- 1Groupe de recherche en épidémiologie des zoonoses et santé publique (GREZOSP), Faculté de médecine vétérinaire, Université de Montréal, Saint-Hyacinthe, QC, Canada
- 2Faculté de médecine et des sciences de la santé, Université de Sherbrooke, Sherbrooke, QC, Canada
- 3Centre de recherche en santé publique de l'Université de Montréal et du CIUSSS du Centre-Sud-de-l'île-de-Montréal (CReSP), Montréal, QC, Canada
- 4Public Health Risk Sciences Division, National Microbiology Laboratory, Public Health Agency of Canada, St. Hyacinthe, QC, Canada
Objectives: With vector-borne diseases emerging across the globe, precipitated by climate change and other anthropogenic changes, it is critical for public health authorities to have well-designed surveillance strategies in place. Sentinel surveillance has been proposed as a cost-effective approach to surveillance in this context. However, spatial design of sentinel surveillance system has important impacts on surveillance outcomes, and careful selection of sentinel unit locations is therefore an essential component of planning.
Methods: A review of the available literature, based on the realist approach, was used to identify key decision issues for sentinel surveillance planning. Outcomes of the review were used to develop a decision tool, which was subsequently validated by experts in the field.
Results: The resulting decision tool provides a list of criteria which can be used to select sentinel unit locations. We illustrate its application using the case example of designing a national sentinel surveillance system for Lyme disease in Canada.
Conclusions: The decision tool provides researchers and public health authorities with a systematic, evidence-based approach for planning the spatial design of sentinel surveillance systems, taking into account the aims of the surveillance system and disease and/or context-specific considerations.
Background
The geographical distribution of vector-borne diseases (VBDs) is increasing all around the world; some VBD are re-emerging in areas where they had disappeared for some time (e.g., malaria in Asia) whilst others are appearing in new locations (e.g., West Nile Virus in North America) (1, 2). Factors including climate change and globalization have expedited the process of disease emergence, as they have created favorable conditions for these diseases to evolve (2). As these facilitating factors are impossible to control in a timely fashion to stop and reverse geographical expansion of VBDs, public health authorities must adapt their practices and act further down the line of disease emergence– in preventing the transmission of pathogens from vectors to human populations.
To implement timely and efficient against VBDs, public health authorities require surveillance systems which provide a defined spatio-temporal portrait of the disease and vectors on their territory, over a time period of sufficient length to assess trends and intervention outcomes. Concurrently, for surveillance system to stay sustainable, the surface area and granularity of the surveillance performed are limited by finite resources, requiring that specific areas be prioritized when the whole of the territory cannot be fully surveyed. This issue is further emphasized when disease prevalence increases. This phenomenon imposes additional stress on surveillance systems and resources, which may further restrict surveillance coverage of the study area e.g., passive tick surveillance in Canada, which was gradually reduced in endemic regions (3).
Sentinel surveillance offers the opportunity to target specific locations to inform about risk across larger study areas, thus reducing resources required by limiting sampling units and effort (3). Sentinels are a finite subunit of a population which are measured repeatedly through time. However, as the sample size is restricted, the sentinel units and their locations must be carefully chosen during the planning phase to effectively answer to surveillance objectives and avoid suboptimal use of resources or even inaccurate results. For instance, some locations may be better suited to following disease and pathogen trends, while others may be more effective at capturing early warnings of disease emergence. Furthermore, if vector surveillance is carried out in ecologically unsuitable environments, absence of vectors may falsely indicate low risk of VBDs across the surveillance zone.
In the context of VBDs, sentinel surveillance has been both successful and unsuccessful in monitoring disease risk for human populations. In some cases, the use of sentinel animals (e.g., chicken, horse, crow) has allowed for early detection of West Nile virus; however, this has not always been replicated and sentinels occasionally fail to emit a signal prior to the diagnosis of the first human cases (4–8). Dogs can also serve as effective sentinels to track the risk of Lyme disease (LD) in endemic regions, but research has shown that in non-endemic regions, canine seroprevalence is not a representative measure of the risk to humans in the context of emergence (9–11). These examples highlight the complexity of decision-making in sentinel surveillance for VBDs and the fact that although surveillance may work in a particular setting, the application of the same protocol may not be effective in another context.
One of the first decisions to be taken by public health authorities in establishing a sentinel surveillance system is to determine which type of sentinel unit will be used. We will define a sentinel unit as the statistical unit of the surveillance system associated with a known geographical location. As such, sentinel units can be diverse and include individual animals, animal herds, medical/veterinary clinics, physicians, laboratories, zoos, etc. To support researchers in choosing the right sentinel species, a framework has been previously generated (12). Once the type of sentinel unit has been chosen, it must be distributed spatially across the study zone. The importance of geographical location of the sentinel units for the effectiveness of the sentinel system has been highlighted in a previous framework (13). For sentinel surveillance of influenza, the WHO has established guidelines for selection of sentinel sites (14). However, such guidelines (or similar decision tools) are missing for sentinel surveillance of VBDs.
In Canada the emergence of Lyme disease is a public health priority (15, 16), and a national sentinel surveillance network for active acarological surveillance is being implemented. However, to ensure effective surveillance across a large study zone, a decision tool to support the selection of geographical locations of sentinel sites (from here on, this concept will be referred to as sentinel unit locations) should be utilized to ensure a systematic approach to surveillance system design; such an approach would ensure reproducibility of the surveillance design and homogeneity in the decision-making steps, encouraging comparability of results. In response to this problem, our research team previously conducted a scoping review to extract selection criteria used in choosing sentinel unit locations across different epidemiological contexts. As epidemiological context and surveillance objectives may influence spatial design of a sentinel surveillance system, we identified the need for a systematic approach to ensure key decision issues are addressed during the planning phases of the surveillance system.
The first aim of this study was to develop a decision aid tool to support the selection of sentinel unit locations, by identifying relevant criteria to consider for geographical distribution of sentinel units within the study zone. The second aim was to demonstrate the functionality of the decision tool by applying it to the design of a national sentinel surveillance system for emerging LD risk in Canada as a case study. Our research will support public health authorities in transparent decision-making for planning of sentinel surveillance of VBDs, allowing the integration of spatially explicit information in surveillance design (17).
Methods
Development of the decision tool
To identify the decisional requirements to include in the spatial design of a sentinel surveillance system, based on the context of the surveillance initiative, we carried out a review based on a realist approach. Realist reviews have been used in the past to develop the conceptual basis and operations requirements for surveillance frameworks in vector-borne diseases, as these are designed to gain an understanding of how complex programs work in different settings (18, 19). We adapted this approach to meet our review needs, to allow us to evaluate how different criteria for choosing sentinel site locations are used in different contexts.
A recent scoping review provided the scope and the exploratory background search for this current review (20). The database of articles built up during the scoping review was used for the purposive sampling steps, as the search strategy corresponded to the need of our review (20). The primary studies were appraised to extract key decision points related to sentinel surveillance planning. These findings were synthesized and integrated as foundational aspects of the decision tool. Full details of the realist-type approach are provided in the Supplementary material 1.
Planning a surveillance system is a complex problem which requires several important decisions. Firstly, the type of sentinel unit should be decided upon e.g., site where vector surveillance will take place or where animal herd will be positioned, or a medical/veterinary clinic. Our decision tool will provide insight into how to distribute sentinel units across the study zone. However determining the number of sentinel units which will form the sentinel surveillance system are beyond the scope of the tool. Public health authorities and researchers may decide on this point based on resources available, surveillance objectives, and disease situation.
Validation of the decision tool
To ensure the functional validity of our decision tool, 14 experts in public health surveillance of VBDs were contacted and asked to assess the tool for functionality, as done in previous methodological research (21). Experts were required to complete an individual web-based questionnaire, with the aim of assessing whether the proposed tool was relevant, complete, and self-explanatory. At the end of the questionnaire, text boxes were available for final comments and suggestions (Supplementary material 2). A total of six experts responded (43% response rate) and questionnaires were examined by the research team and the results were used to update and improve the decision tool. The final version includes findings from our literature analysis and modifications following the validation by experts.
Application of the tool: A case study
Lyme disease cases in Canada have shown a significant expansion during the 21st century; since its addition to the notifiable disease registry, the number of reported cases went from 144 cases reported in 2009 to close to 3,000 cases in 2021 (22). In response to this increasing risk, the Canadian Lyme Disease Research Network (CLyDRN) was created. As part of its research objectives, the CLyDRN had the mandate to build a sentinel surveillance system to provide comparable LD risk measures across the country, based on active surveillance of ticks. A sentinel approach was advocated as it allows for a feasible surveillance strategy across a vast study zone. The selection of criteria to guide final geographical location of sites for this LD sentinel surveillance system at a national level was used as a case study to illustrate the application of the decision tool in supporting selection of sentinel unit locations.
Results
Identification of a decision path and key decisions issues
In the previous scoping review, criteria were classified into six categories: past information, risk, environment, human population characteristics, distribution of sites, and logistics (20). This classification was kept as a skeleton for the decision tool and was used to identify key decisions that should be considered during sentinel site selection, to account for fundamental aspects of the epidemiological situation. The decision issues identified for each criterion, which constituted a key element of the tool were identified from the review (Table 1).
