bOPV, two-sIPV-one-bOPV, and three-sIPV groups were 100%, except for the positive conversion rates of poliovirus type II antibody, which were 81, 96, and 99%, respectively. Therefore, according to the above results, the rate reduction in the incidence of VAPP after the first dose of sIPV inoculation was set to 80% in this study; after the second dose, it was set to 100%.
Cost of VAPP-disabled cases/case = (direct cost + indirect cost)/case = [(medical expenses + transportation expenses of patients in hospital + transportation and accommodation expenses of escorts) + (income reduced due to disability + government subsidies + time lost for escorts)]/case.
Cost of death from VAPP = (direct cost + indirect cost)/case = [(medical expenses + hospitalization transportation expenses + transportation and accommodation expenses of escorts) + (income reduced due to disability + government subsidies + time lost for escorts)]/case.
Dai et al. (25) investigated the disease burden of polio cases in 1993, after which no other relevant research data were available in China. This study aimed to compound the use cost data in this study, using the Net Present Value method to translate medical expenses, accommodation expenses, and transportation expenses into the present value of 2018, and using the price index R for medical care and personal goods at the discount rate (26). For the days lost in the study, the government should refer to the Zhejiang Province compensation method for abnormal reactions to vaccination: one-off compensation will be provided in case of death caused by an abnormal reaction to vaccination. The amount of economic compensation shall be calculated according to the following items and standards: 10 years for the death of urban residents age < 18 years (including those aged 18 years), calculated according to the per capita disposable income of urban residents in the previous year, and 15 years of compensation for death of those aged > 18 years: Economic compensation amount = per capita disposable income of urban residents in Zhejiang province in the previous year × compensation years. VAPP is prevalent among people aged < 18 years; therefore, the compensation period is 10 years. One-off compensation should be provided to residents with disability caused by an abnormal reaction to the vaccination. The economic compensation amount is calculated according to the following items and standards: disability grade based on the “medical accident classification standard (trial),” per capita disposable income of urban residents in Zhejiang province in the previous year, compensation period (i.e., the longest compensation is 20 years from the date of disability), and disability coefficient (1–0.1, which corresponds to disability grades 1 B to grade 3 E). The disability grade was grade 3B, the disability coefficient was 0.4, and the compensation period was calculated based on a 20-year period:
Medical expenses/case = Medical expenses ×
Inpatient transportation cost/case = inpatient transportation cost (25) ×
Transportation and accommodation costs of attendants/case = transportation and accommodation costs of attendants (25) ×
Attendant missing work = number of days attendants missed work (25) × per capita GDP in 2018/365 days.
Income lost due to disability = 2018 GDP per capita × 30 years of service × VAPP disability coefficient.
According to the above formula, the burden of VAPP-disabling diseases is CNY 3,211,803.00, and the burden of fatal VAPP diseases is CNY 5,616,005.49 (Table 2).
In S0, the average annual disease burden of VAPP was CNY 704,219.34. In S1/S2/S3, more than 2 doses of sIPV were administered, no VAPP occurred, and the disease burden of VAPP was CNY 0. In S4, 50% of the population was vaccinated with only 1 dose of sIPV. Hence, the average annual disease burden of S4 VAPP was CNY 352,109.67. The disposal of adverse reactions after S1/S2/S3/S4 PV inoculation were as follows: CNY −704,219.34, −704,219.34, −704,219.34, and −352,109.67, respectively.
VDPV-Related IC
VDPV-related IC of S1/S2/S3/S4 = VDPV-related cost of S1/S2/S3/S4-VDPV-related cost of S0.
VDPV-related cost =circulating vaccine-derived viruses (cVDPV) cost + immunodeficiency vaccine-derivcd poliovirus (iVDPV) cost + ambiguous vaccine-derivcd poliovirus (aVDPV) cost.
According to surveys (27–29), no aVDPV was found in China and Hangzhou, so the aVDPV cost was regarded as zero in this paper.
