- 1Instituto de Parasitología y Biomedicina López-Neyra, Consejo Superior de Investigaciones Científicas (IPBLN, CSIC), Granada, Spain
- 2Institut de Recherche en Sciences de la Santé (IRSS), Bobo-Dioulasso, Burkina Faso
- 3Institut des Sciences et Techniques (INSTech), Bobo-Dioulasso, Burkina Faso
- 4Instituto de Biomedicina de Valencia, Consejo Superior de Investigaciones Científicas (IBV, CSIC), Valencia, Spain
- 5Centre MURAZ, Bobo-Dioulasso, Burkina Faso
- 6Centro de Investigación Biomédica en Red en Epidemiología y Salud Pública (CIBER), Madrid, Spain
- 7Division of Virology, Department of Pathology, University of Cambridge, Cambridge, United Kingdom
Africa accounts for 1.5% of the global coronavirus disease 2019 (COVID-19) cases and 2.7% of deaths, but this low incidence has been partly attributed to the limited testing capacity in most countries. In addition, the population in many African countries is at high risk of infection with endemic infectious diseases such as malaria. Our aim is to determine the prevalence and circulation of SARS-CoV-2 variants, and the frequency of co-infection with the malaria parasite. We conducted serological tests and microscopy examinations on 998 volunteers of different ages and sexes in a random and stratified population sample in Burkina-Faso. In addition, nasopharyngeal samples were taken for RT-qPCR of SARS-CoV-2 and for whole viral genome sequencing. Our results show a 3.2 and a 2.5% of SARS-CoV-2 seroprevalence and PCR positivity; and 22% of malaria incidence, over the sampling period, with marked differences linked to age. Importantly, we found 8 cases of confirmed co-infection and 11 cases of suspected co-infection mostly in children and teenagers. Finally, we report the genome sequences of 13 SARS-CoV-2 isolates circulating in Burkina Faso at the time of analysis, assigned to lineages A.19, A.21, B.1.1.404, B.1.1.118, B.1 and grouped into clades; 19B, 20A, and 20B. This is the first population-based study about SARS-CoV-2 and malaria in Burkina Faso during the first wave of the pandemic, providing a relevant estimation of the real prevalence of SARS-CoV-2 and variants circulating in this Western African country. Besides, it highlights the non-negligible frequency of co-infection with malaria in African communities.
Introduction
To date (mid-November 2022), the SARS-CoV-2 coronavirus, responsible of the coronavirus disease 19 (COVID-19), has caused more than 660 million cases and 6,6 million deaths worldwide, a small proportion of these (9,3 million cases [1.5%] and around 174,407 deaths [2.7%]) have occurred in Africa (data as of September 13th 2022, WHO1). Despite their under-funded health systems, cases and deaths reported in Africa appear low compared to other parts of the world. In addition, most of cases cluster in few Northern and Southern countries (i.e., South Africa [4,01M cases], Morocco [1,26M cases] and Tunisia [1,14M cases]), while data in most Western African countries is scarce or absent (i.e., the number of accumulative cases considering all countries in Western Africa is ~936 K). However, these estimations should be considered with caution given the low number of tests conducted in most African countries compared to data available for Europe (one or two orders of magnitude higher). A more proper effort to estimate the real incidence is needed to make conclusions about the extent of this pandemic in the African continent.
To prevent the emergence and spread of new variants across the world, sequencing of SARS-CoV-2 lineages circulating in Africa is critical (1). Indeed, the higher rates of COVID-19 testing and genomic surveillance in the south of the continent have permitted the early identification of several variants of concern (VOC) such as Beta (B.1.351) and Omicron (B.1.1.529); and different variants of interest (VOI) such as C.1.1 (2, 3). To date, most of the deposited sequences from Africa in the GISAID database come from the South African region (47%), while sequences from Western African countries only account for 17.6% (GISAID2 as of 31 May 2022) (4). Such an uneven African genomic surveillance holds a weakness in our global surveillance system and raises the concern of not leaving Africa behind in the global pandemic response (1).
Besides the direct effects of COVID-19 in terms of cases and deaths, the pandemic has shown to have important collateral effects on several other infectious diseases such as HIV/AIDS, tuberculosis and malaria (5). According to the World Health Organization (WHO), half of all deaths in Africa are caused by these three infectious diseases (~2.4 million people/year) compared to only 2% in Europe. The Sub-Saharan Africa is one of the most affected regions with significant drops in notifications of all three diseases. According to the latest WHO World malaria report3, 15 malaria endemic countries reported more than 20% reduction in malaria testing and treatment between April and June of 20203.
Malaria is a life-threatening disease caused by unicellular eukaryotic parasites of the genus Plasmodium that are transmitted to humans through the bites of infected female Anopheles mosquitoes. Sub-Saharan Africa carries a disproportionate portion of the global malaria burden. In 2020, the region was home to 95% of malaria cases and deaths. Children below 5 years are the most vulnerable group affected by malaria; accounting for 80% of all malaria deaths in the region3. At the beginning of the COVID-19 pandemic, studies projected that malaria deaths could double in 2020 due to health service disruptions (6). However, many countries took urgent actions to avoid the worst projections. In 2020, there were an estimated 241 million cases of malaria and 627,000 deaths worldwide, this is 14 million more cases and 69,000 more deaths than in 2019. Two-thirds of this increase have been associated to disruption in malaria prevention campaigns, diagnosis and treatment during the first wave of the pandemic3.
Burkina Faso is a Western African country that confirmed its first community transmission cases of SARS-CoV-2 on March 15, 2020. To date, this country has reported 21,128 total cases which is a very low incidence compared with the 4M of total cases reported from South Africa, for example. Whether this rate is representative of the real epidemiological situation of the country, or if it is due to insufficient testing, remains and it will probably remain unanswered. On the other hand, malaria that is endemic across the country, is a major health issue with a seasonal peak from June to October. During the COVID-19 pandemic, malaria case incidence (cases per 1,000 population at risk) increased from 366.1 in 2019 to 389.9 in 20204. The high malaria incidence in this country and the increase in prevalence detected during the first wave of the COVID-19 pandemic raised the question about how frequent SARS-CoV-2 and malaria co-infection has been.
In order to address this, 998 volunteers from different rural and urban areas in the south of Burkina Faso were screened for malaria by microscopy, and for SARS-CoV-2 using rapid diagnostic serology tests and RT-qPCR. In addition, SARS-CoV-2 PCR positive samples were whole-genome sequenced and analyzed through comparative genomics and phylogenetics. We found a 3.2% seroprevalence and 2.5% of PCR positivity for SARS-CoV-2 over the studied period. Although age groups mostly affected by each disease do not overlap, 19 cases of SARS-CoV-2/malaria co-infection mostly in younger age groups were found. Finally, we reported 13 SARS-CoV-2 whole genomes circulating in Burkina Faso at the time of sampling. Viral genomes clustered into 3 early clades (i.e., 19B, 20A, and 20B) and lineages found were A.19, A.21, B.1.1.404, B.1.1.118, and B.1.
Altogether, our data shed new light on the incidence of COVID-19 and the different SARS-CoV-2 circulating variants in a Western African country, and its co-occurrence with another endemic infectious disease of utter importance such as malaria.