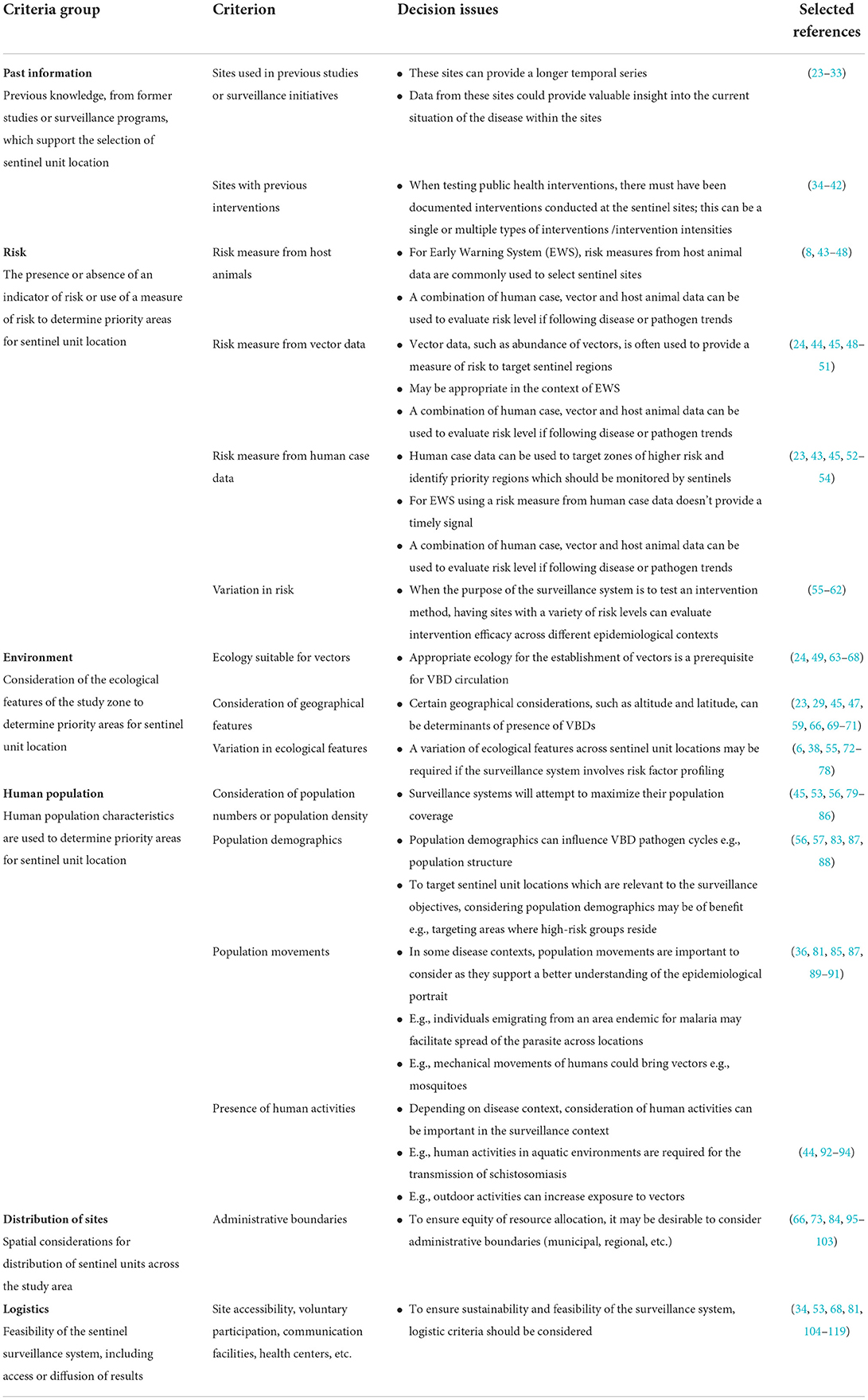
Table 1. Selection criteria for choosing sentinel unit locations within a surveillance system for vector-borne diseases; each criterion has associated decision issues which public health authorities or academics must consider in light of their surveillance context.
The starting point for the tool is to consider any previous studies (past information) or unit locations which have been used in the predetermined study area. We propose to include these sites as a starting basis if they have been used to answer objectives similar to those of the sentinel system being developed. This will contribute to a longer temporal sequence for surveillance and previously collected data could provide valuable insight into the current situation of the VBDs within the sites (23–33). Next, if the objective of the system will be to evaluate a public health intervention, it is important to know whether there have been previous interventions conducted within the sites and choose sites accordingly (34–42).
The next category of criteria to consider is risk-level, that is, the presence or absence of an indicator/measure of risk to determine priority areas for sentinel unit locations. Sentinel sites are often sampled using a risk-based approach targeting subgroups of a population where disease or the pathogen is more likely to be present (13). Many different types of sources of data can be used for evaluating risk to humans, e.g., using data from vectors, host animals, or human cases (23, 24, 43–45, 49, 52–54). Often, these can be integrated together to obtain an overall risk signal. Publicly available databases e.g., the Expanded Special Project for Elimination of Neglected tropical diseases (ESPEN) (120), can provide large scale risk data and can be used to understand the variation in risk across space. Early warning systems (EWS) constitute a special case for which human case data may not provide a signal in a timely manner and for which other data sources, such as host animal data should be prioritized; their use has been frequently reported in the literature and has resulted in sensitive surveillance systems (8, 43–48). Another valid alternative is vector data, including vector abundance or pathogen prevalence in vector populations. Data availability and accessibility may affect the selection of risk-based criteria.
Because environment plays such a crucial role in the transmission cycle of vector-borne pathogens, it constitutes an important category and criteria pertaining to it are involved in key decisions issues. Ecological suitability for presence of vectors and climatic conditions are predominant criteria (24, 49, 63–67). Larger variation in ecological features may allow for risk factor profiling (6, 38, 55, 72–78). For use of surveillance systems as EWS, we recommend that the selection criteria be orientated toward a risk-based measure, as opposed to environmental criteria, to improve specificity.
In public health surveillance, population-oriented approaches are advocated. To get the best representativeness, surveillance system will aim to maximize population coverage (45, 53, 56, 79–86). Other human population criteria which may be utilized by the researcher are dependent on the surveillance objectives and disease context (56, 57, 83, 87, 88). For instance, does the surveillance initiative target a particular population structure? Is population stability of key importance in the transmission cycle, as seen in lymphatic filariasis (36, 81, 85, 87, 89–91)? is the presence of certain human activities required for disease transmission, for instance human water reservoir contact for schistosomiasis (44, 92–94)?
In the distribution of site category the main criterion identified was equity of resources allocation for distribution of sentinel units across the study area. For instance, although risk may be concentrated in a particular area, it may be necessary to characterize and follow the risk in different areas. Administrative boundaries (e.g., municipal, county, or regional) can be used to ensure equity and presence of a sentinel unit in different or priority administrative sectors (66, 73, 84, 95–103).
Logistics criteria were incorporated as a decision step within the decision path to enhance the feasibility and sustainability of the system. This last group of criteria can also be used as a discriminatory feature to select between multiple potential sites which are equal in terms of the previous selection criteria. This group of criteria mostly deals with any logistical constraints related to the sentinel unit location including the need of voluntary participation, presence of specialist centers, stakeholder opinions, or adequate communication facilities (34, 53, 68, 81, 104–119).
A decision tool for sentinel surveillance of vector-borne diseases
Broad criteria categories were organized in a decision path to form a logical sequence of checkpoints and act as the tool for criteria selection. The user can follow each step of the path, however it may be used in an iterative manner. Within each step, key considerations identified through the review are presented as decision issues; these strategic questions can be answered by users during the planning process. Finally, the decision tool was assessed by experts and adjusted accordingly to ensure its validity (Figure 1). The functionality of the decision tool is demonstrated using a case study (section Sentinel surveillance for Lyme disease in Canada: A case study).
