- 1Department of Marine Geosciences, Rosenstiel School of Marine and Atmospheric Science, University of Miami, Miami, FL, United States
- 2Isobar Science-Beta Analytic, Research and Development Department, Miami, FL, United States
- 3Division of Pulmonary and Critical Care Medicine, Johns Hopkins University, Baltimore, MD, United States
- 4Department of Public Health Sciences, University of Miami, Miami, FL, United States
- 5Division of Pulmonary and Critical Care Medicine, College of Medicine-Jacksonville, University of Florida, Jacksonville, FL, United States
One-sentence summary
Our simulation shows widespread radioactive contamination in the event of nuclear accident(s) during the Ukraine war, but the extent and direction of the impact will depend on the timing of the accident.
Introduction
Thirty-six years have passed since the largest uncontrolled radioactive release at the Chernobyl nuclear power plant in Ukraine. Large amounts of iodine (I) and cesium (Cs) radioisotopes were scattered over a wide area. The total radioactive dose from Chernobyl is estimated at 80,000 man-sieverts, and the death toll was more than 200,000 (1). The current Ukraine war raises serious intentional or unintentional risk of another nuclear disaster. Thus, this research examines the spatiotemporal distribution of potential radioactive dust in the event of accidents in power plants in Ukraine. Here, we modeled air mass movements over the region using the HYSPLIT model and archived meteorological data from April 2021 to March 2022 under the assumption of explosion and release of radioactive particles after referring to as “incident” in any or all Ukrainian nuclear power plants at the same time. Furthermore, we used model outputs to study which countries are likely to be affected by the radioactive dust 3 days after the incident. The health effects of radioactive dust exposure are also discussed based on data collected after the Chernobyl incident.
Materials and methods
Site locations
Ukraine has four operational nuclear power plants with a capacity ranging from 2,000 to 6,000 megawatts and located in the northwest and south of the country [Figure 1 and Table 1 from Global Power Plant Database (2)]. The Rivne (Rouno) and Khmelnytska power plants are located in the northwest almost at the same longitude but 130 km apart, while the South Ukraine and Zaporozhye power plants are located at the same latitude but 255 km away from each other.
HYSPLIT forward-trajectory climate simulations
Three-days air mass forward trajectory ensemble plots at 500 m starting altitudes were computed for the first week of each month from April 2021 to March 2022 using the online web version of the HYSPLIT (Hybrid Single-Particle Lagrangian Integrated Trajectory) model (3, 4). HYSPLIT is one of the most extensively used atmospheric transport and dispersion models on earth by the atmospheric sciences community, which has evolved for more than 30 years. Its calculation method is a hybrid between the Lagrangian approach and the Eulerian methodology. HYSPLIT proved to be a complete system for computing not only a simple air parcel trajectory but also complex transport, dispersion, and deposition simulations, and it was successfully used to model the fallout from nuclear clouds (5).
Similarity between trajectories for different timeframes was tested for one of the cites (Rivne) for the first week of each month from April 2020 to March 2021 and from April 2021 to March 2022. The dispersion pattern and movement of air parcel originated from the Rivne site during the aforementioned time frames shows similar pattern (Supplementary Figure S1). Therefore, the time window of April 2021-March 2022 was chosen to represent the atmospheric circulation during the recent 12 months. The starting altitude range for the HYSPLIT model was chosen to be 500–2,000 m to provide maximum coverage for aerosol-bearing air masses that pass over the study area. To incorporate diverse instrumental data into a gridded, 3-D model space, the Global Data Assimilation System with one degree resolution (GDAS1) (https://ready.arl.noaa.gov/archives.php) was used to provide a comprehensive meteorological dataset for the HYSPLIT model. The coordinates of each nuclear power plant were used as the emission source of air masses.
