- 1Technische Universität Braunschweig, Institut für Biochemie, Biotechnologie und Bioinformatik, Abteilung Biotechnologie, Braunschweig, Germany
- 2Leibniz Institute DSMZ-German Collection of Microorganisms and Cell Cultures, Braunschweig, Germany
- 3Universidade Federal do Agreste de Pernambuco, Curso de Medicina Veterinária, Garanhuns, Brazil
- 4Laboratório de Imunologia Aplicada, Núcleo de Biotecnologia, Centro de Desenvolvimento Tecnológico, Universidade Federal de Pelotas, Pelotas, Brazil
Listeria monocytogenes is the causative agent of listeriosis, a highly lethal disease initiated after the ingestion of Listeria-contaminated food. This species comprises different serovars, from which 4b, 1/2a, and 1/2b cause most of the infections. Among the different proteins involved in pathogenesis, the internalins A (InlA) and B (InlB) are the best characterized, since they play a major role in the enterocyte entry of Listeria cells during early infection. Due to their covalent attachment to the cell wall and location on the bacterial surface, along with their exclusive presence in the pathogenic L. monocytogenes, these proteins are also used as detection targets for this species. Even though huge advancements were achieved in the enrichment steps for subsequent Listeria detection, few studies have focused on the improvement of the antibodies for immunodetection. In the present study, recombinant InlA and InlB produced in Escherichia coli were used as targets to generate antibodies via phage display using the human naïve antibody libraries HAL9 and HAL10. A set of five recombinant antibodies (four against InlA, and one against InlB) were produced in scFv-Fc format and tested in indirect ELISA against a panel of 19 Listeria strains (17 species; including the three main serovars of L. monocytogenes) and 16 non-Listeria species. All five antibodies were able to recognize L. monocytogenes with 100% sensitivity (CI 29.24–100.0) and specificity (CI 88.78–100.0) in all three analyzed antibody concentrations. These findings show that phage display-derived antibodies can improve the biological tools to develop better immunodiagnostics for L. monocytogenes.
Introduction
The Listeria genus contains a total of 20 species, from which 14 have been described in the last decade (1). Among these, Listeria monocytogenes is the major causative agent of listeriosis in humans. This species has 13 different serovars, from which the ones named 4b, 1/2a, and 1/2b are responsible for at least 95% of the infections (2). Due to the ubiquitous nature of this Gram-positive, facultative anaerobic, non-sporulating, bacterium it is virtually impossible to eliminate it from certain environments. This is a problem in particular for food production facilities since the resistance to a broad range of pH, salt concentration, biofilm formation, and temperature change allows this bacterium to be often present in food, especially those uncooked or ready-to-eat products (3–5).
The recent description of many Listeria species already indicates the growing importance of studies about this bacterial genus (1, 6–8). In addition, despite the food safety surveillance performed in Europe and the USA, there is still a considerable number of outbreaks being reported. In 2021 alone, one outbreak was reported in the USA, with 1 death amongst 11 infected people (9), while in Europe a surveillance report indicates that listeriosis will tend to increase in the coming years after 2,502 cases and 222 deaths were described across several European countries in 2017 (10).
As a foodborne disease, the development of listeriosis requires the bacterial cells to reach the gastrointestinal tract to start the infection. Receptors on intestinal cells are recognized by a group of proteins on the bacterial surface that promotes entry into the host's cells (11). The main bacterial proteins involved with this host's cell invasion are internalins A (InlA) and B (InlB), which are covalently attached to the cell wall and are only present in members of the pathogenic species L. monocytogenes and some strains of Listeria ivanovii (12). Therefore, as these proteins play a major role in pathogenesis and are easily accessed on the bacterial surface, they are the most studied targets on pathogenesis and development of biological reagents for the detection of Listeria.
The standard Listeria detection is based on microbiological culture and biochemical characterization, which involve an initial enrichment step followed by the detection itself (13). Although this method is very precise, it takes about seven days from sample collection to result, which often is too slow for monitoring many food production processes. To overcome this problem, strategies to improve the enrichment of bacteria from samples have been successful, such as the use of a Pathogen Enrichment Device (PED) (14). For the following detection step, molecular techniques such as PCR have also shown a good performance, although it requires trained personal, and specialized infrastructure to perform the tests, e.g., thermocycler, fluorescence detector, and electrophoresis apparatus (15). To overcome these limitations for Listeria detection, immunodetection with ELISA or lateral flow-based techniques, which rely on specific and sensitive monoclonal antibodies (mAbs), offer a way for improvement.
While the recent years brought significant advancements on the enrichment step for Listeria identification, the generation of suitable mAbs for Listeria detection has not been addressed accordingly. Most of the molecules already described relied on classical hybridoma technology for their production, which, although useful, is often limited in providing high number of potentially useful binders (16–18). In contrast, phage display explores a vast repertoire of binders using a library-based system for acquiring antibodies (19) and, thus, is a technique that allows the generation of antibodies against virtually any target when using a naïve antibody phage library, the so-called “single-pot” antibody libraries (20). However, this procedure has been scarcely explored for the generation of suitable antibodies for Listeria detection.
In addition to the detection of the pathogenic Listeria species, distinction from the non-pathogenic species is also important, as the same food sample source may contain both of them (21). This way, it has been described that non-pathogenic species, mainly Listeria innocua, can overgrow L. monocytogenes, leading to potentially false-negative results (21). To avoid these unwanted results, targets present in all Listeria species, such as flagellin (18) or FBA (22) can be used. Aligned with this, we have previously described the new biomarker pyruvate dehydrogenase complex–enzyme 2 (PDC-E2) for Listeria spp., and used phage display for the generation of antibodies against it, which allowed the detection of all tested Listeria species (23).