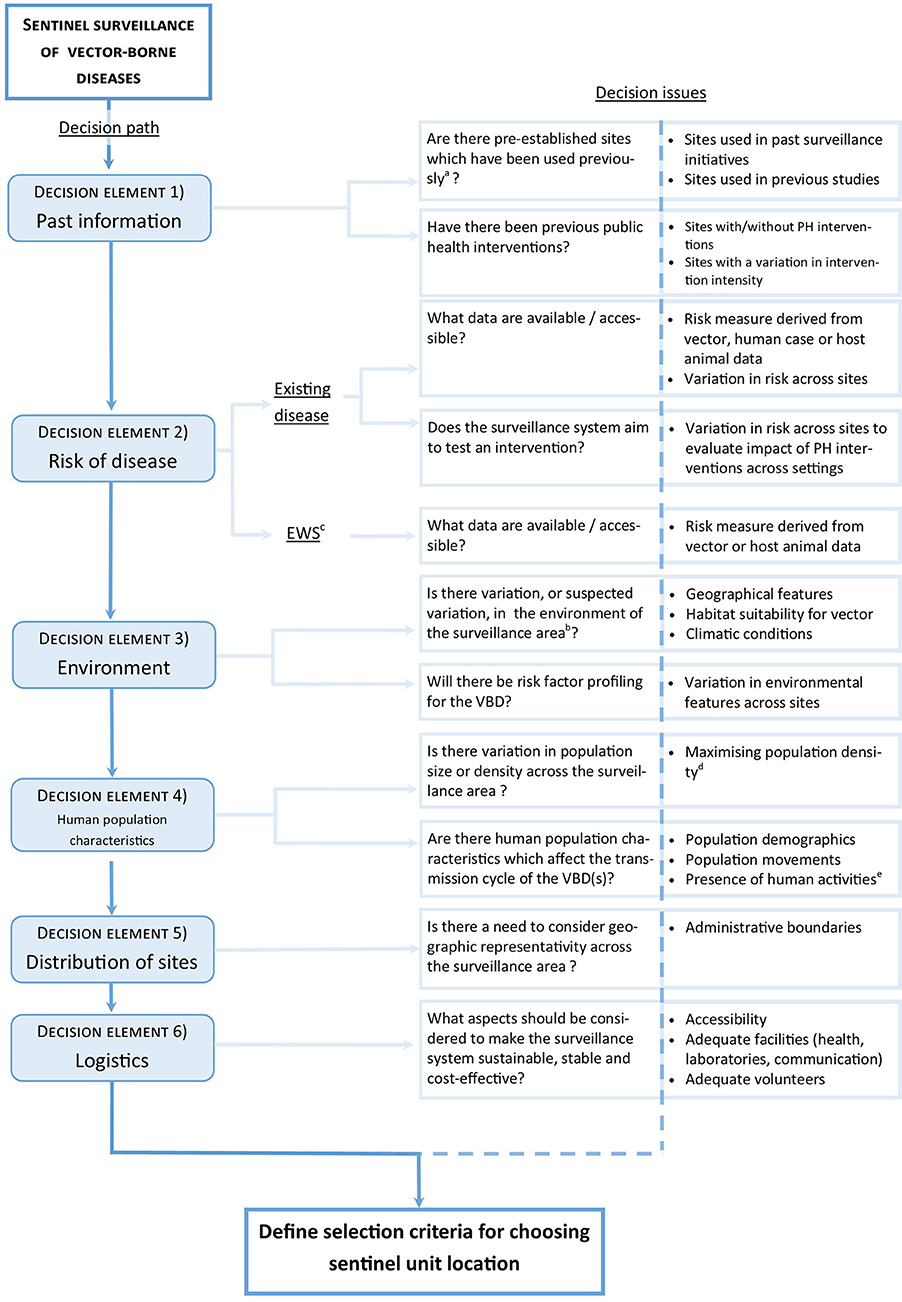
Figure 1. Decision tool for determining key criteria in developing a protocol for the selection of sentinel unit locations for vector-borne diseases. aSite should have been used for a similar objective. bThe variation in the environment is judged significant by the investigators. cEarly warning system. dIt is also relevant to consider potential important population influx e.g., from tourism, occupational reasons. eHuman activities which influence exposure to vectors/vector-borne diseases.
Sentinel surveillance for Lyme disease in Canada: A case study
The objective of the Canadian Lyme Sentinel Network (CaLSeN) is to follow spatiotemporal LD risk trends in Canada. Following the decision to build a sentinel surveillance network, the Surveillance Working Group began by deciding upon the basic network structure. The sentinel unit within the network will consist of a sentinel region, where active surveillance efforts (drag sampling for ticks) will be concentrated. Sentinel regions will consist of a 50 km radius-wide area in close proximity to a population center and will contain 5–10 individual sampling sites. LD risk is very different across provinces and to provide a comparative portrait of LD, at least one sentinel region will be selected in each province. This will ensure that all provinces are represented, meeting CLyDRN's mission statement. The number of sentinel regions will depend on the size of the province, which varies greatly, and on each province's capacity to carry out fieldwork (human resources). As part of the initial planning phases for the surveillance network, we should consider how the spatial design of the network will be constructed.
The decision tool was used to determine how sentinel regions will be distributed across Canada. The decision path was used (Figure 2). At the first checkpoint (past information), we considered if previous sites have been used in similar surveillance initiatives. Although there has been past active surveillance done in most of the Canadian provinces, there are no sentinel regions established for more intensive active surveillance initiatives. There are no planned public health interventions as part of the surveillance system. Thus, at this checkpoint, no criteria were retained. For the second checkpoint (risk), we are monitoring an existing disease, and the focus is not to act as an EWS but to provide a representative epidemiological portrait across Canada. Human case data are difficult to obtain, due to their sensitive nature, and we have resorted to passive tick submissions, available across the territory. Passive tick submissions have been determined to be a good signal for LD risk in human population in past studies (121). This was the only risk criterion retained, as currently no interventions are planned within the surveillance system. For the third checkpoint (environment), as we see important variations in environment-type within provinces, this criterion should be incorporated in the decision-making process. Furthermore, as climate change is an important factor for tick range expansion and tick population establishment, climate, in the form of temperature, was also retained. Risk factor profiling is not a primary aim of the surveillance system, hence variation in ecology was not kept as a criterion. For checkpoint 4 (human population characteristics), we wished to maximize the human population covered by the surveillance system i.e., we aimed to select sentinel regions with higher population density such as urban centers. We decided not to consider population characteristics, as access to demographic data at the municipal level across the whole of Canada posed challenges. Nonetheless, this could also be retained e.g., to consider populations with higher risk of exposure to black-legged ticks such as forest workers, indigenous communities, etc. (122, 123). For checkpoint 5 (distribution), the design of the sentinel system already determines how resources are allocated: we aim for at least one sentinel region per province. However, within each province, there is no need to consider administrative boundaries. Lastly, for checkpoint 6 (logistics), the main determinants of sustainability of the network are related to cost. Communication and laboratory facilities will not be impacted by the location of the sentinel region. Sampling material costs are not impacted by choice of sentinel region, but important variation travel costs and human resources will be associated with travel distance between CLyDRN collaborating centers and the sentinel region.
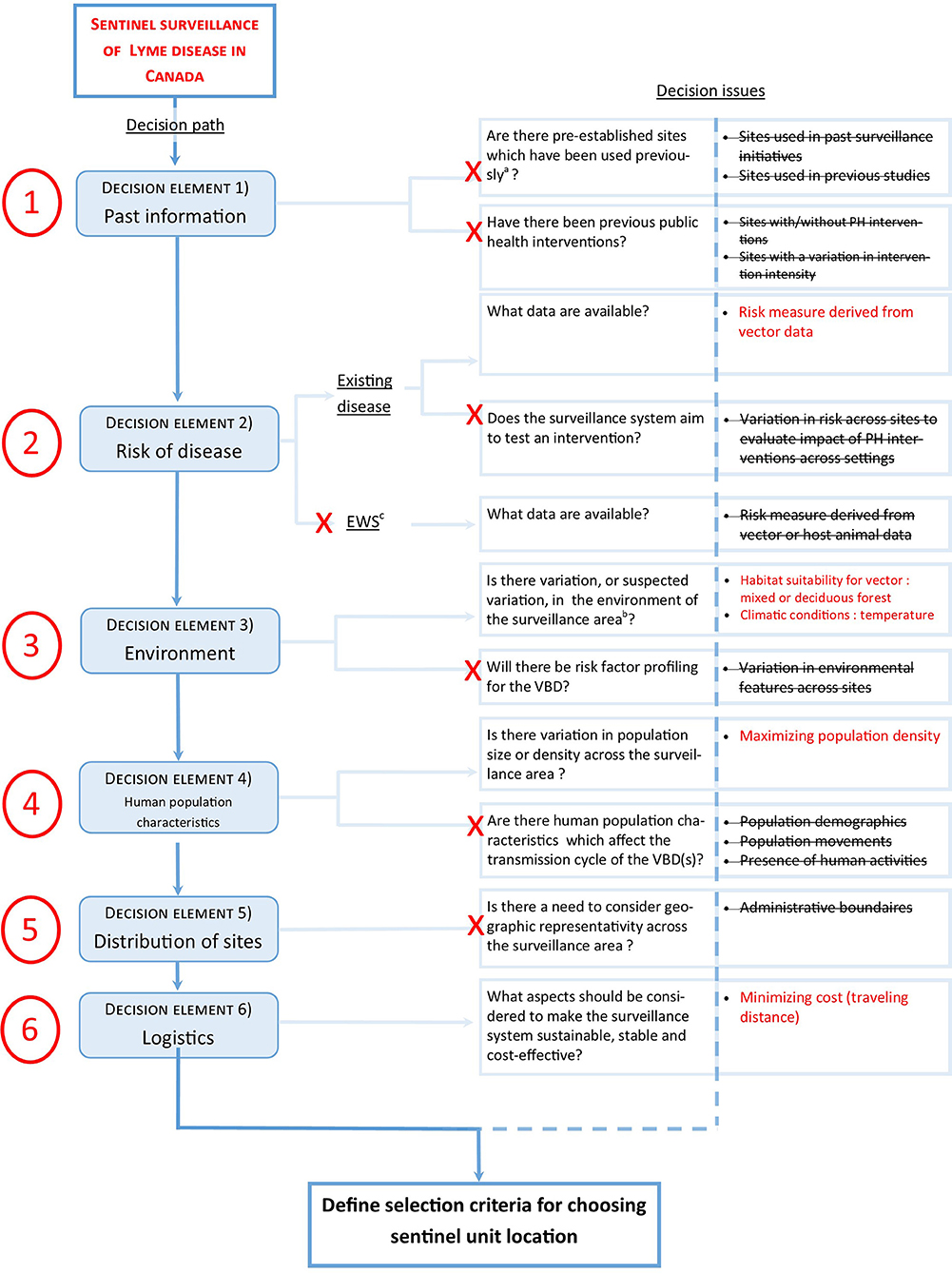
Figure 2. Demonstration of the functionality of the decision tool for determining key criteria for selecting spatial design for a national sentinel surveillance network for Lyme disease in Canada (case study). aSite should have been used for a similar objective. bThe variation in the environment is judged significant by the investigators. cEarly warning system.
Using the decision tool, a total of five criteria were retained (Table 2). These criteria can subsequently be used within a multi-criteria decision analysis (MCDA). The MCDA encourages the participation of multiple stakeholders and provides a transparent decision-making approach. Such an analysis is the object of ongoing work in the context of this case study.
