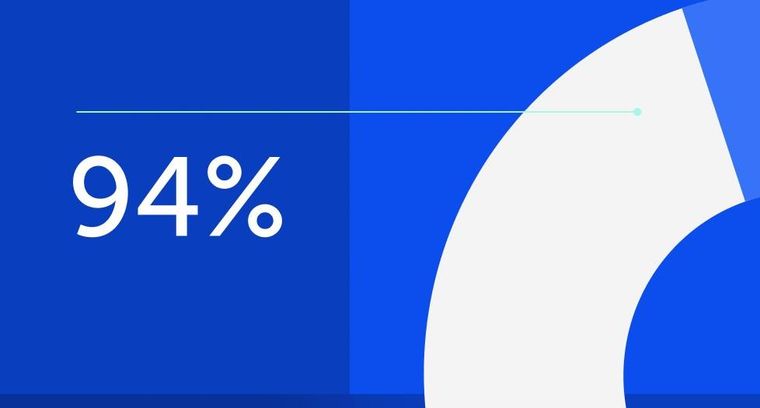
94% of researchers rate our articles as excellent or good
Learn more about the work of our research integrity team to safeguard the quality of each article we publish.
Find out more
METHODS article
Front. Public Health, 22 November 2022
Sec. Occupational Health and Safety
Volume 10 - 2022 | https://doi.org/10.3389/fpubh.2022.1076461
Prefabricated construction is one of the solutions to the problem of balancing environmental improvements with the new buildings in the construction industry. Some work originally done on site is transferred to the front end, and the occupational health risks to industrial workers during the production of prefabricate concrete components are thus aggravated. This study aims to propose a framework to simulate the occupational health risks of workers in prefabricate concrete component plants from the perspective of risk identification, risk assessment, and risk control. Through the following 4 steps, including environmental release monitoring, diffusion and human inhalation mechanism analysis, occupational health risk evaluation, and full-path health risk simulation, this study maps physical entities to virtual reality. The proposed method tends to address the root causes behind occupational health risks, such as the lack of measurement, assessment and prevention criteria, and providing new ideas for theoretical research and innovative practice of HSE management and risk management in the construction industry.
The construction industry faces the problem of balancing economic growth and environmental impact. The concept of prefabricated building is an innovative solution to this problem. Compared to traditional forms of construction, prefabricated building has the advantages of increasing efficiency, shortening periods, lowering costs, saving energy, reducing consumption, reducing environmental pollution and sustainability in the production, construction and use processes (1, 2). Therefore, the prefabricated buildings are being promoted and used worldwide, which contribute to the construction industry's vision of a “carbon-free future.” Taking China as an example, the development of prefabricated buildings has been adopted as an important national strategy. The Chinese government began to focus on the promotion of prefabricated buildings with the Green Building Action Plan released in 2013. In 2018, the Chinese government further made the vigorous development of prefabricated buildings a key task and overall requirement for the future. In 2021, the Chinese government released the Action Plan for Carbon Peaking by 2030, which aims to accelerate the application of prefabricated buildings and the industrialization of construction. Even under the influence of the epidemic, the total building area of new prefabricated building in China reached 740 million square meters in 2021, accounting for about 25% of total area of new construction with more than 500,000 workers employed.
Prefabricated buildings can be divided into concrete structures, steel structures, bamboo and timber structures according to the type of structure, among which prefabricated internal/external wall panels, prefabricated floor slabs, prefabricated staircases and prefabricated balconies as the main components of prefabricated concrete structures are the most common, accounting for over 90% of the market share (3). Unlike traditional on-site construction methods, the production of prefabricate concrete components is mainly carried out in fully/semi-enclosed industrial plants. Industrial workers are required to put in intense physical work and are exposed to specific environments for long periods of time (4). During the production of prefabricate concrete components, the use of materials, machinery and energy leads to the generation of many types of harmful environmental release such as dust, noise, volatile organic compounds (VOCs) and radioactive elements (5).
The environmental protection authorities in most country will review the environmental assessment before the project goes into operation. However, the focus is on the impact of the plant's emissions on the external environment, while neglecting the occupational health of workers. For example, although a certain prefabricated concrete (PC) component manufacturer acquired ISO 9001 and ISO 14001 certification and meet the 17 elements of environmental management, there are only a few words of discussion concerning the occupational health of workers. In addition, through the preliminary site survey and visits, some PC component production enterprises, especially the small plants, have problems such as dust, noise and odor, and workers lack the necessary protective measures and awareness of health protection, which exposed them to serious occupational health risks. A series of occupational health-related laws and regulations are enacted worldwide, including the Occupational Health and Safety Management System Guide in the UK, the Occupational Health and Safety Management System in the US, the Occupational Health Inspection Management Regulations in China, the Occupational Health and Safety Management System General Guide in Australia, the Occupational Health and Safety Management System Guidelines in Japan and the international standard Occupational Health and Safety Management System Requirements and Guidelines for Use. However, the risk regulation system and standards for the assembly construction industry, which lies between manufacturing and construction industry, are still immature and require systematic theoretical research results to support the development of risk evaluation criteria and the control of the overall process.