The aim of this study was to generate recombinant antibodies against the targets internalin A (InlA) and B (InlB), which are known to be involved in Listeria pathogenesis. These proteins are mainly present in L. monocytogenes and, thus, are capable of providing species-specificity. In line with this, four recombinant mAbs against InlA and one against InlB showed high sensitivity and specificity when tested against the three most prevalent L. monocytogenes serovars (4b, 1/2a, and 1/2b), as well as against most of the remaining Listeria spp. and 15 non-Listeria species. Thus, the combination of antibodies against InlA and InlB with those previously described against Listeria spp. could be employed to perform tests that distinguish pathogenic from non-pathogenic Listeria in parallel.
Materials and Methods
Expression in Escherichia coli, and Purification of Recombinant InlA and InlB
The plasmids pAE-inlA and pET28a-inlB for the recombinant protein expression of InlA and InlB in E. coli, respectively, were constructed and described previously (24, 25). E. coli BLR(DE3) (Novagen) containing the individual plasmids was grown in Luria-Bertani (LB) broth with 100 μg/mL ampicillin for 16 h at 37°C while shaking at 200 RPM. Cells were inoculated in 500 mL LB and grown until OD600 = 0.5–0.8 before IPTG was added to a final concentration of 0.15 mM. Expression was induced for 4 h under the same conditions before the cells were harvested (16,000 x g, 10 min, 4°C), suspended in lysis buffer (Tris 20 mM, NaCl 0.5 M, Imidazole 5 mM, pH 7.9), sonicated, and the supernatant was used for Ni+2-affinity purification using Ni-Sepharose (GE Healthcare). Purification washing was performed with the same buffer containing 20 mM Imidazole, while elution contained 500 mM imidazole. Eluted fractions were quantified via spectrophotometry at 280 nm, pooled, and dialyzed against phosphate-buffered saline (PBS) using a previously described protocol for the gradual removal of NaCl and Imidazole (26).
Antibody Panning on Purified rInlA and rInlB and Monoclonal scFv Screening
For the generation of antibodies against rInlA and rInlB, one well of an ELISA Costar plate was coated with 1 μg of the protein diluted in 150 μL of PBS. In parallel, one additional well was coated with Panning Block solution [1% (w/v) skimmed milk powder, 1% (w/v) BSA, diluted in PBS containing 0.05% (v/v) Tween20] for pre-incubation. The remaining procedure was identical to that described elsewhere (27) with libraries HAL9 and HAL10 (28) mixed in the same panning well, using three panning rounds. The strain E. coli TG1 was used to perform the panning rounds, while E. coli XL1-Blue MRF' was used to produce soluble scFv in 96-well plates. Screening of soluble monoclonal scFv in ELISA was performed as previously described, using L. monocytogenes ATCC 7644, and Bacillus subtilis 168 NCIB 10106 as living bacteria coated onto the plates. The latter species was chosen because Bacillus is phylogenetically related to Listeria (29), and, thus, could provide a different set of antigens as negative control of the binders. Additional plates coated with 200 ng/well of either the respective recombinant protein or BSA, diluted in PBS, were used as a positive and negative control, respectively.
Production of scFv-Fc in HEK293 Cells, Immunoblot and Indirect ELISA
The scFv binding specifically to L. monocytogenes in the screening ELISA were sequenced and subcloned into pCSE2.6 vector (30) for expression in Expi293F (Thermo Scientific). The scFv-Fc were produced with mouse IgG2a Fc using a previous protocol (31). When using this expression vector, the Fc region does not cause a change in the binding properties even though it does not have the same species of origin as the scFv (30). Thus, the murine Fc was chosen because the potential application of this mAbs would require mouse Fc detection and because there was no plan to use them for human treatment. Later, the mAbs were purified with protein A-affinity and buffer exchanged to PBS. The applicability of the antibodies in immunoblot was tested after running 1 μg of recombinant protein in a 12% SDS-PAGE, and transferring the proteins to a PVDF membrane (Roth) activated with methanol. The membrane was blocked with 2% (w/v) skimmed milk powder diluted in PBS with 0.05% (v/v) Tween20 (2% MPBS-T) for 16 h at 4°C. Afterwards, 1 μg/mL of each of the scFv-Fc was diluted in blocking buffer and incubated for 1 h at RT, followed by the incubation of goat anti-mouse IgG Fc-specific HRP-conjugated (1:40,000; Sigma), and DAB solution (6 mg 3.3-diaminobenzidine tetrahydrochloride; 10 μL 30% (v/v) H2O2; 9 mL PBS; 1 mL NiSO4 250 mM) development. The indirect ELISA procedure was similar to the screening with additional use of Listeria innocua DSM 20649 as negative control strain (preparation of living cells suspension is detailed in the next topic). This time, the scFv-Fc were diluted in 2% MPBS-T using √10-fold series and incubated for 1 h at RT, followed by incubation with the same secondary antibody from the immunoblot.
Indirect ELISA for Listeria spp. Detection
The bacteria included in this study were acquired from the Leibniz Institute DSMZ-German Collection of Microorganisms and Cell Cultures, which gives a catalog number (DSM) to every strain of its collection (Table 1). They were cultured in BHI, except for Lactobacillus paracasei, for which MRS medium was used, according to the recommendations of the strain manuals. The assay procedure, dilution of mAbs, and list of species were as described (23). Briefly, cells were cultured, washed with PBS, suspended in Carbonate-Bicarbonate 150 mM, pH 9.6, to OD600 = 1.0, and coated onto Costar ELISA plates (Corning). Antibodies were diluted in 2% MPBS-T in three concentrations: (1) the approximate EC50 (EC50); (2) a √10 dilution above the EC50 (EC); and (3) a √10 dilution below the EC50 (EC50-). Goat anti-mouse IgG Fc-specific HRP-conjugated (1:30,000; Jackson ImmunoResearch Laboratories) was used as the secondary antibody. Each antibody concentration was tested twice against each bacterial strain in individual plates. However, only one of these plates was used for the statistical analysis. A signal-to-noise ratio was calculated for each well by using the signal of secondary antibody alone against the bacteria as a reference for noise. The data were analyzed with GraphPad software (Prism, v 5.01) for the Receiver Operating Characteristic (ROC) determination. This way, the sensitivity, specificity, and confidence intervals (CI) were obtained.