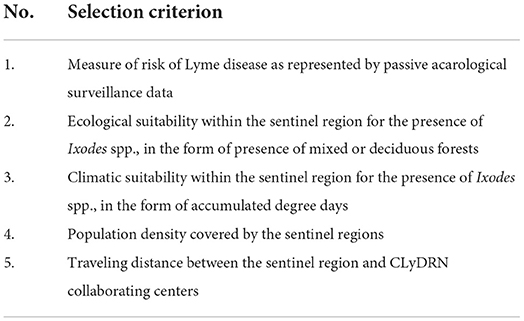
Table 2. Criteria to consider for planning the spatial design of a sentinel surveillance system for Lyme disease in Canada, retained after use of the decision tool.
Discussion
Our study has permitted the development of a new decision tool to guide spatial design of sentinel surveillance systems. As sentinels are a limited sample of a population, careful selection of sentinel unit location is essential for the system to be effective. Although such decision tools were available for other types of infectious diseases, it was not the case for VBDs (124). As VBDs require complex interactions between pathogens, vectors and animal hosts, risk distribution becomes heterogenous in space (125). Careful selection of sentinel location becomes even more crucial to ensure data from sentinel sites is representative of the epidemiological portrait across the study area.
Conducting a review of the material obtained from previous work (20) allowed the identification of key decisions issues. We based our decision tool development approach on previous papers dealing with VBDs (13, 19). Being based on a broad literature search, a strength of our review was the inclusivity of research papers, providing a thoughout insight into key decision issues to consider for elaborating the spatial design of VBD sentinel surveillance systems. Despite this inclusivity, it is important to note that many papers in the literature do not explicit the decisional process behind selection of sentinel unit location; thus, these papers would have been excluded in the original scoping review database (20). Furthermore, some VBDs e.g., malaria, West Nile virus, are overrepresented (20). However, validation by experts working on different VBDs helped strengthen the decision path.
Vector-borne diseases represent a vast and heterogenous group of infectious diseases: their transmission cycles are complex and vary considerably from one disease to another. By keeping the scope of the decision tool wide, it is amenable to various VBDs but it could mean that some of the criteria suggested by the tool may not be relevant the specific disease or pathogen under surveillance. For instance, some VBDs do not rely on animal reservoirs e.g., malaria (126), whilst that others, such as West Nile Virus or LD, depend greatly on animal reservoirs to persist in the environment (127). Some criteria provided by the decision tool may be too broad for application and should be refined appropriately e.g., climatic conditions, habitat suitability. We acknowledge that to maximize the utility of the tool, users must have expertise in the field of the VBD under surveillance and also, knowledge about surveillance systems. Nonetheless, we believe the decision issues can be regarded as transversal: public health authorities or academics should follow the decision path regardless of the VBD(s) they are planning on surveying; this will ensure that key decision issues are not overlooked. The user must keep an open mind and flexible approach and use the tool as an aid as opposed to a strict procedural algorithm. The tool may be used in an iterative manner.
The inclusion of a vast scope of the literature has allowed the development of a decision tool that is not only adaptable to VBDs but also different contexts and surveillance objectives. However, the assessment of how the stage of the disease's emergence process may impact decision issues was not a focus of our realist-type review. The surveillance strategy may indeed diverge depending on whether a disease is absent, emerging or endemic. Although this could have an impact on sentinel unit locations, we would recommend first to evaluate the relevance of using a sentinel surveillance approach. Clow et al. (19) have developed a framework for adapting surveillance approaches across different stages of the emergence process. Such frameworks are complementary to our work and should be used conjointly during the planning phases of the surveillance systems.
In planning a public health surveillance system, the surveillance objectives should be decided on initially as these will have an impact on the system structure (128, 129). Using sentinel surveillance as an EWS can be a difficult endeavor; due to restricted sampling, sentinel surveillance has more often been used for monitoring temporal changes in frequently occurring diseases/pathogens or to detect disease outbreaks (129). Indeed, from our review, a very small proportion of studies had the aim of acting as an EWS (20); therefore, we recommend that the tool be used with caution if the aim of the sentinel surveillance system is to act as an EWS. In this case, we advise that the decision tool could be used alongside literature dealing with sentinel surveillance as EWS, specific to the VBD under investigation (13, 110, 130).
The functionality of the decision tool was demonstrated using our case example of building a new sentinel surveillance system for LD in Canada. Using the decision tool, we believe we were able to extract all relevant decision issues related to our case study. A total of five different criteria were retained from the decision tool (Table 2). Further use of the decision tool will contribute to validating its functionality, especially in differing contexts e.g., in developing countries, where access to data and research realities may be very different to the case study presented.
Although the tool does not integrate the relative importance of each criterion, additional processes can easily overcome this limitation. For example, MCDA have been used to address complex problems relating to vector-borne diseases, such as the development of intervention plans, where multiple and conflicting criteria are applied (17, 131). MCDA has also been used to map out risk areas for infectious diseases, such as avian influenza (132); we suggest that a similar approach could be utilized, in conjunction with criteria obtained from our decision tool, to identify sentinel locations. Indeed, MCDA is an inclusive, transparent, and systematic approach for incorporating different levels of information and could be used to integrate retained criteria from the decision tool in a practical manner.
Our decision tool has consolidated information from global VBD sentinel surveillance systems worldwide and channeled it into a methodical diagram which can aid in the selection process of sentinel unit locations in versatile circumstances. The selected criteria can be integrated in an MCDA model, allowing a participative approach with stakeholders concerned by the surveillance issue. In the future, the use of the decision tool in the establishment of sentinel surveillance systems for VBDs should be evaluated to demonstrate its operational strengths and limitations; new surveillance systems created with the support of this decision tool will require evaluation to provide additional insight into spatial design of sentinel surveillance for VBD for optimization of the decision tool.
Data availability statement
The original contributions presented in the study are included in the article/Supplementary material, further inquiries can be directed to the corresponding author/s.
Author contributions
CG: conceptualization, methodology, interpretation, writing original draft, review, and editing. CB and PL: supervision, conceptualization, methodology, interpretation, review, and editing. CA: conceptualization, methodology, interpretation, review, and editing. PB: interpretation, review, and editing. FM: supervision, review, and editing. All authors contributed to the article and approved the submitted version.
Funding
This work was supported by the Canadian Lyme Disease Research Network (CLyDRN), supported by the Canadian Institutes of Health Research (CIHR), and the Faculty of Veterinary Medicine, Université de Montréal, Québec, Canada.
Acknowledgments
We would like to thank Mariola Mascarenhas, Caroline Sauvé, and Carol-Anne Villeneuve for their contribution in the scoping review. Furthermore, thanks to the Public Health Library of Public Health Agency of Canada and Katherine Merucci for their support in conducting our literature search.
Conflict of interest
The authors declare that the research was conducted in the absence of any commercial or financial relationships that could be construed as a potential conflict of interest.
Publisher's note
All claims expressed in this article are solely those of the authors and do not necessarily represent those of their affiliated organizations, or those of the publisher, the editors and the reviewers. Any product that may be evaluated in this article, or claim that may be made by its manufacturer, is not guaranteed or endorsed by the publisher.
