- 1College of Pharmaceutical Science, Zhejiang University of Technology, Hangzhou, China
- 2Institute of Information Resource, Zhejiang University of Technology, Hangzhou, China
- 3Hangzhou Aeronautical Sanatorium for Special Service of Chinese Air Force, Hangzhou, China
- 4Department of Chinese Medicine, Macau University of Science and Technology, Taipa, Macau SAR, China
Antibiotic-resistant bacteria (ARB) are a serious threat to the health of people and the ecological environment. With this problem becoming more and more serious, more countries made research on the ARB, and the research number has been sharply increased particularly over the past decade. Therefore, it is quite necessary to globally retrace relevant researches on the ARB published from 2010 to 2020. This will help researchers to understand the current research situation, research trends and research hotspots in this field. This paper uses bibliometrics to examine publications in the field of ARB from 2010 to 2020 that were retrieved from the Web of Science (WOS). Our study performed a statistical analysis of the countries, institutions, journals, authors, research areas, author keywords, Essential Science Indicators (ESI) highly cited papers, and ESI hotspots papers to provide an overview of the ARB field as well as research trends, research hotspots, and future research directions in the field. The results showed that the number of related studies is increasing year by year; the USA is most published in the field of ARB; China is the most active in this field in the recent years; the Chinese Acad Sci published the most articles; Sci. Total Environ. published the greatest number of articles; CM Manaia has the most contributions; Environmental Sciences and Ecology is the most popular research area; and “antibiotic resistance,” “antibiotics,” and “antibiotic resistance genes” were the most frequently occurring author keywords. A citation analysis showed that aquatic environment-related antibiotic resistance is a key research area in this field, while antimicrobial nanomaterial-related research is a recent popular topic.
Introduction
Antibiotic-resistant bacteria are resistant to both natural and synthetic antibiotics (1) and thus have become a health concern worldwide. Multi-drug resistant bacteria (MDRB) with stronger resistance can be resistant to 3 or more antibiotics in clinic (2–5). Bacteria can develop intrinsic resistance to certain antibiotics, but can also acquire resistance to antibiotics (6). Among them, the path for bacteria to acquire or development antibiotic resistance which roots in the irrational usage of antibiotics is to prevent antibiotics from entering target, change the antibiotic targets and inactivate antibiotics (6–9). The irrational usage of antibiotics can lead to the prolonged exposure of bacteria to sublethal concentrations of antibiotics which is a key to the resistance selection (10, 11). Because antibiotics with sublethal concentrations cannot kill bacteria, but can affect the frequency of mutations, horizontal gene transfer (HGT) and gene recombination of bacteria, and have a chance to enrich existing low-level resistant mutations or improve the level of drug resistance mutation. The spread of antibiotic resistance among different bacterial populations is achieved through HGT (12). HGT refers to the transfer of antibiotic resistance genes (ARGs) between bacteria by transformation, transduction, and conjugation with the help of plasmids, integrons, transposons and so on (13). A large number of bacterial species are resistant to macrolides, sulfonamides, tetracyclines, and other antibiotics in the biological systems (14). Antibiotic has become synonymous with “antibacterial drug” in some degree, therefore, in this review antibiotic has been used.
Antibiotics are not completely metabolized in the human body, and some are excreted into the sewage with urine and feces in prototype (10). As the sewage treatment process has created a potential environment suitable for the development and spread of antibiotic resistance, such as high bacterial density, pressure caused by pollutants such as heavy metals and antibiotics, etc. Therefore, the discharge of treated sewage gives rise to a large number of ARB and ARGs in the surrounding ecological environment (e.g., aquatic system and soil) (12, 15–21). Moreover, the proportion of antibiotic resistance in chickens, pigs, and wild animals has also increased greatly (22), thus causing a serious burden of infection to human beings (23–25), and greatly affecting the ecological environment (26). Humans can be infected with ARB in different ways. For example, ARB in communities and medical settings can be transmitted through person-to-person contact (27). Healthcare associated infections (HAIs) are infections caused to patients by invasive devices or surgical procedures, such as catheter-associated urinary tract infections, surgical site infections, and ventilator-associated pneumonia (28), which are also common infections with ARB. Antibiotic-resistant bacteria can also be transmitted to people through the environment. For example, driven by hydrological processes such as runoff and infiltration, the treated sewage enters the sources of drinking water, such as surface water and groundwater, after being discharged into the environment, resulting in ARB and ARGs in the drinking water sources (29). However, conventional drinking water treatment is mainly designed to remove contaminants such as heavy metals, solid particles and pathogenic microorganisms, rather than to remove ARB, which may even promote the transmission of ARB from the environment to humans (29, 30). Soil may lead to the transfer of resistance determinants from the environment or zoonotic bacteria to humans (31). When the ARB infect the human body, it can transfer to the human pathogenic bacteria. Once the pathogenic bacteria develop resistance, it is harder to control and treat bacterial infections (29). For example, antibiotic resistance may lead to increased virulence and pathogenicity, increased morbidity and mortality, longer hospital stays, and reduced availability of antibiotics (32, 33). According to the WHO, 10 million people may die from ARB infections every year by 2050. In 2010, the Infectious Diseases Society of America started the “10 × ‘20 Initiative”, with the goal of developing 10 effective antibacterial medications by 2020 (34). The WHO published a priority list in 2018 to guide the creation of new antibiotics (35). However, the rate of new antibiotic research and development is surprisingly slow (36). Very few new structural classes of antibiotics have been introduced since 2000 (37, 38), e.g., cyclic lipopeptide (daptomycin) (39, 40), oxazolidinone (linezolid) (41), etc. Yet more and more bacteria are resistant to many antibiotics used clinically (42, 43). We are no longer confident in the face of more and more bacterial infections (6). Therefore, new antimicrobial strategies are particularly important (44). In the early stage, it was mainly treated in combination with other antibiotics, such as streptomycin and penicillin. The combination of antibiotics has a synergistic effect, which not only has better efficacy than a single drug, but also can inhibit the drug resistance selection of a single drug (45, 46). With the development of multi-drug resistant bacteria, antibiotic substitutes (47) such as phage therapy (48–50), nanomaterials (51–54), bacteriocins (55), antibodies, and probiotics (56) have been attracted more attention.
The earliest monographic study in the field of ARB was published in 1990, and it provided an initial description of the antibiotic resistance mechanism (57). Findings over the subsequent decade included the identification of ARB in aquaculture for the first time (58–60), which was based on irrational antibiotic use in aquaculture (61). In addition, preliminary studies on the spread of ARB (62, 63), doctors' prescriptions (64) as well as phage therapy (65) were performed. During the period from 2000 to 2009, the findings focused on the fact that ARB and ARGs were discovered in wastewater and drinking water (66, 67). Antibiotic resistance (68–70), nanorods (71), phage therapy (72), and rational antibiotic use interventions (73) were further studied. In the last decade, with the development and application of polymerase chain reaction (PCR) assays (74, 75) and metagenomic analysis (76–79), the abundance of multiple ARGs could be identified. Consequently, ARB and ARGs were detected in aquatic systems, such as wastewater (80, 81), rivers (82–85), lakes (86), seawater (87), drinking water (88), reclaimed wastewater (89), and aquaculture (90), as well as animal husbandry (91, 92), compost (93), soil (94, 95), and vegetables (96, 97). For the sake of preventing the spread of ARB and ARGs in the environment and mitigating the damage to humans, animals and the ecological environment, an increasing number of researchers have devoted themselves to finding solutions to this difficult problem. Hence, a large number of processes for removing antibiotics, ARB and ARGs from wastewater have emerged, including chlorination (98, 99), ultraviolet (UV) (100, 101), advanced oxidation processes (AOPs) (102, 103), ozonation (104), solar photo-Fenton (105–107), photocatalytic oxidation (108, 109), constructed wetlands (CWs) (110), and membrane bioreactors (MBRs) (111). Even though studies on ARB and ARGs in wastewater and drinking water were carried out from 2000 to 2009 and from 2010 to 2020, the research content from 2010 to 2020 was more focused. Since the comparison and analysis of ARB and ARGs were generally conducted from 2000 to 2009, most of the samples collected in this stage were from source water, effluent from sewage treatment plants or rivers, while the research from 2010 to 2020 targeted more on the sewage treatment process. The samples collected in this stage may come from different treatment steps in the sewage process. For example, it may come from sand filtration and peracetic acid treatment (112) or various sewage treatment methods, e.g., chlorination (99), ozone (104), etc. Moreover, the detection technologies employed during 2010–2020 are more efficient, such as high-throughput sequencing technology (14).
ARB is highly interrelated to human and ecological health, and there has been more extensive previous studies in this field, the priority list of ARB (35), ARB persistence (113), the challenge of ARB in the food industry (114), the antibiotic resistance profiles (19, 22) antimicrobial strategies (115–117) and antibiotics discovery (36). ARB are a serious threat to the health of people and the ecological environment. With this problem becoming more and more serious, more countries made research on the ARB, and the research number has been sharply increased particularly over the past decade. Therefore, it is quite necessary to globally retrace relevant researches on the ARB in recent 10 years. This will help researchers to understand the current research situation, research trends and research hotspots in this field.
Bibliometric analysis is an effective method for quantitatively assessing academic papers and can be used to investigate the evolution of certain fields, and the results can provide an overview of a certain field as well as research trends, hot topics, distribution of research power and future research directions (118–122). The advantage of bibliometric is that it is not limited by geography, allowing data to be collected by country in a particular area to analyze research globally (123). In addition, specific data analysis software can process the results of bibliometric analyses and present them in a more three-dimensional form (124–127). Therefore, bibliometric analyses have been applied to many fields, such as medicine (128–130), chemistry (131), psychology (132), computer science (133, 134), and robotics (120). In addition, bibliometrics is also widely applied to the aspect of research method, for example, the publications related to such research methods as TOPSIS (135), Analytic Hierarchy Process (136), and ordered weighted averaging operator (137) can also make knowledge recreation by bibliometrics.