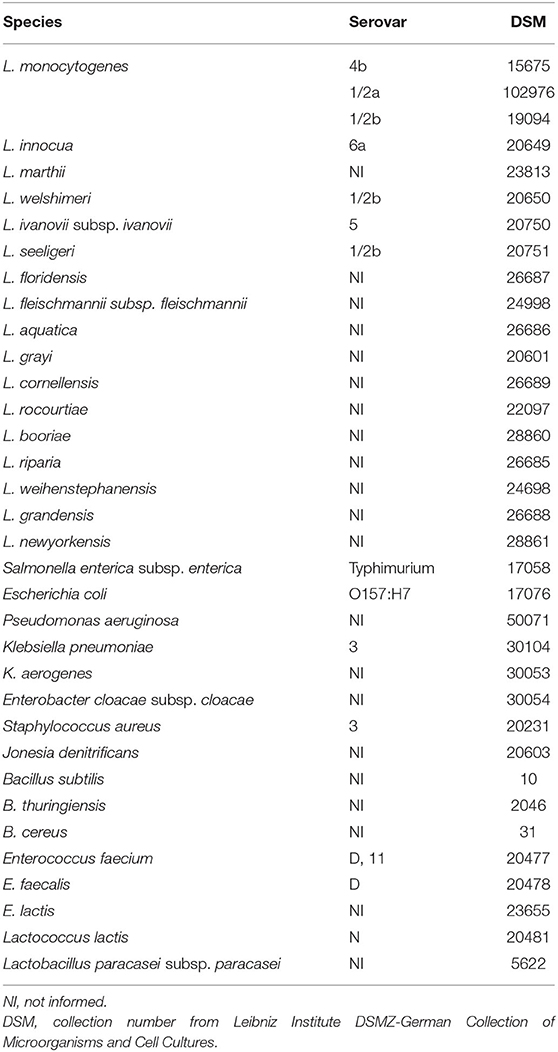
Table 1. List of the Listeria and non-Listeria species used in indirect ELISA for diagnostic performance assessment.
Results and Discussion
Panning With Human Naïve Antibody Library Over rInlA and rInlB Provides Binders Reactive in Immunoblot and ELISA
For antibody selection, the human naïve antibody gene libraries HAL9 and HAL10 were used. After the panning procedure, 52.2% (48/92) of clones randomly selected after three panning rounds on InlA were able to bind living L. monocytogenes in the screening ELISA using soluble scFv. From these, 30 clones were selected based on signal intensity and sequenced. On the other hand, against InlB, only one binder (1/92) was identified. Interestingly, three of the anti-InlA antibodies originated from the same germline sequences for the variable regions of the heavy and light chains (Table 2). While the only anti-InlB sequence had an Amber stop codon at the beginning of its sequence, which was later corrected for mammalian cell expression.
This low number of selected scFv against InlB may have some explanations. Since six of the 92 binders were able to bind the recombinant protein, but only one recognized the live cells, this may indicate significant differences in the structure of the antigen when coated (Supplementary Figure S1). Recent studies described considerable differences between panning on plates, used in this study, and in solution, indicating that some proteins might better keep their properties when not exposing their hydrophobic regions for coating (32). The same InlB used in this study showed to be functional in cellular assays from a previous work (25). Another possibility may be due to a low number of human antibody germline sequences against this antigen. Even though it is unlikely that phage display does not provide antibodies against bacterial antigens (33), previous works described regions of bacterial antigens, including Listeria spp., that provide few antibodies (23). This indicates that InlB may be substantially different from InlA (identity = 28%), which leads to low number of hits when performing panning. In addition, the fact that the only selected antibody against InlB in this work has a stop codon indicates that some VH germlines against this antigen might have been produced in low amounts or even excluded from the library.
The four InlA and one InlB binders with unique sequence were produced as scFv-Fc fusions and purified on Protein A-affinity chromatography. These antibodies were initially tested in Western blot against 1 μg of the respective recombinant antigens (Figure 1A). Despite the observation that one of the anti-InlA with different germline sequence showed slightly weaker staining, all antibodies detected bands of the expected molecular mass in immunoblot. This is an indication that the epitopes recognized by these antibodies are resistant to the harsh conditions applied during sample preparation, i.e., boiling and reducing environment. In addition, the scFv-Fc were titrated over Listeria living cells (Figures 1B–F), where only one of the four anti-InlA (GSM29-D3) and the anti-InlB (GSM30-D2) reached saturation against living cells. Interestingly, the three mAbs not showing saturation were from the same germline. Although no connection between germline and titration behavior had been established yet, the lack of saturation may help to explain the antibody behavior in different immunoassays. In any case, all five mAbs were specific to L. monocytogenes in this initial assay.