cVDPV costGlobally, an average of 76 cVDPV cases occur each year, mainly in 23 less developed countries (30). Considering the previous cVDPV incidence in China, cVDPV I occurred in Zhenfeng County, Buyi, and Miao Autonomous Prefecture, Guizhou Province in 2004, and two cases of VDPV I were reported. A circulating polio-hypervariable virus was detected in Aba County, Aba Tibetan, and Qiang Autonomous Prefecture, Sichuan Province in 2012. One case of cVDPV II and three cases of VDPV II were reported. The national cVDPV incidence is one case every 5 years, with three polio cases per case. According to a comparison between the population of Hangzhou and the national population, the number of cVDPV cases per year was 0.01. For reference, the cost of emergency work after the occurrence of cVDPVs in Guizhou province in August 2004 = the cost of OPV enhanced immunization + the cost of fecal specimen collection, transportation, and testing = CNY 52.85 million (24); the average annual cost of emergency work caused by cVDPV in Hangzhou is CNY 2,114 million. Disease burden of polio cases = 0.004 cases × (disease burden of VAPP disability cases × (1-VAPP fatality rate) + disease burden of VAPP fatality cases × VAPP fatality rate). The average annual burden of cVDPV in China was CNY 12,597.03. The cVDPV incidence of S0/S1/S2/S4 is the same, the cVDPV cost of S0/S1/S2/S4 is CNY 223,977.03, and the cVDPV incidence and related costs of S3 are 0. The annual cVDPV-related IC of S1/S2/S3/S4 were as follows: CNY 0, 0, −223,977.03, and 0.
iVDPV costOne case of iVDPV was reported in Anhui in 2005, Ningxia in 2011, Tianjin in 2012, and Jiangxi in 2013 (31), while one iVDPV case was reported every 2 years in China. According to the results of the comparison between the population of Hangzhou and the national population, the annual iVDPV of Hangzhou is 0.0033. Based on the cost of carrying out emergency immunization and case surveillance after the occurrence of iVDPVs in Anhui province in 2005, iVDPV emergency work cost = OPV emergency immunization cost + stool specimen collection, transportation, and testing cost + construction of sanitary toilets + disinfection cost + personnel subsidy = CNY 4.49 million/case. The disease burden of iVDPV cases is regarded as VAPP fatality cases = CNY 5,616,005.49/case. Before OPV use is terminated, the risk of iVDPV always exists. The incidence of iVDPV of S0/S1/S2/S4 is the same. The iVDPV cost of S0/S1/S2/S4 = (CNY 4.488 million/case +CNY 5,616,005.49/case) × 0.0033 = CNY 33,343.22/year. The incidence and cost of iVDPV in S3 were zero, while the annual iVDPV-related ICs of S1/S2/S3/S4 were CNY 0, 0, −33,343.22, and 0, respectively.
The annual VDPV-related ICs of S1/S2/S3/S4 were CNY 0, 0, −257, 320.25, and 0, respectively.
Traffic cost and IC of missed work for parents taking their children for vaccination
The cost of traffic and cost of delayed work only had an impact on S4. In the immunization program, OPV was administered at the same time at 3, 4, and 18 months of age, and 50% of children in S4 went to the vaccination clinic one less time compared with those in S0. Traffic cost and time cost of parents per inoculation = estimated traffic cost per inoculation + number of accompanying personnel × number of missed work days × per capita GDP in 2018/365 days = CNY 20/time +1.5 accompanying personnel/time × 0.5 days × per capita GDP in 2018/365 = CNY 308.04/time. The total cost = number of newborns in 2018 × PV coverage rate × 0.5, doses × traffic cost and time cost of parents per vaccination = CNY 11,811,890.36. In S1/S2/S3/S4, the traffic and delayed work costs of parents taking their children to vaccinate were CNY 0, 0, 0, and −11,811,890.36, respectively.
Sensitivity analysis
A univariate sensitivity analysis was conducted to explore the impact of parameter uncertainty by considering simultaneous changes within plausible ranges. The incidence of VAPP cases with the five schedules, compensation cost of VAPP cases, and cost of IPV were included according to the range of values to evaluate the influence of changes in these parameters on the ICER.
Results
Incremental cost
The proposed variable cost composition of this study includes 7 items, while the ICs of S1/S2/S3/S4 are CNY 30.77, 68.58, 103.82, and 219.82 million, respectively (Table 3).
Incremental effectiveness
S1/S2/S3/S4 IEVAPP = S0 annual number of VAPP cases—S1/S2/S3/S4 annual number of VAPP cases.
IEDALY of S1/S2/S3/S4 = annual DALY lost to VAPP cases in S0–annual DALY lost to VAPP cases in S1/S2/S3/S4.
DALY loss caused by VAPP = (number of VAPP disability cases × disability coefficient + number of VAPP fatality cases) × average life expectancy.
In S0, the number of VAPP cases per case was 0.22; in S4, the number of VAPP cases per case was 0.11; and in S1/S2/S3, no VAPP cases were reported.
The IEVAPP cases for S1/S2/S3/S4 were 0.22, 0.22, 0.22, and 0.11, respectively.
The IEDALY of S1/S2/S3/S4 were 8.98, 8.98, 8.98, and 4.49, respectively.