To our knowledge bibliometric analysis of publications in the field of ARB has been conducted, but related studies only focused on antibiotics in soil (138) and ARGs (139). Since the study of ARB is multifaceted, such as generation (6), impact (23), control (140), and treatment (55) of ARB, and so on, a comprehensive analysis of ARB research from a bibliometric perspective remains necessary. The goal of this paper is to apply a bibliometric approach to review the leading countries, institutions, authors, and journals, research areas, national and institutional collaborations, author keywords, and ESI highly cited and hot papers to provide research situation, research trends and research hotspots in the field of ARB between 2010 and 2020 globally and then propose future research directions.
Materials and methods
A bibliometric analysis of publications in the field of ARB published between 2010 and 2020 is presented in this paper. Data were obtained from the Science Citation Index Expanded database (SCI-E) and Social Sciences Citation Index database (SSCI). Scopus, Pubmed and Google Academic indeed cover more publications than Web of Science. However, the publications included into the core complications of WOS generally receive higher recognition and it is the most widely accepted database for analysis of science publications (141). Therefore, WOS was chosen as the data source for this study. First, the subject field was set to “antibiotic resistant bacteria”, the date range was set to 2010-01-01 to 2020-12-31, and the document type was set to “article” and “review” for the search. The corresponding country, institution, journal, author, author keywords, and research area of publications meeting the search criteria are listed. The same data were extracted from ESI highly cited and hot papers. Then, the Derwent Data Analyzer (DDA10.0 build 27,330, Search Technology Inc., Norcross, GA, USA), which is a tool for data cleaning, mining and visual processing, was used to clean the derived data.
Although ARB is an acronym for antibiotic resistant bacteria, it was not included in the search formula because the acronym is used in other fields. Antimicrobial include antibiotics, however it was not included in the search formula, because antimicrobial is not only effective against ARB, it is also effective against mycoplasma, chlamydia, viruses, etc. Articles from Scotland, Wales, England, and Northern Ireland are included as papers from the UK. Each journal's impact factor is derived from the 2020 JCR. Not all relevant articles were included in this analysis, and those that did not match the search rules were excluded. In this review DDA has been used to make matrix map, cluster map, bubble chart and cross-correlation plot. Since publications are time-sensitive, this paper only analyzed the literature published from 2010 to 2020.
Results
From 2010 to 2020, 2,823 papers in the ARB field were published by authors in 116 countries, including 99 ESI highly cited papers and 3 ESI hot papers. These publications can be divided into 11 languages, including 2,793 in English (98.94%), 10 in German (0.35%), 6 in Spanish (0.213%), 3 in French and Polish (0.106%), 2 in Hungarian and Portuguese (0.071%), and 1 in Chinese, Dutch, Italian and Turkish (0.035%). The growth trend of articles related to the ARB field from 2010 to 2020 was described (Figure 1). During this period, the number of articles published in this field increased by more than seven-fold, with the number of articles published from 2018 to 2020 increasing significantly. This finding indicates that ARB has attracted increasing concern year by year, and it also shows that the impact of ARB on human beings is increasing.
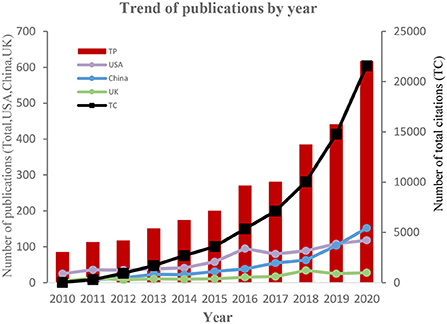
Figure 1. Trends in the number of published articles related to ARB by year. TP, total papers; TC, total citations.
Contribution of leading countries/regions
The top 20 countries in terms of total quantity of publications in the ARB field between 2010 and 2020 were identified (Table 1). The USA is the country with the most publications in this field, followed by China and the UK, whose publications account for 25.61, 18.17, and 6.23% of the total publications, respectively. The same result can be seen in the ranking of total citations; that is, the USA is first, followed by China and the UK. Figure 2 shows the number of ARB-related publications per year from 2010 to 2020 in the USA, China and the UK. It can be seen that China issued very few publications from 2010 to 2013, less than the UK and the USA, while in 2019 the number of publications in China rose significantly. In 2020 China has already surpassed the USA in the number of relevant publications. This indicates that China is considerably more active in this research field during recent years. It is likely related to the large population in China, the high prevalence of antibiotic abuse (142), the relevant policies (143, 144) and higher scientific research fund support (145). Among the top 20 countries, 11 countries were in Europe, 5 countries were in Asia, and 4 countries were in the Americas, which shows that ARB have attracted global attention.
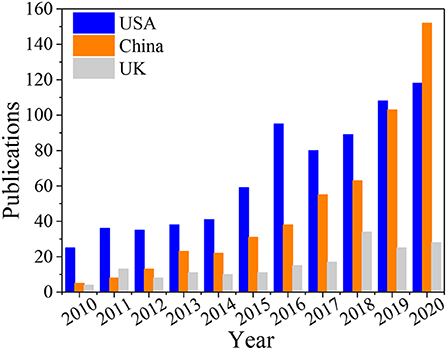
Figure 2. Number of ARB-related publications per year from 2010 to 2020 in the USA, China and the UK.
Cooperation of leading countries/regions
The most impactful science comes from international collaboration (146), which is based on the flow and integration of knowledge. Different countries/regions may have different emphases when studying ARB, although resource complementarity and continuous innovation impulses can be achieved by collaboration. International collaborative publications are joint papers written by scholars from multiple countries. The number of cooperative countries (nCC) refers to how many countries a country has cooperated with in a certain field. It can be concluded from Table 1 that among all countries, the USA, the UK, Germany, Spain and France have more cooperation with other countries. To better understand the current state of international collaboration in the ARB field, a network graph between the top 10 countries/regions was created using the DDA software (Figure 3). The circle size symbolizes the countries' contributions, the lines connecting the circles indicate cooperation between countries, and the thickness of the lines indicates the number of collaborative publications. It can be seen from Figure 3 that almost all of the top 10 countries in publications have ever cooperated with each other. The line between the USA and China is the thickest, which indicates that the number of cooperative publications between the USA and China is the largest in this field, followed by the number of cooperative publications between the USA and Canada.
Contribution of leading institutions
Statistics on the contributions of leading institutions can help us identify the most authoritative professional institutions in the ARB field. There are 3,430 institutions involved in ARB research, and the top 20 are summarized (Table 2). Among these 20 institutions, there are 40% institutions in Europe and Asia, respectively, while the majority of those in Asia are from China. Although the Chinese Acad Sci has published a large amount of articles, the total citations and average citations per paper are not the highest. Although several European institutes do not have a large number of publications, such as Univ Catolica Portuguesa and Univ Cyprus, the quality of articles is relatively high, which can be seen from their high total citations and average citations per paper.
The output and quality of scientific research were positively correlated with the degree of international collaboration (147). A cluster map of the collaboration among the top 15 institutions was created with DDA software (Figure 4). Obviously, Gothenburg University, the Chinese Acad Sci and Tsinghua University showed the most extensive collaborations with other institutions in the ARB field. In addition, the USDA ARS, Karolinska Inst and Univ Queensland have a greater number of collaborations with institutions in different countries; thus, their degree of internationalization was high. The collaborations between the Chinese Acad Sci and Univ Chinese Acad Sci and between Univ Porto and Univ Catolica Portuguesa were the most frequent. Institutions in European countries were more closely connected with those in neighboring countries/regions, which was similar to that in Asia, possibly because of factors such as institutional relationships and geographical proximity.
Contribution of leading journals
The collation of published journals revealed that a total of 983 journals published ARB-related research from 2010 to 2020. The top 30 journals by the number of articles are displayed (Table 3). These 30 journals have published a total of 911 articles on ARB, accounting for 45.86% of the total literature. Forty-three percent of these journals were related to the environment, 20% were related to microbiology, 13% were related to medicine, 10% were related to engineering technology, and 3% was related to materials and chemistry each. The breadth of disciplines involved reflects that ARB represent an interdisciplinary research field.
Contribution of leading authors
Statistics on leading authors can help us understand the top experts in the ARB field. A total of 13,966 authors were counted among 2,823 articles, of which 12,086 authors only published one article, 337 authors published three articles, and 15 authors published 10 or more articles. The top 20 authors in the number of articles and their institutions are summarized (Table 4). These authors published 245 articles, accounting for 8.67% of all articles. CM Manaia has published the most articles in this field and made important contributions to the presence and removal process of antibiotics, ARB and ARG in wastewater and antibiotic resistance in the environment. L Rizzo mainly studied sewage treatment processes, such as photocatalysis and UV. In addition to the study of sewage treatment processes, D Fatta-Kassinos also contributed to the reuse of wastewater.
Contribution of leading research areas
Statistics on the research areas can help us grasp the shift of research emphasis in a specific field. There are 90 study areas associated with ARB, and the top 20 based on the number of articles are concluded (Table 5). The research areas of ARB are not only related to microorganisms, diseases, drugs, and chemistry but also related to the environment, engineering, agriculture, materials and oceanography, with the greatest number of publications related to the ecological environment. The top 5 areas accounted for 76.83% of all articles published, indicating that the environment, microbiology, engineering, drug and chemistry are the top research areas in the ARB field.
The bubble chart can show the research trends and emphasis in a specific field more stereoscopically (205). A bubble chart is depicted to showing the top 20 ARB research areas (Figure 5). The numbers on the bubbles reflect the number of publications. “Environmental Sciences and Ecology” is the dominant research direction in the ARB field. From 2010 to 2020, the number of publications in this field increased and was the greatest overall, and it showed significant annual growth since 2017. “Microbiology” is also a research direction of increasing concern. The number of publications related to “Microbiology” every year is also on the rise, although a certain gap is observed. Compared with “Environmental Sciences and Ecology,” “Microbiology” received greater attention in the initial stage. Previously, the number of publications in the “Engineering” direction increased slowly but substantially between 2018 and 2020. The number of publications related to “Materials Science” was low in the initial phase but increased significantly after 2015, reaching a peak in the last 2 years.
