- 1Heidelberg Institute of Global Health, Heidelberg University Hospital, Heidelberg, Germany
- 2Clinical Research Unit of Nanoro, Institut de Recherche en Sciences de la Santé, Nanoro, Burkina Faso
- 3Centre for Infectious Diseases, Parasitology, Heidelberg University Hospital, Heidelberg, Germany
- 4KEMRI-Wellcome Trust Research Programme, Centre for Geographic Medicine Research-Coast, Kilifi, Kenya
- 5Francis I Proctor Foundation, University of California, San Francisco, San Francisco, CA, United States
- 6Department of Ophthalmology, University of California, San Francisco, San Francisco, CA, United States
- 7Department of Epidemiology and Biostatistics, University of California, San Francisco, San Francisco, CA, United States
- 8Deptartment of Pediatrics, Stanford School of Medicine, Stanford, CA, United States
- 9Department of Global Health and Population, Harvard T.H. Chan School of Public Health, Boston, MA, United States
- 10Africa Health Research Institute (AHRI), KwaZulu-Natal, South Africa
- 11Center for Global Health, Colorado School of Public Health, Aurora, CO, United States
- 12Department of Epidemiology, Colorado School of Public Health, Aurora, CO, United States
- 13Department of Public Health, Erasmus MC, University Medical Center Rotterdam, Rotterdam, Netherlands
Background: Young children in Sub-Saharan Africa (SSA), particularly those from resource-limited settings, are heavily burdened by anemia and malaria. While malaria infected children frequently become anemic (hemoglobin <110 g/L), anemia is a strongly multifactorial disease with many other risk factors than malaria. Due to the complex and often overlapping contributors to anemia, it remains challenging to isolate the true impact of malaria on population level hemoglobin concentrations.
Methods: We quantified the malaria-induced effect on hemoglobin levels in children under 5 years of age, leveraging data from 7,384 twins and other multiples, aged 6 to 59 months, from 57 nationally representative Demographic and Health Surveys (DHSs) from 23 SSA countries from 2006 to 2019. The quasi-experimental twin fixed-effect design let us minimize the impact of potential confounders that do not vary between twins.
Results: Our analyses of twins revealed a malaria-induced hemoglobin decrease in infected twins of 9 g/L (95% CI -10; -7, p<0.001). The relative risk of severe anemia was higher (RR = 3.01, 95% CI 1.79; 5.1, p<0.001) among malaria positive children, compared to malaria negative children. Conversely, malaria positive children are only half as likely to be non-anemic (RR = 0.51, 95% CI 0.43; 0.61, p<0.001).
Conclusion: Even after rigorous control for confounding through a twin fixed-effects study design, malaria substantially decreased hemoglobin levels among SSA twins, rendering them much more susceptible to severe anemia. This effect reflects the population-level effect of malaria on anemia.
Introduction
Young children from low- and middle-income countries frequently suffer from a wide range of health burdens specific to their under-resourced settings. In Sub-Saharan Africa (SSA) these often include anemia of multifactorial origin and endemic infectious diseases such as malaria, where recent estimates have suggested a prevalence of up to 60% (Supplementary material S1) (1–4). Childhood anemia (WHO definition: hemoglobin <110 g/L) has complex etiologies, including malaria, socioeconomic factors, nutritional deficits like iron deficiency, genetic disorders such as sickle cell disease, and other infectious diseases including helminths, intestinal and respiratory diseases (1, 2, 5, 6). Anemia impairs the cognitive and physical development of young children, subsequently reducing educational achievements, labor market opportunities and, ultimately, perpetuating cycles of poverty by limiting the potential of young generations to flourish (7–10). Prieto-Patron et al. (11) estimated the total average cost of iron deficiency anemia in Cote d'Ivoire alone to exceed 890 million USD (2.5% of GDP), and 214 700 disability-adjusted life years (11). Assuming that iron deficiency anemia constitutes only one quarter to one half of the total anemia burden in SSA, the projected total costs of anemia (all etiologies) are devastatingly high (12). Furthermore, according to a recent analysis malaria might account for up to a third of iron-deficiency anemia cases in endemic countries (13).
Malaria infections frequently lead to anemia, acutely, through erythrocyte hemolysis, and in chronic and recurring forms through persisting bone-marrow depression and inflammation (14–19). Malaria is caused by the Plasmodium parasite genus whose species differ in both geographic distribution and severity of the resulting disease (20, 21). Globally, more than 95% of annual malaria cases occur in SSA, partly because it is almost exclusively affected by the particularly aggressive Plasmodium falciparum (22–24). In 2020 P. falciparum caused over 227 million cases of malaria in SSA, with 602 000 fatal outcomes out of which 80% were children younger than 5 years old (24). Despite the clear links between malaria and anemia, the true impact of malaria on population hemoglobin levels is obscured by the complex and often overlapping contributors to anemia.
To bridge this knowledge gap and isolate the impact of malaria on hemoglobin, we pooled nationally representative survey data from 23 SSA countries. Mining these datasets for pairs of twins and higher order multiples (triplets and quadruplets; for ease of reading henceforth also referred to as “twins”, Figure 1) offered the opportunity to account for factors that are only shared between twins and are otherwise nearly impossible to measure in large trials such as in-utero, perinatal and early childhood exposures (25, 26). To achieve this level of control, we adapted an econometric statistical method for our main analysis, fixed effect regression, to rigorously control for most of the major risk factors of anemia that are identical between twins, including temporal and regional constants, mother-dependent variables, and socioeconomic status (25–29).
