- 1Department of Urology, Affiliated Zhongda Hospital of Southeast University, Nanjing, China
- 2School of Basic Medical Sciences, Weifang Medical University, Weifang, China
Background: Heterocyclic aromatic amines (HAAs) are a group of harmful substances produced while cooking meat at high temperatures. This study aimed to investigate the relationship between HAAs and the occurrence of kidney stones.
Methods: Data on the level of four HAAs, including 2-Amino-9H-pyrido [2, 3-b] indole (A-α-C), 1-Methyl-9H-pyrido [3, 4-b] indole (Harman), 9H-Pyrido [3, 4-b] indole (Norharman), and 2-Amino-1-methyl-6-phenylimidazo [4, 5-b] pyridine (PhIP), in the urine from adult participants were extracted from the 2013–2014 NHANES database. Propensity score matching (PSM) was used to balance confounding variables between the stone former and non-stone former groups, and logistic regression analysis was performed to analyze the relationship between HAAs and the occurrence of kidney stones.
Results: Of the 1,558 eligible participants, a history of kidney stones was self-reported by 140 (9.0%). Compared to non-stone formers, stone formers had higher concentrations of A-α-C, Harman, and Norharman and lower concentrations of PhlP in urine. After adjusting for all other confounding variables, multivariate logistic regression analysis showed that the high-Harman group had a higher risk of kidney stones than the low-Harman group [adjusted odds ratios (aOR) = 1.618, 95% CI: 1.076–2.433, p = 0.021]. After PSM analysis, Harman concentration remained a risk factor for kidney stones (high-Harman group vs. low-Harman group: aOR = 1.951, 95% CI: 1.059–3.596, p = 0.032).
Conclusion: Increased urinary Harman concentrations are associated with an increased risk of kidney stones in the general US population.
Introduction
Kidney stones are a common type of urological disease, accounting for 40–50% of all urinary stone diseases (1). The presence of kidney stones varies depending on geography, race, and lifestyle habits. The presence of kidney stones in developed countries is 5–15%, and the presence of kidney stones in China is gradually increasing, with a prevalence of 6.4% in adults and a younger age of onset (2, 3). Kidney stones are mineral deposits in the junction of renal calyces, renal pelvis, or pelvic ureter that cause hematuria and back pain, leading to urological infection, urinary tract obstruction, and even uremia (4, 5).
The pathogenesis of kidney stones is more complicated, with more predisposing variables such as dietary, metabolic, pharmacological, and environmental factors. Additionally, genetic mutations play an important role in the formation of kidney stones (6, 7). Meat intake is prevalent in the Western diet, and high-temperature cooking of meat products produces mutagenic and carcinogenic chemicals such as lipid and protein oxidation products, heterocyclic aromatic amines (HAAs), and polycyclic aromatic hydrocarbons (PAHs) (8–11). In the 1970s, HAAs, a class of mutagenic compounds, were detected in cooked meat (12). HAAs are a class of nitrogen-containing heterocyclic hazardous substances, and studies have found that more than 25 HAAs are formed in cooked red meat (13, 14). In the Maillard reaction system, amino acids, sugars, and creatine are the main components involved in the formation of such culinary toxicants (15). Our previous study found that increased concentrations of PAHs in urine were associated with an increased risk of kidney stones (16). However, no studies have examined the relationship between HAAs and the occurrence of kidney stones.
In the current study, we used data from the 2013–2014 National Health and Nutrition Examination Survey (NHANES) database to explore the relationship between urinary concentrations of HAAs and the risk of kidney stones, which was validated by propensity score matching (PSM) analysis.
Patients and Methods
Data Sources and Preparation
Data for this study were obtained from the NHANES database, which is a cross-sectional, nationally based survey conducted in the US to assess the health and nutritional status of adults and children. The interview component of the NHANES database included demographic information, socioeconomic information, dietary status, and health-related questions. The examination component included medical health-related physical examinations and laboratory tests. Beginning in 1999, the NHANES database publishes data files from the survey online on a 2-year cycle (17). All NHANES protocols were reviewed and approved by the National Center for Health Statistics (NCHS) Ethics Committee, and written informed consent was obtained from relevant participants for any data collection.
The 2013–2014 cycle contains data on HAAs. A total of 5,769 adults (≥20 years) participated in the survey during 2013–2014. The following exclusion criteria were included: (a) incomplete stone survey (n = 11); (b) unknown/abnormal HAAs (n = 4,023); (c) unknown body mass index (BMI), family income, education level, diabetes, renal function (creatinine, blood urea nitrogen, and uric acid levels) and urinary creatinine (n = 168). Based on these criteria, a total of 1,558 study subjects were finally included in this study.
Study Variables and Definitions
The presence of kidney stones was determined based on the KIQ026 question from the Kidney Conditions-Urology survey in the questionnaire data, which was “Have you ever had a kidney stone?” and participants who answered “yes” were defined as having a history of kidney stones.
