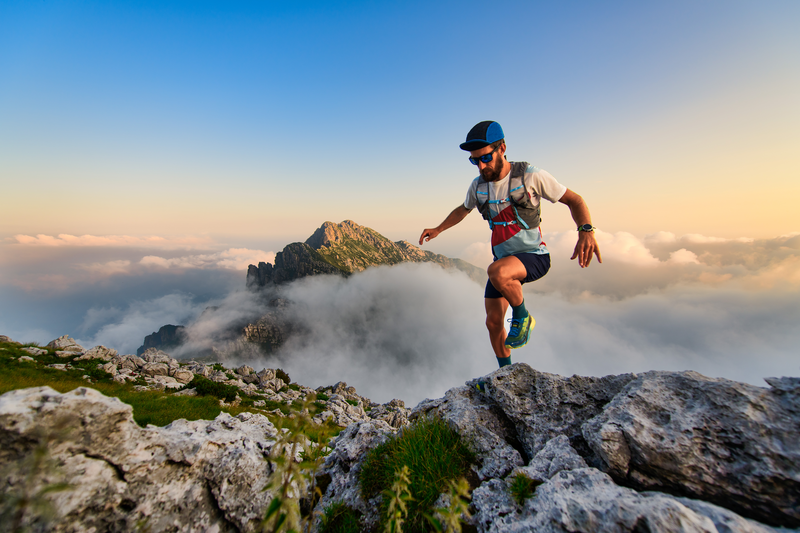
94% of researchers rate our articles as excellent or good
Learn more about the work of our research integrity team to safeguard the quality of each article we publish.
Find out more
ORIGINAL RESEARCH article
Front. Public Health , 26 October 2022
Sec. Public Health and Nutrition
Volume 10 - 2022 | https://doi.org/10.3389/fpubh.2022.1022928
Background: Clinical research results on the relationship between folate and non-alcoholic fatty liver disease are contradictory. Metabolic dysfunction-associated fatty liver disease (MAFLD) is a recently proposed concept. Evidence about the relationship between serum folate and MAFLD, especially considering the status of serum vitamin C, is scarce. This study was aimed to investigate the association of serum folate levels with the prevalence of MAFLD, and further to analyze the potential impact of serum vitamin C status on their association.
Methods: Totally 2,797 participants from National Health and Nutrition Examination Survey (NHANES) 2017–2018 were included. Vibration-controlled transient elastography was used to detect liver steatosis and fibrosis. Participants were divided in groups based on the tertiles of serum folate or vitamin C, and the serum folate or vitamin C level in T1 was low. Logistic regression analysis in the complex sample module was performed to illustrate the association of serum folate levels with the prevalence of MAFLD. Stratification analysis by serum vitamin C status was performed as well.
Results: Compared with the serum folate levels of T1 group, participants in the T3 group had 47.9% lower risk of MAFLD [OR = 0.521 (95% CI: 0.401–0.677)]. However, when participants were stratified by serum vitamin C levels, there was no association between the serum folate levels and MAFLD in the T1 or T2 group. Among participants in the T3 group of vitamin C status, participants in the T3 group of serum folate had a 63.6% lower risk of MAFLD compared with the T1 group [OR = 0.364 (95% CI: 0.147–0.903)].
Conclusions: High serum folate level is associated with lower prevalence of MAFLD, especially in participants with sufficient vitamin C.
Non-alcoholic fatty liver disease (NAFLD) is a very common chronic liver disease with a global prevalence about 25% (1), and affects over 30% of people in the United States (2). In addition to the gradual progression into fibrosis, cirrhosis and hepatocellular carcinoma, NAFLD is also associated with major adverse cardiovascular events and increased all-cause mortality (3–5). Based on the new understanding of fatty liver disease, some international experts recently proposed to replace the term NALFD with metabolic dysfunction-associated fatty liver disease (MAFLD) (6, 7). MAFLD is an inclusive disease that emphasizes the relationship between fatty liver and metabolic status. The definition of MAFLD helps better identify and manage patients at risk of cardiovascular complications, and is more clinically valuable than the definition of NAFLD (8, 9). Therefore, it is crucial to identify the possible risk factors of MAFLD.
Folate is a water-soluble B-complex family of vitamins. The word “folate” includes polyglutamates that are naturally found in foods and folic acids, and is a synthetic form taken as a supplement (10, 11). Folate plays an important role in hepatic DNA methylation, oxidative stress and fat metabolism, and has a major impact on the pathogenesis of NAFLD (12–14). However, the clinical research results on the relationship between folate and NAFLD are contradictory. A small investigation concludes that severe NAFLD is associated with lower serum folate concentration in obese individuals (15). Nevertheless, a study with 30 NAFLD patients and 24 healthy controls reveals no significant inter-group difference in folate levels and no association between folate levels and the severity of liver disease (16). However, the numbers of people observed in these epidemiological studies are relatively small. Furthermore, MAFLD is a recently proposed concept, and its relationship with serum folate has not been reported. Thus, it is essential to clarify the association between serum folate and MAFLD.