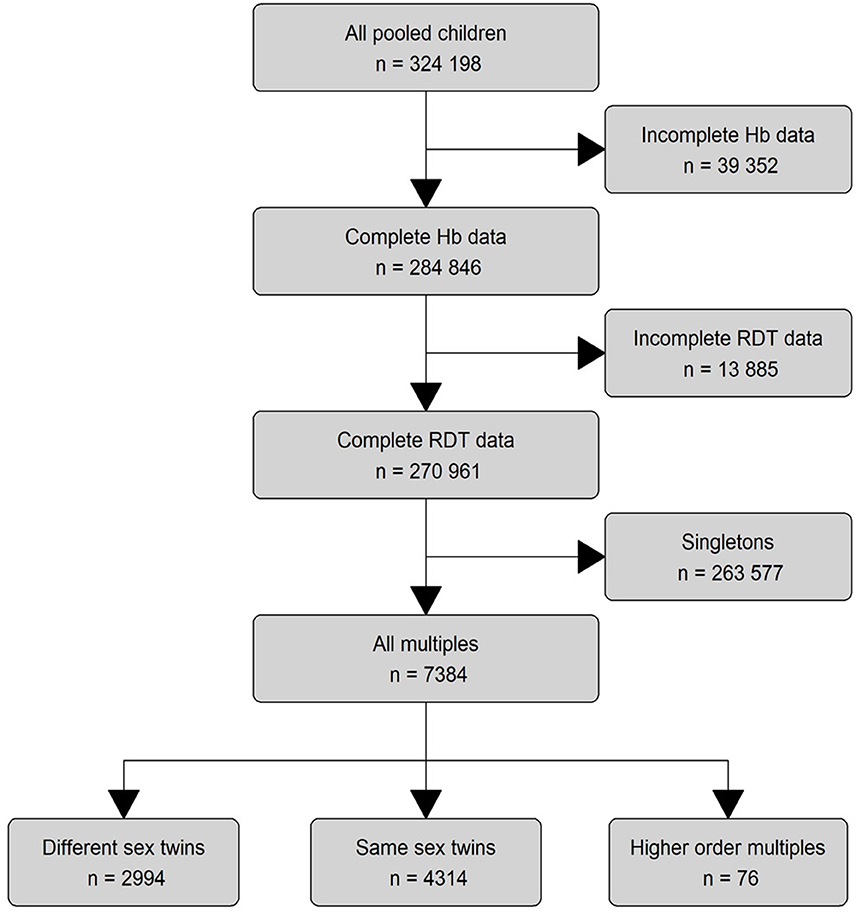
Figure 1. Eligibility assessment and data processing. We pooled 324,198 children from all available DHS surveys (57 surveys from 23 countries) that contained hemoglobin and malaria outcomes. We analyzed the data on three different levels: The main descriptive analysis was performed on all children with complete RDT data (n = 270,961). The main regression model was run on all multiples (n = 7,384), while the subset analyses were performed on the lowest level for different-sex twins alone (n = 4,314) and same-sex and higher level multiples combined (n = 3,070). Blind ended boxes show cases that did not meet subset criteria and were not considered in any analysis. Boxes with continued lines show cases used for analysis.
Our study aims to isolate the exact malaria-induced change in hemoglobin levels in endemic settings of SSA. This is currently of particular relevance as recent promising malaria-vaccine trials and the consecutive recommendation of the WHO to vaccine young children give hope of an imminent improvement of the malaria burden to endemic countries (24, 30, 31). Our study will shed light on the potential gains against anemia secondary to an improved or successful malaria elimination process.
Methods
Data and variables
We used nationally representative survey data which are available through USAID's Demographic and Health Surveys (DHSs) and Malaria Indicator Surveys (MISs) (DHS Program, RRID:SCR_000905). During the surveys, trained field workers conducted extensive interviews, collected biomarkers, and gathered anthropometric data from a representative cluster-based sample of households in the respective survey country (Figure 2). All households and participants receive new identifiers for each survey, thus the pooled data is strictly cross-sectional, as it is impossible to trace participants across surveys. All data and details on the data generation process are freely available from the DHS website (32).
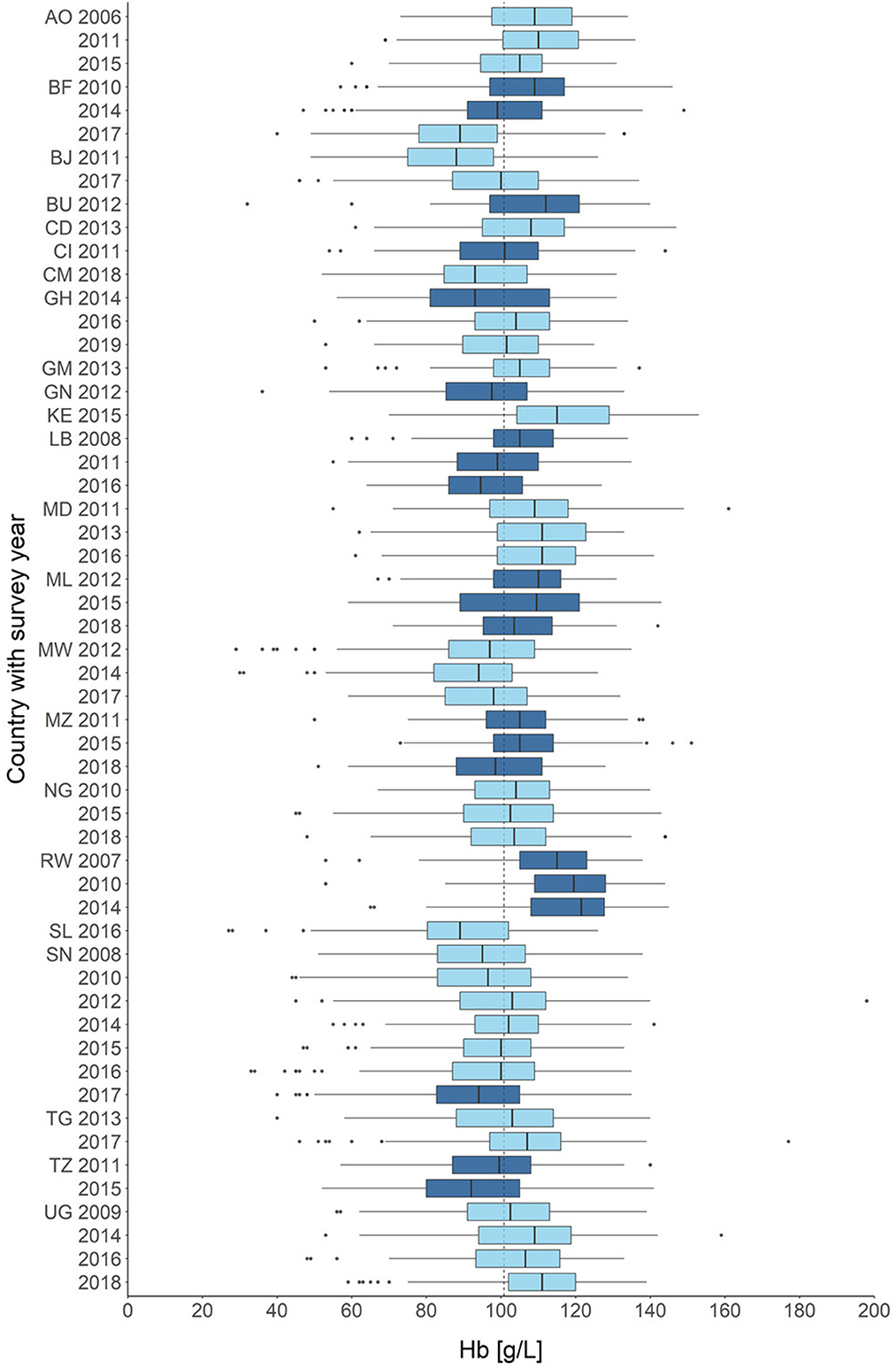
Figure 2. Distribution of hemoglobin across all children in all included surveys (n = 270,961). Countries are color-coded, surveys with years are given on the y-axis. Hemoglobin concentrations are given in g/L on the x-axis. AO, Angola; BF, Burkina Faso; BJ, Benin; BU, Burundi; CD, Côte d'Ivoire; CM, Cameroon; GH, Ghana; GM, Gambia; GN, Guinea; KE, Kenya; LB, Liberia; MD, Madagascar; ML, Mali; MW, Malawi; MZ, Mozambique; NG, Nigeria; RW, Rwanda; SL, Sierra Leone; SN, Senegal; TG, Togo; TZ, Tanzania; UG, Uganda. Survey year is set when the survey was begun, some surveys crossed over into the following years.