Thus, this article is grounded in the intersection of engineering, environmental, and health management. A logical framework of occupational health risks was formed using risk management concept to identify the full pathway process of environmental release, combined with a personal exposure assessment method to form a simulation model of occupational health risk assessment for the whole process workers. It is also applied to real-life cases to form a complete set of long-term management models of occupational health risks caused by environmental release, providing a new paradigm for research in the field of occupational health risk management.
The environmental release in this study refers to the hazardous substances or energy spilling, leaking, emitting or escaping into the external environment in physical or chemical form. The construction phase is long with many upstream and downstream industries, complex construction elements, and huge amounts of construction materials, machinery and energy, thus generating various types of environmental release at all stages of building production, transportation, construction, decoration and renovation (6). The main environmental release listed in order from the construction industry are: carbon dioxide (and other greenhouse gases), inorganic dust (minerals, metals, artificial dust), formaldehyde, noise, VOCs, high temperature and high humidity, organic dust, ammonia, radon, etc. A summary of the above environmental release sources and health impacts is shown in Table 1. According to the EU statistical report, more than 15% of construction workers deal with or contact dangerous substances for a long time, which induces various skin diseases; 32% of construction workers are exposed to fumes and vapors more than half of their working hours, causing respiratory diseases, silicosis, and even cancer (25). In 2019, 79,000 people in the UK construction sector suffered from occupational diseases. About 3,500 people died of cancer each year, and 5,900 people suffered from cancer (26). Based on the above facts, the incidence of occupational diseases among construction workers is 2–6 times higher than the average level of the whole industry, among which the health damage of workers caused by environmental release is particularly serious.
Traditional building products, especially concrete components, are mostly cast on site in an outdoor open environment, and the resulting harmful environmental release are easily dissipated into the atmosphere, so the exposure concentration/intensity of industrial workers is relatively low. The production of PC components is conducted in industrial plants, and the production process produces various harmful environmental release, such as dust, noise and VOCs. Industrial workers have long been in this semi-enclosed space with high environmental release and high intensity, suffering serious potential threat to the health in the short or long term.
“Occupational health risk” refers to the possibility of work-related diseases or occupational diseases caused by workers' exposure to occupational hazard factors in the process of occupational activities, and its health damage consequences have specific probability and severity (27). Occupational health risks mainly come from the following three aspects: occupational diseases, safety accidents and mental health (28). Occupational health risk assessment is a comprehensive and system through the workplace occupational hazards identification and analysis of the specific application of risk assessment methods, assessment of laborers in the professional activities caused by exposure to occupational hazard factors in the process of the possibility of work related diseases or occupational disease, to predict its occupational health risk level, provide the basis for the corresponding risk control measures (29).
Occupational health risk assessment can be divided into qualitative, semi-quantitative and quantitative risk assessment methods. In the 1990's, European and American countries and international organizations successively issued occupational health risk assessment guidelines or norms to assess and manage the risk of hazardous substances in the workplace, For example: ICMM Operational Guidelines for Occupational Health Risk Assessment, Occupational Exposure Limits Evaluation Methodology, New Guidance on Inhalation Risk Assessment, Simple Elements of Chemical Occupational Hazard Classification and Control Techniques, Romanian Risk Assessment Methodology for Occupational Accidents and Occupational Diseases, Australian Guidance on Occupational Health and Safety Risk Assessment Management, International Council on Mining and Metals Occupational Health Risk Assessment Guidance, Risk Assessment Methodology for Occupational Exposure to Toxic Chemicals, ICI Mond Toxicity Index Evaluation Method and so on (30–33). In addition to these classical models, Monte Carlo simulation, exposure proportional assessment method, integrated index assessment method, Physiologically-based pharmacokinetic (PBPK) model, fuzzy Bayesian network, and risk assessment method under operational conditions are also widely used (34–40).
The United States National Institute for Occupational Safety and Health (NOSH) research report indicated that there are as many as 39 categories related to occupational health and safety in the construction industry, such as: sandblasting, blood lead, asbestos, asphalt, carbon monoxide, eye diseases, high temperature and pressure, quartz, skin exposure, noise and so on. At present, in addition to Australia, Sweden, Germany and other countries with good occupational health management, there are still some countries in the world with relatively weak research on occupational health management in the construction industry, and there is still a great room for improvement in occupational health management in the construction industry.
Previous research OHSAS and HSE management system as the core, aiming at comprehensive evaluation of the construction industry practitioners of occupational safety and health risks. In fact, multi-source harmful environmental release is one of the causes of occupational health risks. From the perspective of building types, studies mainly focus on traditional building forms and their construction processes, while studies on prefabricated buildings, especially the PC component production are relatively few. In terms of the type of emissions, the vast majority of studies focus on emissions of carbon dioxide and other greenhouse gases that have profound effects on human health and the environment. However, there are few studies on environmental release such as dust, noise and VOCs that pose acute/chronic threats to the health of construction workers. In addition, previous studies lack real-time and long-term monitoring and dynamic optimization of emission sources and their surrounding environment, resulting in the lack of universality of static data obtained from a single measure, and the lack of referential results and conclusions.
