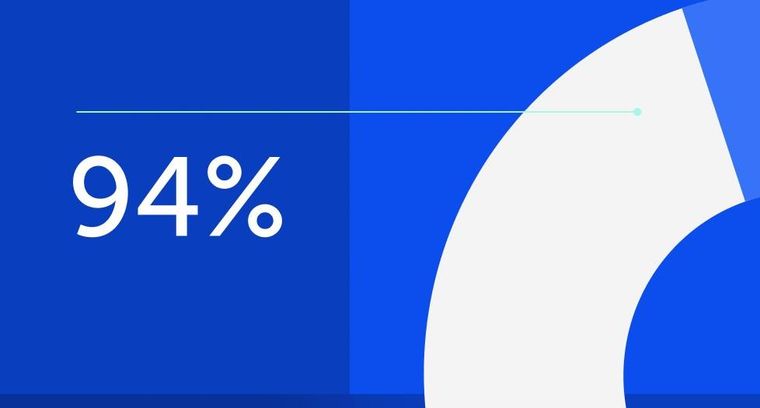
94% of researchers rate our articles as excellent or good
Learn more about the work of our research integrity team to safeguard the quality of each article we publish.
Find out more
REVIEW article
Front. Public Health, 26 April 2022
Sec. Occupational Health and Safety
Volume 10 - 2022 | https://doi.org/10.3389/fpubh.2022.828845
The emergence of SARS-CoV2 in 2019 showed again that the world's healthcare system is not fully equipped and well-designed for preventing the transmission of nosocomial respiratory infections. One of the great tools for preventing the spread of infectious organisms in hospitals is the anteroom. Several articles have investigated the role of the anteroom in disease control but the lack of a comprehensive study in this field prompted us to provide more in-depth information to fill this gap. Also, this study aimed to assess the necessity to construct an anteroom area for hospital staff members at the entrance of each ward of the hospital, and specify the equipment and facilities which make the anteroom more efficient. Articles were identified through searches of Scopus, Web of Sciences, PubMed, and Embase for studies published in English until May 2020 reporting data on the effect of the anteroom (vestibule) area in controlling hospital infections. Data from eligible articles were extracted and presented according to PRISMA's evidence-based data evaluation search strategy. Also, details around the review aims and methods were registered with the PROSPERO. From the database, 209 articles were identified, of which 25 studies met the study criteria. Most studies demonstrated that an anteroom significantly enhances practical system efficiency. The results showed that the equipment such as ventilation system, high-efficiency particulate absorption filter, hand dispensers, alcohol-based disinfection, sink, mirror, transparent panel, UVC disinfection, and zone for PPE change, and parameters like temperature, door type, pressure, and size of the anteroom are factors that are effective on the safety of the hospital environment. Studies demonstrated that providing an anteroom for changing clothing and storing equipment may be useful in reducing the transmission of airborne infections in hospitals. Since the transmission route of SARS-CoV2 is common with other respiratory infectious agents, it can be concluded that a well-designed anteroom could potentially decrease the risk of SARS-CoV2 transmission during hospitalization as well.
The SARS-CoV2 has been at the forefront of all infectious agents that humans have ever encountered in terms of socioeconomic impacts. In most countries, the number of patients hospitalized with COVID-19 was more than the ICU bed capacity and the risk of hospital-acquired infections among healthcare staff is higher than ever. Hospitals play an essential role in responding to communicable disease epidemics and infectious disease control. In the case of COVID-19, the focus of the hospitals is on symptomatic patients and isolating them. Face masks, gloves, long-sleeved gowns, and goggles are known as personal protective equipment (PPE) in dealing with the spread of infectious agents (1–3). However, asymptomatic and presymptomatic individuals may serve as a reservoir in hospital-acquired infections including SARS-CoV2 (4, 5).
It was found that reinfections of some pathogens such as SARS-CoV2 among healthcare workers would more likely to be asymptomatic. So, asymptomatic healthcare workers can cause person-to-person transmission and should be considered as a source of infection in hospital wards to prevent potential outbreaks and the infection of different types of patients. Therefore, all necessary designs and facilities to minimize the risk of nosocomial infections should be considered especially in the respiratory isolation wards.
Hospital staff members work in the wards and are in direct contact with other staff members and asymptomatic patients and are more likely to be infected at work. On the other hand, infected hospital staff without any symptoms can infect their family members, other healthcare personnel, and visitors through person-to-person transmission, and also via indirect contact transmission involving contaminated objects or surfaces (6, 7). In addition, infectious microscopic organisms can transmit through the air in an indoor environment when the infected people produce respiratory droplets by breathing, talking, coughing, and sneezing (8–10).
It seems that in hospitals the focus of infection prevention programs is on preventing infection transmission through direct contact, and the indirect transmission of infections in hospitals has been neglected, except for isolation rooms (4).
One of the most important concerns of hospital staff members is the transmission of infectious organisms from the work environment to their homes and other wards (11). Factors such as reluctance to use leisure time due to the possibility of getting infected, fear of infecting others, lack of facilities to disinfect themselves and their equipment, and high load of airborne pathogens in the air of the hospital environment have caused excessive job burnout in medical staff members that endangers their physical and emotional health (12).
Scientific studies specified that well-designed physical settings can be an important tool in making hospitals safer and less risky and stressful for patients, their families, and hospital staff members (13, 14). The design of hospital physical environments should help to prevent the spread of infections and improve the safety, and quality of hospitals (12, 13). One of the great tools contributing to the prevention of infectious diseases is the anteroom (also known as vestibule) (15, 16). It is a hospital physical environment that is mostly used at the entrance of the isolation room and diminishes or prevents the spread of airborne infectious organisms and reduces the movement of contaminated air or other dangerous particles from the isolation room to the hallway (17). The anteroom is a negative or positive pressurized area containing cabinets for supplies, a sink for handwashing, and enough space for donning and doffing of PPE (18). The role of anteroom with negative pressure is different from that with positive pressure. Infectious patients' isolation rooms must be maintained at a negative pressure relative to the anteroom so that air flows into the room and not in the anteroom, theoretically preventing the escape of infectious aerosols from the isolation room. But the cancer patients' isolation room should be positively pressurized compared with the anteroom to prevent the movement of contaminated air to the isolation room (12–14, 17).
Several articles have investigated the role of the anteroom (vestibule) in disease control and prevention but until now there is no comprehensive study in this field. Considering the ongoing pandemic of Coronavirus, it was necessary to conduct a systematic review to provide a complete understanding and summarize the evidence associated with the effect of the anteroom (vestibule) area in decreasing hospital infections including SARS-CoV2 across and examine the necessity of reconsideration in the space division of hospitals to provide a healthier space for hospital staff members and patients. Also, this study aims to answer the following questions:
1- Is it necessary to construct an anteroom area for hospital staff members at the entrance of each ward of the hospital?
2- How is the effect of equipment such as ventilation system, high-efficiency particulate absorption (HEPA) filter, hand dispensers, alcohol-based disinfection, sink, mirror, transparent panel, UVC disinfection, and zone for PPE change, and parameters like temperature, door type, pressure, and size of the anteroom on the safety of the hospital environment?