All surveys from the SSA region that contained hemoglobin measurements and rapid diagnostic tests (RDT)-based malaria measurements were eligible for analysis. We downloaded the survey data using the rdhs (v0.7.1) R package and extracted the variabes sex, hemoglobin and RDT result for analysis. Furthermore, we extracted the survey identifier, the household identifier, the date of birth and the mother identifier to identify the twin pairs. The datasets were restricted to children with complete RDT and hemoglobin measurements.
We limited the data to children aged 6–59 months as younger children typically benefit from pregnancy related IgG-transfers, i.e., they are still protected by the mother's immune system, while older children will have had significantly more exposure to malaria and begin to develop partial immunity of their own (26, 33). We further regionally restricted the data to Sub-Saharan Africa because of its high prevalence of malaria and almost exclusive endemicity of P. falciparum (22, 23). We used blood hemoglobin levels as a proxy for anemia in line with the WHO definition of anemia for children (hemoglobin ≥110 g/L healthy; 109–100 g/L mild anemia; 99–70 g/L moderate anemia; <70 g/L severe anemia) (5). Hemoglobin levels were measured using HemoCue test systems (32).
We used the malaria test results from established RDT systems to identify the children with parasitemia, understood as a binary indicator for parasite presence. Unfortunately, there was no quantitative measurement of parasitemia (i.e., parasite density) available in the given data. RDTs are frequently used in remote clinical settings and surveys because they work well-with minimal laboratory equipment while providing solid and immediate results on site. The tests detect plasmodium-specific antigens in a lateral flow system to diagnose malaria (34, 35).
Unfortunately, the data did not contain reliable information on potentially confounding causes of anemia such as iron status, coinfections or genetic disorders.
Data preparation
The flowchart illustrates the process of data preparation and eligibility assessment (Figure 1). The eligibility criteria of SSA-region, hemoglobin and RDT-results were met by 57 surveys from 23 countries, spanning a time frame from 2006 to 2019 (Figure 2).
Since there was no predefined twin-identifier in the given data, we manually constructed a twin-identifier variable from the country code, survey year, cluster identifier, household identifier, mother identifier and date of birth (century month code) of the child, thus ensuring that only twins and higher order multiples shared a common indicator. The resulting main dataset contained 7,384 monozygotic and dizygotic twins with complete data and was used in the analysis of the main model.
With the given data it is not possible to exclusively identify monozygotic twins. Instead, we approached a selection of monozygotic twins by creating a subset excluding twin pairs with differing sex and all multiples (same-sex and different-sex alike), to maximize the probability of genetic similarity (36). This subset of same-sex twins included 4,314 individuals out of which 1,320 (30.6%) will likely be monozygotic. We provide a more in depth explanation of this approach in the Supplementary material S2.
Statistical analysis
We first described the overall patterns in the population structure of all children and of the twin subsets to enable easier comparison between the groups and assess the representativeness of the twins.
We further added a visual analysis of the hemoglobin distributions among all children for each included survey and created prevalence maps for anemia and malaria for the most recent survey in every country (Supplementary material S1). We used only the twins and sex-subsets for the inferential analysis, not the pooled data of all children. For each of the three regression datasets (main, same-sex, different-sex) we constructed a linear twin-fixed effect regression model. Each of the models estimated the expected difference in hemoglobin of twins with parasitemia compared to those without, with a separate intercept for each pair of twins. In more descriptive terms, the model considers a twin pair as a single entity, where the individual siblings within a twin pair represent two simultaneous measurements of the same entity in two different exposure-states (25, 28). The quasi-experimental objective was to analyse the parasitemia-associated hemoglobin change in two counter-factual realities of one child (as represented through a twin pair). The twin pairs that did not differ in parasitemia status could not be used to estimate the hemoglobin change, however they provided power to the overall analysis. This approach is increasingly popular in epidemiologic research and allowed us to minimize the impact of potential confounders that do not vary between twins, including most relevant risk factors of anemia (27, 29). The formula for the main model is provided with the Supplementary material S3.
We adjusted our main and different-sex models for sex and the twin fixed-effect, the same-sex model for the twin fixed-effect only. All variables that did not vary between twins, such as country, age, test method, etc. were perfectly colinear with the twin-fixed effect and were therefore excluded from the statistical model (28). We further added subset analyses for each individual country and stratified by age group to account modulating effects of these factors (Supplementary material S4).
The quasi-poisson models are four separate extensions of the main model structure that allowed us to estimate the malaria effect on the relative risk of mild or worse (<110 g/L), moderate or worse (<90 g/L) and severe anemia (<70 g/L). These analyses illustrate in a more tangible way the consequences that are implied in the malaria-induced hemoglobin reductions. All analyses were performed using R version 4.0.2. We extracted, cleaned and analyzed the data with the help of the following r packages (rdhs 0.6.3; plm 2.2.4, fixest 0.8.3).
Results
An overview of the demographic composition of the datasets used for analysis is provided in Table 1. Demographic summaries of each survey are provided in the Supplementary materials S5, S6. Across all surveys, most children (62.9%) suffered from at least mild anemia (Hb <110 g/L). The prevalence of anemia was higher in plasmodium positive children than in negative ones (82.4 vs. 56.2%) (Supplementary material S7). The average hemoglobin across the included surveys decreased marginally but statistically significant over time (2006–2010: 106 g/L, 2015–2019: 103 g/L; unpaired T-test: p<0.001). The pooled prevalence of plasmodium parasitemia also remained largely constant between survey years (2006–2010: 18.4%, 2015–2019: 18.8%; two proportions Z-test: p = 0.002). The distribution of hemoglobin in each survey is shown in Figure 2. In total 547 (14.89%) of the total 3,679 twin pairs had disconcordant parasitemia status, which was leveraged for the fixed-effect model. Finally, there was no statistically significant difference in the hemoglobin distributions of same-sex and different-sex twins in a two sample unpaired T-test (95% CI -0.7; 0.9, p = 0.808).