Result and discussion
Results of the HYSPLIT three-days air mass forward-trajectory originated from the locations of the nuclear power plants in Ukraine are shown in Figure 2. As illustrated in Figure 2, the patterns of the air mass movement over the region show a large spatial and temporal variability. This suggests that the radioactive dust generated from the point sources will affect Ukraine, west of Russia, and other countries depending on the timing of the incident during the year (or season). Table 2 summarizes the countries that will be impacted by contaminated air masses during different months of the year. The HYSPLIT trajectories suggest that during the winter months 3 days after the occurrence of an incident in Ukrainian nuclear power plants, countries in central, south, and southeastern Europe as well as countries in the Middle East may receive radioactive-contaminated aerosol. During the fall, the air parcels containing radioactive-contaminated aerosols may hover over the countries in central, north, south, and southeastern Europe. In summer months, air parcels with radioactive-contaminated aerosols may travel over countries in central, north, south, and southeastern Europe, part of the Middle East, north Asia, and even North Africa. Finally, countries in central, north, south, and southeastern Europe may receive contaminated air parcels if the incident happens in Ukrainian nuclear power plants during spring. Major water bodies that could be affected by contaminated air parcels are the Black Sea, Baltic Sea, Mediterranean Sea, and, to a lesser extent, Caspian Sea. The contaminated dust would not reach the United States, at least after the first few days of the potential incident.
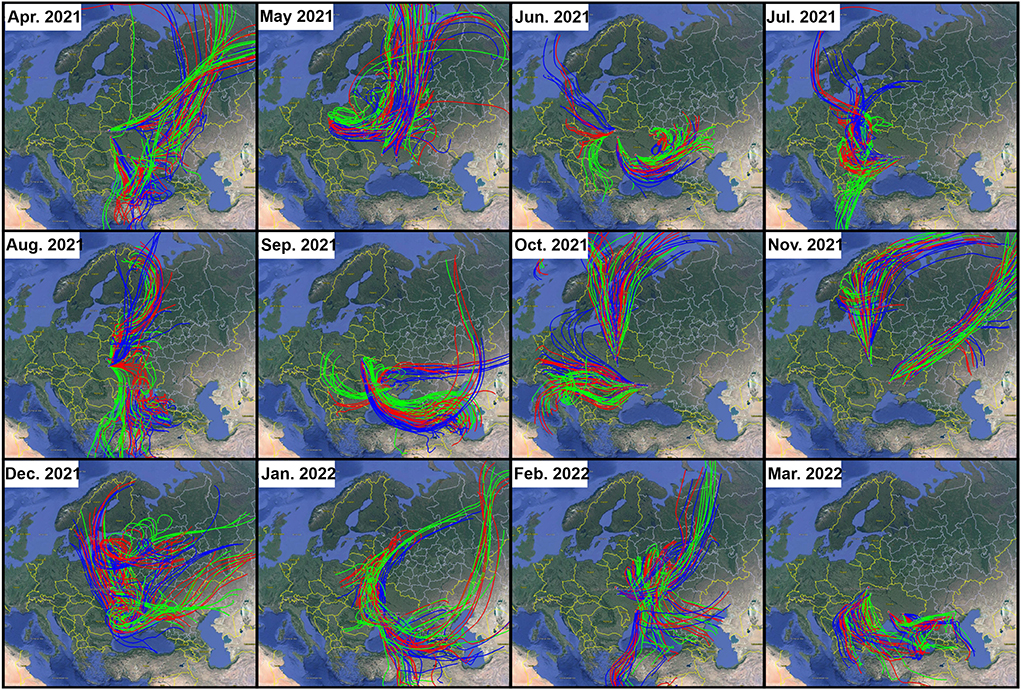
Figure 2. Three-day forward trajectory for the studied sites for the first week of each month starting from April 2021 to March 2022. Base map is from Google Earth.