Concentrations of HAAs in urine were measured by isotope-dilution high-performance liquid chromatography/electrospray ionization tandem mass spectrometry (ID HPLC-ESI MS/MS). The urine samples were fortified and hydrolyzed at 70°C under alkaline conditions for 5 h. The samples were then extracted by solid-phase extraction, after which the analytes were eluted and analyzed by LC/MS/MS (https://wwwn.cdc.gov/Nchs/Nhanes/2013-2014/HCAAS_H.htm). Only the following 10 HAAs appeared in the 2013–2014 cycle: 2-Amino-9H-pyrido [2, 3-b] indole (A-α-C), 2-Amino-3-methyl-9H-pyriodo [2, 3-b] indole (MeA-α-C), 1-Methyl-9H-pyrido [3, 4-b] indole (Harman), 9H-Pyrido [3, 4-b] indole (Norharman), 2-Amino-6-methyldipyrido [1, 2-a:3', 2'-d] imidazole (Glu-P-1), 2-Aminodipyrido [1, 2-a:3', 2'-d] imidazole (Glu-P-2), 3-Amino-1,4-dimethyl-5H-pyrido [4, 3-b] indole (Trp-P-1), 1-Methyl-3-amino-5H-pyrido [4, 3-b] indole (Trp-P-2), 2-Amino-3-methyl-3H-imidazo [4, 5-f] quinoline (IQ), and 2-Amino-1-methyl-6-phenylimidazo [4, 5-b] pyridine (PhIP). The lower limit of detection (LOD) for the 10 HAAs was 0.62, 0.33, 4.59, 12.6, 0.31, 0.83, 0.79, 0.63, 0.37, and 0.34 pg/mL, respectively. Since the percentage of ≥ LOD for Glu-P-1, Glu-P-2, IQ, MeA-α-C, Trp-P-1, and Trp-P-2 was below 20%, further analysis was not considered. In addition, age, sex, marital status, race, family income, education level, BMI, hypertension, diabetes, physical activities, smoking status, urinary creatinine, and renal function [creatinine, blood urea nitrogen, uric acid levels, and estimated glomerular filtration rate (eGFR)] were also included in the study. The BMI was calculated as weight (kg)/[height (m2)*height (m2)]. Hypertension and diabetes mellitus was diagnosed by a physician or other health professional. The estimated glomerular filtration rate (eGFR) was measured in our previous study (5, 18).
Statistical Analysis
The categorical data were described by frequency (n) and percentage (%), and continuous data were described by the mean ± SD. In processing the data, a weighted analysis the data was performed. HAAs were divided into high and low concentration groups according to the median. A univariate and multivariate logistic regression was performed to evaluate the association between different concentration groups of HAAs and kidney stones, and the results were expressed as adjusted odds ratios (aORs) and 95% CI intervals, and the same analysis was performed in all subgroups. We constructed three multivariate logistic regression models: the base model included participant demographic variables, including age, sex, race, education level, marital status, and family income; the core model added variables related to participants' living conditions, such as BMI, diabetes, hypertension, physical activity status, and smoking status; and the extended model added creatinine, blood urea nitrogen, uric acid, eGFR, and urinary creatinine, which are indicators related to renal function.
Additionally, a 1:1 PSM analysis was performed to balance the differences between stone former and non-stone former groups, with the following adjusted confounding variables: age, sex, race, education level, marital status, family income, BMI, hypertension, diabetes, physical activity status, and smoking status. To further test the correctness of the results, the data after PSM were reanalyzed. All data and figures in this study were organized and analyzed using R software (version 3.5.3) and SPSS software (version 24). Statistical significance was set at p < 0.05.
Results
Based on the criteria in the flow chart (Figure 1), 1,558 participants were eventually included in the analysis, of whom 140 (9%) self-reported history of kidney stones, and 1,418 (91%) had no history of kidney stones. The clinicopathological characteristics of all participants are shown in Table 1. A chi-square test revealed significant differences between the stone former and the non-stone former groups in six variables: age, race, BMI, hypertension, diabetes, and physical activity (all p < 0.05). In addition, the stone former group had higher levels of blood urea nitrogen, blood creatinine, uric acid, urine creatinine, and lower levels of eGFR compared with the non-stone former group.
Table 2 demonstrates the concentrations of HAAs in the urine of stone formers and non-stone formers. Since the percentage of ≥ LOD for Glu-P-1, Glu-P-2, IQ, MeA-α-C, Trp-P-1, and Trp-P-2 was below 20%, further analysis was not considered. We found higher concentrations of A-α-C, Harman, and Norharman and lower concentrations of PhlP in the urine of stone formers than in non-stone formers.
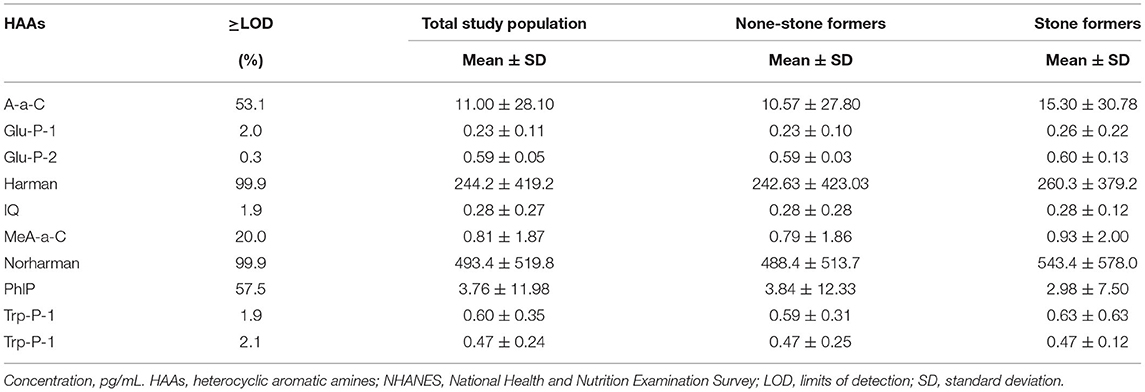
Table 2. The distribution levels of urinary HAAs in the study population with and without kidney stones in NHANES 2013–2014.