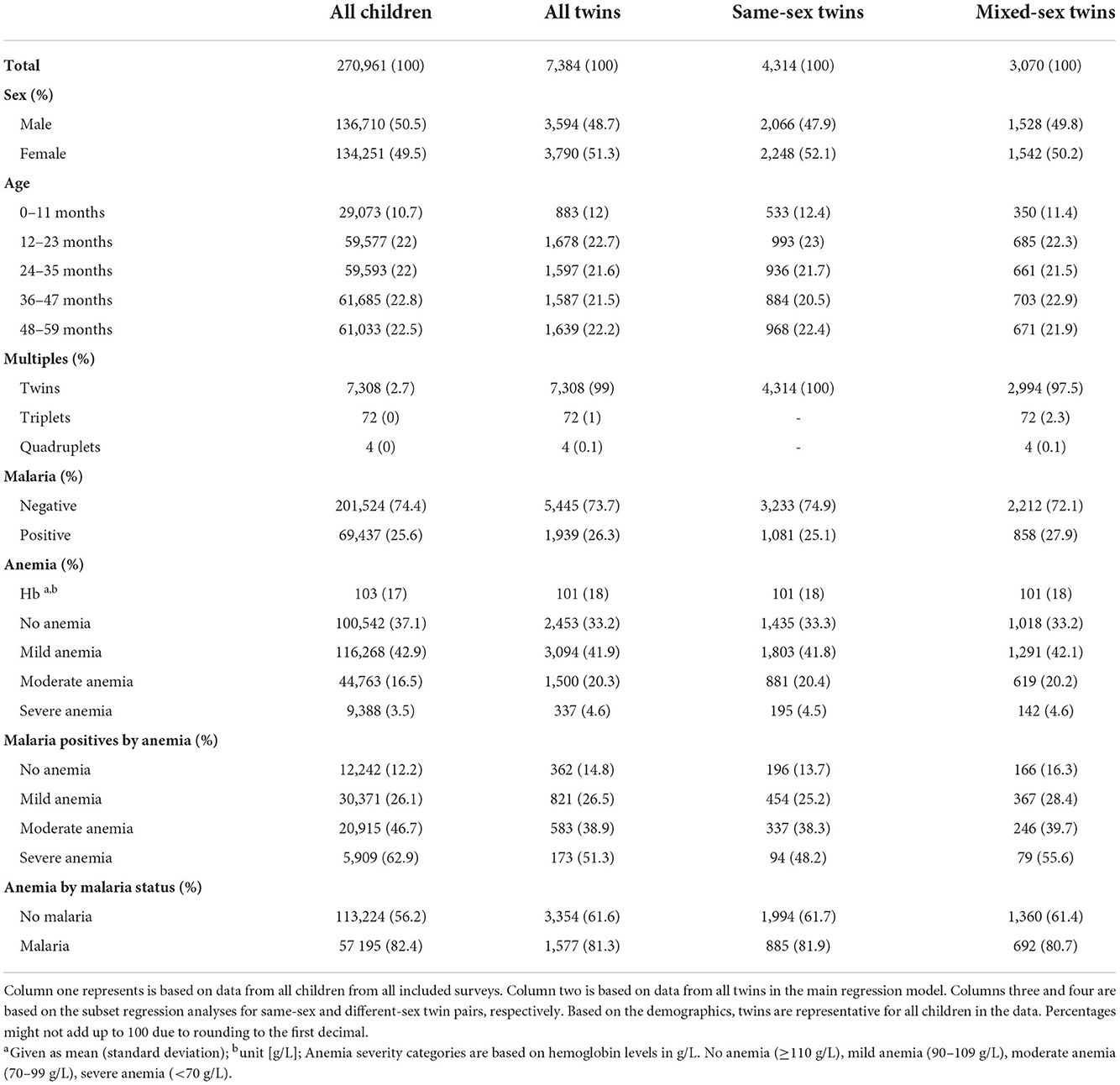
Table 1. Demographic and health characteristics of the study populations, separately for each level of analysis.
The main model, adjusted for sex and the twin fixed effect, showed a 9 g/L (95% CI -10; -7, p<0.001) hemoglobin decrease in children with positive RDT compared to their RDT-negative twins. Female sex increased hemoglobin by 2 g/L (95% CI 1; 3, p<0.001). The main model outcome and the outcomes for each separate country analysis are illustrated in Figure 3, the age subset analysis is appended to the Supplementary materials S4, S8.
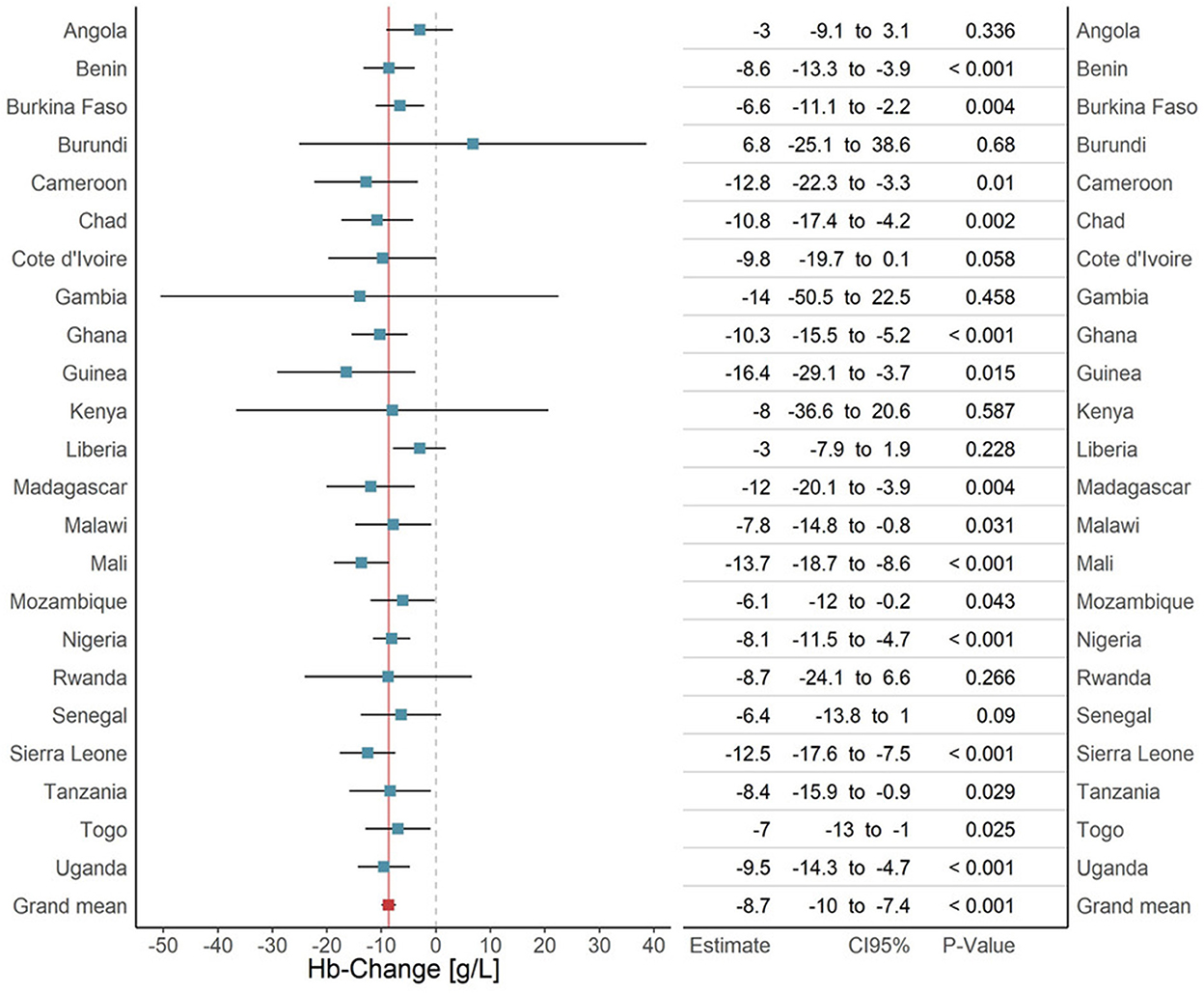
Figure 3. Analysis of the malaria effect on hemoglobin, overall effect and country specific effects. Each bar represents the result of a subset-analysis that used all surveys of a given country (blue). The errorbars represent the corresponding 95% confidence intervals. The bottom row (red) shows the outcome of the main pooled analysis across all twins from all surveys in all countries. The continuous dark red vertical line is the extension of the main pooled analysis result. The dashed line is the line of no effect. The table to the right adds the exact regression results for every country with 95% confidence intervals and p-values.
We adjusted the second, the same-sex model, for the twin fixed effect only and found a malaria-induced hemoglobin decrease of 9 g/L (95% CI -11; -7, p<0.001). Based on the Weinberg rule we estimated that a third of the same-sex data set are monozygotic twins (Supplementary material S2). The monozygotic twins thus weigh more heavily in the same-sex model than in the main model data where the monozygotic twins likely represent around one fifth.
The third, the different-sex model, was adjusted for the twin fixed effect and sex and included only those twins who were definitely dizygotic. We found a 8 g/L (95% CI −10; −6, p<0.001) hemoglobin reduction induced by plasmodium presence among the different-sex twins. The effect of parasitemia in the different-sex model is thus marginally smaller than the effect of the main and same-sex model (Supplementary material S9).
Based on the main model we also conducted a quasi-poisson regression with anemia severity as binary outcomes. In this model series plasmodium positive children where at higher relative risk of mild anemia or worse (Hb <110 g/L), (RR = 1.28, 95% CI 1.2; 1.36, p<0.001), moderate anemia or worse (Hb <90 g/L), (RR = 1.76, 95% CI 1.49; 2.08, p<0.001) and severe anemia (Hb <70 g/L), (RR = 3.01, 95% CI 1.79; 5.1, p<0.001). At the same time, their “risk” of being healthy (Hb≥110) was reduced (RR = 0.51, 95% CI 0.43; 0.61, p<0.001). The outcomes of the quasi-poisson regression are illustrated in Supplementary material S10.
Discussion
Our models consistently demonstrated a malaria-induced reduction in hemoglobin when comparing plasmodium-infected children to their healthy twin counterparts and confirmed that malaria substantially impacts population-level hemoglobin. Plasmodium presence was associated with a 9 g/L hemoglobin decrease among all twins and among the same sex twins. Among the different-sex twins the parasitemia-associated hemoglobin decrease was marginally smaller. Since the anemia severity groups are