Methods
Ethics
This protocol was carried out by the Institut des Sciences et Techniques de Bobo-Dioulasso and was approved by the Spanish National Research Council (CSIC), the Ethics Committee of Biomedical Research in Andalusia (CCEIBA), and the Center National de la Recherche Scientifique et Technologique (CNRST) of Burkina-Faso. The protocol is in conformity with the declaration of Helsinki and all international regulations about the ethical principles in biomedical research involving human subjects. SARS-CoV-2 seropositive and malaria positive individuals were provided with treatment and assisted by medical professionals. During the screening, local authorities, school directors and families of volunteers were informed about the objectives of the study and protocols performed. As the study involved children, a signed declaration of conformity for the participation in the study was obtained from their parents and/or legal representatives.
Study design
The study was conducted in 11 villages from different rural and urban areas in the south of Burkina Faso (Supplementary Figure S1). From August to November 2020, a total of 998 asymptomatic volunteers were enrolled and evaluated. Demographic and clinical information collected included age, sex and temperature. For each participating subject, two drops of blood were obtained by capillary puncture of the fingertip. This blood was used for malaria detection by microscopy and PCR, and for COVID-19 serology testing using a point of care rapid test (INgezim COVID-19 CROM Easy). When there was consent, nasopharyngeal samples were also collected in AVL buffer (Qiagen) for molecular testing of SARS-CoV-2 using RT-qPCR.
SARS-CoV-2 diagnosis
Detection of anti-SARS-CoV-2 antibodies was performed on volunteers' capillary blood using the INgezim COVID-19 CROM Easy kit (Gold Standard Diagnostics), following manufacturer's instructions. This serology-based finger-prick test is based on a dual-recognition immunochromatographic assay that determines the presence of total antibodies (IgG, IgA, and IgM) specific to SARS-CoV-2 in a single blood sample by using the nucleoprotein (N protein) as antigen. The manufacturer reported specificity of 99.3% and sensitivity of 94.5%. No cross-reactivity with other coronaviruses or agents related to human respiratory illnesses have been reported. In this study, we estimated seroprevalence as the proportion of individuals who had a positive result in the point-of-care test after 10 min.
Nasopharyngeal samples were taken using a sterile swab. The swab was immediately placed in a pre-filled tube containing 0.5 mL of inactivating lysis buffer (AVL, Qiagen). Samples were stored at 4°C for 24h and at −20°C for prolonged storage for subsequent analysis. Molecular detection of SARS-CoV-2 by RT-qPCR on seropositive volunteers was carried out in the Institut de Recherche en Sciences de la Santé/Direction Régionale de l'Ouest (IRSS DRO) of Burkina Faso using the FastPlexTriplex SARS-CoV-2 detection kit (gene target identified: ORF1ab, N and the human RNase P as control) (PreciGenome, LLC).
Swab samples of seronegative volunteers were stored at −80°C and processed in the Institute of Parasitology and Biomedicine Lopez-Neyra (IPBLN) as follows: RNA was extracted from 140 μL of pooled swab samples using the QIAamp viral RNA extraction kit (Qiagen). A pool size of five samples was used in the villages with seropositive subjects (Bobo-Dioulasso and Dandé), whereas samples from villages with negative seroprevalence were processed in pools of 10 samples. RT-qPCR analysis was performed using the one step Direct SARS-CoV-2 Realtime PCR Kit (Vircell S.L) following the manufacturer's instructions. Targets identified with this kit are N, E and hRNase P genes. RT-qPCR was performed in 20 μL final volume reactions (5 μL of RNA sample) using a CFX96 Real-Time PCR Detection System (Bio-Rad, Hercules, CA, USA). Cycle threshold (Ct) values were analyzed using the BIORAD CFX manager software. Samples of positive pools were extracted individually and retested by RT-qPCR. Samples were considered positive when the N and E target genes had a Ct <40. Positive and negative controls were included in all experiments.
Whole genome sequencing and phylogenetic analysis
RNA from SARS-CoV-2 positive samples with a Ct value ≤35 was sent to sequencing to the Institute of Biomedicine of Valencia (IBV-CSIC). RNA was retrotranscribed into cDNA and SARS-CoV-2 complete genome amplification was performed in two multiplex PCR, accordingly to openly available protocol developed by the ARTIC network5 using the V4.1 multiplex primers scheme (artic-network n.d.). Two resulting amplicon pools were combined and used for library preparation. Genomic libraries were constructed with the Nextera DNA Flex Sample Preparation kit (Illumina Inc., San Diego, CA) according to the manufacturer's protocol with 5 cycles for indexing PCR. Whole genome sequencing was carried out in a MiSeq platform (2 × 150 cycles paired-end run; Illumina). Sequences obtained went through an open source bioinformatic pipeline based on IVAR6 (7). The different steps of the pipeline are as follows: (1) removal of human reads with Kraken (8); (2) filtering of the fastq files using fastp v 0.20.1 (9) (arguments: –cut tail, –cut-window-size, –cut-mean-quality, -max_len1, -max_len2); (3) mapping and variant calling using IVAR v 1.2; and 4) quality control assessment with MultiQC (10). Consensus sequences generated by this pipeline were aligned against the SARS-CoV-2 reference sequence (11) with MAFFT (12). Problematic positions were masked using the mask_alignment.py script from the repository maintained by Rob Lanfear7. Lineages and clade nomenclature were assigned using Pangolin (13) and Nexclade online tool (14). Phylogenetic tree was generated with Nextclade v2.5.08, the tree was downloaded in JSON format and visualized using Auspice v2.37.3 online tool9 (15, 16).
Malaria diagnosis
Malaria prevalence was estimated in the same population sample of 998 volunteers simultaneously to SARS-CoV-2 detection. Detection of malaria was performed by microscopic examination of blood thick smears that were prepared following standard methods (17). Blood thick smears were screened for the presence of Plasmodium falciparum asexual and gametocyte stages in the blood.
Additionally, to have a more precise estimation of malaria positivity on the set of SARS-CoV-2 positive samples, we performed molecular detection of P. falciparum by PCR as follows: DNA was extracted from dried blood samples collected on filter paper using the DNeasy Blood & Tissue Kit (Qiagen). PCR detection of P. falciparum was carried out as previously described (18). Briefly, primers Pf1 5'-GGAATGTTATTGCTAACAC and Pf2 5'-AATGAAGAGCTGTGTATC targeting a region of the mitochondrial cox1 gene (19) were used in a PCR master mix with 2 μL of extracted DNA. PCRs were performed twice and products were visualized by gel electrophoresis. A sample was considered co-infection, when positive in at least one of the two PCRs.
Statistical analysis
We tested the association between variables with the Wilcoxon Sum Rank tests (for continuous quantitative variables) and the Kruskal-Wallis chi-squared test.
Results and discussion
COVID-19 transmission in Africa has been marked by relatively fewer infections, mostly asymptomatic, and lower death rate compared to developed countries. A younger population structure and a variety of socio-ecological factors (i.e., warm weather, low population density and mobility and trained immunity by previous high burden infectious diseases), as well as early public health measures taken by governments (i.e., early lockdown sub-Saharan countries) (20), have been suggested to explain such lower incidence (21). Surprisingly, the pandemic has been more pronounced in a few countries (e.g., South Africa, Morocco and Tunisia) suggesting country-specific drivers of SARS-CoV-2 spread and morbidity, but this could also be largely explained by a low and unequal testing capacity. Reported cases of COVID-19 are dissimilar between countries like South Africa (4.01 million cases and 102,129 deaths), Kenia (338,301 cases and 5,674 deaths) or Ethiopia (493,353 cases and 7,572 deaths). Testing also differs greatly, with 50,000 tests/day in South Africa vs. 5,000 tests/day in Kenia or Ethiopia. In Burkina Faso, with a population of 20,903,278, only 21,128 cases and 387 deaths have been reported [as of September 13th 2022, WHO (see text footnote 1)]. However, no data about the testing effort in Burkina Faso are available for comparison.
