- 1Department of Clinical Laboratory, Dermatology Hospital, Southern Medical University, Guangzhou, China
- 2Department of Clinical Laboratory, Shanghai Fourth People's Hospital Affiliated to Tongji University School of Medicine, Shanghai, China
- 3Guangzhou Key Laboratory for Sexually Transmitted Diseases Control, Guangzhou, China
The global incidence of genital Chlamydia trachomatis infection increased rapidly as the primary available treatment of C. trachomatis infection being the use of antibiotics. However, the development of antibiotics resistant stain and other treatment failures are often observed in patients. Consequently, novel therapeutics are urgently required. Rhein is a monomer derivative of anthraquinone compounds with an anti-infection activity. This study investigated the effects of rhein on treating C. trachomatis infection. Rhein showed significant inhibitory effects on the growth of C. trachomatis in multiple serovars of C. trachomatis, including D, E, F and L1, and in various host cells, including HeLa, McCoy and Vero. Rhein could not directly inactivate C. trachomatis but could inhibit the growth of C. trachomatis by regulating pathogen-host cell interactions. Combined with azithromycin, the inhibitory effect of rehin was synergistic both in vitro and in vivo. Together these findings suggest that rhein could be developed for the treatment of C. trachomatis infections.
Introduction
Chlamydia trachomatis (C. trachomatis), a Gram-negative bacterial pathogen, is a causative agent of sexually transmitted infections in humans. There are an estimated 127 million new cases of C. trachomatis genital infection annually worldwide (1). C. trachomatis is classified into 15 serovars, with serovars D to K causing urinary or genital tract infections and serovars L1 to L3 being associated with lymphogranuloma venereum (2, 3). The developmental cycle of C. trachomatis is unique and biphasic, featuring an infective, metabolically inactive, elementary body (EB) and a metabolically active, intracellular, reproductive reticular body (RB) (4). Many individuals infected with C. trachomatis are asymptomatic, but chlamydia infections can have serious consequences. Untreated or recurrent chlamydial urogenital infections can lead to severe complications such as pelvic inflammatory disease and infertility (5). Genital infection with C. trachomatis also facilitates other sexually transmitted infections such as HIV (6) and human papillomavirus (7, 8). Thus, the potential risk chlamydia poses to human health should not be underestimated.
Currently, 1 g azithromycin (AZM) or 100 mg doxycycline twice a day for 7 days is the unanimously recommended, first-line treatment for uncomplicated C. trachomatis infections of the urogenital tract in the United States (9), China (10, 11), Europe (12), and Australia (13). Nevertheless, antibiotic resistance or treatment failure is not uncommon in C. trachomatis infections (10, 14, 15). For example, AZM treatment failure has been reported in 5% to 23% of Chlamydia-positive men with non-gonococcal urethritis and women with cervicitis not at risk of reinfection (16). A partner-treatment study reported that 8% of women had persistent chlamydial infection despite stating they had no sexual contact after treatment (17). These treatment failures may be due to resistance in members of the genus C. trachomatis and persistent infection. The tet (M) gene confers resistance to tetracycline antibiotics, while mutations in the 23S rRNA gene confer resistance to macrolides (18–20). The rates of 23S rRNA gene mutations and the abundance of tet (M) in C. trachomatis were higher in a treatment-failure group than in a treatment-success group (21). Furthermore, following exposure to antimicrobial drugs at sub-inhibitory concentrations, C. trachomatis can transform into a surviving reticulate with an almost persistently quiescent metabolism, which further increases the resistance to antibiotics (22). The emergence of antibiotic resistance and treatment failure indicated the need to identify novel anti-chlamydial agents.
Phytochemicals have attracted attention in recent years because of their therapeutic potential against a wide variety of pathogenic microorganisms (23). Compounds extracted from biomaterials and phytochemicals include flavones (24), terpenoids (25), alkaloids (26), and essential oils (27), and many of these compounds have antimicrobial properties. In a previous study, rhubarb inhibited C. trachomatis infection (28). Rhein (4, 5-dihydroxyanthraquinone-2-carboxylic acid; Figure 1A) is a monomer primarily extracted from rhubarb (29, 30). This lipophilic, naturally occurring compound has numerous pharmacological properties, including antitumor, antioxidant, anti-inflammatory, antimicrobial, hepatoprotective, and nephroprotective activities (31). As an antimicrobial, rhein has effects against Staphylococcus aureus (32, 33), Pseudomonas aeruginosa (34), Porphyromonas gingivalis (35), and influenza virus (36), among others. In the current study, the effects of rhein on C. trachomatis replication in cell culture and in mice were investigated with the aim of determining whether rhein had potential as a novel therapeutic against C. trachomatis infections.
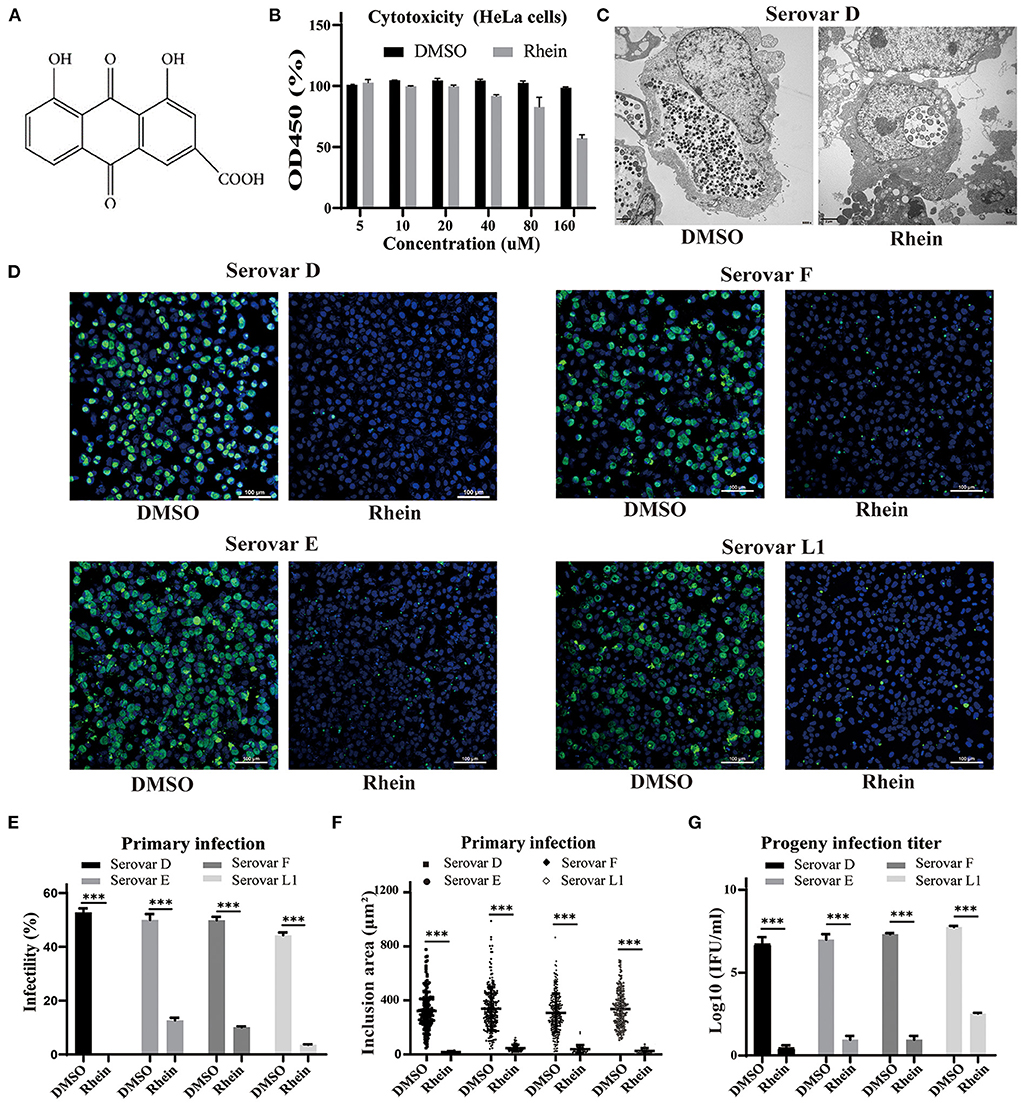
Figure 1. Rhein efficiently inhibited C. trachomatis replication. (A) Chemical structure of rhein. (B) Cytotoxic effect of rhein on HeLa cells assessed using a Cell Counting Kit-8. (C) Representative transmission electron micrographs of HeLa cells infected with C. trachomatis serovar D and exposed to 40 μm rhein or DMSO. Scale bars, 2 μm. (D) Immunofluorescent staining of HeLa cells infected with C. trachomatis serovars D, E, F, and L1 and exposed to 40 μM rhein for 48 h. C. trachomatis inclusions were stained with FITC-conjugated MOMP antibody (green) and nuclei were counterstained with DAPI (blue). Fluorescent images were captured on a confocal microscope at ×200 magnification. Scale bars, 100 μm. (E) Infectivity. (F) Inclusion area. (G) Infectious progeny titer. Data bars in b, e, and g represent the mean ± standard deviation. *p< 0.05, **p < 0.01, ***p< 0.001.
