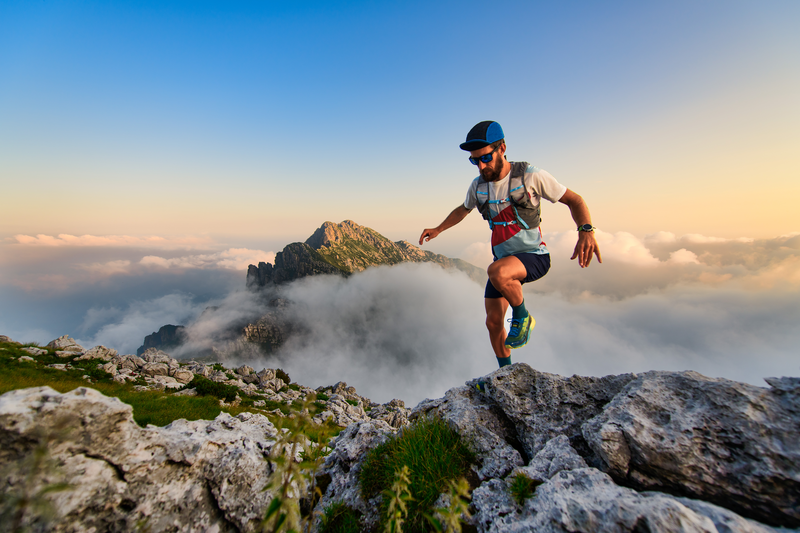
94% of researchers rate our articles as excellent or good
Learn more about the work of our research integrity team to safeguard the quality of each article we publish.
Find out more
ORIGINAL RESEARCH article
Front. Public Health , 23 September 2022
Sec. Infectious Diseases – Surveillance, Prevention and Treatment
Volume 10 - 2022 | https://doi.org/10.3389/fpubh.2022.964741
This article is part of the Research Topic The One Health Approach in the Context of Public Health View all 12 articles
Arisaema jacquemontii Blume is a highly medicinal and poisonous plant belong to the family Araceae. It is used to treat several deadly diseases, including viral infections. It has antioxidant, anti-cancerous, antimalarial, anti-vermicidal, and antiviral activities. Therefore, five parts of the Arisaema jacquemontii Blume plant, such as leaf, seed, stem, pulp, and rhizome extract, were evaluated for metabolic and in silico characterization of probable compounds using gas chromatography-mass spectrometry (GC-MS) analysis. A total of 22 compounds were isolated from the methanolic extracts of A. jacquemontii Blume. A selected antiviral COVID-19 protein i.e., protease (6LU7) was docked against the obtained compounds. Different affinities were obtained through various compounds. The best results were shown by three different compounds identified in the rhizome. The maximum binding affinity of these compounds is 8.1 kJ/mol. Molecular docking (MD) indicate that these molecules have the highest binding energies and hydrogen bonding interactions. The binding mode of interaction was discovered to be reasonably effective for counteracting the SARS virus COVID-19. The findings of this study could be extremely useful in the development of more phytochemical-based COVID-19 therapeutics.
Phytochemistry outlines the morphology of a large number of secondary metabolites. Many active ingredients have been discovered and isolated from different parts of plant species, including carotenoids, sterols, aliphatic compounds, monoterpenes and sesquiterpenes, triterpenoids, and other miscellaneous chemicals (1, 2). More than 4,000 phytochemicals have been described and categorized (3). The plant-originated chemicals contain secondary metabolites. These are secreted against when different calamities are faced (4), for example, antimicrobial effects, antioxidant activities, a decrease in platelets, detoxification of enzymes for modulation, anti-cancerous properties, and hormonal modulation (5, 6). Medicinal plants play an essential role in the provision of various phytochemicals. The era of modern research started in the early 19th century after herbal preparation. The first isolated active alkaloids were morphine, quinine, and strychnine (7). The primary emphasis was made on plant-derived drugs with the tremendous development of synthetic pharmaceutical chemistry and microbial fermentation (8–12).
Metabolomics is the large-scale study of small molecules known as metabolites within cells, bio-fluids, tissues, or organisms. These molecules and their interactions within the biological system are collectively known as metabolomes (13–16). These biomolecules provide biologically relevant endpoints of metabolic processes focusing on the products of interactions between gene expression, protein expression, and cellular environment (17, 18). Metabolomics is the most modern omics technology in which the patterns are identified to function and balance the metabolomic changes observed in their pathways (19). Metabolomics is the most comprehensive analysis used to study the diverse metabolites throughout the globe. These are present in different cells of the organisms, vastly expanded to fingerprinting and profiling metabolites through their selected and recognized markers (20). Metabolomics is the most fundamental classical and biological technique that quickly reflects the genotype (21–26). Metabolomics provides information about the qualitative and quantitative data and exhibits a compound's point and specific time. There are more than 200,000 metabolites extracted from plants (27). In the post-genomic era, 30–50% of the sequenced data was not interpreted correctly. Metabolomics is one of the most crucial areas contributing much toward the prokaryotic genome elucidation (28, 29). While out of 1–5 gene functions, only one gene's functions seem to be understood and illustrated in the bacterial genome, such as E. coli has an unknown sequence (30). These sequences helped to find out the functional and non-functional genes.