Patients were categorized into high and low concentration groups according to the median level of HAAs. Logistic regression was performed to analyze the relationship between HAAs and the occurrence of kidney stones. Univariate logistic regression analysis showed that the risk of kidney stones was 65.2% (95% CI: 1.157–2.358, p = 0.006) higher in the high Harman group compared to that in the low Harman group; 66.6% (95% CI: 1.167–2.378, p = 0.005) higher in the high Norharman group compared to the low Norharman group. Multivariate logistic regression analysis that high Harman was an independent risk factor for kidney stones in both the base model (aOR = 1.511, 95% CI: 1.041–2.194, p = 0.03), core model (aOR = 1.622, 95% CI: 1.106–2.38, p = 0.013), and expanded model (aOR = 1.618, 95% CI: 1.076–2.433, p = 0.021) (Table 3). In addition, all subgroup analyses also found an increased risk of kidney stones in the high Harman group compared to the low Harman group (Figure 2).
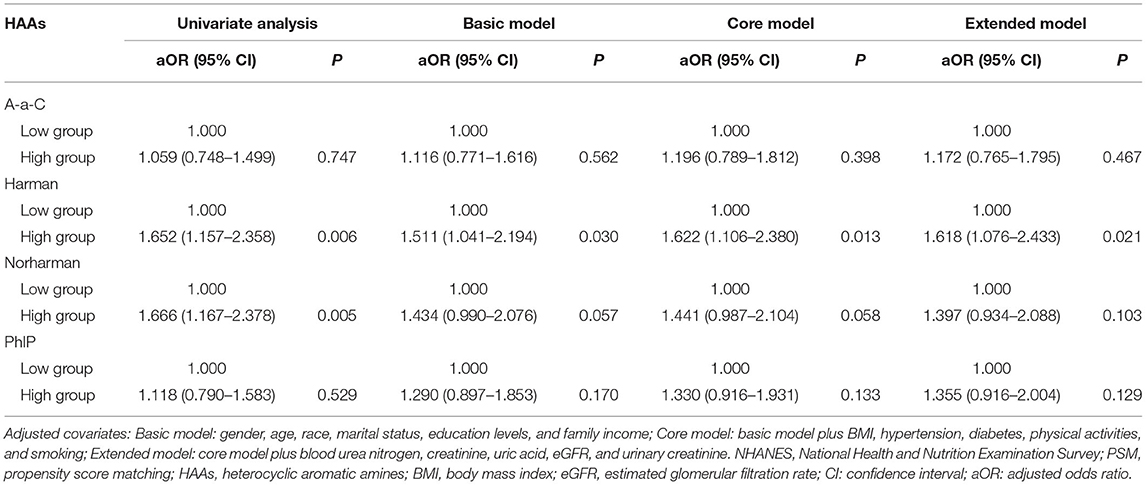
Table 3. Adjusted odds ratios for associations between the urinary HAAs and the presence of kidney stones in NHANES 2013–2014 before PSM.
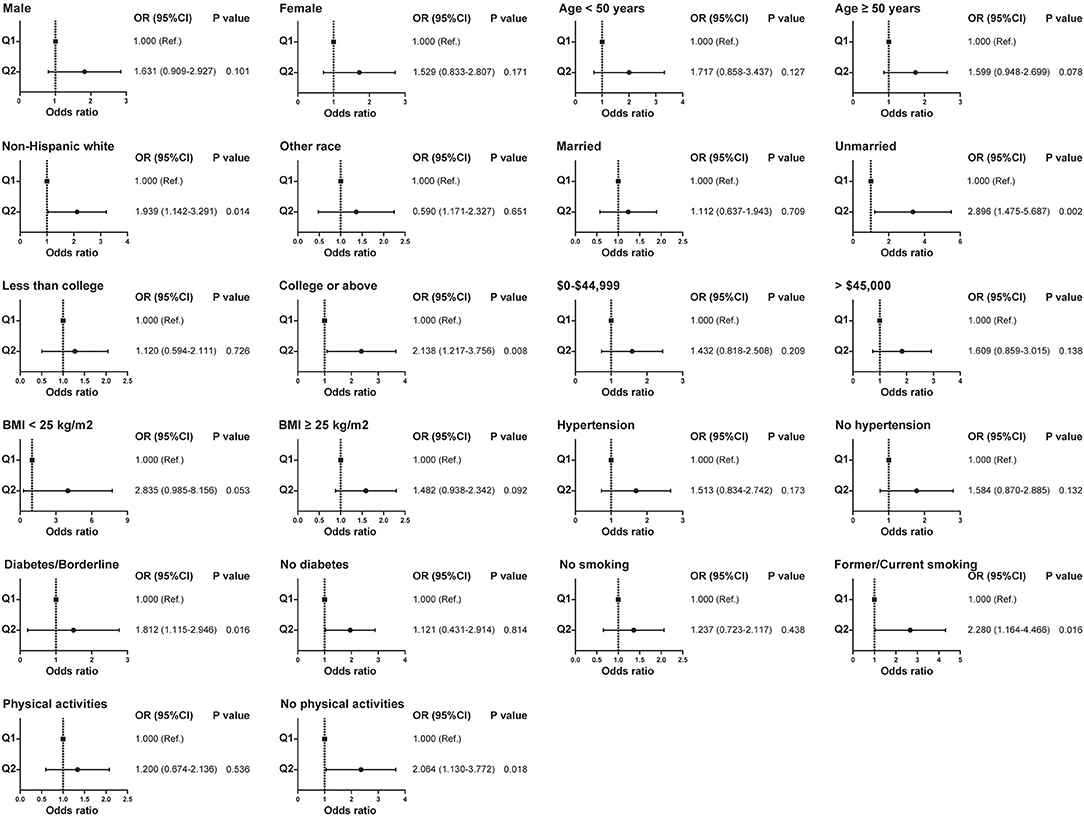
Figure 2. Relationship between Harman and kidney stones in subgroups with different clinical characteristics before propensity score matching.