Materials and methods
Cell culture and C. trachomatis strains
Human epithelial carcinoma cells (HeLa) (ATCC CCL-2.1), mouse fibroblast cells (McCoy) (ATCC CTL-1696) provided by Dr. Lifang Jiang (Sun Yat-sen University, China) and African green monkey kidney cells (Vero) (CCTCC GDC062) were maintained in Dulbecco's modified Eagle's medium (Gibco, St. Louis, MO, USA) with 10% heat-inactivated fetal bovine serum (Gibco) at 37°C in an incubator supplied with 5% CO2 (Sanyo, Tokyo, Japan). C. trachomatis serovars D, E, F and L1 were provided by Dr. Joke Spaargaren (Public Health Laboratory of the Municipal Health Service of Amsterdam, Netherlands).
To obtain sufficient quantity of C. trachomatis, confluent HeLa cells were infected with C. trachomatis and centrifuged for 60 min at 1,000 × g. After centrifugation, the supernatants were replaced with 1 ml maintenance medium supplemented with 1.0 μg/ml cycloheximide (MedChemExpress, Monmouth Junction, NJ, USA). At 48 hpi, infected cells were sonicated and resuspended in sucrose–phosphate–glutamate. Stocks were divided into small aliquots and stored frozen at −70°C.
Compounds and drugs
Rhein (MedChemExpress) and AZM (North China Pharmaceutical Group Corporation, Hebei, China) were dissolved according to the manufacturers' instructions and stored at −70°C. DMSO (Sigma, St. Louis, USA) was stored at 4°C.
Cytotoxicity assays with rhein
Cytotoxicity of rhein in HeLa cells was assessed using a Cell Counting Kit-8 (CCK-8) (Dojindo, Tokyo, Japan) according to the manufacturer's instructions. Briefly, HeLa cells were seeded at 1 × 104 cells per well in 96-well plates and incubated overnight. Cell monolayers were exposed to various concentrations of rhein (0, 5, 10, 20, 40, 80, and 160 μM) for 48 h, then the CCK-8 kit was utilized, measuring the absorbance of the cells, and the results were expressed as percent viable cells.
Immunofluorescent staining
Confluent HeLa cells were centrifuged at 1,000 × g with C. trachomatis for 1 h and then placed at 37°C in an incubator supplied with 5% CO2 for 1 h. The medium was then changed to maintenance medium in the presence of 40 μM rhein or DMSO. Infected HeLa cells were cultured on cell slides for 48 h and fixed with 4% paraformaldehyde for 20 min at room temperature. Cells were permeabilized with 0.1% Triton X-100 for 20 min at room temperature and were then incubated with 1% bovine serum albumin in phosphate-buffered saline with Tween (PBS + 0.1% Tween 20) for 60 min to block non-specific binding of the antibodies. Cells were incubated with a fluorescein isothiocyanate (FITC)-conjugated antibody against the major outer membrane protein (MOMP) of C. trachomatis (Abcam, Cambridge, UK) and were then counterstained with DAPI (Solarbio, Beijing, China).
Confocal microscopy
Samples were examined under a confocal microscopy at ×200 magnification (Nikon, Tokyo, Japan) and the number of inclusions and nuclei were counted in 15 random fields from triplicate samples in each experiment. The number of inclusions and nuclei were used to assess infectivity by calculating the inclusion/nuclei percent with Nikon AR NIS 5.02.00 software. The software was also used to measure the area of inclusion bodies.
Electron microscopy
Infected HeLa cells were centrifuged at 1,000 × g for 1 h and then placed at 37°C in an incubator supplied with 5% CO2 for 1 h. The medium was then changed to maintenance medium in the presence of 40 μM rhein or DMSO. At 48 hpi, cells treated with rhein or DMSO were collected, pelleted by centrifugation at 1,000 × g for 5 min, and were then embedded and examined by transmission electron microscopy (Japan Electron Optics Laboratory, Tokyo, Japan).
Titer of infectious progeny assay
C. trachomatis-infected cells were collected and sonicated. EBs were released from the cells and used to infect fresh cell monolayers. Total inclusions were counted, and numbers of inclusion-forming units (IFU/ml) were calculated at 48 hpi.
Direct interaction assay
EBs of C. trachomatis were co-incubated with 40 μM rhein for 12, 24, 36, or 48 h at 4°C before infection (37); DMSO was used as a positive control. C. trachomatis was washed with PBS to remove the residual rhein and was then used to infect HeLa cells in 24-well plates. At 48 hpi, cells were fixed with paraformaldehyde and observed by confocal microscopy.
Influence-on-cell assay
HeLa cells were seeded in 24-well plates at 1 × 105 cells/well and 40 μM rhein was added to the culture medium and incubated for 24 h. Cell monolayers were washed three times with PBS, then the pretreated cells were infected with C. trachomatis; DMSO treatment served as a positive control. At 48 hpi, cells were stained with MOMP antibody and observed using confocal microscopy.
Influence-on-adsorption assay
HeLa cells were infected with C. trachomatis and simultaneously exposed to 40 μM rhein in the culture medium; a control group received the equivalent amount of DMSO. The culture plate was centrifuged at 1,500 × g for 1 h and then placed at 37°C in an incubator supplied with 5% CO2 for 1 h. The medium containing rhein was then discarded, and cells were washed with PBS three times before addition of the maintenance medium. Immunofluorescence staining was conducted at 48 hpi.
Western blotting
Treated cells were incubated for 0, 12, 24, 36, or 48 h, then the cellular proteins were lysed by RIPA (Invitrogen, 89900) supplemented with a protease and phosphatase inhibitor cocktail (Invitrogen, 78440), and incubated with SDS-PAGE loading buffer (Reducing) (Cwbio, CW0027) at 100°C for 10 min. Antibodies used for western blotting were as follows: anti-RSK1 p90 (phospho T359 + S363) antibody (1:1,000, ab32413, Abcam), anti-RSK1 p90 antibody (1:1,000, ab32114, Abcam), anti-Phospho-p44/42 MAPK (Erk1/2) (1:1,000, 4370S, Cell signaling), anti- p44/42 MAPK (Erk1/2) (1:1,000, 4695S, Cell signaling), anti-cHSP60 (1:2,000, sc-57840, Santa Cruz), anti-GAPDH (1:1,0000, ab181602, Abcam), anti-rabbit IgG-HRP-linked antibody (1:5,000, 7074S, Cell signaling), and anti-mouse IgG-HRP-linked antibody (1:5,000, 7076S, Cell signaling). Blots were imaged on a ChemiDoc MP Imaging System (Bio-Rad).
Animals
Female BALB/c mice (4–6-week-old) were purchased from the Southern Medical University (Guangzhou, China). At 10 and 7 days before infection, all mice were injected subcutaneously with 2.5 mg medroxyprogesterone acetate (Bayunshan Pharmaceutical Company, Guangzhou, China) to synchronize estrus (38). After treatment, the mice were vaginally infected with 1 × 107 C. trachomatis IFU or an equal volume of sucrose–phosphate–glutamate. Experiments were conducted in the Experimental Animal Center of South China Agricultural University and in accordance with the National Institutes of Health Guide for the Care and Use of Laboratory Animals. All procedures performed in studies involving experiments on animals were approved by the Ethics Committee of South China Agricultural University (SCAU, Guangzhou, China and approval number: 2020c035).
Drug treatment in vivo
Mice were divided into five groups: negative control, positive control, rhein treatment, AZM treatment and rhein + AZM combined treatment. Rhein was dissolved in DMSO at 10 mg/ml, and AZM was dissolved in ethanol at 0.084 mg/ml. Mice were treated with 120 mg/kg rhein, 1.0 mg/kg AZM or a combination of 120 mg/kg rhein and 1.0 mg/kg AZM in 0.5% carboxymethylcellulose sodium (CMC-Na) once daily by gavage from day 4 to day 10. Control mice were gavaged with 0.5% CMC-Na. Vaginal swabs were taken for cell culture on day 4 (before gavage), day 7 and day 10 after infection, and the number of inclusions were measured.