The Life Cycle Assessment (LCA) can be used to assess the impact on human health of various pollutants emitted from the entire process of product production, with emphasis on a long term and cyclical evaluation concept (41). Risk Management Theory is to maintain a certain state of affairs when risk is deemed acceptable and to ensure maximum return. And when the risk is determined to be unacceptable, corresponding measures are taken to reduce the risk. Based on the above two theories, a life cycle risk management evaluation model is proposed in this paper to establish a risk management evaluation system as a long-term effect. The conceptual process is shown in Figure 1.
The risk identification is divided into three parts through the investigation and literature review to understand the mechanism of environmental release from the perspective of LCA. In the Risk Assessment stage, the occupational health risk assessment method and a simulation model adapted to the PC component production phase are built, so that the risks can be can be quantified and optimized. Finally, the modeled risk assessment system is put into empirical analysis. We will control the risk status of all aspects accurately and put forward policy advice. With the records of the database, the effect of measures on risk reduction are tracked and monitored, and the information is feedbacked to the risk evaluation and risk management system to achieve dynamic risk control (42).
Given that the uncertain factors occur as probabilistic phenomenon, the individual exposure assessment method is used in this study. The individual exposure assessment method is used to simulate the probability of inhalation, exposure and exposure to hazardous environmental release during a worker's long-term work, and thus to assess occupational health risks (43). It can be calculated after a large number of iterations to make the results true and reliable within a certain confidence interval. According to the US EPA risk assessment model, the relationship between pollutant concentrations emitted by production and the intake dose of workers is calculated as Equation (1) (44).
Where CDIi denotes the chronic daily inhalation/intake of emission i (mg/kg/d), Ci is the concentration of emission i (mg/m3), IR is inhalation rate (m3/h), ED represents exposure duration (h/d), EF is exposure frequency (d/a), EL is years of exposure, BW is body weight (kg), and AL is average life cycle (years).
In the available health risk assessment literature, hazardous compounds are usually classified as non-carcinogens or carcinogens (45). For carcinogenic substances, the carcinogenic damage quantification method is established the carcinogenic risk model based on the given carcinogenic slope factor and reference concentration data (46); for non-carcinogenic substances, a chronic disease damage quantification method is established for workers' occupational health risks by calculating chronic hazard factors based on reference concentration data (47). The carcinogenic risk model and the chronicity risk model of the occupational health risk model were quantified using Equations (2) and (3), respectively (48).
Where CRi denotes carcinogenic risk of emission i, ISFi is inhalation slope factor for emission i (kg·d /mg), HQi means Hazard Quotient, namely the ratio of the concentration of emission i to its reference concentration RfCi in a given time period, with HQi ≥1 indicates that the emission concentration is high enough to cause chronic non-carcinogenic effects. RfCi symbolizes the reference concentration factor of emission i (mg/m3). ISFi and RfCi are captured in the US Integrated Risk Information System (IRIS).
The disability-adjusted life year (DALY) is used to quantify the disease burden and injuries in human populations in the Global Burden of Disease Study and perform a quantitative assessment of health damage (49). Health risks are proportionally distributed with the diseases suffered through impact and damage analysis and unified into the DALY as shown in Equation (4) (50).
Where YLL denotes the year of life lost. YLD is the year of life lived with a disability. It is a time-based measure that combines the time lost due to premature mortality and the duration of disability caused by illness in survivors.
According to the three stages of risk management theory, this study builds an occupational health risk framework, starting from the three stages of risk identification, risk assessment and risk control, including research content, technical roadmap and methods, as shown in Figure 2.
Identifying the source of environmental release and confirming the emission characteristics. Specifically, it includes the following two contents: (1) Sorting out the current production process and process characteristics of typical prefabricate concrete component plants assembly lines. (2) Determining the type, location and extent of environmental release resulting from operations such as material fugitive, energy consumption and mechanical equipment operation during the production of prefabricate concrete components. Taking PC exterior wall panels as an example, their production contains more than 30 processes such as cleaning the mold, installing reinforcement cages, pouring and vibrating, and spray release agent, etc. The potential harmful environmental release during the process mainly contains dust, noise, and VOCs, as shown in Figure 3. For other types of PC components, such as PC floor slabs, PC staircases, PC balcony slabs required for housing construction, and PC shield pieces required for underground projects, are slightly different in process, but the main types of environmental release are the same.
Figure 3. Types of potentially harmful environmental release from the production of precast concrete facade panels.
Detection and analysis of environmental release properties in precast concrete components plants includes: (1) Field sampling of dust, VOCs gas and other aerosol substances using cyclone particulate collectors, vacuum sampling pumps, Teflon filter membranes, etc. Samples collected in the field are sent to the laboratory under light-free, low-temperature and dry conditions. (2) The material class and particle size interval of the solids in the filter membrane, and the composition and proportion of the gas in the sampler, respectively, with the aid of gas chromatography/mass spectrometry (GC/MS), according to the standard test method provided by the National Institute for Occupational Safety and Health Research (NIOSH). (3) Toxicological and human potential damage analysis was conducted for all detected substances, and the substances with a large proportion and heavy hazard were finally selected as the final environmental release types (Note: Noise is analyzed separately for its impact on human health and is included as one of the environmental release types).