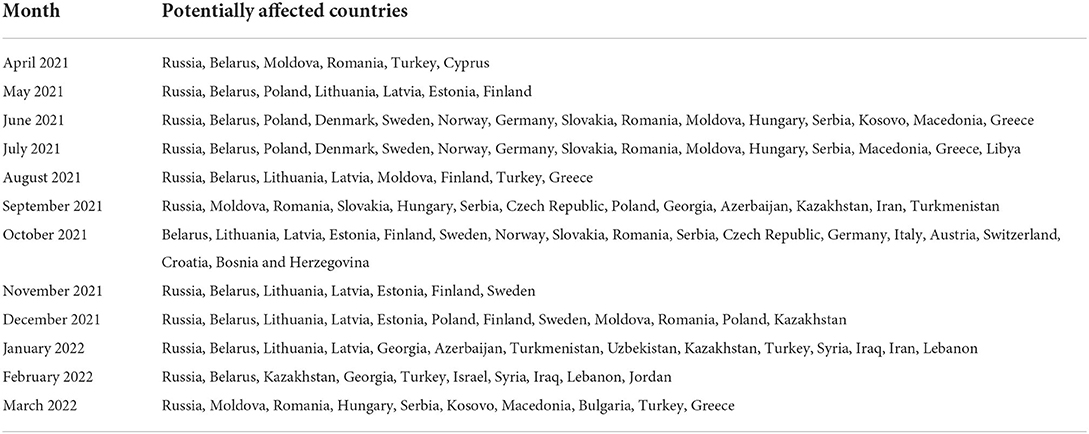
Table 2. List of countries which contaminated air mass will potentially travel over their air space from April 2021 to March 2022.
The health effects of radioactive dust may be classified as early and late health effects. Acute radiation syndrome (ARS) may occur in the 30 km2 around each burst plant resulting in death mainly due to significant skin injuries and bone marrow failure. People who survive from ARS may suffer from cataract, skin injuries, respiratory illnesses such as emphysema and bronchitis, and sexual dysfunction (6–8). Data show that 134 of 600 workers were diagnosed with ARS within hours after the Chernobyl accident. The sickness was seen among the power plant employees and first responders but not in the evacuated population or the general population. Nearly all people with a whole-body dose of more than 6.5 gray (Gy) died within 3 months (9).
Late health effects mainly occur because of development of malignant lesions. A marked increase in thyroid cancer incidence was reported in Belarus, Ukraine, and the four most contaminated regions of Russia (Bryansk, Kaluga, Orel, Tula); among those were younger than 18 years at the time of the Chernobyl nuclear accident. The total number of cases of thyroid cancer due the Chernobyl accident registered between 1991 to 2015 among 18 years or younger in Belarus, Ukraine, and the most contaminated regions of Russia exceeded 19,000 (10). Children and adolescents received high radiation doses to the thyroid mainly because of consumption of fresh milk containing 131I, specifically in the 8 weeks following the disaster, and because of iodine's 8-day half-life (11). Individual thyroid doses due to radioactive iodine ingestion fluctuated up to 42 gray (Gy) and depended on the age of the individual, region of exposure, and individual's dairy product consumption habits. Population-average thyroid doses among children of youngest age reached up to 0.75 Gy in Belarus most contaminated area, the Gomel Oblast (12–15).
A Chernobyl cohort of registered recovery and cleanup workers who received radioactive doses varying from 20 to 500 mSv and were followed up over the period 1992–2009 showed a significant increase of 18% in all solid cancers. Furthermore, the dose-response relationship was confirmed (16). In contrast to the substantial increase in thyroid cancer incidence among those exposed at under 18 y, except in clean-up workers, no meaningful evidence of any statistical association between radionuclide exposure and leukemia risk yet exists. Previous studies couldn't establish a consistent correlation between radiation and leukemia incidences in the exposed populations (9, 17, 18).
Nonmalignant diseases may develop in victims of remote exposure to radionuclide dust particles. Since the release of the WHO 2006 report, there has been emerging evidence that radiation as low as 20 mSv can lead to radiation-induced cataracts (19–21) and potential cardiovascular disease (22, 23).
According to experts, psychosocial impacts are the disaster's main public health issues that have affected the largest number of people. Common problems include cerebrovascular diseases, organic mental and mood disorders, and cognitive impairments such as dementia that increased with irradiation dose exposure. Interestingly, radiation exposure level was found to be a common risk factor for mental health effects of the Chernobyl, Fukushima, and 3-mile island nuclear disasters (9, 24, 25).
The current trajectory models are based on the archived data and only present the movement of air masses for the first week of each month. To better understand the movement of air masses that could originate from the Ukrainian power plants and the possibility of countries receiving contaminated air parcels, we recommend a weekly trajectory frequency for each month of the year to be utilized.