Analysis of author keywords
A keyword collection based on abundant academic findings in a research field over a long period of time can reveal the overall characteristics, developmental trends, and internal connections of such research. The top 30 author keywords from 2,823 publications were sorted and displayed in a bubble chart (206–209) in this study (Figure 6). The number on the bubble represents the times that the author keywords appeared in the corresponding year. In this paper, we combined author keywords with the same meaning through the DDA. Eventually, a total of 5,506 author keywords were obtained. Among them, 4,276 author keywords appeared only once, which accounted for 77.67%; 573 author keywords appeared twice, which accounted for 10.41%; and 6 author keywords appeared more than 100 times, which accounted for ~0.11%. Among them, “Antibiotic resistance,” “Antibiotic-resistant bacteria,” “Antibiotics,” and “Antibiotic resistance genes” had the highest appearance frequency. Much of the research on “Antibiotic resistance” has focused on the existence of “Antibiotics,” “Antibiotic-resistant bacteria,” and “Antibiotic resistance genes” in “Wastewater” and the environment and associated removal techniques. There are also many related studies on “Antibiotics,” “Antimicrobials,” “Antimicrobial peptides,” “MRSA,” “Nanoparticles,” and “Muti-drug resistant bacteria”.
The cross-correlation plot shows that two keywords occurred in one paper at the same time. Through the co-occurrence analysis of author keywords, the cross-connection between each author keywords can be better revealed. We designed a cross-correlation plot of the leading 30 author keywords by DDA (Figure 7). The size of the circle reflects how frequently the author keywords appear in total articles; the line connecting the two circles indicates that the two author keywords appear in the same article. The dashed line indicates a correlation between the two author keywords ranging from 0.25 to 0.5, and the solid line means 0.5–0.75. Undoubtedly, the author keywords with the highest frequency also correspond to the largest circles. We can also clearly discover that the author keywords appearing at the same time as “Antibiotic resistance” are the most, indicating that their research scope is wider. Among them, “Antibiotic-resistant bacteria” and “Antibiotic resistance genes,” “Resistance” and “Antibiotics,” “Phage therapy” and “Bacteriophage,” “Enterobacteriaceae” and “ESBL”, and “Antibiotic resistance genes” and “Tetracycline” are five pairs of closely related keywords, indicating that those two keywords had a high frequency of appearing simultaneously in an article.
Analysis of ESI highly cited papers
The frequency of citations is a valuable metric for evaluating the impact of scientific papers (210, 211). The ESI highly cited papers refer to papers published in the last decade that presented a citation frequency ranked within the top 1% worldwide within the previous 2 months. Therefore, this paper adopts ESI highly cited papers to explore the hot topics of recent studies. The top 20 most cited papers in the ARB field from 2010 to 2020 are revealed (Table 6). Among these papers, the USA contributed 4 papers and the UK, Sweden and China each contributed 3 papers. Investigations to determine how antibiotic resistance develops in bacteria is the most frequently subject. Studies have focused on the main mechanisms of antibiotic resistance. The impact of ARB infection on humans is also of particular concern. In 2015, ARB infections were estimated to cause numerous deaths in Europe, with a high burden in infants and elderly individuals. Antibiotic resistance in wastewater has been a hot research topic in the last decade, with many studies related to Enterococcus and Escherichia coli. In addition, Acinetobacter baumannii, Pseudomonas aeruginosa (218), vancomycin-resistant Enterococcus (VRE) and methicillin-resistant Staphylococcus aureus (MRSA) (219) have a relatively large impact on humans and have recently received more attention. Guidelines for biological risk assessments of ARB production and transmission in the environment have also been controversial subjects in recent years because of their important roles in controlling antibiotic resistance in the environment.
Analysis of ESI hot papers
ESI hot papers are papers published in the last 2 years that have a citation frequency ranked within the top 0.1% worldwide in the previous 2 months. Three ESI hot papers published in 2020 were identified (Table 7). The hottest papers in the last 2 years describe the generation and fate of antibiotics, ARB and ARGs in sewage treatment plants around the world. The second paper reviews the research progress of antimicrobial nanofiber wound dressings since 2015, especially recent advances in biohybrid dressings made from cross species. The last hot paper summarizes the physicochemical properties of 5 photothermal agents and their application in antimicrobial photothermal therapy.
Latest developments
From January 2021 to 2022, 19 highly cited papers in total met the search conditions, among which 2 were hot papers. The research contents of these highly cited papers mainly focus on the three aspects as follows. Initially, there are many researches on substances and preparations that can play an antibacterial role. For example, the antibacterial mechanism of nanomaterials (222), and molecularly imprinted polymers (223), the research review of antibacterial peptides in the source, structure, clinical trials (224), etc., the mechanism of prebiotics to remove intestinal pathogens (225), as well as the activity and antibacterial mechanism of antimicrobial agents from plants (226). Secondly, there are also many studies on the existence of micro pollutants, including the distribution and concentration of antibiotic resistance genes in the environment (227), the pollution status, sources and potential risks of antibiotics in surface water (228), and the production and removal of resistant microorganisms in hospital wastewater (229). What's more, these studies also touched upon aspects of water treatment technology, such as the mechanism of action of photocatalytic removal of antibiotics and inactivated bacteria (230), the effect of ozone removal of ARB and ARGs (231), and the overview of microalgae for environmental remediation (232).
Discussion
Emerging research elements
According to the statistical analysis of author keywords from 2010 to 2020, new author keywords have emerged in this field. Since the new author keywords appear less frequently, which has not shown in the chart. Here only introduce the new author keywords that appear comparatively more frequently. The 2019 COVID-19 pandemic, triggered by SARS-CoV-2 (233–236), has placed a tremendous burden on both the health care system and human society (237–239). It was found that the incidence of carbapenem-resistant Enterobacteriaceae infections have rapidly increased in critically ill patients with COVID-19 (240). Surprisingly, maintaining social distance has been shown to help reduce the transmission of SARS-CoV-2 and ARB (241). In addition, polypeptides are not only potential substitutes for the treatment of ARB infection but are also effective in the treatment of COVID-19 (242). Nanoparticles are not only effective antibacterial agents but also antibacterial drug delivery carriers. Electrospinning represents a new technology for preparing nanofibers in the last 2 years, and it is very suitable for generating antibacterial nanomaterials because nanomaterials produced using this technology have a large specific surface area and controllable structure (221, 243). In the past 2 years, studies have linked machine learning with ARB identification. Compared with traditional DNA sequencing, spectral diagnostic data are analyzed by machine learning algorithms to accurately identify ARB and ARGs (244, 245). In addition, studies have applied machine learning models for the early prediction of subclinical mastitis to reduce the risk of ARB (246).
Future research directions
It is well known that the goal of studying antibiotic resistant bacteria is to resist ARB by understanding the mechanisms of the generation, evolution as well as transmission of the antibiotic resistance, such as the implement of sewage treatment processes; to find effective methods to reduce the harm caused by antibiotic resistant bacteria to global humankind and ecosystem, such as the research and development of new antibiotics, antibiotic substitutes, adjuvants.
According to the author keywords bubble chart (Figure 6), cross-correlation graph (Figure 7) and ESI highly cited papers (Table 6), it can be found that the research on antibiotic resistance has been the first place and plays a leading role in this field for the last decade. The scope of research mainly includes the existence of antibiotic resistance in the aquatic systems (247), sewage treatment processes, and negative effects (248, 249). This may be related to the early abuse of antibiotics (250) in many countries, such as China (142, 251–254), USA (255, 256), India (257), Italy (258), and so on. It is undeniable that those studies play a significant role in the understanding of antibiotic resistance. However, some studies have pointed out that MRSA existed long before the antibiotics was used (259). Mutations in microbial metabolism can also lead to antibiotic resistance (260). This just goes to show that our understanding of antibiotic resistance is not thorough enough. Further research on the induction factors and relevant mechanisms that lead to antibiotic resistance is required in the future.
According to the ESI hot papers (Table 7), nanomaterials have been the hottest topic in this field in the last 2 years, which is closely related to their superior antibacterial properties. However, according to the author keywords bubble chart (Figure 6) and cross-correlation graph (Figure 7), it can be found that the research on antimicrobial peptides and bacteriophages has gradually increased in the last decade but has not received enough attention. Peptide-based antibiotics have been found to be effective against MDRB because bacterial resistance responds slowly to the action mode of peptide natural products (261). Encrypted peptide kills bacteria by targeting the cell membranes of pathogenic bacteria and is not susceptible to selective resistance (262). At present, research has found candidate peptide antibiotics in human intestinal flora using machine learning (263), which breaks through the path dependence on the traditional antibiotic discovery. Bacteriophages have been found in human intestines either, which are in a harmonious symbiotic relationship with intestinal flora, rather than an antagonistic mode (264). Bacteriophage related therapies are in the concern once more (265). In addition, there has been also some progress in the relationship between intestinal flora and antibiotic resistance (266), the effect of antibiotics on intestinal flora (267), the effect of vaccines on antibiotic resistance (268), and antibiotic-resistant bacterial inhibitors (269). However, these studies are not thorough enough (270, 271). Therefore, it is necessary to pay attention to the diversification of research and strengthen the research on antibiotic substitutes, human intestinal flora and adjuvants in the future.