distinguished by steps of 20 g/L hemoglobin, the effect sizes are large enough to be clinically relevant, particularly if considering that our calculations are based on cross-sectional data. This is also illustrated in the poisson regression, where children with parasitemia are only about half as likely to be non-anemic and three times more likely to be severely anemic than their plasmodium-negative peers. The slightly larger effect of the same-sex model in comparison to the different-sex model might point toward a relevant genetic predisposition, e.g., sickle cell anemia, G6PD-deficiency or thalaessaemias (13, 37). However, the hemoglobin difference between the groups is neither statistically significant nor clinically relevant.
To our knowledge, this is the first large scale twin study on the impact of malaria on anemia. It complements an extensive body of research on the clinical impact of malaria on individual, and, to a lesser extent, on population hemoglobin. Previous research has described the population impact in a wide range from -13 g/L (WHO, 2019 malaria report), to a marginal -1 g/L among adults in a regional setting in south-east Asia (38, 39). In vivo studies have described the course of anemia during a plasmodium infection in adults with a sharp initial drop of -15 g/L hemoglobin followed by a rapid recovery to a plateau of -5 g/L (40). Korenromp et al. found successful malaria control strategies to improve population hemoglobin in children by 7 g/L (41). Our point estimate is located at the center of the range described in the literature and we confidently attribute this to the strict control for confounding in our modeling approach. The sex-associated hemoglobin difference is not backed well by current literature. Normally, we would expect to see a difference at the onset of puberty, not in children younger than five. However, sex dependent differences in early childhood have been recently described by Fulgoni et al. and are in line with our previous findings in Burkina Faso (42, 43). The high prevalence of anemia among malaria-negative children is within the expected expected range given the regional circumstances and has been previously described in other large studies (4, 44, 45).
Our study benefits from several strong innovations. It draws data from a large, representative sample of children in SSA, the region where the greatest gains against malaria have been made and yet much remains to be done. Additionally, from this representative sample we identified a very large number of twins as a basis for our fixed effect statistical model, controlling for most relevant confounding that is shared between twins (25, 28).
Our study has several limitations. Firstly, the nature of cross-sectional data is vulnerable to certain types of bias. This includes survival bias, where children with severe anemia are likely treated in clinics to receive intensified medical care and therefore cannot be included in the surveys (20). We expect survival bias to modify our results toward a more conservative estimation. Furthermore, the manual construction of the twin identifier could possibly introduce classification bias, where a child with an absent or deceased twin sibling is not correctly identified as a twin.