Statistical analyses
GraphPad Prism 8 (GraphPad Software, La Jolla, CA, USA) was used to generate graphs, and statistical analyses were conducted using SPSS 15.0 (SPSS Inc., Chicago, IL, USA). Quantitative data are presented as mean ± standard deviation. The Shapiro–Wilk test was used to test the normality of quantitative data. Fisher's exact test and Bonferroni's multiple comparisons were used to assess infectivity. Kruskal–Wallis and Dunn's multiple comparisons tests were used to evaluate the area of inclusions. An unpaired t-test was used to analyze the difference in EB titer between groups. P-values were calculated using one-way ANOVA followed by Bonferroni correction for multiple comparisons. A nonparametric Wilcoxon test was used for mouse model statistics. Differences were considered significant at P <0.05 (*), P <0.01 (**) and P <0.001 (***).
Results
Rhein effectively inhibited C. trachomatis replication
Cell viability was approximately 95% in samples exposed to 40 μM rhein (Figure 1B). The anti-chlamydial effects of rhein were investigated in HeLa cells infected with the more prevalent serovars of C. trachomatis (serovars D, E and F) and the L1 serovar that can lead to venereal lymphogranuloma (39). A few aberrant RBs were observed by transmission electron microscopy in HeLa cells infected with C. trachomatis serovar D and treated with 40 μM rhein, compared with many small, mature EB particles within the inclusion of DMSO-treated cells at 48 hpi (Figure 1C). Immunofluorescent staining revealed that the inclusion bodies became smaller and the infectivity, inclusion size and infectious progeny decreased in the presence of 40 μM rhein (Figures 1D–G). These results demonstrated that rhein effectively inhibited the growth and reproduction of different serovars of C. trachomatis in HeLa cells.
The effect of rhein on C. trachomatis was dose- and time-dependent
The effect of different concentrations of rhein (0, 5, 10, 20, 40, and 80 μM) on HeLa cells infected with C. trachomatis was examined, and the infectivity, inclusion area and infectious progeny are decreased in the presence of rhein in a dose-dependent manner (Figure 2A). HeLa cells infected with C. trachomatis were also exposed to 40 μM rhein at various time points (0, 6, 12, 18, and 24 h) after infection. C. trachomatis inclusions were larger and more numerous with rhein exposure at 24 hpi compared with rhein exposure at 0 hpi (Figure 2B). The titer of infectious progeny also increased with the delay in exposure to rhein (Figure 2B). These findings indicated that rhein inhibited the replication of C. trachomatis in a dose- and time-dependent manner, and suggested that the earlier cells are treated with rhein, the better the inhibition of C. trachomatis.
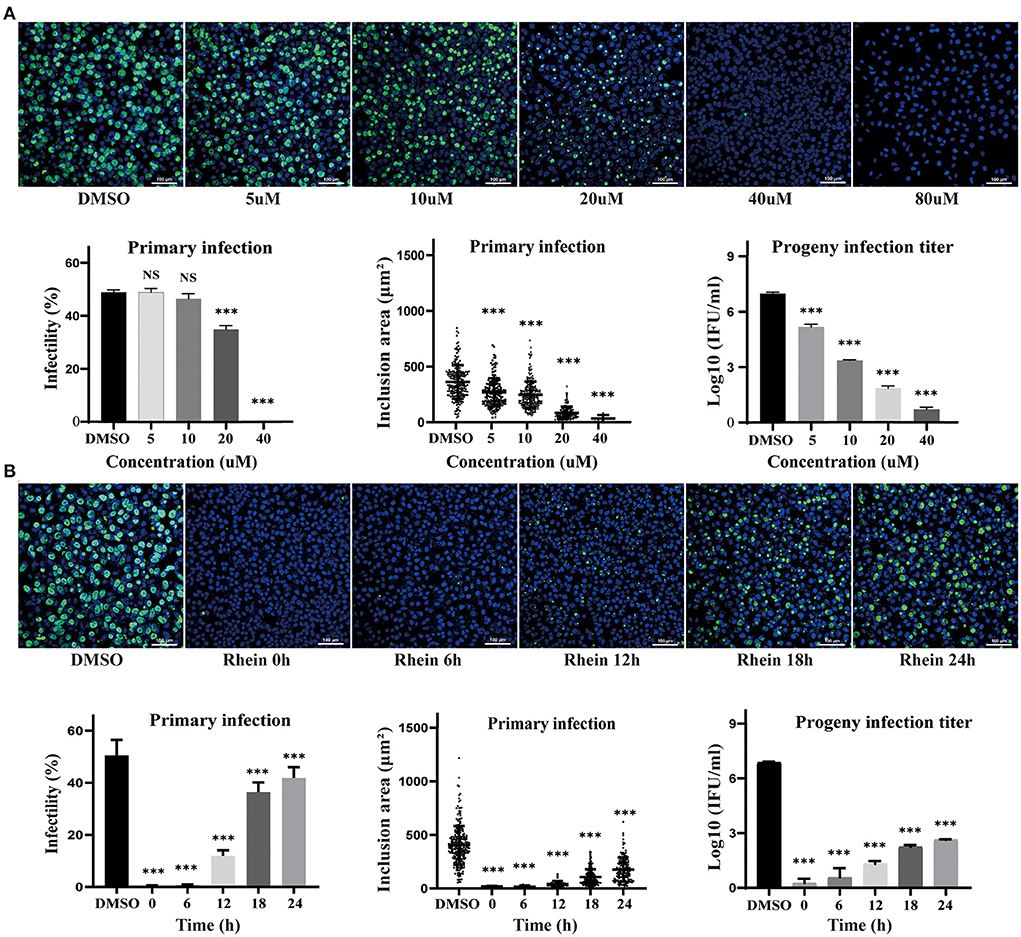
Figure 2. Inhibitory effects of rhein on C. trachomatis infection were dose- and time-dependent. (A) HeLa cells were infected with C. trachomatis serovar D at multiplicity of infection (MOI) 5 and were exposed to various concentrations of rhein (5, 10, 20, 40, and 80 μM) or DMSO for 48 h before fixation and immunostaining. (B) HeLa cells infected with C. trachomatis were exposed to rhein (40 μM) at 0, 6, 12, 18, and 24 hpi. Cells were fixed, and C.trachomatis were stained with a FITC-conjugated anti-MOMP antibody (green), while host cell nuclei were counterstained with DAPI (blue). Scale bars, 100 μm. Data bars in the graphs represent the mean ± standard deviation. NS, not significant; *p< 0.05, **p < 0.01, ***p< 0.001.
Rhein did not directly inactivate C. trachomatis elementary bodies
Rhein and other anthraquinone drugs, including emodin and aloe-emodin, have been extracted from rhubarb. Emodin and aloe-emodin have antibacterial or virucidal activity by destroying the envelope of bacteria or viruses (40–43). Rhein was previously demonstrated to directly inhibit the growth of S. aureus (33). To determine whether rhein could directly impair C. trachomatis activity, 40 μM rhein was co-incubated with C. trachomatis serovar D for 12, 24, 36, and 48 h, respectively (36, 40). The infectivity and inclusion area of C. trachomatis exposed to rhein were not significantly different from those of the corresponding DMSO control (P > 0.05; Figure 3). This suggested that rhein did not directly inactivate C. trachomatis EBs.