3- What are the differences in the role of the anteroom with negative pressure from that with positive pressure?
The results of this study were reported according to the PRISMA (Preferred Reporting Items for Systematic Reviews and Meta-Analyses) guidelines (19). Also, details around the review aims and methods were registered with the PROSPERO (registration number: CRD42021257048). We searched Scopus, Web of Science, PubMed, and Embase databases for articles regarding the effect of the anteroom (vestibule) area in controlling hospital infections. Searches were performed without date restriction until 7th March 2021. Articles written in English were searched. We also used references of included primary articles for search.
The search terms used were as follows: “anteroom OR vestibule,” AND “hospital,” AND “infection control.” Two independent researchers screened titles and abstracts of papers and any disagreements were resolved by a third reviewer. A PRISMA flowchart showing the study selection process is depicted in Figure 1.
Studies were included in this review if they were peer-reviewed and written in the English language. Studies must have investigated the effect of the anteroom (vestibule) area in controlling hospital infections. Furthermore, studies must have clearly described the methodology and environments in which the study was performed.
All search results were managed with EndNote X7.1 (Clarivate Analytics, Philadelphia, PA, USA). Duplicates were deleted and the title and abstract of the remaining citations were reviewed to exclude irrelevant articles. For the remaining citations, full texts were downloaded and evaluated. Authors have independently assessed the risk of bias in the included studies, according to the criteria from the modified STROBE checklist, as a validated method for assessing the quality of observational and case-control studies (20). The instrument used a system to evaluate cross-sectional studies based on some criteria: title and abstract, background, objectives, study design, setting, participants, variables, data sources, and measurements, bias, study size, quantitative variables, statistical methods, participants' result, descriptive data result, outcome data and main results, other analyses, key results, limitations, interpretation, and generalizability (20).
For each domain, the following description was used for management of the risk of bias: “Yes,” “No,” and “Unclear.” We graded the quality of included studies and the risk of bias, using grading 1 for “yes” and 0 for “no and unclear,” and disagreement was resolved by discussion.
The following information/data were extracted from studies that met the inclusion criteria: name of the first author, year of publication, data collection period, continent, geographical location (country), study design, type of hospital infection (viral/fungal/bacterial), type of infectious organisms, interventions, study objectives, major findings, recommendation, and citation. When the reported data were insufficient or in the case of articles whose full text was not available, we contacted the corresponding by email to request additional information or full text. After three emails with a week's interval, these studies were excluded.
Systematically searching multiple data sources identified a total of 209 records. After the removal of duplicates, 134 papers remained and after screening by title and abstract 97 articles were excluded based on inclusion and exclusion criteria. Finally, 37 studies reporting data on the effect of the anteroom (vestibule) area in controlling hospital infections were assessed for eligibility and 25 study papers (21–44) were included in the systematic review after full-text scrutiny. The main characteristics of the included studies are summarized in Table 1.
All included papers were published between 1993 and 2020. One research reported data from 14 European countries (Denmark, Greece, Spain, Ireland, Austria, Slovenia, Bulgaria, Luxembourg, Finland, France, Malta, Italy, Norway, and Poland) as a single study (23). Results from a single study were showed data from 2 different countries (Finland and Singapore) (28). Also, data from one study was uncategorizable (44). Regarding the continent, the majority of the studies were conducted in Europe (n = 11) and North America (n = 9) (Figure 2), and regarding the country the highest numbers of reports were available for the USA (n = 7/25) and Nordic countries (n = 7/25).
Figure 2. Several studies conducted in different continents reporting data on the effect of the anteroom (vestibule) area in controlling hospital infections.
Of the 11 studies reporting data for the effect of the anteroom (vestibule) area in controlling hospital infections caused by infectious organisms (17, 21, 26, 29, 32–36, 42, 43), 8 had separate data for viral infections (17, 21, 32, 34–36, 42, 43). Also, 2 studies reported data on bacterial infections (29, 33), and one study included fungal infections (26).
Studies highlighted that an anteroom enhances practical system efficiency. It acts as a buffer zone and a layer between the isolation room and the corridor to maintain the pressure gradient and prevent pressure loss (17, 23, 32, 37). The anteroom is considered necessary for reducing the virus particle migration and air transmission from the isolation room to the corridor. It enhances environmental control and safety by reducing the risk of infection transferring (17, 32, 37). Frequently disinfection of the anteroom causes less virus transmission to corridors (32). The environmental design of the access point of hospital wards with spaces such as anteroom that are well-equipped and is able to exclude airflow, enhance infection transmission prevention and air load control (26).
Eleven studies highlighted the effects of pressure on preventing air transmission and reducing the risk of infection (17, 26, 27, 31, 35–37, 39, 41, 43, 44). Results of a study conducted by Jan Styczynski et al., demonstrated that among 177 centers from 36 countries, the numbers of existing centers which use positive and negative pressure for their anterooms pressure gradient are about the same number of 19–20% (44). Three studies mentioned that anterooms equipped with negative pressure prevent airborne transmission and high particle air load (26, 27, 35). In one study, it was reported that the air exchange during door opening and closing between an isolation room and anteroom with negative pressure of −2.5 Pa was reduced compared to the neutral situation (27). In another study, the positive pressure rooms environment was changed to negative pressure to prevent airborne transmission to near areas. The −1.2 Pa pressure was used for the anteroom environment (35).
Two studies emphasize that the positive pressure of an anteroom makes the system reliable and acts as an airlock to minimize the air and particle flow (31, 39). In one study, it was shown that the particles can transfer to the isolation room through the anteroom with negative pressure, while anterooms with positive pressure disrupt the particle flow way. The positive pressure acts as a barrier and reduces the concentration of particles (39). The other study mentioned that positive pressure was preferred to the negative pressure anteroom. The anteroom pressured set at 10 Pa. The pressure level of the room can be seen from the work area and nursing station (31).
Four studies focused on the pressure difference gradient between rooms (17, 36, 41, 43). In one study, a −8 Pa pressure difference between an anteroom and isolation room resulted in better function and effective infection prevention (36). In another study, it was mentioned that the pressure difference between the anteroom and the isolation room with the 20 Pa results in lower infection particle transmission than the difference pressure gradient of 1.5 Pa (41). In the other study, the pressure difference was −8 Pa up to −15 Pa based on the combination of the pressure difference between the isolation room and anteroom, and the difference between the anteroom and corridor (17). While a study reported a pressure difference of more than 15 Pa between anteroom and isolation room and between anteroom and other areas as a suitable pressure difference (43).
Four studies emphasized the practical and beneficial aspect of implementing the pressure device monitor for pressure checks (17, 31, 43, 44). A pressure device on the wall helps health staff to control the pressure difference easily and change air measurement (17, 44). A monitoring system for controlling the pressure difference between the anteroom and the patient room or anteroom and hallway to not fall under the determined gradient Pa would help to guarantee the maintenance of continuous positive pressure by alerting the staff (44). Checking tools such as visual pressure check gauges and alarms are set to control the performance of ventilation systems. Scheduled protocol plans are designed to have high prevention maintenance and keep the required standards (31).