Vitamin C, a powerful antioxidant, can scavenge the free radicals produced by the body, and may be related to the pathogenesis of MAFLD (17, 18). The association between serum Vitamin C levels and the prevalence of MAFLD in populations was also demonstrated recently (19). Therefore, it can be reasonably hypothesized that vitamin C status may protect against MAFLD from low serum folate. The use of antioxidant combination therapy may have a positive effect on the improvement of MAFLD and liver fibrosis (20). Furthermore, more than 50% of elders in US took a multivitamin supplement containing both folate and vitamin C, aiming to improve or maintain health (21). Hence, it is necessary to investigate the impact of vitamin C status on the association between folate levels and MAFLD.
Therefore, this study was aimed to examine the association of serum folate levels with the prevalence of MAFLD, and further analyze the effect of serum vitamin C status on the association between serum folate and MAFLD. We present the following article in accordance with the STROBE reporting checklist.
The National Health and Nutrition Examination Survey (NHANES) 2017–2018 was adopted, which is a national survey conducted by the National Center for Health Statistics (NCHS) in the United States. To represent the civilian and non-institutionalized U.S. population, NHANES used a stratified, multistage, and clustered probability sampling design. Oversampling was also conducted in certain population subgroups to improve the reliability and accuracy of indicator estimates for these subgroups. This survey met the requirements of the NCHS institutional review board, and all participants signed written informed consent. Our analysis population consisted of 5,856 participants aged ≥18 years from the 2017 to 2018 survey cycle. Participants without Mobile Examination Center (MEC) exam were excluded (n = 323). Then 787 participants were excluded because vibration-controlled transient elastography (VCTE) was not performed or their VCTE data was incomplete. We also excluded one person who missed the median controlled attenuation parameter (CAP) scores. Finally, we excluded participants with missing data in serum folate level (n = 1,911) or serum vitamin C (n = 37), resulting in 2,797 participants in the final analysis (Figure 1).
The staff collected whole blood samples and stored them under appropriate conditions. The specimens were shipped to the laboratory and analyzed by trained phlebotomists according to standard protocols. The NHANES quality assurance and quality control (QA/QC) protocols were mandated by the 1,988 Clinical Laboratory Improvement Act.
Serum folate levels were measured using isotope-dilution high performance liquid chromatography coupled to tandem mass spectrometry (LC-MS/MS). Detection was performed by combining each specimen (150 μL of serum) with an ammonium formate buffer and an internal standard mixture. Quantitation was based on peak area ratios interpolated against a five-point aqueous linear calibration curve using 1/x2 weighting.
Isocratic ultra-high performance liquid chromatography (UPLC) was used to determine vitamin C levels. The peak area of the unknown amount in serum specimens was compared with that of the known amount in the calibration solution to determine the quantitative data of vitamin C.
In household interviews, information such as age, gender, and daily alcohol consumption was collected. Races were classified as Mexican American, Other Hispanic, Non-Hispanic White, Non-Hispanic Black, Non-Hispanic Asian, and others. Education levels were divided into less than high school, high school, and above high school. Smoking status was divided into current, ever, and never. During physical examinations in a mobile examination center, waist circumference and body mass index (weight in kilograms divided by height in meters squared) were collected. Diabetes was defined as a fasting blood glucose level ≥ 7.0 mmol/L, HbA1c ≥6.5%, or a prior diagnosis of diabetes. Hypertension was determined as systolic blood pressure ≥ 140 mmHg, diastolic blood pressure ≥ 90 mmHg, or self-reported hypertension diagnosis. The laboratory methods for measuring total cholesterol (TC) (mg/dL), triglyceride (TG) (mg/dL), high-density lipoproteins cholesterol (HDL-C), and low-density lipoproteins cholesterol (LDL-C) are available in NHANES.
Compared to the NHANES from other years, participants in 2017–2018 underwent VCTE examination. VCTE was performed using the FibroScan model 502 V2 Touch (Echosens, Paris, France) equipped with medium (M) or extra-large (XL) probes. Medium probes were used first unless the manufacturer instructions recommended to use XL probes. The detailed procedure manual was described in the Liver Ultrasound Transient Elastography Procedures Manual. Liver steatosis and fibrosis were determined by using the controlled attenuation parameter (CAP) and liver stiffness measurements (LSM) from the VCTE assessment, respectively.
MAFLD was defined on the basis of hepatic steatosis evidence in addition to one of the following three criteria: overweight/obesity, presence of type 2 diabetes mellitus, or evidence of metabolic dysregulation (6). Metabolic dysregulation was defined as the presence of at least two risky metabolic abnormalities: (1) waist circumference ≥ 102 cm in men and ≥ 88 cm women, (2) blood pressure ≥ 130/85 mmHg or specific drug treatment, (3) plasma triglycerides ≥ 1.70 mmol/l or specific drug treatment, (4) plasma HDL-cholesterol < 1.0 mmol/L for men and < 1.3 mmol/L for women or specific drug treatment, (5) prediabetes (i.e., fasting glucose level 5.6 to 6.9 mmol/L, or 2-h post-load glucose level 7.8 to 11.0 mmol or HbA1c 5.7% to 6.4%), (6) homeostasis model assessment (HOMA)-insulin resistance score ≥ 2.5, and (7) plasma high-sensitivity C-reactive protein (hs-CRP) level > 2 mg/L. Liver steatosis was defined as the CAP score ≥ 248 dB/m or ≥ 274 dB/m (22, 23).