The most common technique for measuring metabolism is mass spectrometry and nuclear magnetic resonance (NMR) spectroscopy (31, 32). These two approaches easily observe different compounds from the plant at various stages. Gas chromatography-mass spectrometry is the oldest technique and the most sensitive, robotic, intensive, and developed technique mainly used to identify the primary metabolic compounds in mass spectrometry, such as carbohydrates, lipids, amino acids, fatty acids, and organic acids (33). In addition, molecular docking is a molecular modeling approach in which we evaluate the interaction of two molecules and anticipate how a protein interacts with a tiny molecule called a ligand, and the modeling technique is called molecular docking. In this developing world of complexity, the area of rational drug design has played a critical role in the development of novel medications (34). At the same time, selecting the biomolecule of interest is the most essential and critical step in rational drug design (35).
A. jacquemontii Blume is a popular Himalayan medicinal plant used to treat a variety of infections in traditional medicine (36–43). It is used as a foodstuff, an antidote for snakebite, and a therapy for respiratory infections, eczema, and rashes (44–49). It has anti-proliferative, anticonvulsant, anti-insect, and antiviral properties (50). It was hypothesized that the A. jacquemontii Blume plant possesses specific compounds that can be used as an antiviral. Therefore, this study was carried out to isolate and identify compounds from A. jaquemontii Blume with antiviral activity and combating ability against COVID-19 protein (6LU7). It will also contribute to the sustainable development goals by maintaining life on land and supporting the consumption and production of A. jacquemontii Blume, which will benefit the community medicinally and increase economic growth by reducing poverty.
The plant samples were collected from the Western Himalaya Mountains at an elevation of 4,500 m (51–53). All plant parts, such as rhizome stem and leaves, were washed properly with distilled water, while in the case of seed, the pulp was separated and samples were kept at room temperature (25°C) for proper drying. Some samples were frozen at −80°C for further use.
The A. jacquemontii Blume samples were weighed and shade dried for 20 days. After drying, different parts of A. jacquemontii Blume, such as rhizome, seed, pulp, leaves, and stem, were thoroughly dried and then ground with the help of a grinder into powder form. The dried samples were weighed, mixed with ethanol at 1 g:10 ml, added methanol, and kept in an electric shaker at 200 rpm (round per minute) for 48 h. After two successive days of shaking, the solution was filtered with the help of Whatmann's No. 1 filter paper. The obtained filtrate from the solution was poured into the Petri plate and placed at room temperature for 1 day to evaporate methanol or chloroform (54). A thin extract layer was obtained in the Petri plate collected in the Eppendorf for future use. After drying and measuring, layer extractions were collected through a spatula in the Eppendorf. This procedure was repeated three times, and the maximum extract was collected for experimentation. The extract was preserved in Eppendorf and used for further research purposes.
The extract prepared from different parts of the plant species was subjected to standard phytochemical analysis so that various chemical compounds such as alkaloids, flavonoids, tannins, and saponins could be detected (54).
Methanol and chloroform extracts of different parts of the A. jacquemontii Blume were carried out for chromatographic analysis to confirm the presence of phytoconstituents in their active solvent extracts.
GC-MS of the plant samples was performed using a PerkinElmer GC Clarus 500 system and chromatograph coupled to a mass spectrometer equipped with an Elite-I, fused silica capillary column (30 mm 90.25 mm ID 91 IMdf, consisting of 100% dimethyl polysiloxane). The sample was dissolved in n-hexane and GC-MS identification was operated in electron impact mode with an ionization energy of 70 eV. The National Institute of Standard and Technology (NIST) library database was used for the interpretation of the compounds detected on a mass spectrum (GC-MS) through which the weighed, molecular formula and structural formula of the samples were determined (55).
Molecular docking is a type of bioinformatics modeling where interactions of two or more molecules give us a stable product. Phytocompounds separate from the highly medicinal plant A. jacquemontii Blume using GC-MS analyses, docked against the targeted coronavirus protein to detect the compounds binding with the active site of the targeted 6LU7 protein at chain C (56). This protein is an essential enzyme in the Coronavirus and plays a crucial role in mediating viral replication in the transcription, making it an attractive drug target against SARS-COVID-2. This chain is considered the binding site on the protein for ligand (57). Ligand structures in SDF format were downloaded from PubChem and converted into PDB format through PY mole software. The protein was downloaded directly in PDB format. This format was used to carry out ligand–protein interactions in AutoDock 4.2.6. The protein was prepared for docking by removing water, adding hydrogen, adding kollarman charges, and computing atomic solvation parameters. The protein–ligand complex was designed by creating a grid box in AutoDock 4.2.6 in which all the x, y, and z dimensions are 60, 60, and 60, respectively, with a spacing of 0.5 Å. We have used blind docking to cover the overall protein and grid box. Results in the form of a summary were obtained in the grid.txt file in the respective folder. In this way, protein–ligand interactions were further analyzed by command prompt software to check the affinity of their bonding by running a protein–ligand summary file (58). The LIG PLOT software was used to analyze that protein–ligand complex in three-dimensional structure, their number of hydrogen bonds, the name of that hydrogen bond, and affinity.
A total of 22 compounds were recorded from A. jacquemontii Blume through GC-MS (Table 1). Out of which a leaves extract contains one compound, i.e., 2-fluoro-6-(trifluoromethyl)-acetophenone, pulp extract contains three compounds like triallymethylsilane, propane-nitrile, 3-(methylthio), octane, 1-(propylthio) and seed extract possess three compounds such as 2, 5-dimethyl-3-isopropylpyrazine, phenol, 2, 5-bis (1,1-dimethyl ethyl)-pentadecanoic acid methyl ester (Figures 1–5).