Antibiotic resistance imposes a heavy burden on human beings. A study on the worldwide burden of antibiotic resistance (272) found that the mortality in the whole age interval caused by antibiotic resistance is the highest in the Africa. Pseudomonas aeruginosa, MRSA and other MDRB have caused a large number of deaths. This suggests that low-resource settings bear the heaviest burden, which is consistent with the statistical analysis of this study in the leading countries or regions (Table 1), leading institutions (Table 2) and leading authors (Table 4). Although countries in Africa have made some contributions in this field (273–278), the relevant research is not sufficient and is not in the leading position, the understanding of antibiotic resistant bacteria is not enough. According to the author keywords bubble chart (Figure 6), it can be found that MRSA, Pseudomonas aeruginosa and other MDRB have received more attention in recent 2 years (279). The extremely strong resistance not only causes great losses to humans, but also threatens the existing antibiotics. Studies have shown that the COVID-19 pandemic has led to overuse of antibiotics in many areas, which will aggravate the antibiotic resistance (280, 281). Therefore, every country needs to establish strict antibiotic prescription guidelines to regulate antibiotic use. However, one study has shown that reducing antibiotic prescriptions cannot stop the spread of antibiotic resistant (282). There is a gap between antibiotic stewardship in the paper and in practice (283). Even treatments that match susceptibility of pathogens may result in resistance, because the development of antibiotic resistance is essentially driven by rapid re-infection of different strains of the patient with prescription resistance (284), and they suggest that the personalized antibiotic treatment suggestions can be given by predicting the patient's past infection or history using the machine learning, thus reducing the emergence of ARB. However, ARB can circulate and transfer between humans and animals. Therefore, it is not enough to reduce the propagation of antibiotic resistance by simply managing the use of antibiotic in human beings. There is no boundary among environment, animal and human beings. The control of antibiotic resistance requires simultaneous communication and cooperation of these three fields, rather than the separation of them (285).
In conclusion, this research proposed the possible future research direction in the field of ARB by starting from the aspects of controlling the transmission of ARB and developing new antibiotics. Aspect of relevant research on new antibacterial agents: As peptide-based antibiotics have potential to defend against the ARB, many scholars are paying attention to its design and development (286–288). However, studies show that some problems occur after this kind of antibiotics are used, for example, it causes short half lives in vivo, protease degradation and others (289). Therefore, the research on the interaction between peptide-based antibiotics and human bodies (290, 291) and the decoration of its chemical structure (261) shall be further conducted in the future. In addition, it is inevitable for peptide-based antibiotics to become drug-resistant, despite its relatively low possibility of becoming antibiotic resistant. So, it is required to concern how to limit the drug resistance rate of new peptide-based antibiotics in the future. In the future, it is possible to research how to use bacteriophages to recover the complexity of damaged microbiota and how to use bacteriophages to operate HGT microbial genomes in microbial flora from the mutual beneficial aspect between intestinal bacteria and bacteriophages (264). Aspect of controlling the transmission of ARB: In conclusion, corresponding measures shall be taken on three aspects including humans, animals and environment to control the transmission of ARB in the future. On the aspect of humans, concerning the gap between antibiotics management and research and the actual situation (283), it is required to research the actual using condition antibiotics in humans across the world. In addition, it is equally important to reduce the use of antibiotics so as to control the generation and transmission of antibiotic resistance, especially in countries short of resources (292). Therefore, it is demanded to research the measures on how to reduce the use of antibiotics in the future, for instance, to develop relevant vaccines or hygiene system (293, 294), etc. On the aspect of environment, wastewater can transmit ARB and ARGs not only to humans, but also to the ecological environment (19). Despite the growing number of studies on sewage treatment, there is still a lack of a unified standard and program for sewage treatment. In terms of animal husbandry and aquaculture, a global policy is required to control the use of antibiotics on animals and prevent the ARB and ARGs from spreading to humans through food chains (295). What's more, we should also research how to use and manage antibiotics jointly from the three aspects of humans, animals and environment. It is possible to develop toward the direction of constructing the biological risk assessment platform (296) and electronic monitoring system (293, 297).
Conclusions
In this study, we provided a research overview of the field of ARB. Over time, ARB have become a global threat, and an increasing amount of related research has been carried out. Both developed countries, represented by the USA, and developing countries, represented by China, have made significant contributions to this field. There are relatively few relevant studies from Africa, but antibiotic-resistant bacterial infections in Africa are of great concern (298). ARB represent an interdisciplinary research field, with most studies focused on environmental and microbial aspects. Particularly, antibiotic resistance is not only a research focus in this field but also a research hotspot. Although some progress has been made with novel antibiotics, further research is still needed (299–301). In the future, we can strengthen the financial support (302) and technical and knowledge cooperation (303) for the research and development of new antibacterial drugs (304–306), etc. In this case, bacteriocins, phage therapy, nanomaterials, human intestinal flora and machine learning have inspired hope for the treatment of ARB infection. However, further relevant studies are still needed in the future. Since 2021–2022 related publications are not included, this study provides an overview of the latest research progress in this field based on the 2021–2022 ESI highly cited papers in the field of ARB.
Certain limitations were observed in this study. For example, articles without authors keywords were not included in the analysis. In summary, this study will hopefully inspire researchers in the field of ARB and assist them in further understanding the research trends, research hotspots, and future research directions in this field. Although WOS has covered many publications, however, some publications from database such as Scopus, PubMed, may not be included in this study.
Author contributions
YW and QZ contributed to the conception and design of the study and wrote the first draft of the manuscript. QZ organized the database and performed the statistical analysis. GS and DD reviewed and edited the manuscript. GS and ZD provided financial support. All authors contributed to manuscript revision, read, and approved the submitted version.
Funding
This research was funded by Science Technology Department of Zhejiang Province (No. 2022C25007).
Conflict of interest
The authors declare that the research was conducted in the absence of any commercial or financial relationships that could be construed as a potential conflict of interest.
Publisher's note
All claims expressed in this article are solely those of the authors and do not necessarily represent those of their affiliated organizations, or those of the publisher, the editors and the reviewers. Any product that may be evaluated in this article, or claim that may be made by its manufacturer, is not guaranteed or endorsed by the publisher.
References
1. Coates A, Hu Y, Bax R, Page C. The future challenges facing the development of new antimicrobial drugs. Nat Rev Drug Discov. (2002) 1:895–910. doi: 10.1038/nrd940
2. Kuenzli E, Jaeger VK, Frei R, Neumayr A, DeCrom S, Haller S, et al. High colonization rates of extended-spectrum beta-lactamase (Esbl)-producing Escherichia coli in Swiss travellers to South Asia- a prospective observational multicentre cohort study looking at epidemiology, microbiology and risk factors. BMC Infect Dis. (2014) 14:528. doi: 10.1186/1471-2334-14-528