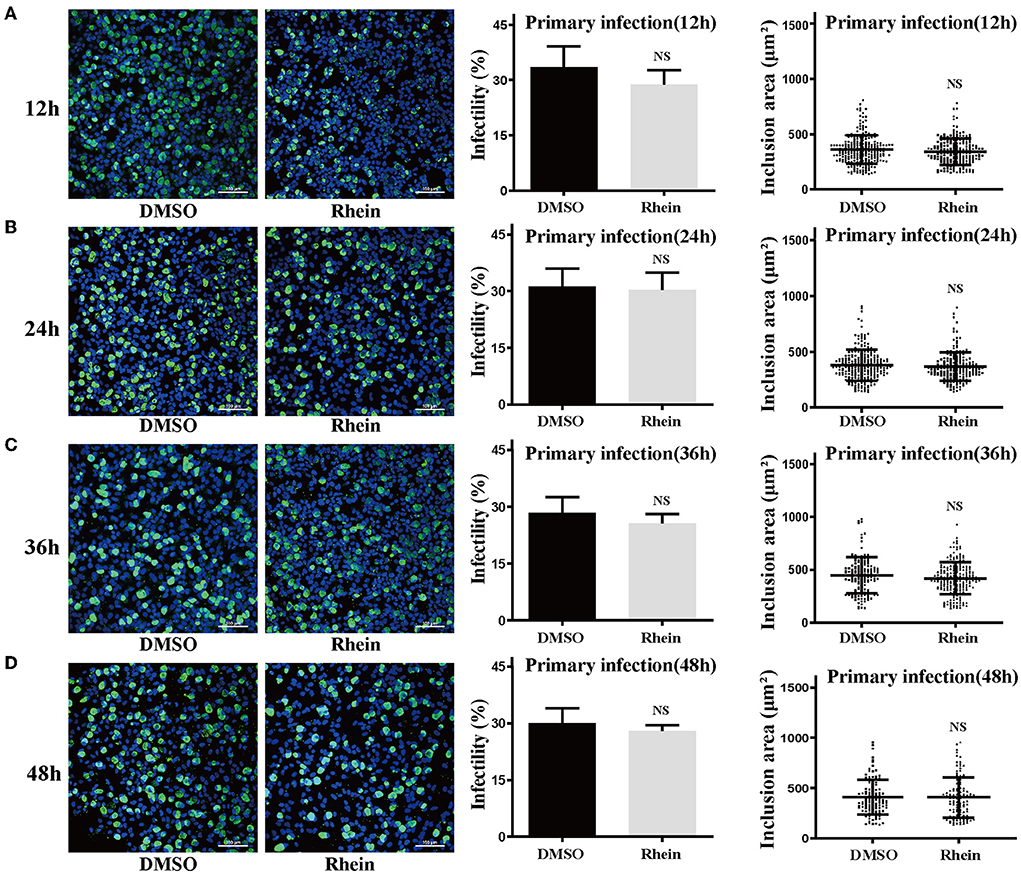
Figure 3. Pretreatment with rhein did not impair C. trachomatis particles. Elementary bodies of C. trachomatis were respectively co-incubated with 40 μM rhein for 12, 24, 36, or 48 h at 4°C before infection. At 48 hpi, C. trachomatis inclusions, infectivity, and inclusion areas were observed by confocal microscopy. (A) 12 h; (B) 24 h; (C) 36 h; and (D) 48 h. Images were captured at ×200 magnification. Scale bars, 100 μm. Data represent the mean ± standard deviation of triplicate samples. NS, not significant. *p< 0.05, **p < 0.01, ***p< 0.001.
Rhein inhibited C. trachomatis through regulation of host cells
C. trachomatis is an obligate intracellular parasitic pathogen that needs to combine with host surface receptors to enter a cell. The pathogen then uses host cell nutrients to replicate and reproduce by regulating the interaction with the host cell (44). Rhein did not have a direct inactivation effect on C. trachomatis. To elucidate the potential inhibitory mechanism of rhein, a set of influence-on-cell, influence-on-adsorption, and influence-on-post-adsorption assays were designed (37, 45) (Figure 4A). The first two assays were used to determine whether rhein affected the adhesion and binding of EB particles to cell membranes, while the third assay was used to determine whether rhein inhibited C. trachomatis during its replication stage. The influence-on-post-adsorption assay showed a significant inhibitory effect of rhein (Figures 4B–D). Our previous study demonstrated that the extracellular signaling-regulated kinase (ERK)/ribosomal S6 kinase (RSK) signaling pathway was important in C. trachomatis infection (46). To investigate the mechanism of action of rhein in C. trachomatis infection, the protein of ERK and RSK were performed by western blotting in the current study on C. trachomatis-infected cells treated with rhein for different times post-infection. P-RSK expression was up-regulated at 12 h after C. trachomatis infection in the presence or absence of rhein. P-ERK and P-RSK were both down-regulated in the presence of rhein at 36 h and 48 h post-infection (Figures 4E,F). The total ERK and RSK remain constant. Moreover, in cell lines of murine (McCoy) and primate (Vero) origin, the antibacterial activity of rhein was also exerted during the replication stage of C.trachomatis (47) (Supplementary Figure S1). These observations suggest that the inhibitory activity of rhein may not be host cell-specific and that rhein may regulate host cells and change the environment to inhibit C. trachomatis replication.
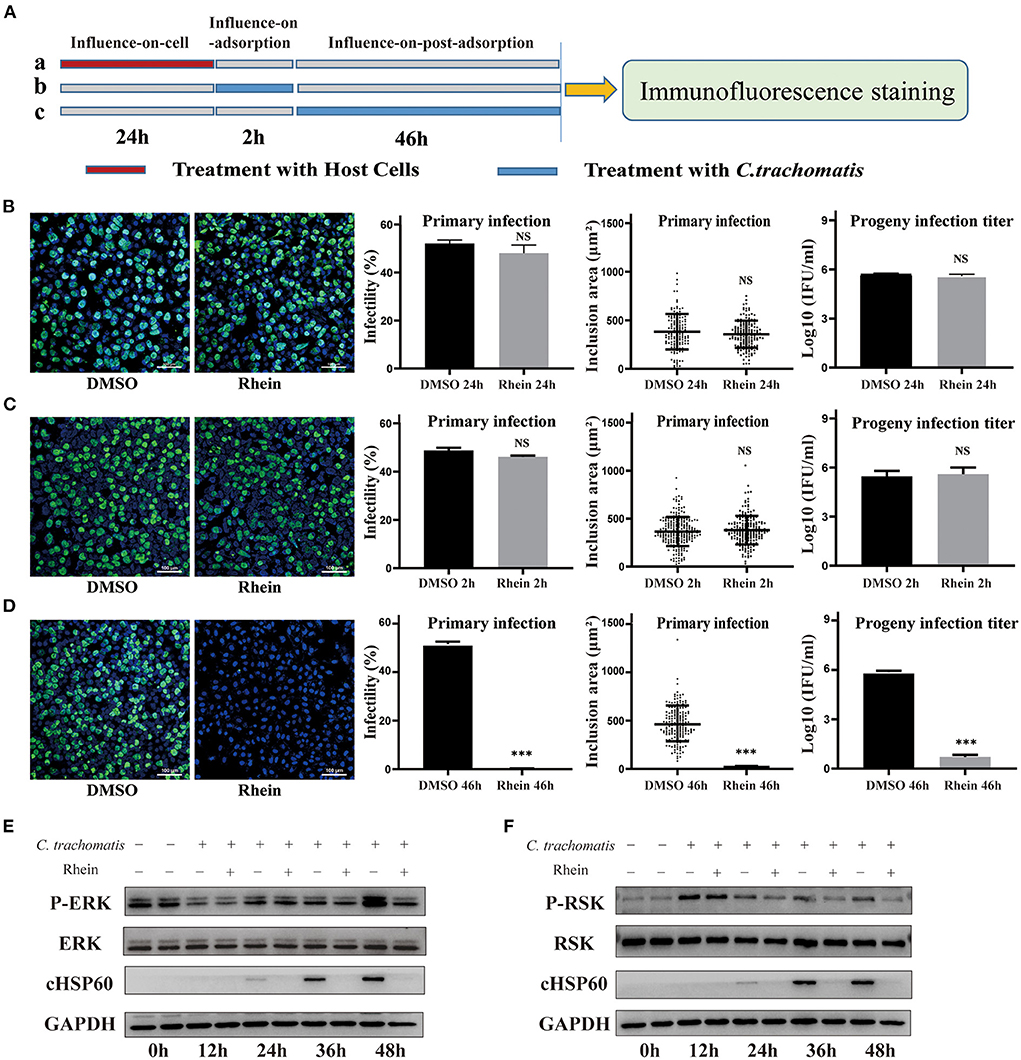
Figure 4. Rhein inhibited C. trachomatis infection by regulating host cells. (A) Three treatment conditions (each row is a treatment): (A) influence-on-cell, cells were pretreated with 40 μM rhein for 24 h; (B) influence-on-adsorption, cells were exposed to 40 μM rhein for 2 h during the period of adsorption; (C) influence-on-post-adsorption, cells were exposed to 40 μM rhein for 46 h after adsorption. (B–D) Immunofluorescent images (×200 magnification), infectivity, inclusion area, and infectious progeny titer. DMSO was used as positive control. (B) Cells were pretreated with 40 μM rhein for 24 h (treatment a). (C) Cells were exposed 40 μM rhein for 2 h during the period of adsorption (treatment b). (D) Cells were exposed 40 μM rhein for 46 h after adsorption (treatment c). (E) Western blots of p-ERK, ERK, cHSP60 and GAPDH protein expression in C. trachomatis-infected cells with or without rhein at different time points post-infection. The bands were cropped from different parts of the same gel. (F) Western blots of p-RSK, RSK, cHSP60 and GAPDH protein expression in C. trachomatis-infected cells with or without rhein at different time points post-infection. The bands were cropped from different parts of the same gel. Data in the graphs represent the mean ± standard deviation of triplicate samples. NS, not significant; *p< 0.05, **p < 0.01, ***p< 0.001.