Eleven studies considered that the air systems help to improve efficiency by removing particles (17, 21, 23, 26, 31, 33–36, 43, 44). The ventilation system of the isolation unit is separated from other wards and connected to a backup power source. The airflow and pressure gradient flow from the cleanest to the most contaminated area. The air does not circulate again and is exhausted in a site far from the building to reduce the risk of infection transmission for occupants and the community (43). An anteroom is ventilated by the incoming and outgoing air device (31). The location and type of air device have an impact on directing airflow and reducing the infection transmission (17). Air intake and air exhaust happen at two opposite sides of the room (44).
The rate of air exchange was examined by five studies. One study indicates that the rate of air exchanges between 5 and 10 times per hour is efficient, based on the type of patients. The normal situation required 5 times and 10 times per hour was used for patients with airborne transmission disease (31). Another study reported that an airflow exchange in an anteroom between 14 and 18 times per hour was effective (35). Two studies considered a minimum of 12-air exchange per hour to be mandatory for having an efficient ventilation system (43, 44). Room ventilation of 30 min was used to clean the area after a high-risk connection with the infected patient (35). The other study stated that the implemented air supply on the ceiling and wall exhaust that function with the 12-air rate change per hour helped in the extraction of contaminants. The increase in the number of air supply is not beneficial (36).
Five studies emphasized that a HEPA filtration system enhances infection air load prevention (23, 26, 33, 34, 44). Anterooms that are equipped with HEPA filtration system at positive airflow showed a very low level of particles (26). HEPA filtration systems discharge the air, protect the environment, and individuals (23). One study mentioned that the desirable function system was achieved when the filters were checked, cleaned, and replaced frequently. Correcting the position and checking the function of dampers and controlling the exhaust ventilation is necessary (33). HEPA filters with an efficiency rate of 99.97% are able to remove particles ≤ 0.3 μm in diameter. Having a procedure for regular replacement and maintenance of filters by staff to control the efficiency of filters is suggested (44).
Four studies emphasized the efficient and appropriate size of the anteroom to enhance its capability and usability (30, 31, 38, 42). The small size of the anteroom without ventilation and with basic equipment causes a pause during transit and increases the particles transmission (30). The anteroom physical environment and proper size of the anteroom provide the potential to have zones in the anteroom area and divide the zones into spaces to enhance infection control (38, 42). The standard size of the anteroom makes it possible to store PPE and supplies (31).
Two studies pointed out that temperature plays a significant role in controlling airborne contamination. The 2°C temperature difference between the anteroom and isolation room significantly affected the air transfer, especially in the rooms which had sliding doors. When the difference between the two rooms' temperatures is more than 2°C, the risk of transporting contaminated sources to the next room is higher due to thermal convective flows (24, 28).
Eleven studies provided information about the effects of anteroom's equipment on infection control (17, 23, 29, 31, 32, 34, 35, 38, 42–44). The proper environmental design and the placement of equipment significantly affect the contamination risk (38). Anterooms that are well-equipped act as a protective safe barrier for health workers and patients for infection control (34). Seven of eleven studies emphasized hand hygiene importance in the anteroom. Hand disinfection products such as a sink, hand dispensers, and alcohol-based disinfection are considered a protective process necessary for better healthcare workers' protection and prevention of infection transmission (17, 31, 32, 34, 38, 42, 44). In three studies, the placement of hand hygiene dispensers was considered an important factor. The hand dispensers should be implemented on the specific points of the wall which are secured, well-supported, and reachable (38, 42). Automatic dispensers are considered desirable and useful for healthcare workers. Putting several alcohol hand hygiene products is reachable and easy-access points enhance the infection control process (31). Using a hand-free sink for clinical use is recommended for healthcare staff safety (44).
Six studies emphasized the effect of the anteroom and its equipment for donning and doffing the PPE and its impact on infection transmission prevention. Healthcare workers use the anteroom as a place for applying or removing their PPE (23, 32, 35, 42). The donning and doffing area in the anteroom and implementing a border zone for separating this area from others reduce the infection risk and enhance the safety of the environment (35, 42). The suitable settlement of equipment provides a safe doffing situation such as the position of the removal bin that should be in arm's reach (38). Placing a hand railway in the area of donning and doffing, save the healthcare workers' balance and it is easier to disinfect. It also helps to manage and save space (17, 42). Using a mirror in the anteroom helps the medical staff to check and control their PPE material for patient care, anesthesia, medical devices, and sample bottles are placed or disinfected in the anteroom (17, 32, 34, 35). Having a transparent panel such as a window between the isolation room and anteroom enhances the safety of the environment since medical staff can communicate through the transparent panel and the doors are remained closed (17, 38). Windows in the wall or door help the staff to communicate or watch over the patient (44). One study stated that using UVC disinfection considerably decreases the bacterial population on surfaces that were exposed to UVC light directly or indirectly in a short exposure time. The usage of UVC for disinfection decreases the environmental bacterial infection and works as complementary chemical disinfection. The organisms that are not in the direct path of UVC light or in the shadowed area will not be destroyed and need chemical disinfection (42). The packages of equipment should be removed in a clean area and then transfer to the clinical area. Before disposal, solid waste should be disinfected. There are three methods that are suggested for liquid waste disposal, namely, solidification and autoclaving, disinfection with chlorine, and direct disposal to a dirty drain system (43).
Fourteen studies stressed on the necessity and importance of door in the anteroom for infection control and the environmental safety (17, 21, 22, 24, 25, 27, 28, 31, 33, 34, 36, 38, 40, 44). The type of the door and the way that health workers use the door are factors that affect the pressure difference and performance of the anteroom (40). The air and particles exchange happens in the last second of the door closing cycle. With the increase in the door cycle speed, the airflow exchanges between the anteroom and isolation room increased significantly (27). The door of the anteroom should be closed to open the door of the next room. A 30-s pause is needed for stabilizing ventilation before the door opens (31). A self-closing door is recommended for maintaining a constant pressure gradient (44). Two studies emphasized the use of springs or manual systems that are applied to the door to control the unintended door opening and to reduce the door opening time (17, 33). In one study the door gaps between the isolation room and anteroom were shown as a factor that reduces the risk of infection. A 0.003 mm3 door gap reduces the risk of infection transmission compared to a 0.002 mm3 door gap (36).