3. Wang Z, Han M, Li E, Liu X, Wei H, Yang C, et al. Distribution of antibiotic resistance genes in an agriculturally disturbed lake in China: their links with microbial communities, antibiotics, and water quality. J Hazard Mater. (2020) 393:122426. doi: 10.1016/j.jhazmat.2020.122426
4. Sapkota AR, Curriero FC, Gibson KE, Schwab KJ. Antibiotic-resistant Enterococci and fecal indicators in surface water and groundwater impacted by a concentrated swine feeding operation. Environ Health Perspect. (2007) 115:1040–5. doi: 10.1289/ehp.9770
5. Magiorakos AP, Srinivasan A, Carey RB, Carmeli Y, Falagas ME, Giske CG, et al. Multidrug-resistant, extensively drug-resistant and pandrug-resistant bacteria: an international expert proposal for interim standard definitions for acquired resistance. Clin Microbiol Infect. (2012) 18:268–81. doi: 10.1111/j.1469-0691.2011.03570.x
6. Blair JM, Webber MA, Baylay AJ, Ogbolu DO, Piddock LJ. Molecular mechanisms of antibiotic resistance. Nat Rev Microbiol. (2015) 13:42–51. doi: 10.1038/nrmicro3380
7. Zhang J, Zheng Y, Yang Y. Antibiotic prescription patterns in children and neonates in China. Lancet Glob Health. (2019) 7:e1496. doi: 10.1016/S2214-109X(19)30406-1
8. Al-Halawa DA, Sarama R, Abdeen Z, Qasrawi R. Knowledge, attitudes, and practices relating to antibiotic resistance among pharmacists: a cross-sectional study in the West Bank, Palestine. Lancet. (2019) 393:S7. doi: 10.1016/S0140-6736(19)30593-8
9. Fleming-Dutra KE, Hersh AL, Shapiro DJ, Bartoces M, Enns EA, File TM Jr, et al. Prevalence of inappropriate antibiotic prescriptions among us ambulatory care visits, 2010-2011. JAMA. (2016) 315:1864–73. doi: 10.1001/jama.2016.4151
10. Andersson DI, Hughes D. Microbiological effects of sublethal levels of antibiotics. Nat Rev Microbiol. (2014) 12:465–78. doi: 10.1038/nrmicro3270
11. Andersson DI, Hughes D. Evolution of antibiotic resistance at non-lethal drug concentrations. Drug Resist Updat. (2012) 15:162–72. doi: 10.1016/j.drup.2012.03.005
12. Davies J, Davies D. Origins and evolution of antibiotic resistance. Microbiol Mol Biol Rev. (2010) 74:417–33. doi: 10.1128/MMBR.00016-10
13. Michael-Kordatou I, Karaolia P, Fatta-Kassinos D. The role of operating parameters and oxidative damage mechanisms of advanced chemical oxidation processes in the combat against antibiotic-resistant bacteria and resistance genes present in urban wastewater. Water Res. (2018) 129:208–30. doi: 10.1016/j.watres.2017.10.007
14. Makowska N, Koczura R, Mokracka J. Class 1 integrase, sulfonamide and tetracycline resistance genes in wastewater treatment plant and surface water. Chemosphere. (2016) 144:1665–73. doi: 10.1016/j.chemosphere.2015.10.044
15. Auerbach EA, Seyfried EE, McMahon KD. Tetracycline resistance genes in activated sludge wastewater treatment plants. Water Res. (2007) 41:1143–51. doi: 10.1016/j.watres.2006.11.045
16. Karkman A, Do TT, Walsh F, Virta MP. Antibiotic-resistance genes in waste water. Trends Microbiol. (2018) 26:220–8. doi: 10.1016/j.tim.2017.09.005
17. Udikovic-Kolic N, Wichmann F, Broderick NA, Handelsman J. Bloom of resident antibiotic-resistant bacteria in soil following manure fertilization. Proc Natl Acad Sci U S A. (2014) 111:15202–7. doi: 10.1073/pnas.1409836111
18. Rizzo L, Manaia C, Merlin C, Schwartz T, Dagot C, Ploy MC, et al. Urban wastewater treatment plants as hotspots for antibiotic resistant bacteria and genes spread into the environment: a review. Sci Total Environ. (2013) 447:345–60. doi: 10.1016/j.scitotenv.2013.01.032
19. Hultman J, Tamminen M, Parnanen K, Cairns J, Karkman A, Virta M. Host range of antibiotic resistance genes in wastewater treatment plant influent and effluent. FEMS Microbiol Ecol. (2018) 94:fiy038. doi: 10.1093/femsec/fiy038
20. Martinez JL. The role of natural environments in the evolution of resistance traits in pathogenic bacteria. Proc Biol Sci. (2009) 276:2521–30. doi: 10.1098/rspb.2009.0320
21. Wu N, Qiao M, Zhang B, Cheng W-D, Zhu Y-G. Abundance and diversity of tetracycline resistance genes in soils adjacent to representative swine feedlots in China. Environ Sci Technol. (2010) 44:6933–9. doi: 10.1021/es1007802
22. Van Boeckel TP, Pires J, Silvester R, Zhao C, Song J, Criscuolo NG, et al. Global trends in antimicrobial resistance in animals in low-and middle-income countries. Science. (2019) 365:eaaw1944. doi: 10.1126/science.aaw1944
23. Cassini A, Högberg LD, Plachouras D, Quattrocchi A, Hoxha A, Simonsen GS, et al. Attributable deaths and disability-adjusted life-years caused by infections with antibiotic-resistant bacteria in the Eu and the European economic area in 2015: a population-level modelling analysis. Lancet Infect Dis. (2019) 19:56–66. doi: 10.1016/s1473-3099(18)30605-4
24. de Kraker ME, Davey PG, Grundmann H, Group BS. Mortality and hospital stay associated with resistant Staphylococcus aureus and Escherichia coli bacteremia: estimating the burden of antibiotic resistance in Europe. PLoS Med. (2011) 8:e1001104. doi: 10.1371/journal.pmed.1001104
25. Jernigan JA, Hatfield KM, Wolford H, Nelson RE, Olubajo B, Reddy SC, et al. Multidrug-resistant bacterial infections in us hospitalized patients, 2012–2017. N Engl J Med. (2020) 382:1309–19. doi: 10.1056/NEJMoa1914433
26. Grenni P, Ancona V, Barra Caracciolo A. Ecological effects of antibiotics on natural ecosystems: a review. Microchem J. (2018) 136:25–39. doi: 10.1016/j.microc.2017.02.006
27. Peabody MA, Van Rossum T, Lo R, Brinkman FS. Evaluation of shotgun metagenomics sequence classification methods using in silico and in vitro simulated communities. BMC Bioinformatics. (2015) 16:362. doi: 10.1186/s12859-015-0788-5
28. Fontana C, Favaro M, Minelli S, Bossa MC, Testore GP, Leonardis F, et al. Acinetobacter baumannii in intensive care unit: a novel system to study clonal relationship among the isolates. BMC Infect Dis. (2008) 8:79. doi: 10.1186/1471-2334-8-79
29. Sanganyado E, Gwenzi W. Antibiotic resistance in drinking water systems: occurrence, removal, and human health risks. Sci Total Environ. (2019) 669:785–97. doi: 10.1016/j.scitotenv.2019.03.162
30. Wu Q, Li S, Zhao X, Zhao X. Interaction between typical sulfonamides and bacterial diversity in drinking water. J Water Health. (2018) 16:914–20. doi: 10.2166/wh.2018.210
31. Pendleton JN, Gorman SP, Gilmore BF. Clinical relevance of the eskape pathogens. Expert Rev Anti Infect Ther. (2013) 11:297–308. doi: 10.1586/eri.13.12
32. Ashbolt NJ, Amézquita A, Backhaus T, Borriello P, Brandt KK, Collignon P, et al. Human health risk assessment (Hhra) for environmental development and transfer of antibiotic resistance. Environ Health Perspect. (2013) 121:993–1001. doi: 10.1289/ehp.1206316
33. Chamosa LS, Álvarez VE, Nardelli M, Quiroga MP, Cassini MH, Centrón D. Lateral Antimicrobial Resistance Genetic Transfer Is Active in the Open Environment. Sci Rep. (2017) 7:513. doi: 10.1038/s41598-017-00600-2
34. Boucher HW, Talbot GH, Benjamin DK Jr, Bradley J, Guidos RJ, Jones RN, et al. 10 X ‘20 progress–development of new drugs active against gram-negative bacilli: an update from the Infectious Diseases Society of America. Clin Infect Dis. (2013) 56:1685–94. doi: 10.1093/cid/cit152
35. Tacconelli E, Carrara E, Savoldi A, Harbarth S, Mendelson M, Monnet DL, et al. Discovery, research, and development of new antibiotics: the who priority list of antibiotic-resistant bacteria and tuberculosis. Lancet Infect Dis. (2018) 18:318–27. doi: 10.1016/s1473-3099(17)30753-3
36. Stokes JM, Yang K, Swanson K, Jin W, Cubillos-Ruiz A, Donghia NM, et al. A deep learning approach to antibiotic discovery. Cell. (2020) 180:688–702. doi: 10.1016/j.cell.2020.01.021
37. Hover BM, Kim SH, Katz M, Charlop-Powers Z, Owen JG, Ternei MA, et al. Culture-independent discovery of the malacidins as calcium-dependent antibiotics with activity against multidrug-resistant gram-positive pathogens. Nat Microbiol. (2018) 3:415–22. doi: 10.1038/s41564-018-0110-1
38. Walsh C. Where will new antibiotics come from? Nat Rev Microbiol. (2003) 1:65–70. doi: 10.1038/nrmicro727
39. Fowler VG Jr, Boucher HW, Corey GR, Abrutyn E, Karchmer AW, Rupp ME, et al. Daptomycin versus standard therapy for bacteremia and endocarditis caused by Staphylococcus aureus. N Engl J Med. (2006) 355:653–65. doi: 10.1056/NEJMoa053783
40. Steenbergen JN, Alder J, Thorne GM, Tally FP. Daptomycin: a lipopeptide antibiotic for the treatment of serious gram-positive infections. J Antimicrob Chemother. (2005) 55:283–8. doi: 10.1093/jac/dkh546
41. Tsiodras S, Gold HS, Sakoulas G, Eliopoulos GM, Wennersten C, Venkataraman L, et al. Linezolid resistance in a clinical isolate of Staphylococcus aureus. Lancet. (2001) 358:207–8. doi: 10.1016/S0140-6736(01)05410-1
42. Gonzales RD, Schreckenberger PC, Graham MB, Kelkar S, DenBesten K, Quinn JP. Infections due to vancomycin-resistant enterococcus faecium resistant to linezolid. Lancet. (2001) 357:1179. doi: 10.1016/S0140-6736(00)04376-2