Rhein and AZM had a synergistic inhibitory effect against C. trachomatis
AZM is a first-line drug for treating C. trachomatis infections, but treatment failure has been reported (15, 17). Although rhein alone impaired growth of C. trachomatis, an experiment was conducted to investigate whether rhein and AZM had a synergistic suppressive effect on C. trachomatis infection. Sub-inhibitory concentrations of 20 μM rhein and 0.005 μg/ml AZM were tested. The infectivity, the area of inclusions and infectious progeny of C. trachomatis were reduced by the two individual treatments (rhein alone and AZM alone) (Figure 5). However, a greater inhibitory effect on C. trachomatis replication was observed when rhein was combined with AZM compared with rhein alone and AZM alone (Figure 5). Thus, the combination of rhein and AZM potentially has great clinical value.
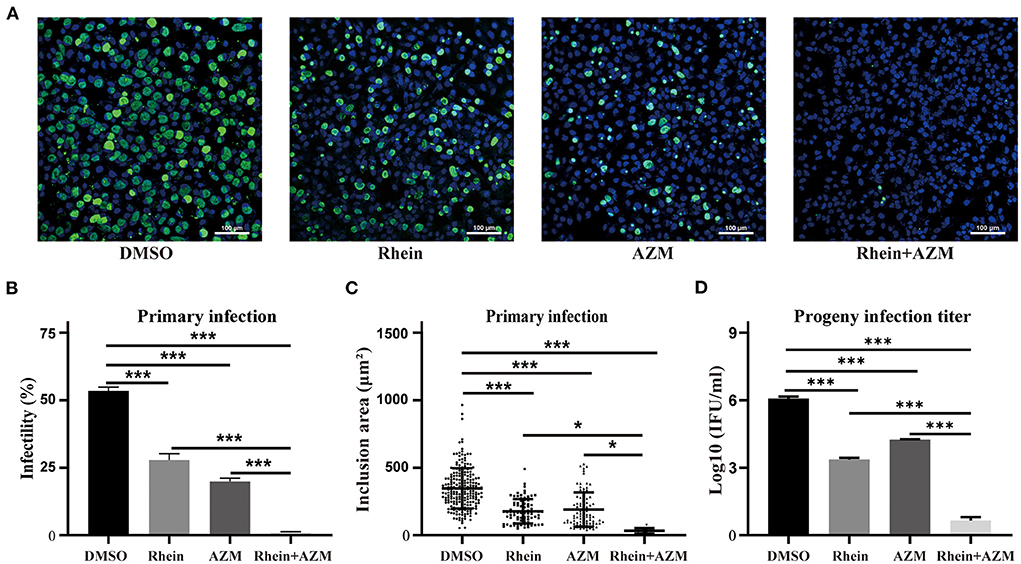
Figure 5. Rhein and AZM combined had synergistic inhibitory effects on C. trachomatis. (A) Immunofluorescence images (×200 magnification; scale bars, 100 μm) of control (DMSO), 20 μM rhein, 0.005 mg/l AZM, and 20 μM rhein + 0.005 mg/l AZM treatments are shown from left to right. (B) Infectivity, (C) inclusion area, and (D) infectious progeny titer of C. trachomatis according to treatments. Data represent the mean ± standard deviation from three independent experiments. *p< 0.05, **p < 0.01, ***p< 0.001.
Rhein combined with AZM inhibited C. trachomatis infection in mouse models
The in vitro experiments demonstrated that rhein effectively inhibited C. trachomatis infection, and when combined with AZM, there was a synergistic inhibitory effect. The inhibitory effect of rhein on C. trachomatis was therefore tested in vivo in a mouse model. Six-week-old female BALB/c mice were infected with C. trachomatis serovar D, then DMSO, AZM, rhein and AZM + rhein were administered orally from day 4 to day 10 post-infection. Vaginal swabs were taken on days 4, 7 and 10 for cell culture and to determine the number of infectious progenies. The number of infectious progenies in the DMSO control and rhein-treated group was not significantly different between days 4, 7, and 10 (Figures 6A,B). However, the number of infectious progenies in the AZM-treated group decreased significantly from day 4 to day 10 (Figure 6C), and the number of infectious progenies in the AZM + rhein treatment group decreased significantly from day 4 to day 7 (Figure 6D). Murine tissues were examined by hematoxylin and eosin (H&E) staining on day 22 after C. trachomatis infection. Edema and hypertrophy were observed in the uterus of infected mice (Figure 6E), but the uterine edema was relieved in the rhein and/or AZM treatment groups. There were no pathological changes in the heart, liver, spleen, or kidney of mice in any treatment group as revealed by H&E staining (Supplementary Figure S2).
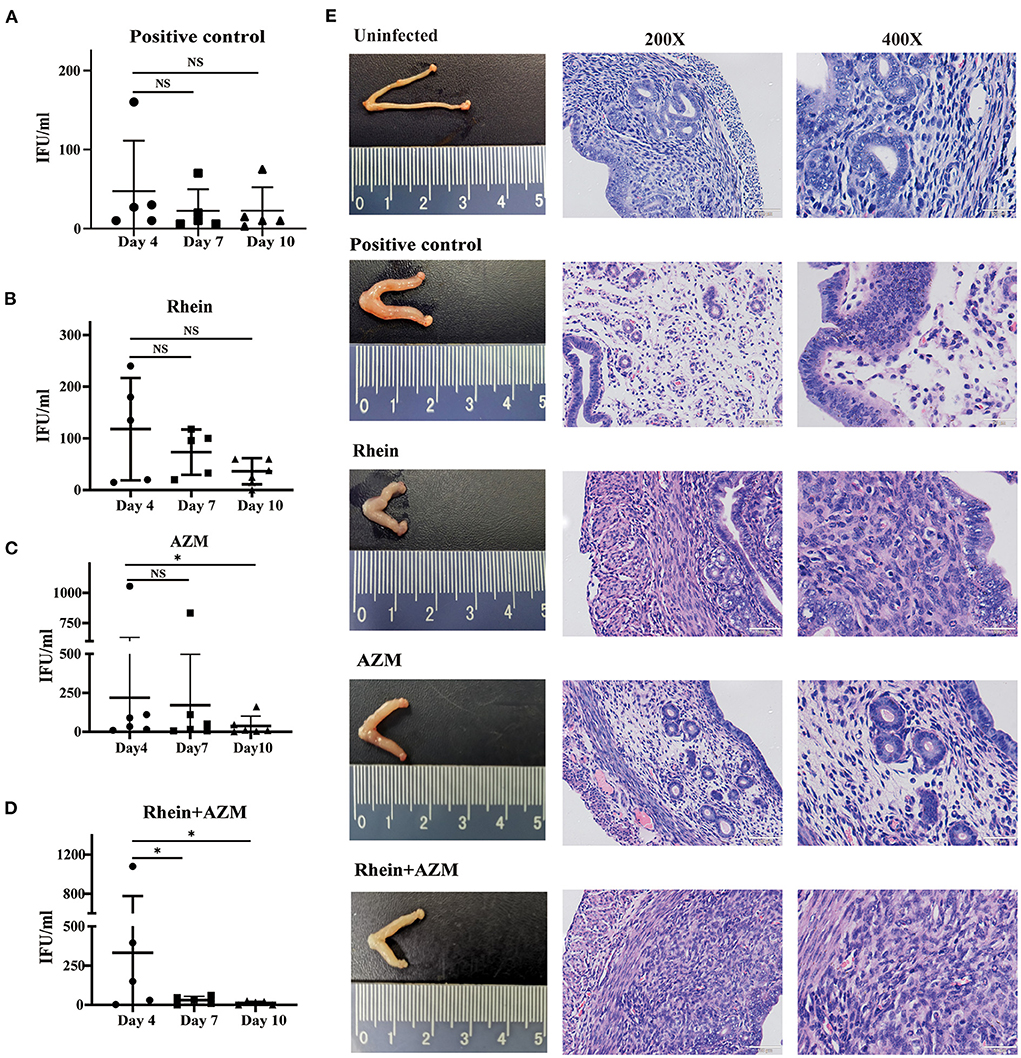
Figure 6. Rhein combined with AZM inhibited C. trachomatis infection in mouse models. Vaginal swabs were taken on days 4, 7, and 10 after infection for cell culture and determination of the number of inclusion bodies. (A) Positive control group. (B) Rhein treatment group. (C) AZM treatment group. (D) AZM + rhein treatment group. (E) Pathological changes in the gross morphology of the uterus (×200 magnification; scale bars, 100 μm. ×400 magnification; scale bars, 50 μm). The nonparametric Wilcoxon test was used for statistical analysis. NS, not significant; *p< 0.05, **p < 0.01, ***p< 0.001.