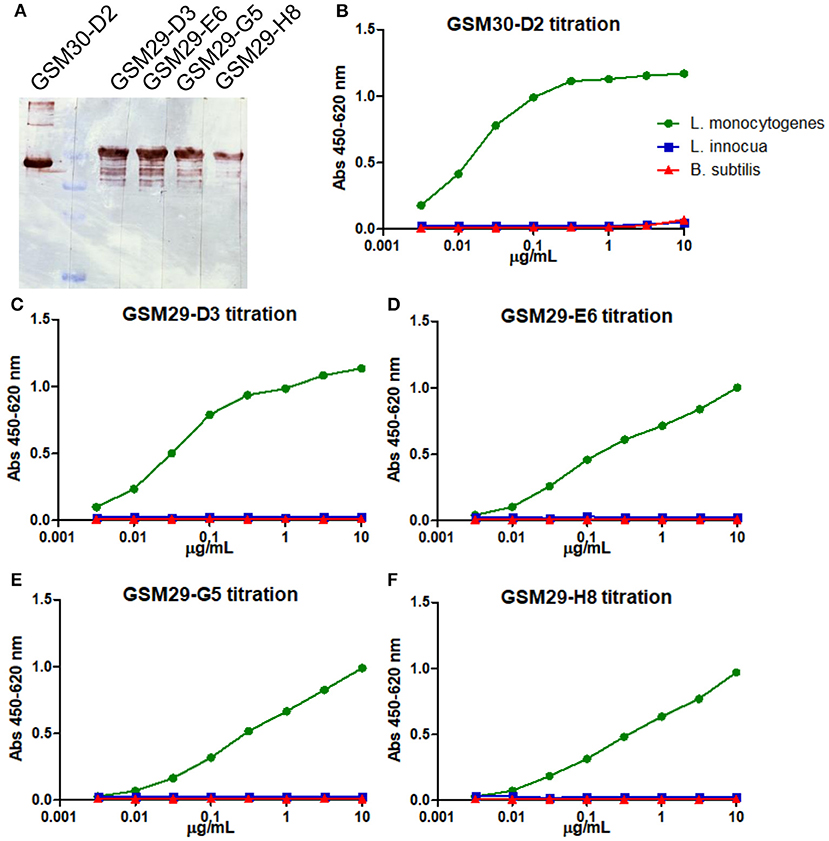
Figure 1. Immunoblot and indirect ELISA for titration and initial specificity screening of the scFv-Fc antibodies against recombinant InlA and InlB. The five mAbs were diluted to 1 μg/mL and tested against 1 μg of the recombinant protein via immunoblot (A). The anti-InlB scFv-Fc GSM30-D2 (B), and the anti-InlA GSM29-D3 (C), GSM29-E6 (D), GSM29-G5 (E), and GSM29-H8 (F) were tested against three strains coated alive onto ELISA plates: L. monocytogenes ATCC 7644 (green), L. innocua DSM 20649 (blue), and B. subtilis 168 NCIB 10106 (red).
Recombinant scFv-Fc mAbs Are Specific to L. monocytogens via Indirect ELISA
The five initially characterized antibodies (four against InlA, and one against InlB) were tested using indirect ELISA against a panel of Listeria spp., including the most virulent serovars 4b, 1/2a, and 1/2b. To access the detection performance, a ROC analysis was performed, showing that all five mAbs have 100% sensitivity and specificity (Table 2). When it comes to the reactivity of the antibodies, there is a clear distinction between the detection of InlA and InlB. The four antibodies against InlA showed a signal-to-noise ratio ranges between 11 to 20 for serotypes 4b and 1/2b, and 31 to 38 against 1/2a (Figure 2, Supplementary Table S1). The antibody against InlB had signal-to-noise ratios higher than 30 for serotypes 4b and 1/2a and slightly below 5 against 1/2b. All the non-target strains showed signal-to-noise ratios below 2.
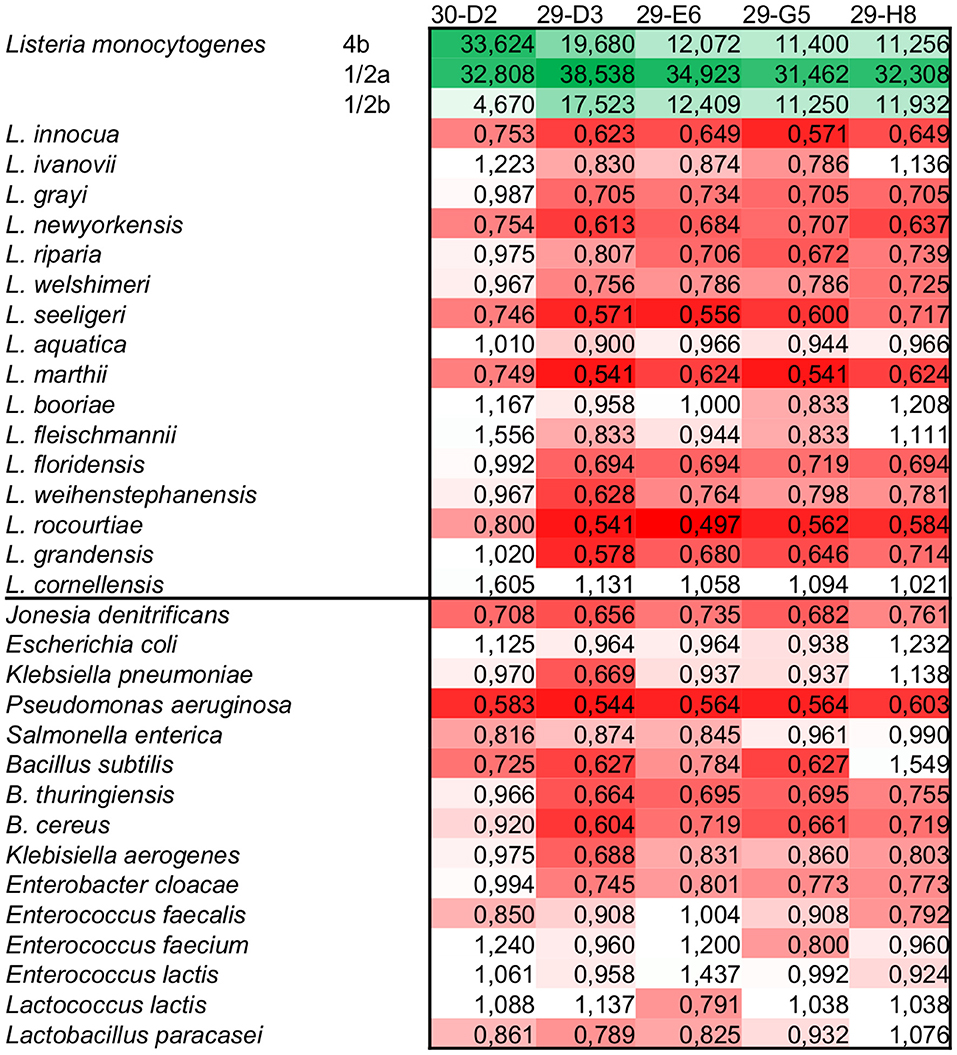
Figure 2. Signal-to-noise ratio of the scFv-Fc tested using the highest concentration (EC50+) in indirect ELISA. All five mAbs showed specific reaction against L. monocytogenes. Depending on the serotype and target used, the signals were from 4 up to more than 35 times higher than the negative reactions. The color scale goes from green (higher reaction) to red (lower reaction) going through white (signal-to-noise ratio = 1).