Table 1. GC-MS analysis and characterization of different compounds in various parts of A. jacquemontii Blume.
The same number of compounds such as ortho-methoxyacetophenone, 4'-diethylaminoacetanilide, nonanoic acid, methyl ester, and 2, 5-dimethylcyclohexanol were also extracted from the stem. A total of 11 compounds such as beta, methyl xyloside, nonanoic acid, 3-decanol, phenol, 2, 5-bis (1,1-dimethyl ethyl)-, benzene, 2-methyl-1, 3, 5-trimethyl, nonanedioic acid, dimethyl ester, tridecanoic acid, methyl ester, n-hexadecanoic acid, octadecanoic acid, methyl ester, p-hexyloxy nitrobenzene, and gamma-sitosterol were observed in the rhizome of A. jacquemontii Blume (Figure 3). Different peaks along with their retention time were observed along with the given names of the compounds (Table 1). According to the literature, only five of these 22 compounds are known for their antiviral activities.
The GC-MS extract of different parts of A. jacquemontii Blume was AutoDocked against antiviral protein 6LU7. Among all, compounds obtained from rhizome extract yield the highest binding interaction affinity. Ligand–protein interaction was stronger in those compounds (Table 2). The 6LU7 consists of 3C-like protease (3CLpro) and spike protein, a striking goal for medical production in COVID-19. Of all these three chains, the C chain is the binding site for the ligand on protein. This protein is further used in binding interaction with the ligand through which binding affinity can be analyzed in Auto Dock vina (Figure 6).
Table 2. Docking results of different parts of A. jacquemontii Blume along with their binding affinity.
The recorded 22 compounds have different binding affinities for the selected protein. These compounds were considered as ligands against interaction with proteins in molecular docking. All the ligands, their structural formula, and their CID number obtained through PubChem and binding affinity calculation are explained in Table 2. Three-dimensional visualization of this entire compound's structure illustrates the number of hydrogen bonds, the name of the hydrogen bond, and hydrophobic interactions with other amino acids. The binding affinities of all the compounds are 2-fluoro-6-(trifluoromethyl)– acetophenone has −7.4 kJ/mol affinity value having only one (1) hydrogen bond O-NH2 having a distance of 2.89 Å and hydrophobic interactions were Phe 219(A), Trp 218(A), Leu 271(A), Gly 275(A), Glu 270(A), and Asn 277(A). Propanenitrile, 3-(methylthio) has −3.4 kJ/mol ligand–protein affinity having two hydrogen bonds N-N, N-OG1 with bond distance 3.20 Å and 2.96 Å, respectively, with hydrophobic interactions Asn 151 (A), Phe 294 (A), Thr 292 (A), and Gln 110 (A). Octane, 1-(propylthio) has a bonding affinity of −5.1 kJ/mol with no hydrogen bond present in it, having hydrophobic interactions as His 246 (A), Val 202 (A), Gln 110(A), Pro 293 (A), Ile 249 (A), and Phe 294 (A), and in the same way, no hydrogen bond was present in 2, 5-dimethyl-3-isopropyl-pyrazine and seven different types of hydrophobic interactions were observed. In the same way, all the compounds showed different numbers and types of hydrogen bonds to varying distances, with quite different binding affinities. The highest binding affinity was −8.1 kJ/mol that was demonstrated in two compounds of rhizome such as p-Hexyloxy nitrobenzene and Gamma-Sitosterol, while no hydrogen bond was present in the first compound and the second one had two hydrogen bonds that were O-O and O-O, with bond distances of 3.03 Å and 3.09 Å, respectively (Supplementary Table 1, Table 3).
Table 3. Summary of docking results of 22 compounds, functional residues involved in hydrophobic interactions, hydrogen bonds, and their binding affinities against 6LU7 target protein.
The Arisaema genus has been previously analyzed to have antibacterial, antioxidant, and cancer-fighting properties. It has a physiological effect on the human respiratory syncytial virus. The Herpes simplex virus was also examined in the Arisaema genus (59). The Japanese encephalitis (JE) condition was caused by the Japanese encephalitis virus (JEV) and affects millions worldwide. The genus Arisaema was used to cure this deadly virus, by which nearly 70,000 people per year are affected (60). A methanolic extract of the roots of A. jacquemontii Blume was proven to have antiviral properties (17). The GC-MS results of A. jacquemontii Blume showed the presence of 22 compounds in it. All the compounds have a good binding affinity as shown after molecular docking. Only one compound trimethylsilane revealed no docking results because Si is a sensitive case in docking. While, according to Kant et al. (59), only three compounds were observed from Arisaema that are 2-hydroxydiplopterol, 30-nor-lanost-5-ene-3β-ol and 30-nor-lanost-5-ene-3-one and out of all these three compounds only one compound showed docking results with−3.83 binding affinity and no results were shown by rest of the two compounds. GC-MS analysis of methanolic extracts of Salacia oblonga roots revealed the presence of compounds such as n-hexadecanoic acid (11.94), 6-octadecanoic acid (2.24), hexadecanoic acid, 3-hydroxy methyl ester (1.84), n-methoxy-n-methylacetamide (17.38), phytol (0.58), 1, 2 benzene dicarboxylic acid (61). While GC-MS identified the same chemicals in A. jacquemontii Blume with variable binding affinity, indicating that they might be used to treat COVID-19.