Incremental cost-effectiveness ratio
The ICERVAPP of S1/S2/S3/S4 increased gradually to CNY 13.99, 31.17, 47.19, 199.83 million/VAPP, respectively. The ICERDALY of S1/S2/S3/S4 also increased gradually, which were CNY 0.34, 0.76, 1.16, and 4.90 million/DALY, respectively (Table 4).
ICERVAPP and ICERDALY were substantially high for S3 (four-sIPV) and S4 (replacement of self-funded sIPV based on one-sIPV-three-bOPV). The recommendations of WHO on pharmacoeconomic evaluation (32): ICERDALY < per capita GDP, with high cost-effectiveness; per capita GDP < ICERDALY < 3 times GDP per capita, cost-effectiveness; and ICERDALY >3 times per capita GDP, without cost-effectiveness advantage. In 2018, the per capita GDP of the Hangzhou Statistics Bureau was CNY 0.14 million/year. S1 (two-sIPV-two-bOPV) had a cost-effectiveness advantage, whereas S2/S3/S4 had no cost-effectiveness advantage.
Discussion
The economic benefits of IPV-containing immunization schedules need to be further clarified. This study evaluated the cost-effectiveness of different IPV-containing immunization schedules in Hangzhou, which could provide convincing evidence to further switch PV immunization strategies in China. In this study, we found that two-sIPV-two-bOPV has a cost-effectiveness advantage, whereas other schedules have no cost-effectiveness advantage. IPV-containing schedules are currently cost-effective in Hangzhou. They may be cost-effective by reducing the prices of sIPV, which may accelerate polio eradication in China.
Recent studies have shown that sIPV inclusion in the immunization schedule remains cost-effectiveness, although it differs extensively between studies (33–36). Studies have shown that Global OPV cessation offered the possibility of large future health and economic benefits compared with continued OPV use (37). OPV remains the PV of choice in most countries because of their low cost (38). Most high-income countries use IPV exclusively for RI, while middle-income countries continue to adopt IPV for RI using a sequential schedule of IPV followed by OPV (IPV/OPV) or an IPV dose co-administered with the third non-birth OPV dose (38). IPV remains much more expensive than OPV but does not come with VAPP or cVDPV risks because it does not contain a live poliovirus (39). In upper-middle-income countries, PV immunization schedules are cost-effective in addition to their efficacy in preventing diseases, which means that the expected cost of treatment exceeds the difference in the cost between the schedules (40), with the best estimates suggest incremental net benefits for the intervention of between ~$40 and $50 billion when compared to Routine vaccination, assuming reasonable economic values for the prevention or treatment of paralysis (40). By contrast, in low-income countries, the cost of immunization schedules remains relatively high and the treatment cost is much lower, which implies the need to pay additional costs to obtain effectiveness (40). In addition, projections of the cost of the vaccine are greatly dependent on supply and the ability to negotiate a competitive price (17). Estimates of vaccine costs were largely driven by the assumption that middle-income countries would gradually switch to IPV in any scenario. With the large population of middle-income countries, including China, and the higher cost of IPV, middle-income countries contribute significantly to the difference in the global cost of the different policy scenarios. If the price of IPV decreased over time or if fewer middle-income countries were to switch to IPV, the cost of universal IPV would be lower. In this study, ICERDALY of S3 (4 doses sIPV) = 1.16 million, had no cost-effectiveness advantage. If 0.14 million < ICERDALY of S3(4 doses sIPV) < 0.42 million, S3 would had a cost-effectiveness advantage. Assuming everything else is equal, the price for sIPV is between CNY 10 to CNY 17 could make 4 doses sIPV schedule have cost-effectiveness advantage. Vaccine costs are not the only factor to be considered in policy decision-making. This suggests the possible limitations of utilizing the ICER in vaccine evaluation. The fundamental issues that should be taken into account when deciding to introduce a new vaccine include the type of vaccine, the target disease, and the strength of immunization program (41).