Although the present study could not involve a large panel of L. monocytogenes strains, some characteristics in the pattern of antibody reactivity are notable. Both 4b and 1/2b serotypes are part of the genetic lineage I of pathogenic Listeria (34), and the reactivity against InlA antibodies proves that both show similar levels of this target on the bacterial surface, while for InlB, serotype 4b shows a much superior amount of this target in comparison to 1/2b serotype. Considering that both serotypes are frequently isolated from human samples, this reactivity correlates to the fact that 4b serotype is highly infective (35, 36). On the other hand, serotype 1/2a is part of genetic lineage II, which is mainly isolated from food samples. However, the strain DSM 102976 strain used in the present study was isolated from an infected Guinea pig. This indicates that contrary to the fact that this serotype is usually less infective than 4b, the high levels of both InlA and InlB showed in the reactivity profile may have been a result of previous infective passages of this strain. Indeed, although these two internalins are the main factors known to promote intestinal cells invasion, other genes involved in the pathogenesis and survival of Listeria are important to determine virulence (37–39). Other environmental factors, such as temperature, may also influence the expression level of these targets, although the culture conditions used in the present study were the most appropriate for growth and both InlA and InlB in general (40).
Both InlA and InlB are the most studied proteins for L. monocytogenes detection. In other studies, mAbs against InlA were generated by hybridoma technology and were able to provide molecules with variable sensitivity. When using different highly sensitive biosensors, some works described the limit of detection (LOD) of 107 cells/mL (41), while others described 3 x 102 CFU/mL (24). An aptamer binder against InlA allowed detecting 103 CFU/mL when combined with other mAbs in SPR (42). Regarding InlB, a previous work described scFv binders that could be used for L. monocytogenes staining with quantum dots, as well as anti-InlA scFv (43). Phage display has also been previously used to obtain VHH antibodies from llamas immunized with InlB, although the applicability of this nanobody in immunodetection was not further investigated (44). Binders toward other targets were described, such as the hybridoma-derived mAbs against LapB, which were able to recognize more than 46 of the 53 L. monocytogenes strains included in the experiment (45). The use of a phage display human synthetic antibody library to generate antibodies against L. monocytogenes resulted in one scFv targeting ActA found to specifically recognize 6 out of 8 tested pathogenic strains (46). This same molecule was also tested in SPR showing LOD of 2 x 106 CFU/mL (47). Nonetheless, similarly to the VHH generated via phage display, this molecule was not further assessed for the development of a detection immunoassay. Considering that commercial lateral flow tests are already able to detect 104-106 CFU/mL, all the newly generated antibodies offer a chance for improving the minimal amount of Listeria cells detected.
In the present study, the generation of recombinant antibodies from a human naïve antibody library via phage display against InlA and InlB is described. Taking this into account, although the main goal was to generate a detection method for L. monocytogenes, the fully human origin of these antibodies would also facilitate the therapeutic application of these mAbs against Listeria infection in humans. This is especially important considering that, even though listeriosis has a low incidence overall, its mortality rate can be as high as 25%, with most outbreaks describing more than 10% mortality (48). Moreover, the increasing number of Listeria isolates that are resistant to antibiotics is already causing concern in the medical community (49). In this aspect, antibodies may offer an interesting alternative to antibiotics in the near future. Indeed, mAbs against Ferritin-like protein (Flp), ActA, or Listeriolysin O (LLO) have shown promising results in protecting mice against Listeria infection (50, 51). Thus, the recombinant human mAbs presented here could be an interesting alternative for passive immunization to reduce the number of deaths caused by listeriosis.
The panel of L. monocytogenes strains used here included the three most prevalent serovars in clinical cases (34). Besides that, no cross-reaction against any of the other 31 tested species was observed, indicating the high applicability of these antibodies for detection. As to the characteristics of L. monocytogenes, it is known that some isolates contain either truncated or mutated InlA or InlB (52, 53). Even though the number of isolates included in the study was low, no relevant strain containing both antigens altered has ever been described, indicating that the combination of mAbs targeting InlA and InlB still allow the detection of such strains. Therefore, the recognition profile of the generated mAbs described here indicates they have high differentiation capability, allowing the specific detection of L. monocytogenes in further studies with a detection procedure which is close to the final detection method (e.g., lateral flow). In this way, the current study indicates that the use of the anti-InlA e InlB mAbs in combination with those previously described against PDC-E2 could allow the proper detection of both pathogenic and non-pathogenic Listeria species.
Conclusion
Considering the growing importance of the genus Listeria in recent years, it is essential to increase studies aiming to develop tools to identify and control it. Since listeriosis is a foodborne disease that can be highly fatal, proper testing of food and food production facilities is the best way to prevent outbreaks. Hence, the present work shows the use of antibody phage display to generate suitable antibodies that can further improve the detection of L. monocytogenes. In future works, it might be possible to combine them with broadly-specific antibodies allowing the detection of the entire Listeria genus to create an immunoassay that differentiates between pathogenic and non-pathogenic Listeria.
Data Availability Statement
The datasets presented in this study can be found in online repositories. The names of the repository/repositories and accession number(s) can be found at: https://publikationsserver.tu-braunschweig.de/receive/dbbs_mods_00066984.
Author Contributions
GM, MM, ÂM, FC, and MH: conceptualization and funding acquisition. GM: investigation and writing—original draft. GM, SG, SD, MM, FC, and MH: methodology and writing—editing. SG, FC, ÂM, SD, and MH: resources. SD, MM, FC, and MH: supervision. All authors contributed to the article and approved the submitted version.