Based on the source emission characteristics, the diffusion and dispersion mechanisms of environmental release are analyzed using spread and dispersion laws as follows: (1) Using monitoring equipment such as laser dust meter, acoustic meter and photo-ionization detector to monitor the emission sources and the surrounding environment at fixed points to obtain real-time data on the change in concentration of dust or gas emissions during the production of prefabricate concrete components and the change in intensity of noise, respectively. (2) Collecting and measuring data on potential influencing factors such as spatial boundaries, wind speed, wind direction, temperature and humidity within the plant during the same production time period using temperature/humidity meters, anemometers and so on. (3) Establish an environmental release propagation/diffusion model, and simulate the transport trajectory, diffusion path and concentration distribution of dust and gas, as well as the propagation distance and decreasing degree of noise, respectively, with Fluent software. (4) Determine the propagation or dispersion pattern of various types of environmental release during the production of prefabricate concrete components.
The human intake mechanism of environmental release includes the following four steps: (1) Real-time monitoring of noise intensity and emission concentration data at the ear, mouth and nose of workers of different job types during production operations by requiring workers to wear portable devices. (2) Deploy RFID sensor devices at designated locations to collect and record data on workers' job characteristics, behavioral habits, movement range and other potential influencing factors. (3) Modeling the inhalation/exposure dose of workers to simulate their inhalation, exposure dose, or exposure level to short-lived environmental release under specific constraints. (4) Determine the way of environmental release from human intake during the production of prefabricate concrete components. A number of instruments and equipment are used in these processes, which are highly specialized and sophisticated. The main instruments and equipment mentioned above are shown in Figure 4.
On the basis of the risk identification and assessment methods, the simulation model was established to establish the “Emission-Transmission-Inhalation” whole process of worker occupational health risk simulation and evaluation model. The simulation module of each part is established, specifically including: (1) Establish a basic simulation module of prefabricate concrete component production process and emission source location based on building information model. (2) Establish a numerical simulation module based on computational fluid dynamics to simulate the propagation and dispersion of environmental release in component plants. (3) Establish a multi-intelligence-based worker production behavior and health injury simulation module. (4) Create a multiple sources database module containing information on environmental release monitoring, worker health data, emission factors and so on. (5) The application development platform is selected to integrate the above simulation modules and database to build a quarriable, editable and calculable occupational health risk simulation and evaluation model, as shown in Figure 5.
Based on the life cycle risk management evaluation method, combined with the above simulation model, the long-term control of occupational health risks caused by environmental release is formed. For the identification of risk control strategies, the target case analysis takes into account the results of risk evaluation and identifies control strategies based on the classification of carcinogenic risk and chronic disease risk respectively. Then, based on the Critical success factors (CSF) analysis method, the key success variables of environmental release risk control are searched from three aspects: emission sources, transmission paths and recipient individuals.
Drawing a fishbone diagram to identify, define and develop specific measures included in the above three CSFs, such as reducing dust/gas release due to handling, disturbance or vibration of parts, installing sound insulation and noise reduction equipment, installing sprinkler systems, optimizing ventilation systems, temperature and humidity regulation systems, regular cleaning and decontamination, requiring workers of specific jobs to wear protective equipment, strictly setting daily/yearly working hours for workers of certain special jobs, adopting a job rotation system on a regular basis, etc. Fishbone diagram through critical success factor analysis, the gray correlation algorithm is used to determine the gray correlation between the above measures and to develop the optimal combination strategy. The process is shown in Figure 6.
The simulation model contains five key elements as shown in Equation (5), where: SM is the simulation model, PE is physical entity, VE is virtual entity, SS is service, DB is data, and CN is the connection between the parts.
Physical layer element identification and data acquisition. The accurate analysis of PE is the basis for building the simulation model. In the project, a single PC component production machinery and equipment can be regarded as a unit-level PE, which is the smallest unit for function realization; a full set of PC component production line can be regarded as a system-level PE, which can complete the component production task; the whole plant composed of production line, environmental Emission and workers can be regarded as a system-level PE, which is a comprehensive system including material flow, emission flow and information flow, including four parts of human-machine-material-environment, as shown in Equation (6).
Hp describes the scope of action and behavior of industrial workers in the production process of components, and the data are obtained through RFID and image recognition technologies; Mp describes the operation of production machinery and production lines, and is portrayed and simulated through preliminary research and continuous probability events; Pp describes the production process of PC components, and is built through the material flow model; Ep describes the intensity and propagation law of environmental release, and is measured through sensors and portable devices.
Model layer model building and rules making. The establishment of the model layer is the core of the simulation technology. The digital model of this project includes geometric model, behavioral model and rule model, which describes and portrays each physical entity in the production process of PC components from multiple time scales and multiple space scales, as shown in Equation (7).
Gv is a 3D model describing the geometric parameters and relationships of PE, with good spatial and temporal consistency with PE, which can be realized by Fluent 3D modeling software with Revit and Auto CAD; Bv describes the real-time response and behavior of PE under the joint action of external environmental impact and internal operation mechanism in different time scales, using a multi-intelligence-based simulation model. Rv describes how physical entities operate, including rules based on historical data, experience based on tacit knowledge summaries, and process flow standards, enabling VE to map the PC component production process and environmental release in real time.