The study could add to the expanding body of evidence that the Arisaema genus has antiviral properties, owing to phytoconstituents produced from these medicinal plants' ability to inhibit viral mediators such as HSV. Molecular docking is one of the most effective approaches for creating novel ligands for proteins with specified functions, and it is widely utilized in drug development. The antiviral activity of Arisaema, a plant from the Araceae family, was tested by docking phytochemical compounds with the 6LU7 protein to see if they might attach to the enzyme's active site and prevent it from working. Antiviral proteins were docked against these 22 compounds to see how much binding impact they had on finding a new drug or inhibitor to limit or decrease disease infection. Each molecule was docked using topoisomerase IV type B and spectroscopically identified using GC–MS. Swiss PDB Viewer identified the active site additives as Leu 209, Thr 207, Arg 176, Val 160, Leu 135, Ile 134, Asp 121, Pro 119, Met 118, Gly 117, Arg 116, Asp 113, Glu 90, Asp 89, Val 88, Ser 87, Asn 86, Asp 85, and Val 83 and used −8.5 kcal/mol energy (62–64). Similarly, 8.1 kJ/mol affinities were found in two A. jacquemontii Blume rhizome's compounds, namely, p-hexyloxy nitrobenzene and gamma-sitosterol, whereas the first compound had no hydrogen bonds and the second had two hydrogen bonds, O-O and O-O with bond distances of 3.03 A and 3.09 A, respectively (Table 3).
Docking analysis revealed that nelfinavir forms H-bonds with the 6LU7 amino acids Glu 166, Gln 189, and Gln 192 obtained from different medicinal plants in the tropical region (65). However, nonanoic acid, methyl ester, also forms a hydrogen bond with Gln 110, and this compound was obtained from the methanolic extract of the stem of A. jacquemontii Blume. The hydrophobic interactions obtained through ligand–protein interaction are Phe 294 (A), Thr 111 (A) Asn 151 (A), Ile 106 (A), Val 104 (A), Arg 105 (A), and Asp 295 (A). The amino acid residue interactions at the active site of COVID-19 Mpro are predicted to be mediated by hydroxy groups (-OH), ketone groups (=O), and ether groups (-O-) in luteolin and kaempferol compounds. The same bonding groups were observed in Mpro protein with different compounds of A. jacquemontii Blume such as 2,5-Dimethylcyclohexanol, beta-methyl xyloside, 3-decanol, and gamma-sitosterol with binding affinities −5.4, −6.3, −5.8, and −8.11, respectively (65).
COVID-19 has stressed our healthcare system and underlined the essential role of laboratory medicine and herbal medicine in tackling the spread of new transmissible agents. Networks of COVID-19 laboratories have been set up to support the specific needs of citizens and patients, and they will continue to be fundamental during the restarting of social and work activities (66, 67). Plants, therefore, play an important role in its cure because different herbal teas were considered effective in treating this deadly disease (68, 69). This study helped us to discover an inhibitor against protease so that it can be helpful in the cure of COVID-19. To our knowledge, there is currently no study on the COVID-19 infection in terms of A. jaquemontii, although scientists are working on it. After comprehensively reviewing the literature and implementing our own research, we observed that A. jacquemontii Blume has a high potential for combating COVID-19.
This study satisfies a number of the sustainable development goals (SDGs) by playing a key role in fulfilling these goals. It helped us to maintain life on land. Because this is a medicinal plant and is used to treat several diseases not only in humans but also in animals. After knowing the importance of this medically important species, our utmost desire should be to support the local community in its consumption and production so that it can be further utilized to treat many disorders. As its consumption and production were supported by the local community, it opened up a number of livelihoods for the poor, which will provide income resources for the community group.
A total of 22 compounds were extracted from A. jaquemontii from five different parts of the plant, including seed, stem, pulp, leaf, and rhizome. The antiviral activity of these 22 potential drugs was studied via in silico characterization. The 6LU7 Mpro protein has been identified as a key and effective target for inhibiting the new COVID-19. Of all these chemicals, two had the highest binding affinity of −8.1 kJ/mol. Based on these selected compounds' intermolecular interaction and binding energy, it has also been concluded that these chemical compounds could be a potential drug for SARS-CoV-2 infection. Because there is no experimental technique in in silico characterization using molecular docking, it explains the potential of isolated chemicals through different software. These isolated chemicals have such high binding energy values that they can be further processed for experimental purposes, which will be another study and more support for this effort. It could be an effective drug by blocking the replication in maturity of the virus in the host body.
These findings focus on bioactive phytochemicals synthetically modified to their structural motifs that could be potentially used against infectious diseases. This study's findings might be utilized to generate additional phytochemicals to be used against COVID-19 in the future. The docked compounds are recommended for in vitro and in vivo studies in the future to possibly overcome the infectious viral diseases of humans as well as animals.
The original contributions presented in the study are included in the article/Supplementary material, further inquiries can be directed to the corresponding author/s.
Conceptualization, methodology, validation, formal analysis, investigation, resources, data curation, and writing—original draft preparation: SS, SK, GM, AA, IK, and ZA. Writing—review and editing: SS, SK, GM, AA, IK, ZA, HH, JP, JY, and AR. Visualization: SS, SK, GM, AA, IK, ZA, and AR. Supervision: SK, GM and AR. Project administration: SK, GM, HH, JP, JY, and AR. All authors have read and agreed to the published version of the manuscript.