IBM SPSS statistical 25 and R 4.1.0 were used for all analyses. According to the NHANES recommendations, appropriate weighting was used for all data analysis. Continuous variables were expressed as mean ± standard error, and categorical variables as frequency (percentage). Differences between continuous and categorical variables were assessed using weighted Student's t-test and weighted chi-squared test. A two-tailed P < 0.05 was considered significant. Serum folate level was divided into tertiles (24), of which tertile 1 (T1) was used as the reference. Logistic regression analysis in the complex sample module was performed to determine the associations of serum folate levels with the prevalence of MAFLD/NAFLD and liver fibrosis. Logistic regression was also utilized to assess the associations between folate and MAFLD/NAFLD and liver fibrosis stratified by serum vitamin C status. Serum vitamin C levels were also categorized by tertiles (25). The vitamin C levels in T1, T2, and T3 groups were ≤ 39.5 μmol/L, 39.5–61.9 μmol/L, and >61.9 μmol/L, respectively. Model 1 was adjusted for age, sex and race. Model 2 was further adjusted for education, smoking status, and daily alcohol consumption. Stratified analysis was conducted by age and gender to further analyze the association between MAFLD and serum folate, vitamin C. In addition, the associations between serum folate and NAFLD were analyzed. NAFLD was defined as hepatic steatosis excluding viral hepatitis, significant alcohol consumption or the use of steatogenic medications (2). Significant alcohol consumption was defined as >30 g/day for men and >20 g/day for women (26). Liver steatosis was also defined as the CAP scores ≥ 248 dB/m or ≥ 274 dB/m (22, 23). The study population of NAFLD was shown in Supplementary Figure 1. Liver fibrosis was defined as the LSM score ≥ 7 KPa or ≥ 8 KPa (27, 28).
Baseline characteristics were compared based on MAFLD (Table 1). A total of 2,797 participants were involved, including 1,318 non-MAFLD participants and 1479 MAFLD participants. Compared with the non-MAFLD group, the MAFLD group tended to be men, older and smokers, and had lower vitamin C serum levels and folate levels.
Table 2 showed the associations of serum folate with MAFLD. When liver steatosis was defined as CAP score ≥248 dB/m, univariate regression analysis showed that in terms of serum folate, the T3 group had a 27.4% lower risk of MAFLD compared with the T1 group [OR = 0.726 (95% CI: 0.576–0.915)]. After adjusting for sex, age and race, the prevalence of MAFLD in the T3 group decreased by 49.2% compared with the T1 group [OR = 0.508 (95% CI: 0.394, 0.654)]. When we further adjusted for education, smoking status, and daily alcohol consumption, the T3 group of serum folate had a 47.9% lower risk of MAFLD compared with the T1 group [OR = 0.521 (95% CI: 0.401–0.677)]. When liver steatosis was defined as CAP score ≥274 dB/m, the negative association of serum folate with MAFLD was marginally significant in the fully adjusted model [OR = 0.627 (95% CI: 0.366–1.074)]. In gender-stratified analyses, MAFLD was not associated with serum folate in men. However, folate concentrations were inversely associated with MAFLD in women (Supplementary Table 1). In age-stratified analyses, serum folate was inversely associated with MAFLD in participants < 40 years old, but not in participants ≥ 40 years old (Supplementary Table 2).
The association of serum folate levels with NAFLD was defined as different cutoff values for steatosis (Supplementary Table 3). When liver steatosis was defined as CAP score ≥248 dB/m, T3 group of serum folate had a 38.4% lower risk of NAFLD compared with the T1 group after adjusting for age, sex and race, education, smoking status, and daily alcohol consumption [OR = 0.616 (95% CI: 0.451–0.842)].
Table 3 showed the effect of vitamin C status on the association between serum folate and MAFLD. In the T1 and T2 groups of vitamin C status, there was no significant association between serum folate and MAFLD in the fully adjusted model, no matter liver steatosis was defined as CAP scores ≥ 248 dB/m or ≥ 274 dB/m. However, in the T3 group of vitamin C status, the T3 group of serum folate had a 63.6% lower risk of MAFLD compared with the T1 group after adjusting for age, sex and race, education, smoking status, and daily alcohol consumption [OR = 0.364 (95% CI: 0.147–.903)], when liver steatosis was defined as CAP score ≥248 dB/m. The result is similar to the above finding when liver steatosis was defined as CAP score ≥ 274 dB/m [OR = 0.209 (95% CI: 0.089–0.491)]. In women, among subjects of T3 group vitamin C status, the T3 group of serum folate was not associated with MAFLD in the fully adjusted model in women when liver steatosis was defined as with CAP scores ≥ 248 dB/m [OR = 0.271 (95% CI: 0.055–1.330)] (Supplementary Table 4). In participants < 40 years old, among subjects of T3 group vitamin C status, the T3 group of serum folate was associated with MAFLD in the fully adjusted model when liver steatosis was defined as with CAP scores ≥ 248 dB/m [OR = 0.283 (95% CI: 0.096–0.837)] (Supplementary Table 5).