Functional and business module design of the service layer. The service layer is designed to encapsulate various types of data, models, algorithms, and results in the simulation model in a service-oriented manner, to support the operation of the internal functions of the model and to provide functional services (Bs). At the same time, the service (Fs) needs of PC component manufacturing enterprise managers for environmental release and worker health risk information acquisition are met through application software and clients, as shown in equation (8).
Fs includes: model management services provided for VE such as modeling simulation, model assembly and fusion; data management and processing services provided for DB such as data storage, encapsulation, cleaning, correlation and fusion; comprehensive connection services provided for CN such as data collection, sense access, data transmission, protocols and interfaces, etc. Bs mainly include: environmental release monitoring and occupational health risk assessment services for management personnel and other services; abnormal concentration monitoring and alarm services for industrial workers; data statistics and industry standard cap monitoring for government regulatory departments.
Database establishment and data are interconnected in the data layer. The establishment of the database in the data layer is the key to realize the information linkage and transmission among other layers, mainly including PE data, VE data and SS data, as shown in Equation (9).
Dp mainly includes physical element attribute data reflecting PE specifications, functions, performance, relationships, etc., as well as dynamic process data reflecting PE operating conditions, real-time performance, environmental parameters, sudden disturbances, etc., which can be collected through testing equipment, sensors and other equipment; Dv mainly includes data related to geometric models, physical models, behavioral models and rule models in VE, as well as simulation data based on the above models Ds mainly includes data related to Fs (such as algorithms, models, data processing methods, etc.) and Bs (query, analysis and management related data).
Inter-layer connection method design. After the above four layers are established, how to connect the layers together is another key technique to realize the proper operation of the simulation model. The connection (CN) between the above four layers is shown in Equation (10).
PD realizes the interaction between PE and DB, using emission monitoring instruments, FRID, image recognition, etc. to collect PE data in real time and transmit them to DB through OPC-UA protocol specification; PV realizes the interaction between PE and VE, transmitting the collected PE real time data to VE for updating and correcting the model; PS realizes the interaction between PE and SS, transmitting the collected PE real time data to SS for updating and correcting the model. Realize the update and optimization of SS. At the same time, the operation guidance, analysis and decision optimization results generated by SS are provided to the manager in the form of client, and the optimization of PE is realized through manual operation; VD realizes the interaction between VE and DB, and stores the simulation and related data generated by VE into DB in real time through the database interface, and reads the associated data from DB in real time; VS realizes the interaction between VE and SS, and completes the instruction VS implements the interaction between VE and SS, completing commands such as instruction transmission, data sending and receiving, message synchronization, etc.; SD implements the interaction between SS and DB, storing SS data to DB in real time, and reading historical data and rule data in DB in real time. The three processes, PV in implementing the interaction between PE and VE, PS in implementing the interaction between PE and SS, and VS in implementing the interaction between VE and SS, are repeated over and over again, both iteratively, and the result of each iteration is used as the initial value for the next iteration. The model driven architecture of occupational health risk assessment is shown in Figure 7.
On the one hand, finding the balance of economic benefits, environmental release and health risks based on the empirical research. Firstly, adding external constraints such as capital cost, time cost, and human cost that enterprises need to invest for environmental release control and risk prevention, corresponding to each measure in the target case study. Secondly, constructing a multi-objective decision model based on greedy algorithm to find the local optimal solution under the above constraints of different cost inputs and risk levels, and establish a set of risk control and input strategies. For example, if an enterprise wants to reduce the probability of workers' cancer to < 3 per 100,000, the total amount of risk prevention funds to be invested and the investment strategy can be obtained by the decision model under the condition that the number of workers and the duration of work are determined.
On the other hand, the study helps to facilitate the establishment of a long-term occupational health risk management system and the introduction of more accurate environmental release and worker occupational health risk assessment criteria for the production of PC components, as well as more accurate occupational health risk monitoring and early warning by the government. Occupational health risk long-term management system construction, risk monitoring and early warning aims to establish a set of long-term monitoring and early warning management system based on the above research results, specifically including: (1) Integrate the multi-objective model with the simulation model, combine simulation experiments with parallel experiments, and establish environmental release and worker occupational health risk evaluation criteria for PC component production for different products (wall/floor slabs, stairs, shield pieces, etc.) and different types of work (reinforcement tying, cement pouring, component maintenance workers, etc.), respectively. (2) Determine the number, location and scope of environmental release monitoring points according to the standards, set the concentration/intensity monitoring thresholds for different categories of emissions, and establish a multi-level risk warning system for workers' carcinogenesis and chronic diseases. (3) Compare the monitoring data of several PC component plants, summarize the characteristics of environmental release and workers' health risks, further optimize the monitoring program of monitoring points, thresholds and types of work, and form evaluation standards with high timeliness and universality, and promote and apply them in the whole industry.
The occupational health risks of construction industry workers have been much higher than the social average. With the growing scale of prefabricated buildings, the work originally required to be done on site is shifting to the front end, and the health risks of on-site construction workers are shifting to industrial workers in prefabricate concrete component plants.