We are thankful to the indigenous community who initially identified the potential uses of this plant against viral diseases, especially respiratory diseases. We are also thankful to Mr. Jehan Sher Khan for helping in the collection of this plant from various mountains. Author acknowledge the Pakistan Academy of Sciences and URF QAU for partial funding to the experimental part of this research work. Dr Farman Ullah, Department of Chemistry, Quaid-i-Azam University Islamabad for facilitation in GCMS analyses.
The authors declare that the research was conducted in the absence of any commercial or financial relationships that could be construed as a potential conflict of interest.
All claims expressed in this article are solely those of the authors and do not necessarily represent those of their affiliated organizations, or those of the publisher, the editors and the reviewers. Any product that may be evaluated in this article, or claim that may be made by its manufacturer, is not guaranteed or endorsed by the publisher.
The Supplementary Material for this article can be found online at: https://www.frontiersin.org/articles/10.3389/fpubh.2022.964741/full#supplementary-material
Supplementary Table 1. Three dimensional images showing protein - Ligand complex, bond present, and different hydrophobic interactions.
1. Rather LJ, Mohammad F. Phytochemistry, biological activities and potential of annatto in natural colorant production for industrial applications–a review. J Adv Res. (2016) 7:499–514. doi: 10.1016/j.jare.2015.11.002
2. Yang B, Li Q, Cheng K, Fang J, Mustafa G, Pan J, et al. Proteomics and metabolomics reveal the mechanism underlying differential antioxidant activity among the organs of two base plants of Shiliang tea (Chimonanthus salicifolius and Chimonanthus zhejiangensis). Food Chem. (2022) 385:132698. doi: 10.1016/j.foodchem.2022.132698
3. Costa MA, Xia Z-Q, Davin LB, Lewis NG. Toward engineering the metabolic pathways of cancer-preventing lignans in cereal grains and other crops. In: Phytochemicals in Human Health Protection, Nutrition, and Plant Defense. Springer. (1999) p. 67–87. doi: 10.1007/978-1-4615-4689-4_4
4. Gryglewski RJ, Korbut R, Robak J. Swi?s JJBp. On the mechanism of antithrombotic action of flavonoids. Biochem Pharmacol. (1987) 36:317–22. doi: 10.1016/0006-2952(87)90288-7
5. Rao BNJAPJocn. Bioactive phytochemicals in Indian foods and their potential in health promotion and disease prevention. Asia Pac J Clin Nutr. (2003) 12:9–22.
6. Zhang Z, Cui F, Cao C, Wang Q, Zou Q. Single-cell RNA analysis reveals the potential risk of organ-specific cell types vulnerable to SARS-CoV-2 infections. Comput Biol Med. (2022) 140:105092. doi: 10.1016/j.compbiomed.2021.105092
7. Saxena M, Saxena J, Nema R, Singh D, Gupta AJJop. Phytochemistry Phytochemistry of Medicinal Plants. (2013) p. 1.
8. Akunyili DJASotNSoP. The Role of Regulation of Medicinal Plants and Phytomedicine in Socio-Economic Development. (2003) p. 1–7.
9. Li H, Wang F. Core-shell chitosan microsphere with antimicrobial and vascularized functions for promoting skin wound healing. Mater Des. (2021) 204:109683. doi: 10.1016/j.matdes.2021.109683
10. Liu G, Nie R, Liu Y, Mehmood A. Combined antimicrobial effect of bacteriocins with other hurdles of physicochemic and microbiome to prolong shelf life of food: a review. Sci Total Environ. (2022) 154058. doi: 10.1016/j.scitotenv.2022.154058
11. Wang Q, Sha H, Cao S, Zhao B, Wang G, Zheng P. Tourmaline enhanced methane yield via regulating microbial metabolic balance during anaerobic co-digestion of corn stover and cow manure. Bioresour Technol. (2022) 359:127470. doi: 10.1016/j.biortech.2022.127470
12. Yang Y, Dou Y, Wang B, Wang Y, Liang C, An S, et al. Increasing contribution of microbial residues to soil organic carbon in grassland restoration chronosequence. Soil Biology and Biochemistry. (2022) 170:108688. doi: 10.1016/j.soilbio.2022.108688
13. Fukusaki E, Kobayashi A. Plant metabolomics: potential for practical operation. J Biosci Bioeng. (2005) 100:347–54. doi: 10.1263/jbb.100.347
14. Dunn WB, Bailey NJ, Johnson HE. Measuring the metabolome: current analytical technologies. Analyst. (2005) 130:606–25. doi: 10.1039/b418288j
15. Villas-Boas SG, Nielsen J, Smedsgaard J, Hansen MA, Roessner-Tunali U. Metabolome Analysis: an Introduction. New Jersey, U.S.: John Wiley & Sons. (2007). doi: 10.1002/0470105518
16. Villas-Bôas SG, Mas S, Åkesson M, Smedsgaard J, Nielsen J. Mass spectrometry in metabolome analysis. Mass Spectrom Rev. (2005) 24:613–46. doi: 10.1002/mas.20032