Discussion
Rhein significantly inhibited C. trachomatis replication across various serovars and in HeLa, McCoy and Vero host cells. In combination with AZM, rhein exerted a synergistic suppressive effect on C. trachomatis infection, both in vitro and in vivo. In addition, rhein may regulate host cells and change the environment to inhibit C. trachomatis replication. Taken together, the findings of this study suggest that rhein may be a potential treatment for C. trachomatis infection.
Rhein was previously reported to have effective antibacterial and antiviral activity against S. aureus, Helicobacter pylori, influenza A virus, and hepatitis B virus (HBV) (33, 36, 46). The mechanism of action of rhein was shown to involve direct impairment of pathogens or regulation of host cell signaling pathways. Rhein increased the transcription of genes encoding the iron-regulated surface determinants system and genes involved in the ribonucleotide reductase systems of S. aureus (33). In addition, rhein exerted its antimicrobial activity against S. aureus by reducing the transcription of genes responsible for anaerobic respiration and fermentation (33). Rhein inhibited DNA polymerase activity in HBV (48). In above studies, the mechanisms of action of rhein involve direct impairment of pathogens. However, rhein also significantly inhibited influenza A virus-induced oxidative stress and decreased influenza A virus-induced expression of Toll-like receptor 2 (TLR2), TLR3 and TLR4. Moreover, rhein suppressed influenza A virus-induced activation of host signaling pathways including the Akt, p38/JNK MAPK and NF-κB pathways in A549 cells (36). In the current study, rhein did not have a direct inactivating effect on C. trachomatis, but rather inhibited this pathogen in a post-adsorption replication stage. C. trachomatis is an intracellular pathogen that is heavily dependent on host cells, thus the mechanism of rhein inhibition of C. trachomatis may be similar to that of influenza A virus whereby host cells are regulated to affect the growth and development of pathogens (49).
Rhein has multiple targets and consequently regulates multiple pathways at the molecular level, including the MAPK signaling pathway, the PI3K-AKT signaling pathway, and the Wnt signaling pathway (31). Among these pathways involved in the pharmacological activity of rhein, the MAPK signaling pathway can be considered one of the most interactive pathways and rhein can regulate the Ras/Raf/MEK/ERK pathway to inhibit the phosphorylation of ERK1/2 (50, 51). The ERK pathway is considered crucial in cell proliferation and migration and RSK is an important downstream effector of the Ras/Raf/MEK/ERK signaling pathway (52, 53). Phosphorylated substrates of RSK are involved in diverse cellular processes including gene transcription, protein synthesis, cell cycle regulation, and cell survival (54, 55). ERK signaling pathways are the most prominent kinase signaling network utilized by C. trachomatis and have been characterized as being instrumental in nutrient acquisition, host cell apoptosis resistance, immune responses, and even pathology associated with chlamydial infections (56–58). Moreover, our previous study suggested that ERK/RSK may be a novel target for C. trachomatis therapeutics (46). In this study, phosphorylated ERK/RSK was reduced upon exposure to rhein, suggesting that rhein may inhibit C. trachomatis infection by regulating the ERK/RSK pathway.
In the process of infectious disease treatment and drug development, host-directed therapy (HDT) is a novel strategy for treating bacterial and viral infections. Biological products or small molecules are used to interfere with replication or persistence of the pathogen by regulating host factors (59). Currently, small-molecule drugs have been proposed for the management of tuberculosis, HBV and HIV by HDT (60–62). C. trachomatis development requires host cell energy and nutrients and may therefore be a suitable pathogen for the development of HDT (63–65). The small molecule mycophenolate mofetil was recently demonstrated to effectively inhibit C. trachomatis growth by targeting the rate-limiting enzyme inosine-5′-monophosphate dehydrogenase in the biosynthesis of guanine nucleotides in host cells (66). In addition, our research team reported that inhibitors targeting ERK/RSK had potential in the treatment of C. trachomatis infection (46). Findings from the current study indicated that rhein may regulate host cells and change the environment to inhibit C. trachomatis replication. Moreover, rhein and AZM had a synergistic inhibitory effect on C. trachomatis in vitro and in vivo. Rhein may therefore be a potential drug for a HDT strategy of managing chlamydial infections.
Although rhein was demonstrated to inhibit C. trachomatis infection, the precise molecular mechanism of rhein on C. trachomatis has not yet been elucidated. Current research suggests that rhein inhibits C. trachomatis survival most likely through targeting host factors. Future work will explore the molecular mechanism by which rhein affects C. trachomatis replication.
In summary, this study provided evidence that rhein reduced C. trachomatis replication in vitro and in vivo and indicated that rhein may have potential in drug development for the treatment of C. trachomatis.
Data availability statement
The raw data supporting the conclusions of this article will be made available by the authors, without undue reservation.
Ethics statement
The animal study was reviewed and approved by the Ethics Committee of South China Agricultural University (SCAU, Guangzhou, China and approval number: 2020c035).
Author contributions
XY and QX performed most of the experiments in this study and jointly wrote the draft manuscript. WC and ZM were responsible for the initial data analysis. XS and LM compiled figure preparation and statistical analysis. JO and YL provided experimental assistance and constructive comments to this study. HZ and YX had the leading contribution to the design of studies and interpretation of the whole dataset. All authors read and approved the manuscript.
Funding
This work was supported by grants from the National Natural Science Foundation of China (81974307), the Natural Science Foundation of Guangdong Province (2018A030313662, 2019A1515011827, and 2021A1515012255), Research Foundation of Department of Education of Guangdong Province (2021KTSCX014), Science and Technology Program of Guangzhou (202201011579), and Guangdong Province Bureau of Traditional Chinese Medicine Scientific Research Project (20211276).
Acknowledgments
We thank Dr. Bin Yang for helpful scientific discussions.
Conflict of interest
The authors declare that the research was conducted in the absence of any commercial or financial relationships that could be construed as a potential conflict of interest.
Publisher's note
All claims expressed in this article are solely those of the authors and do not necessarily represent those of their affiliated organizations, or those of the publisher, the editors and the reviewers. Any product that may be evaluated in this article, or claim that may be made by its manufacturer, is not guaranteed or endorsed by the publisher.
Supplementary material
The Supplementary Material for this article can be found online at: https://www.frontiersin.org/articles/10.3389/fpubh.2022.1002029/full#supplementary-material
References
1. Rowley J, Vander Hoorn S, Korenromp E, Low N, Unemo M, Abu-Raddad LJ, et al. Chlamydia, gonorrhoea, trichomoniasis and syphilis: global prevalence and incidence estimates, 2016. Bull World Health Organ. (2019) 97:548–562P. doi: 10.2471/BLT.18.228486
2. Gjurasin B, Lepej SZ, Cole MJ, Pitt R. Begovac J.Chlamydia trachomatis in cervical lymph node of man with lymphogranuloma venereum, croatia, 2014. Emerg Infect Dis. (2018) 24:806–8. doi: 10.3201/eid2404.171872
3. Mackern-Oberti JP, Motrich RD, Breser ML, Sánchez LR, Cuffini C, Rivero VE. Chlamydia trachomatis infection of the male genital tract: an update. J Reprod Immunol. (2013) 100:37–53. doi: 10.1016/j.jri.2013.05.002
4. Gitsels A, Sanders N, Vanrompay D. Chlamydial infection from outside to inside. Front Microbiol. (2019) 10:2329. doi: 10.3389/fmicb.2019.02329
5. Cheok YY, Lee CYQ, Cheong HC, Looi CY, Wong WF. Chronic inflammatory diseases at secondary sites ensuing urogenital or pulmonary chlamydia infections. Microorganisms. (2020) 8:127. doi: 10.3390/microorganisms8010127
6. Peterman TA, Newman DR, Maddox L, Schmitt K, Shiver S. Risk for HIV following a diagnosis of syphilis, gonorrhoea or chlamydia: 328,456 women in Florida, 2000-2011. Int J STD AIDS. (2015) 26:113–9. doi: 10.1177/0956462414531243
7. Escarcega-Tame MA, López-Hurtado M, Escobedo-Guerra MR, Reyes-Maldonado E, Castro-Escarpulli G, Guerra-Infante FM. Co-infection between genotypes of the human papillomavirus and Chlamydia trachomatis in Mexican women. Int J STD AIDS. (2020): 31:1255–62. doi: 10.1177/0956462420947587
8. Seraceni S, De Seta F, Colli C, Del Savio R, Pesel G, Zanin V, et al. High prevalence of hpv multiple genotypes in women with persistent chlamydia trachomatis infection. Infect Agent Cancer. (2014) 9:30. doi: 10.1186/1750-9378-9-30
9. Kohlhoff SA, Hammerschlag MR. Treatment of Chlamydial infections: 2014 update. Expert Opin Pharmacother. (2015) 16:205–12. doi: 10.1517/14656566.2015.999041
10. Qi M-L, Guo Y-L, Wang Q-Q, Chen X-S, Han J-D, Su X-H, et al. Consensus by Chinese expert panel on chlamydia trachomatis-resistant and chlamydia trachomatis-persistent infection. Chin Med J (Engl). (2017) 130:2852–6. doi: 10.4103/0366-6999.219159
11. Workowski KA, Bolan GA. Centers for disease control and prevention. Sexually transmitted diseases treatment guidelines, 2015. MMWR Recomm Rep. (2015) 64:1–37.