Secondly, we decided to use RDT result as our indicator for malaria status, rather than microscopy although microscopy is considered the gold standard. We made this choice because the RDTs most effectively reflect the diagnostic situation in SSA. RDTs are used in most DHS surveys, are a staple in clinical settings of rural Africa where microscopy is frequently not feasible and have very similar sensitivity and specificity (46, 47). The downside of RDTs is that they are susceptible to false positives, particularly in the presence of rheumatoid factor and in latent malaria infections (48–50). Some RDTs have been shown to remain positive in children for up to 30 days even after an infection is under immune system or medical control (51). Since the included survey time span ranges from 2006–2019, the quality of deployed RDTs will likely have improved over the years (52). The reliance on RDTs that developed over time, the different test models and target-antigens used, pose a risk of systematic measurement erros that can't be fully addressed through the study design as the error rates might vary between surveys.
Thirdly, we were not able to exclusively identify monozygotic twins within the given data set. This is important because the genetic relationship of dizygotic twins is roughly that of regular siblings, therefore our main model does not control for genetic differences that might vary between twins and still cause anemia, e.g., sickle-cell anemia trait. However, the twin fixed-effect approach is superior to a mother- or household-fixed-effect approach because it controls for all temporal and regional covariates that are associated with the twin birth, including in-utero and perinatal exposures, the mother's education, location of birth, age of the child, early childhood exposures etc (26). Similarly, the models lack explicit control for coinfections and malnutrition which were not covered in the data but might differ between twins. However, since these potential confounders are likely not systematically linked to the malaria status, their impact on the final results should be limited. Nevertheless, future surveys will hopefully address these gaps in the data to enable a clearer picture on anemia in the study populations.
The burden of anemia and malaria to SSA countries is substantial (53). However, the combined risks of malaria and anemia put affected children in a double-jeopardy situation where those that survive the acute infection frequently suffer anemia with corresponding detriments to development and increased infectious disease susceptibility (54, 55). In the long run, this close interaction can perpetuate cycles of poverty where anemia diminishes a child's chances at future upward social mobility and their future offspring will therefore be exposed to poverty, malaria, and anemia again. Hence, successfully combating malaria reduces the burden of anemia and proffers critical secondary benefits for the health and development of children and, ultimately, the economic stability of their countries. These benefits do not seem to be as far out of reach anymore as a first malaria vaccine gives hope of improved success in decreasing the burden of malaria and secondary to that, the burden of anemia (31).
In conclusion, we were able to demonstrate the substantial, malaria-induced reduction of population hemoglobin in one of the largest studies of SSA twins to date, while rigorously controlling for almost all confounders that are relevant to anemia and malaria. Since malaria's impact on hemoglobin reflects up to the population level it can be reasonably assumed that malaria delays development of individuals, regions, and entire countries. The work on eradicating malaria remains valuable, particularly those efforts that target the high risk group of young, anemic children. These programs should be extended rather than neglected, despite the current difficulties in the global political and health landscape.
Data availability statement
Publicly available datasets were analyzed in this study. This data can be found at: https://dhsprogram.com/ (RRID:SCR_000905).
Author contributions
TS and TB designed the study. TS performed the data analysis and drafted the manuscript with support of CB. All co-authors contributed significantly to the revision of the manuscript and provided scientific guidance. All authors contributed to the article and approved the submitted version.
Funding
For the publication fee we acknowledge financial support by Deutsche Forschungsgemeinschaft within the funding programme Open Access Publikationskosten as well as by Heidelberg University and the Heidelberg Institute of Global Health.
Acknowledgments
We would kindly like to thank Prof. Till Bärnighausen for scientific advice and his support in refining the research question and methodology of this paper. We would further like to thank Prof. Daniel Haydon for his advice on the statistical methods and Barbara Helm for her constructive feedback in the writing process. The developers of the rdhs (OJ Watson, Jeff Eaton), feglm (Berge L, Krantz S, McDermott G) and plm (Y Croissant, G Millo) R packages have made our work substantially easier and less computing intensive. We would further like to extend our gratitude to the DHS program, the local survey organizers and the field workers for generating, processing and providing the data. Most importantly though, our thanks are owed to all participants of the DHS surveys that agreed to be part of the studies.
Conflict of interest
The authors declare that the research was conducted in the absence of any commercial or financial relationships that could be construed as a potential conflict of interest.
Publisher's note
All claims expressed in this article are solely those of the authors and do not necessarily represent those of their affiliated organizations, or those of the publisher, the editors and the reviewers. Any product that may be evaluated in this article, or claim that may be made by its manufacturer, is not guaranteed or endorsed by the publisher.
Supplementary material
The Supplementary Material for this article can be found online at: https://www.frontiersin.org/articles/10.3389/fpubh.2022.1009865/full#supplementary-material
References
1. Hotez PJ, Kamath A. Neglected tropical diseases in Sub-Saharan Africa: review of their prevalence, distribution, and disease burden. PLoS Negl Trop Dis. (2009) 3:e412. doi: 10.1371/journal.pntd.0000412
2. Balarajan Y, Ramakrishnan U, Ozaltin E, Shankar AH, Subramanian SV. Anaemia in low-income and middle-income countries. Lancet. (2011) 378:2123–35. doi: 10.1016/S0140-6736(10)62304-5
3. Snow RW, Craig M, Deichmann U, Marsh K. Estimating mortality, morbidity and disability due to malaria among africa's non-pregnant population. Bull World Health Organ. (1999) 77:624–40.
4. The World Bank. Prevalence of Anemia among Children (% of Children Ages 6-59 Months) - Sub-Saharan Africa (2022) [08.10.2022]. Available online at: https://data.worldbank.org/indicator/SH.ANM.CHLD.ZS?locations=ZG (accessed October 08, 2022).
5. WHO. Haemoglobin Concentrations for the Diagnosis of Anaemia and Assessment of Severity. Geneva: World Health Organization (2011). Available online at: http://www.who.int/vmnis/indicators/haemoglobin.pdf (accessed March 03, 2021).