Five studies focused on the importance of door types (21, 22, 24, 25, 28). Four studies indicated that swinging doors, also known as hinged doors weakens the function of the anterooms and poses a risk of infection. The possibility of infection transmission increased during the door rotation (21, 22, 25, 28). In one study it was mentioned that the use of a filter and fan system (airflow door barrier) near a hinged door area significantly removed the risk of contamination control failure by hinged door movement and reduced the infection risk, but not removed the person transmission. The sliding doors have less leakage compared to the hinged door (25). But both the sliding door and hinged door transfer significant airflow and pathogen from the isolation room to the anteroom and corridor. The volume of air exchange through sliding doors compared to hinged doors is notably lower. The duration of door-open had a relation to air transfer. When the total cycle increased, the amount of air volume exchange also significantly increased (28). In one study it was shown that when the sliding door is placed between the anteroom and the treatment room and the temperature difference is more than 2°C, thermal convection happens through the warm air gathered at the top of the door to the anteroom. So, significant contaminated air exchange between two rooms occurs. An air exhaust duct over the doors will be helpful to control thermal convective flows (24).
In general, according to the data presented in this article, we believe that there is sufficient evidence of an association between the presence of the anteroom (vestibule) area in each ward of the hospital and the decrease in transmission of airborne infectious diseases.
The COVID-19 pandemic and the lightning-fast spread of the extremely deadly virus SARS-CoV2 have impacted the lives of people all over the world. In previous studies, the airborne transmission of SARS-CoV2 has been confirmed (45, 46). The anteroom (vestibule) can be an essential tool to control airborne infections caused by viruses, bacteria, and fungi. Patient rooms, pharmacies, radiology suites, surgical suites, and cafeterias are all situations where an anteroom can help control the airflow between the sensitive patient-occupied area and other physical environments of the hospital. Despite its importance, until now there is no comprehensive study regarding the rule of the anteroom (vestibule) in disease control and prevention in hospitals. In this study, we reviewed all documents published in the English language on the topic across the world.
Our results showed that most of the studies included in this systematic review were conducted in the USA (n = 7/25) and Nordic countries (n = 7/25). This confirmed the fact that these countries are those in which much attention has been paid to the anteroom as an infection control area. Most USA hospitals use anterooms for hospitalized patients with suspected or confirmed airborne transmissible infections. In high-income countries like the USA and Nordic countries, 15% of hospital expenditure goes to the designation of hospital physical environments (e.g., anterooms) to prevent hospital-acquired infections (47). On the other hand, the results demonstrated that the application of anterooms in most countries, particularly low- and middle-income countries, is neglected. So, there is a need for a global platform to share knowledge in this field.
In this study, the predominant investigated infectious organisms in the majority of the imported studies fall into the category of viral infections (n = 8). Viruses, bacteria, and fungi are the etiologic agents of airborne diseases that are caused via transmission through the air. The main reason that in this systematic review viral infections contained most of the studies is the fact that in comparison with fungal and bacterial respiratory infections, respiratory viral infections are more contagious. In other words, in most cases, the mere demonstration of viable fungal or bacterial microorganisms in the air does not establish the occurrence of airborne transmission (48).
On the other hand, prior findings have emphasized the importance of an anteroom on health staff safety and efficiency of hospital systems. Anteroom's equipment has a significant effect on its performance and in reducing virus transmission. Equipment and parameters such as door type, air system, pressure, HEPA filter, temperature, hand dispensers, alcohol-based disinfection, sink, mirror, transparent panel, UVC disinfection, and zone for PPE change are factors that enhance the safety of the environment.
According to our investigation, pressure has a major effect on infection control (17, 26, 27, 31, 35–37, 39, 41). In the guidelines of different countries, various pressure gradients are suggested for the anteroom pressure between the range of negative and positive pressure. The USA, Nordic countries (Norway, Sweden, Finland, and Denmark), and Australia guidelines recommend an isolation room with negative pressure compared to the anteroom, and an anteroom with negative pressure compared with the corridor (47–49), while the UK recommends positive pressure for the anteroom compared to both the isolation room and the corridor (50). Some centers prefer the UK suggestion since an anteroom with positive pressure act as a barrier, blocks the air, and prevents air transmission (31, 51). As a reason, there are centers with different protocols for their anteroom. The centers differ in having positive or negative pressure and in the number of the pressure gradient (44). The various range of pressure gradients through all the centers indicate a need in revising the guidelines (51). The theory behind a negative pressure anteroom is that an anteroom with negative pressure acts as an empty space. So, when the door between an anteroom and corridor is opened, the air from the corridor with positive pressure transfers to the anteroom filling the space (26, 27, 35). By transmitting air from corridor to anteroom, pathogens existing in the hospital environment will transfer to the anteroom. If there is a leakage in an anteroom environment with negative pressure, air and pathogens from other areas would transmit to the anteroom. Then the polluted anteroom with higher pressure than the isolation room will be a danger to the patient who is vulnerable due to being infected with another virus and their weekend immune system (52). Positive pressure anteroom act like an airlock, preventing infection transmission from both corridor and isolation room entering the anteroom (31). Positive pressure anteroom reduces the fungal growth and protects the environment from infections ingress (53). So, the anteroom does not allow the infection from the isolation room to run to the corridor and vice versa. The same goes for the anteroom at the entrance of wards where staff could change their cloth and disinfect themselves after visiting patients. Positive pressure for this anteroom protects the healthcare staff and prevents infection transmission from the corridor to the safe area.
The range of pressure difference between isolation room and anteroom varied between 2.5 and 15 Pa (17, 36, 41), and was sometimes defined as 30 Pa (31). Several factors affect the maintenance of pressure difference such as door opening and closing (41, 54). The low-pressure difference puts a risk on maintaining the pressure condition and increases the risk of pathogens transmission from the isolation room space to the anteroom (55, 56). To ensure pressure difference maintenance and the safety of the anteroom environment, the higher-pressure difference will be more appropriate. It is recommended to set a monitor and alarm system for staff to check the pressure gradient of the environment regularly.
The ventilation system of the isolation unit is another factor that mostly affects the efficiency of the system (21, 23, 26, 31, 33–36, 40). The exhaust and intake air should be on the opposite sides of the room (44). The ventilation system of the isolation unit is separated from other wards and connected to a backup power source. The airflow and pressure gradient flow from the cleanest to the most contaminated area. The air does not circulate again and is exhausted in a site far from the building to reduce the risk of infection transmission for occupants and the community (43, 57).
The rate of air exchange per hour used for the anteroom environment varied between 5 and 18 times per hour (53, 58, 59). The rate of 5 and 6 is considered for a normal situation (60). The rate of more than 12 times per hour is mostly used when a patient with an infectious disease is hospitalized (61, 62). However, with viral infections such as COVID-19, patients may be asymptomatic or suspected to be infected. So, defining a normal situation would be doubtful. Setting the normal based on an air exchange more than 12 times per hour is required to have an efficient ventilation system and to be ensured patients' and healthcare staff's safety (43, 63). Room size is directly related to the amount of air conditioning per hour. As the volume of the room increases, the number of air changes also increases (64). The rate of air exchange for anterooms at the entrance of each ward should be regulated based on high human traffic.
