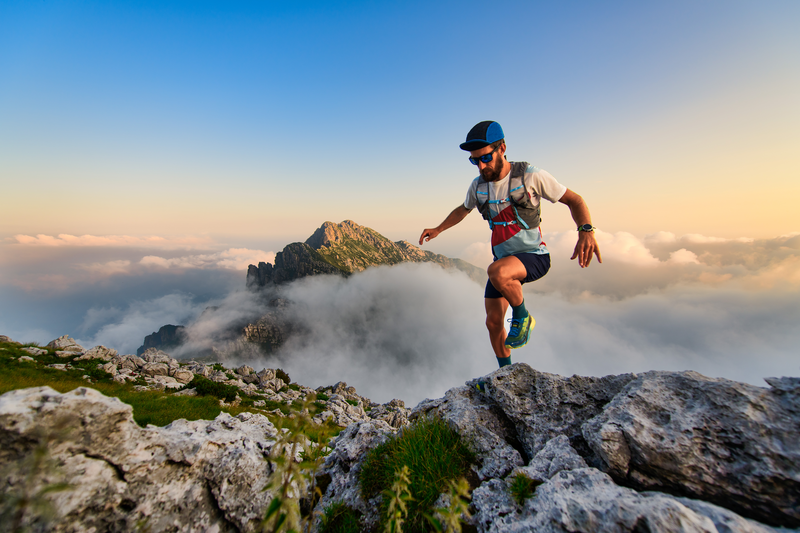
95% of researchers rate our articles as excellent or good
Learn more about the work of our research integrity team to safeguard the quality of each article we publish.
Find out more
HYPOTHESIS AND THEORY article
Front. Public Health , 24 November 2022
Sec. Public Health Policy
Volume 10 - 2022 | https://doi.org/10.3389/fpubh.2022.1021901
This article is part of the Research Topic Women in Science: Public Health Policy 2022 View all 6 articles
The prenatal environment plays a critical role in shaping fetal development and ultimately the long-term health of the child. Here, we present data linking prenatal health, via maternal nutrition, comorbidities in pregnancy (e.g., diabetes, hypertension), and infectious and inflammatory exposures, to lifelong health through the developmental origins of disease framework. It is well-established that poor maternal health puts a child at risk for adverse outcomes in the first 1,000 days of life, yet the full health impact of the in utero environment is not confined to this narrow window. The developmental origins of disease framework identifies cognitive, neuropsychiatric, metabolic and cardiovascular disorders, and chronic diseases in childhood and adulthood that have their genesis in prenatal life. This perspective highlights the enormous public health implications for millions of pregnancies where maternal care, and therefore maternal health and fetal health, is lacking. Despite near universal agreement that access to antenatal care is a priority to protect the health of women and children in the first 1,000 days of life, insufficient progress has been achieved. Instead, in some regions there has been a political shift toward deprioritizing maternal health, which will further negatively impact the health and safety of pregnant people and their children across the lifespan. In this article we argue that the lifelong health impact attributed to the perinatal environment justifies policies aimed at improving access to comprehensive antenatal care globally.
The first 1,000 days of life, from conception to 1 years of age, is a period of incredible vulnerability that can dramatically alter the life trajectory of a child (1, 2). From the time of conception, the developmental course of the embryo, and subsequently the fetus, is dependent on the health of the mother. Pre- and post-natal care policies that promote maternal health have the potential to shape a child's path to survive and thrive with intergenerational benefits. Most programs focusing on the first 1,000 days promote early childhood interventions that support children in reaching their developmental milestones (1). However, taking a view of a person's lifelong health trajectory through the lens of the developmental origins of health and disease framework identifies a broader scope of impact for maternal health interventions (Figure 1). A growing body of evidence has documented the impact of the perinatal environment on lifelong health. Diverse prenatal exposures can impact fetal growth and development in utero and alter long-term health outcomes. These include environmental exposures, medications, maternal nutrition, comorbidities in pregnancy including gestational diabetes and hypertension, and infectious and inflammatory exposures (3–6). During pregnancy, early patterning decisions followed by exquisitely regulated developmental processes are required for healthy fetal growth and development (7). While some disturbances in these carefully regulated processes may lead to fetal demise and pregnancy loss, other non-lethal perturbations may subtly alter organ formation and maturation. The consequences of these alterations may manifest later in life during periods of rapid growth and development (e.g., adolescence), or into adulthood, leading to the unmasking of health problems or premature onset of non-communicable diseases (7).
Figure 1. Developmental origins of health and disease. From the time of conception, and throughout gestation, the health of the mother and resulting changes in the in utero environment can impact the health and development of the child and last well into adulthood. Maternal health, including stress, nutritional status, metabolic disorders, infection, and inflammation during pregnancy, can impact in utero development via multiple pathways including altered placental vascular development, nephrogenesis, and microbiome. This can result in an increased risk of poor health outcomes in the child, including a potential impact on neurodevelopmental processes, or may manifest in adulthood as an increased risk of metabolic disorders, cardiovascular disorders, or acute kidney injury. Created using Biorender.com.
As evidence documents the impact of the perinatal environment on lifelong health, there is a need to translate this evidence into policy that promotes comprehensive and accessible antenatal care (ANC) and allied reproductive and pediatric healthcare. Here, we present evidence of developmental origins of disease and propose that enhanced access to ANC is a crucial pathway to improve prenatal health, healthy in utero development, and healthy outcomes across the lifespan. At present there are no globally uniform standards for the provision of ANC. In 2002, the WHO recommended focused antenatal care (FANC), which suggests a minimum of four scheduled visits for uncomplicated pregnancies (8). After an evidence-based policy review in 2015 showed increased risk for perinatal death with FANC compared to eight-visit models, the WHO updated its minimum recommended number of ANC visits from four to eight (9). Despite the WHO's updated recommendations, FANC, or modified versions, remains the most common model of ANC, based on widespread use in low- and middle-income countries (LMIC). Lack of consistency around ANC policies and recommendations, combined with shifting healthcare priorities and limited resources, leaves far too many pregnant people and children at risk. Recognizing the long-term impact of exposures in pregnancy on human health and wellbeing, there is an urgent need to prioritize policies around maternal health and pregnancy care. We propose that the developmental origins of disease framework provides even more evidence for the need to protect health at this vulnerable time.
Multiple cardiovascular, metabolic, immune, and neurological morbidities have been linked to developmental, or in utero, origins (4, 5, 10). Among the most direct consequences of compromised in utero development are adverse pregnancy outcomes such as preterm birth (PTB; birth prior to 37 completed gestational weeks of pregnancy), being born with low birth weight (LBW; birth weight <2,500 g), or small-for-gestational age (SGA; birth weight below the 10th percentile for gestational age). Each of these adverse pregnancy outcomes confers an excess risk of morbidity and mortality in the immediate period and throughout the lifespan. PTB is now the leading cause of neonatal mortality worldwide, and a leading cause of death in children under five (11). In addition to perinatal mortality, multiple long-term health outcomes are associated with PTB including neurodevelopmental disorders as well as cardiovascular and metabolic disease in adulthood (12–14). An estimated 27% of all births in LMIC are SGA as a result of intrauterine growth restriction (15). Like PTB, SGA is associated with a greatly increased risk of neonatal morbidity and mortality and can have lifelong consequences including growth delay, neurodevelopmental impairment, and cerebral palsy (16, 17). The risk of PTB, LBW, and SGA is closely tied to maternal health and multiple factors including maternal malnutrition, exposure to infectious diseases, or maternal metabolic disorders, increase the risk of poor birth outcomes. The role of identifiable birth outcomes, such as PTB and LBW, on developmental origins of disease is more easily quantifiable, as the association between adverse birth outcomes and childhood health is well-established. However, research is demonstrating childhood and lifelong health outcomes associated with maternal health, in the absence identifiable poor birth outcomes (Table 1).
Table 1. Maternal exposures during pregnancy that may impact long-term health outcomes and potential interventions.
Multiple studies have linked maternal infection with poor neurocognitive outcome and with neuropsychiatric disorders in children (3, 18). Prenatal infection or inflammatory disorders are associated with an increased risk of schizophrenia, autism spectrum disorder, and depression in exposed children. This occurs in the absence of congenital infection and has been linked to the impact of maternal immune activation on fetal neurodevelopment. For example, malaria in pregnancy, one of the most common maternal infections globally, has been associated with impaired language development in exposed offspring (19).