43. Cui L, Tominaga E, Neoh H-m, Hiramatsu K. Correlation between reduced daptomycin susceptibility and vancomycin resistance in vancomycin-intermediate Staphylococcus aureus. Antimicrob Agents Chemother. (2006) 50:1079–82. doi: 10.1128/AAC.50.3.1079-1082.2006
44. Chen Y, Gao Y, Chen Y, Liu L, Mo A, Peng Q. Nanomaterials-based photothermal therapy and its potentials in antibacterial treatment. J Control Release. (2020) 328:251–62. doi: 10.1016/j.jconrel.2020.08.055
45. Gandhi TN, Malani PN. Combination therapy for methicillin-resistant Staphylococcus aureus bacteremia: not ready for prime time. JAMA. (2020) 323:515–6. doi: 10.1001/jama.2019.21472
46. Tyers M, Wright GD. Drug combinations: a strategy to extend the life of antibiotics in the 21st century. Nat Rev Microbiol. (2019) 17:141–55. doi: 10.1038/s41579-018-0141-x
47. Czaplewski L, Bax R, Clokie M, Dawson M, Fairhead H, Fischetti VA, et al. Alternatives to antibiotics—a pipeline portfolio review. Lancet Infect Dis. (2016) 16:239–51. doi: 10.1016/S1473-3099(15)00466-1
48. Kortright KE, Chan BK, Koff JL, Turner PE. Phage therapy: a renewed approach to combat antibiotic-resistant bacteria. Cell Host Microbe. (2019) 25:219–32. doi: 10.1016/j.chom.2019.01.014
49. Mcvay CS, Velasquez M, Fralick JA. Phage therapy of pseudomonas aeruginosa infection in a mouse burn wound model. Antimicrob Agents Chemother. (2007) 51:1934–8. doi: 10.1128/AAC.01028-06
50. Krylov V, Shaburova O, Pleteneva E, Bourkaltseva M, Krylov S, Kaplan A, et al. Modular approach to select bacteriophages targeting Pseudomonas aeruginosa for their application to children suffering with cystic fibrosis. Front Microbiol. (2016) 7:1631. doi: 10.3389/fmicb.2016.01631
51. Gupta A, Mumtaz S, Li CH, Hussain I, Rotello VM. Combatting antibiotic-resistant bacteria using nanomaterials. Chem Soc Rev. (2019) 48:415–27. doi: 10.1039/C7CS00748E
52. Barros CHN, Fulaz S, Stanisic D, Tasic L. Biogenic nanosilver against multidrug-resistant bacteria (Mdrb). Antibiotics. (2018) 7:69. doi: 10.3390/antibiotics7030069
53. Huang YS, Wang JT, Tai HM, Chang PC, Huang HC, Yang PC. Metal nanoparticles and nanoparticle composites are effective against haemophilus influenzae, Streptococcus pneumoniae, and multidrug-resistant bacteria. J Microbiol Immunol Infect. (2022) 55:708–15. doi: 10.1016/j.jmii.2022.05.003
54. Chen Y, Ren L, Sun L, Bai X, Zhuang G, Cao B, et al. Amphiphilic silver nanoclusters show active nano–bio interaction with compelling antibacterial activity against multidrug-resistant bacteria. NPG Asia Mater. (2020) 12:56. doi: 10.1038/s41427-020-00239-y
55. Cotter PD, Ross RP, Hill C. Bacteriocins - a viable alternative to antibiotics? Nat Rev Microbiol. (2013) 11:95–105. doi: 10.1038/nrmicro2937
56. Pamer EG. Resurrecting the intestinal microbiota to combat antibiotic-resistant pathogens. Science. (2016) 352:535–8. doi: 10.1126/science.aad9382
57. Hamilton-Miller JMT. The emergence of antibiotic resistance: myths and facts in clinical practice. Intens Care Med. (1990) 16:S206–S11. doi: 10.1007/BF01709702
58. Husevag B, Lunestad BT, Johannessen PJ, Enger O, Samuelsen OB. Simultaneous occurrence of vibrio salmonicida and antibiotic-resistant bacteria in sediments at abandoned aquaculture sites. J Fish Dis. (1991) 14:631–40. doi: 10.1111/j.1365-2761.1991.tb00621.x
59. Sandaa R-A, Torsvik VL, Goksoyr J. Transferable drug resistance in bacteria from fish-farm sediments. Can J Microbiol. (1992) 38:1061–5. doi: 10.1139/m92-174
60. McKeon DM, Calabrese JP, Bissonnette GK. Antibiotic resistant gram-negative bacteria in rural groundwater supplies. Water Res. (1995) 29:1902–8. doi: 10.1016/0043-1354(95)00013-B
61. Boogaard AE, Stobberingh EE. Antibiotic usage in animals- impact on bacterial resistance and public health. Drugs. (1999) 58:589–607. doi: 10.2165/00003495-199958040-00002
62. Austin DJ, Kakehashi M, Anderson RM. The transmission dynamics of antibiotic–resistant bacteria: the relationship between resistance in commensal organisms and antibiotic consumption. Proc R Soc Lond B Biol Sci. (1997) 264:1629–38. doi: 10.1098/rspb.1997.0227
63. Gopal Rao G. Risk factors for the spread of antibiotic-resistant bacteria. Drugs. (1998) 55:323–30. doi: 10.2165/00003495-199855030-00001
64. Nyquist A-C, Gonzales R, Steiner JF, Sande MA. Antibiotic prescribing for children with colds, upper respiratory tract infections, and bronchitis. JAMA. (1998) 279:875–7. doi: 10.1001/jama.279.11.875
65. Alisky J, Iczkowski K, Rapoport A, Troitsky N. Bacteriophages show promise as antimicrobial agents. J Infect. (1998) 36:5–15. doi: 10.1016/S0163-4453(98)92874-2
66. Xi C, Zhang Y, Marrs CF, Ye W, Simon C, Foxman B, et al. Prevalence of antibiotic resistance in drinking water treatment and distribution systems. Appl Environ Microbiol. (2009) 75:5714–8. doi: 10.1128/AEM.00382-09
67. Schwartz T, Kohnen W, Jansen B, Obst U. Detection of antibiotic-resistant bacteria and their resistance genes in wastewater, surface water, and drinking water biofilms. FEMS Microbiol Ecol. (2003) 43:325–35. doi: 10.1111/j.1574-6941.2003.tb01073.x
68. Wright GD. The antibiotic resistome: the nexus of chemical and genetic diversity. Nat Rev Microbiol. (2007) 5:175–86. doi: 10.1038/nrmicro1614
69. Piddock LJ. Clinically relevant chromosomally encoded multidrug resistance efflux pumps in bacteria. Clin Microbiol Rev. (2006) 19:382–402. doi: 10.1128/CMR.19.2.382-402.2006
70. Brandl K, Plitas G, Mihu CN, Ubeda C, Jia T, Fleisher M, et al. Vancomycin-resistant enterococci exploit antibiotic-induced innate immune deficits. Nature. (2008) 455:804–7. doi: 10.1038/nature07250
71. Norman RS, Stone JW, Gole A, Murphy CJ, Sabo-Attwood TL. Targeted photothermal lysis of the pathogenic bacteria, Pseudomonas aeruginosa, with gold nanorods. Nano Lett. (2008) 8:302–6. doi: 10.1021/nl0727056
72. Lu TK, Collins JJ. Engineered bacteriophage targeting gene networks as adjuvants for antibiotic therapy. Proc Natl Acad Sci U S A. (2009) 106:4629–34. doi: 10.1073/pnas.0800442106
73. Gruson D, Hilbert G, Vargas F, Valentino R, Bebear C, Allery A, et al. Rotation and restricted use of antibiotics in a medical intensive care unit: impact on the incidence of ventilator-associated pneumonia caused by antibiotic-resistant gram-negative bacteria. Am J Respir Crit Care Med. (2000) 162:837–43. doi: 10.1164/ajrccm.162.3.9905050
74. Tao CW, Hsu BM, Ji WT, Hsu TK, Kao PM, Hsu CP, et al. Evaluation of five antibiotic resistance genes in wastewater treatment systems of swine farms by real-time Pcr. Sci Total Environ. (2014) 496:116–21. doi: 10.1016/j.scitotenv.2014.07.024
75. Messacar K, Dominguez SR. Blood Pcr testing for enteroviruses in young children. Lancet Infect Dis. (2018) 18:1299–301. doi: 10.1016/S1473-3099(18)30492-4
76. Makowska N, Philips A, Dabert M, Nowis K, Trzebny A, Koczura R, et al. Metagenomic analysis of beta-lactamase and carbapenemase genes in the wastewater resistome. Water Res. (2020) 170:115277. doi: 10.1016/j.watres.2019.115277
77. Zhao R, Yu K, Zhang J, Zhang G, Huang J, Ma L, et al. Deciphering the mobility and bacterial hosts of antibiotic resistance genes under antibiotic selection pressure by metagenomic assembly and binning approaches. Water Res. (2020) 186:116318. doi: 10.1016/j.watres.2020.116318
78. Guo J, Li J, Chen H, Bond PL, Yuan Z. Metagenomic analysis reveals wastewater treatment plants as hotspots of antibiotic resistance genes and mobile genetic elements. Water Res. (2017) 123:468–78. doi: 10.1016/j.watres.2017.07.002
79. Xu R, Yang ZH, Zheng Y, Wang QP, Bai Y, Liu JB, et al. Metagenomic analysis reveals the effects of long-term antibiotic pressure on sludge anaerobic digestion and antimicrobial resistance risk. Bioresour Technol. (2019) 282:179–88. doi: 10.1016/j.biortech.2019.02.120
80. Ding H, Qiao M, Zhong J, Zhu Y, Guo C, Zhang Q, et al. Characterization of antibiotic resistance genes and bacterial community in selected municipal and industrial sewage treatment plants beside Poyang Lake. Water Res. (2020) 174:115603. doi: 10.1016/j.watres.2020.115603
81. Hrenovic J, Ivankovic T, Ivekovic D, Repec S, Stipanicev D, Ganjto M. The fate of carbapenem-resistant bacteria in a wastewater treatment plant. Water Res. (2017) 126:232–9. doi: 10.1016/j.watres.2017.09.007
82. Singh R, Singh AP, Kumar S, Giri BS, Kim K-H. Antibiotic resistance in major rivers in the world: a systematic review on occurrence, emergence, and management strategies. J Clean Prod. (2019) 234:1484–505. doi: 10.1016/j.jclepro.2019.06.243
83. Jia S, Zhang X-X, Miao Y, Zhao Y, Ye L, Li B, et al. Fate of antibiotic resistance genes and their associations with bacterial community in livestock breeding wastewater and its receiving river water. Water Res. (2017) 124:259–68. doi: 10.1016/j.watres.2017.07.061