Supplementary material
The Supplementary Material for this article can be found online at: https://www.frontiersin.org/articles/10.3389/fpubh.2022.1003949/full#supplementary-material
References
1. Savic S, Potkonjak A, Zekic-Stosic M, Petrovic T. Chapter 4 - The emergence of vector-borne diseases in new locations. In:Roig B, Weiss K, Thireau V, editors. Management of Emerging Public Health Issues and Risks. Cambridge, MA: Academic Press (2019), p. 89–99. doi: 10.1016/B978-0-12-813290-6.00004-4
2. Watson TG, Anderson RC. Ixodes scapularis say on white-tailed deer (Odocoileus virginianus) from Long Point, Ontario. J Wildl Dis. (1976) 12:66–71. doi: 10.7589/0090-3558-12.1.66
3. Gilbert R, Cliffe SJ. Public health surveillance. In:Regmi k, Gee I, editors. Public Health Intelligence: Issues of Measure and Method. New York, NY: Springer (2016), p. 91–110. doi: 10.1007/978-3-319-28326-5_5
4. McCluskey BJ. Use of sentinel herds in monitoring and surveillance systems. In:Salman MD, editor. Animal Disease Surveillance and Survey Systems. Hoboken, NJ: Wiley (2008), p. 119–33. doi: 10.1002/9780470344866.ch8
5. Rizzoli A, Rosà R, Rosso F, Buckley A, Gould E. West Nile virus circulation detected in northern Italy in sentinel chickens. Vector Borne Zoonotic Dis. (2007) 7:411–7. doi: 10.1089/vbz.2006.0626
6. Kwan JL, Kluh S, Madon MB, Nguyen DV, Barker CM, Reisen WK. Sentinel chicken seroconversions track tangential transmission of West Nile virus to humans in the greater Los Angeles area of California. Am J Trop Med Hyg. (2010) 83:1137–45. doi: 10.4269/ajtmh.2010.10-0078
7. Eidson M, Komar N, Sorhage F, Nelson R, Talbot T, Mostashari F, et al. Crow deaths as a sentinel surveillance system for West Nile virus in the northeastern United States, 1999. Emerg Infect Dis. (2001) 7:615–20. doi: 10.3201/eid0704.017402
8. Petrović T, Šekler M, Petrić D, Lazić S, Debeljak Z, Vidanović D, et al. Methodology and results of integrated WNV surveillance programmes in Serbia. PLoS ONE. (2018) 13:e0195439. doi: 10.1371/journal.pone.0195439
9. Duncan AW, Correa MT, Levine JF, Breitschwerdt EB. The dog as a sentinel for human infection: prevalence of Borrelia burgdorferi C6 antibodies in dogs from southeastern and mid-Atlantic states. Vector Borne Zoonotic Dis. (2004) 4:221–9. doi: 10.1089/1530366042162542
10. Little SE, Heise SR, Blagburn BL, Callister SM, Mead PS. Lyme borreliosis in dogs and humans in the USA. Trends Parasitol. (2010) 26:213–8. doi: 10.1016/j.pt.2010.01.006
11. Millen K, Kugeler KJ, Hinckley AF, Lawaczeck EW, Mead PS. Elevated Lyme disease seroprevalence among dogs in a nonendemic county: harbinger or artifact? Vector Borne Zoonotic Dis. (2013) 13:340–1. doi: 10.1089/vbz.2012.1025
12. Halliday JEB, Meredith AL, Knobel DL, Shaw DJ, Bronsvoort BMC, Cleaveland S. A framework for evaluating animals as sentinels for infectious disease surveillance. J R Soc Interface. (2007) 4:973–84. doi: 10.1098/rsif.2007.0237
13. Racloz V, Griot C, Stärk KD. Sentinel surveillance systems with special focus on vector-borne diseases. Anim Health Res Rev. (2006) 7:71–9. doi: 10.1017/S1466252307001120
14. Susilarini NK, Sitorus M, Praptaningsih CY, Sampurno OD, Bratasena A, Mulyadi E, et al. Application of WHO's guideline for the selection of sentinel sites for hospital-based influenza surveillance in Indonesia. BMC Health Serv Res. (2014) 14:424. doi: 10.1186/1472-6963-14-424
15. Otten A, Fazil A, Chemeris A, Breadner P, Ng V. Prioritization of vector-borne diseases in Canada under current climate and projected climate change. Microb Risk Anal. (2020) 14:100089. doi: 10.1016/j.mran.2019.100089
16. Kulkarni MA, Berrang-Ford L, Buck PA, Drebot MA, Lindsay LR, Ogden NH. Major emerging vector-borne zoonotic diseases of public health importance in Canada. Emerg Microbes Infect. (2015) 4:e33. doi: 10.1038/emi.2015.33
17. Hongoh V, Hoen AG, Aenishaenslin C, Waaub J-P, Bélanger D, Michel P, et al. Spatially explicit multi-criteria decision analysis for managing vector-borne diseases. Int J Health Geogr. (2011) 10:70. doi: 10.1186/1476-072X-10-70
18. Pawson R, Greenhalgh T, Harvey G, Walshe K. Realist review–a new method of systematic review designed for complex policy interventions. J Health Serv Res Policy. (2005) 10:21–34. doi: 10.1258/1355819054308530
19. Clow KM, Leighton PA, Pearl DL, Jardine CM, A. framework for adaptive surveillance of emerging tick-borne zoonoses. One Health. (2019) 7:100083. doi: 10.1016/j.onehlt.2019.100083
20. Guillot C, Bouchard C, Berthiaume P, Mascarenhas M, Sauvé C, Villeneuve CA, et al. A portrait of sentinel surveillance networks for vector-borne diseases: a scoping review supporting sentinel network design. Vector Borne Zoonotic Dis. (2021) 21:827–38. doi: 10.1089/vbz.2021.0008
21. Aenishaenslin C, Häsler B, Ravel A, Parmley EJ, Mediouni S, Bennani H, et al. Evaluating the integration of one health in surveillance systems for antimicrobial use and resistance: a conceptual framework. Front Vet Sci. (2021) 8:611931. doi: 10.3389/fvets.2021.611931
22. Goverrnement of Canada. Lyme disease: Monitoring. Available online at: https://www.canada.ca/en/public-health/services/diseases/lyme-disease/surveillance-lyme-disease.html#a2 (accessed February 25, 2022).
23. Courtin F, Camara O, Camara M, Kagbadouno M, Bucheton B, Solano P, et al. Sleeping sickness in the historical focus of forested Guinea: update using a geographically based method. Parasite. (2019) 26:61. doi: 10.1051/parasite/2019061
24. Maquart M, Boyer S, Rakotoharinome VM, Ravaomanana J, Tantely ML, Heraud JM, et al. High prevalence of west nile virus in domestic birds and detection in 2 new mosquito species in Madagascar. PLoS ONE. (2016) 11:e0147589. doi: 10.1371/journal.pone.0147589
25. Kelen PTV, Downs JA, Burkett-Cadena ND, Ottendorfer CL, Hill K, Sickerman S, et al. Habitat associations of eastern equine encephalitis transmission in Walton County Florida. J Med Entomol. (2012) 49:746–56. doi: 10.1603/ME11224
26. Sserwanga A, Harris JC, Kigozi R, Menon M, Bukirwa H, Gasasira A, et al. Improved malaria case management through the implementation of a health facility-based sentinel site surveillance system in Uganda. PLoS ONE. (2011) 6:e16316. doi: 10.1371/journal.pone.0016316
27. Barrera R, MacKay A, Amador M, Vasquez J, Smith J, Díaz A, et al. Mosquito vectors of West Nile virus during an epizootic outbreak in Puerto Rico. J Med Entomol. (2010) 47:1185–95. doi: 10.1603/ME10038
28. Barrera R, Hunsperger E, Muñoz-Jordán JL, Amador M, Diaz A, Smith J, et al. First isolation of West Nile virus in the Caribbean. Am J Trop Med Hyg. (2008) 78:666–8. doi: 10.4269/ajtmh.2008.78.666
29. Barrera R, Torres N, Freier JE, Navarro JC, García CZ, Salas R, et al. Characterization of enzootic foci of Venezuelan equine encephalitis virus in western Venezuela. Vector Borne Zoonotic Dis. (2001) 1:219–30. doi: 10.1089/153036601753552585
30. Clavijo A, Munroe F, Zhou EM, Booth TF, Roblesky K. Incursion of bluetongue virus into the Okanagan Valley, British Columbia. Canad Vet J. (2000) 41:312–4.
31. Abu Elzein EME, Al-Afaleq AI, Mellor PS, El-Bashir AM, Hassanein MM. Study of Akabane infection in Saudi Arabia by the use of sentinel ruminants. J Comp Pathol. (1998) 119:473–8. doi: 10.1016/S0021-9975(98)80041-8
32. George TDS, Cybinski DH, Standfast HA, Gard GP, Della-Porta AJ. The isolation of five different viruses of the epizootic haemorrhagic disease of deer serogroup. Aust Vet J. (1983) 60:216–7. doi: 10.1111/j.1751-0813.1983.tb09587.x
33. Amerasinghe FP, Ariyasena TG. Survey of adult mosquitoes (diptera: culicidae) during irrigation development in the mahaweli project, Sri Lanka. J Med Entomol. (1991) 28:387–93. doi: 10.1093/jmedent/28.3.387
34. Botto C, Basañez M-G, Escalona M, Villamizar NJ, Noya-Alarcón O, Cortez J, et al. Evidence of suppression of onchocerciasis transmission in the Venezuelan Amazonian focus. Parasit Vectors. (2016) 9:40. doi: 10.1186/s13071-016-1313-z
35. Rodríguez-Pérez MA, Domínguez-Vázquez A, Unnasch TR, Hassan HK, Arredondo-Jiménez JI, Orozco-Algarra ME, et al. Interruption of transmission of onchocerca volvulus in the Southern Chiapas Focus, México. PLoS Negl Trop Dis. (2013) 7:e2133. doi: 10.1371/journal.pntd.0002133
36. Leang R, Barrette A, Bouth DM, Menard D, Abdur R, Duong S, et al. Efficacy of dihydroartemisinin-piperaquine for treatment of uncomplicated Plasmodium falciparum and Plasmodium vivax in Cambodia, 2008 to 2010. Antimicrob Agents Chemother. (2013) 57:818–26. doi: 10.1128/AAC.00686-12