Maternal stress is also associated with the development of neuropsychiatric disorders later in life (20–23). Maternal anxiety and/or depression has been linked with an increased risk of childhood emotional and behavioral problems in early adulthood, independent of postpartum symptoms or socioeconomic factors (24). Children born to mothers who experienced maternal anxiety have demonstrated epigenetic age acceleration at 10 years of age (24). Imaging studies have associated prenatal maternal distress with structural changes in the infant brain, specifically hippocampal connectivity and cortical morphology (25, 26). Investigators have begun to propose early interventions to teach and facilitate stress management for at-risk mothers (27).
Development of metabolic disorders such as type 2 diabetes and cardiovascular disease have been linked to maternal nutritional status (28). Maternal undernutrition is often associated with intrauterine growth restriction, which increases the risk of type 2 diabetes and cardiovascular disease in offspring in later life. Although a large proportion of children whose mothers experience nutritional deficiencies will not show a birth phenotype, fetal hormonal adaptations and epigenetic changes are evident. Maternal obesity is also a risk factor for metabolic and cardiovascular disorders in children (29). Non-communicable diseases are rising globally with the fastest rates in LMIC, where weak health systems make it difficult to provide adequate care for people living with chronic conditions. This results in more women at risk of malnutrition in pregnancy and an increasing number of children at risk.
Hypotheses around the mechanisms underlying developmental origins of disease propose that as the fetus aims to optimize growth in response to energy and nutrient availability in utero, fetal physiology undergoes structural and functional adaptations that persist after birth. Therefore, in conditions of nutrient deficiency, the fetus adapts to prioritize glucose and lipid storage (30). However, when the post-natal environment is nutritionally abundant, these in utero adaptations become maladaptive and can contribute to the development of metabolic syndromes and cardiovascular disorders in later life. Several studies have established an association between individuals exposed to maternal nutrient deprivation in utero, and risk of LBW, obesity and cardiovascular disease in adulthood (30–32). In particular, individuals born LBW who demonstrate compensatory “catch up” growth in infancy and early childhood appear to have increased risk of adverse cardiovascular outcomes in adulthood. Maternal overweight, obesity, and increased gestational weight gain, are associated with an increased risk of large-for-gestational-age newborns and abnormal adiposity (33–35). Despite differences in birth phenotype compared to children of undernourished women, these offspring are also at increased risk of obesity, metabolic syndrome in childhood (36) and adulthood (37), and of early cardiovascular death.
The in utero environment plays a critical role in a child's post-natal health, behavior, and long-term development but remains a neglected area of research, due at least in part, to a lack of prioritization of maternal health and the inherent risks and challenges associated with conducting research in pregnancy. From conception through to delivery, each stage of in utero development can have profound implications on the healthy growth and development of the child. Full reviews of in utero development have been reported in detail (38, 39). After an initial period of embryonic development, fetal development begins at 8 weeks of gestation. The first 12 weeks of fetal development are marked by formation of all major organ systems, which are established by 12 weeks with ongoing maturation and development through to term and into childhood. In the second trimester, essential processes in lung development are initiated, as well as the formation of hearing and facial features. Throughout the third trimester numerous maturation processes occur, including formation of muscle and fat stores, until the fetus is considered to be at term by 37 completed weeks of gestation. Across embryonic and fetal development, central nervous system development proceeds by sequential periods of neurogenesis, cell migration, synapse formation, and synaptic pruning. Functional neural circuitry undergoes essential periods of development in the third trimester. Each of these developmental periods require an orchestrated and tightly regulated set of initiation and completion signals. Given the unique developmental events occurring within each trimester, the timing of adverse maternal health events such as an infection in pregnancy could have varying impacts on fetal health based on gestational age. Alternatively, conditions such as sustained maternal malnutrition, have the potential for cumulative impact on the in utero environment. Collectively, these data reveal that disruption to the tightly regulated in utero environment modified early developmental processes (6, 21). Thus the nature and timing of events that affect maternal health and modify the intrauterine environment have the potential to disrupt essential and tightly regulated processes of embryonic and fetal development.
Poor maternal health and resulting changes to the in utero environment have been associated with altered fetal organ structure, epigenetic modification of gene expression, regulation of cellular aging, and the composition of the microbiome (5) (Table 1). For example, three processes that begin in utero and contribute to life-long human health are placental vascular development, fetal nephrogenesis, and the establishment of the baby's microbiome.
Fetal development is dependent on the growth, structure, and function of the placental vasculature. By term, placental vasculature is over 500 km in length and 15 m2 in surface area (40). Several prominent pregnancy-related pathologies including gestational diabetes mellitus, intrauterine growth restriction, and pre-eclampsia have been linked to placental vasculature dysfunctions including inadequate perfusion, and villous morphological alterations and infarction (41–43). The placenta is formed through sequential processes of vasculogenesis (the formation of new vasculature) and angiogenesis (refinement of existing blood vessels). Once established, the placental vasculature undergoes processes of transformation (branching and elongation of capillaries) and maturation of vessels throughout gestation. The rate of capillary elongation increases dramatically beginning at gestational week 25 and total length increases at an exponential rate until term (44). The accelerated angiogenic processes occurring in the third trimester mirror and support the rapid fetal growth occurring at this time. Placental pathologies are closely tied to maternal health and therefore provide a potential mechanistic pathway for developmental origins of disease (10).
Similar to the ordered processes that characterize placental development, early kidney development involves sequential steps of ureteric bud outgrowth followed by ureteric branching and branching nephrogenesis. Nephrogenesis begins at 5 weeks of gestation and is completed by 36 weeks of gestation, so an individual is born with their entire complement of nephrons or “nephron endowment.” Nephron endowment in humans ranges from 200,000 to 2 million nephrons per kidney. An estimated 90% of nephrons are formed between weeks 26 and 36 of gestation where initial ureteric branching and nephrons undergo massive expansion as chains of nephrons with multiple inter-connecting nephrons. Interruptions to nephrogenesis during this critical period of fetal development have a substantial impact on nephron endowment. The number of nephrons at birth impact the normal lifespan of the kidney and people with reduced nephron endowment are at increased risk of developing hypertension and chronic kidney disease.
In the pre-natal and post-natal periods, numerous studies are providing evidence that the microbiome plays a critical role in development and future risk of metabolic, immunologic/inflammatory, and neurodevelopmental conditions. Most of the research in this field has studied the role of intestinal microbiome in these processes, which is the focus of the following section.
In early life, the intestinal microbiota regulates immune development and homeostasis, including immune tolerance and the life-long bias of the immune Th1/Th2 response [reviewed in (45, 46)]. The critical window for the establishment of the intestinal microbiome after birth is generally held to extend to ~2 years of age, though there continues to be considerable dynamism through childhood and adolescence (47). Whether the infant microbiome is seeded in utero remains the subject of debate (48). Intrapartum exposure to maternal genital and intestinal microbiota during vaginal delivery further enriches the microbial communities that will ultimately colonize the newborn (49). During the post-natal period, the maternal influence over the newborn microbiome continues with breastfeeding (50). Events that disrupt these periods of maternal microbial transfer and hasten the introduction of organisms from other maternal niches (e.g., skin) or environmental sources (e.g., formula, water, food) such as PTB (51, 52), caesarian section delivery (53–55), or the early cessation of breastfeeding (56), have a significant impact on the composition of the newborn intestinal microbiome. Notably, the microbiome of both mother and fetus have been associated with maternal health status. For example, the composition of the placental microbiome has been associated with maternal stress and gestational diabetes, whereas the infant intestinal microbiome is associated with maternal obesity, maternal diet (independent of obesity), gestational diabetes, antibiotic exposure, and other environmental exposures including maternal smoking and tobacco smoke exposure [reviewed in (57)].
The composition of the intestinal microbiome in early life has been implicated in an increasing array of disease states in childhood and later life. In preterm infants, perturbations in the intestinal microbial composition precedes the development of enterocolitis (52). In other studies, the composition of the intestinal microbiome during the early “critical window” has been associated with the development of allergy and atopy (including asthma, anaphylaxis, allergic rhinitis, atopic dermatitis) as well as immune-mediated conditions such as type 1 diabetes and inflammatory bowel disease later in childhood (45, 50, 58–62).
In addition to its impact on these conditions, intestinal dysbiosis in the early life period has been associated with neurodevelopmental outcomes. The critical window for the establishment of the microbiome is aligned with periods of rapid brain development, for example, the peak of synaptic pruning in the brain coincides with the maturation of the intestinal microbiota (63–66). Synaptic pruning associated with changes in intestinal microbiota results in alterations in specific white matter structures in the brain (67). In recent studies, intestinal diversity at 1 year of age was associated with cognitive abilities at 2 (68), 3 (69), and 4 years of age (70). Intestinal microbiota have also been found to be associated with behavioral outcomes (71) and neuropsychiatric outcomes such Attention Deficit Hyperactivity Disorder (ADHD) (72, 73) and autism [reviewed in (74)].