Policymakers should consider not only the costs of global policies but also potential benefits such as prevention of VAPP cases. The risk of VAPP continues to exist for policies that involve OPV use, and the continuous use OPV increases the number of VAPP cases. Findings from the health economics evaluation on replacing OPV with IPV varied widely: ICERVAPP ranged between USD 0.74 and 80.0 million, while ICERDALY caused by VAPP ranged between USD 0.01 and 5.60 million (37, 42). If the introduction of sIPV doses is associated with the replacement of the respective OPV doses, the cost increase will be approximately USD 20 and 59 million, respectively (35). Replacing the first three OPV doses with IPV on RI with discontinuation of supplemental immunization reduced the incidence of VAPP to 51 (35). In this study, we found that ICERVAPP and ICERDALY were substantially high for four-sIPV (CNY 47.19 million and 1.16 million), and the replacement of self-funded sIPV based on one-sIPV-three-bOPV (CNY 199.83 and 4.90 million), compared with those of the previous one-sIPV-three-bOPV schedule in < city>Hangzhou < /city>, China. Similar results were reported in previous studies. The two-IPV-two-bOPV and four-IPV schedules could prevent 1.20–1.35 VAPP cases and avert 16.83–18.96 DALYs per year, compared with the four-tOPV schedule in Shanghai, China (43). In Russia, the incidence of VAPP cases was much lower for sequential immunization schedule of OPV and IPV (1 case/4.18 million doses) than that for the OPV-only schedule (1 case/1.59 million OPV doses) (44). In the United States, no VAPP occurred in the IPV-containing schedule, while 2.9 VAPP cases/million occurred in the OPV schedule (45). ICERVAPP and averting DALYVAPP varied widely (40, 42, 46). In this study, the two-sIPV-two-bOPV immunization schedule was cost-effective, according to the WHO guidelines (47). Our findings suggest that the lower cost of sIPV and higher VAPP incidence may contribute to the lower ICER and higher cost-effectiveness. The ICER varies across countries with different economic status (33, 42). In 1996, an American study of children aged < 6 years reported an ICERVAPP of USD 3.1 million per VAPP for the two-IPV-two-bOPV schedule and USD 3 million per VAPP for the four-IPV schedule (46). By contrast, a 2001 Australian study conducted in children aged < 6 years showed that the ICERVAPP were USD 10 million per VAPP and USD 29 million per VAPP for these two schedules (48). In addition, the ICERDALY in Columbia was USD 0.071 million per DALY (42). The disparity in ICERVAPP across countries may be related to the differing costs of IPV and OPV, incidence of VAPP, and compensation costs for VAPP in different countries (33–36).
However, IPV does not protect against infections or from participation in asymptomatic fecal-oral poliovirus transmission, and its ability to stop or prevent poliovirus transmission were not tested in developing countries (39, 49). Consistent with the data from clinical trials showing limited intestinal immunity provided by IPV (49), despite an IPV-only RI coverage of over 90%, Israel recently detected intense asymptomatic WPV1 transmission for 12 months, likely due to the relatively low hygienic conditions in the Bedouin populations in the South (50, 51). IPV may offer a relatively greater reduction in long-term global risks associated with iVDPV introduction or other releases and may help prevent sustained transmission of OPV-related viruses and thus cVDPV emergence in settings with higher RI coverage and less fecal-oral transmission (52). Although the plan anticipates the simultaneous globally coordinated cessation of serotype 1-containing OPV (OPV1) and serotype 3-containing OPV (OPV3) after 2018 (OPV13 cessation), the possibility of certification of global WPV3 interruption in 2016, while WPV1 may continue to circulate, raising the potential for phased withdrawal of OPV3 and then OPV1 (30). Duintjer's study showed that IPV may prevent initial transmissions that lead to the establishment of population-wide transmission (37). No viable outbreak response strategy exists to stop poliovirus spread if it occurs more than 5–10 years after OPV cessation and in the absence of a large mOPV stockpile in populations highly susceptible to fecal-oral poliovirus transmission. The cost of maintaining an outbreak response capacity represents about 10% of the total program cost. However, an increase in the price of OPV would increase the cost of maintaining stockpiles and responding to outbreaks. This analysis aims to show policymakers the relative costs associated with various sIPV immunization policies with different doses. This analysis presents some of the costs including vaccine IC, vaccination IC, adverse reactions to PV vaccination IC, VDPV related IC, and parents traffic delay IC that will affect the IC of sIPV immunization program with different doses. The four PV immunization schedules in this study have both advantages and disadvantages in terms of safety, effectiveness, accessibility, and cost of administration (46, 53). To achieve the goal of polio eradication, VAPP and VDPV caused by OPV use should be eliminated (54). IPV provides protection against all three poliovirus serotypes, which facilitates the maintenance of polio-free status (55). Therefore, the possible social and economic impact of VAPP cannot ignore, such as the vaccine hesitancy in Nigeria in 2003 (56), although the four-IPV schedule was cost-ineffective in our study. In Shanghai in 2019, in the context of implementing the free two-IPV-two-bOPV schedule, 47% of children's parents chose the four-IPV schedule at their own expense or an IPV-containing combined vaccine (unpublished data), suggesting a high intention to receive IPV. This report indicates the feasibility of including the four-IPV immunization schedule in local EPI in resource-rich Chinese settings, such as the metropolitan areas like Shanghai (43). Other key uncertainties that affect the probability of outbreaks and/or their consequences include the long-term survival of immunodeficient patients with lower income levels, the impact of IPV-induced immunity on transmission and/or extent of fecal-oral spread in different populations, the quality and frequency of tOPV rounds until OPV2 cessation and bOPV rounds leading up to OPV13 cessation, the future rate of release of WPV or sIPV production sites in the context of different levels of containment, the unpredictable occurrence of other extremely rare events after OPV cessation with very large consequences, and the potential for OPV used during outbreak response to generate new VDPV outbreaks elsewhere (53, 57).