Due to the differences in some variables between the stone former and non-stone former groups, we used a 1:1 PSM to correct for differences between the two groups (Figure 3 and Supplementary Figure S1). A total of 132 participants were included in the stone former and non-stone former groups, respectively. The clinicopathological characteristics of all participants after PSM are shown in Table 4. We found higher concentrations of A-α-C, Harman, and Norharman and lower concentrations of PhlP in the urine of participants in the stone former group than in the non-stone former group; however, the differences were not statistically significant. We likewise performed a multivariate logistic regression analysis and found that high Harman was an independent risk factor (aOR = 1.951, 95% CI: 1.059–3.596, p = 0.032) for kidney stones (Table 5), and similar results were found for subgroup analysis (Figure 4).
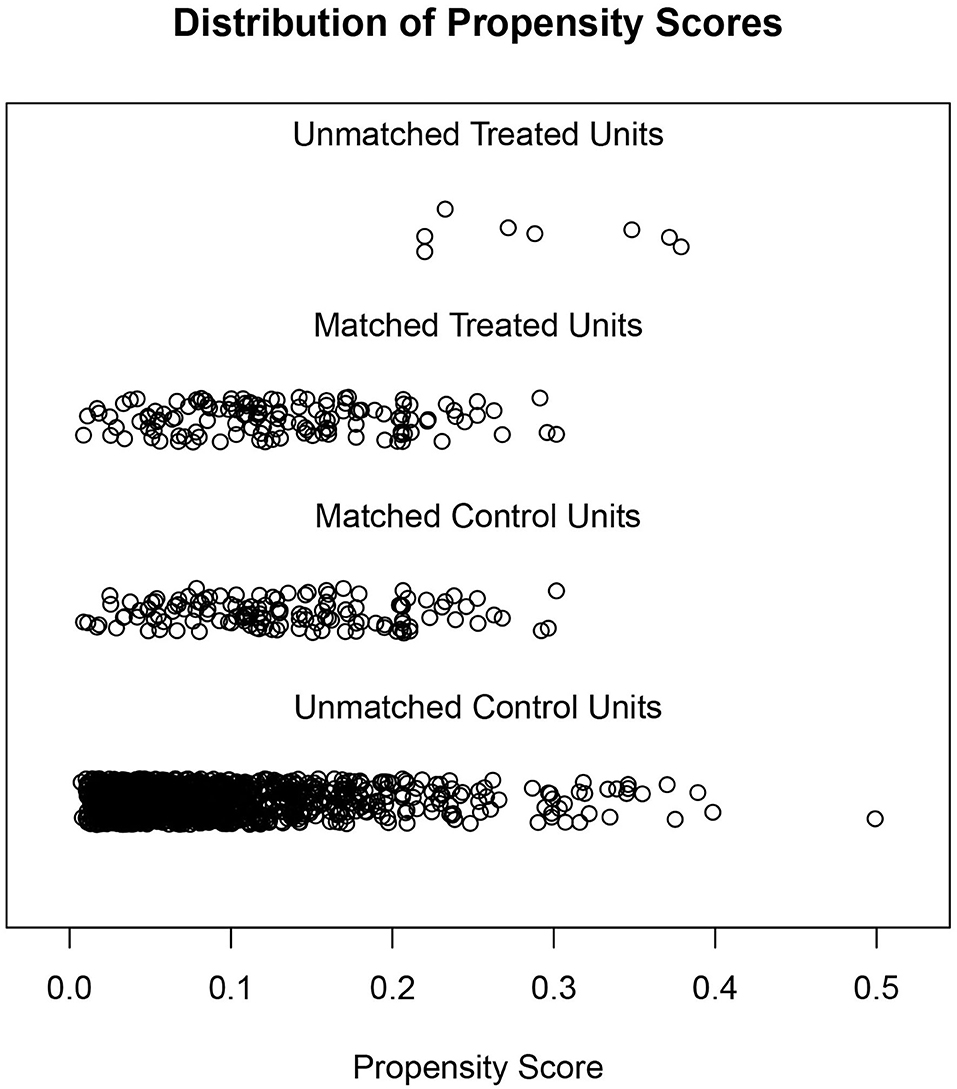
Figure 3. Distribution of the stone former and non-stone former groups before and after propensity score matching.