At the beginning of the pandemic, serological surveillance was used as a prospective tool to define the cumulative incidence of COVID-19, particularly in the presence of asymptomatic or mild infections (22). At present, seven countries in Western Africa, four countries in Eastern Africa and four countries in Central Africa have conducted seroprevalence studies that are published or available as preprint (Supplementary Table S1). Serological surveys in African countries show highly heterogeneous results with SARS-CoV-2 seroprevalence ranging from 0.9 to 45% during 2020 (Supplementary Table S1). To our knowledge, no data have been reported from Burkina Faso for any period.
In this study, we report the results of a population-based and stratified survey involving a total of 998 volunteers that were recruited during a period of 3 months in 2020 (August 22nd to November 19th). The sampling was conducted in 11 villages from different rural and urban areas in the south of Burkina Faso (Supplementary Figure S1). Fifty Five percent of the participants (549 out of 998) were female, and 45% (449 out of 998) were male. The samples were distributed equally into four age groups: (A) 5–12 years (25.2%); (B) 13–20 years (24.9%); (C) 21–40 years (25.2%); and (D) >40 years (24.7%). Importantly, all the participants were asymptomatic. The distribution of positive cases by age and gender classes is detailed in Table 1.
A total of 32 individuals out of 998 tested positive for SARS-CoV-2 total antibodies (IgG/A/M), resulting in an overall seroprevalence rate of 3.2% (95% CI 2.1–4.3). However, most of seropositives came from the urban city of Bobo-Dioulasso where local seroprevalence was of 4.96% at that time. A higher seroprevalence in urban areas in Africa has been reported previously (23–26). Apart from urban vs. rural differences, we report significant differences between age groups in SARS-CoV-2 seropositivity (Kruskal-Wallis chi-squared = 16.285, df = 3, p-value < 0.001). The highest seroprevalence was in the group age of >40 years (6.9% [95% CI 3.7–10.1]), followed by teenagers aged 13–20 years (2.8% [95% CI 0.7–4.9]) and children aged 5–12 years (2.4% [95% CI 0.5–4.3]), whereas the lowest seroprevalence was observed in the group of 21–40 years (0.8% [95% CI 0–1.9]) (Figure 1A, Table 1). However, there were no significant differences in SARS-CoV-2 seroprevalence between female and male individuals (Wilcoxon rank sum test W = 15,690, p-value = 0.78) (Supplementary Figure S2), which agrees with other population-wide seroprevalence surveys in Sub-Saharan Africa (24, 27, 28). Finally, we also observed that SARS-CoV-2 seroprevalence varied markedly with time with a significant increase in the incidence with a maximum of ~5.5% in November 2020 (Supplementary Figure S3). This increase in infection rate agrees with previously published reports from the African CDC and WHO (see text footnote 1) that observed a gradually increase in cases since September 2020.
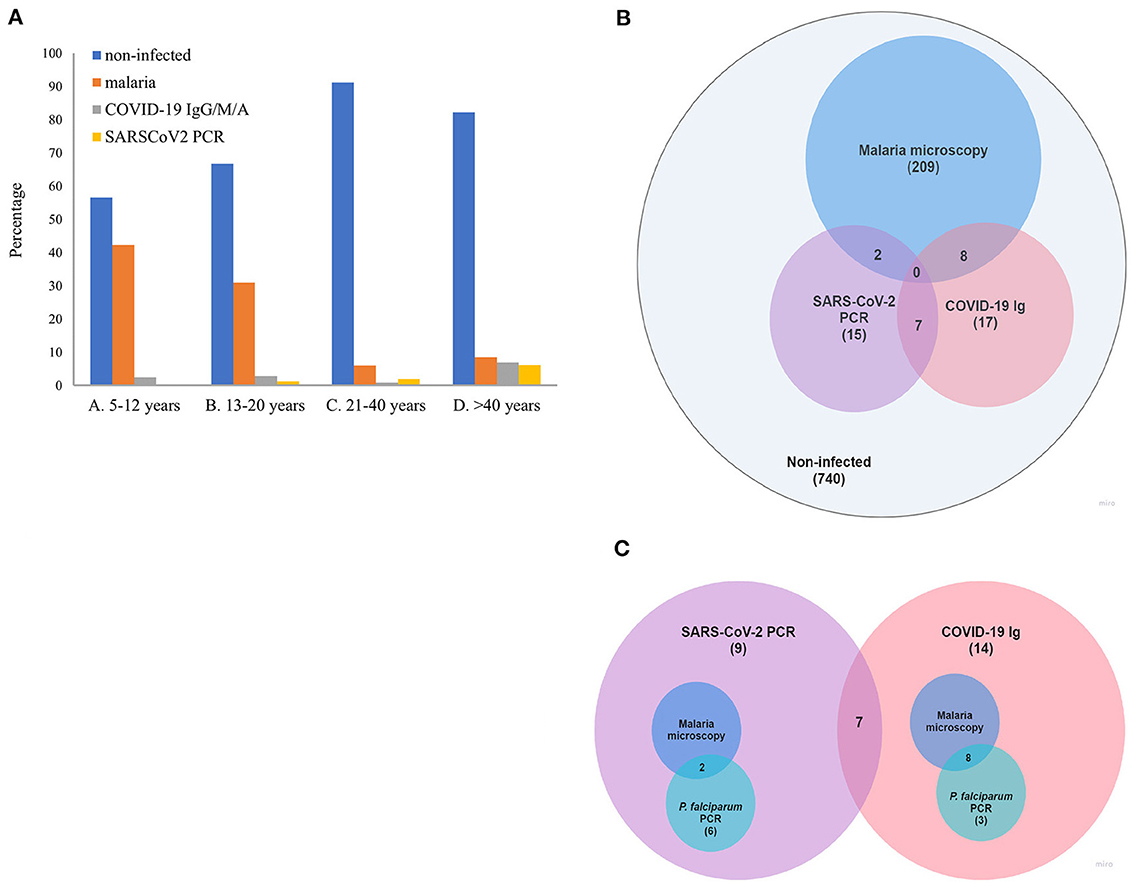
Figure 1. (A) Prevalence of SARS-CoV-2 and malaria by age group. (B) Venn diagram representation of positive SARS-CoV-2 and malaria cases in the whole sampled population. (C) Venn diagram representation of SARS-CoV-2/malaria co-infection in the set of SARS-CoV-2 positive samples.
Furthermore, seroprevalence estimates for SARS-CoV-2 obtained in this study are also comparable with results reported in other African countries during 2020 (although sample period and the testing effort might be different) (Supplementary Table S1). For example, in a serosurvey of asymptomatic people conducted in May 2020 in Addis Ababa (Ethiopia), 3.0% tested positive for SARS-CoV-2 IgG (29). Similarly, a seroprevalence study of 3,098 Kenyan blood donors sampled between April-June 2020 reported a national seroprevalence of 4.3%, with some urban regions reaching around 8% (e.g., Nairobi and Mombasa) (23). Another study in Ethiopia during July 2020 in 14 urban areas found a seroprevalence of 3.5% from 16,932 samples (24).