As with any model, this study had some limitations. Our analysis did not consider all the costs. For example, we did not estimate the expected cost of an outbreak; doing so would entail the generation of values for the risks of political re-emergence. However, one would anticipate that the costs of responding to an outbreak would include a mass immunization response, increased surveillance and case investigation, training of responders, and replenishing the stockpile. The management of these risks and the expected costs and benefits will ultimately drive policy decisions. The cost of cold-chain services, human resources, administration cost of human resources, and administration of VAPP surveillance, treatment, follow-up, and compensation are difficult to accurately estimate. Thus, we hypothesized that they would remain identical across the four PV immunization schedules. However, this study provided better estimations based on previous findings. Further studies will need to consider the full set of prospective vaccines and other policy options, and the impacts of immunization policy decisions on expected future polio endgame health and economic costs.
Conclusion
This study found that ICERVAPP and ICERDALY were substantially high for S3 (four-sIPV) and S4 (replacement of self-funded sIPV based on one-sIPV-three-bOPV). Two-sIPV-two-bOPV had a cost-effectiveness advantage, whereas S2/S3/S4 had no cost-effectiveness advantage, which might be attributable to the difference in the cost between sIPV and OPV and rare VAPP cases. However, they may be cost-effective by decreasing sIPV prices. Considering the risk of VAPP in the four-tOPV schedule, IPV-containing schedules may be a feasible strategy to accelerate polio eradication efforts in China. In addition, further evaluation of the long-term effectiveness and benefits of IPV-containing schedules in China is warranted.
Data availability statement
The original contributions presented in the study are included in the article/Supplementary material, further inquiries can be directed to the corresponding author.
Author contributions
YX and YL: formal analysis and methodology. JW, XC, XuZ, and WJ: investigation. YL: project administration and writing—review and editing. YX: software and writing—original draft. JD, XiZ, and WG: validation. All authors contributed to the article and approved the submitted version.
Funding
This study was funded by the Zhejiang Provincial Basic Public Welfare Research Projects (Grant No. LGF18H260002) and Hangzhou Research Projects for Agricultural and Social Development (Grant No. 20180533B94).
Conflict of interest
The authors declare that the research was conducted in the absence of any commercial or financial relationships that could be construed as a potential conflict of interest.
Publisher's note
All claims expressed in this article are solely those of the authors and do not necessarily represent those of their affiliated organizations, or those of the publisher, the editors and the reviewers. Any product that may be evaluated in this article, or claim that may be made by its manufacturer, is not guaranteed or endorsed by the publisher.
Supplementary material
The Supplementary Material for this article can be found online at: https://www.frontiersin.org/articles/10.3389/fpubh.2022.990042/full#supplementary-material
References
1. Liu JH, Tong GM. Progress of poliomyelitis vaccine research. Prog Microbiol Immunol. (2011) 39:72–4. doi: 10.3969/j.issn.1005-5673.2011.02.017
2. Kew OM, Sutter RW, De Gourville EM, Dowdle WR, Pallansch MA. Vaccine-derived polioviruses and the endgame strategy for global polio eradication. Annu Rev Microbiol. (2005) 59:587–635. doi: 10.1146/annurev.micro.58.030603.123625
3. Polio Global Eradication Initiative,. Polio Eradication Endgame Strategic Plan. Polio Global Eradication Initiative. Elektrobit/OL (2013–2018). Available online at: http://www.Polioeradication.org/Portals/0/Document/Resources/StrategyWork/Peesp_ES_EN_A4.pdf (accessed May 8, 2013).