17. Zamboni N, Saghatelian A. Patti GJ. Defining the metabolome: size, flux, and regulation. Mol Cell. (2015) 58:699–706. doi: 10.1016/j.molcel.2015.04.021
18. Chen F, Aqeel M, Maqsood MF, Khalid N, Irshad MK, Ibrahim M, et al. Mitigation of lead toxicity in Vigna radiata genotypes by silver nanoparticles. Environ Pollut. (2022) 308:119606. doi: 10.1016/j.envpol.2022.119606
19. Holmes E, Wilson ID, Nicholson JKJC. Metabolic phenotyping in health and disease. Cell. (2008) 134:714–7. doi: 10.1016/j.cell.2008.08.026
20. Okada T, Mochamad Afendi F, Altaf-Ul-Amin M, Takahashi H, Nakamura K. Kanaya SJCc-add. Metabolomics of medicinal plants: the importance of multivariate analysis of analytical chemistry data. Curr Comput Aided Drug Des. (2010) 6:179–96. doi: 10.2174/157340910791760055
21. Fiehn O, Kopka J, Dörmann P, Altmann T, Trethewey RN. Willmitzer LJNb. Metabolite profiling for plant functional genomics. Nat Biotechnol. (2000) 18:1157–61. doi: 10.1038/81137
22. Nikou T, Witt M, Stathopoulos P, Barsch A, Halabalaki M. Olive oil quality and authenticity assessment aspects employing FIA-MRMS and LC-Orbitrap MS metabolomic approaches. Front Public Health. (2020) 8:558226. doi: 10.3389/fpubh.2020.558226
23. Zhang A, Sun H, Wang P, Han Y, Wang X. Recent and potential developments of biofluid analyses in metabolomics. J Proteomics. (2012) 75:1079–88. doi: 10.1016/j.jprot.2011.10.027
24. Verpoorte R, Choi Y, Mustafa N, Kim H. Metabolomics: back to basics. Phytochemist Rev. (2008) 7:525–37. doi: 10.1007/s11101-008-9091-7
25. Böttcher T, Pitscheider M, Sieber SA. Natural products and their biological targets: proteomic and metabolomic labeling strategies. Angewandte Chemie Int Edition. (2010) 49:2680–98. doi: 10.1002/anie.200905352
26. Weckwerth W. Metabolomics: an integral technique in systems biology. Bioanalysis. (2010) 2:829–36. doi: 10.4155/bio.09.192
27. Weckwerth W, Fiehn OJ. Can we discover novel pathways using metabolomic analysis? Curr Opin Biotechnol. (2002) 13:156–60. doi: 10.1016/S0958-1669(02)00299-9
28. Siew N, Azaria Y, Fischer DJ. The ORFanage: an ORFan database. Nucleic Acids Res. (2004) 32:D281–D283. doi: 10.1093/nar/gkh116
29. Xu Q, Zeng Y, Tang W, Peng W, Xia T, Li Z, et al. Multi-task joint learning model for segmenting and classifying tongue images using a deep neural network. IEEE J Biomed Health Inform. (2020) 24:2481–9. doi: 10.1109/JBHI.2020.2986376
30. Liang P, Labedan B. Riley MJ. Physiological genomics of Escherichia coli protein families. Physiol Genomics. (2002) 9:15–26. doi: 10.1152/physiolgenomics.00086.2001
31. Dettmer K, Hammock BD. Metabolomics–a new exciting field within the” omics” sciences. Environ Health Perspect. (2004) 112:A396–7. doi: 10.1289/ehp.112-1241997
32. Li H, Peng R, Wang Z. On a diffusive susceptible-infected-susceptible epidemic model with mass action mechanism and birth-death effect: analysis, simulations, and comparison with other mechanisms. SIAM Journal on Applied Mathematics. (2018) 78:2129–53. doi: 10.1137/18M1167863
33. Gullberg J, Jonsson P, Nordström A, Sjöström M, Moritz TJAb. Design of experiments: an efficient strategy to identify factors influencing extraction and derivatization of Arabidopsis thaliana samples in metabolomic studies with gas chromatography/mass spectrometry. Anal Biochem. (2004) 331:283–95. doi: 10.1016/j.ab.2004.04.037
34. Liljefors T, Krogsgaard-Larsen P, Madsen U. Textbook of Drug Design and Discovery. London, UK. CRC Press. (2002).
35. Oshiro C, Bradley EK, Eksterowicz J, Evensen E, Lamb ML, Lanctot JK, et al. Performance of 3D-database molecular docking studies into homology models. J Med Chem. (2004) 47:764–7. doi: 10.1021/jm0300781
36. Khan SM, Page S, Ahmad H, Shaheen H, Ullah Z, Ahmad M, et al. Medicinal flora and ethnoecological knowledge in the Naran Valley, Western Himalaya, Pakistan. J Ethnobiol Ethnomed. (2013) 9:1–13. doi: 10.1186/1746-4269-9-4
37. Khan SM. Plant Communities and Vegetation Ecosystem Services in the Naran Valley, Western Himalaya. University of Leicester. (2012).
38. Ahmad H, Öztürk M, Ahmad W, Khan SM. Status of natural resources in the uplands of the Swat Valley Pakistan. In: Climate change impacts on high-altitude ecosystems. Springer. (2015). p. 49–98. doi: 10.1007/978-3-319-12859-7_2
39. Roshan R, Ahmed S, Hasan MM. Arisaema jacquemontii Blume (Araceae): A review of medicinal uses, phytochemistry and pharmacology. J Pharmacogn Phytochem. (2017) 6:429–32.