As outlined above, investing in the promotion of perinatal care has the potential to improve outcomes far beyond acute complications associated with poor birth outcomes. An investment in widespread access to comprehensive ANC, on a global scale, has the potential to improve health outcomes for children well past the first 1,000 days of life. However, globally, only 66% of pregnant women (less in LMIC) are accessing the absolute minimum of 4 ANC visits (75). Comprehensive prenatal care generally incorporates a more rigorous visit schedule (Table 2), including a first early visit before 12 weeks' gestation, a visit every 4 weeks up until 28 weeks' gestation, a visit every 2–3 weeks from 28 to 36 weeks' gestation, and weekly visits thereafter, until delivery (76). Therefore, in high-income countries, pregnant women typically attend 7–12 routine prenatal visits across pregnancy (76, 77).
In addition to services provided via basic ANC, a comprehensive approach to care includes screening for a wider array of risk factors for adverse pregnancy and long-term offspring outcomes, including perinatal anxiety, depression, and other psychosocial conditions, intimate partner violence, detailed nutritional screens and interventions, gestational diabetes, haemoglobinopathies, and a panel of high-risk infections (e.g., asymptomatic bacteriuria, syphilis, or infection with hepatitis B virus, Chlamydia trachomatis, Neisseria gonorrhea, cytomegalovirus, Group B streptococcus, etc.). Vaccinations and boosters including Tdap (tetanus, diphtheria, and pertussis) and influenza are also recommended as part of comprehensive ANC to prevent severe infections that could negatively impact in utero development and neonatal survival.
Ultrasound scans are an important component of comprehensive ANC. At the first visit (<12 weeks' gestation), ultrasound is recommended for accurate gestational dating. Accurate gestational dating is necessary to time screening and interventions, to identify and manage complications, and to inform precise birth plans that ensure safe facility deliveries (78, 79). Routine complete second trimester ultrasound scans (18–22 weeks) are used to screen for and/or diagnose fetal and placental anatomical abnormalities, identify the number of fetuses, and confirm gestational age. Furthermore, the second trimester anatomical scan can be used in conjunction with genetic screening (i.e., multiple marker serum screening) to establish risk of a chromosomal or genetic abnormality.
Inadequate ANC (defined as late or inconsistent access) increases the risk for PTB and LBW, as well as other triggers for the developmental origins of disease (i.e., missed vaccinations, and untreated maternal infections, metabolic disorders, and psychosocial conditions) in both LMIC and high-income countries (80–83).
The continuum of care for optimizing maternal-child health encompasses much more than ANC (84). The period of labor and delivery, and the first days of a newborn's life, are a high-risk period for both mother and child. To optimize maternal and child outcomes, attendance of a skilled provider at delivery, access to emergency obstetric care, and postnatal care are important to long-term maternal-child outcomes. The continuum of care for pregnant women and all women of reproductive age should also extend to sexual education, family planning (e.g., modern contraception), and safe abortion. There is abundant evidence that sexual education and family planning programs protect the in utero environment and reduce the burden of maternal-child morbidity and mortality by preventing acquisition of sexually transmitted infections (STIs), reducing rates of unintended pregnancies that are associated with inadequate ANC use, and decreasing the number of high-risk pregnancies more likely to end in PTB, LBW, and SGA (e.g., pregnancies in women <18 and >35 years of age, short birth intervals (<24 months), and high parity births) (84–86). Gaps in healthcare that increase the risk of poor health outcomes for the mother have the potential to translate into poor birth outcomes and could increase the risk of developmental origins of disease.
Barriers to comprehensive ANC and reproductive care are multi-faceted and include both social and structural barriers. These barriers have been extensively studied and reviewed (87–95). In brief, they include socioeconomic, educational, and geographical obstacles (e.g., cost and distance to a health facility, misperceptions surrounding pregnancy, inability to recognize danger signs), sociocultural obstacles (e.g., stigma around pregnancy, gender norms and inequities), and negative experiences with ANC (e.g., lack of privacy, long wait times, poor quality of care and/or care providers). Evidence also suggests use of ANC increases with maternal age, education, socioeconomic status, exposure to media, and contraception use, and decreases with parity and unintended pregnancies.
While the above studies investigated barriers to care in LMIC, many of the same barriers (e.g., socioeconomic, educational, and geographical obstacles, health system barriers, negative experiences with ANC) are also reflected in high-income countries including Canada and the USA (96–99). In high-income countries, these disparities often fall along racial lines with ethnic minorities carrying a disproportionate burden of inadequate access to ANC and reproductive health (99).
Legal restrictions and intimate partner violence are two further systemic barriers to adequate reproductive health and ANC. Legal restrictions on abortion, for example, trigger barriers to maternal-fetal health in several ways. Rates of unsafe abortion are directly correlated to restrictiveness of abortion laws (100). Legal restrictions on abortion also have indirect effects on in utero health with implications for the developmental origins of disease, including higher rates of unintended or unwanted pregnancies that are linked with reduced utilization of ANC and higher risk of adverse birth outcomes (101). Women who experience intimate partner violence are less likely to have control over their reproductive health (i.e., access to contraception and/or protection, forced pregnancy or forced termination, etc.), less likely to have access to ANC, especially early initiation of ANC, and are at higher risk for STIs, mental health disorders, and adverse birth outcomes (86, 102–106). At the most extreme end of intimate partner violence, homicide is a leading cause of death amongst pregnant women and women of reproductive age in the US (106, 107). Finally, in addition to baseline systemic issues, acute barriers that exacerbate existing inequities in access to ANC, including humanitarian crises, armed conflict, and global events (e.g., COVID-19 pandemic), must also be acknowledged for their impact on the in utero environment.
Many policies focus on individual aspects of maternal-child healthcare, rather than the continuum of care necessary for long-term positive pregnancy outcomes. Approximately 92% of all pregnancies occur in LMIC, where barriers to reproductive health are frequent, and access to ANC is most limited (101). ANC policies in many LMIC have incorporated versions of the WHO's four-visit FANC model, which has since been proven inadequate (9). In sub-Saharan Africa, only 7% of pregnant women attend the currently recommended 8+ ANC visits (93). National policies must be updated according to evidence-based recommendations. There are also disconnects between policy existence and implementation. Effective policy implementation requires underlying shifts in attitudes and behavior. Even with national ANC policies in place, unmet need for ANC remains overwhelming: in LMIC, an estimated 50 million pregnant women attend <4 ANC visits, 31 million do not deliver at a health facility, and 133 million do not receive the treatments needed for common STIs (108).
There has been substantial progress in improving maternal-child health over the past decade. Despite growing evidence highlighting the long-term, intergenerational impacts of the in utero environment, current socio-political indications in some regions of the world threaten a slide toward deprioritizing women's health. UNICEF reports that half of all maternal deaths and over half of all neonatal deaths are directly attributable to poor quality ANC (109). There is an urgent need to reinforce the developmental origins of disease and shift policy toward the prioritization of maternal-child health in global action and policies.
The most straightforward strategy to improve maternal-child health is investment. While standardized policy is required to guide global progress, policy change will be ineffective without simultaneously investing in strengthening overall health systems. Investment in quality of ANC health services (e.g., increasing health center staff, specialized personnel, and infrastructure; equitable distribution of medical commodities including medicines, vaccines, and technologies, etc.), infrastructure critical to accessing health services (e.g., roads connecting rural and urban communities, affordable transportation, etc.), and governmental investments to promote utilization (e.g., free maternal healthcare, primary resource allocation) could remove barriers to access for pregnant women (88, 110). The Guttmacher institute estimates that increasing annual investment in LMICs by $4.80 per capita would close existing gaps in sexual and reproductive health services (108). Increasing investment in the full continuum of reproductive health care would also have significant cascading impacts—for example, for every additional dollar invested in contraceptive services, an estimated three dollars in costs from maternal, neonatal, and abortion care would be saved, by averting unintended pregnancies (108).
Advocacy and community engagement are cornerstones to the behavior and attitude changes required to support policy. Women's empowerment and access to information/education directly correlate with early and consistent utilization of ANC and access to important pregnancy interventions (90, 93, 95), and promoting gender equity in both healthcare seekers and healthcare providers increases utilization of ANC (95, 111).