The 32 SARS-CoV-2 seropositive individuals were subjected to individual RT-qPCR. SARS-CoV-2 active infection was confirmed in 7 samples (seropositive/PCR positive). Due to the low sensitivity of rapid antibody tests to detect early infections, all the seronegative samples were also analyzed by RT-qPCR. These swab samples were first processed in pools, and then, positive pools were processed individually. Pooled testing is a useful approach to reduce time, cost and increase testing capacity in areas with low prevalence of COVID-19. PCR pooling works with maximum efficiency with a prevalence <3% and a pool size <10 (30, 31). First, we tested the sensitivity of our “pool-test” in groups of five (one positive sample diluted in four negative ones) and ten (one positive sample diluted in nine negative ones) samples (Supplementary Table S2). With these results, we decided to group samples in pools of five in areas with seropositive subjects (i.e., Bobo-Dioulasso and Dandé); whereas samples from areas with zero seroprevalence, pools of 10 swabs were processed instead. RNA was extracted from pooled swab samples and RT-PCR was performed. Then, samples in positive pools were extracted and tested individually. With this procedure, we found 17 additional SARS-CoV-2 positive samples by RT-qPCR, most of them from the urban locality of Bobo-Dioulasso (Table 1, Figure 1B). In concordance with serological tests, there were not significant differences in SARS-CoV-2 PCR positivity between females and males (Wilcoxon rank sum test W = 125,348, p-value = 0.08114) (Supplementary Figure S2). However, there were significant differences between age groups (Kruskal-Wallis chi-squared = 20.155, df = 3, p-value < 0.001) with the highest prevalence in the age group of >40 years decreasing with age. The lowest prevalence was observed in children between 5 and 12 years (Figure 1A, Table 1).
19 out of 24 SARS-CoV-2 PCR positive samples had a Ct value ≤35 and they were processed for whole-genome sequencing. After genome assembly and quality control processes, good quality genomes were obtained from 13 samples. To assign the sequences to lineages and clades, we used the dynamic lineage classification method called Phylogenetic Assignment of Named Global Outbreak Lineages (PANGOLIN) (13) and the sequence analysis web tool, Nextclade (14). Phylogenetic analysis showed that our SARS-CoV-2 genomes clustered into three clades: 19B, 20A, and 20B (Table 2, Figure 2). Most of the samples belong to clade 19B, this one along with 19A were the clades that emerged in Wuhan in late 2019 and dominated the early outbreak. Clade 20A emerged from 19A and dominated the European outbreak in March 2020, and 20B is a sub-clade of 20A (32). Lineages found were A.19, A.21, B.1.1.404, B.1.1.118, and B.1. The distribution and description of these lineages10 are summarized in Supplementary Table S3. Lineages A.19, A.21, and B.1.1.404 were common in Burkina Faso at the time of analysis, and importantly, were described there for the first time (see text footnote 10). However, lineages B.1.1.118 and B.1 were not common in Burkina Faso nor-even in Africa at that time (see text footnote 10). As these lineages presented a worldwide distribution, they probably correspond to imported lineages. Indeed, B.1 was reported in Morocco, Saudi Arabia, Spain, France and Brazil on February 202011. A genomic study of SARS-CoV-2 conducted in Ghana with samples from March 12 to April 1 2020 and the end of May 2020, reports the same clades that we found circulating in Burkina Faso from August to November 2020, moreover they also report the linage B.1 (33). Although little divergence was observed (Figure 2) as sequences comes from early days in the pandemic, we detected a common spike mutation in most of the Burkina Faso genomes, D614G mutation (Table 2). A SARS-CoV-2 variant carrying this mutation became dominant during the early days of the pandemic at global level and had a presumed fitness advantage (34). The D614G mutation was also dominant in Guinea genomes (33) and north-middle African genomes (35).
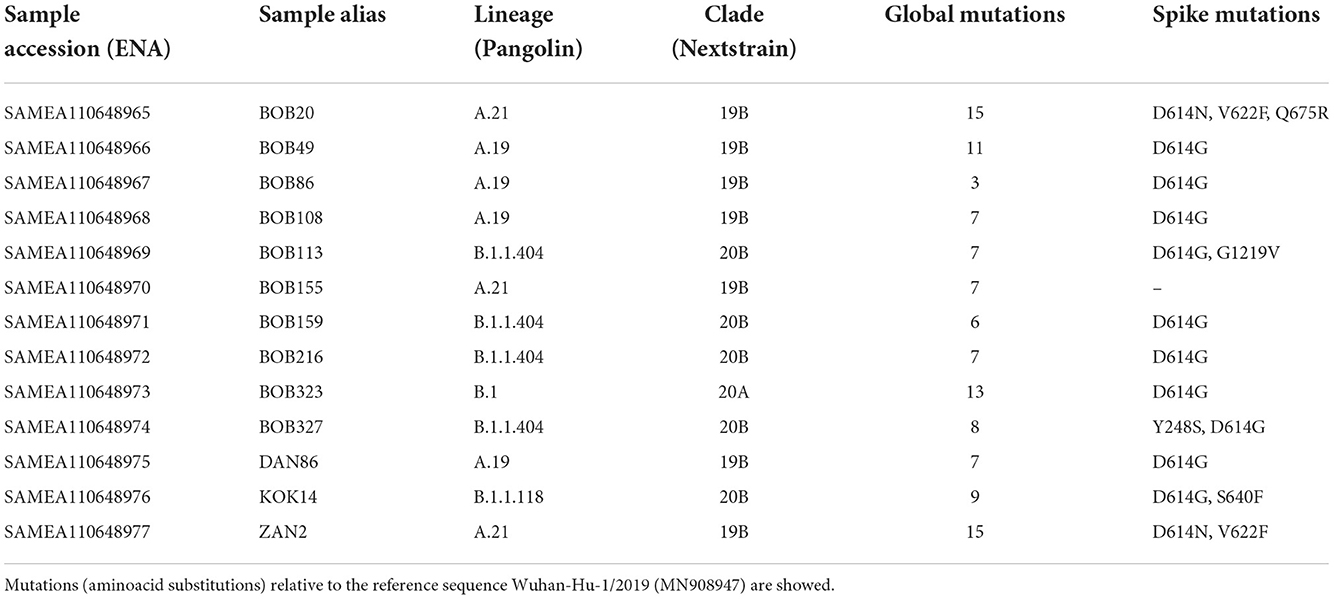
Table 2. Circulating lineages and clades of SARS-CoV-2 in Burkina Faso from August to November 2020.