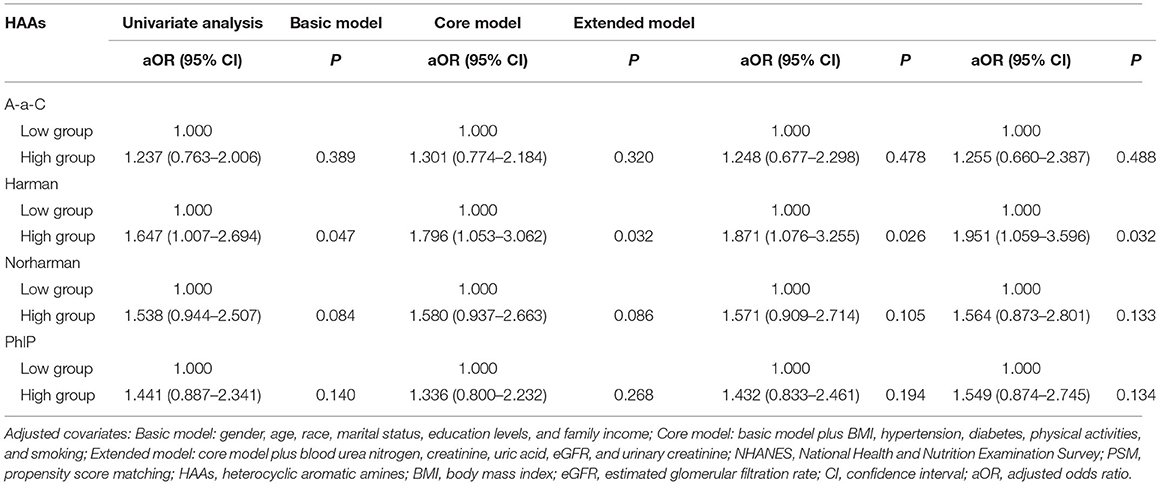
Table 5. Adjusted odds ratios for associations between the urinary HAAs and the presence of kidney stones in NHANES 2013–2014 after PSM.
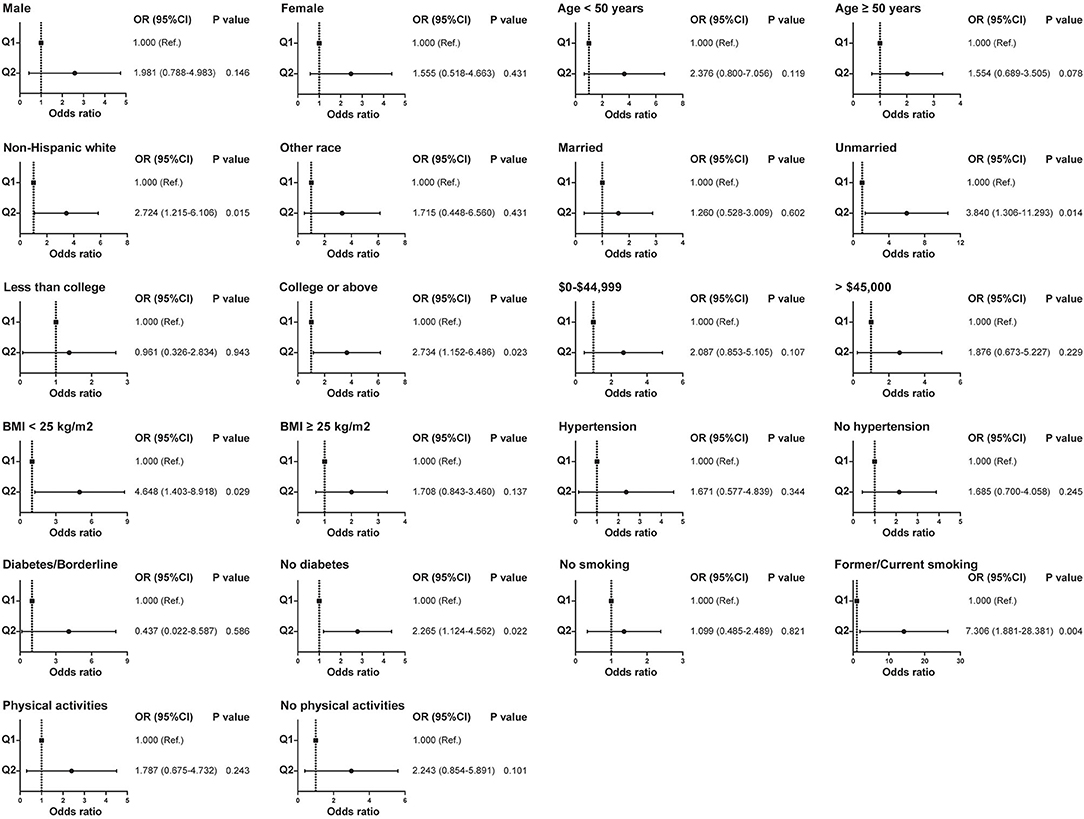
Figure 4. Relationship between Harman and kidney stones in subgroups with different clinical characteristics after propensity score matching.