Finally, to enact effective policy change, we need to optimize the evidence base used to inform decision-making. There exist many gaps in our full scope of understanding of the biological, policy, and implementation issues facing maternal-child health, and even more gaps in how to solve them (112–117). Continued data collection to address these neglected areas, quality interventional research, and monitoring and evaluation of policies and implementation to allow for contextual adaptations, are critical to informing evidence-based approaches to improving immediate and long-term maternal-child health. We must continue to promote and support research in the field that fosters innovative, scalable, and empowering strategies to fill existing gaps in care [e.g., self-care interventions for provision of sexual and reproductive health in humanitarian crisis settings (117); integrated packages of care covering the full reproductive health continuum (84); interventions that acknowledge and improve maternal mental health (27), etc.].
Despite well-characterized barriers to ANC and allied healthcare, a devastating number of maternal-child dyads do not receive adequate, if any, ANC. Most studies highlighting provision of ANC and barriers to access have focused on its impacts on maternal and neonatal mortality. In this review, we show that mortality is only the tip of the iceberg. If we do not prioritize reproductive health and ANC, millions more children will experience preventable, lifelong, and intergenerational morbidities including chronic kidney disease and neurocognitive deficits that are triggered by preventable causes in utero. Current global maternal health policies and attitudes are failing our most vulnerable populations.
The evidence on the developmental origins of many chronic diseases support investments in maternal health to achieve broad impacts on population health. Most data on the developmental origins of health and disease come from high-income settings where there are differences in maternal health and nutritional status prior to and during pregnancy and differences in the nature and frequency of infectious exposures during pregnancy. An estimated 90% of global pregnancies occur in LMIC with an estimated 125 million pregnancies at risk of malaria annually (118). Here, we discuss developmental origins of disease and the rationale this provides for expanded ANC and allied reproductive and pediatric healthcare, without a principal focus on LMIC. However, we recognize that the burden of developmental origins of disease occurs principally in resource constrained settings, which justify enhanced attention and resource allocation (1, 2).
We know that investment in women and children living in poverty show enormous returns. In the face of conclusive evidence that comprehensive ANC improves health outcomes for mother and child, far too many women are not receiving adequate ANC. While the principal focus is and should remain on acute outcomes for mother and child, we propose that we can no longer ignore the vital importance of the in utero environment on the lifelong health and development of every child. Perhaps enhancing the focus on developmental origins of disease will further strengthen the evidence base required to shift focus and resources to reduce barriers and expand services of ANC. Protecting pregnant women and the in utero environment has the potential to promote healthy intergenerational outcomes.
The original contributions presented in the study are included in the article/supplementary material, further inquiries can be directed to the corresponding author/s.
All authors listed have made a substantial, direct, and intellectual contribution to the work and approved it for publication.
This work was supported by the Canadian Institutes of Health Research Foundation (grant no. FDN-148439 to KK), the Canada Research Chair Program (to KK), Open Philanthropy (to KK), and donations from Kim Kertland (to KK).
The authors declare that the research was conducted in the absence of any commercial or financial relationships that could be construed as a potential conflict of interest.
All claims expressed in this article are solely those of the authors and do not necessarily represent those of their affiliated organizations, or those of the publisher, the editors and the reviewers. Any product that may be evaluated in this article, or claim that may be made by its manufacturer, is not guaranteed or endorsed by the publisher.
1. Engle PL, Fernald LC, Alderman H, Behrman J, O'Gara C, Yousafzai A, et al. Strategies for reducing inequalities and improving developmental outcomes for young children in low-income and middle-income countries. Lancet. (2011) 378:1339–53. doi: 10.1016/S0140-6736(11)60889-1
2. Walker SP, Wachs TD, Grantham-McGregor S, Black MM, Nelson CA, Huffman SL, et al. Inequality in early childhood: risk and protective factors for early child development. Lancet. (2011) 378:1325–38. doi: 10.1016/S0140-6736(11)60555-2
3. Al-Haddad BJS, Oler E, Armistead B, Elsayed NA, Weinberger DR, Bernier R, et al. The fetal origins of mental illness. Am J Obstet Gynecol. (2019) 221:549–62. doi: 10.1016/j.ajog.2019.06.013
4. Barker DJ, Eriksson JG, Forsen T, Osmond C. Fetal origins of adult disease: strength of effects and biological basis. Int J Epidemiol. (2002) 31:1235–9. doi: 10.1093/ije/31.6.1235
5. Martin-Gronert MS, Ozanne SE. Mechanisms underlying the developmental origins of disease. Rev Endocr Metab Disord. (2012) 13:85–92. doi: 10.1007/s11154-012-9210-z
6. Bilbo SD, Schwarz JM. Early-life programming of later-life brain and behavior: a critical role for the immune system. Front Behav Neurosci. (2009) 3:14. doi: 10.3389/neuro.08.014.2009
7. Andrew DJ, Yelon D. Editorial overview: developmental mechanisms, patterning and organogenesis. Curr Opin Genet Dev. (2015) 32:5–8. doi: 10.1016/j.gde.2015.05.002
8. World Health Organization. WHO Antenatal Care Randomized Trial : Manual for the Implementation of the New Model. World Health Organization (2002). Available online at: https://apps.who.int/iris/handle/10665/42513 (accessed August 10, 2022).
9. Dowswell T, Carroli G, Duley L, Gates S, Gülmezoglu AM, Khan-Neelofur D, et al. Alternative versus standard packages of antenatal care for low-risk pregnancy. Cochrane Database Syst Rev. (2015) 2015:CD000934. doi: 10.1002/14651858.CD000934.pub3
10. Bilbo SD. How cytokines leave their mark: the role of the placenta in developmental programming of brain and behavior. Brain Behav Immun. (2011) 25:602–3. doi: 10.1016/j.bbi.2011.01.018
11. Cao G, Liu J, Liu M. Global, regional, and national incidence and mortality of neonatal preterm birth, 1990-2019. JAMA Pediatr. (2022) 176:787–96. doi: 10.1001/jamapediatrics.2022.1622
12. Parkinson JRC, Hyde MJ, Gale C, Santhakumaran S, Modi N. Preterm birth and the metabolic syndrome in adult life: a systematic review and meta-analysis. Pediatrics. (2013) 131:e1240–63. doi: 10.1542/peds.2012-2177