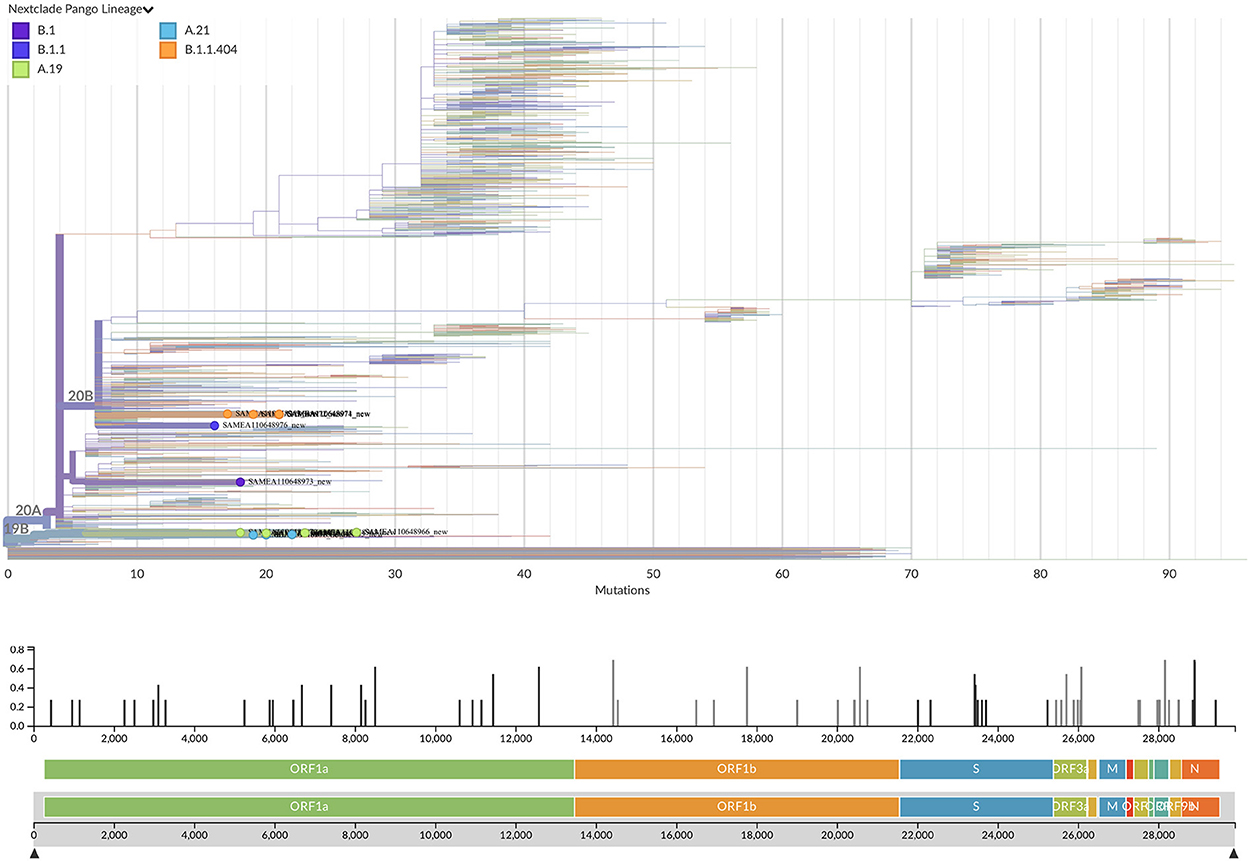
Figure 2. Phylogenetic tree with the 13 new SARS-CoV-2 Burkina Faso genomes placed on a reference tree of 2022 published sequences from all over the world along with the diversity panel below, a bar-chart showing the variation (i.e., mutations) of the 13 sequences in the genome relative to the reference sequence Wuhan-Hu-1/2019 (MN908947). Phylogenetic tree generated with Nextclade online software v2.5.0 (https://clades.nextstrain.org) (accessed on 13 September 2022) and visualized using Auspice v2.37.3 (https://auspice.us/) (accessed on 13 September 2022).
In order to estimate the frequency of SARS-CoV-2/malaria co-infection for the same period and subjects, malaria was screened by microscopy. Out of the 998 sampled individuals, 219 tested positive for malaria by microscopy (22% CI 19.4–24.5). Like in the case of SARS-CoV-2, there were significant differences in malaria prevalence between age groups (Kruskal-Wallis chi-squared = 132.18, df = 3, p-value < 0.001), but in this case, malaria was mostly detected in children below 12 years old (42.2%; 95% CI 36.1–48.4) (Figure 1A, Table 1). In addition, a small sex difference was observed with a higher prevalence in male subjects (W = 92,508, p-value < 0.05) (Supplementary Figure S2). Similar to the temporal trend observed for SARS-CoV-2, malaria cases also showed a significant increase from 5.26% at the beginning of the study to 29.2% at the end of the sampled period (Supplementary Figure S3). This could suggest an impact in malaria control due to the pandemic. Indeed, according to official reports, the incidence of malaria in Burkina Faso (cases per 1, 000 population at risk) increased from 366.1 in 2019 to 389.9 in 202012.
Although thick blood smear examination by microscopy is considered the gold standard for malaria diagnosis in the field, molecular detection by PCR has shown to be more sensitive (18, 36), especially when low-density parasitaemias are present. Therefore, to detect more precisely co-infections, all SARS-CoV-2 positive cases (n = 49) were analyzed by P. falciparum PCR.
Of the 17 SARS-CoV-2 seronegative/PCR positive samples, 8 co-infections with malaria (by microscopy and/or P. falciparum PCR) were reported, whereas for the 25 seropositive/PCR negative subjects, 11 were positive for malaria (Figures 1B,C, Supplementary Table S5). Since the serology test measures total antibodies (IgG/A/M) for SARS-CoV-2, it cannot differentiate recent or past infections, therefore, we cannot rule out whether these data correspond to a co-infection or a sequential infection.
To summarize, we found 8 cases of confirmed SARS-CoV-2 (PCR positive) co-infection with malaria, and 11 cases of suspected co-infection (SARS-CoV-2 seropositive/PCR negative). Of them, twelve were children and teenagers below 20 years, three were adults of 21–40 years and four were adults of >40 years (Supplementary Table S5). There were no significant differences in coinfection frequency between females and males (10 males, 9 females). For five SARS-CoV-2 PCR positive co-infected samples, we had good quality genomes that corresponded to lineages A.19, A.21, B.1.1.404, and B.1.1.118.
Similar low frequency for SARS-CoV-2 and malaria co-infections found in our study (1.9 %) has been also reported in other studies (37–41) (Supplementary Table S4). A systematic review of COVID-19 and malaria coinfection studies has estimated 11% of pooled prevalence (data of 5 studies) with a high degree of heterogeneity (42). However, it is important to note that these studies examined symptomatic patients at the hospital and our study tested stratified asymptomatic people randomly. A recent study reported a higher prevalence of co-infection (12%) with highest prevalence in the age group of 0–20 years (22%) and above 60 years (20%), in this case malaria infection was evaluated in a cohort of hospitalized patients in Uganda with SARS-CoV-2 PCR-confirmed infection (43). As for the symptomatology of co-infections, preliminary data suggest that patients with SARS-CoV-2 and malaria did not seem to have a worst disease outcome but previous malaria exposure seems to be related to less frequency of severe COVID-19 (43). Another study reports that patients co-infected with malaria had significantly faster recovery compared to those not co-infected (41). One limitation of our study to analyze and draw conclusions about clinical outcomes of the co-infections is that all volunteers were asymptomatic. Another limitation in the estimation of SARS-CoV-2 and malaria single infections in the Burkina Faso population could be the smaller sample number of adults >60 years (n = 52), and the fact that children under the age of 5 were not included in the study because of ethical reasons. These two groups are the most hit by COVID-19 and malaria, respectively. In addition, the low frequency of SARS-CoV-2 and malaria parasite single infections in the Burkina population (small sample size of volunteers that were positive for either disease), reduces the probability of find them together.