84. Marathe NP, Pal C, Gaikwad SS, Jonsson V, Kristiansson E, Larsson DGJ. Untreated urban waste contaminates Indian river sediments with resistance genes to last resort antibiotics. Water Res. (2017) 124:388–97. doi: 10.1016/j.watres.2017.07.060
85. Low A, Ng C, He J. Identification of antibiotic resistant bacteria community and a geochip based study of resistome in urban watersheds. Water Res. (2016) 106:330–8. doi: 10.1016/j.watres.2016.09.032
86. Tran NH, Hoang L, Nghiem LD, Nguyen NMH, Ngo HH, Guo W, et al. Occurrence and risk assessment of multiple classes of antibiotics in urban canals and lakes in Hanoi, Vietnam. Sci Total Environ. (2019) 692:157–74. doi: 10.1016/j.scitotenv.2019.07.092
87. Barros J, Igrejas G, Andrade M, Radhouani H, Lopez M, Torres C, et al. Gilthead seabream (Sparus aurata) carrying antibiotic resistant Enterococci. A potential bioindicator of marine contamination? Mar Pollut Bull. (2011) 62:1245–8. doi: 10.1016/j.marpolbul.2011.03.021
88. Bai X, Ma X, Xu F, Li J, Zhang H, Xiao X. The drinking water treatment process as a potential source of affecting the bacterial antibiotic resistance. Sci Total Environ. (2015) 533:24–31. doi: 10.1016/j.scitotenv.2015.06.082
89. Lu J, Zhang Y, Wu J, Wang J, Cai Y. Fate of antibiotic resistance genes in reclaimed water reuse system with integrated membrane process. J Hazard Mater. (2020) 382:121025. doi: 10.1016/j.jhazmat.2019.121025
90. Gao P, Mao D, Luo Y, Wang L, Xu B, Xu L. Occurrence of sulfonamide and tetracycline-resistant bacteria and resistance genes in aquaculture environment. Water Res. (2012) 46:2355–64. doi: 10.1016/j.watres.2012.02.004
91. Sapkota AR, Hulet RM, Zhang G, McDermott P, Kinney EL, Schwab KJ, et al. Lower prevalence of antibiotic-resistant Enterococci on US conventional poultry farms that transitioned to organic practices. Environ Health Perspect. (2011) 119:1622–8. doi: 10.1289/ehp.1003350
92. Brooks JP, Adeli A, McLaughlin MR. Microbial ecology, bacterial pathogens, and antibiotic resistant genes in swine manure wastewater as influenced by three swine management systems. Water Res. (2014) 57:96–103. doi: 10.1016/j.watres.2014.03.017
93. Cui P, Bai Y, Li X, Peng Z, Chen D, Wu Z, et al. Enhanced removal of antibiotic resistance genes and mobile genetic elements during sewage sludge composting covered with a semi-permeable membrane. J Hazard Mater. (2020) 396:122738. doi: 10.1016/j.jhazmat.2020.122738
94. Chen Z, Zhang W, Yang L, Stedtfeld RD, Peng A, Gu C, et al. Antibiotic resistance genes and bacterial communities in cornfield and pasture soils receiving swine and dairy manures. Environ Pollut. (2019) 248:947–57. doi: 10.1016/j.envpol.2019.02.093
95. Li H, Li B, Ma J, Ye J, Guo P, Li L. Fate of antibiotic-resistant bacteria and antibiotic resistance genes in the electrokinetic treatment of antibiotic-polluted soil. Chem Eng J. (2018) 337:584–94. doi: 10.1016/j.cej.2017.12.154
96. Gao FZ, He LY, He LX, Zou HY, Zhang M, Wu DL, et al. Untreated swine wastes changed antibiotic resistance and microbial community in the soils and impacted abundances of antibiotic resistance genes in the vegetables. Sci Total Environ. (2020) 741:140482. doi: 10.1016/j.scitotenv.2020.140482
97. Murray R, Tien Y-C, Scott A, Topp E. The impact of municipal sewage sludge stabilization processes on the abundance, field persistence, and transmission of antibiotic resistant bacteria and antibiotic resistance genes to vegetables at harvest. Sci Total Environ. (2019) 651:1680–7. doi: 10.1016/j.scitotenv.2018.10.030
98. Zheng J, Su C, Zhou J, Xu L, Qian Y, Chen H. Effects and mechanisms of ultraviolet, chlorination, and ozone disinfection on antibiotic resistance genes in secondary effluents of municipal wastewater treatment plants. Chem Eng J. (2017) 317:309–16. doi: 10.1016/j.cej.2017.02.076
99. Lin W, Zhang M, Zhang S, Yu X. Can chlorination co-select antibiotic-resistance genes? Chemosphere. (2016) 156:412–9. doi: 10.1016/j.chemosphere.2016.04.139
100. Guo MT, Yuan QB, Yang J. Microbial selectivity of UV treatment on antibiotic-resistant heterotrophic bacteria in secondary effluents of a municipal wastewater treatment plant. Water Res. (2013) 47:6388–94. doi: 10.1016/j.watres.2013.08.012
101. Zhang T, Hu Y, Jiang L, Yao S, Lin K, Zhou Y, et al. Removal of antibiotic resistance genes and control of horizontal transfer risk by UV, chlorination and UV/chlorination treatments of drinking water. Chem Eng J. (2019) 358:589–97. doi: 10.1016/j.cej.2018.09.218
102. Li H, Yang X-L, Song H-L, Zhang S, Long X-Z. Effects ofdirect current on Klebsiella spp. viability and corresponding resistance gene expression in simulative bio-electrochemical reactors. Chemosphere. (2018) 196:251–9. doi: 10.1016/j.chemosphere.2017.12.176
103. Hu Y, Zhang T, Jiang L, Yao S, Ye H, Lin K, et al. Removal of sulfonamide antibiotic resistant bacterial and intracellular antibiotic resistance genes by UVC-activated peroxymonosulfate. Chem Eng J. (2019) 368:888–95. doi: 10.1016/j.cej.2019.02.207
104. Oh J, Salcedo DE, Medriano CA, Kim S. Comparison of different disinfection processes in the effective removal of antibiotic-resistant bacteria and genes. J Environ Sci. (2014) 26:1238–42. doi: 10.1016/S1001-0742(13)60594-X
105. Michael SG, Michael-Kordatou I, Beretsou VG, Jäger T, Michael C, Schwartz T, et al. Solar photo-fenton oxidation followed by adsorption on activated carbon for the minimisation of antibiotic resistance determinants and toxicity present in urban wastewater. Appl Catal B-Environ. (2019) 244:871–80. doi: 10.1016/j.apcatb.2018.12.030
106. Fiorentino A, Esteban B, Garrido-Cardenas JA, Kowalska K, Rizzo L, Aguera A, et al. Effect of solar photo-fenton process in raceway pond reactors at neutral ph on antibiotic resistance determinants in secondary treated urban wastewater. J Hazard Mater. (2019) 378:120737. doi: 10.1016/j.jhazmat.2019.06.014
107. Giannakis S, Le TM, Entenza JM, Pulgarin C. Solar photo-fenton disinfection of 11 antibiotic-resistant bacteria (Arb) and elimination of representative Ar genes. Evidence that antibiotic resistance does not imply resistance to oxidative treatment. Water Res. (2018) 143:334–45. doi: 10.1016/j.watres.2018.06.062
108. Zhou Z, Shen Z, Cheng Z, Zhang G, Li M, Li Y, et al. Mechanistic insights for efficient inactivation of antibiotic resistance genes: a synergistic interfacial adsorption and photocatalytic-oxidation process. Sci Bull. (2020) 65:2107–19. doi: 10.1016/j.scib.2020.07.015
109. Moreira NFF, Narciso-da-Rocha C, Polo-Lopez MI, Pastrana-Martinez LM, Faria JL, Manaia CM, et al. Solar treatment (H2o2, Tio2-P25 and Go-Tio2 Photocatalysis, Photo-Fenton) of organic micropollutants, human pathogen indicators, antibiotic resistant bacteria and related genes in urban wastewater. Water Res. (2018) 135:195–206. doi: 10.1016/j.watres.2018.01.064
110. Huang X, Zheng J, Liu C, Liu L, Liu Y, Fan H. Removal of antibiotics and resistance genes from swine wastewater using vertical flow constructed wetlands: effect of hydraulic flow direction and substrate type. Chem Eng J. (2017) 308:692–9. doi: 10.1016/j.cej.2016.09.110
111. Le TH, Ng C, Tran NH, Chen H, Gin KY. Removal of antibiotic residues, antibiotic resistant bacteria and antibiotic resistance genes in municipal wastewater by membrane bioreactor systems. Water Res. (2018) 145:498–508. doi: 10.1016/j.watres.2018.08.060
112. Zanotto C, Bissa M, Illiano E, Mezzanotte V, Marazzi F, Turolla A, et al. Identification of antibiotic-resistant Escherichia coli isolated from a municipal wastewater treatment plant. Chemosphere. (2016) 164:627–33. doi: 10.1016/j.chemosphere.2016.08.040
113. Andersson DI. Persistence of antibiotic resistant bacteria. Curr Opin Microbiol. (2003) 6:452–6. doi: 10.1016/j.mib.2003.09.001
114. Capita R, Alonso-Calleja C. Antibiotic-resistant bacteria: a challenge for the food industry. Crit Rev Food Sci Nutr. (2013) 53:11–48. doi: 10.1080/10408398.2010.519837
115. Krzeminski P, Tomei MC, Karaolia P, Langenhoff A, Almeida CMR, Felis E, et al. Performance of secondary wastewater treatment methods for the removal of contaminants of emerging concern implicated in crop uptake and antibiotic resistance spread: a review. Sci Total Environ. (2019) 648:1052–81. doi: 10.1016/j.scitotenv.2018.08.130
116. Hiller CX, Hubner U, Fajnorova S, Schwartz T, Drewes JE. Antibiotic microbial resistance (AMR) removal efficiencies by conventional and advanced wastewater treatment processes: a review. Sci Total Environ. (2019) 685:596–608. doi: 10.1016/j.scitotenv.2019.05.315
117. Sharma VK, Johnson N, Cizmas L, McDonald TJ, Kim H. A review of the influence of treatment strategies on antibiotic resistant bacteria and antibiotic resistance genes. Chemosphere. (2016) 150:702–14. doi: 10.1016/j.chemosphere.2015.12.084