Discussion
Kidney stone is a common urological disease that seriously affects human health and imposes a substantial medical burden on the patient's family. Therefore, early identification of risk factors for kidney stones has important clinical significance. In the present study, we used the NHANES database, which is representative of the US population, and found that the concentration of some HAAs (A-α-C, Harman, and Norharman) in the urine of patients with kidney stones was higher than that of patients without kidney stones. In addition, after adjusting for other confounding variables, multivariate logistic regression analysis showed that the concentration of Harman in urine was positively associated with the risk of kidney stones, with a 61.8% increased risk in the high Harman group compared to the low Harman group. To exclude the effects of other confounding variables and to test the validity of these results, we used PSM analysis to adjust for differences between the stone former and non-stone former groups. Subsequent multivariate logistic regression and subgroup analysis confirmed that increased concentrations of Harman in the urine might be a risk factor for kidney stones.
Heterocyclic aromatic amines are a class of polycyclic aromatic compounds that are formed naturally during the heating of protein-based foods such as meat (19). The average exposure to meat HAAs varies by the nation due to differences in the diets of the East and West, especially the amount of meat consumed (20). Studies have shown that the average daily exposure to HAAs is 565.3 ng/d in Americans, 69.4 ng/d in Europeans, and 49.94 ng/d in the Chinese population (21–23). Exposure to meat HAAs is correlated with the presence of kidney stones.
Numerous experiments have shown that HAAs can cause gene mutations or cancer by disrupting hydrogen bonds in DNA strands through the insertion/deletion of gene loci (24, 25). Excessive consumption of foods containing HAAs, especially cooked protein meats, is associated with an increased risk of several cancers (26, 27). Components of HAAs in grilled meat can be transformed into possibly carcinogenic genotoxic chemicals, and human bodies can detoxify HAAs by conjugation and eliminate the metabolites through urine (28, 29). Urinary HAAs are biomarkers that can be used to determine the exposure and the relative biological activity of detoxification in the organism (30).
Oxidative stress is closely associated with the formation of kidney stones (31). Lawana et al. (32) found that low doses of Harmane caused a 1.6-fold increase in reactive oxygen species (ROC) production in SH-SY5Y cells, which increased to 3.5-fold at higher doses. Furthermore, there was a dose-dependent increase in ROC synthesis in SH-SY5Y cells in response to Phlp and its metabolites. In addition, increased exposure to HAAs was associated with increased dopamine oxidation metabolites in vivo, which in turn caused oxidative stress in cells (33). Our findings provide a potential clinical basis for investigating the relationship between HAAs and the presence of kidney stones, and the accumulation of HAAs may increase the presence of kidney stones through increased oxidative stress.
Several findings of the current study are noteworthy. First, the participants included in this study were representative of the national population of the US, and the findings warrant generalization. Second, we constructed multiple multivariate logistic regression analysis models, adjusting for other confounding variables separately in the statistical analysis. Finally, to verify the reliability of our findings, we also conducted a 1:1 PSM analysis, which was used to eliminate the effects caused by quantitative differences between groups. However, there are certain limitations to the study. First, the NHANES database is a cross-sectional retrospective questionnaire with its inherent limitations. Second, the type of kidney stones is not available in the NHANES database, and different stone types may yield different results. Finally, the causal relationship between the concentration of urinary HAAs and kidney stones is difficult to determine, thus warranting further basic experiments for determination.
Conclusion
Higher concentrations of urinary HAAs are associated with the development of kidney stones, and increased urinary Harman concentration may be a risk factor for kidney stones.
Data Availability Statement
The datasets presented in this study can be found in online repositories. The names of the repository/repositories and accession number(s) can be found in the article/Supplementary Material.
Ethics Statement
The studies involving human participants were reviewed, approved and was carried out in accordance with the recommendations of NHANES committee with written informed consent from all subjects. All subjects gave written informed consent in accordance with the Declaration of Helsinki. The protocol was approved by the NHANES committee. The patients/participants provided their written informed consent to participate in this study.
Author Contributions
All authors contributed to data analysis, drafting or revising the article, have agreed on the journal to which the article will be submitted, gave final approval of the version to be published, and agree to be accountable for all aspects of the work.
Funding
This work was supported by National Natural Science Foundation of China (81900618, 82170703), Tai-Shan Scholar Program from Shandong Province (tsqn202103116), Jiangsu Provincial Key Research and Development Program (BE2019751), Innovative Team of Jiangsu Provincial (2017ZXKJQW07), and the National Key Research and Development Program of China (SQ2017YFSF090096).
Conflict of Interest
The authors declare that the research was conducted in the absence of any commercial or financial relationships that could be construed as a potential conflict of interest.
Publisher's Note
All claims expressed in this article are solely those of the authors and do not necessarily represent those of their affiliated organizations, or those of the publisher, the editors and the reviewers. Any product that may be evaluated in this article, or claim that may be made by its manufacturer, is not guaranteed or endorsed by the publisher.
Acknowledgments
We thank Bullet Edits for editing this manuscript.
Supplementary Material
The Supplementary Material for this article can be found online at: https://www.frontiersin.org/articles/10.3389/fpubh.2022.935739/full#supplementary-material
Supplementary Figure S1. The proportion of the stone former and non-stone former groups before and after propensity score matching.