7. WHO. Expanded program on immunization. Poliomyelitis in 1987, 1988 and 1989-Part. WER. (1991) 66:49–54.
8. Aylward B, Tangermann R. The global polio eradication initiative lessons learned and prospects for success. Vaccine. (2011) 29 (Suppl 14):D80–5. doi: 10.1016/j.vaccine.2011.10.005
9. Liang X, Zhang Y, Xu W, Wen N, Zuo S, Lee LA, et al. An outbreak of poliomyelitis caused by typeIvaccine-derived poliovirus in China. J Infect Dis. (2006) 194:545–51. doi: 10.1086/506359
10. Polio Eradication,. WHO Global Action Plan to Minimize Poliovirus Facility-Associated Risk After Type-Specific Eradication of Wild Polioviruses Sequential Cessation of OPV Use. Geneva: World Health Organization (2014). Available online at: http://www.polioeradication.org/Portals/0/Document/Resources/PostEradication/GAPIII_2014.pdf (accessed July 2015)
11. Lane EJ. The world's first inactivated vaccine for the Sabin strain of poliomyelitis was approved for marketing. Eval Anal Drug. (2015) 15:18.
12. WHO. Meeting of the strategic advisory group of experts on immunization, April 2016– conclusions and recommendations. WER. (2016) 91:265–84.
13. Liao G, Li R, Li C, Sun M, Jiang S, Li Y, et al. Phase 3 trial of a sabin strain-based inactivated poliovirus vaccine. J Infect Dis. (2016) 214:1728–34. doi: 10.1093/infdis/jiw433
14. *Qiu J, Yang Y, Huang L, Wang L, Jiang Z, Gong J, et al. Immunogenicity and safety evaluation of bivalent types 1 and 3 oral poliovirus vaccine by comparing different poliomyelitis vaccination schedules in China: a randomized controlled non-inferiority clinical trial. Hum Vaccin Immunother. (2017) 13:1–10. doi: 10.1080/21645515.2017.1288769
15. *Drummond MF, Sculpher MJ, Torrance GW, O'Brien BJ, Stoddart GL. Methods for the Economic Evaluation of Health Care Programmes. Oxford: Oxford University Press (1997).
16. *Zhang N, Shi XF, Wu J. The application of incremental cost-effectiveness ratio to health technology assessment. Chin J Health Policy. (2012) 5:64–8. doi: 10.3969/j.issn.1674-2982.2012.02.012
17. *Sangrujee N, Cáceres VM, Cochi SL. Cost analysis of post-polio certification immunization policies. Bull World Health Organ. (2004) 82:9–15.
18. *Tao LN, Liu JC. Evaluation method of cold chain volume based on vaccine volume and inoculation number. Chin J Vaccine Immun. (2014) 20:454–8.
19. *WHO. User Guide for WHO Vaccine Volume Calculator [EB/OL]. WHO. Available online at: http://www.who.int/immunization/programmes_systems/supply_chain/resources/tools/en/index4.html
20. *World Health Organization. The world health organization (WHO) about the use of oral polio vaccine introduced inactivated polio inactivated opinion. Epidemiol Wkly. Q(2003) 78.
21. *Platt LR, Estivariz CF, Sutter RW. Vaccine-Associated paralytic poliomyelitis: a review of the epidemiology and estimation of the global burden. J Infect Dis. (2014) 210 (Suppl. 1):S380–9. doi: 10.1093/infdis/jiu184
22. *Liu Y, Wang J, Liu S, Du J, Wang L, Gu W, et al. Introduction of inactivated poliovirus vaccine leading into the polio eradication endgame strategic plan; Hangzhou, China, 2010-2014. Vaccine. (2017) 35:1281–6. doi: 10.1016/j.vaccine.2017.01.034
23. *Ulla KG, Lindsay, Barry D. The cost-effectiveness of alternative polio immunizationpolicies in South Africa. Vaccine. (2006) 24:5670–8. doi: 10.1016/j.vaccine.2006.05.032
24. *Li RP, Xu AQ. Strategic Analysis and Cost Estimation of Using SABin-IPV Instead of OPV in The Late Stage of Poliomyelitis Eradication in China. Jinan: Shandong University (2007).
25. *Dai F, Zhang RZ. Economic burden of poliomyelitis. Zhonghua Liu Xing Bing Xue Za Zhi. (1996) 17:169–71.
26. *Cheng XM. In the Field of Medical and Health Care Cost—Benefit Analysis. Shanghai: Shanghai Medical University Press (1994).