The association between serum folate and NAFLD stratified by vitamin C status was presented in Supplementary Table 6. When liver steatosis was defined as CAP score ≥ 248 dB/m, among subjects of T3 group of vitamin C status, the T3 group of serum folate had a 57.8% lower risk of NAFLD compared with the T1 group in the fully adjusted model [OR = 0.422 (95% CI: 0.223–0.797)]. The association remained unchanged when CAP score ≥274 dB/m was defined as liver steatosis [OR = 0.328 (95% CI: 0.142–0.755)].
The associations between serum vitamin C levels and the prevalence of MAFLD were also investigated (Supplementary Table 7). When liver steatosis was defined as CAP score ≥ 248 dB/m, compared with the serum vitamin C level of T1 group, the prevalence of MAFLD decreased by 50.5% [OR = 0.495 (95% CI: 0.289–0.848)] in the T3 group after adjusting for age, sex and race, education, smoking status, and daily alcohol consumption. The association was marginally significant when liver steatosis was defined as CAP score ≥ 274 dB/m [OR = 0.514 (95% CI: 0.242–1.095)]. In gender-stratified analyses, serum vitamin C was inversely associated with MAFLD in women, but not in men (Supplementary Table 8). In age-stratified analyses, serum vitamin C was inversely associated with MAFLD in participants < 40 years old, but not in participants ≥ 40 years old (Supplementary Table 9).
There was no association between serum folate and the prevalence of liver fibrosis (Supplementary Table 10). When stratified by serum vitamin C levels, we also did not observe a relationship between serum folate levels and liver fibrosis (Supplementary Table 11). And there was the association between vitamin C and liver fibrosis in T2 group, but not in T3 group (Supplementary Table 12).
As far as we know, it is the first study to investigate the impact of vitamin C status on the association between serum folate and the prevalence of MAFLD, which illustrated that the serum folate level was inversely associated with the risk of developing MAFLD in adults after adjusting for potential confounders. More importantly, when stratified by serum vitamin C levels, the inverse correlation between the serum folate level and MAFLD was only maintained at high serum vitamin C level, but not at low serum vitamin C levels. Based on the interesting phenomenon, we speculate that the protective effect of increased serum folate on MAFLD may be largely dependent on serum vitamin C levels.
To our knowledge, no epidemiologic study with a large number of samples has investigated the association between the serum folate level and MAFLD, and the results of previous studies are contradictory. A single-center cross-sectional study with 30 NAFLD patients and 24 healthy controls matched for sex, age, BMI, and waist circumference showed serum folate levels were not significantly different between the two groups (16). However, serum folate levels were lower in obese patients with severe NAFLD compared with subjects with normal liver or mild liver alterations (15). A study of Chinese adults (70 subjects undergoing liver biopsy, and 130 subjects undergoing proton magnetic resonance spectroscopy (1H-MRS) for liver fat measurement) demonstrated that serum folic acid levels were inversely correlated with the degree of hepatic steatosis (29). In addition, the addition of serum folic acid to the NAFLD prediction score significantly improved diagnostic performance (29). Similar to these two studies, we concluded that serum folate levels were inversely associated with the prevalence of MAFLD in populations with a relatively large number of samples. Animal studies also support these findings. As reported, chronic folate deficiency led to liver steatosis in mice (30). Moreover, folic acid supplementation reduced liver lipid accumulation and liver inflammation induced by a high-fat diet in mice (31). An 8-week short-term animal study showed that folic acid supplementation significantly reduced steatosis and improved liver function in the rats fed with a high-fructose diet (32).
Reportedly, the occurrence and development of NAFLD are related to epigenetic mechanisms, one of which is DNA methylation (33). Hypermethylation and hypomethylation are associated with gene silencing and gene activation, respectively (34, 35). Folate is considered to be a methyl donor for DNA methylation, and can affect the expression of cellular genes, resulting in a series of effects. The underlying mechanism may be that folate plays an important role in the synthesis of purine and thymidylate and in DNA methylation (36), and folate deficiency can lead to high expression of genes related to hepatic fat synthesis, resulting in hepatic steatosis (37). In addition, folate deficiency reduces the level of phosphatidy-lethanolamine N-methyltransferase, thereby reducing the methylation of phosphatidylethanolamine to phosphatidylcholine, which ultimately affects low-density lipoprotein secretion and causes liver fat accumulation (38, 39). Folic acid supplementation can regulate the transcription of NADPH oxidase, effectively reduce the oxidative stress caused by high-fat diet (40), increase AMP levels, and activate LKB1 to restore AMPK activation in the liver, thereby improving cholesterol and glucose metabolism, which may slow down the pathogenesis of NAFLD (41).