12. Lanjouw E, Ossewaarde JM, Stary A, Boag F, van der Meijden WI. 2010 European guideline for the management of Chlamydia trachomatis infections. Int J STD AIDS. (2010) 21:729–37. doi: 10.1258/ijsa.2010.010302
13. Kong FY, Hocking JS. Treatment challenges for urogenital and anorectal Chlamydia trachomatis. BMC Infect Dis. (2015) 15:293. doi: 10.1186/s12879-015-1030-9
14. Tamarelle J, Ma B, Gajer P, Humphrys MS, Terplan M, Mark KS, et al. Nonoptimal vaginal microbiota after azithromycin treatment for chlamydia trachomatis infection. J Infect Dis. (2020) 221:627–35. doi: 10.1093/infdis/jiz499
15. O'Brien KS, Emerson P, Hooper PJ, Reingold AL, Dennis EG, Keenan JD, et al. Antimicrobial resistance following mass azithromycin distribution for trachoma: a systematic review. Lancet Infect Dis. (2019) 19:e14–25. doi: 10.1016/S1473-3099(18)30444-4
16. Pitt RA, Alexander S, Horner PJ, Ison CA. Presentation of clinically suspected persistent chlamydial infection: a case series. Int J STD AIDS. (2013) 24:469–75. doi: 10.1177/0956462412472815
17. Golden MR, Whittington WL, Handsfield HH, Hughes JP, Stamm WE, Hogben M, et al. Effect of expedited treatment of sex partners on recurrent or persistent gonorrhea or chlamydial infection. N Engl J Med. (2005) 352:676–85. doi: 10.1056/NEJMoa041681
18. Misyurina OY, Chipitsyna EV, Finashutina YP, Lazarev VN, Akopian TA, Savicheva AM. et al. Mutations in a 23S rRNA gene of Chlamydia trachomatis associated with resistance to macrolides. Antimicrob Agents Chemother. (2004) 48:1347–9. doi: 10.1128/AAC.48.4.1347-1349.2004
19. Li M, Zhang X, Huang K, Qiu H, Zhang J, Kang Y. et al. Presence of Chlamydia trachomatis and Mycoplasma spp, but not Neisseria gonorrhoeae and Treponema pallidum, in women undergoing an infertility evaluation: high prevalence of tetracycline resistance gene tet(M). AMB Expr. (2017) 7:206. doi: 10.1186/s13568-017-0510-2
20. Mestrovic T, Ljubin-Sternak S. Molecular mechanisms of Chlamydia trachomatis resistance to antimicrobial drugs. Front Biosci (Landmark Ed). (2018) 23:656–70. doi: 10.2741/4611
21. Shao L, You C, Cao J, Jiang Y, Liu Y, Liu Q. High treatment failure rate is better explained by resistance gene detection than by minimum inhibitory concentration in patients with urogenital Chlamydia trachomatis infection. Int J Infect Dis. (2020) 96:121–7. doi: 10.1016/j.ijid.2020.03.015
22. Panzetta ME, Valdivia RH, Saka HA. Chlamydia persistence:a survival strategy to evade antimicrobial effects in-vitro and in-vivo. Front Microbiol. (2018) 9:3101. doi: 10.3389/fmicb.2018.03101
23. AlSheikh HMA, Sultan I, Kumar V, Rather IA, Al-Sheikh H, Tasleem Jan A, et al. Plant-based phytochemicals as possible alternative to antibiotics in combating bacterial drug resistance. Antibiotics (Basel). (2020) 9:480. doi: 10.3390/antibiotics9080480
24. Adamczak A, Ozarowski M, Karpiński TM. Antibacterial activity of some flavonoids and organic acids widely distributed in plants. J Clin Med. (2019) 9:109. doi: 10.3390/jcm9010109
25. Cappiello F, Loffredo MR, Del Plato C, Cammarone S, Casciaro B, Quaglio D, et al. The revaluation of plant-derived terpenes to fight antibiotic-resistant infections. Antibiotics (Basel). (2020) 9:325. doi: 10.3390/antibiotics9060325
26. Casciaro B, Mangiardi L, Cappiello F, Romeo I, Loffredo MR, Iazzetti A, et al. Naturally-occurring alkaloids of plant origin as potential antimicrobials against antibiotic-resistant infections. Molecules. (2020) 25:3619. doi: 10.3390/molecules25163619
27. Dorman HJ, Deans SG. Antimicrobial agents from plants: antibacterial activity of plant volatile oils. J Appl Microbiol. (2000) 88:308–16. doi: 10.1046/j.1365-2672.2000.00969.x
28. Huang M, Zheng H, Duan X, Xue Y, Li J. Study on the Anti-chlamydia trachomatis activity of single chinese medicine and 7 heat-clearing drugs of bazheng powder in vitro. Chin J Leprosy Skin Dis. (2015) 31:15–7.
29. Zhou YX, Xia W, Yue W, Peng C, Rahman K, Zhang H. Rhein: A Review of Pharmacological Activities. Evid Based Complement Alternat Med. (2015) 2015:578107. doi: 10.1155/2015/578107
30. Zhang XR, Wang JB, Xiao XH, Liu TS, Chu XH, Zhou CP, et al. Antimicrobial activity and chemical differences between the two chemotypes of rhubarbs. Yao Xue Xue Bao. (2010) 45:1144–8. doi: 10.16438/j.0513-4870.2010.09.005
31. Sun H, Luo G, Chen D, Xiang Z. A Comprehensive and system review for the pharmacological mechanism of action of rhein, an active anthraquinone ingredient. Front Pharmacol. (2016) 7:247. doi: 10.3389/fphar.2016.00247
32. Zheng H, Xue Y, Bai S, Qin X, Lu P, Yang B. Association of the in vitro susceptibility of clinical isolates of chlamydia trachomatis with serovar and duration of antibiotic exposure. Sex Transm Dis. (2015) 42:115–9. doi: 10.1097/OLQ.0000000000000241
33. Yu L, Xiang H, Fan J, Wang D, Yang F, Guo N, et al. Global transcriptional response of Staphylococcus aureus to rhein, a natural plant product. J Biotechnol. (2008) 135:304–8. doi: 10.1016/j.jbiotec.2008.04.010
34. Saito ST, Trentin Dda S, Macedo AJ, Pungartnik C, Gosmann G, Silveira Jde D, et al. Bioguided fractionation shows cassia alata extract to inhibit staphylococcus epidermidis and pseudomonas aeruginosa growth and biofilm formation. Evid Based Complement Alternat Med. (2012) 2012:867103. doi: 10.1155/2012/867103
35. Azelmat J, Larente JF, Grenier D. The anthraquinone rhein exhibits synergistic antibacterial activity in association with metronidazole or natural compounds and attenuates virulence gene expression in Porphyromonas gingivalis. Arch Oral Biol. (2015) 60:342–6. doi: 10.1016/j.archoralbio.2014.11.006
36. Wang QW, Su Y, Sheng JT, Gu LM, Zhao Y, Chen XX, et al. Anti-influenza A virus activity of rhein through regulating oxidative stress, TLR4, Akt, MAPK, and NF-κB signal pathways. PLoS ONE. (2018) 13:e0191793. doi: 10.1371/journal.pone.0191793
37. Li JL, Yang N, Huang L, Chen D, Zhao Y, Tang MM, et al. Pyocyanin inhibits chlamydia infection by disabling infectivity of the elementary body and disrupting intracellular growth. Antimicrob Agents Chemother. (2018) 62:e02260–17. doi: 10.1128/AAC.02260-17
38. Shaw JH, Behar AR, Snider TA, Allen NA, Lutter EI. Comparison of murine cervicovaginal infection by chlamydial strains: identification of extrusions shed in vivo. Front Cell Infect Microbiol. (2017) 7:18. doi: 10.3389/fcimb.2017.00018
39. Henning TR, Morris M, Ellis S, Kelley K, Phillips C, Ritter J, et al. Development of a rectal sexually transmitted infection (STI) Model in Rhesus macaques using Chlamydia trachomatis serovars E and L[[sb]]2[[/s]]. J Med Primatol. (2017) 46:218–27. doi: 10.1111/jmp.12272
40. Batista MN, Braga ACS, Campos GRF, Souza MM, Matos RPA, Lopes TZ, et al. Natural products isolated from oriental medicinal herbs inactivate zika virus. Viruses. (2019) 11:49. doi: 10.3390/v11010049