Conflict of Interest
SG was employed by German Collection of Microorganisms and Cell Cultures GmbH (DSMZ).
The remaining authors declare that the research was conducted in the absence of any commercial or financial relationships that could be construed as a potential conflict of interest.
Publisher's Note
All claims expressed in this article are solely those of the authors and do not necessarily represent those of their affiliated organizations, or those of the publisher, the editors and the reviewers. Any product that may be evaluated in this article, or claim that may be made by its manufacturer, is not guaranteed or endorsed by the publisher.
Acknowledgments
The authors would like to thank Prof. Dr. Arun K. Bhunia for kindly providing the plasmid pET28b/inlB constructed by Dr. Pascale Cossart (Institut Pasteur, France) and Conselho Nacional de Desenvolvimento Científico e Tecnológico (CNPq) from Brazil for funding the project and providing the scholarship of GMSGM (process 204693/2014-4).
Supplementary Material
The Supplementary Material for this article can be found online at: https://www.frontiersin.org/articles/10.3389/fpubh.2022.712657/full#supplementary-material
References
1. Leclercq A, Moura A, Vales G, Tessaud-Rita N, Aguilhon C, Lecuit M. Listeria thailandensis sp. nov. Int J Syst Evol Microbiol. (2019) 69:74–81. doi: 10.1099/ijsem.0.003097
2. Datta AR, Burall LS. Serotype to genotype: the changing landscape of listeriosis outbreak investigations. Food Microbiol. (2018) 75:18–27. doi: 10.1016/j.fm.2017.06.013
3. Yin Y, Yao H, Doijad S, Kong S, Shen Y, Cai X, et al. A hybrid sub-lineage of Listeria monocytogenes comprising hypervirulent isolates. Nat Commun. (2019) 10:4283. doi: 10.1038/s41467-019-12072-1
4. Zhang Q, Feng Y, Deng L, Feng F, Wang L, Zhou Q, et al. SigB plays a major role in Listeria monocytogenes tolerance to bile stress. Int J Food Microbiol. (2011) 145:238–43. doi: 10.1016/j.ijfoodmicro.2010.12.028
5. Lee BH, Cole S, Badel-Berchoux S, Guillier L, Felix B, Krezdorn N, et al. Biofilm formation of Listeria monocytogenes strains under food processing environments and pan-genome-wide association study. Front Microbiol. (2019) 10:2698. doi: 10.3389/fmicb.2019.02698
6. den Bakker HC, Warchocki S, Wright EM, Allred AF, Ahlstrom C, Manuel CS, et al. Listeria floridensis sp. nov, Listeria aquatica sp nov, Listeria cornellensis sp nov, Listeria riparia sp nov and Listeria grandensis sp nov, from agricultural and natural environments. Int J Syst Evol Microbiol. (2014) 64:1882–9. doi: 10.1099/ijs.0.052720-0
7. Doijad SP, Poharkar KV, Kale SB, Kerkar S, Kalorey DR, Kurkure NV, et al. Listeria goaensis sp. nov. Int J Syst Evol Microbiol. (2018) 68:3285–91. doi: 10.1099/ijsem.0.002980
8. Weller D, Andrus A, Wiedmann M, den Bakker HC. Listeria booriae sp. nov and Listeria newyorkensis sp nov, from food processing environments in the USA. Int J Syst Evol Microbiol. (2015) 65:286–92. doi: 10.1099/ijs.0.070839-0
9. Center for Disease ans Control (CDC). Listeria Outbreak Linked to Hispanic-Style Fresh Soft Cheeses. (2021). Available online at: https://www.cdc.gov/listeria/outbreaks/hispanic-soft-cheese-02-21/index.html [accessed May 14, 2021].
10. European Centre for Disease Prevention and Control. Listeriosis. in Annual epidemiological report for 2017, ed. ECDC (Stockholm: ECDC).
11. Pizarro-Cerdá J, Kühbacher A, Cossart P. Entry of Listeria monocytogenes in mammalian epithelial cells: an updated view. Cold Spring Harb Perspect Med. (2012) 2:a010009. doi: 10.1101/cshperspect.a010009
12. Guillet C, Join-Lambert O, Le Monnier A, Leclercq A, Mechaï F, Mamzer-Bruneel MF, et al. Human listeriosis caused by Listeria ivanovii. Emerg Infect Dis. (2010) 16:136–8. doi: 10.3201/eid1601.091155
13. Jadhav S, Bhave M, Palombo EA. Methods used for the detection and subtyping of Listeria monocytogenes. J Microbiol Methods. (2012) 88:327–41. doi: 10.1016/j.mimet.2012.01.002
14. Hahm B-K, Kim H, Singh AK, Bhunia AK. Pathogen enrichment device (PED) enables one-step growth, enrichment and separation of pathogen from food matrices for detection using bioanalytical platforms. J Microbiol Methods. (2015) 117:64–73. doi: 10.1016/j.mimet.2015.07.016
15. Janzten MM, Navas J, Corujo A, Moreno R, López V, Martínez-Suarez VJ. Specific detection of Listeria monocytogenes in foods using commercial methods: from chromogenic media to real-time PCR. Spanish J Agric Res. (2006) 4:235–47. doi: 10.5424/sjar/2006043-198
16. Bhunia AK, Ball PH, Fuad AT, Kurz BW, Emerson JW, Johnson MG. Development and characterization of a monoclonal antibody specific for Listeria monocytogenes and Listeria innocua. Infect Immun. (1991) 59:3176–84. doi: 10.1128/iai.59.9.3176-3184.1991
17. Yu K-Y, Noh Y, Chung M, Park H-J, Lee N, Youn M, et al. Use of monoclonal antibodies that recognize p60 for identification of Listeria monocytogenes. Clin Diagn Lab Immunol. (2004) 11:446–51. doi: 10.1128/CDLI.11.3.446-451.2004