37. Fonseca DM, Unlu I, Crepeau T, Farajollahi A, Healy SP, Bartlett-Healy K, et al. Area-wide management of Aedes albopictus. Part 2: gauging the efficacy of traditional integrated pest control measures against urban container mosquitoes. Pest Manag Sci. (2013) 69:1351–61. doi: 10.1002/ps.3511
38. Abuaku B, Duah N, Quaye L, Quashie N, Malm K, Bart-Plange C, et al. Therapeutic efficacy of artesunate-amodiaquine and artemether-lumefantrine combinations in the treatment of uncomplicated malaria in two ecological zones in Ghana. Malar J. (2016) 15:6. doi: 10.1186/s12936-015-1080-x
39. Richards FO, Eigege A, Miri ES, Kal A, Umaru J, Pam D, et al. Epidemiological and entomological evaluations after six years or more of mass drug administration for lymphatic filariasis elimination in Nigeria. PLoS Negl Trop Dis. (2011) 5:e1346. doi: 10.1371/journal.pntd.0001346
40. Boyd A, Won KY, McClintock SK, Donovan CV, Laney SJ, Williams SA, et al. A community-based study of factors associated with continuing transmission of lymphatic Filariasis in Leogane, Haiti. PLoS Negl Trop Dis. (2010) 4:e640. doi: 10.1371/journal.pntd.0000640
41. Rodríguez-Pérez MA, Unnasch TR, Domínguez-Vázquez A, Morales-Castro AL, Richards F Jr., Peña-Flores GP, et al. Lack of active Onchocerca volvulus transmission in the northern Chiapas focus of Mexico. Am J Trop Med Hyg. (2010) 83:15–20. doi: 10.4269/ajtmh.2010.09-0626
42. Ramzy RMR, Hafez ON, Gad AM, Faris R, Harb M, Buck AA, et al. Efficient assessment of filariasis endemicity by screening for filarial antigenaemia in a sentinel population. Trans R Soc Trop Med Hyg. (1994) 88:41–4. doi: 10.1016/0035-9203(94)90490-1
43. Healy JM, Reisen WK, Kramer VL, Fischer M, Lindsey NP, Nasci RS, et al. Comparison of the efficiency and cost of West Nile virus surveillance methods in California. Vector Borne Zoonotic Dis. (2015) 15:147–55. doi: 10.1089/vbz.2014.1689
44. Ruiz-Arrondo I, McMahon BJ, Hernández-Triana LM, Santibañez P, Portillo A, Oteo JA. Surveillance of mosquitoes (Diptera, Culicidae) in a Northern Central Region of Spain: implications for the medical community. Front Vet Sci. (2019) 6:86. doi: 10.3389/fvets.2019.00086
45. Yang K, Sun LP, Liang YS, Wu F, Li W, Zhang JF, et al. Schistosoma japonicum risk in Jiangsu province, People's Republic of China: identification of a spatio-temporal risk pattern along the Yangtze River. Geospat Health. (2013) 8:133–42. doi: 10.4081/gh.2013.61
46. Ortiz S, Ceballos MJ, González CR, Reyes C, Gómez V, García A, et al. Trypanosoma cruzi diversity in infected dogs from areas of the north coast of Chile. Vet Parasitol Reg Stud Reports. (2016) 5:42–7. doi: 10.1016/j.vprsr.2016.09.004
47. Luanda L, Mihu-Pintilie A, Anită D, Adriana A, Lecollinet S, Savuta G. West Nile Virus Reemergence in Romania: a serologic survey in host species. Vector Borne Zoonotic Dis. (2014) 14:330–7. doi: 10.1089/vbz.2013.1405
48. Lefrançois T, Blitvich BJ, Pradel J, Molia S, Vachiéry N, Martinez D. West Nile virus in Guadeloupe: introduction, spread, and decrease in circulation level: 2002-2005. Ann N Y Acad Sci. (2006) 1081:206–15. doi: 10.1196/annals.1373.025
49. Baldacchino F, Marcantonio M, Manica M, Marini G, Zorer R, Delucchi L, et al. Mapping of Aedes albopictus abundance at a local scale in Italy. Remote Sens. (2017) 9:749. doi: 10.3390/rs9070749
50. Cherenet T, Sani RA, Speybroeck N, Panandam JM, Nadzr S, Van den Bossche P, et al. comparative longitudinal study of bovine trypanosomiasis in tsetse-free and tsetse-infested zones of the Amhara Region, northwest Ethiopia. Vet Parasitol. (2006) 140:251–8. doi: 10.1016/j.vetpar.2006.04.004
51. Chaskopoulou A, Dovas CI, Chaintoutis SC, Kashefi J, Koehler P, Papanastassopoulou M. Detection and early warning of West Nile Virus circulation in Central Macedonia, Greece, using sentinel chickens and mosquitoes. Vector Borne Zoonotic Dis. (2013) 13:723–32. doi: 10.1089/vbz.2012.1176
52. Amdouni J, Monaco F, Portanti O, Sghaier S, Conte A, Hassine TB, et al. Detection of enzootic circulation of a new strain of West Nile virus lineage 1 in sentinel chickens in the north of Tunisia. Acta Trop. (2020) 202:105223. doi: 10.1016/j.actatropica.2019.105223
53. Rodriguez-Rodriguez D, Maraga S, Lorry L, Robinson LJ, Siba PM, Mueller I, et al. Repeated mosquito net distributions, improved treatment, and trends in malaria cases in sentinel health facilities in Papua New Guinea. Malar J. (2019) 18:364. doi: 10.1186/s12936-019-2993-6
54. Liu Z, Soe T, Zhao Y, Than A, Cho C, Aung P, et al. Geographical heterogeneity in prevalence of subclinical malaria infections at sentinel endemic sites of Myanmar. Parasit Vectors. (2019) 12:83. doi: 10.1186/s13071-019-3330-1
55. Mishra N, Singh JPN, Srivastava B, Arora U, Shah NK, Ghosh SK, et al. Monitoring antimalarial drug resistance in India via sentinel sites: outcomes and risk factors for treatment failure, 2009-2010. Bull World Health Organ. (2012) 90:895–904. doi: 10.2471/BLT.12.109124
56. El Amin SB, Malik EFM, Ahmed EDS, El Abadi EK, Mohamad TA. Efficacy of chloroquine and sulfadoxine/pyrimethamine mono-and combined therapy against falciparum malaria in Sudan. East Mediterr Health J. (2007) 13:25–34.
57. Regis L, Monteiro A, Melo-Santos M, Silveirajr J, Furtado A, Acioli R, et al. Developing new approaches for detecting and preventing Aedes aegypti population outbreaks: basis for surveillance, alert and control system. Mem Inst Oswaldo Cruz. (2008) 103:50–9. doi: 10.1590/S0074-02762008000100008
58. Elamin SB, Awad AI, Eltayeb IB, Elmardi KA, Hassan AH, Mohamed AO, et al. Descriptive study on the efficacy of artemether-lumefantrine in the treatment of uncomplicated Plasmodium falciparum malaria in Sudan. Eur J Clin Pharmacol. (2010) 66:231–7. doi: 10.1007/s00228-009-0750-4
59. Zhou G, Lee M-C, Githeko AK, Atieli HE, Yan G. Insecticide-treated net campaign and malaria transmission in Western Kenya: 2003-2015. Front Public Health. (2016) 4:153–153. doi: 10.3389/fpubh.2016.00153
60. Gray DJ, Thrift AP, Williams GM, Zheng F, Li Y-S, Guo J, et al. Five-year longitudinal assessment of the downstream impact on schistosomiasis transmission following closure of the Three Gorges Dam. PLoS Negl Trop Dis. (2012) 6:e1588. doi: 10.1371/journal.pntd.0001588
61. Zhao GM, Zhao Q, Jiang QW, Chen XY, Wang LY, Yuan HC. Surveillance for schistosomiasis japonica in China from 2000 to 2003. Acta Trop. (2005) 96:288–95. doi: 10.1016/j.actatropica.2005.07.023
62. Menard D, Ratsimbasoa A, Randrianarivelojosia M, Rabarijaona L-P, Raharimalala L, Domarle O, et al. Assessment of the efficacy of antimalarial drugs recommended by the National Malaria Control Programme in Madagascar: up-dated baseline data from randomized and multi-site clinical trials. Malar J. (2008) 7:55. doi: 10.1186/1475-2875-7-55
63. Ilboudo-Sanogo E, Tiono BA, Sagnon N, Cuzin Ouattara N, Nébié I, Sirima SB. Temporal dynamics of malaria transmission in two rural areas of Burkina Faso with two ecological differences. J Med Entomol. (2010) 47:618–24. doi: 10.1093/jmedent/47.4.618
64. Casati S, Racloz V, Delécolle JC, Kuhn M, Mathis A, Griot C, et al. An investigation on the Culicoides species composition at seven sites in southern Switzerland. Med Vet Entomol. (2009) 23:93–8. doi: 10.1111/j.1365-2915.2009.00803.x
65. Tohon ZB, Mainassara HB, Garba A, Mahamane AE, Bosqué-Oliva E, Ibrahim ML, et al. Controlling schistosomiasis: significant decrease of anaemia prevalence one year after a single dose of praziquantel in Nigerian schoolchildren. PLoS Negl Trop Dis. (2008) 2:e241. doi: 10.1371/journal.pntd.0000241
66. Racloz V, Presi P, Vounatsou P, Schwermer H, Casati S, Vanzetti T, et al. Use of mapping and statistical modeling for the prediction of Bluetongue occurrence in Switzerland based on vector biology. Vet Ital. (2007) 43:513–8.
67. Ompusunggu S, Hills SL, Maha MS, Moniaga VA, Susilarini NK, Widjaya A, et al. Confirmation of Japanese encephalitis as an endemic human disease through sentinel surveillance in Indonesia. Am J Trop Med Hyg. (2008) 79:963–70. doi: 10.4269/ajtmh.2008.79.963
68. Alba A, Allepuz A, Napp S, Soler M, Selga I, Aranda C, et al. Ecological surveillance for West Nile in Catalonia (Spain), learning from a five-year period of follow-up. Zoonoses Public Health. (2014) 61:181–91. doi: 10.1111/zph.12048
69. Sun L-P, Liang Y-S, Wu H-H, Tian Z-X, Dai J-R, Yang K, et al. A Google Earth-based surveillance system for schistosomiasis japonica implemented in the lower reaches of the Yangtze River, China. Parasit Vectors. (2011) 4:223. doi: 10.1186/1756-3305-4-223
70. Ford-Jones EL, Fearon M, Leber C, Dwight P, Myszak M, Cole B, et al. Human surveillance for West Nile virus infection in Ontario in 2000. Canad Med Assoc J. (2002) 166:29–35.