41. Chang SJ, Huang SH, Lin YJ, Tsou YY, Lin CW. Antiviral activity of Rheum palmatum methanol extract and chrysophanol against Japanese encephalitis virus. Arch Pharm Res. (2014) 37:1117–23. doi: 10.1007/s12272-013-0325-x
42. Sydiskis RJ, Owen DG, Lohr JL, Rosler KH, Blomster RN. Inactivation of enveloped viruses by anthraquinones extracted from plants. Antimicrob Agents Chemother. (1991) 35:2463–6. doi :10.1128/AAC.35.12.2463. doi: 10.1128/AAC.35.12.2463
43. Alves DS, Pérez-Fons L, Estepa A, Micol V. Membrane-related effects underlying the biological activity of the anthraquinones emodin and barbaloin. Biochem Pharmacol. (2004) 68:549–61. doi: 10.1016/j.bcp.2004.04.012
44. Elwell C, Mirrashidi K, Engel J. Chlamydia cell biology and pathogenesis. Nat Rev Microbiol. (2016) 14:385–400. doi: 10.1038/nrmicro.2016.30
45. Osaka I, Hefty PS. Lipopolysaccharide-binding alkylpolyamine DS-96 inhibits Chlamydia trachomatis infection by blocking attachment and entry. Antimicrob Agents Chemother. (2014) 58:3245–54. doi: 10.1128/AAC.02391-14
46. Xue Y, Chen W, Mai Z, Yu X, Wu Q, Wan C, et al. Inhibition of the extracellular signal-regulated kinase/ribosomal S6 kinase cascade limits Chlamydia Trachomatis infection. J Invest Dermatol. (2021) 141:852–862.e6. doi: 10.1016/j.jid.2020.07.033
47. Sandoz KM, Valiant WG, Eriksen SG, Hruby DE, Allen RD. 3rd, Rockey DD. The broad-spectrum antiviral compound ST-669 restricts chlamydial inclusion development and bacterial growth and localizes to host cell lipid droplets within treated cells. Antimicrob Agents Chemother. (2014) 58:3860–6. doi: 10.1128/AAC.02064-13
48. Li Z, Li LJ, Sun Y, Li J. Identification of natural compounds with anti-hepatitis B virus activity from Rheum palmatum L. ethanol Extract Chemother. (2007) 53:320–6. doi: 10.1159/000107690
49. Drosten M, Barbacid M. Targeting the MAPK pathway in KRAS-driven tumors. Cancer Cell. (2020) 37:543–50. doi: 10.1016/j.ccell.2020.03.013
50. Lin ML, Chung JG, Lu YC, Yang CY, Chen SS. Rhein inhibits invasion and migration of human nasopharyngeal carcinoma cells in vitro by down-regulation of matrix metalloproteinases-9 and vascular endothelial growth factor. Oral Oncol. (2009) 45:531–7. doi: 10.1016/j.oraloncology.2008.07.012
51. Wu C, Cao H, Zhou H, Sun L, Xue J, Li J, et al. Research progress on the antitumor effects of rhein: literature review. Anticancer Agents Med Chem. (2017) 17:1624–32. doi: 10.2174/1871520615666150930112631
52. Anjum R, Blenis J. The RSK family of kinases: emerging roles in cellular signalling. Nat Rev Mol Cell Biol. (2008) 9:747–58. doi: 10.1038/nrm2509
53. Kun E, Tsang YTM, Ng CW, Gershenson DM, Wong KK. MEK inhibitor resistance mechanisms and recent developments in combination trials. Cancer Treat Rev. (2020) 92:102137. doi: 10.1016/j.ctrv.2020.102137
54. Lin L, White SA, Hu K. Role of p90RSK in kidney and other diseases. Int J Mol Sci. (2019) 20:972. doi: 10.3390/ijms20040972
55. Casalvieri KA, Matheson CJ, Backos DS, Reigan P. Selective targeting of RSK isoforms in cancer. Trends Cancer. (2017) 3:302–12. doi: 10.1016/j.trecan.2017.03.004
56. Kun D, Xiang-Lin C, Ming Z, Qi L. Chlamydia inhibit host cell apoptosis by inducing Bag-1 via the MAPK/ERK survival pathway. Apoptosis. (2013) 18:1083–92. doi: 10.1007/s10495-013-0865-z
57. Su H, McClarty G, Dong F, Hatch GM, Pan ZK, Zhong G. Activation of Raf/MEK/ERK/cPLA2 signaling pathway is essential for chlamydial acquisition of host glycerophospholipids. J Biol Chem. (2004) 279:9409–16. doi: 10.1074/jbc.M312008200
58. Mehlitz A, Banhart S, Mäurer AP, Kaushansky A, Gordus AG, Zielecki J, et al. Tarp regulates early Chlamydia-induced host cell survival through interactions with the human adaptor protein SHC1. J Cell Biol. (2010) 190:143–57. doi: 10.1083/jcb.200909095
59. Zumla A, Rao M, Wallis RS, Kaufmann SHE, Rustomjee R, Mwaba P, et al. Host-directed therapies for infectious diseases: current status, recent progress, and future prospects. Lancet Infect Dis. (2016) 16:e47–63. doi: 10.1016/S1473-3099(16)00078-5
60. Passioura T, Watashi K, Fukano K, Shimura S, Saso W, Morishita R, et al. De novo macrocyclic peptide inhibitors of hepatitis b virus cellular entry. Cell Chem Biol. (2018) 25:906–15.e5. doi: 10.1016/j.chembiol.2018.04.011
61. Verrier ER, Schuster C, Baumert TF. Advancing hepatitis B virus entry inhibitors. J Hepatol. (2017) 66:677–9. doi: 10.1016/j.jhep.2016.11.028
62. Andersson JA, Sha J, Kirtley ML, Reyes E, Fitts EC, Dann SM, et al. Combating multidrug-resistant pathogens with host-directed nonantibiotic therapeutics. Antimicrob Agents Chemother. (2017) 62:e01943–17. doi: 10.1128/AAC.01943-17
63. Rother M, Teixeira da Costa AR, Zietlow R, Meyer TF, Rudel T. Modulation of host cell metabolism by Chlamydia trachomatis. Microbiology spectrum. (2019) 7:7-3. doi: 10.1128/microbiolspec.BAI-0012-2019
64. Olive AJ, Haff MG, Emanuele MJ, Sack LM, Barker JR, Elledge SJ, et al. Chlamydia trachomatis-induced alterations in the host cell proteome are required for intracellular growth. Cell Host Microbe. (2014) 15:113–24. doi: 10.1016/j.chom.2013.12.009
65. Mehlitz A, Eylert E, Huber C, Lindner B, Vollmuth N, Karunakaran K, et al. Metabolic adaptation of Chlamydia trachomatis to mammalian host cells. Mol Microbiol. (2017) 103:1004–19. doi: 10.1111/mmi.13603
Keywords: Chlamydia trachomatis, antibacterial activity, rhein, host-directed therapy, azithromycin, in vivo
Citation: Yu X, Xu Q, Chen W, Mai Z, Mo L, Su X, Ou J, Lan Y, Zheng H and Xue Y (2022) Rhein inhibits Chlamydia trachomatis infection by regulating pathogen-host cell. Front. Public Health 10:1002029. doi: 10.3389/fpubh.2022.1002029
Received: 24 July 2022; Accepted: 07 September 2022;
Published: 26 September 2022.
Edited by:
Weiming Tang, University of North Carolina at Chapel Hill, United StatesReviewed by:
Fengshi Jing, University of North Carolina, United StatesGifty Marley, University of North Carolina China-Project (SESH Global), China
Copyright © 2022 Yu, Xu, Chen, Mai, Mo, Su, Ou, Lan, Zheng and Xue. This is an open-access article distributed under the terms of the Creative Commons Attribution License (CC BY). The use, distribution or reproduction in other forums is permitted, provided the original author(s) and the copyright owner(s) are credited and that the original publication in this journal is cited, in accordance with accepted academic practice. No use, distribution or reproduction is permitted which does not comply with these terms.
*Correspondence: Heping Zheng, zhhpf@hotmail.com; Yaohua Xue, yaohuaxue2015@163.com
†These authors have contributed equally to this work and share first authorship