It has been suggested that the low incidence and mortality of COVID-19 in malaria endemic regions could be related to cross-immunity and common immunodominant epitopes between malaria and SARS-CoV-2 (43–46). In silico analysis have identified potential shared targets for immune response between SARS-CoV-2 and Plasmodium falciparum proteins which could generate cross-reactivity through HLA and CD8+ T-cell activation (45). Moreover, tuberculosis and malaria prevalence had been significantly associated with reduced COVID-19 mortality (46). Previous studies have also indicated that malaria-induced immunomodulation could be protective against respiratory viruses, reducing pulmonary inflammatory response inflammation (47, 48). Alternatively, the higher burden of infectious diseases in sub-Saharan Africa has been suggested to mediate the asymptomatic SARS-CoV-2 infections by induction of immunological tolerance or trained immunity to other infections such as tuberculosis, other human coronaviruses and even Bacillus Calmette-Guérin (BCG) vaccination (21, 46). In addition, other studies have reported that bacterial, fungal and viral co-infections with SARS-CoV-2 are also uncommon, however when present, they may cause a worse outcome (49–51). In the case of coinfections with HIV, the prevalence seems higher (26,6%) and HIV subjects showed an increased risk of hospital admission for COVID-19 (52). However, the studies are still preliminary and further research is needed to test these hypotheses. Therefore, additional prospective studies are required to establish a cause-effect relationship between the SARS-CoV-2 disease outcome and co-infection with other circulating pathogens. These should include more patients, control groups, clinical and immunological information, tracing on SARS-CoV-2 severity when co-occurrence with other prevalent infectious diseases.
Conclusion
To our knowledge, this is the first population-based SARS-CoV-2 prevalence study performed in Burkina-Faso during the first wave of the COVID-19 pandemic. In addition, this is also one of the few studies that examines the co-occurrence of SARS-CoV-2 infection with the malaria parasite in an asymptomatic population.
The findings from this seroprevalence study for SARS-CoV-2 indicate that the prevalence of antibodies against the new coronavirus was around 3% in Burkina Faso at the time of analysis, with large differences in prevalence at local level and between various age classes. This supports the hypothesis that Africa had one of the lowest coronavirus case rates and that would not be due, at least entirely, to a lower testing capacity. Another important finding of our study is the low frequency of co-infection with malaria among the sampled population (1.9 %). Of these, 12 out of the 19 cases reported were in children (4) or teenagers (8), and 4 cases in adults of >40 years.
Finally, the SARS-CoV-2 whole genome sequencing analysis revealed that the circulating lineages found in Burkina Faso during the first wave of the pandemic were early clades derived from the Wuhan strain. Most of the lineages reported have been previously described in Burkina Faso or the neighbor countries. However, we also identified two less frequent lineages that were probably imported to Burkina-Faso from USA or Europe. We believe that data presented in this study help to fill the gap of the SARS-CoV-2 epidemiological situation in sub-Saharan Africa, where interactions with endemic infectious diseases are common.
Data availability statement
The datasets generated for this study can be found in the European Nucleotide Archive (ENA) database (https://www.ebi.ac.uk/ena/browser/home) with project ID PRJEB55393 and sample IDs SAMEA110648965-SAMEA110648977.
Ethics statement
The studies involving human participants were reviewed and approved by Ethics Committee of the Spanish National Research Council (CSIC), the Ethics Committee of Biomedical Research in Andalusia (CCEIBA), and the Ethics Committee of the Center National de la Recherche Scientifique et Technologique (CNRST) of Burkina-Faso. Written informed consent to participate in this study was provided by the participants' legal guardian/next of kin.
Author contributions
Conceptualization was the responsibility of EG-D that also supervised the project with NI. RY and JB provided infrastructure and organized fieldwork activities in Burkina Faso. RY, DS, and AY performed data collection and malaria microscopy and PCR. DL-F and MP-M performed RT-qPCR assays. IC, IG-N, and MT-P conducted whole genome sequencing and phylogenetic analysis. DL-F and EG-D analyzed the data and wrote the manuscript. NI, RY, and IC reviewed the manuscript. All authors read and approved the final manuscript.
Funding
This research work received funding from by the European Commission- NextGenerationEU (Regulation EU 2020/2094) and grant: 202020E159, through CSIC's Global Health Platform (PTI Salud Global) and the Spanish Ministry of Science and Innovation (grant: PID2019-111109RB-I00).
Acknowledgments
We would like to thank all volunteers who participated in this study, as well as the local authorities and communities in Burkina Faso for their support. We also thank the IPBLN, IRSS and IBV core facilities for their support to project activities.
Conflict of interest
The authors declare that the research was conducted in the absence of any commercial or financial relationships that could be construed as a potential conflict of interest.
Publisher's note
All claims expressed in this article are solely those of the authors and do not necessarily represent those of their affiliated organizations, or those of the publisher, the editors and the reviewers. Any product that may be evaluated in this article, or claim that may be made by its manufacturer, is not guaranteed or endorsed by the publisher.
Supplementary material
The Supplementary Material for this article can be found online at: https://www.frontiersin.org/articles/10.3389/fpubh.2022.1048404/full#supplementary-material
Footnotes
2. ^ https://gisaid.org/submission-tracker-global/
3. ^ https://www.who.int/teams/global-malaria-programme/reports/world-malaria-report-2021
4. ^ https://data.worldbank.org/indicator/SH.MLR.INCD.P3?locations=BF, accessed on 27 April 2022.
5. ^ https://www.protocols.io/view/ncov-2019-sequencing-protocol-bp2l6n26rgqe/v1?version_warning=no
6. ^ https://gitlab.com/fisabio-ngs/sars-cov2-mapping
7. ^ https://zenodo.org/record/4069557#.X37nuXUzaWg
8. ^ https://clades.nextstrain.org (accessed on 13 September 2022).