Based on the intersection of engineering, environmental, and health management, this study tries to solve the root problems behind occupational health risks from risk identification, risk assessment, and risk control, the three processes such as the lack of measurement, assessment, and prevention standards. Through mapping physical entities to virtual simulation, feeding back the quantification and optimization results to practical applications, and then expanding individual projects to the whole industry, this study provides new ideas for theoretical research and innovative practice of HSE management and risk management in the construction industry. Firstly, the logical framework of occupational health risk is formed using the concept of life cycle risk management, which provides a new paradigm for the research in the field of occupational health risk management. Secondly, identifying the characteristics of the source of emissions and analyzing the diffusion propagation law and diffusion mechanism to understand the whole path process of environmental release. Afterwards, clarifying the human intake mechanism combined with the personal exposure assessment method that considers uncertainty factors. Finally, the simulation model of occupational health risk assessment for the whole process workers is formed, and finally it is applied to real cases to form a complete set of long-term management model of occupational health risks caused by environmental release.
A reasonable risk assessment simulation model and a long-term management model are proposed in this study. In the future, more empirical researches need to be verified in the model to improve the measurement accuracy, refine the human exposure assessment method, and adjust the simulation parameters, and so as to help decision makers to formulate occupational health risk policy. Furthermore, the production of PC components is only one phase of the assembled building industry. Transport, construction and other phases also include a large number of workers whose health risks need to be considered in future studies.
The original contributions presented in the study are included in the article/supplementary material, further inquiries can be directed to the corresponding author/s.
PC led the overall study. HZ, ZD, and XJ conducted the analysis. HZ, ZD, XJ, PZ, and SZ drafted the manuscript with input from the other authors. All authors contributed to the concept and design of the study, the interpretation of data, revision of the manuscript for important intellectual content, and have read and approved the final version of the manuscript.
This research was funded by Jiangsu Natural Science Fund (BK20200782) and Innovation and entrepreneurship training program for college students in Jiangsu Province (202110298026Z).
The authors declare that the research was conducted in the absence of any commercial or financial relationships that could be construed as a potential conflict of interest.
All claims expressed in this article are solely those of the authors and do not necessarily represent those of their affiliated organizations, or those of the publisher, the editors and the reviewers. Any product that may be evaluated in this article, or claim that may be made by its manufacturer, is not guaranteed or endorsed by the publisher.
1. Kamali M, Hewage K. Development of performance criteria for sustainability evaluation of modular versus conventional construction methods. J Clean Prod. (2017) 142:3592–606. doi: 10.1016/j.jclepro.2016.10.108
2. Hong J, Shen GQ, Li Z, Zhang B, Zhang W. Barriers to promoting prefabricated construction in China: a cost–benefit analysis. J Clean Prod. (2018) 172:649–60. doi: 10.1016/j.jclepro.2017.10.171
3. Liu WH, Zhang H, Wang Q, Hua TR, Xue H. A review and scientometric analysis of global research on prefabricated buildings. Adv Civ Eng. (2021) 2021:18. doi: 10.1155/2021/8869315
4. Man SS, Chan AHS, Alabdulkarim S. Quantification of risk perception: development and validation of the construction worker risk perception (CoWoRP) scale. J Safety Res. (2019) 71:25–39. doi: 10.1016/j.jsr.2019.09.009
5. Franks E. Safety and Health in Prefabricated Construction: A New Framework for Analysis. Seattle: University of Washington (2018).
6. Jones W, Gibb A, Haslam R, Dainty A. Work-related ill-health in construction: the importance of scope, ownership and understanding. Saf Sci. (2019) 120:538–50. doi: 10.1016/j.ssci.2019.07.038
7. Szamocki N, Kim M-K, Ahn CR, Brilakis I. Reducing greenhouse gas emission of construction equipment at construction sites: field study approach. J Constr Eng Manag. (2019) 145:05019012. doi: 10.1061/(ASCE)CO.1943-7862.0001690
8. Pervez H, Ali Y, Petrillo A. A quantitative assessment of greenhouse gas (GHG) emissions from conventional and modular construction: a case of developing country. J Clean Prod. (2021) 294:126210. doi: 10.1016/j.jclepro.2021.126210
9. Melia P, Ruggieri G, Sabbadini S, Dotelli G. Environmental impacts of natural and conventional building materials: a case study on earth plasters. J Clean Prod. (2014) 80:179–86. doi: 10.1016/j.jclepro.2014.05.073
10. Luo QM, Huang LP, Xue XY, Chen ZS, Zhou FB, Wei LH, et al. Occupational health risk assessment based on dust exposure during earthwork construction. J Build Eng. (2021) 44:103186. doi: 10.1016/j.jobe.2021.103186
11. Tong R, Cheng M, Zhang L, Liu M, Yang X, Li X, et al. The construction dust-induced occupational health risk using Monte-Carlo simulation. J Clean Prod. (2018) 184:598–608. doi: 10.1016/j.jclepro.2018.02.286
12. Zhai L, Zhao J, Xu B, Deng Y, Xu Z. Influence of indoor formaldehyde pollution on respiratory system health in the urban area of Shenyang, China. Afr Health Sci. (2013) 13:137–43. doi: 10.4314/ahs.v13i1.19
13. Tang XJ, Bai Y, Duong A, Smith MT, Li LY, Zhang LP. Formaldehyde in China: production, consumption, exposure levels, and health effects. Environ Int. (2009) 35:1210–24. doi: 10.1016/j.envint.2009.06.002
14. Liu JH, Guo YM, Pan XC. Study of the current status and factors that influence indoor air pollution in 138 houses in the urban area in Xi'an. In: Carpenter DO, editor. Environmental Challenges in The Pacific Basin. Chapter 12th International Conference of the Pacific-Basin-Consortium-for-Environment-and-Health-Sciences. (2008). p. 246–55.