6. Ekvall H. Malaria and anemia. Curr Opin Hematol. (2003) 10:108–14. doi: 10.1097/00062752-200303000-00002
7. Holding PA, Snow RW. Impact of plasmodium falciparum malaria on performance and learning: review of the evidence. Am J Trop Med Hyg. (2001) 64(1-2 Suppl):68–75. doi: 10.4269/ajtmh.2001.64.68
8. Sudfeld CR, McCoy DC, Danaei G, Fink G, Ezzati M, Andrews KG, et al. Linear growth and child development in low- and middle-income countries: a meta-analysis. Pediatrics. (2015) 135:e1266–75. doi: 10.1542/peds.2014-3111
9. Sachs J, Malaney P. The economic and social burden of malaria. Nature. (2002) 415:680–5. doi: 10.1038/415680a
10. Soliman AT, Al Dabbagh MM, Habboub AH, Adel A, Humaidy NA, Abushahin A. Linear growth in children with iron deficiency anemia before and after treatment. Journal of Tropical Pediatrics. (2009) 55:324–7. doi: 10.1093/tropej/fmp011
11. Prieto-Patron AZ VH, Fattore G, Sabatier M, Detzel P. Reducing the burden of iron deficiency anemia in cote D'ivoire through fortification. J Health Popul Nutr. (2020) 39:1. doi: 10.1186/s41043-020-0209-x
12. Petry N, Olofin I, Hurrell RF, Boy E, Wirth JP, Moursi M, et al. The proportion of anemia associated with iron deficiency in low, medium, and high human development index countries: a systematic analysis of national surveys. Nutrients. (2016) 8:1–17. doi: 10.3390/nu8110693
13. Muriuki JM, Mentzer AJ, Mitchell R, Webb EL, Etyang AO, Kyobutungi C, et al. Malaria is a cause of iron deficiency in African children. Nat Med. (2021) 27:653–8. doi: 10.1038/s41591-021-01238-4
15. Price RN, Simpson JA, Nosten F, Luxemburger C, Hkirjaroen L., ter Kuile F, et al. Factors contributing to anemia after uncomplicated falciparum malaria. Am J Trop Med Hyg. (2001) 65:614–22. doi: 10.4269/ajtmh.2001.65.614
16. Douglas NM, Lampah DA, Kenangalem E, Simpson JA, Poespoprodjo JR, Sugiarto P, et al. Major burden of severe anemia from non-falciparum malaria species in southern papua: a hospital-based surveillance study. PLoS Med. (2013) 10:e1001575. doi: 10.1371/journal.pmed.1001575
17. Phillips MA, Burrows JN, Manyando C, van Huijsduijnen RH, Van Voorhis WC, Wells TNC. Malaria. Nat Rev Dis Prim. (2017) 3:17050. doi: 10.1038/nrdp.2017.50
18. Ekvall H, Premji Z, Bennett S, Bjorkman A. Hemoglobin concentration in children in a malaria holoendemic area is determined by cumulated plasmodium falciparum parasite densities. Am J Trop Med Hyg. (2001) 64:58–66. doi: 10.4269/ajtmh.2001.64.58
19. Jaenisch T, Sazawal S, Dutta A, Deb S, Ramsan M, Sullivan DJ. Contributions of polyclonal malaria, gametocytemia, and pneumonia to infant severe anemia incidence in malaria hyperendemic pemba, Tanzania. Am J Trop Med Hyg. (2012) 86:925–30. doi: 10.4269/ajtmh.2012.11-0164
20. Ashley EA, Pyae Phyo A, Woodrow CJ. Malaria. Lancet. (2018) 391:1608–21. doi: 10.1016/s0140-6736(18)30324-6
21. McElroy PD, Ter Kuile FO, Lal AA, Bloland PB, Hawley WA, Oloo AJ, et al. Effect of plasmodium falciparum parasitemia density on hemoglobin concentrations among full-term, normal birth weight children in Western Kenya, Iv. The Asembo Bay Cohort Project. Am J Trop Med Hyg. (2000) 62:504–12. doi: 10.4269/ajtmh.2000.62.504
22. Battle KE, Lucas TCD, Nguyen M, Howes RE, Nandi AK, Twohig KA, et al. Mapping the global endemicity and clinical burden of Plasmodium Vivax, 2000–17: a spatial and temporal modelling study. Lancet. (2019) 394:332–43. doi: 10.1016/S0140-6736(19)31096-7
23. Weiss DJ, Lucas TCD, Nguyen M, Nandi AK, Bisanzio D, Battle KE, et al. Mapping the global prevalence, incidence, and mortality of plasmodium falciparum, 2000–17: a spatial and temporal modelling study. Lancet. (2019) 394:322–31. doi: 10.1016/S0140-6736(19)31097-9
25. Barnighausen T, Oldenburg C, Tugwell P, Bommer C, Ebert C, Barreto M, et al. Quasi-Experimental study designs series-paper 7: assessing the assumptions. J Clin Epidemiol. (2017) 89:53–66. doi: 10.1016/j.jclinepi.2017.02.017
26. Dobbs KR, Dent AE. Plasmodium malaria and antimalarial antibodies in the first year of life. Parasitology. (2016) 143:129–38. doi: 10.1017/S0031182015001626
27. Anekwe TD, Newell ML, Tanser F, Pillay D, Barnighausen T. The causal effect of childhood measles vaccination on educational attainment: a mother fixed-effects study in Rural South Africa. Vaccine. (2015) 33:5020–6. doi: 10.1016/j.vaccine.2015.04.072
28. Gunasekara FI, Richardson K, Carter K, Blakely T. Fixed effects analysis of repeated measures data. Int J Epidemiol. (2014) 43:264–9. doi: 10.1093/ije/dyt221
29. Fujiwara T, Kawachi I. Is Education causally related to better health? A twin fixed-effect study in the United States. Int J Epidemiol. (2009) 38:1310–22. doi: 10.1093/ije/dyp226
30. Datoo MS, Natama MH, Somé A, Traoré O, Rouamba T, Bellamy D, et al. Efficacy of a low-dose candidate malaria vaccine, R21 in adjuvant matrix-M, with seasonal administration to children in burkina faso: a randomised controlled trial. Lancet. (2021) 397:1809–18. doi: 10.1016/S0140-6736(21)00943-0
31. WHO. WHO Recommends Groundbreaking Malaria Vaccine for Children at Risk. Geneva: World Health Organization (2021). Available online at: https://www.who.int/news/item/06-10-2021-who-recommends-groundbreaking-malaria-vaccine-for-children-at-risk (accessed October 07, 2021).
32. ICF. The Dhs Program: Methodology: The DHS Program website. Funded by United StatesID. (2020) [updated 2020/11/242020/11/30]. Available online at: https://dhsprogram.com (accessed November 30, 2020).
33. Doolan DL, Dobano C, Baird JK. Acquired Immunity to Malaria. Clin Microbiol Rev. (2009) 22:13–36. doi: 10.1128/CMR.00025-08
34. WHO. Guidelines for the Treatment of Malaria. Geneva: World Health Organization, (2015). Available online at: https://www.who.int/publications/i/item/9789241549127 (accessed December 10, 2020).