71. Jegal S, Jun H, Kim-Jeon M-D, Park SH, Ahn SK, Lee J, et al. Three-year surveillance of culicine mosquitoes (Diptera: Culicidae) for flavivirus infections in Incheon Metropolitan City and Hwaseong-si of Gyeonggi-do Province, Republic of Korea. Acta Trop. (2020) 202:105258. doi: 10.1016/j.actatropica.2019.105258
72. Seidahmed OM, Abdelmajed MA, Mustafa MS, Mnzava AP. Insecticide susceptibility status of the malaria vector anopheles arabiensis in Khartoum city, Sudan: differences between urban and periurban areas. East Mediterr Health J. (2012) 18:769–76. doi: 10.26719/2012.18.7.769
73. Dadzie SK, Chabi J, Asafu-Adjaye A, Owusu-Akrofi O, Baffoe-Wilmot A, Malm K. et al. Evaluation of piperonyl butoxide in enhancing the efficacy of pyrethroid insecticides against resistant Anopheles gambiae sl in Ghana. Malar J. (2017) 16:342. doi: 10.1186/s12936-017-1960-3
74. Pitzer JB, Byford RL, Vuong HB, Steiner RL, Creamer RJ, Caccamise DF. Potential vectors of West Nile virus in a semiarid environment: Doña Ana County, New Mexico. J Med Entomol. (2009) 46:1474–82. doi: 10.1603/033.046.0634
75. Lernout T, Cardinale E, Jego M, Desprès P, Collet L, Zumbo B, et al. Rift valley fever in humans and animals in Mayotte, an endemic situation? PLoS ONE. (2013) 8:e74192–e74192. doi: 10.1371/journal.pone.0074192
76. Moyou-Somo R, Essomba P, Songue E, Tchoubou NN, Ntambo A, Hiol HN, et al. A public private partnership to fight against malaria along the Chad-Cameroon pipeline corridor: I. Baseline data on socio-anthropological aspects, knowledge, attitudes and practices of the population concerning malaria. BMC Public Health. (2013) 13:1023. doi: 10.1186/1471-2458-13-1023
77. Ren Z, Wang D, Hwang J, Bennett A, Sturrock HJW, Ma A, et al. Spatial-temporal variation and primary ecological drivers of anopheles sinensis human biting rates in malaria epidemic-prone regions of China. PLoS ONE. (2015) 10:e0116932. doi: 10.1371/journal.pone.0116932
78. Möhlmann TWR, Wennergren U, Tälle M, Favia G, Damiani C, Bracchetti L, et al. Community analysis of the abundance and diversity of mosquito species (Diptera: Culicidae) in three European countries at different latitudes. Parasit Vectors. (2017) 10:510. doi: 10.1186/s13071-017-2481-1
79. Knope K, Doggett SL, Jansen CC, Johansen CA, Kurucz N, Feldman R, et al. Arboviral diseases and malaria in Australia, 2014–15: annual report of the National Arbovirus and Malaria Advisory Committee. Commun Dis Intell. (2019) 43:71. doi: 10.33321/cdi.2019.43.14
80. Mengistu B, Deribe K, Kebede F, Martindale S, Hassan M, Sime H, et al. The National Programme to eliminate lymphatic filariasis from Ethiopia. Ethiop Med J. (2017) 55:45–54.
81. Nyunt MH, Soe MT, Myint HW, Oo HW, Aye MM, Han SS, et al. Clinical and molecular surveillance of artemisinin resistant falciparum malaria in Myanmar (2009-2013). Malar J. (2017) 16:333. doi: 10.1186/s12936-017-1983-9
82. Knope K, Whelan P, Smith D, Johansen C, Moran R, Doggett S, et al. Arboviral diseases and malaria in Australia, 2010-11: annual report of the national arbovirus and malaria advisory committee. Commun Dis Intell Q Rep. (2013) 37:E1–20.
83. Teixeria MG, Lima Barreto M, Conceicao N, Costa M, Alves Ferreira LD, Morato V, Fernando Vasconcelos P, et al. Exposure to the risk of dengue virus infection in an urban setting: ecological versus individual heterogeneity. WHO Regional Office for South-East Asia (2007). Available online at: https://apps.who.int/iris/handle/10665/170454 (accessed October 17, 2022).
84. Koroma JB, Bangura MM, Hodges MH, Bah MS, Zhang Y, Bockarie MJ. Lymphatic filariasis mapping by Immunochromatographic Test cards and baseline microfilaria survey prior to mass drug administration in Sierra Leone. Parasit Vectors. (2012) 5:10. doi: 10.1186/1756-3305-5-10
85. Modi A, Gamit S, Jesalpura BS, Kurien G, Kosambiya JK. Reaching endpoints for lymphatic filariasis elimination- results from mass drug administration and nocturnal blood surveys, South Gujarat, India. PLoS Negl Trop Dis. (2017) 11:e0005476. doi: 10.1371/journal.pntd.0005476
86. Sanou AS, Dirlikov E, Sondo KA, Kagoné TS, Yameogo I, Sow HE, et al. Building laboratory-based arbovirus sentinel surveillance capacity during an ongoing dengue outbreak, Burkina Faso. 2017. Health Secur. (2018) 16(S1):S103–10. doi: 10.1089/hs.2018.0048
87. Msyamboza K, Ngwira B, Banda R, Mkwanda S, Brabin B. Sentinel surveillance of lymphatic filariasis, schistosomiasis soil transmitted helminths and malaria in rural southern Malawi. Malawi Med J. (2010) 22:12–4. doi: 10.4314/mmj.v22i1.55901
88. Kabula B, Tungu P, Matowo J, Kitau J, Mweya C, Emidi B, et al. Susceptibility status of malaria vectors to insecticides commonly used for malaria control in Tanzania. Trop Med Int Health. (2012) 17:742–50. doi: 10.1111/j.1365-3156.2012.02986.x
89. Ojha CR, Joshi B, Kc KP, Dumre SP, Yogi KK, Bhatta B, et al. Impact of mass drug administration for elimination of lymphatic filariasis in Nepal. PLoS Negl Trop Dis. (2017) 11:e0005788. doi: 10.1371/journal.pntd.0005788
90. Gunathilaka N, Abeyewickreme W, Hapugoda M, Wickremasinghe R. Determination of demographic, epidemiological, and socio-economic determinants and their potential impact on malaria transmission in Mannar and Trincomalee districts of Sri Lanka. Malar J. (2016) 15:330. doi: 10.1186/s12936-016-1390-7
91. Biritwum NK, Frempong KK, Verver S, Odoom S, Alomatu B, Asiedu O, et al. Progress towards lymphatic filariasis elimination in Ghana from 2000-2016: analysis of microfilaria prevalence data from 430 communities. PLoS Negl Trop Dis. (2019) 13:e0007115. doi: 10.1371/journal.pntd.0007115
92. Chu KY, Vanderburg JA, Klumpp RK. Transmission dynamics of miracidia of Schistosoma haematobium in the Volta Lake. Bull World Health Organ. (1981) 59:555–60.
93. Barnish G, Jordan P, Bartholomew RK, Grist E. Routine focal mollusciciding after chemotherapy to control Schistosoma mansoni in Cul de Sac valley, Saint Lucia. Trans R Soc Trop Med Hyg. (1982) 76:602–9. doi: 10.1016/0035-9203(82)90220-6
94. Jánová E. Emerging and threatening vector-borne zoonoses in the world and in Europe: a brief update. Pathog Glob Health. (2019) 113:49–57. doi: 10.1080/20477724.2019.1598127
95. Koroma JB, Sesay S, Conteh A, Koudou B, Paye J, Bah M, et al. Impact of five annual rounds of mass drug administration with ivermectin on onchocerciasis in Sierra Leone. Infect Dis Pov. (2018) 7:30. doi: 10.1186/s40249-018-0410-y
96. Kakolwa MA, Mahende MK, Ishengoma DS, Mandara CI, Ngasala B, Kamugisha E, et al. Efficacy and safety of artemisinin-based combination therapy, and molecular markers for artemisinin and piperaquine resistance in Mainland Tanzania. Malar J. (2018) 17:369. doi: 10.1186/s12936-018-2524-x
97. Coleman S, Dadzie SK, Seyoum A, Yihdego Y, Mumba P, Dengela D, et al. A reduction in malaria transmission intensity in Northern Ghana after 7 years of indoor residual spraying. Malar J. (2017) 16:324. doi: 10.1186/s12936-017-1971-0
98. Ouedraogo H, Drabo F, Zongo D, Bagayan M, Bamba I, Pima T, et al. Schistosomiasis in school-age children in Burkina Faso after a decade of preventive chemotherapy. Bull World Health Organ. (2016) 94:37–45. doi: 10.2471/BLT.15.161885
99. Hetzel MW, Morris H, Tarongka N, Barnadas C, Pulford J, Makita L, et al. Prevalence of malaria across Papua New Guinea after initial roll-out of insecticide-treated mosquito nets. Trop Med Int Health. (2015) 20:1745–55. doi: 10.1111/tmi.12616
100. Nana-Djeunga HC, Tchatchueng-Mbougua JB, Bopda J, Mbickmen-Tchana S, Elong-Kana N, Nnomzo'o E, et al. Mapping of bancroftian filariasis in Cameroon: prospects for elimination. PLoS Negl Trop Dis. (2015) 9:e0004001. doi: 10.1371/journal.pntd.0004001
101. Santman-Berends IM, Bartels CJ, van Schaik G, Stegeman JA, Vellema P. The increase in seroprevalence of bluetongue virus (BTV) serotype 8 infections and associated risk factors in Dutch dairy herds, in 2007. Vet Microbiol. (2010) 142:268–75. doi: 10.1016/j.vetmic.2009.10.026
102. Raeisi A, Ringwald P, Safa O, Shahbazi A, Ranjbar M, Keshavarz H, et al. Monitoring of the therapeutic efficacy of chloroquine for the treatment of uncomplicated, Plasmodium falciparum malaria in Iran. Ann Trop Med Parasitol. (2006) 100:11–6. doi: 10.1179/136485906X86220
103. Bangs MJ, Larasati RP, Corwin AL, Wuryadi S. Climatic factors associated with epidemic dengue in Palembang, Indonesia: implications of short-term meteorological events on virus transmission. Southeast Asian J Trop Med Public Health. (2006) 37:1103–16.