15. Hadei M, Hopke PK, Rafiee M, Rastkari N, Yarahmadi M, Kermani M, et al. Indoor and outdoor concentrations of BTEX and formaldehyde in Tehran, Iran: effects of building characteristics and health risk assessment. Environ Sci Pollut Res. (2018) 25:27423–37. doi: 10.1007/s11356-018-2794-4
16. Choi J, Kang H, Hong T, Baek H, Lee D-E. Automated noise exposure assessment model for the health of construction workers. Autom Constr. (2021) 126:103657. doi: 10.1016/j.autcon.2021.103657
17. Li X, Song Z, Wang T, Zheng Y, Ning X. Health impacts of construction noise on workers: a quantitative assessment model based on exposure measurement. J Clean Prod. (2016) 135:721–31. doi: 10.1016/j.jclepro.2016.06.100
18. Dabirian S, Han SH, Lee J. Stochastic-based noise exposure assessment in modular and off-site construction. J Clean Prod. (2020) 244:118758. doi: 10.1016/j.jclepro.2019.118758
19. Chong D, Wang Y, Zhao K, Wang D, Oeser M. Asphalt fume exposures by pavement construction workers: current status and project cases. J Constr Eng Manag. (2018) 144:05018002. doi: 10.1061/(ASCE)CO.1943-7862.0001454
20. Yin X. Pollution pattern of formaldehyde and TVOC in indoor air and its control measures. Nat Environ Pollut Technol. (2017) 16:579–85. Available online at: https://neptjournal.com/upload-images/NL-60-34-(32)G-113com.pdf
21. Acharya P, Boggess B, Zhang K. Assessing heat stress and health among construction workers in a changing climate: a review [review]. Int J Environ Res Public Health. (2018) 15:16. doi: 10.3390/ijerph15020247
22. Li XD, Chow KH, Zhu YM, Lin Y. Evaluating the impacts of high-temperature outdoor working environments on construction labor productivity in China: a case study of rebar workers. Build Environ. (2016) 95:42–52. doi: 10.1016/j.buildenv.2015.09.005
23. Yi W, Chan AP. Optimizing work–rest schedule for construction rebar workers in hot and humid environment. Build Environ. (2013) 61:104–13. doi: 10.1016/j.buildenv.2012.12.012
24. Chan AP, Yam MC, Chung JW, Yi W. Developing a heat stress model for construction workers. J Facil Manag. (2012) 10:59–74. doi: 10.1108/14725961211200405
25. Health in the Construction Industry. Netherlands: European Agency for Safety and Health at Work (2013).
27. Ilbahar E, Karasan A, Cebi S, Kahraman C. A novel approach to risk assessment for occupational health and safety using Pythagorean fuzzy AHP and fuzzy inference system. Saf Sci. (2018) 103:124–36. doi: 10.1016/j.ssci.2017.10.025
28. Ajslev J, Dastjerdi EL, Dyreborg J, Kines P, Jeschke KC, Sundstrup E, et al. Safety climate and accidents at work: cross-sectional study among 15,000 workers of the general working population. Saf Sci. (2017) 91:320–5. doi: 10.1016/j.ssci.2016.08.029
29. Liu H, Li J, Li HY, Li H, Mao P, Yuan JF. Risk perception and coping behavior of construction workers on occupational health risks-a case study of Nanjing, China. Int J Environ Res Public Health. (2021) 18:7040. doi: 10.3390/ijerph18137040
30. USEPA. Risk Assessment Guidance for Superfund Volume I: Human Health Evaluation Manual. Office of Emergency and Remedial Response, US Environmental Protection Agency. EPA/540/1-89/002 Washington, DC (1989). Available online at: https://www.epa.gov/sites/default/files/2015-09/documents/rags_a.pdf
31. Pece S, Dascalescu A, Ruscu O. Risk Assessment Method for Occupational Accidents Diseases. Bucharest: Ministry of Labor Social Protection (1998). Available online at: http://protectiamuncii.ro/pdfs/risk_assessment_method.pdf
32. Xie BB, Chang W, Wu K, Guo LL, Mei Y. Application of three risk assessment methods to noise risk assessment in an automobile foundry enterprise. Chin J Indust Hygiene Occup Dis. (2022) 40:271–5. doi: 10.3760/cma.j.cn121094-20210109-00036
33. Zhao X, Zeng Q, Liu J, Ni Y, Wang X, Gu Q. Application of five methods in the occupational health risk assessment of workers exposed to welding fumes. Chin J Indust Hygiene Occup Dis. (2021) 39:375–8. doi: 10.3760/cma.j.cn121094-20200630-00368
34. Zhang X, Huang GH. Assessment of BTEX-induced health risk under multiple uncertainties at a petroleum-contaminated site: an integrated fuzzy stochastic approach. Water Resour Res. (2011) 47:11–3. doi: 10.1029/2011WR010682
35. Hassim MH. Comparison of methods for assessing occupational health hazards in chemical process development and design phases. Curr Opin Chem Eng. (2016) 14:137–49. doi: 10.1016/j.coche.2016.10.001