Author contributions
Conceptualization: AS and MM. Methodology and visualization: AS. Investigation and writing-original draft: AS, RD, and MM. Supervision: MM. Writing-review and editing: AS, RD, NK, and MM. All authors contributed to the article and approved the submitted version.
Conflict of interest
The authors declare that the research was conducted in the absence of any commercial or financial relationships that could be construed as a potential conflict of interest.
Publisher's note
All claims expressed in this article are solely those of the authors and do not necessarily represent those of their affiliated organizations, or those of the publisher, the editors and the reviewers. Any product that may be evaluated in this article, or claim that may be made by its manufacturer, is not guaranteed or endorsed by the publisher.
Supplementary material
The Supplementary Material for this article can be found online at: https://www.frontiersin.org/articles/10.3389/fpubh.2022.959668/full#supplementary-material
References
2. Global Energy Observatory Google KTH KTH Royal Institute of Technology in Stockholm Enipedia. World Resources Institute. Global Power Plant Database. Published on Resource Watch and Google Earth Engine (2018). Available online at: http://resourcewatch.org/https://earthengine.google.com/
3. Stein AF, Draxler RR, Rolph GD, Stunder BJB, Cohen MD, Ngan F. NOAA's HYSPLIT atmospheric transport and dispersion modeling system. Bull Am Meteorol Soc. (2015) 96:2059–77. doi: 10.1175/BAMS-D-14-00110.1
4. Rolph G, Stein A, Stunder B. Real-time Environmental Applications and Display System: READY. Environ Model Softw. (2017) 95:210–28. doi: 10.1016/j.envsoft.2017.06.025
5. Rolph GD, Ngan F, Draxler RR. Modeling the fallout from stabilized nuclear clouds using the HYSPLIT atmospheric dispersion model. J Environ Radioact. (2014) 136:41–55. doi: 10.1016/j.jenvrad.2014.05.006
6. Belyi D, Kovalenko A, Bazyka D, Bebeshko V. Non-cancer effects in acute radiation syndrome survivors in Ukraine. Health Phys. (2010) 98:876–84. doi: 10.1097/HP.0b013e3181d270e4
7. Steinert M, Weiss M, Gottlöber P, Belyi D, Gergel O, Bebeshko V, et al. Delayed effects of accidental cutaneous radiation exposure: fifteen years of follow-up after the Chernobyl accident. J Am Acad Dermatol. (2003) 49:417–23. doi: 10.1067/S0190-9622(03)02088-7
8. Mettler FA Jr, Gus'kova AK, Gusev I. Health effects in those with acute radiation sickness from the Chernobyl accident. Health physics. (2007) 93:462–9. doi: 10.1097/01.HP.0000278843.27969.74
10. UNSCEAR. Evaluation Of Data On Thyroid Cancer In Regions Affected By The Chernobyl Accident, Unscear (2017).
11. Unscear. Health effects due to radiation from the Chernobyl accident, UNSCEAR assessments of the Chernobyl accident (2008).