13. Crump C, Winkleby MA, Sundquist K, Sundquist J. Risk of diabetes among young adults born preterm in Sweden. Diabetes Care. (2011) 34:1109–13. doi: 10.2337/dc10-2108
14. Kajantie E, Strang-Karlsson S, Hovi P, Wehkalampi K, Lahti J, Kaseva N, et al. Insulin sensitivity and secretory response in adults born preterm: the Helsinki Study of Very Low Birth Weight Adults. J Clin Endocrinol Metab. (2015) 100:244–50. doi: 10.1210/jc.2014-3184
15. Black RE. Global prevalence of small for gestational age births. Nestle Nutr Ins Workshop Ser. (2015) 81:1–7. doi: 10.1159/000365790
16. Vollmer B, Edmonds CJ. School age neurological and cognitive outcomes of fetal growth retardation or small for gestational age birth weight. Front Endocrinol. (2019) 10:186. doi: 10.3389/fendo.2019.00186
17. Savchev S, Sanz-Cortes M, Cruz-Martinez R, Arranz A, Botet F, Gratacos E, et al. Neurodevelopmental outcome of full-term small-for-gestational-age infants with normal placental function. Ultrasound Obstet Gynecol. (2013) 42:201–6. doi: 10.1002/uog.12391
18. Knuesel I, Chicha L, Britschgi M, Schobel SA, Bodmer M, Hellings JA, et al. Maternal immune activation and abnormal brain development across CNS disorders. Nat Rev Neurol. (2014) 10:643–60. doi: 10.1038/nrneurol.2014.187
19. Weckman AM, Conroy AL, Madanitsa M, Gnaneswaran B, McDonald CR, Kalilani-Phiri L, et al. Neurocognitive outcomes in Malawian children exposed to malaria during pregnancy: an observational birth cohort study. PLoS Med. (2021) 18:e1003701. doi: 10.1371/journal.pmed.1003701
20. Bale TL. Epigenetic and transgenerational reprogramming of brain development. Nat Rev Neurosci. (2015) 16:332–44. doi: 10.1038/nrn3818
21. Bale TL, Baram TZ, Brown AS, Goldstein JM, Insel TR, McCarthy MM, et al. Early life programming and neurodevelopmental disorders. Biol Psychiatry. (2010) 68:314–9. doi: 10.1016/j.biopsych.2010.05.028
22. Faa G, Manchia M, Pintus R, Gerosa C, Marcialis MA, Fanos V. Fetal programming of neuropsychiatric disorders. Birth Defects Res C Embryo Today. (2016) 108:207–23. doi: 10.1002/bdrc.21139
23. Fanni D, Gerosa C, Rais M, Ravarino A, van Eyken P, Fanos V, et al. The role of neuropathological markers in the interpretation of neuropsychiatric disorders: focus on fetal and perinatal programming. Neurosci Lett. (2018) 669:75–82. doi: 10.1016/j.neulet.2016.10.063
24. McGill MG, Pokhvisneva I, Clappison AS, McEwen LM, Beijers R, Tollenaar MS, et al. Maternal prenatal anxiety and the fetal origins of epigenetic aging. Biol Psychiatry. (2022) 91:303–12. doi: 10.1016/j.biopsych.2021.07.025
25. Scheinost D, Spann MN, McDonough L, Peterson BS, Monk C. Associations between different dimensions of prenatal distress, neonatal hippocampal connectivity, and infant memory. Neuropsychopharmacology. (2020) 45:1272–9. doi: 10.1038/s41386-020-0677-0
26. Qiu A, Tuan TA, Ong ML, Li Y, Chen H, Rifkin-Graboi A, et al. COMT haplotypes modulate associations of antenatal maternal anxiety and neonatal cortical morphology. Am J Psychiatry. (2015) 172:163–72. doi: 10.1176/appi.ajp.2014.14030313
27. Arabin B, Hellmeyer L, Maul J, Metz GAS. Awareness of maternal stress, consequences for the offspring and the need for early interventions to increase stress resilience. J Perinat Med. (2021) 49:979–89. doi: 10.1515/jpm-2021-0323
28. Barker DJP. Maternal nutrition and cardiovascular disease. Nutr Health. (1993) 9:99–106. doi: 10.1177/026010609300900206
29. Armitage J, Poston L, Taylor P. Developmental origins of obesity and the metabolic syndrome: the role of maternal obesity. Front Horm Res. (2008) 36:73–84. doi: 10.1159/000115355
30. Hales CN, Barker DJP. Type 2 (non-insulin-dependent) diabetes mellitus: the thrifty phenotype hypothesis. Diabetologia. (1992) 35:595–601. doi: 10.1007/BF00400248
31. Barker DJP. The intrauterine origins of cardiovascular disease. Acta Paediatr Suppl. (1993) 82(Suppl. 391):93–9. doi: 10.1111/j.1651-2227.1993.tb12938.x
32. Barker DJ. Fetal origins of coronary heart disease. BMJ. (1995) 311:171. doi: 10.1136/bmj.311.6998.171
33. Hull HR, Dinger MK, Knehans AW, Thompson DM, Fields DA. Impact of maternal body mass index on neonate birthweight and body composition. Am J Obstet Gynecol. (2008) 198:416.e1–6. doi: 10.1016/j.ajog.2007.10.796
34. Sewell MF, Huston-Presley L, Super DM, Catalano P. Increased neonatal fat mass, not lean body mass, is associated with maternal obesity. Am J Obstet Gynecol. (2006) 195:1100–3. doi: 10.1016/j.ajog.2006.06.014
35. Starling AP, Brinton JT, Glueck DH, Shapiro AL, Harrod CS, Lynch AM, et al. Associations of maternal BMI and gestational weight gain with neonatal adiposity in the Healthy Start study. Am J Clin Nutr. (2015) 101:302–9. doi: 10.3945/ajcn.114.094946
36. Boney CM, Verma A, Tucker R, Vohr BR. Metabolic syndrome in childhood: association with birth weight, maternal obesity, and gestational diabetes mellitus. Pediatrics. (2005) 115:e290–6. doi: 10.1542/peds.2004-1808
37. Hochner H, Friedlander Y, Calderon-Margalit R, Meiner V, Sagy Y, Avgil-Tsadok M, et al. Associations of maternal prepregnancy body mass index and gestational weight gain with adult offspring cardiometabolic risk factors: the Jerusalem Perinatal Family Follow-up Study. Circulation. (2012) 125:1381–9. doi: 10.1161/CIRCULATIONAHA.111.070060
38. Mayer C, Joseph KS. Fetal growth: a review of terms, concepts and issues relevant to obstetrics. Ultrasound Obstet Gynecol. (2013) 41:136–45. doi: 10.1002/uog.11204
39. Thomason ME. Development of brain networks in utero: relevance for common neural disorders. Biol Psychiatry. (2020) 88:40–50. doi: 10.1016/j.biopsych.2020.02.007
40. Karimu AL, Burton GJ. The distribution of microvilli over the villous surface of the normal human term placenta is homogenous. Reprod Fertil Dev. (1995) 7:1269–73. doi: 10.1071/RD9951269
41. Mifsud W, Sebire NJ. Placental pathology in early-onset and late-onset fetal growth restriction. Fetal Diagn Ther. (2014) 36:117–28. doi: 10.1159/000359969
42. Salafia CM. Placental pathology of fetal growth restriction. Clin Obstet Gynecol. (1997) 40:740–9. doi: 10.1097/00003081-199712000-00008
43. Huynh J, Dawson D, Roberts D, Bentley-Lewis R. A systematic review of placental pathology in maternal diabetes mellitus. Placenta. (2015) 36:101–14. doi: 10.1016/j.placenta.2014.11.021
44. Mayhew TM. Fetoplacental angiogenesis during gestation is biphasic, longitudinal and occurs by proliferation and remodelling of vascular endothelial cells. Placenta. (2002) 23:742–50. doi: 10.1016/s0143-4004(02)90865-9
45. Stiemsma L, Reynolds L, Turvey S, Finlay B. The hygiene hypothesis: current perspectives and future therapies. Immunotargets Ther. (2015) 4:143. doi: 10.2147/ITT.S61528
46. Shreiner A, Huffnagle GB, Noverr MC. The “Microflora Hypothesis” of allergic disease. Adv Exp Med Biol. (2008) 635:113–34. doi: 10.1007/978-0-387-09550-9_10
47. Yatsunenko T, Rey FE, Manary MJ, Trehan I, Dominguez-Bello MG, Contreras M, et al. Human gut microbiome viewed across age and geography. Nature. (2012) 486:222–7. doi: 10.1038/nature11053