40. Mir AY, Yaqoob U, Hassan M, Bashir F, Zanit SB, Haq SM, et al. Ethnopharmacology and phenology of high-altitude medicinal plants in Kashmir, Northern Himalaya. Ethnobotany Res Appl. (2021) 22:1–15. doi: 10.32859/era.22.17.1-15
41. Shah A, Sarvat R, Shoaib S, Ayodele A, Nadeem M, Qureshi T, et al. An ethnobotanical survey of medicinal plants used for the treatment of snakebite and scorpion sting among the people of Namal valley, Mianwali district, Punjab, Pakistan. Appl Ecol Environ Res. (2018) 16:111–43. doi: 10.15666/aeer/1601_111143
42. Dutta A, Singh K, Singh B, Sharma YP, Bussmann RW. Documentation of veterinary practices from Gujjar and Bakarwal tribes of District Poonch, Jammu & Kashmir: A boon for animals from our ancestors. Ethnobotany Res Appl. (2021) 21:1–18. doi: 10.32859/era.21.45.1-18
43. Su F, Sun Y, Zhu W, Bai C, Zhang W, Luo Y, et al. Comprehensive review of research progress on the genus Arisaema: botany, uses, phytochemistry, pharmacology, toxicity and pharmacokinetics. J Ethnopharmacol. (2022) 285:114798. doi: 10.1016/j.jep.2021.114798
44. Singh K, Rup PJ, Saxena A, Khan RH, Ashraf MT, Kamboj SS, et al. tuber lectin from Arisaema helleborifolium Schott with anti-insect activity against melon fruit fly, Bactrocera cucurbitae (Coquillett) and anti-cancer effect on human cancer cell lines. Arch Biochem Biophys. (2006) 445:156–65. doi: 10.1016/j.abb.2005.10.021
45. Rahman SU, Ullah Z, Ali A, Aziz MA, Alam N, Sher H, et al. Traditional knowledge of medicinal flora among tribal communities of Buner Pakistan. Phytomedicine Plus. (2022) 2:100277. doi: 10.1016/j.phyplu.2022.100277
46. Bhattarai S, Chaudhary RP, Taylor RS. Wild edible plants used by the people of Manang district, central Nepal. Ecol Food Nutr. (2009) 48:1–20. doi: 10.1080/03670240802034996
47. Verma H, Lal V, Pant K. Evaluation of anxiolytic activity in four selected species of Arisaema. J Pharm Res. (2015) 9:569–72.
48. Ratha KK, Joshi GC, Rungsung W, Hazra J. Use pattern of high altitude medicinal plants by Bhotiya tribe of Niti valley, Uttarakhand. World J Pharm Pharm Sci. (2015) 4:1042–61.
49. Asmat S, Khan SM, Ahmad Z, Manan F, Noor R, Zaman IU. Role of Chitral Gol National Park in Maintaining and Conserving Plant Diversity of the Region. In: Biodiversity, Conservation and Sustainability in Asia. Springer. (2022) p. 199–217. doi: 10.1007/978-3-030-73943-0_12
50. Jeelani S, Khuroo MA, Razadan T. New triterpenoids from Arisaema jacquemontii. J Asian Nat Prod Res. (2010) 12:157–61. doi: 10.1080/10286020903505057
51. Khan W, Khan SM, Ahmad H, Ahmad Z, Page S. Vegetation mapping and multivariate approach to indicator species of a forest ecosystem: a case study from the Thandiani sub Forests Division (TsFD) in the Western Himalayas. Ecol Indic. (2016) 71:336–51. doi: 10.1016/j.ecolind.2016.06.059
52. Manan F, Khan SM, Ahmad Z, Kamran S, Haq ZU, Abid F, et al. Environmental determinants of plant associations and evaluation of the conservation status of Parrotiopsis jacquemontiana in Dir, the Hindu Kush Range of Mountains. Trop Ecol. (2020) 61:509–26. doi: 10.1007/s42965-020-00109-2
53. Nazakat S, Khan SM, Noor R, Arif M, Khalid N, Ahmad Z. Floral Composition, Sustainable Utilization, and Conservation of Important Medicinal Plants in the Ayubia National Park, Abbottabad, Khyber Pakhtunkhwa, Pakistan. In: Ethnobiology of Mountain Communities in Asia. Springer. (2021) p. 103–123. doi: 10.1007/978-3-030-55494-1_6
54. Rajalakshmy M, Maniyan AK, Sruthi C, Sindhu A. Standardisation of a polyherbal anti-diabetic Ayurvedic medicine Diajith. Ayurpharm Int J Ayur Alli Sci. (2014) 3:186–94.
55. George TK, Tomy A, Jisha MS. Molecular docking study of bioactive compounds of Withania somnifera extract against topoisomerase IV type B. Proc Natl Acad Sci India Sect B Biol Sci. (2020) 90:381–90. doi: 10.1007/s40011-019-01110-z
56. Narkhede RR, Cheke RS, Ambhore JP, Shinde SD. The molecular docking study of potential drug candidates showing anti-COVID-19 activity by exploring of therapeutic targets of SARS-CoV-2. Eurasian J Med. (2020) 4:185–95.