35. Mathison BA, Pritt BS. Update on malaria diagnostics and test utilization. J Clin Microbiol. (2017) 55:2009–17. doi: 10.1128/JCM.02562-16
36. Fellman J, Eriksson AW. Weinberg's differential rule reconsidered. Hum Biol. (2006) 78:253–75. doi: 10.1353/hub.2006.0044
37. Elguero E, Delicat-Loembet LM, Rougeron V, Arnathau C, Roche B, Becquart P, et al. Malaria continues to select for sickle cell trait in central Africa. Proc Natl Acad Sci U S A. (2015) 112:7051–4. doi: 10.1073/pnas.1505665112
39. Kotepui M, Phunphuech B, Phiwklam N, Chupeerach C, Duangmano S. Effect of malarial infection on haematological parameters in population near thailand-myanmar border. Malar J. (2014) 13:218. doi: 10.1186/1475-2875-13-218
40. Bickerton Blackburn CR. Observations on the development of resistance to vivax malaria. Trans R Soc Trop Med Hyg. (1948) 42:117–62. doi: 10.1016/0035-9203(48)90020-0
41. Korenromp EL, Armstrong-Schellenberg JR, Williams BG, Nahlen BL, Snow RW. Impact of malaria control on childhood anemia in Africa – a quantitative review. Trop Med Int Health. (2004) 9:1050–65. doi: 10.1111/j.1365-3156.2004.01317.x
42. Fulgoni VL 3rd, Agarwal S, Kellogg MD, Lieberman HR. Establishing pediatric and adult rbc reference intervals with Nhanes data using piecewise regression. Am J Clin Pathol. (2019) 151:128–42. doi: 10.1093/ajcp/aqy116
43. Starck T, Bulstra CA, Tinto H, Rouamba T, Sie A, Jaenisch T, et al. The effect of malaria on haemoglobin concentrations: a nationally representative household fixed-effects study of 17,599 children under 5 years of age in Burkina Faso. Malar J. (2021) 20:416. doi: 10.1186/s12936-021-03948-zz
44. Safiri S, Kolahi A-A, Noori M, Nejadghaderi SA, Karamzad N, Bragazzi NL, et al. Burden of Anemia and its underlying causes in 204 countries and territories, 1990–2019: results from the global burden of disease study 2019. J Hematol Oncol. (2021) 14:185. doi: 10.1186/s13045-021-01202-2
45. Jonker FAM., te Poel E, Bates I, Boele van Hensbroek M. Anaemia, iron deficiency and susceptibility to infection in children in sub-saharan africa, guideline dilemmas. Br J Haematol. (2017) 177:878–83. doi: 10.1111/bjh.14593
46. Boyce MR, O'Meara WP. Use of malaria rdts in various health contexts across sub-saharan africa: a systematic review. BMC Public Health. (2017) 17:470. doi: 10.1186/s12889-017-4398-1
47. Bruxvoort KJ, Leurent B, Chandler CIR, Ansah EK, Baiden F, Bjorkman A, et al. The impact of introducing malaria rapid diagnostic tests on fever case management: a synthesis of ten studies from the act consortium. Am J Trop Med Hyg. (2017) 97:1170–9. doi: 10.4269/ajtmh.16-0955
48. Gatton ML, Ciketic S, Barnwell JW, Cheng Q, Chiodini PL, Incardona S, et al. An Assessment of false positive rates for malaria rapid diagnostic tests caused by non-plasmodium infectious agents and immunological factors. PLoS ONE. (2018) 13:e0197395. doi: 10.1371/journal.pone.0197395
49. Ayogu EE, Ukwe CV, Nna EO. Assessing the reliability of microscopy and rapid diagnostic tests in malaria diagnosis in areas with varying parasite density among older children and adult patients in Nigeria. J Postgrad Med. (2016) 62:150–6. doi: 10.4103/0022-3859.183167
50. Fançony C, Sebastião YV, Pires JE, Gamboa D, Nery SV. Performance of microscopy and rdts in the context of a malaria prevalence survey in angola: a comparison using pcr as the gold standard. Malaria j. (2013) 12:284. doi: 10.1186/1475-2875-12-284
51. Dalrymple U, Arambepola R, Gething PW, Cameron E. How long do rapid diagnostic tests remain positive after anti-malarial treatment? Malar J. (2018) 17:228. doi: 10.1186/s12936-018-2371-9
52. Cunningham J, Jones S, Gatton ML, Barnwell JW, Cheng Q, Chiodini PL, et al. A review of the who malaria rapid diagnostic test product testing programme (2008–2018): performance, procurement, and policy. Malar J. (2019) 18:387. doi: 10.1186/s12936-019-3028-z
53. Global R. and National incidence, prevalence, and years lived with disability for 310 diseases and injuries, 1990–2015: a systematic analysis for the global burden of disease study 2015. Lancet. (2016) 388:1545–602. doi: 10.1016/S0140-6736(16)31678-6
54. Oh TK, Song KH, Song IA. History of anemia and long-term mortality due to infection: a cohort study with 12 years follow-up in South Korea. BMC Infect Dis. (2021) 21:674. doi: 10.1186/s12879-021-06377-0
Keywords: twins, multiples, fixed-effect, Sub-Sahara Africa, hemoglobin, DHS, anemia-etiology, malaria
Citation: Starck T, Dambach P, Rouamba T, Tinto H, Osier F, Oldenburg CE, Adam M, Bärnighausen T, Jaenisch T and Bulstra CA (2022) The effect of malaria on childhood anemia in a quasi-experimental study of 7,384 twins from 23 Sub-Saharan African countries. Front. Public Health 10:1009865. doi: 10.3389/fpubh.2022.1009865
Received: 02 August 2022; Accepted: 18 October 2022;
Published: 06 December 2022.
Edited by:
Gisely Melo, Fundação de Medicina Tropical Doutor Heitor Vieira Dourado (FMT-HVD), BrazilReviewed by:
Jaishree Raman, National Institute of Communicable Diseases (NICD), South AfricaEvans Raballah, Masinde Muliro University of Science and Technology, Kenya
Copyright © 2022 Starck, Dambach, Rouamba, Tinto, Osier, Oldenburg, Adam, Bärnighausen, Jaenisch and Bulstra. This is an open-access article distributed under the terms of the Creative Commons Attribution License (CC BY). The use, distribution or reproduction in other forums is permitted, provided the original author(s) and the copyright owner(s) are credited and that the original publication in this journal is cited, in accordance with accepted academic practice. No use, distribution or reproduction is permitted which does not comply with these terms.
*Correspondence: Tim Starck, c3RhcmNrJiN4MDAwNDA7c3R1ZC51bmktaGVpZGVsYmVyZy5kZQ==
†Present address: Faith Osier, Department of Life Sciences, Imperial College London, London, United Kingdom