12. Drozdovitch V. Radiation exposure to the thyroid after the chernobyl accident. Front Endocrinol. (2020) 11:569041. doi: 10.3389/fendo.2020.569041
13. Stezhko VA, Buglova EE, Danilova LI, Drozd VM, Krysenko NA, Lesnikova NR, et al. A cohort study of thyroid cancer and other thyroid diseases after the Chornobyl accident: objectives, design and methods. Radiat Res. (2004) 161:481–92. doi: 10.1667/3148
14. Drozdovitch V, Minenko V, Golovanov I, Khrutchinsky A, Kukhta T, Kutsen S, et al. Thyroid dose estimates for a cohort of belarusian children exposed to (131)I from the chernobyl accident: assessment of uncertainties. Radiat Res. (2015) 184:203–18. doi: 10.1667/RR13791.1
15. Likhtarov I, Kovgan L, Masiuk S, Talerko M, Chepurny M, Ivanova O, et al. Thyroid cancer study among Ukrainian children exposed to radiation after the Chornobyl accident: improved estimates of the thyroid doses to the cohort members. Health Phys. (2014) 106:370–96. doi: 10.1097/HP.0b013e31829f3096
16. Kashcheev VV, Chekin SY, Maksioutov MA, Tumanov KA, Kochergina EV, Kashcheeva PV, et al. Incidence and mortality of solid cancer among emergency workers of the Chernobyl accident: assessment of radiation risks for the follow-up period of 1992–2009. Radiat Environ Biophys. (2015) 54:13–23. doi: 10.1007/s00411-014-0572-3
17. Romanenko AY, Finch SC, Hatch M, Lubin JH, Bebeshko VG, Bazyka DA, et al. The Ukrainian-American study of leukemia and related disorders among Chornobyl cleanup workers from Ukraine: III. Radiation risks. Radiat Res. (2008) 170:711–20. doi: 10.1667/RR1404.1
18. Howe GR. Leukemia following the chernobyl accident. Health Phys. (2007) 93:512–5. doi: 10.1097/01.HP.0000281178.75068.e3
19. Chumak VV, Worgul BV, Kundiyev YI, Sergiyenko NM, Vitte PM, Medvedovsky C, et al. Dosimetry for a study of low-dose radiation cataracts among chernobyl clean-up workers. Radiat Res. (2007) 167:606–14. doi: 10.1667/RR0302.1
20. Shore RE, Neriishi K, Nakashima E. Epidemiological studies of cataract risk at low to moderate radiation doses: (not) seeing is believing. Radiat Res. (2010) 174:889–94. doi: 10.1667/RR1884.1
21. Hammer GP, Scheidemann-Wesp U, Samkange-Zeeb F, Wicke H, Neriishi K, Blettner M. Occupational exposure to low doses of ionizing radiation and cataract development: a systematic literature review and perspectives on future studies. Radiat Environ Biophys. (2013) 52:303–19. doi: 10.1007/s00411-013-0477-6
22. Little MP, Azizova TV, Bazyka D, Bouffler SD, Cardis E, Chekin S, et al. Systematic review and meta-analysis of circulatory disease from exposure to low-level ionizing radiation and estimates of potential population mortality risks. Environ Health Perspect. (2012) 120:1503–11. doi: 10.1289/ehp.1204982
23. Little MP, Azizova TV, Hamada N. Low- and moderate-dose non-cancer effects of ionizing radiation in directly exposed individuals, especially circulatory and ocular diseases: a review of the epidemiology. Int J Radiat Biol. (2021) 97:782–803. doi: 10.1080/09553002.2021.1876955
24. Loganovsky K, Marazziti D. Mental Health and Neuropsychiatric Aftermath 35 Years After the Chernobyl Catastrophe: Current State and Future Perspectives. Clinical Neuropsychiatry. (2021) 18:101–6. doi: 10.36131/cnfioritieditore20210204
Keywords: health effects, radioactive dust, Ukraine, nuclear accident, HYSPLIT
Citation: Sharifi A, Dinparastisaleh R, Kumar N and Mirsaeidi M (2022) Health effects of radioactive contaminated dust in the aftermath of potential nuclear accident in Ukraine. Front. Public Health 10:959668. doi: 10.3389/fpubh.2022.959668
Received: 01 June 2022; Accepted: 22 July 2022;
Published: 22 August 2022.
Edited by:
Stephen Waller, Uniformed Services University, United StatesReviewed by:
Mariia Pyshkina, Institute of Industrial Ecology Ural Brunch of Russian Academy of Science, RussiaCopyright © 2022 Sharifi, Dinparastisaleh, Kumar and Mirsaeidi. This is an open-access article distributed under the terms of the Creative Commons Attribution License (CC BY). The use, distribution or reproduction in other forums is permitted, provided the original author(s) and the copyright owner(s) are credited and that the original publication in this journal is cited, in accordance with accepted academic practice. No use, distribution or reproduction is permitted which does not comply with these terms.
*Correspondence: Mehdi Mirsaeidi, bS5taXJzYWVpZGkmI3gwMDA0MDt1ZmwuZWR1