27. *Alleman MM, Jorba J, Henderson E, Diop OM, Shaukat S, Traoré MA, et al. Update on vaccine-derived poliovirus outbreaks - worldwide, January 2020-June 2021. MMWR Morb Mortal Wkly Rep. (2021) 70:1691–9. doi: 10.15585/mmwr.mm7049a1
28. *Zhao H, Ma X, Tang H, Zhang Y, Chen N, Kaisaier W, et al. Circulation of type 2 vaccine-derived poliovirus in China in 2018-2019. Open Forum Infect Dis. (2021) 8:ofab535. doi: 10.1093/ofid/ofab535
29. *Zhang Y, Zhu SL, Yan DM, Wang DY, Li XL, Zhu H, et al. Type I vaccine-derived polioviruses in China from 1995 to 2019. Biosaf Health. (2019) 3:155–8. doi: 10.1016/j.bsheal.2019.12.002
30. *Thompson KM, Tebbens RJ. Current polio global eradication and control policy options: perspectives from modeling and prerequisites for oral poliovirus vaccine cessation. Expert Rev Vaccines. (2012) 11:449–59. doi: 10.1586/erv.11.195
31. *Wen N, Fan CX, Yan DM, Zhu SL, Wang HB, Liu B, et al. Epidemiological characteristics of vaccine-derived poliovirus and its cases in China from 2001 to 2013. Chin J Vaccine Immun. (2014) 20:210–5.
32. *WHO. WHO Guide to Generalized Cost-Effectiveness Analysis [EB/OL]. WHO. Available online at: http://www.who.int/choice/publications/p_2003_generalised_cea.PDF
33. *Yang LX. Incremental Cost Study of Inactivated Poliovirus Vaccine into National Immunization Program. Beijing: Chinese Center for Disease Control and Prevention (2015)
34. *Sartori AM, Vicentine MP, Gryninger LC, Soárez PC, Novaes HM. Polio inactivated vaccine costs into routine childhood immunization in Brazil. Rev Saude Publica. (2015) 49:8. doi: 10.1590/S0034-8910.2015049005492
35. *Khan MM, Sharma S, Tripathi B, Alvarez FP. Budget impact of polio immunization strategy for India: introduction of one dose of inactivated poliomyelitis vaccine and reductions in supplemental polio immunization. Public Health. (2017) 142:31–8. doi: 10.1016/j.puhe.2016.10.016
36. *Domingues CM, de Fátima Pereira S, Cunha Marreiros AC, Menezes N, Flannery B. Introduction of sequential inactivated polio vaccine-oral polio vaccine schedule for routine infant immunization in Brazil's national immunization program. J Infect Dis. (2014) 210 (Suppl. S1):S143–51. doi: 10.1093/infdis/jit588
37. *Duintjer Tebbens RJ, Pallansch MA, Cochi SL, Wassilak SG, Thompson KM. An economic analysis of poliovirus risk management policy options for 2013-2052. BMC Infect Dis. (2015) 15:389. doi: 10.1186/s12879-015-1112-8
38. †World Health Organization. World schedule as of . WHO (2013). Available online at: http://www.who.int/immunization/monitoring_surveillance/data/en/ (accessed May 19, 2014).
39. †Thompson KM, Duintjer Tebbens RJ. National choices related to inactivated poliovirus vaccine, innovation, and the end game of global polio eradication. Exp Rev Vaccines. (2014) 13:221–34. doi: 10.1586/14760584.2014.864563
40. †Tebbens RJD, Pallansch MA, Cochi SL, Wassilak SG, Linkins J, Sutter RW, et al. Economic analysis of the global polio eradication initiative. Vaccine. (2010) 29:334–43. doi: 10.1016/j.vaccine.2010.10.026
41. †World Health Organization Department Immunization. Principles and Considerations for Adding a Vaccine to a National Immunization Programme: From Decision to Implementation and Monitoring. Geneva: WHO (2014).
42. †Alvis N, De LHF, Narváez J. Economic impact of introducing the injectable inactivated polio vaccine in Colombia. Rev Panam Salud Publica. (2010) 27:352–9. doi: 10.1590/S1020-49892010000500005
43. †Ren J, Maimaiti H, Sun X, Huang Z, Liu J, Yang J, et al. Cost-Effectiveness of three poliovirus immunization schedules in Shanghai, China. Vaccines. (2021) 9:1062. doi: 10.3390/vaccines9101062
44. †Ivanova OE, Eremeeva TP, Morozova NS. Vaccine-associated paralytic poliomyelitis in the Russian federation in 1998–2014. Int J Infect Dis. (2018) 76:64–9. doi: 10.1016/j.ijid.2018.08.017
45. †Alexander LN, Seward JF, Santibanez TA, Pallansch MA, Kew OM, Prevots DR, et al. Vaccine policy changes and epidemiology of poliomyelitis in the United States. JAMA. (2004) 292:1696–701. doi: 10.1001/jama.292.14.1696
46. †Miller MA, Sutter RW, Strebel PM, Hadler SC. Cost-effectiveness of incorporating inactivated poliovirus vaccine into the routine childhood immunization schedule. JAMA. (1996) 276:967–71. doi: 10.1001/jama.276.12.967
47. †Circulating Vaccine-Derived Poliovirus (cVDPV). Available online at: https://polioeradication.org/wp-content/uploads/2020/12/20201118_cVDPV2_Global_Update_EN.pdf (accessed September 21, 2021).