High-efficiency particulate absorption (HEPA) filters are suggested to be used in the anteroom with the high efficiency at the exhaust air and are mandatory for places with the risk of circulation of exhaust air (23, 26, 33, 34, 43, 57, 65). The HEPA filters should be secured by prefilters and placed to have easy access for maintenance and checking (43, 66). Having a procedure for regular replacement and maintenance of filters by staff to control the efficiency of filters is suggested (44, 67). In the anteroom environment, HEPA filters are suggested for implementation on supply air (43). It seems that for the anteroom environment HEPA filters are used for both intake and exhaust air, and if the infrastructure is not accessible, portable HEPA filters would be a suitable solution (21). However, the portable filters might affect the circulation of airflow. Also, the regular changing and checking of filters have high importance in making a system reliable and efficient.
In addition, the temperature and size of the anteroom affect the factors mentioned above (68, 69). Increasing the temperature difference between the rooms, cause a convection current which increases the air transmission (24, 28, 70). It is recommended that the temperature difference between the anteroom and surrounding environments be minimized. The size of the anteroom plays an important role in infection prevention. The small anterooms do not provide a safe environment (71). The anteroom should be large enough to separate clean areas from contaminated spaces, such as spaces for used clothing, by zoning (38). Also, the anteroom should have places for storage of patient care equipment such as sampling glasses and staff PPE is required (42, 72). Therefore, it is suggested to set a standard for the anteroom space in the guidelines. So, people can have proper circulation in the designated zones and the possibility to do safety protection protocols.
A well-equipped anteroom assists the healthcare staff to enhance the efficiency and safety of the system (73). Most studies emphasize the practical usage of storage for supplies, PPE, mirrors, hand railways, hand-free sinks, several alcohol-based hand dispensers, transparent panels or windows, and zones for clean or contaminated areas (17, 23, 30–32, 34, 35, 38, 42). The anteroom is necessary for hospital staff to change and wear PPE, gloves, headwear, plastic footwear cover, and eye protection. Materials used for the patient should be disinfected with soap or bleach (74). A door or panel with a large sealed window is beneficial for healthcare workers for checking patients and communicating with other healthcare workers (59). Implementing UVC light for disinfection would be a suitable solution to disinfect anteroom areas in compliance with chemical disinfection materials as it reduces the air pollution while no one is present in the anteroom (75, 76). It is recommended that communication devices be placed between the two spaces so that people can talk through them and see the opposite side through the glass space to minimize the opening of the doors. For future research, researchers could investigate the UVC light implementation in the anteroom. Determining the number and distance of UVC lights based on the anteroom dimensions for maximum safety might be examined.
Another factor for maintaining the safety, pressure gradient, and preventing air transmission of anteroom is the door type (17, 18, 21, 24, 25, 27, 28, 31, 33, 34, 36, 38, 40). Research showed the use of sliding doors reduced the particle transmission between environments (77). Self-closing doors are recommended for applying in the anteroom (78). Also, when the two doors of the anteroom and isolation room are interlocked, it avoids air transmission and enhances safety and infection prevention (79). Using electronic identity cards for controlling access reduces the unnecessary door opening (59).
In this study, we aimed to assess the necessity to construct an anteroom area for hospital staff members at the entrance of each ward of the hospital, and specify the equipment and facilities which make the anteroom more efficient. Based on our analyses, anterooms are effective in controlling infections and providing a suitable environment for staff's health and safety. For a reason, implementing spaces that perform and are equipped as an anteroom, and having a shower, at the entrance of each ward for health staff safety can significantly increase the safety of the healthcare staff at work. Furthermore, the results showed that the equipment such as ventilation system, HEPA filter, hand dispensers, alcohol-based disinfection, sink, mirror, transparent panel, UVC disinfection, and zone for PPE change, and parameters like temperature, door type, pressure, and size of the anteroom are factors that are effective on the safety of the hospital environment. Despite their importance, guidance on the construction of anterooms is less than clear. Also, the lack of education and communication about guidelines, infection control, and methods and equipment for safety such as PPE might cause problems and worries for healthcare staff. Setting an education about protocols and systems would be a helpful source to cope with crisis conditions. To the best of our knowledge, this study was the first comprehensive study to assess the necessity to construct an anteroom area for controlling airborne nosocomial infections during hospitalization and specify the equipment and facilities which make the anteroom more efficient. Since SARS-CoV2 share the common modes of transmission of most airborne viruses, namely, SARS-CoV, MERS-CoV, and influenza virus, it can be concluded that a well-designed anteroom could potentially decrease the risk of SARS-CoV2 transmission from person to person during hospitalization as during the current pandemic.
The necessity to construct an anteroom area in crowded places of hospitals, and specifying the equipment and facilities which make the anteroom more efficient in this situation was not described in this study that is suggested to be examined in future studies.
EA and ZR supervised the study and wrote the manuscript. MF, SZ, MS, and MK were involved in planning and supervising the findings of this work. HS contributed to the search strategy. All authors discussed the results, contributed to the final manuscript, and approved the submitted version.
The authors declare that the research was conducted in the absence of any commercial or financial relationships that could be construed as a potential conflict of interest.
All claims expressed in this article are solely those of the authors and do not necessarily represent those of their affiliated organizations, or those of the publisher, the editors and the reviewers. Any product that may be evaluated in this article, or claim that may be made by its manufacturer, is not guaranteed or endorsed by the publisher.
1. WHO. Interim Infection Prevention and Control Guidance for Care of Patients with Suspected or Confirmed Filovirus Haemorrhagic Fever in Health-Care Settings, With Focus on Ebola. World Heal Organ (2014). p. 1–27. Available online at: http://apps.who.int/iris/bitstream/10665/130596/1/WHO_HIS_SDS_2014.4_eng.pdf?ua=1&ua=1&ua=1 (accessed April 08, 2022).
2. Prevention C for DC and Guidance for Donning and Doffing Personal Protective Equipment (PPE) During Management of Patients With Ebola Virus Disease in US Hospitals (2015).
3. Riediker M, Tsai DH. Estimation of Viral Aerosol Emissions From Simulated Individuals With Asymptomatic to Moderate Coronavirus Disease 2019. JAMA Netw open. (2020) 3:e2013807. doi: 10.1001/jamanetworkopen.2020.13807
4. Moradian N, Ochs HD, Sedikies C, Hamblin MR, Camargo CA, Martinez JA, et al. The urgent need for integrated science to fight COVID-19 pandemic and beyond. J Transl Med. (2020) 18:1–7. doi: 10.1186/s12967-020-02364-2
5. Salehi M, Ahmadikia K, Badali H, Khodavaisy S. Opportunistic fungal infections in the epidemic area of COVID-19: a clinical and diagnostic perspective from Iran. Mycopathologia. (2020) 185:607–11. doi: 10.1007/s11046-020-00472-7
6. Jensen PA, Lambert LA, Iademarco MF, Ridzon R. Guidelines for preventing the transmission of Mycobacterium tuberculosis in health-care settings. MMWR Recomm Rep. (2005) 54:1–141.