48. Walter J, Hornef MW. A philosophical perspective on the prenatal in utero microbiome debate. Microbiome. (2021) 9:1–9. doi: 10.1186/s40168-020-00979-7
49. Korpela K, Helve O, Kolho KL, Saisto T, Skogberg K, Dikareva E, et al. Maternal fecal microbiota transplantation in cesarean-born infants rapidly restores normal gut microbial development: a proof-of-concept study. Cell. (2020) 183:324–34.e5. doi: 10.1016/j.cell.2020.08.047
50. Stiemsma LT, Michels KB. The role of the microbiome in the developmental origins of health and disease. Pediatrics. (2018) 141:e20172437. doi: 10.1542/peds.2017-2437
51. Hiltunen H, Collado MC, Ollila H, Kolari T, Tölkkö S, Isolauri E, et al. Spontaneous preterm delivery is reflected in both early neonatal and maternal gut microbiota. Pediatr Res. (2022) 91:1804–11. doi: 10.1038/s41390-021-01663-8
52. Pammi M, Cope J, Tarr PI, Warner BB, Morrow AL, Mai V, et al. Intestinal dysbiosis in preterm infants preceding necrotizing enterocolitis: a systematic review and meta-analysis. Microbiome. (2017) 5:1–15. doi: 10.1186/s40168-017-0248-8
53. Bokulich NA, Chung J, Battaglia T, Henderson N, Jay M, Li H, et al. Antibiotics, birth mode, and diet shape microbiome maturation during early life. Sci Transl Med. (2016) 8:343ra82. doi: 10.1126/scitranslmed.aad7121
54. Chu DM, Ma J, Prince AL, Antony KM, Seferovic MD, Aagaard KM. Maturation of the infant microbiome community structure and function across multiple body sites and in relation to mode of delivery. Nature Med. (2017) 23:314–26. doi: 10.1038/nm.4272
55. Dominguez-Bello MG, Costello EK, Contreras M, Magris M, Hidalgo G, Fierer N, et al. Delivery mode shapes the acquisition and structure of the initial microbiota across multiple body habitats in newborns. Proc Natl Acad Sci USA. (2010) 107:11971–5. doi: 10.1073/pnas.1002601107
56. Harmsen HJM, Wildeboer-Veloo ACM, Raangs GC, Wagendorp AA, Klijn N, Bindels JG, et al. Analysis of intestinal flora development in breast-fed and formula-fed infants by using molecular identification and detection methods. J Pediatr Gastroenterol Nutr. (2000) 30:61–7. doi: 10.1097/00005176-200001000-00019
57. Kapourchali FR, Cresci GAM. Early-life gut microbiome-the importance of maternal and infant factors in its establishment. Nutr Clin Pract. (2020) 35:386–405. doi: 10.1002/ncp.10490
58. Abrahamsson TR, Jakobsson HE, Andersson AF, Björkstén B, Engstrand L, Jenmalm MC. Low gut microbiota diversity in early infancy precedes asthma at school age. Clin Exp Allergy. (2014) 44:842–50. doi: 10.1111/cea.12253
59. Azad MB, Konya T, Guttman DS, Field CJ, Sears MR, Hayglass KT, et al. Infant gut microbiota and food sensitization: associations in the first year of life. Clin Exp Allergy. (2015) 45:632–43. doi: 10.1111/cea.12487
60. Hviid A, Svanström H, Frisch M. Antibiotic use and inflammatory bowel diseases in childhood. Gut. (2011) 60:49–54. doi: 10.1136/gut.2010.219683
61. Mejía-León ME, Petrosino JF, Ajami NJ, Domínguez-Bello MG, de La Barca AMC. Fecal microbiota imbalance in Mexican children with type 1 diabetes. Sci Rep. (2014) 4:1–5. doi: 10.1038/srep03814
62. Shaw SY, Blanchard JF, Bernstein CN. Association between the use of antibiotics in the first year of life and pediatric inflammatory bowel disease. Am J Gastroenterol. (2010) 105:2687–92. doi: 10.1038/ajg.2010.398
63. Agans R, Rigsbee L, Kenche H, Michail S, Khamis HJ, Paliy O. Distal gut microbiota of adolescent children is different from that of adults. FEMS Microbiol Ecol. (2011) 77:404–12. doi: 10.1111/j.1574-6941.2011.01120.x
64. Erny D, de Angelis ALH, Jaitin D, Wieghofer P, Staszewski O, David E, et al. Host microbiota constantly control maturation and function of microglia in the CNS. Nat Neurosci. (2015) 18:965–77. doi: 10.1038/nn.4030
65. Cowan CSM, Dinan TG, Cryan JF. Annual research review: critical windows - the microbiota-gut-brain axis in neurocognitive development. J Child Psychol Psychiatry. (2020) 61:353–71. doi: 10.1111/jcpp.13156
66. Eltokhi A, Janmaat IE, Genedi M, Haarman BCM, Sommer IEC. Dysregulation of synaptic pruning as a possible link between intestinal microbiota dysbiosis and neuropsychiatric disorders. J Neurosci Res. (2020) 98:1335–69. doi: 10.1002/jnr.24616
67. Ong IM, Gonzalez JG, McIlwain SJ, Sawin EA, Schoen AJ, Adluru N, et al. Gut microbiome populations are associated with structure-specific changes in white matter architecture. Transl Psychiatry. (2018) 8:1–11. doi: 10.1038/s41398-017-0022-5
68. Carlson AL, Xia K, Azcarate-Peril MA, Goldman BD, Ahn M, Styner MA, et al. Infant gut microbiome associated with cognitive development. Biol Psychiatry. (2018) 83:148–59. doi: 10.1016/j.biopsych.2017.06.021
69. Rothenberg SE, Chen Q, Shen J, Nong Y, Nong H, Trinh EP, et al. Neurodevelopment correlates with gut microbiota in a cross-sectional analysis of children at 3 years of age in rural China. Sci Rep. (2021) 11:1–11. doi: 10.1038/s41598-021-86761-7
70. Streit F, Prandovszky E, Send T, Zillich L, Frank J, Sabunciyan S, et al. Microbiome profiles are associated with cognitive functioning in 45-month-old children. Brain Behav Immun. (2021) 98:151–60. doi: 10.1016/j.bbi.2021.08.001
71. Laue HE, Karagas MR, Coker MO, Bellinger DC, Baker ER, Korrick SA, et al. Sex-specific relationships of the infant microbiome and early-childhood behavioral outcomes. Pediatr Res. (2022) 92:580–91. doi: 10.1038/s41390-021-01785-z
72. Wan L, Ge WR, Zhang S, Sun YL, Wang B, Yang G. Case-control study of the effects of gut microbiota composition on neurotransmitter metabolic pathways in children with attention deficit hyperactivity disorder. Front Neurosci. (2020) 14:127. doi: 10.3389/fnins.2020.00127
73. Prehn-Kristensen A, Zimmermann A, Tittmann L, Lieb W, Schreiber S, Baving L, et al. Reduced microbiome alpha diversity in young patients with ADHD. PLoS ONE. (2018) 13:e0200728. doi: 10.1371/journal.pone.0200728
74. Ronan V, Yeasin R, Claud EC. Childhood development and the microbiome-the intestinal microbiota in maintenance of health and development of disease during childhood development. Gastroenterology. (2021) 160:495–506. doi: 10.1053/j.gastro.2020.08.065
75. UNICEF. Antenatal Care - UNICEF DATA. (2022). Available online at: https://data.unicef.org/topic/maternal-health/antenatal-care/ (accessed August 10, 2022).
77. Public Health Agency of Canada. “Chapter 3: Care During Pregnancy.” Maternity-Newborn Care Guidelines. (2020). Available online at: https://www.canada.ca/content/dam/hc-sc/documents/services/publications/healthy-living/maternity-newborn-care-guidelines-chapter-3/maternity-newborn-care-guidelines-chapter-3-en.pdf (accessed August 10, 2022).
78. Fulcher I, Hedt K, Marealle S, Tibaijuka J, Abdalla O, Hofmann R, et al. Errors in estimated gestational ages reduce the likelihood of health facility deliveries: results from an observational cohort study in Zanzibar. BMC Health Serv Res. (2020) 20:1–10. doi: 10.1186/s12913-020-4904-5
79. Morgan JA, Cooper DB. Pregnancy Dating. StatPearls (2021). Available online at: https://www.ncbi.nlm.nih.gov/books/NBK442018/ (accessed August 10, 2022).