As an antioxidant, vitamin C reduces oxidative stress and prevents chronic inflammatory diseases (42). The relationship between vitamin C and liver steatosis has been investigated. An observational study with 72 NAFLD patients indicated that the prevalence of serum vitamin C deficiency and insufficient vitamin C intake was very high (43). Multifactorial regression analysis demonstrated vitamin C intake was negatively associated with the prevalence of NAFLD in 797 subjects ≥18 years old (44). In another cross-sectional study of 4,494 subjects, the increased serum vitamin C concentrations were negatively association with MAFLD among adults in the United States (19), which is consistent to our results. More interestingly, we found that the inverse correlation between the serum folate concentration and MAFLD was maintained only in subjects with higher serum vitamin C status, but not in subjects with low serum vitamin C levels. Therefore, sufficient serum vitamin C may be an important prerequisite for the protective effect of serum folate on MAFLD. It implies that the simultaneous supplementation of VC and folic acid in multivitamin tablets may be reasonable. Our results may provide new ideas for clinical nutritional treatment of MAFLD.
In gender-stratified analyses, although serum folate and vitamin C were inversely associated with MAFLD in women, the inverse correlation between the serum folate level and MAFLD was not maintained at high serum vitamin C level when stratified by serum vitamin C levels. We speculate that this may be caused by the small number of participants after stratification. In age-stratified analyses, the inverse correlation between the serum folate level and MAFLD was maintained at high serum vitamin C level when stratified by serum vitamin C levels.
There are several studies on the association of folate and liver fibrosis. A study showed that folate supplementation can increase the level of fusion protein Syntaxin 17 in the liver, which is important in reversing the development of liver inflammation and fibrosis (45). However, folate levels did not differ significantly in fibrosis stages in a study of 30 patients with biopsy-proven NAFLD and 24 healthy controls (16). The results of this study are consistent with our result, but we need further research.
The association between vitamin C and liver fibrosis has also been investigated. Forty-five NASH patients participated in the 6-month trial, with 23 receiving vitamin E (1000IU) and vitamin C (1,000 mg), and 22 receiving placebo. The fibrosis scores significantly improved in the vitamin group (46). However, a previous cross-sectional study of 789 people confirmed that dietary vitamin C intake was not associated with liver fibrosis (47). When stratified by serum vitamin C levels, we did not observe the association between serum folate levels and liver fibrosis. It is suggested that supplementing vitamin C and folate at the same time has no effect on liver fibrosis.
There are several strengths of this research. Firstly, it is the first study to investigate the impact of serum vitamin C status on the association of serum folate levels with the prevalence of MAFLD. Secondly, the recently proposed definition MAFLD was adopted, which further assures the novelty of our research. Thirdly, NHANES is representative of the civilian and non-institutionalized national population, which makes our results more representative and persuasive. However, there are some limitations. First, it is a cross-sectional study, so no causal relationship between serum folate levels or serum vitamin C levels and MAFLD can be established. Second, although we tried our best to adjust for the potential confounders, not all of the confounding factors can be included. Third, data such as drug therapy, lifestyle and dietary habit were not available, which may have impacted our findings.
In conclusion, serum folate level is inversely associated with the prevalence of MAFLD, especially in participants with sufficient serum vitamin C. It may be necessary to maintain sufficient serum vitamin C for ensuring the protective effect of increased folate on MALFD. However, more studies are needed to validate this.
The datasets presented in this study can be found in online repositories (https://www.cdc.gov/nchs/nhanes/index.htm).
The studies involving human participants were reviewed and approved by National Center for Health Statistics. The patients/participants provided their written informed consent to participate in this study. Written informed consent was obtained from the individual(s), and minor(s)' legal guardian/next of kin, for the publication of any potentially identifiable images or data included in this article.
YJ, HC, XC, GY, CS, HD, and FT participated in the data collection and analysis. XC and GY validated the data. YJ and HC drafted the manuscript. HW and JS revised the manuscript and served as scientific advisors. All authors contributed to the study conception and design.
This work was supported by the National Natural Science Foundation of China (82170800), Guangdong Basic and Applied Basic Research Foundation (2021A1515110682), and Research Initiation Project of Shunde Hospital of Southern Medical University (SRSP2021001).
We thank the subjects and staff for their contributions to this study.
The authors declare that the research was conducted in the absence of any commercial or financial relationships that could be construed as a potential conflict of interest.
All claims expressed in this article are solely those of the authors and do not necessarily represent those of their affiliated organizations, or those of the publisher, the editors and the reviewers. Any product that may be evaluated in this article, or claim that may be made by its manufacturer, is not guaranteed or endorsed by the publisher.
The Supplementary Material for this article can be found online at: https://www.frontiersin.org/articles/10.3389/fpubh.2022.1022928/full#supplementary-material
MAFLD, Metabolic dysfunction-associated fatty liver disease; NHANES, National Health and Nutrition Examination Survey; NAFLD, Non-alcoholic fatty liver disease; NCHS, National Center for Health Statistics; MEC, Mobile Examination Center; VCTE, vibration-controlled transient elastography; CAP, controlled attenuation parameter; LSM, liver stiffness measurements; TC, total cholesterol; TG, triglyceride; HDL-C, high-density lipoproteins cholesterol; LDL-C, low-density lipoproteins cholesterol; BMI, body mass index.