7. Morawska L. Droplet fate in indoor environments, or can we prevent the spread of infection? Indoor Air. (2006) 16:335–47. doi: 10.1111/j.1600-0668.2006.00432.x
8. Embil JM, Dyck B, Plourde P. Prevention and control of infections in the home. CMAJ. (2009) 180:E82–6. doi: 10.1503/cmaj.071898
9. Stelfox HT, Bates DW, Redelmeier DA. Safety of Patients Isolated for Infection Control. J Am Med Assoc. (2003) 290:1899–905. doi: 10.1001/jama.290.14.1899
10. Garner JS. Guideline for Isolation Precautions in Hospitals. Infect Control Hosp Epidemiol. (1996) 17:54–80. doi: 10.1017/S0195941700006123
11. Van Doremalen N, Bushmaker T, Morris DH, Holbrook MG, Gamble A, Williamson BN, et al. Aerosol and surface stability of SARS-CoV-2 as compared with SARS-CoV-1. N Engl J Med. (2020) 382:1564–7. doi: 10.1056/NEJMc2004973
12. Amin S. The psychology of coronavirus fear: are healthcare professionals suffering from corona-phobia? Int J Healthc Manag. (2020) 13:1–8. doi: 10.1080/20479700.2020.1765119
13. Ulrich RS, Zimring C, Zhu X, DuBose J, Seo HB, Choi YS, et al. A Review of the Research Literature on Evidence-Based Healthcare Design (Part I) Abstract Introduction Background. Health Environ Res Des. (2008) 1(Part I):61–125. doi: 10.1177/193758670800100306
14. Montecalvo MA, Jarvis WR, Uman J, Shay DK, Petrullo C, Rodney K, et al. Infection-control measures reduce transmission of vancomycin-resistant Enterococci in an endemic setting. Ann Intern Med. (1999) 131:269–72. doi: 10.7326/0003-4819-131-4-199908170-00006
15. Tay JK, Khoo MLC, Loh WS. Surgical Considerations for Tracheostomy During the COVID-19 Pandemic: Lessons Learned From the Severe Acute Respiratory Syndrome Outbreak. JAMA Otolaryngol Neck Surg. (2020) 146:517–8. doi: 10.1001/jamaoto.2020.0764
16. Ong SWX, Tan YK, Chia PY, Lee TH, Ng OT, Wong MSY, et al. Air, Surface Environmental, and Personal Protective Equipment Contamination by Severe Acute Respiratory Syndrome Coronavirus 2 (SARS-CoV-2) From a Symptomatic Patient. JAMA. (2020) 323:1610–2. doi: 10.1001/jama.2020.3227
17. Lee JK, Jeong HW. Rapid expansion of temporary, reliable airborne-infection isolation rooms with negative air machines for critical COVID-19 patients. Am J Infect Control. (2020) 48:822–4. doi: 10.1016/j.ajic.2020.04.022
18. Shon S, Cho H, Sung M, Kang J, Choi Y, Lee S, et al. Operationalization of an Expanded Anteroom in a COVID-19 Dedicated Hospital, South Korea. Preprints. (2020) 1:2020120485. doi: 10.20944/preprints202012.0485.v1
19. D. Moher L, Shamseer M, Clarke D, Ghersi A, Liebrati M, Petticrew, et al. Preferred reporting items for systematic review and meta-analysis protocols (PRISMA-P) 2015 statement. Syst Rev. (2015) 4:1. doi: 10.1186/2046-4053-4-1
20. Vandenbroucke JP, von Elm E, Altman DG, Gøtzsche PC, Mulrow CD, Pocock S, et al. Strengthening the Reporting of Observational Studies in Epidemiology (STROBE): explanation and elaboration. Int J Surg. (2014) 12:1500–24. doi: 10.1016/j.ijsu.2014.07.014
21. Mousavi ES, Pollitt KJ, Sherman J, Martinello RA. Performance analysis of portable HEPA filters and temporary plastic anterooms on the spread of surrogate coronavirus. Build Environ. (2020) 183:107186. doi: 10.1016/j.buildenv.2020.107186
22. Hang J, Li Y, Ching WH, Wei J, Jin R, Liu L, et al. Potential airborne transmission between two isolation cubicles through a shared anteroom. Build Environ. (2015) 89:264–78. doi: 10.1016/j.buildenv.2015.03.004
23. Fusco FM, Schilling S, De Iaco G, Brodt HR, Brouqui P, Maltezou HC, et al. Infection control management of patients with suspected highly infectious diseases in emergency departments: data from a survey in 41 facilities in 14 European countries. BMC Infect Dis. (2012) 12:1–7. doi: 10.1186/1471-2334-12-27
24. Beauchêne C, Laudinet N, Choukri F, Rousset JL, Benhamadouche S, Larbre J, et al. Accumulation and transport of microbial-size particles in a pressure protected model burn unit: CFD simulations and experimental evidence. BMC Infect Dis. (2011) 11:1–8. doi: 10.1186/1471-2334-11-58
25. Venås B, Vikan AW, Lind MC, Harsem TT. Air flow door barrier for airborne infection isolation rooms. (2019). Available online at: https://www.scopus.com/inward/record.uri?eid=2-s2.0-85071885783&doi=10.1051%2Fe3sconf%2F201911102063&partnerID=40&md5=7040f698f745cc3a7e5a1d55fa16af79 (accessed April 08, 2022).