References
1. Howles SA, Thakker RV. Genetics of kidney stone disease. Nat Rev Urol. (2020) 17:407–21. doi: 10.1038/s41585-020-0332-x
2. Norsworthy AN, Pearson MM. From catheter to kidney stone: the uropathogenic lifestyle of proteus mirabilis. Trends Microbiol. (2017) 25:304–15. doi: 10.1016/j.tim.2016.11.015
3. Zeng G, Mai Z, Xia S, Wang Z, Zhang K, Wang L, et al. Prevalence of kidney stones in China: an ultrasonography based cross-sectional study. BJU Int. (2017) 120:109–16. doi: 10.1111/bju.13828
4. Denburg MR, Koepsell K, Lee JJ, Gerber J, Bittinger K, Tasian GE. Perturbations of the gut microbiome and metabolome in children with calcium oxalate kidney stone disease. J Am Soc Nephrol. (2020) 31:1358–69. doi: 10.1681/ASN.2019101131
5. Mao W, Hu Q, Chen S, Chen Y, Luo M, Zhang Z, et al. Polyfluoroalkyl chemicals and the risk of kidney stones in US adults: a population-based study. Ecotoxicol Environ Saf. (2021) 208:111497. doi: 10.1016/j.ecoenv.2020.111497
6. Nettuwakul C, Praditsap O, Sawasdee N, Rungroj N, Ruamyod K, Watanapa WB, et al. Loss-of-function mutations of SCN10A encoding NaV1. 8 alpha subunit of voltage-gated sodium channel in patients with human kidney stone disease. Sci Rep. (2018) 8:10453. doi: 10.1038/s41598-018-28623-3
7. Singh P, Harris PC, Sas DJ, Lieske JC. The genetics of kidney stone disease and nephrocalcinosis. Nat Rev Nephrol. (2022) 18:224–40. doi: 10.1038/s41581-021-00513-4
8. Ozsarac N, Kolsarici N, Demirok Soncu E, Haskaraca G. Formation of heterocyclic aromatic amines in doner kebab cooked with different methods at varying degrees of doneness. Food Addit Contam Part A Chem Anal Control Expo Risk Assess. (2019) 36:225–35. doi: 10.1080/19440049.2018.1562230
9. Nogacka AM, Gomez-Martin M, Suarez A, Gonzalez-Bernardo O, de Los Reyes-Gavilan CG, Gonzalez S. Xenobiotics formed during food processing: their relation with the intestinal microbiota and colorectal cancer. Int J Mol Sci. (2019) 20:2051. doi: 10.3390/ijms20082051
10. Khan MR, Azam M. Shrimp as a substantial source of carcinogenic heterocyclic amines. Food Res Int. (2021) 140:109977. doi: 10.1016/j.foodres.2020.109977
11. Alsohaimi IH, Khan MR, Ali HM, Azam M. Emergence of mutagenic/carcinogenic heterocyclic amines in traditional Saudi chicken dishes prepared from local restaurants. Food Chem Toxicol. (2019) 132:110677. doi: 10.1016/j.fct.2019.110677
12. Khan MR. Influence of food condiments on the formation of carcinogenic heterocyclic amines in cooked chicken and determination by LC-MS/MS. Food Addit Contam Part A Chem Anal Control Expo Risk Assess. (2015) 32:307–14. doi: 10.1080/19440049.2015.1008057
13. Gibis M. Heterocyclic aromatic amines in cooked meat products: causes, formation, occurrence, and risk assessment. Compr Rev Food Sci Food Saf. (2016) 15:269–302. doi: 10.1111/1541-4337.12186
14. Zhang Y, Wu WJ, Zhou WE, Ren ZQ, Feng XS, Zhang F. Determination of 14 heterocyclic aromatic amines in meat products using solid-phase extraction and supercritical fluid chromatography coupled to triple quadrupole mass spectrometry. J Sep Sci. (2020) 43:1372–81. doi: 10.1002/jssc.201900816
15. Khan MR, Busquets R, Naushad M, Puignou L. Cooking with elaborate recipes can reduce the formation of mutagenic heterocyclic amines and promote co-mutagenic amines. Food Addit Contam Part A Chem Anal Control Expo Risk Assess. (2019) 36:385–95. doi: 10.1080/19440049.2019.1571286
16. Sun S, Mao W, Tao S, Zou X, Tian S, Qian S, et al. Polycyclic aromatic hydrocarbons and the risk of kidney stones in us adults: an exposure-response analysis of NHANES 2007–2012. Int J Gen Med. (2021) 14:2665–76. doi: 10.2147/IJGM.S319779
17. Mao W, Wu J, Zhang Z, Xu Z, Xu B, Chen M. Neutrophil-lymphocyte ratio acts as a novel diagnostic biomarker for kidney stone prevalence and number of stones passed. Transl Androl Urol. (2021) 10:77–86. doi: 10.21037/tau-20-890
18. Mao W, Wang K, Xu B, Zhang H, Sun S, Hu Q, et al. ciRS-7 is a prognostic biomarker and potential gene therapy target for renal cell carcinoma. Mol Cancer. (2021) 20:142. doi: 10.1186/s12943-021-01443-2
19. Dong H, Xian Y, Li H, Bai W, Zeng X. Potential carcinogenic heterocyclic aromatic amines (HAAs) in foodstuffs: formation, extraction, analytical methods, and mitigation strategies. Compr Rev Food Sci Food Saf. (2020) 19:365–404. doi: 10.1111/1541-4337.12527
20. Syeda T, Cannon JR. Potential role of heterocyclic aromatic amines in neurodegeneration. Chem Res Toxicol. (2022) 35:59–72. doi: 10.1021/acs.chemrestox.1c00274