80. Vintzileos AM, Ananth C v., Smulian JC, Scorza WE, Knuppel RA. The impact of prenatal care in the United States on preterm births in the presence and absence of antenatal high-risk conditions. Am J Obstet Gynecol. (2002) 187:1254–7. doi: 10.1067/mob.2002.127140
81. Kuhnt J, Vollmer S. Antenatal care services and its implications for vital and health outcomes of children: evidence from 193 surveys in 69 low-income and middle-income countries. BMJ Open. (2017) 7:e017122. doi: 10.1136/bmjopen-2017-017122
82. Nkoka O, Chuang TW, Chen YH. Association between timing and number of antenatal care visits on uptake of intermittent preventive treatment for malaria during pregnancy among Malawian women. Malar J. (2018) 17:1–11. doi: 10.1186/s12936-018-2360-z
83. Raut S, Apte A, Srinivasan M, Dudeja N, Dayma G, Sinha B, et al. Determinants of maternal influenza vaccination in the context of low- and middle-income countries: a systematic review. PLoS ONE. (2022) 17:e0262871. doi: 10.1371/journal.pone.0262871
84. Kerber KJ, de Graft-Johnson JE, Bhutta ZA, Okong P, Starrs A, Lawn JE. Continuum of care for maternal, newborn, and child health: from slogan to service delivery. Lancet. (2007) 370:1358–69. doi: 10.1016/S0140-6736(07)61578-5
85. Brown W, Ahmed S, Roche N, Sonneveldt E, Darmstadt GL. Impact of family planning programs in reducing high-risk births due to younger and older maternal age, short birth intervals, and high parity. Semin Perinat. (2015) 39:338–44. doi: 10.1053/j.semperi.2015.06.006
86. Glasier A, Gülmezoglu AM, Schmid GP, Moreno CG, van Look PF. Sexual and reproductive health: a matter of life and death. Lancet. (2006) 368:1595–607. doi: 10.1016/S0140-6736(06)69478-6
87. Downe S, Finlayson K, Tunçalp Ö, Gülmezoglu AM. Provision and uptake of routine antenatal services: a qualitative evidence synthesis. Cochrane Database Syst Rev. (2019) 6:CD012392. doi: 10.1002/14651858.CD012392.pub2
88. Dahab R, Sakellariou D. Barriers to accessing maternal care in low income countries in Africa: a systematic review. Int J Environ Res Public Health. (2020) 17:1–17. doi: 10.3390/ijerph17124292
89. Kyei-Nimakoh M, Carolan-Olah M, McCann Tv. Access barriers to obstetric care at health facilities in sub-Saharan Africa-a systematic review. Syst Rev. (2017) 6:110. doi: 10.1186/s13643-017-0503-x
90. Adedokun ST, Yaya S. Correlates of antenatal care utilization among women of reproductive age in sub-Saharan Africa: evidence from multinomial analysis of demographic and health surveys (2010-2018) from 31 countries. Arch Public Health. (2020) 78:134. doi: 10.1186/s13690-020-00516-w
91. Gabrysch S, Campbell OMR. Still too far to walk: literature review of the determinants of delivery service use. BMC Pregnancy Childbirth. (2009) 9:34. doi: 10.1186/1471-2393-9-34
92. Okedo-Alex IN, Akamike IC, Ezeanosike OB, Uneke CJ. Determinants of antenatal care utilisation in sub-Saharan Africa: a systematic review. BMJ Open. (2019) 9:e031890. doi: 10.1136/bmjopen-2019-031890
93. Tessema ZT, Teshale AB, Tesema GA, Tamirat KS. Determinants of completing recommended antenatal care utilization in sub-Saharan from 2006 to 2018: evidence from 36 countries using Demographic and Health Surveys. BMC Pregnancy Childbirth. (2021) 21:192. doi: 10.1186/s12884-021-03669-w
94. Tessema ZT, Tesema GA, Yazachew L. Individual-level and community-level factors associated with eight or more antenatal care contacts in sub-Saharan Africa: evidence from 36 sub-Saharan African countries. BMJ Open. (2022) 12:e049379. doi: 10.1136/bmjopen-2021-049379
95. Kareem YO, Morhason-Bello IO, OlaOlorun FM, Yaya S. Temporal relationship between Women's empowerment and utilization of antenatal care services: lessons from four National Surveys in sub-Saharan Africa. BMC Pregnancy Childbirth. (2021) 21:1–14. doi: 10.1186/s12884-021-03679-8
96. Heaman MI, Sword W, Elliott L, Moffatt M, Helewa ME, Morris H, et al. Barriers and facilitators related to use of prenatal care by inner-city women: perceptions of health care providers. BMC Pregnancy Childbirth. (2015) 15:2. doi: 10.1186/s12884-015-0431-5
97. Feijen-De Jong EI, Jansen DE, Baarveld F, van der Schans CP, Schellevis FG, Reijneveld SA. Determinants of late and/or inadequate use of prenatal healthcare in high-income countries: a systematic review. Eur J Public Health. (2012) 22:904–13. doi: 10.1093/eurpub/ckr164
98. Heaman MI, Martens PJ, Brownell MD, Chartier MJ, Thiessen KR, Derksen SA, et al. Inequities in utilization of prenatal care: a population-based study in the Canadian province of Manitoba. BMC Pregnancy Childbirth. (2018) 18:1–18. doi: 10.1186/s12884-018-2061-1
99. Grand-Guillaume-Perrenoud JA, Origlia P, Cignacco E. Barriers and facilitators of maternal healthcare utilisation in the perinatal period among women with social disadvantage: a theory-guided systematic review. Midwifery. (2022) 105:103237. doi: 10.1016/j.midw.2021.103237
100. Ganatra B, Gerdts C, Rossier C, Johnson BR, Tunçalp Ö, Assifi A, et al. Global, regional, and subregional classification of abortions by safety, 2010-14: estimates from a Bayesian hierarchical model. Lancet. (2017) 390:2372–81. doi: 10.1016/S0140-6736(17)31794-4
101. Bearak J, Popinchalk A, Ganatra B, Moller AB, Tunçalp Ö, Beavin C, et al. Unintended pregnancy and abortion by income, region, and the legal status of abortion: estimates from a comprehensive model for 1990-2019. Lancet Glob Health. (2020) 8:e1152–61. doi: 10.1016/S2214-109X(20)30315-6
102. Bahati C, Izabayo J, Niyonsenga J, Sezibera V, Mutesa L. Intimate partner violence as a predictor of antenatal care services utilization in Rwanda. BMC Pregnancy Childbirth. (2021) 21:754. doi: 10.1186/s12884-021-04230-5
103. Aboagye RG, Seidu AA, Asare BYA, Adu C, Ahinkorah BO. Intimate partner violence and timely antenatal care visits in sub-Saharan Africa. Arch Public Health. (2022) 80:124. doi: 10.1186/s13690-022-00853-y
104. Tura H, Licoze A. Women's experience of intimate partner violence and uptake of Antenatal Care in Sofala, Mozambique. PLoS ONE. (2019) 14:e0217407. doi: 10.1371/journal.pone.0217407
105. Alhusen JL, Ray E, Sharps P, Bullock L. Intimate partner violence during pregnancy: maternal and neonatal outcomes. J Womens Health. (2015) 24:100–6. doi: 10.1089/jwh.2014.4872
106. Fay KE, Onwuzurike C, Finley A, Miller E. Integrating anti-violence efforts into sexual and reproductive health: reproductive coercion as a case example. Contraception. (2022) 115:75–9. doi: 10.1016/j.contraception.2022.06.006
107. Wallace M, Gillispie-Bell V, Cruz K, Davis K, Vilda D. Homicide during pregnancy and the postpartum period in the United States, 2018-2019. Obstet Gynecol. (2021) 138:762–9. doi: 10.1097/AOG.0000000000004567
108. Sully EA, Biddlecom A, Darroch JE, Riley T, Ashford LS, Lince-Deroche N, et al. Adding It Up: Investing in Sexual and Reproductive Health 2019. New York, NY (2020). doi: 10.1363/2020.31593
109. UNICEF WHO. Ending Preventable Newborn Deaths Stillbirths by 2030: Moving Faster Towards High-Quality Universal Health Coverage 2020-2025. (2020). Available online at: https://data.unicef.org/resources/ending-preventable-newborn-deaths-and-stillbirths-by-2030/ (accessed August 10, 2022).