1. Younossi ZM, Koenig AB, Abdelatif D, Fazel Y, Henry L, Wymer M. Global epidemiology of nonalcoholic fatty liver disease-Meta-analytic assessment of prevalence, incidence, and outcomes. Hepatology. (2016) 64:73–84. doi: 10.1002/hep.28431
2. Ciardullo S, Perseghin G. Prevalence of NAFLD, MAFLD and associated advanced fibrosis in the contemporary United States population. Liver Int. (2021) 41:1290–3. doi: 10.1111/liv.14828
3. Kim D, Konyn P, Sandhu KK, Dennis BB, Cheung AC, Ahmed A. Metabolic dysfunction-associated fatty liver disease is associated with increased all-cause mortality in the United States. J Hepatol. (2021) 75:1284–91. doi: 10.1016/j.jhep.2021.07.035
4. Lindenmeyer CC, McCullough AJ. The natural history of nonalcoholic fatty liver disease-an evolving view. Clin Liver Dis. (2018) 22:11–21. doi: 10.1016/j.cld.2017.08.003
5. Simon TG, Roelstraete B, Hagström H, Sundström J, Ludvigsson JF. Non-alcoholic fatty liver disease and incident major adverse cardiovascular events: results from a nationwide histology cohort. Gut. (2021) 2021:1–19. doi: 10.1136/gutjnl-2021-325724
6. Eslam M, Newsome PN, Sarin SK, Anstee QM, Targher G, Romero-Gomez M, et al. A new definition for metabolic dysfunction-associated fatty liver disease: An international expert consensus statement. J Hepatol. (2020) 73:202–9. doi: 10.1016/j.jhep.2020.03.039
7. Eslam M, Sanyal AJ, George J. MAFLD: A consensus-driven proposed nomenclature for metabolic associated fatty liver disease. Gastroenterology. (2020) 158:1999–2014.e1. doi: 10.1053/j.gastro.2019.11.312
8. George J, Gish RG, Geier A. MAFLD and cardiovascular events: What does the evidence show? Clin Gastroenterol Hepatol. (2021) 19:2025–8. doi: 10.1016/j.cgh.2021.02.027
9. Lee H, Lee YH, Kim SU, Kim HC. Metabolic dysfunction-associated fatty liver disease and incident cardiovascular disease risk: a nationwide cohort study. Clin Gastroenterol Hepatol. (2021) 19:2138–47.e10. doi: 10.1016/j.cgh.2020.12.022
10. Iyer R, Tomar SK. Folate: a functional food constituent. J Food Sci. (2009) 74:R114–22. doi: 10.1111/j.1750-3841.2009.01359.x
11. Wright AJ, Dainty JR, Finglas PM. Folic acid metabolism in human subjects revisited: potential implications for proposed mandatory folic acid fortification in the UK. Br J Nutr. (2007) 98:667–75. doi: 10.1017/S0007114507777140
12. Cordero P, Gomez-Uriz AM, Campion J, Milagro FI, Martinez JA. Dietary supplementation with methyl donors reduces fatty liver and modifies the fatty acid synthase DNA methylation profile in rats fed an obesogenic diet. Genes Nutr. (2013) 8:105–13. doi: 10.1007/s12263-012-0300-z
13. da Silva RP, Kelly KB, Al Rajabi A, Jacobs RL. Novel insights on interactions between folate and lipid metabolism. BioFactors. (2014) 40:277–83. doi: 10.1002/biof.1154
14. Sid V, Siow YL, O K. Role of folate in nonalcoholic fatty liver disease. Canad J Physiol Pharmacol. (2017) 95:1141–8. doi: 10.1139/cjpp-2016-0681
15. Hirsch S, Poniachick J, Avendaño M, Csendes A, Burdiles P, Smok G, et al. Serum folate and homocysteine levels in obese females with non-alcoholic fatty liver. Nutrition. (2005) 21:137–41. doi: 10.1016/j.nut.2004.03.022
16. Polyzos SA, Kountouras J, Patsiaoura K, Katsiki E, Zafeiriadou E, Zavos C, et al. Serum vitamin B12 and folate levels in patients with non-alcoholic fatty liver disease. Int J Food Sci Nutr. (2012) 63:659–66. doi: 10.3109/09637486.2011.649249
17. Padayatty SJ, Katz A, Wang Y, Eck P, Kwon O, Lee JH, et al. Vitamin C as an antioxidant: evaluation of its role in disease prevention. J Am College Nutr. (2003) 22:18–35. doi: 10.1080/07315724.2003.10719272
18. Valdecantos MP, Pérez-Matute P, Quintero P, Martínez JA. Vitamin C, resveratrol and lipoic acid actions on isolated rat liver mitochondria: all antioxidants but different. Redox Rep. (2010) 15:207–16. doi: 10.1179/135100010X12826446921464
19. Xie ZQ, Li HX, Tan WL, Yang L, Ma XW, Li WX, et al. Association of Serum Vitamin C With NAFLD and MAFLD among adults in the United States. Front Nutr. (2021) 8:795391. doi: 10.3389/fnut.2021.795391