10. ^ https://cov-lineages.org/lineage_list.html
11. ^ https://gisaid.org/phylodynamics/global/nextstrain/
12. ^ https://data.worldbank.org/indicator/SH.MLR.INCD.P3?locations=BF
References
1. Wilkinson E, Giovanetti M, Tegally H, San JE, Lessells R, Cuadros D, et al. A year of genomic surveillance reveals how the SARS-CoV-2 pandemic unfolded in Africa. Science. (2021) 374:423–31. doi: 10.1126/science.abj4336
2. Kannan S, Shaik Syed Ali P, Sheeza A. Omicron (B 11529)—variant of concern—molecular profile and epidemiology: a mini review. Eur Rev Med Pharmacol Sci. (2021) 25:8019–22. doi: 10.26355/eurrev00_202112_27653
3. Tegally H, Wilkinson E, Lessells RJ, Giandhari J, Pillay S, Msomi N, et al. Sixteen novel lineages of SARS-CoV-2 in South Africa. Nat Med. (2021) 27:440–6. doi: 10.1038/s41591-021-01255-3
4. Khare S, Gurry C, Freitas L, Schultz MB, Bach G, Diallo A, et al. GISAID's role in pandemic response. China CDC Wkly. (2021) 3:1049. doi: 10.46234/ccdcw2021.255
5. Hogan AB, Jewell BL, Sherrard-Smith E, Vesga JF, Watson OJ, Whittaker C, et al. Potential impact of the COVID-19 pandemic on HIV, tuberculosis, and malaria in low-income and middle-income countries: a modelling study. Lancet Glob Heal. (2020) 8:e1132–41. doi: 10.1016/S2214-109X(20)30288-6
6. Sherrard-Smith E, Hogan AB, Hamlet A, Watson OJ, Whittaker C, Winskill P, et al. The potential public health consequences of COVID-19 on malaria in Africa. Nat Med. (2020) 26:1411–6. doi: 10.1038/s41591-020-1025-y
7. Grubaugh ND, Gangavarapu K, Quick J, Matteson NL, De Jesus JG, Main BJ, et al. An amplicon-based sequencing framework for accurately measuring intrahost virus diversity using PrimalSeq and iVar. Genome Biol. (2019) 20:1–19. doi: 10.1186/s13059-018-1618-7
8. Wood DE, Salzberg SL. Kraken: Ultrafast metagenomic sequence classification using exact alignments. Genome Biol. (2014) 15:1–12. doi: 10.1186/gb-2014-15-3-r46
9. Chen S, Zhou Y, Chen Y, Gu J. Fastp: an ultra-fast all-in-one FASTQ preprocessor. Bioinformatics. (2018) 34:i884. doi: 10.1093/bioinformatics/bty560
10. Ewels P, Magnusson M, Lundin S, Käller M. MultiQC: summarize analysis results for multiple tools and samples in a single report. Bioinformatics. (2016) 32:3047–8. doi: 10.1093/bioinformatics/btw354
11. Wu F, Zhao S, Yu B, Chen YM, Wang W, Song ZG, et al. A new coronavirus associated with human respiratory disease in China. Nature. (2020) 579:265–9. doi: 10.1038/s41586-020-2008-3
12. Katoh K, Standley DM. MAFFT multiple sequence alignment software version 7: improvements in performance and usability. Mol Biol Evol. (2013) 30:772–80. doi: 10.1093/molbev/mst010
13. O'Toole Á, Scher E, Underwood A, Jackson B, Hill V, McCrone JT, et al. Assignment of epidemiological lineages in an emerging pandemic using the pangolin tool. Virus Evol. (2021) 7:veab064. doi: 10.1093/ve/veab064
14. Aksamentov I, Roemer C, Hodcroft EB, Neher RA. Nextclade: clade assignment, mutation calling and quality control for viral genomes. J Open Source Softw. (2021) 6:3773. doi: 10.21105/joss.03773
15. Hadfield J, Megill C, Bell SM, Huddleston J, Potter B, Callender C, et al. NextStrain: real-time tracking of pathogen evolution. Bioinformatics. (2018) 34:4121–3. doi: 10.1093/bioinformatics/bty407
16. Sagulenko P, Puller V, Neher RA. TreeTime: maximum-likelihood phylodynamic analysis. Virus Evol. (2018) 4:vex042. doi: 10.1093/ve/vex042
18. Fabre R, Berry A, Magnaval JF. Comparative assessment of conventional PCR with multiplex real-time PCR using SYBR Green I detection for the molecular diagnosis of imported malaria. Parasitology. (2003) 128:15–21. doi: 10.1017/S0031182003004219
19. Tan TMC, Nelson JS, Ng HC, Ting RCY, Kara UAK. Direct PCR amplification and sequence analysis of extrachromosomal Plasmodium DNA from dried blood spots. Acta Trop. (1997) 68:105–14. doi: 10.1016/S0001-706X(97)00080-6
20. Haider N, Osman AY, Gadzekpo A, Akipede GO, Asogun D, Ansumana R, et al. Lockdown measures in response to COVID-19 in nine sub-Saharan African countries. BMJ Glob Heal. (2020) 5:1–10. doi: 10.1136/bmjgh-2020-003319
21. Kusi KA, Frimpong A, Partey FD, Lamptey H, Amoah LE, Ofori MF. High infectious disease burden as a basis for the observed high frequency of asymptomatic SARS-CoV-2 infections in sub-Saharan Africa. AAS Open Res. (2021) 4:2. doi: 10.12688/aasopenres.13196.2
22. Wu X, Fu B, Chen L, Feng Y. Serological tests facilitate identification of asymptomatic SARS-CoV-2 infection in Wuhan, China. J Med Virol. (2020) 92:1795–6. doi: 10.1002/jmv.25904
23. Uyoga S, Adetifa IMO, Karanja HK, Nyagwange J, Tuju J, Wanjiku P, et al. Seroprevalence of anti-SARS-CoV-2 IgG antibodies in Kenyan blood donors. Science. (2021) 371:79–82. doi: 10.1126/science.abe1916
24. Tadesse EB, Endris AA, Solomon H, Alayu M, Kebede A, Eshetu K, et al. Seroprevalence and risk factors for SARS-CoV-2 Infection in selected urban areas in Ethiopia: a cross-sectional evaluation during July 2020. Int J Infect Dis. (2021) 111:179–85. doi: 10.1016/j.ijid.2021.08.028
25. Barrie MB, Lakoh S, Kelly JD, Kanu JS, Squire JS, Koroma Z, et al. SARS-CoV-2 antibody prevalence in Sierra Leone, March 2021: a cross-sectional, nationally representative, age-stratified serosurvey. BMJ Glob Heal. (2021) 6:7271. doi: 10.1101/2021.06.27.21259271
26. Abdella S, Riou S, Tessema M, Assefa A, Seifu A, Blachman A, et al. Prevalence of SARS-CoV-2 in urban and rural Ethiopia: Randomized household serosurveys reveal level of spread during the first wave of the pandemic. EClinicalMed. (2021) 35:100880. doi: 10.1016/j.eclinm.2021.100880
27. Wiens KE, Mawien PN, Rumunu J, Slater D, Jones FK, Moheed S, et al. Seroprevalence of severe acute respiratory syndrome coronavirus 2 IgG in Juba, South Sudan, 2020. Emerg Infect Dis. (2021) 27:1598–606. doi: 10.3201/eid2706.210568
28. Mulenga LB, Hines JZ, Fwoloshi S, Chirwa L, Siwingwa M, Yingst S, et al. Prevalence of SARS-CoV-2 in six districts in Zambia in July, 2020: a cross-sectional cluster sample survey. Lancet Glob Heal. (2021) 9:e773–81. doi: 10.1016/S2214-109X(21)00053-X
29. Kempen JH, Abashawl A, Suga HK, Difabachew MN, Kempen CJ, Debele MT, et al. SARS-CoV-2 serosurvey in Addis Ababa, Ethiopia. Am J Trop Med Hyg. (2020) 103:2022–3. doi: 10.4269/ajtmh.20-0816
30. Daniel EA, Esakialraj L BH, S A, Muthuramalingam K, Karunaianantham R, Karunakaran LP, et al. Pooled testing strategies for SARS-CoV-2 diagnosis: a comprehensive review. Diagn Microbiol Infect Dis. (2021) 101:115432. doi: 10.1016/j.diagmicrobio.2021.115432
31. de Salazar A, Aguilera A, Trastoy R, Fuentes A, Alados JC, Causse M, et al. Sample pooling for SARS-CoV-2 RT-PCR screening. Clin Microbiol Infect. (2020) 26:1687.e1–e5. doi: 10.1016/j.cmi.2020.09.008
32. Alm E, Broberg EK, Connor T, Hodcroft EB, Komissarov AB, Maurer-Stroh S, et al. Geographical and temporal distribution of SARS-CoV-2 clades in the WHO European Region, January to June 2020. Eurosurveillance. (2020) 25:2001410. doi: 10.2807/1560-7917.ES.2020.25.32.2001410
33. Ngoi JM, Quashie PK., Morang'a CM, Bonney JHK, Amuzu DSY, Kumordjie S, et al. Genomic analysis of SARS-CoV-2 reveals local viral evolution in Ghana. Exp Biol Med. (2021) 246:960–70. doi: 10.1177/1535370220975351
34. Korber B, Fischer WM, Gnanakaran S, Yoon H, Theiler J, Abfalterer W, et al. Tracking changes in SARS-CoV-2 spike: evidence that D614G increases infectivity of the COVID-19 virus. Cell. (2020) 182:812–827.e19. doi: 10.1016/j.cell.2020.06.043
35. Sallam M, Ababneh NA, Dababseh D, Bakri FG, Mahafzah A. Temporal increase in D614G mutation of SARS-CoV-2 in the Middle East and North Africa. Heliyon. (2021) 7:e06035. doi: 10.1016/j.heliyon.2021.e06035
36. Aydin-Schmidt B, Xu W, Gonzá Lez IJ, Polley SD, Bell D, Shakely DR, et al. Loop mediated isothermal amplification (LAMP) accurately detects malaria DNA from filter paper blood samples of low density parasitaemias. PLoS ONE. 9:e103905. doi: 10.1371/journal.pone.0103905
37. Bakamutumaho B, Cummings MJ, Owor N, Kayiwa J, Namulondo J, Byaruhanga T, et al. Severe COVID-19 in uganda across two epidemic phases: a prospective cohort study. Am J Trop Med Hyg. (2021) 105:740–4. doi: 10.4269/ajtmh.21-0551
38. Morton B, Barnes KG, Anscombe C, Jere K, Matambo P, Mandolo J, et al. Distinct clinical and immunological profiles of patients with evidence of SARS-CoV-2 infection in sub-Saharan Africa. Nat Commun. (2021) 12:1–12.