21. Pouzou JG, Costard S, Zagmutt FJ. Probabilistic assessment of dietary exposure to heterocyclic amines and polycyclic aromatic hydrocarbons from consumption of meats and breads in the United States. Food Chem Toxicol. (2018) 114:361–74. doi: 10.1016/j.fct.2018.02.004
22. Rohrmann S, Zoller D, Hermann S, Linseisen J. Intake of heterocyclic aromatic amines from meat in the European Prospective Investigation into Cancer and Nutrition (EPIC)-Heidelberg cohort. Br J Nutr. (2007) 98:1112–5. doi: 10.1017/S000711450778145X
23. Wong KY, Su J, Knize MG, Koh WP, Seow A. Dietary exposure to heterocyclic amines in a Chinese population. Nutr Cancer. (2005) 52:147–55. doi: 10.1207/s15327914nc5202_5
24. Guinez M, Canales R, Talio C, Gomez D, Smichowski P. Determination of heterocyclic aromatic amines in ashes from biomass burning by UHPLC-MS/MS after ultrasound-assisted dispersive solid-liquid microextraction. Talanta. (2020) 206:120182. doi: 10.1016/j.talanta.2019.120182
25. Skaljac S, Jokanovic M, Tomovic V, Ivic M, Tasic T, Ikonic P, et al. Influence of smoking in traditional and industrial conditions on colour and content of polycyclic aromatic hydrocarbons in dry fermented sausage “Petrovská klobása”. LWT. (2017) 87:158–62. doi: 10.1016/j.lwt.2017.08.038
26. Bylsma LC, Alexander DD. A review and meta-analysis of prospective studies of red and processed meat, meat cooking methods, heme iron, heterocyclic amines and prostate cancer. Nutr J. (2015) 14:125. doi: 10.1186/s12937-015-0111-3
27. Ollberding NJ, Wilkens LR, Henderson BE, Kolonel LN, Le Marchand L. Meat consumption, heterocyclic amines and colorectal cancer risk: the multiethnic cohort study. Int J Cancer. (2012) 131:E1125–33. doi: 10.1002/ijc.27546
28. Turesky RJ, Garner RC, Welti DH, Richoz J, Leveson SH, Dingley KH, et al. Metabolism of the food-borne mutagen 2-amino-3,8-dimethylimidazo[4,5-f]quinoxaline in humans. Chem Res Toxicol. (1998) 11:217–25. doi: 10.1021/tx9701891
29. Malfatti MA, Kulp KS, Knize MG, Davis C, Massengill JP, Williams S, et al. The identification of [2-(14)C]2-amino-1-methyl-6-phenylimidazo[4,5-b]pyridine metabolites in humans. Carcinogenesis. (1999) 20:705–13. doi: 10.1093/carcin/20.4.705
30. Turesky RJ, Liu L, Gu D, Yonemori KM, White KK, Wilkens LR, et al. Biomonitoring the cooked meat carcinogen 2-amino-1-methyl-6-phenylimidazo[4,5-b]pyridine in hair: impact of exposure, hair pigmentation, and cytochrome P450 1A2 phenotype. Cancer Epidemiol Biomarkers Prev. (2013) 22:356–64. doi: 10.1158/1055-9965.EPI-12-1206
31. Khan SR. Reactive oxygen species as the molecular modulators of calcium oxalate kidney stone formation: evidence from clinical and experimental investigations. J Urol. (2013) 189:803–11. doi: 10.1016/j.juro.2012.05.078
32. Lawana V, Um SY, Rochet JC, Turesky RJ, Shannahan JH, Cannon JR. Neuromelanin modulates heterocyclic aromatic amine-induced dopaminergic neurotoxicity. Toxicol Sci. (2020) 173:171–88. doi: 10.1093/toxsci/kfz210
33. Wang Y, Peng L, Bellamri M, Langouet S, Turesky RJ. Mass spectrometric characterization of human serum albumin adducts formed with n-oxidized metabolites of 2-amino-1-methylphenylimidazo[4,5-b]pyridine in human plasma and hepatocytes. Chem Res Toxicol. (2015) 28:1045–59. doi: 10.1021/acs.chemrestox.5b00075
Keywords: kidney stone, heterocyclic aromatic amines, Harman, NHANES, propensity score matching
Citation: Zhang G, Zou X, Mao W and Chen M (2022) Heterocyclic Aromatic Amines and Risk of Kidney Stones: A Cross-Sectional Study in US Adults. Front. Public Health 10:935739. doi: 10.3389/fpubh.2022.935739
Received: 04 May 2022; Accepted: 20 June 2022;
Published: 14 July 2022.
Edited by:
Fei Li, Zhongnan University of Economics and Law, ChinaReviewed by:
Mohammad Rizwan Khan, King Saud University, Saudi ArabiaYu-Zheng Ge, Nanjing Medical University, China
Guohua Zeng, First Affiliated Hospital of Guangzhou Medical University, China
Copyright © 2022 Zhang, Zou, Mao and Chen. This is an open-access article distributed under the terms of the Creative Commons Attribution License (CC BY). The use, distribution or reproduction in other forums is permitted, provided the original author(s) and the copyright owner(s) are credited and that the original publication in this journal is cited, in accordance with accepted academic practice. No use, distribution or reproduction is permitted which does not comply with these terms.
*Correspondence: Ming Chen, bWluZ2NoZW5zZXVAMTI2LmNvbQ==; Weipu Mao, bWFvd2VpcHU4OEAxNjMuY29t; Xiangyu Zou, em91eGlhbmd5dWR5QDEyNi5jb20=