48. †Tucker AW, Isaacs D, Burgess M. Cost-effectiveness analysis of changing from live oral poliovirus vaccine to inactivated poliovirus vaccine in Australia. Aust N Z J Public Health. (2001) 25:411–6. doi: 10.1111/j.1467-842X.2001.tb00648.x
49. †Duintjer TRJ, Pallansch MA, Chumakov KM, Halsey NA, Hovi T, Minor PD, et al. Expert review on poliovirus immunity and transmission. Risk Anal. (2013) 33:544–605. doi: 10.1111/j.1539-6924.2012.01864.x
50. †Anis E, Kopel E, Singer SR, Kaliner E, Moerman L, Moran-Gilad J, et al. Insidious reintroduction of wild poliovirus into Israel, 2013. Euro Surveill. (2013) 18:20586. doi: 10.2807/1560-7917.ES2013.18.38.20586
51. †Kalkowska DA, Duintjer Tebbens RJ, Grotto I, Shulman LM, Anis E, Wassilak SG, et al. Modeling options to manage type 1 wild poliovirus imported into Israel in 2013. J Infect Dis. (2015) 211:1800–12. doi: 10.1093/infdis/jiu674
52. †Wahjuhono G, Revolusiana, Widhiastuti D, Sundoro J, Mardani T, Ratih WU, et al. Switch from oral to inactivated poliovirus vaccine in Yogyakarta Province, Indonesia: summary of coverage, immunity, and environmentalsurveillance. J Infect Dis. (2014) 210 (Suppl 1):S347–52. doi: 10.1093/infdis/jiu060
53. †Thompson KM, Duintjer TRJ, Pallansch MA, Kew OM, Sutter RW, Aylward RB, et al. The risks, costs, and benefits of possible future global policies for managing polioviruses. Am J Public Health. (2008) 98:1322–30. doi: 10.2105/AJPH.2007.122192
54. †Huang ZY, Hu JY, Sun XD, Yang JP. Analysis of poliomyelitis eradication and recommendations for immunization strategies. J Microbes Infect. (2020) 15:337–44.
55. †WHO. Polio vaccines: WHO position paper, March 2016—recommendations. Vaccine. (2017) 35:1197–9. doi: 10.1016/j.vaccine.2016.11.017
56. †Nasir UN, Bandyopadhyay AS, Montagnani F, Akite JE, Mungu EB, Uche IV, et al. Polio elimination in Nigeria: a review. Hum. Vaccines Immunother. (2015) 12:658–63. doi: 10.1080/21645515.2015.1088617
57. †Thompson KM, Duintjer Tebbens RJ. Eradication versus control for poliomyelitis: an economic analysis. Lancet. (2007) 369:1363–71. doi: 10.1016/S0140-6736(07)60532-7
Keywords: cost-effectiveness, dose, IPV, immunization schedules, Sabin strain
Citation: Xu Y, Liu Y, Wang J, Che X, Du J, Zhang X, Gu W, Zhang X and Jiang W (2022) Cost-effectiveness of various immunization schedules with inactivated Sabin strain polio vaccine in Hangzhou, China. Front. Public Health 10:990042. doi: 10.3389/fpubh.2022.990042
Received: 09 July 2022; Accepted: 24 August 2022;
Published: 23 September 2022.
Edited by:
Jhon Carlos Castaño, University of Quindío, ColombiaReviewed by:
Li Shi, Chinese Academy of Medical Sciences and Peking Union Medical College, ChinaZhuoying Huang, Shanghai Municipal Center for Disease Control and Prevention (SCDC), China
Konstantin Chumakov, Office of Vaccines Research and Review, Food and Drug Administration, United States
Copyright © 2022 Xu, Liu, Wang, Che, Du, Zhang, Gu, Zhang and Jiang. This is an open-access article distributed under the terms of the Creative Commons Attribution License (CC BY). The use, distribution or reproduction in other forums is permitted, provided the original author(s) and the copyright owner(s) are credited and that the original publication in this journal is cited, in accordance with accepted academic practice. No use, distribution or reproduction is permitted which does not comply with these terms.
*Correspondence: Yan Liu, smileforever81@126.com