104. George TDS, A. sentinel herd system for the study of arbovirus infections in Australia and Papua-New Guinea. Vet Res Commun. (1980) 4:39–51. doi: 10.1007/BF02278479
105. Richards FO, Dung P, Kal A, Gerlong GY, Onyeka J, Sambo Y, et al. Significant decrease in the prevalence of Wuchereria bancrofti infection in anopheline mosquitoes following the addition of albendazole to annual, ivermectin-based, mass treatments in Nigeria. Ann Trop Med Parasitol. (2005) 99:155–64. doi: 10.1179/136485905X19838
106. De Liberato C, Frontoso R, Magliano A, Montemaggiori A, Autorino GL, Sala M, et al. Monitoring for the possible introduction of Crimean-Congo haemorrhagic fever virus in Italy based on tick sampling on migratory birds and serological survey of sheep flocks. Prev Vet Med. (2018) 149:47–52. doi: 10.1016/j.prevetmed.2017.10.014
107. Qian YJ, Li SZ, Xu J, Yang K, Huang YX, Cao ZG, et al. Potential schistosomiasis foci in China: a prospective study for schistosomiasis surveillance and response. Acta Trop. (2015) 141(Pt B):342–8. doi: 10.1016/j.actatropica.2013.08.017
108. Touch S, Hills S, Sokhal B, Samnang C, Sovann L, Khieu V, et al. Epidemiology and burden of disease from Japanese encephalitis in Cambodia: results from two years of sentinel surveillance. Trop Med Int Health. (2009) 14:1365–73. doi: 10.1111/j.1365-3156.2009.02380.x
109. Bett B, Irungu P, Nyamwaro SO, Murilla G, Kitala P, Gathuma J, et al. Estimation of tsetse challenge and its relationship with trypanosomosis incidence in cattle kept under pastoral production systems in Kenya. Vet Parasitol. (2008) 155:287–98. doi: 10.1016/j.vetpar.2008.05.028
110. Randrianasolo L, Raoelina Y, Ratsitorahina M, Ravolomanana L, Andriamandimby S, Heraud J-M, et al. Sentinel surveillance system for early outbreak detection in Madagascar. BMC Public Health. (2010) 10:31. doi: 10.1186/1471-2458-10-31
111. Richards FO, Emukah E, Graves PM, Nkwocha O, Nwankwo L, Rakers L, et al. Community-wide distribution of long-lasting insecticidal nets can halt transmission of lymphatic filariasis in southeastern Nigeria. Am J Trop Med Hyg. (2013) 89:578–87. doi: 10.4269/ajtmh.12-0775
112. Doherty RL, George TD, Carley JG. Arbovirus infections of sentinel cattle in Australia and New Guinea. Aust Vet J. (1973) 49:574–9. doi: 10.1111/j.1751-0813.1973.tb06737.x
113. Yukich JO, Butts J, Miles M, Berhane Y, Nahusenay H, Malone JL, et al. A description of malaria sentinel surveillance: a case study in Oromia Regional State, Ethiopia. Malar J. (2014) 13:88. doi: 10.1186/1475-2875-13-88
114. Vitale M, Lupone CD, Kenneson-Adams A, Ochoa RJ, Ordoñez T, Beltran-Ayala E, et al. A comparison of passive surveillance and active cluster-based surveillance for dengue fever in southern coastal Ecuador. BMC Public Health. (2020) 20:1065–1065. doi: 10.1186/s12889-020-09168-5
115. Sears D, Kigozi R, Mpimbaza A, Kakeeto S, Sserwanga A, Staedke SG, et al. Anti-malarial prescription practices among outpatients with laboratory-confirmed malaria in the setting of a health facility-based sentinel site surveillance system in Uganda. Malar J. (2013) 12:252. doi: 10.1186/1475-2875-12-252
116. Smith FD, Ballantyne R, Morgan ER, Wall R. Estimating Lyme disease risk using pet dogs as sentinels. Comp Immunol Microbiol Infect Dis. (2012) 35:163–7. doi: 10.1016/j.cimid.2011.12.009
117. Dunphy BM, Kovach KB, Gehrke EJ, Field EN, Rowley WA, Bartholomay LC, et al. Long-term surveillance defines spatial and temporal patterns implicating Culex tarsalis as the primary vector of West Nile virus. Sci Rep. (2019) 9:6637. doi: 10.1038/s41598-019-43246-y
118. Pultorak E, Nadler Y, Travis D, Glaser A, McNamara T, Mehta SD. Zoological institution participation in a West Nile Virus surveillance system: implications for public health. Public Health. (2011) 125:592–9. doi: 10.1016/j.puhe.2011.03.013
119. Septfons A, Goronflot T, Jaulhac B, Roussel V, De Martino S, Guerreiro S, et al. Epidemiology of Lyme borreliosis through two surveillance systems: the national Sentinelles GP network and the national hospital discharge database, France, 2005 to 2016. Euro Surveill. (2019) 24:1800134. doi: 10.2807/1560-7917.ES.2019.24.11.1800134
120. World Health Organization. Expanded Special Project for Elimination of Neglected Tropical Diseases. https://espen.afro.who.int/ (accessed August 12, 2022).
121. Ripoche M, Gasmi S, Adam-Poupart A, Koffi JK, Lindsay LR, Ludwig A, et al. Passive tick surveillance provides an accurate early signal of emerging lyme disease risk and human cases in Southern Canada. J Med Entomol. (2018) 55:1016–26. doi: 10.1093/jme/tjy030
122. De Keukeleire M, Robert A, Luyasu V, Kabamba B, Vanwambeke SO. Seroprevalence of Borrelia burgdorferi in Belgian forestry workers and associated risk factors. Parasit Vectors. (2018) 11:277. doi: 10.1186/s13071-018-2860-2
123. Tutt-Guérette MA, Yuan M, Szaroz D, McKinnon B, Kestens Y, Guillot C, et al. Modelling spatiotemporal patterns of lyme disease emergence in Québec. Int J Environ Res Public Health. (2021) 18:9669. doi: 10.20944/preprints202107.0692.v1
124. Hay AJ, McCauley JW. The WHO global influenza surveillance and response system (GISRS)-A future perspective. Influenza Other Respir Viruses. (2018) 12:551–57. doi: 10.1111/irv.12565
125. Campbell-Lendrum D, Manga L, Bagayoko M, Sommerfeld J. Climate change and vector-borne diseases: what are the implications for public health research and policy? Philos Trans R Soc Lond B Biol Sci. (2015) 370:20130552. doi: 10.1098/rstb.2013.0552
126. Centers for Disease Control and Prevention. Malaria: Biology. (2020). Available online at: https://www.cdc.gov/malaria/about/biology/index.html (accessed August 12, 2022).
127. Vidaña B, Busquets N, Napp S, Pérez-Ramírez E, Jiménez-Clavero M, Johnson N. The role of birds of prey in West Nile Virus epidemiology. Vaccines. (2020) 8:550. doi: 10.3390/vaccines8030550
128. Teutsch SM, Thacker SB. Planning a public health surveillance system. Epidemiol Bull. (1995) 16:1–6.
129. European Centre for Disease Prevention and Control. Data Quality Monitoring and Surveillance System Evaluation – A Handbook of Methods and Applications. Stockholm: ECDC (2014).
130. Mostashari F, Kulldorff M, Hartman JJ, Miller JR, Kulasekera V. Dead bird clusters as an early warning system for West Nile virus activity. Emerg Infect Dis. (2003) 9:641–6. doi: 10.3201/eid0906.020794
131. Aenishaenslin C, Hongoh V, Cissé H, Hoen A, Samoura K, Michel P, et al. Multi-criteria decision analysis as an innovative approach to managing zoonoses: results from a study on Lyme disease in Canada. BMC Public Health. (2013) 13:897. doi: 10.1186/1471-2458-13-897
Keywords: sentinel surveillance system, vector-borne disease (VBD), Lyme disease, decision tool, surveillance planning
Citation: Guillot C, Bouchard C, Aenishaenslin C, Berthiaume P, Milord F and Leighton PA (2022) Criteria for selecting sentinel unit locations in a surveillance system for vector-borne disease: A decision tool. Front. Public Health 10:1003949. doi: 10.3389/fpubh.2022.1003949
Received: 26 July 2022; Accepted: 10 October 2022;
Published: 10 November 2022.
Edited by:
Nuno Sepulveda, Warsaw University of Technology, PolandReviewed by:
Lara Ferrero Gómez, Universidade Jean Piaget de Cabo, Cape VerdeEmanuele Giorgi, Lancaster University, United Kingdom
Marieta Braks, National Institute for Public Health and the Environment, Netherlands
Copyright © 2022 Guillot, Bouchard, Aenishaenslin, Berthiaume, Milord and Leighton. This is an open-access article distributed under the terms of the Creative Commons Attribution License (CC BY). The use, distribution or reproduction in other forums is permitted, provided the original author(s) and the copyright owner(s) are credited and that the original publication in this journal is cited, in accordance with accepted academic practice. No use, distribution or reproduction is permitted which does not comply with these terms.
*Correspondence: Camille Guillot, Camille.guillot@umontreal.ca