18. Kim S-H, Park M-K, Kim J-Y, Chuong PD, Lee Y-S, Yoon B-S, et al. Development of a sandwich ELISA for the detection of Listeria spp. using specific flagella antibodies. J Vet Sci. (2005) 6:41–6. doi: 10.4142/jvs.2005.6.1.41
19. Kuhn P, Fühner V, Unkauf T, Moreira GMSG, Frenzel A, Miethe S, et al. Recombinant antibodies for diagnostics and therapy against pathogens and toxins generated by phage display. Proteomics Clin Appl. (2016) 10:922–48. doi: 10.1002/prca.201600002
20. Laustsen AH, Greiff V, Karatt-Vellatt A, Muyldermans S, Jenkins TP. Animal Immunization, in vitro display technologies, and machine learning for antibody discovery. Trends Biotechnol. (2021) 39:1263–73. doi: 10.1016/j.tibtech.2021.03.003
21. Oravcová K, Trncíková T, Kuchta T, Kaclíková E. Limitation in the detection of Listeria monocytogenes in food in the presence of competing Listeria innocua. J Appl Microbiol. (2008) 104:429–37. doi: 10.1111/j.1365-2672.2007.03554.x
22. Mendonça M, Moreira GMSG, Conceição FR, Hust M, Mendonça KS, Moreira ÂN, et al. Fructose 1,6-bisphosphate aldolase, a novel immunogenic surface protein on Listeria Species. PLoS ONE. (2016) 11:e0160544. doi: 10.1371/journal.pone.0160544
23. Moreira GMSG, Köllner SMS, Helmsing S, Jänsch L, Meier A, Gronow S, et al. Pyruvate dehydrogenase complex—enzyme 2, a new target for Listeria spp. detection identified using combined phage display technologies. Sci Rep. (2020) 10:15267. doi: 10.1038/s41598-020-72159-4
24. Mendonça M, Conrad NL, Conceição FR, Moreira AN, da Silva WP, Aleixo JA, et al. Highly specific fiber optic immunosensor coupled with immunomagnetic separation for detection of low levels of Listeria monocytogenes and L. Ivanovii. BMC Microbiol. (2012) 12:275. doi: 10.1186/1471-2180-12-275
25. Drolia R, Tenguria S, Durkes AC, Turner JR, Bhunia AK. Listeria Adhesion Protein Induces intestinal epithelial barrier dysfunction for bacterial translocation. Cell Host Microbe. (2018) 23:470–84.e7. doi: 10.1016/j.chom.2018.03.004
26. Moreira GMSG, Moreira C Jr, da Cunha CEP, Mendonça M, Conceição FR. Recombinant botulinum toxoids: a practical guide for production. Methods Mol Biol. (2016) 1404:621–32. doi: 10.1007/978-1-4939-3389-1_40
27. Russo G, Meier D, Helmsing S, Wenzel E, Oberle F, Frenzel A, et al. Parallelized antibody selection in microtiter plates. Methods Mol Biol. (2018) 1701:273–84. doi: 10.1007/978-1-4939-7447-4_14
28. Kügler J, Wilke S, Meier D, Tomszak F, Frenzel A, Schirrmann T, et al. Generation and analysis of the improved human HAL9/10 antibody phage display libraries. BMC Biotechnol. (2015) 15:1–15. doi: 10.1186/s12896-015-0125-0
29. Chiara M, Caruso M, Erchia AMD, Manzari C, Fraccalvieri R, Goffredo E, et al. Comparative genomics of listeria sensu lato : genus-wide through lateral gene transfer. Genome Biol Evol. (2015) 7:2154–72. doi: 10.1093/gbe/evv131
30. Miethe S, Rasetti-Escargueil C, Liu Y, Chahboun S, Pelat T, Avril A, et al. Development of neutralizing scFv-Fc against botulinum neurotoxin A light chain from a macaque immune library. MAbs. (2014) 6:446–59. doi: 10.4161/mabs.27773
31. Jäger V, Büssow K, Wagner A, Weber S, Hust M, Frenzel A, et al. High level transient production of recombinant antibodies and antibody fusion proteins in HEK293 cells. BMC Biotechnol. (2013) 13:52. doi: 10.1186/1472-6750-13-52
32. Wenzel EV, Bosnak M, Tierney R, Schubert M, Brown J, Dübel S, et al. Human antibodies neutralizing diphtheria toxin in vitro and in vivo. Sci Rep. (2020) 10:571. doi: 10.1038/s41598-019-57103-5
33. Roth KDR, Wenzel EV, Ruschig M, Steinke S, Langreder N, Heine PA, et al. Developing recombinant antibodies by phage display against infectious diseases and toxins for diagnostics and therapy. Front Cell Infect Microbiol. (2021) 11:697876. doi: 10.3389/fcimb.2021.697876
34. Orsi RH, den Bakker HC, Wiedmann M. Listeria monocytogenes lineages: Genomics, evolution, ecology, and phenotypic characteristics. Int J Med Microbiol. (2011) 301:79–96. doi: 10.1016/j.ijmm.2010.05.002
35. Ribeiro VB, Destro MT. Listeria monocytogenes Serotype 1/2b and 4b isolates from human clinical cases and foods show differences in tolerance to refrigeration and salt stress. J Food Prot. (2014) 77:1519–26. doi: 10.4315/0362-028X.JFP-13-548
36. Hasebe R, Nakao R, Ohnuma A, Yamasaki T, Sawa H, Takai S, et al. Listeria monocytogenes serotype 4b strains replicate in monocytes/macrophages more than the other serotypes. J Vet Med Sci. (2017) 79:962–9. doi: 10.1292/jvms.16-0575
37. Su X, Cao G, Zhang J, Pan H, Zhang D, Kuang D, et al. Characterization of internalin genes in Listeria monocytogenes from food and humans, and their association with the invasion of Caco-2 cells. Gut Patho. (2019) 11:30. doi: 10.1186/s13099-019-0307-8