110. Gamberini C, Angeli F, Ambrosino E. Exploring solutions to improve antenatal care in resource-limited settings: an expert consultation. BMC Pregnancy Childbirth. (2022) 22:449. doi: 10.1186/s12884-022-04778-w
111. Bhan N, McDougal L, Singh A, Atmavilas Y, Raj A. Access to women physicians and uptake of reproductive, maternal and child health services in India. EClinicalMedicine. (2020) 20:100309. doi: 10.1016/j.eclinm.2020.100309
112. de Jongh TE, Gurol-Urganci I, Allen E, Zhu NJ, Atun R. Integration of antenatal care services with health programmes in low- and middle-income countries: systematic review. J Global Health. (2016) 6:010403. doi: 10.7189/jogh.06.010403
113. McDonald CR, Weckman AM, Wright JK, Conroy AL, Kain KC. Pregnant women in low- and middle-income countries require a special focus during the COVID-19 pandemic. Front Glob Womens Health. (2020) 1:564560. doi: 10.3389/fgwh.2020.564560
114. Eggleston AJ, Richards A, Farrington E, Tse WC, Williams J, Sella Hewage A, et al. Randomised trials in maternal and perinatal health in low and middle-income countries from 2010 to 2019: a systematic scoping review. BMJ Open. (2022) 12:e059473. doi: 10.1136/bmjopen-2021-059473
115. Singh NS, Aryasinghe S, Smith J, Khosla R, Say L, Blanchet K. A long way to go: a systematic review to assess the utilisation of sexual and reproductive health services during humanitarian crises. BMJ Glob Health. (2018) 3:e000682. doi: 10.1136/bmjgh-2017-000682
116. Chersich M, Blaauw D, Dumbaugh M, Penn-Kekana L, Thwala S, Bijlmakers L, et al. Mapping of research on maternal health interventions in low- and middle-income countries: a review of 2292 publications between 2000 and 2012. Global Health. (2016) 12:52. doi: 10.1186/s12992-016-0189-1
117. Logie CH, Khoshnood K, Okumu M, Rashid SF, Senova F, Meghari H, et al. Self care interventions could advance sexual and reproductive health in humanitarian settings. BMJ. (2019) 365:l1083. doi: 10.1136/bmj.l1083
118. Dellicour S, Tatem AJ, Guerra CA, Snow RW, ter Kuile FO. Quantifying the number of pregnancies at risk of malaria in 2007: a demographic study. PLoS Med. (2010) 7:e1000221. doi: 10.1371/journal.pmed.1000221
119. Cannon TD, van Erp TGM, Rosso IM, Huttunen M, Lönnqvist J, Pirkola T, et al. Fetal hypoxia and structural brain abnormalities in schizophrenic patients, their siblings, and controls. Arch Gen Psychiatry. (2002) 59:35–41. doi: 10.1001/archpsyc.59.1.35
120. Schnurr TM, Ängquist L, Nøhr EA, Hansen T, Sørensen TIA, Morgen CS. Smoking during pregnancy is associated with child overweight independent of maternal pre-pregnancy BMI and genetic predisposition to adiposity. Sci Rep. (2022) 12:3135. doi: 10.1038/s41598-022-07122-6
121. Giussani DA. Breath of life: heart disease link to developmental hypoxia. Circulation. (2021) 144:1429–43. doi: 10.1161/CIRCULATIONAHA.121.054689
122. Al-Haddad BJS, Jacobsson B, Chabra S, Modzelewska D, Olson EM, Bernier R, et al. Long-term risk of neuropsychiatric disease after exposure to infection in utero. JAMA Psychiatry. (2019) 76:594–602. doi: 10.1001/jamapsychiatry.2019.0029
123. Bangirana P, Conroy AL, Opoka RO, Semrud-Clikeman M, Jang JH, Apayi C, et al. Effect of malaria and malaria chemoprevention regimens in pregnancy and childhood on neurodevelopmental and behavioral outcomes in children at 12, 24 and 36 months: a randomized clinical trial. Clin Infect Dis. (2022) ciac815. doi: 10.1093/cid/ciac815
124. Bedu-Addo G, Alicke M, Boakye-Appiah JK, Abdul-Jalil I, van der Giet M, Schulze MB, et al. In utero exposure to malaria is associated with metabolic traits in adolescence: the Agogo 2000 birth cohort study. J Infect. (2017) 75:455–63. doi: 10.1016/j.jinf.2017.08.010
125. Edlow AG, Castro VM, Shook LL, Kaimal AJ, Perlis RH. Neurodevelopmental outcomes at 1 year in infants of mothers who tested positive for SARS-CoV-2 during pregnancy. JAMA Netw Open. (2022) 5:e2215787. doi: 10.1001/jamanetworkopen.2022.15787
126. Abtibol-Bernardino MR, de Almeida Peixoto L de FA, de Oliveira GA, de Almeida TF, Rodrigues GRI, Otani RH, et al. Neurological findings in children without congenital microcephaly exposed to Zika virus in utero: a case series study. Viruses. (2020) 12:1335. doi: 10.3390/v12111335
127. Eberle C, Fasig T, Brüseke F, Stichling S. Impact of maternal prenatal stress by glucocorticoids on metabolic and cardiovascular outcomes in their offspring: a systematic scoping review. PLoS ONE. (2021) 16:e0245386. doi: 10.1371/journal.pone.0245386
128. Mueller NT, Bakacs E, Combellick J, Grigoryan Z, Dominguez-Bello MG. The infant microbiome development: mom matters. Trends Mol Med. (2015) 21:109–17. doi: 10.1016/j.molmed.2014.12.002
129. Kong L, Chen X, Liang Y, Forsell Y, Gissler M, Lavebratt C. Association of preeclampsia and perinatal complications with offspring neurodevelopmental and psychiatric disorders. JAMA Netw Open. (2022) 5:e2145719. doi: 10.1001/jamanetworkopen.2021.45719
130. Huang C, Li J, Qin G, Liew Z, Hu J, László KD, et al. Maternal hypertensive disorder of pregnancy and offspring early-onset cardiovascular disease in childhood, adolescence, and young adulthood: a national population-based cohort study. PLOS Med. (2021) 18:e1003805. doi: 10.1371/journal.pmed.1003805
131. Kong L, Nilsson IAK, Brismar K, Gissler M, Lavebratt C. Associations of different types of maternal diabetes and body mass index with offspring psychiatric disorders. JAMA Netw Open. (2020) 3:e1920787. doi: 10.1001/jamanetworkopen.2019.20787
132. Nogueira Avelar E Silva R, Yu Y, Liew Z, Vested A, Sørensen HT, Li J. Associations of maternal diabetes during pregnancy with psychiatric disorders in offspring during the first 4 decades of life in a population-based Danish Birth Cohort. JAMA Netw Open. (2021) 4:e2128005. doi: 10.1001/jamanetworkopen.2021.28005
133. Yu Y, Arah OA, Liew Z, Cnattingius S, Olsen J, Sørensen HT, et al. Maternal diabetes during pregnancy and early onset of cardiovascular disease in offspring: population based cohort study with 40 years of follow-up. BMJ. (2019) 367. doi: 10.1136/bmj.l6398
134. Mcnamara BJ, Gubhaju L, Chamberlain C, Stanley F, Eades SJ. Early life influences on cardio-metabolic disease risk in aboriginal populations–what is the evidence? A systematic review of longitudinal and case-control studies. Int J Epidemiol. (2012) 41:1661–82. doi: 10.1093/ije/dys190
135. Sirilert S, Tongsong T. Hepatitis B virus infection in pregnancy: immunological response, natural course and pregnancy outcomes. J Clin Med. (2021) 10:2926. doi: 10.3390/jcm10132926
136. Boivin MJ, Maliwichi-Senganimalunje L, Ogwang LW, Kawalazira R, Sikorskii A, Familiar-Lopez I, et al. Neurodevelopmental effects of ante-partum and post-partum antiretroviral exposure in HIV-exposed and uninfected children versus HIV-unexposed and uninfected children in Uganda and Malawi: a prospective cohort study. Lancet HIV. (2019) 6:e518–30. doi: 10.1016/S2352-3018(19)30083-9
137. Benki-Nugent SF, Yunusa R, Mueni A, Laboso T, Tamasha N, Njuguna I, et al. Lower neurocognitive functioning in HIV-exposed uninfected children compared with that in HIV-unexposed children. J Acquir Immune Defic Syndr. (2022) 89:441–7. doi: 10.1097/QAI.0000000000002881
138. Bui DS, Perret JL, Walters EH, Lodge CJ, Bowatte G, Hamilton GS, et al. Association between very to moderate preterm births, lung function deficits, and COPD at age 53 years: analysis of a prospective cohort study. Lancet Respir Med. (2022) 10:478–84. doi: 10.1016/S2213-2600(21)00508-7
139. Villar J, Restrepo-Méndez MC, McGready R, Barros FC, Victora CG, Munim S, et al. Association between preterm-birth phenotypes and differential morbidity, growth, and neurodevelopment at age 2 years: results from the INTERBIO-21st Newborn Study. JAMA Pediatr. (2021) 175:483–93. doi: 10.1001/jamapediatrics.2020.6087
140. Uemura O, Ishikura K, Kaneko T, Hirano D, Hamasaki Y, Ogura M, et al. Perinatal factors contributing to chronic kidney disease in a cohort of Japanese children with very low birth weight. Pediatr Nephrol. (2021) 36:953–60. doi: 10.1007/s00467-020-04791-1
Keywords: pregnancy, in utero development, maternal health, antenatal care, origins of disease
Citation: McDonald CR, Weckman AM, Wright JK, Conroy AL and Kain KC (2022) Developmental origins of disease highlight the immediate need for expanded access to comprehensive prenatal care. Front. Public Health 10:1021901. doi: 10.3389/fpubh.2022.1021901
Received: 17 August 2022; Accepted: 01 November 2022;
Published: 24 November 2022.
Edited by:
Meghna Ranganathan, University of London, United KingdomReviewed by:
Marta Cristina Antonelli, Consejo Nacional de Investigaciones Científicas y Técnicas (CONICET), ArgentinaCopyright © 2022 McDonald, Weckman, Wright, Conroy and Kain. This is an open-access article distributed under the terms of the Creative Commons Attribution License (CC BY). The use, distribution or reproduction in other forums is permitted, provided the original author(s) and the copyright owner(s) are credited and that the original publication in this journal is cited, in accordance with accepted academic practice. No use, distribution or reproduction is permitted which does not comply with these terms.
*Correspondence: Kevin C. Kain, a2V2aW4ua2FpbkB1aG4uY2E=
†These authors share first authorship
Disclaimer: All claims expressed in this article are solely those of the authors and do not necessarily represent those of their affiliated organizations, or those of the publisher, the editors and the reviewers. Any product that may be evaluated in this article or claim that may be made by its manufacturer is not guaranteed or endorsed by the publisher.
Research integrity at Frontiers
Learn more about the work of our research integrity team to safeguard the quality of each article we publish.