39. Matangila JR, Nyembu RK, Telo GM, Ngoy CD, Sakobo TM, Massolo JM, et al. Clinical characteristics of COVID-19 patients hospitalized at Clinique Ngaliema, a public hospital in Kinshasa, in the Democratic Republic of Congo: A retrospective cohort study. PLoS ONE. (2020) 15:1–15. doi: 10.1371/journal.pone.0244272
40. Amoo OS, Aina OO, Okwuraiwe AP, Onwuamah CK, Shaibu JO, Ige F, et al. COVID-19 spread patterns is unrelated to malaria co-infections in Lagos, Nigeria. Adv Infect Dis. (2020) 10:200–15. doi: 10.4236/aid.2020.105018
41. Mahajan NN, Gajbhiye RK, Bahirat S, Lokhande PD, Mathe A, Rathi S, et al. Co-infection of malaria and early clearance of SARS-CoV-2 in healthcare workers. J Med Virol. (2021) 93:2431–8. doi: 10.1002/jmv.26760
42. Wilairatana P, Masangkay FR, Kotepui KU, Milanez GDJ, Kotepui M. Prevalence and characteristics of malaria among covid-19 individuals: a systematic review, meta-analysis, and analysis of case reports. PLoS Negl Trop Dis. (2021) 15:1–18. doi: 10.1371/journal.pntd.0009766
43. Achan J, Serwanga A, Wanzira H, Kyagulanyi T, Nuwa A, Magumba G, et al. Current malaria infection, previous malaria exposure, and clinical profiles and outcomes of COVID-19 in a setting of high malaria transmission: an exploratory cohort study in Uganda. Lancet Microbe. (2022) 3:e62–71. doi: 10.1016/S2666-5247(21)00240-8
44. Osei SA, Biney RP, Anning AS, Nortey LN, Ghartey-Kwansah G. Low incidence of COVID-19 case severity and mortality in Africa; Could malaria co-infection provide the missing link? BMC Infect Dis. (2022) 22:1–11. doi: 10.1186/s12879-022-07064-4
45. Iesa MAM, Osman MEM, Hassan MA, Dirar AIA, Abuzeid N, Mancuso JJ, et al. SARS-CoV-2 and Plasmodium falciparum common immunodominant regions may explain low COVID-19 incidence in the malaria-endemic belt. New Microbes New Infect. (2020) 38:100817. doi: 10.1016/j.nmni.2020.100817
46. Raham TF. Influence of malaria endemicity and tuberculosis prevalence on COVID-19 mortality. Public Health. (2021) 194:33–5. doi: 10.1016/j.puhe.2021.02.018
47. Edwards CL, Zhang V, Werder RB, Best SE, Sebina I, James KR, et al. Coinfection with blood-stage plasmodium promotes systemic type i interferon production during pneumovirus infection but impairs inflammation and viral control in the lung. Clin Vaccine Immunol. (2015) 22:477–83. doi: 10.1128/CVI.00051-15
48. Thompson MG, Breiman RF, Hamel MJ, Desai M, Emukule G, Khagayi S, et al. Influenza and malaria coinfection among young children in western Kenya, 2009–2011. J Infect Dis. (2012) 206:1674–84. doi: 10.1093/infdis/jis591
49. Garcia-Vidal C, Sanjuan G, Moreno-García E, Puerta-Alcalde P, Garcia-Pouton N, Chumbita M, et al. Incidence of co-infections and superinfections in hospitalized patients with COVID-19: a retrospective cohort study. Clin Microbiol Infect. (2021) 27:83–8. doi: 10.1016/j.cmi.2020.07.041
50. Musuuza JS, Watson L, Parmasad V, Putman-Buehler N, Christensen L, Safdar N. Prevalence and outcomes of co-infection and superinfection with SARS-CoV-2 and other pathogens: a systematic review and metaanalysis. PLoS ONE. (2021) 16:e0251170. doi: 10.1371/journal.pone.0251170
51. Feldman C, Anderson R. The role of co-infections and secondary infections in patients with COVID-19. Pneumonia. (2021) 13:1–15. doi: 10.1186/s41479-021-00083-w
Keywords: Western Africa, seroprevalence, COVID-19, Plasmodium falciparum, co-occurrence, genomic surveillance
Citation: López-Farfán D, Yerbanga RS, Parres-Mercader M, Torres-Puente M, Gómez-Navarro I, Sanou DMS, Yao AF, Bosco Ouédraogo J, Comas I, Irigoyen N and Gómez-Díaz E (2022) Prevalence of SARS-CoV-2 and co-infection with malaria during the first wave of the pandemic (the Burkina Faso case). Front. Public Health 10:1048404. doi: 10.3389/fpubh.2022.1048404
Received: 19 September 2022; Accepted: 24 November 2022;
Published: 12 December 2022.
Edited by:
Stephanie Yanow, University of Alberta, CanadaReviewed by:
Alicia Arnott, New South Wales Health Pathology, AustraliaAmanda Maestre, Universidad de Antioquia, Colombia
Copyright © 2022 López-Farfán, Yerbanga, Parres-Mercader, Torres-Puente, Gómez-Navarro, Sanou, Yao, Bosco Ouédraogo, Comas, Irigoyen and Gómez-Díaz. This is an open-access article distributed under the terms of the Creative Commons Attribution License (CC BY). The use, distribution or reproduction in other forums is permitted, provided the original author(s) and the copyright owner(s) are credited and that the original publication in this journal is cited, in accordance with accepted academic practice. No use, distribution or reproduction is permitted which does not comply with these terms.
*Correspondence: Elena Gómez-Díaz, elena.gomez@csic.es