26. Araujo R, Cabral JP, Rodrigues AG. Air filtration systems and restrictive access conditions improve indoor air quality in clinical units: Penicillium as a general indicator of hospital indoor fungal levels. Am J Infect Control. (2008) 36:129–34. doi: 10.1016/j.ajic.2007.02.001
27. Mousavi ES, Grosskopf KR. Airflow patterns due to door motion and pressurization in hospital isolation rooms. Sci Technol Built Environ. (2016) 22:379–84. doi: 10.1080/23744731.2016.1155959
28. Kalliomäki P, Saarinen P, Tang JW, Koskela H. Airflow patterns through single hinged and sliding doors in hospital isolation rooms. Int J Ventilation. (2015) 14:111–26. doi: 10.1080/14733315.2015.11684074
29. Andersen BM, Bånrud H, Bøe E, Bjordal O, Drangsholt F. Comparison of UV C light and chemicals for disinfection of surfaces in hospital isolation units. Infect Cont Hosp Epidemiol. (2006) 27:729–34. doi: 10.1086/503643
30. Johnson DL, Lynch RA, Mead KR. Containment effectiveness of expedient patient isolation units. Am J Infect Control. (2009) 37:94–100. doi: 10.1016/j.ajic.2008.05.011
31. Holmdahl T, Lanbeck P. Design for the post-antibiotic era: Experiences from a new building for infectious diseases in Malmö, Sweden. HERD. (2013) 6:27–52. doi: 10.1177/193758671300600403
32. Bin SY, Heo JY, Song MS, Lee J, Kim EH, Park SJ, et al. Environmental contamination and viral shedding in MERS patients during MERS-CoV outbreak in South Korea. Clin Infect Dis. (2016) 62:755–60. doi: 10.1093/cid/civ1020
33. Fraser VJ, Johnson K, Primack J, Jones M, Medoff G, Dunagan WC. Evaluation of rooms with negative pressure ventilation used for respiratory isolation in seven midwestern hospitals. Infect Cont Hosp Epidemiol. (1993) 14:623–8. doi: 10.2307/30149744
34. Ippolito G, Nicastri E, Capobianchi MR, Di Caro A, Petrosillo N, Puro V. Hospital preparedness and management of patients affected by viral haemorrhagic fever or smallpox at the Lazzaro Spallanzani Institute, Italy. Eurosurveillance. (2005) 10:1–2. doi: 10.2807/esm.10.03.00523-en
35. Park J, Yoo SY, Ko JH, Lee SM, Chung YJ, Lee JH, et al. Infection prevention measures for surgical procedures during a Middle East respiratory syndrome outbreak in a tertiary care hospital in South Korea. Sci Rep. (2020) 10:1–8. doi: 10.1038/s41598-019-57216-x
36. Tung YC, Hu SC. Infection risk of indoor airborne transmission of diseases in multiple spaces. Archit Sci Rev. (2008) 51:14–20. doi: 10.3763/asre.2008.5103
37. Emmerich SJ, Heinzerling D, Choi JI, Persily AK. Multizone modeling of strategies to reduce the spread of airborne infectious agents in healthcare facilities. Build Environ. (2013) 60:105–15. doi: 10.1016/j.buildenv.2012.11.013
38. Herlihey TA, Gelmi S, Flewwelling CJ, Hall TN, Bañez C, Morita PP, et al. Personal protective equipment for infectious disease preparedness: a human factors evaluation. infect Cont Hosp Epidemiol. (2016) 37:1022–8. doi: 10.1017/ice.2016.124
39. Mousavi ES, Grosskopf KR. Secondary exposure risks to patients in an airborne isolation room: Implications for anteroom design. Build Environ. (2016) 104:131–7. doi: 10.1016/j.buildenv.2016.05.010
40. Kokkonen A, Hyttinen M, Holopainen R, Salmi K, Pasanen P. Performance testing of engineering controls of airborne infection isolation rooms by tracer gas techniques. Indoor Built Environ. (2014) 23:994–1001. doi: 10.1177/1420326X13499173
41. Adams NJ, Johnson DL, Lynch RA. The effect of pressure differential and care provider movement on airborne infectious isolation room containment effectiveness. Am J Infect Control. (2011) 39:91–7. doi: 10.1016/j.ajic.2010.05.025
42. Herlihey TA, Gelmi S, Cafazzo JA, Hall TN. The impact of environmental design on doffing personal protective equipment in a healthcare environment: a formative human factors trial. infect Cont Hosp Epidemiol. (2017) 38:712–7. doi: 10.1017/ice.2017.68
43. Bannister B, Puro V, Fusco FM, Heptonstall J, Ippolito G. EUNID Working Group. Framework for the design and operation of high-level isolation units: consensus of the European Network of Infectious Diseases. Lancet infect Dis. (2009) 9:45–56. doi: 10.1016/S1473-3099(08)70304-9
44. Styczynski J, Tridello G, Donnelly JP, Iacobelli S, Hoek J, Mikulska M, et al. Protective environment for hematopoietic cell transplant (HSCT) recipients: The Infectious Diseases Working Party EBMT analysis of global recommendations on health-care facilities. Bone Marrow Transplant. (2018) 53:1131–8. doi: 10.1038/s41409-018-0141-5
45. Rabaan AA, Al-Ahmed SH, Al-Malkey MK, Alsubki RA, Ezzikouri S, Al-Hababi FH, et al. Airborne transmission of SARS-CoV-2 is the dominant route of transmission: droplets and aerosols. Infez Med. (2021) 29:10–9.
46. Rogers B, Buckheit K, Ostendorf J. Ergonomics and nursing in hospital environments. Workplace Health Saf. (2013) 61:429–39. doi: 10.1177/216507991306101003
47. VACIC. Guidelines for the Classification and Design of Isolation Rooms in Health Care Facilities. Vic Advis Comm Infect Control (2007). Available online at: http://www.health.vic.gov.au/ideas/regulations (accessed April 08, 2022).
48. CDC. Healthcare Infection Control Practices Advisory Committee (HICPAC): Guidelines for Environmental Infection Control in Health-Care Facilities. US Dep Heal Hum Serv Centers Dis Control Prev Atlanta, GA 30329. (2003). p. 1–235. Available from: http://www.cdc.gov/hicpac/pdf/guidelines/eic_in_hcf_03.pdf (accessed April 08, 2022).
49. Lingaas E, Rydock JP. Best practice in design and testing of isolation rooms in Nordic hospitals. Round Table Ser - R Soc Med. (2007) 87–107.
50. Department of Health. Health Building Note 04-01. Supplement 1: Isolation Facilities for Infectious Patients in Acute Settings. Natl Heal Serv (2009). Available online at: https://www.gov.uk/government/uploads/system/uploads/attachment_data/file/148503/HBN_04-01_Supp_1_Final.pdf (accessed April 08, 2022).
51. Ms SSS, Baracco G, Fennelly KP. Isolation anterooms: Important components of airborne infection control. Am J Infect Control. (2020) 41:452–5. doi: 10.1016/j.ajic.2012.06.004
52. Al-Benna S. Negative pressure rooms and COVID-19. J Perioper Pract. (2021) 31:18–23. doi: 10.1177/1750458920949453
53. Collier L, Bright-Thomas R, Richardson M, Jones A. P251 Environmental fungal sampling in a cystic fibrosis centre. BMJ. (2017) 72:A220.1-A220. doi: 10.1136/thoraxjnl-2017-210983.393
54. Beggs CB. The Use of Engineering Measures to Control Airborne Pathogens in Hospital Buildings. (2002). Available online at: http://www.efm.leeds.ac.uk/CIVE/MTB/CBB-Nov8.pdf
55. Zia H, Singh R, Seth M, Ahmed A, Azim A. Engineering Solutions for Preventing Airborne Transmission in Hospitals with Resource Limitation and Demand Surge. Indian J Crit Care Med. (2021) 25:453. doi: 10.5005/jp-journals-10071-23792
56. Qian H, Zheng X. Ventilation control for airborne transmission of human exhaled bio-aerosols in buildings. J Thorac Dis. (2018) 10:S2295–304. doi: 10.21037/jtd.2018.01.24
57. Mousavi ES, Kananizadeh N, Martinello RA, Sherman JD. COVID-19 Outbreak and Hospital Air Quality: A Systematic Review of Evidence on Air Filtration and Recirculation. Environ Sci Technol. (2021) 55:4134–47. doi: 10.1021/acs.est.0c03247
58. Wang CH, Kuo NW. Post-occupancy evaluation of negative-pressure isolation rooms: Using the balanced scorecard framework. J Archit Plann Res. (2009) 26:1–13.