20. Di Francia R, Rinaldi L, Troisi A, Di Benedetto F, Berretta M. Effect of anti-oxidant agents in patients with hepatocellular diseases. Euro Rev Med Pharmacol Sci. (2015) 19:3993–5. Available online at: https://www.europeanreview.org/wp/wp-content/uploads/3993-3995-Letter-to-the-Editor.pdf
21. Rautiainen S, Gaziano JM, Christen WG, Bubes V, Kotler G, Glynn RJ, et al. Effect of baseline nutritional status on long-term multivitamin use and cardiovascular disease risk: a secondary analysis of the physicians' health study II randomized clinical trial. JAMA Cardiol. (2017) 2:617–25. doi: 10.1001/jamacardio.2017.0176
22. Eddowes PJ, Sasso M, Allison M, Tsochatzis E, Anstee QM, Sheridan D, et al. Accuracy of fibroscan controlled attenuation parameter and liver stiffness measurement in assessing steatosis and fibrosis in patients with nonalcoholic fatty liver disease. Gastroenterology. (2019) 156:1717–30. doi: 10.1053/j.gastro.2019.01.042
23. Karlas T, Petroff D, Sasso M, Fan JG, Mi YQ, de Lédinghen V, et al. Individual patient data meta-analysis of controlled attenuation parameter (CAP) technology for assessing steatosis. J Hepatol. (2017) 66:1022–30. doi: 10.1016/j.jhep.2016.12.022
24. Chen S, Honda T, Hata J, Sakata S, Furuta Y, Yoshida D, et al. High serum folate concentrations are associated with decreased risk of mortality among Japanese adults. J Nutr. (2021) 151:657–65. doi: 10.1093/jn/nxaa382
25. Deicher R, Ziai F, Bieglmayer C, Schillinger M, Hörl WH. Low total vitamin C plasma level is a risk factor for cardiovascular morbidity and mortality in hemodialysis patients. J Am Soc Nephrol. (2005) 16:1811–8. doi: 10.1681/ASN.2004100850
26. Chalasani N, Younossi Z, Lavine JE, Charlton M, Cusi K, Rinella M, et al. The diagnosis and management of nonalcoholic fatty liver disease: Practice guidance from the American Association for the Study of Liver Diseases. Hepatology. (2018) 67:328–57. doi: 10.1002/hep.29367
27. Lomonaco R, Godinez Leiva E, Bril F, Shrestha S, Mansour L, Budd J, et al. Advanced liver fibrosis is common in patients with type 2 diabetes followed in the outpatient setting: the need for systematic screening. Diabetes Care. (2021) 44:399–406. doi: 10.2337/dc20-1997
28. Roulot D, Roudot-Thoraval F, G NK, Kouacou N, Costes JL, Elourimi G, et al. Concomitant screening for liver fibrosis and steatosis in French type 2 diabetic patients using Fibroscan. Liver Int. (2017) 37:1897–906. doi: 10.1111/liv.13481
29. Xia MF, Bian H, Zhu XP, Yan HM, Chang XX, Zhang LS, et al. Serum folic acid levels are associated with the presence and severity of liver steatosis in Chinese adults. Clin Nutr. (2018) 37:1752–8. doi: 10.1016/j.clnu.2017.06.021
30. Christensen KE, Wu Q, Wang X, Deng L, Caudill MA, Rozen R. Steatosis in mice is associated with gender, folate intake, and expression of genes of one-carbon metabolism. J Nutr. (2010) 140:1736–41. doi: 10.3945/jn.110.124917
31. Sid V, Shang Y, Siow YL, Hewage SM, House JD, O K. Folic acid supplementation attenuates chronic hepatic inflammation in high-fat diet fed mice. Lipids. (2018) 53:709–16. doi: 10.1002/lipd.12084
32. Kim H, Min H. Folic acid supplementation prevents high fructose-induced non-alcoholic fatty liver disease by activating the AMPK and LKB1 signaling pathways. Nutr Res Pract. (2020) 14:309–21. doi: 10.4162/nrp.2020.14.4.309
33. Lee J, Kim Y, Friso S, Choi SW. Epigenetics in non-alcoholic fatty liver disease. Mol Aspects Med. (2017) 54:78–88. doi: 10.1016/j.mam.2016.11.008
34. Rinaldi L, Pafundi PC, Galiero R, Caturano A, Morone MV, Silvestri C, et al. Mechanisms of non-alcoholic fatty liver disease in the metabolic syndrome. A Narrative Review. Antioxidants. (2021) 10:e20270. doi: 10.3390/antiox10020270
35. Sodum N, Kumar G, Bojja SL, Kumar N, Rao CM. Epigenetics in NAFLD/NASH: Targets and therapy. Pharmacol Res. (2021) 167:105484. doi: 10.1016/j.phrs.2021.105484
36. Duthie SJ, Narayanan S, Brand GM, Pirie L, Grant G. Impact of folate deficiency on DNA stability. J Nutr. (2002) 132(8 Suppl):2444s−9. doi: 10.1093/jn/132.8.2444S
37. Champier J, Claustrat F, Nazaret N, Fèvre Montange M, Claustrat B. Folate depletion changes gene expression of fatty acid metabolism, DNA synthesis, and circadian cycle in male mice. Nutr Res. (2012) 32:124–32. doi: 10.1016/j.nutres.2011.12.012