57. Hatada R, Okuwaki K, Mochizuki Y, Handa Y, Fukuzawa K, Komeiji Y, et al. Fragment molecular orbital based interaction analyses on COVID-19 main protease– inhibitor N3 complex (PDB ID: 6LU7). J Chem Inf Model. (2020) 60:3593–602. doi: 10.1021/acs.jcim.0c00283
58. Hückel E. Quantentheoretische Beiträge zum Problem der aromatischen und ungesättigten Verbindungen. III Zeitschrift für Physik. (1932) 76:628–48. doi: 10.1007/BF01341936
59. Kant K, Lal UR, Kumar A, Ghosh M. A merged molecular docking, ADME-T and dynamics approaches towards the genus of Arisaema as herpes simplex virus type 1 and type 2 inhibitors. Comput Biol Chem. (2019) 78:217–26. doi: 10.1016/j.compbiolchem.2018.12.005
60. Campbell GL, Hills SL, Fischer M, Jacobson JA, Hoke CH, Hombach JM, et al. Estimated global incidence of Japanese encephalitis: a systematic review. Bull World Health Organ. (2011) 89:766–74. doi: 10.2471/BLT.10.085233
61. Musini A, Rao JP, Giri A. Phytochemicals of Salacia oblonga responsible for free radical scavenging and antiproliferative activity against breast cancer cell lines (MDA-MB-231). Physiol Mol Biol Plants. (2015) 21:583–90. doi: 10.1007/s12298-015-0317-z
62. Charifson PS, Corkery JJ, Murcko MA, Walters WP. Consensus scoring: A method for obtaining improved hit rates from docking databases of three-dimensional structures into proteins. J Med Chem. (1999) 42:5100–9. doi: 10.1021/jm990352k
63. Charifson PS, Grillot A-L, Grossman TH, Parsons JD, Badia M, Bellon S, et al. Novel dual-targeting benzimidazole urea inhibitors of DNA gyrase and topoisomerase IV possessing potent antibacterial activity: Intelligent design and evolution through the judicious use of structure-guided design and stucture– activity relationships. J Med Chem. (2008) 51:5243–63. doi: 10.1021/jm800318d
64. Hou Q, Huang J, Xiong X, Guo Y, Zhang B. Role of nutrient-sensing receptor GPRC6A in regulating colonic group 3 innate lymphoid cells and inflamed mucosal healing. J Crohn's and Colitis. (2022) 20:1–13. doi: 10.1093/ecco-jcc/jjac020
65. Khaerunnisa S, Kurniawan H, Awaluddin R, Suhartati S, Soetjipto S. Potential inhibitor of COVID-19 main protease (Mpro) from several medicinal plant compounds by molecular docking study. Preprints. (2020) 2020:2020030226. doi: 10.20944/preprints202003.0226.v1
66. Ciotti M, Ciccozzi M, Terrinoni A, Jiang W-C, Wang C-B, Bernardini S. The COVID-19 pandemic. Crit Rev Clin Lab Sci. (2020) 57:365–88. doi: 10.1080/10408363.2020.1783198
67. Hu T, Wang S, She B, Zhang M, Huang X, Cui Y, et al. Human mobility data in the COVID-19 pandemic: characteristics, applications, and challenges. Int J Digital Earth. (2021) 14:1126–47. doi: 10.1080/17538947.2021.1952324
68. Khan SM, Rehman K, Ijaz U, Ali S, Jehangir S, Rehman AU, et al. Showcasing the Internationally Prioritized Medicinal Plants to Counteract the Pandemics—Potential Remedies for COVID-19 and other Forms of SARS: Internationally Prioritized Medicinal Plants to Counteract the COVID-19. Proc Pak Acad Sci: B. Life and Environ Sci. (2021) 58:55–67. doi: 10.53560/PPASB(58-sp1)655
Keywords: antioxidant, in silico, docking, interaction, phytochemical, A. jacquemontii Blume, Cobra lily, Anti-COVID protein
Citation: Shehzadi S, Khan SM, Mustafa G, Abdullah A, Khan I, Ahmad Z, Han H, Yu J, Park J and Raposo A (2022) Antiviral COVID-19 protein and molecular docking: In silico characterization of various antiviral compounds extracted from Arisaema jacquemontii Blume. Front. Public Health 10:964741. doi: 10.3389/fpubh.2022.964741
Received: 09 June 2022; Accepted: 18 August 2022;
Published: 23 September 2022.
Edited by:
Mohiuddin Md. Taimur Khan, Washington State University Tri-Cities, United StatesReviewed by:
Tuerhongjiang Tuxun, First Affiliated Hospital of Xinjiang Medical University, ChinaCopyright © 2022 Shehzadi, Khan, Mustafa, Abdullah, Khan, Ahmad, Han, Yu, Park and Raposo. This is an open-access article distributed under the terms of the Creative Commons Attribution License (CC BY). The use, distribution or reproduction in other forums is permitted, provided the original author(s) and the copyright owner(s) are credited and that the original publication in this journal is cited, in accordance with accepted academic practice. No use, distribution or reproduction is permitted which does not comply with these terms.
*Correspondence: Heesup Han, aGVlc3VwLmhhbkBnbWFpbC5jb20=; António Raposo, YW50b25pby5yYXBvc29AdWx1c29mb25hLnB0
Disclaimer: All claims expressed in this article are solely those of the authors and do not necessarily represent those of their affiliated organizations, or those of the publisher, the editors and the reviewers. Any product that may be evaluated in this article or claim that may be made by its manufacturer is not guaranteed or endorsed by the publisher.
Research integrity at Frontiers
Learn more about the work of our research integrity team to safeguard the quality of each article we publish.