36. Yasli F, Bolat B. A novel risk analysis approach for occupational safety using Bayesian Network and interval type-2 fuzzy sets: the case of underground mining. J Intell Fuzzy Syst. (2022) 42:265–82. doi: 10.3233/JIFS-219191
37. Liu R, Liu Z, Liu HC, Shi H. An improved alternative queuing method for occupational health and safety risk assessment and its application to construction excavation. Autom Constr. (2021) 126:103672. doi: 10.1016/j.autcon.2021.103672
38. van der Voet H, Kruisselbrink JW, de Boer WJ, van Lenthe MS, van den Heuvel JJB, Crepet A, et al. The MCRA toolbox of models and data to support chemical mixture risk assessment. Food Chem Toxicol. (2020) 138:14. doi: 10.1016/j.fct.2020.111185
39. Kuepfer L, Niederalt C, Wendl T, Schlender JF, Willmann S, Lippert J, et al. Applied concepts in PBPK modeling: how to build a PBPK/PD model. CPT Pharmacometrics Syst Pharmacol. (2016) 5:516–31. doi: 10.1002/psp4.12134
40. Ay C, Guler T, Besikci EB. Implementation of ARAMIS methodology in the risk assessment of chemical tankers: the case of loading operation. Ocean Eng. (2022) 261:22. doi: 10.1016/j.oceaneng.2022.112211
42. Slovic P, Peters E, Finucane ML, MacGregor DG. Affect, risk, and decision making. Health Psychol. (2005) 24:S35. doi: 10.1037/0278-6133.24.4.S35
43. Raponi F, Bauleo L, Ancona C, Forastiere F, Paci E, Pigini D, et al. Quantification of 1-hydroxypyrene, 1-and 2-hydroxynaphthalene, 3-hydroxybenzo[a]pyrene and 6-hydroxynitropyrene by HPLC-MS/MS in human urine as exposure biomarkers for environmental and occupational surveys. Biomarkers. (2017) 22:575–83. doi: 10.1080/1354750X.2016.1252959
44. Mohammadi A, Faraji M, Ebrahimi AA, Nemati S, Abdolahnejad A, Miri M. Comparing THMs level in old and new water distribution systems; seasonal variation and probabilistic risk assessment. Ecotoxicol Environ Saf. (2020) 192:110286. doi: 10.1016/j.ecoenv.2020.110286
45. Hope BK. An examination of ecological risk assessment and management practices. Environ Int. (2006) 32:983–95. doi: 10.1016/j.envint.2006.06.005
46. Lewtas J. Air pollution combustion emissions: characterization of causative agents and mechanisms associated with cancer, reproductive, and cardiovascular effects. Mutat Res. (2007) 636:95–133. doi: 10.1016/j.mrrev.2007.08.003
47. Chen HY, Teng YG, Lu SJ, Wang YY, Wang JS. Contamination features and health risk of soil heavy metals in China. Sci Total Environ. (2015) 512:143–53. doi: 10.1016/j.scitotenv.2015.01.025
48. Hoang HG, Chiang CF, Lin C, Wu CY, Lee CW, Cheruiyot NK, et al. Human health risk simulation and assessment of heavy metal contamination in a river affected by industrial activities. Environ Pollut. (2021) 285:117414. doi: 10.1016/j.envpol.2021.117414
49. Murray CJ. Quantifying the burden of disease: the technical basis for disability-adjusted life years. Bull World Health Organ. (1994) 72:427–45.
Keywords: prefabricate concrete, occupational health risks, environmental release, LCA, risk management assessment
Citation: Cui P, Zhao H, Dong Z, Ju X, Zou P and Zhou S (2022) Modeling the occupational health risk of workers caused by environmental release during the production of PC components. Front. Public Health 10:1076461. doi: 10.3389/fpubh.2022.1076461
Received: 21 October 2022; Accepted: 07 November 2022;
Published: 22 November 2022.
Edited by:
Luigi Vimercati, University of Bari Aldo Moro, ItalyCopyright © 2022 Cui, Zhao, Dong, Ju, Zou and Zhou. This is an open-access article distributed under the terms of the Creative Commons Attribution License (CC BY). The use, distribution or reproduction in other forums is permitted, provided the original author(s) and the copyright owner(s) are credited and that the original publication in this journal is cited, in accordance with accepted academic practice. No use, distribution or reproduction is permitted which does not comply with these terms.
*Correspondence: Peng Cui, Y3VpQG5qZnUuZWR1LmNu
Disclaimer: All claims expressed in this article are solely those of the authors and do not necessarily represent those of their affiliated organizations, or those of the publisher, the editors and the reviewers. Any product that may be evaluated in this article or claim that may be made by its manufacturer is not guaranteed or endorsed by the publisher.
Research integrity at Frontiers
Learn more about the work of our research integrity team to safeguard the quality of each article we publish.