38. Chew TW, Jiang X, Yan J, Wang W, Lusa AL, Carrier BJ, et al. Folate intake, MTHFR genotype, and sex modulate choline metabolism in mice. J Nutr. (2011) 141:1475–81. doi: 10.3945/jn.111.138859
39. Vance DE, Li Z, Jacobs RL. Hepatic phosphatidylethanolamine N-methyltransferase, unexpected roles in animal biochemistry and physiology. J Biol Chem. (2007) 282:33237–41. doi: 10.1074/jbc.R700028200
40. Sarna LK, Wu N, Wang P, Hwang SY, Siow YL, O K. Folic acid supplementation attenuates high fat diet induced hepatic oxidative stress via regulation of NADPH oxidase. Canad J Physiol Pharmacol. (2012) 90:155–65. doi: 10.1139/y11-124
41. Sid V, Wu N, Sarna LK, Siow YL, House JD, O K. Folic acid supplementation during high-fat diet feeding restores AMPK activation via an AMP-LKB1-dependent mechanism. Am J Physiol Regulat. (2015) 309:R1215–25. doi: 10.1152/ajpregu.00260.2015
42. Berretta M, Quagliariello V, Maurea N, Di Francia R, Sharifi S, Facchini G, et al. Multiple effects of ascorbic acid against chronic diseases: updated evidence from preclinical and clinical studies. Antioxidants. (2020) 9:e1182. doi: 10.3390/antiox9121182
43. Coelho JM, Cansanção K, Perez RM, Leite NC, Padilha P, Ramalho A, et al. Association between serum and dietary antioxidant micronutrients and advanced liver fibrosis in non-alcoholic fatty liver disease: an observational study. PeerJ. (2020) 8:e9838. doi: 10.7717/peerj.9838
44. Chan R, Wong VW, Chu WC, Wong GL, Li LS, Leung J, et al. Diet-quality scores and prevalence of nonalcoholic fatty liver disease: a population study using proton-magnetic resonance spectroscopy. PLoS ONE. (2015) 10:e0139310. doi: 10.1371/journal.pone.0139310
45. Tripathi M, Singh BK, Zhou J, Tikno K, Widjaja A, Sandireddy R, et al. Vitamin B(12) and folate decrease inflammation and fibrosis in NASH by preventing syntaxin 17 homocysteinylation. J Hepatol. (2022). doi: 10.1016/j.jhep.2022.06.033
46. Harrison SA, Torgerson S, Hayashi P, Ward J, Schenker S. Vitamin E and vitamin C treatment improves fibrosis in patients with nonalcoholic steatohepatitis. Am J Gastroenterol. (2003) 98:2485–90s. doi: 10.1111/j.1572-0241.2003.08699.x
Keywords: folate, vitamin C, metabolic dysfunction-associated fatty liver disease, vibration-controlled transient elastography, National Health and Nutrition Examination Survey
Citation: Jiang Y, Cao H, Chen X, Yu G, Song C, Duan H, Tian F, Wan H and Shen J (2022) Associations of serum folate and vitamin C levels with metabolic dysfunction-associated fatty liver disease in US adults: A nationwide cross-sectional study. Front. Public Health 10:1022928. doi: 10.3389/fpubh.2022.1022928
Received: 19 August 2022; Accepted: 06 October 2022;
Published: 26 October 2022.
Edited by:
Ferdinando Carlo Sasso, University of Campania Luigi Vanvitelli, ItalyReviewed by:
Alfredo Caturano, University of Campania Luigi Vanvitelli, ItalyCopyright © 2022 Jiang, Cao, Chen, Yu, Song, Duan, Tian, Wan and Shen. This is an open-access article distributed under the terms of the Creative Commons Attribution License (CC BY). The use, distribution or reproduction in other forums is permitted, provided the original author(s) and the copyright owner(s) are credited and that the original publication in this journal is cited, in accordance with accepted academic practice. No use, distribution or reproduction is permitted which does not comply with these terms.
*Correspondence: Jie Shen, c2ppZXN5QHNtdS5lZHUuY24=; Heng Wan, d2FuaGRyQDE2My5jb20=
†These authors have contributed equally to this work and share first authorship
‡These authors have contributed equally to this work
Disclaimer: All claims expressed in this article are solely those of the authors and do not necessarily represent those of their affiliated organizations, or those of the publisher, the editors and the reviewers. Any product that may be evaluated in this article or claim that may be made by its manufacturer is not guaranteed or endorsed by the publisher.
Research integrity at Frontiers
Learn more about the work of our research integrity team to safeguard the quality of each article we publish.