59. Mora-Rillo M, Arsuaga M, Ramírez-Olivencia G, de la Calle F, Borobia AM, Sánchez-Seco P, et al. Acute respiratory distress syndrome after convalescent plasma use: Treatment of a patient with Ebola virus disease contracted in Madrid, Spain. Lancet Respir Med. (2015) 3:554–62. doi: 10.1016/S2213-2600(15)00180-0
60. Whicker JJ, Wasiolek PT, Tavani RA. Influence of room geometry and ventilation rate on airflow and aerosol dispersion: implications for worker protection. Health Phys. (2002) 82:52–63. doi: 10.1097/00004032-200201000-00007
61. Li Y, Huang X, Yu ITS, Wong TW, Qian H. Role of air distribution in SARS transmission during the largest nosocomial outbreak in Hong Kong. Indoor Air. (2005) 15:83–95. doi: 10.1111/j.1600-0668.2004.00317.x
62. Pandey N, Kaushal V, Puri GD, Taneja S, Biswal M, Mahajan P, et al. Transforming a General Hospital to an Infectious Disease Hospital for COVID-19 Over 2 Weeks. Front Public Heal. (2020) 8:1–8. doi: 10.3389/fpubh.2020.00382
63. Ninomura P, Bartley J. New ventilation guidelines for health-care facilities. ASHRAE J. (2001) 43:29.
64. Cherrie JW. The Effect of Room Size and General Ventilation on the Relationship Between Near and Far-Field Concentrations. Appl Occup Environ Hyg. (1999) 14:539–46. doi: 10.1080/104732299302530
65. Truesdell M, Guttman P, Clarke B, Wagner S, Bloom J, DuShane J, et al. Conversion of positive-pressure cardiac catheterization and electrophysiology laboratories to a novel 2-zone negative-pressure system during COVID-19 pandemic. J Cardiovasc Electrophysiol. (2020) 31:1901–3. doi: 10.1111/jce.14579
66. Figuerola-Tejerina A, Hernández-Aceituno A, Alemán-Vega G, Orille-García C, Ruiz-Álvarez M, Sandoval-Insausti H. Developing a faster way to identify biocontamination in the air of controlled environment rooms with HEPA filters: airborne particle counting. Sci Rep. (2020) 10:1–8. doi: 10.1038/s41598-020-59367-8
67. Lednicky JA, Lauzardo M, Fan ZH, Jutla A, Tilly TB, Gangwar M, et al. Viable SARS-CoV-2 in the air of a hospital room with COVID-19 patients. Int J Infect Dis. (2020) 100:476–82. doi: 10.1016/j.ijid.2020.09.025
68. Cunha AG, Peixoto TL, Gomes LCP, Bastos VDS, Cavalcanti TP, GusmÃo-Cunha AM. How to prepare the operating room for COVID-19 patients. Rev Col Bras Cir. (2020) 47:e20202575. doi: 10.1590/0100-6991e-20202575
69. Saran S, Gurjar M, Baronia A, Sivapurapu V, Ghosh PS, Raju GM, et al. Heating, ventilation and air conditioning (HVAC) in intensive care unit. Crit Care. (2020) 24:1–11. doi: 10.1186/s13054-020-02907-5
70. Kalliomäki P. Exchange Airflows Through Doorways Induced by Door Opening and Occupant Movement. Docoral thesis: Alto University of Medical Engineering, Finland (2021).
71. Mousavi ES, Bausman D. Renovation in Hospitals: Pressurization Strategies by Healthcare Contractors in the United States. Heal Environ Res Des J. (2020) 13:179–90. doi: 10.1177/1937586719861557
72. Esswein EJ, Kiefer M, Wallingford K, Burr G, Lee LJH, Wang J, et al. Environmental and occupational health response to SARS, Taiwan, 2003. Emerg Infect Dis. (2004) 10:1187–94. doi: 10.3201/eid1007.030728
73. Sykes A. An International Review of High Level Isolation Units. The Newcastle upon Tyne Hospitals NHS Foundation Trust (2018). Avialable online at: https://media.churchillfellowship.org/documents/Sykes_A_Report_2018_Final.pdf
74. Van Paassen J, Bauer MP, Arbous MS, Visser LG, Schmidt-Chanasit J, Schilling S, et al. Acute liver failure, multiorgan failure, cerebral oedema, and activation of proangiogenic and antiangiogenic factors in a case of Marburg haemorrhagic fever. Lancet Infect Dis. (2012) 12:635–42. doi: 10.1016/S1473-3099(12)70018-X
75. Guimera D, Trzil J, Joyner J, Hysmith ND. Effectiveness of a shielded ultraviolet C air disinfection system in an inpatient pharmacy of a tertiary care children's hospital. Am J Infect Control. (2018) 46:223–5. doi: 10.1016/j.ajic.2017.07.026
76. McDevitt JJ, Milton DK, Rudnick SN, First MW. Inactivation of poxviruses by upper-room UVC Light in a simulated hospital room environment. PLoS ONE. (2008) 3:e3186. doi: 10.1371/journal.pone.0003186
77. Kalliomäki P, Saarinen P, Koskela H. Effect of door opening in hospital isolation room. 45th R3 Nordic Symposium: Cleanroom technology, contamination control and cleaning. 3:99.
78. Rao SKM. Designing hospital for better infection control: An experience. Med J Armed Forces India. (2004) 60:63–6. doi: 10.1016/S0377-1237(04)80163-1
Keywords: anteroom, vestibule, airborne infection, nosocomial infection, SARS-CoV2, hospitalization, healthcare safety
Citation: Andalib E, Faghani M, Zia Ziabari SM, Shenagari M, Salehiniya H, Keivanlou MH and Rafat Z (2022) The Effectiveness of the Anteroom (Vestibule) Area on Hospital Infection Control and Health Staff Safety: A Systematic Review. Front. Public Health 10:828845. doi: 10.3389/fpubh.2022.828845
Received: 07 December 2021; Accepted: 22 March 2022;
Published: 26 April 2022.
Edited by:
Andres M. Rubiano, El Bosque University, ColombiaReviewed by:
Marcello Benevento, University of Bari Aldo Moro, ItalyCopyright © 2022 Andalib, Faghani, Zia Ziabari, Shenagari, Salehiniya, Keivanlou and Rafat. This is an open-access article distributed under the terms of the Creative Commons Attribution License (CC BY). The use, distribution or reproduction in other forums is permitted, provided the original author(s) and the copyright owner(s) are credited and that the original publication in this journal is cited, in accordance with accepted academic practice. No use, distribution or reproduction is permitted which does not comply with these terms.
*Correspondence: Zahra Rafat, ZHIuemFocmEtcmFmYXRAZ3Vtcy5hYy5pcg==; cmFmYXQuemFocmEyMDE1QGdtYWlsLmNvbQ==
Disclaimer: All claims expressed in this article are solely those of the authors and do not necessarily represent those of their affiliated organizations, or those of the publisher, the editors and the reviewers. Any product that may be evaluated in this article or claim that may be made by its manufacturer is not guaranteed or endorsed by the publisher.
Research integrity at Frontiers
Learn more about the work of our research integrity team to safeguard the quality of each article we publish.