38. Poimenidou S V, Dalmasso M, Papadimitriou K, Fox EM, Skandamis PN, Jordan K. Virulence gene sequencing highlights similarities and differences in sequences in Listeria monocytogenes serotype 1/2a and 4b strains of clinical and food origin from 3 different geographic locations. Front Microbiol. (2018) 9:1103. doi: 10.3389/fmicb.2018.01103
39. Drolia R, Bhunia AK. Crossing the intestinal barrier via Listeria adhesion protein and internalin A. Trends Microbiol. (2019) 27:408–25. doi: 10.1016/j.tim.2018.12.007
40. Mcgann P, Ivanek R, Wiedmann M, Boor KJ. Temperature-dependent expression of Listeria monocytogenes internalin and internalin-like genes suggests functional diversity of these proteins among the Listeriae † downloaded from. Appl Environ Microbiol. (2007) 73:2806–14. doi: 10.1128/AEM.02923-06
41. Hearty S, Leonard P, Quinn J, O'Kennedy R. Production, characterisation and potential application of a novel monoclonal antibody for rapid identification of virulent Listeria monocytogenes. J Microbiol Methods. (2006) 66:294–312. doi: 10.1016/j.mimet.2005.12.009
42. Ohk SH, Koo OK, Sen T, Yamamoto CM, Bhunia AK. Antibody-aptamer functionalized fibre-optic biosensor for specific detection of Listeria monocytogenes from food. J Appl Microbiol. (2010) 109:808–17. doi: 10.1111/j.1365-2672.2010.04709.x
43. Tully E, Hearty S, Leonard P, O'Kennedy R. The development of rapid fluorescence-based immunoassays, using quantum dot-labelled antibodies for the detection of Listeria monocytogenes cell surface proteins. Int J Biol Macromol. (2006) 39:127–34. doi: 10.1016/j.ijbiomac.2006.02.023
44. MacKenzie CR, Arbabi-Ghahroudi M, Gene RW, van Faassen H, Aroche C, Hall JC, et al. High affinity anti-Internalin B VHH antibody fragments isolated from naturally and artificially immunized repertoires. J Immunol Methods. (2014) 416:29–39. doi: 10.1016/j.jim.2014.10.009
45. Boivin T, Elmgren C, Brooks BW, Huang H, Pagotto F, Lin M. Expression of surface protein LapB by a wide spectrum of Listeria monocytogenes serotypes as demonstrated with anti-LapB monoclonal antibodies. Appl Environ Microbiol. (2016) 82:6768–78. doi: 10.1128/AEM.01908-16
46. Paoli GC, Chen CY, Brewster JD. Single-chain Fv antibody with specificity for Listeria monocytogenes. J Immunol Methods. (2004) 289:147–55. doi: 10.1016/j.jim.2004.04.001
47. Nanduri V, Bhunia AK, Tu SI, Paoli GC, Brewster JD. SPR biosensor for the detection of L. monocytogenes using phage-displayed antibody. Biosens Bioelectron. (2007) 23:248–52. doi: 10.1016/j.bios.2007.04.007
48. Noordhout CM De, Devleesschauwer B, Angulo FJ, Haagsma J, Kirk M, Havelaar A, et al. The global burden of listeriosis: a systematic review and meta- analysis. Lancet Infect Dis. (2015) 14:1073–82. doi: 10.1016/S1473-3099(14)70870-9
49. Olaimat AN, Al-Holy MA, Shahbaz HM, Al-Nabulsi AA, Abu Ghoush MH, Osaili TM, et al. Emergence of antibiotic resistance in Listeria monocytogenes isolated from food products: a comprehensive review. Compr Rev Food Sci Food Saf. (2018) 17:1277–92. doi: 10.1111/1541-4337.12387
50. Mohamed W, Sethi S, Darji A, Mraheil MA, Hain T, Chakraborty T. Antibody targeting the ferritin-like protein controls Listeria infection. Infect Immun. (2010) 78:3306–14. doi: 10.1128/IAI.00210-10
51. Asano K, Sashinami H, Osanai A, Hirose S, Ono HK, Narita K, et al. Passive immunization with anti-ActA and anti-listeriolysin O antibodies protects against Listeria monocytogenes infection in mice. Sci Rep. (2016) 6:1–10. doi: 10.1038/srep39628
52. Cruz CD, Pitman AR, Harrow SA, Fletcher GC. Listeria monocytogenes associated with New Zealand seafood production and clinical cases: unique sequence types, truncated InlA, and attenuated invasiveness. Appl Environ Microbiol. (2014) 80:1489. doi: 10.1128/AEM.03305-13
Keywords: Listeria monocytogenes, monoclonal antibody, internalin A, internalin B, phage display, detection, food safety
Citation: Moreira GMSG, Gronow S, Dübel S, Mendonça M, Moreira ÂN, Conceição FR and Hust M (2022) Phage Display-Derived Monoclonal Antibodies Against Internalins A and B Allow Specific Detection of Listeria monocytogenes. Front. Public Health 10:712657. doi: 10.3389/fpubh.2022.712657
Received: 20 May 2021; Accepted: 14 February 2022;
Published: 15 March 2022.
Edited by:
Sergio Oscar Angel, CONICET Instituto Tecnológico de Chascomús (INTECH), ArgentinaReviewed by:
Andrea Queiroz Maranhão, University of Brasilia, BrazilIrene R. Grant, Queen's University Belfast, United Kingdom
Copyright © 2022 Moreira, Gronow, Dübel, Mendonça, Moreira, Conceição and Hust. This is an open-access article distributed under the terms of the Creative Commons Attribution License (CC BY). The use, distribution or reproduction in other forums is permitted, provided the original author(s) and the copyright owner(s) are credited and that the original publication in this journal is cited, in accordance with accepted academic practice. No use, distribution or reproduction is permitted which does not comply with these terms.
*Correspondence: Michael Hust, bS5odXN0JiN4MDAwNDA7dHUtYnMuZGU=