118. Bourke PF, Butler L. Mapping Australia's basic research in the medical and health sciences. Med J Aust. (1997) 167:610–3. doi: 10.5694/j.1326-5377.1997.tb138912.x
119. Chen Y, Jin Q, Fang H, Lei H, Hu J, Wu Y, et al. Analytic network process: academic insights and perspectives analysis. J Clean Prod. (2019) 235:1276–94. doi: 10.1016/j.jclepro.2019.07.016
120. Bao G, Pan L, Fang H, Wu X, Yu H, Cai S, et al. Academic review and perspectives on robotic exoskeletons. IEEE Trans Neural Syst Rehabil Eng. (2019) 27:2294–304. doi: 10.1109/TNSRE.2019.2944655
121. Fang H, He L, He H, Wang X, Wang Y, Ge H, et al. The 100 most-cited articles in castration-resistant prostate cancer: a bibliometric analysis. J Mens Health. (2022) 18:003. doi: 10.31083/jomh.2021.053
122. Chen H, Wan Y, Jiang S, Cheng Y. Alzheimer's disease research in the future: bibliometric analysis of cholinesterase inhibitors from 1993 to 2012. Scientometrics. (2014) 98:1865–77. doi: 10.1007/s11192-013-1132-3
123. Wu Y, Cheng Y, Yang X, Yu W, Wan Y. Dyslexia: a bibliometric and visualization analysis. Front Public Health. (2022) 10:915053. doi: 10.3389/fpubh.2022.915053
124. Wu Y, Wan Y, Zhang F. Characteristics and trends of C-H activation research: a review of literature. Curr Org Synth. (2018) 15:781–92. doi: 10.2174/1570179415666180426115417
125. Li L, Wan Y, Lu J, Fang H, Yin Z, Wang T, et al. Lattice boltzmann method for fluid-thermal systems: status, hotspots, trends and outlook. IEEE Access. (2020) 8:27649–75. doi: 10.1109/ACCESS.2020.2971546
126. He L, Fang H, Chen C, Wu Y, Wang Y, Ge H, et al. Metastatic castration-resistant prostate cancer: academic insights and perspectives through bibliometric analysis. Medicine. (2020) 99:e19760. doi: 10.1097/MD.0000000000019760
127. Chen H-Q, Wang X, He L, Chen P, Wan Y, Yang L, et al. Chinese energy and fuels research priorities and trend: a bibliometric analysis. Renew Sust Energ Rev. (2016) 58:966–75. doi: 10.1016/j.rser.2015.12.239
128. Loomes DE, van Zanten SV. Bibliometrics of the top 100 clinical articles in digestive disease. Gastroenterology. (2013) 144:673–6. doi: 10.1053/j.gastro.2013.02.013
129. Fusar-Poli P, Solmi M, Brondino N, Davies C, Chae C, Politi P, et al. Transdiagnostic psychiatry: a systematic review. World Psychiatry. (2019) 18:192–207. doi: 10.1002/wps.20631
130. Sun G, Dong D, Dong Z, Zhang Q, Fang H, Wang C, et al. Drug repositioning: a bibliometric analysis. Front Pharmacol. (2022) 13:974849. doi: 10.3389/fphar.2022.974849
131. Zhu S, Meng H, Gu Z, Zhao Y. Research trend of nanoscience and nanotechnology – a bibliometric analysis of nano today. Nano Today. (2021) 39:e101233. doi: 10.1016/j.nantod.2021.101233
132. Lindahl J, Stenling A, Lindwall M, Colliander C. Trends and knowledge base in sport and exercise psychology research: a bibliometric review study. Int Rev Sport Exerc Psychol. (2015) 8:71–94. doi: 10.1080/1750984X.2015.1019540
133. Muhuri PK, Shukla AK, Janmaijaya M, Basu A. Applied soft computing: a bibliometric analysis of the publications and citations during (2004–2016). Appl Soft Comput. (2018) 69:381–92. doi: 10.1016/j.asoc.2018.03.041
134. Garousi V, Mantyla MV. Citations, research topics and active countries in software engineering: a bibliometrics study. Comput Sci Rev. (2016) 19:56–77. doi: 10.1016/j.cosrev.2015.12.002
135. Yu D, Pan T. Tracing knowledge diffusion of TOPSIS: a historical perspective from citation network. Expert Syst Appl. (2021) 168:114238. doi: 10.1016/j.eswa.2020.114238
136. Yu D, Kou G, Xu Z, Shi S. Analysis of collaboration evolution in AHP research: 1982-2018. Int J Inf Technol Decis Mak. (2021) 20:7–36. doi: 10.1142/S0219622020500406
137. He X, Wu Y, Yu D. Merigo J. Exploring the ordered weighted averaging operator knowledge domain: a bibliometric analysis. Int J Intell Syst. (2017) 32:1151–66. doi: 10.1002/int.21894
138. Zheng C, Liao H, Tu C. An improved bibliometric analysis on antibiotics in soil research. Bull Environ Contam Toxicol. (2021) 108:276–83. doi: 10.1007/s00128-021-03395-1
139. Zheng C-L, Su J-Q, Zhu D, Xu Y-Y. Global trends and performances of studies on antibiotic resistance genes. Environ Eng Manag J. (2020) 19:485–95. doi: 10.30638/eemj.2020.046
140. Pruden A, Larsson DG, Amezquita A, Collignon P, Brandt KK, Graham DW, et al. Management options for reducing the release of antibiotics and antibiotic resistance genes to the environment. Environ Health Perspect. (2013) 121:878–85. doi: 10.1289/ehp.1206446
141. Yang L, Chen Z, Liu T, Gong Z, Yu Y, Wang J. Global trends of solid waste research from 1997 to 2011 by using bibliometric analysis. Scientometrics. (2013) 96:133–46. doi: 10.1007/s11192-012-0911-6
142. Wan g Z, Zhang H, Han J, Xing H, Wu M-c, Yang T. Deadly sins of antibiotic abuse in China. Infect Control Hosp Epidemiol. (2017) 38:758–9. doi: 10.1017/ice.2017.60
143. Wang X, Wu D, Xuan Z, Wang W, Zhou X. The influence of a ban on outpatient intravenous antibiotic therapy among the secondary and tertiary hospitals in China. BMC Public Health. (2020) 20:1794. doi: 10.1186/s12889-020-09948-z
144. Wen R, Li C, Zhao M, Wang H, Tang Y. Withdrawal of antibiotic growth promoters in china and its impact on the foodborne pathogen Campylobacter coli of swine origin. Front Microbiol. (2022) 13:3505. doi: 10.3389/fmicb.2022.1004725
145. Li L, Li X, Zhong W, Yang M, Xu M, Sun Y, et al. Gut microbiota from colorectal cancer patients enhances the progression of intestinal adenoma in apcmin/+ mice. EBioMedicine. (2019) 48:301–15. doi: 10.1016/j.ebiom.2019.09.021
147. Abramo G, D'Angelo CA, Solazzi M. The relationship between scientists' research performance and the degree of internationalization of their research. Scientometrics. (2010) 86:629–43. doi: 10.1007/s11192-010-0284-7
148. Vaz-Moreira I, Nunes OC, Manaia CM. Bacterial diversity and antibiotic resistance in water habitats: searching the links with the human microbiome. FEMS Microbiol Rev. (2014) 38:761–78. doi: 10.1111/1574-6976.12062
149. Novo A, Andre S, Viana P, Nunes OC, Manaia CM. Antibiotic resistance, antimicrobial residues and bacterial community composition in urban wastewater. Water Res. (2013) 47:1875–87. doi: 10.1016/j.watres.2013.01.010
150. Moreira NFF, Sousa JM, Macedo G, Ribeiro AR, Barreiros L, Pedrosa M, et al. Photocatalytic ozonation of urban wastewater and surface water using immobilized Tio2 with leds: micropollutants, antibiotic resistance genes and estrogenic activity. Water Res. (2016) 94:10–22. doi: 10.1016/j.watres.2016.02.003
151. Rizzo L, Sannino D, Vaiano V, Sacco O, Scarpa A, Pietrogiacomi D. Effect of solar simulated N-Doped Tio2 photocatalysis on the inactivation and antibiotic resistance of an E. coli strain in biologically treated urban wastewater. Appl Catal B-Environ. (2014) 144:369–78. doi: 10.1016/j.apcatb.2013.07.033
152. Rizzo L, Malato S, Antakyali D, Beretsou VG, Dolic MB, Gernjak W, et al. Consolidated vs. new advanced treatment methods for the removal of contaminants of emerging concern from urban wastewater. Sci Total Environ. (2019) 655:986–1008. doi: 10.1016/j.scitotenv.2018.11.265
153. Rizzo L, Della Sala A, Fiorentino A, Li Puma G. Disinfection of urban wastewater by solar driven and UV lamp - Tio(2) photocatalysis: effect on a multi drug resistant Escherichia coli strain. Water Res. (2014) 53:145–52. doi: 10.1016/j.watres.2014.01.020
154. Fatta-Kassinos D, Kalavrouziotis IK, Koukoulakis PH, Vasquez MI. The risks associated with wastewater reuse and xenobiotics in the agroecological environment. Sci Total Environ. (2011) 409:3555–63. doi: 10.1016/j.scitotenv.2010.03.036
155. Christou A, Aguera A, Bayona JM, Cytryn E, Fotopoulos V, Lambropoulou D, et al. The potential implications of reclaimed wastewater reuse for irrigation on the agricultural environment: the knowns and unknowns of the fate of antibiotics and antibiotic resistant bacteria and resistance genes - a review. Water Res. (2017) 123:448–67. doi: 10.1016/j.watres.2017.07.004
156. Karaolia P, Michael-Kordatou I, Hapeshi E, Drosou C, Bertakis Y, Christofilos D, et al. Removal of antibiotics, antibiotic-resistant bacteria and their associated genes by graphene-based Tio2 composite photocatalysts under solar radiation in urban wastewaters. Appl Catal B-Environ. (2018) 224:810–24. doi: 10.1016/j.apcatb.2017.11.020
157. Larsson DGJ, Andremont A, Bengtsson-Palme J, Brandt KK, de Roda Husman AM, Fagerstedt P, et al. Critical knowledge gaps and research needs related to the environmental dimensions of antibiotic resistance. Environ Int. (2018) 117:132–8. doi: 10.1016/j.envint.2018.04.041
158. Karkman A, Parnanen K, Larsson DGJ. Fecal pollution can explain antibiotic resistance gene abundances in anthropogenically impacted environments. Nat Commun. (2019) 10:80. doi: 10.1038/s41467-018-07992-3
159. Lundstrom SV, Ostman M, Bengtsson-Palme J, Rutgersson C, Thoudal M, Sircar T, et al. Minimal selective concentrations of tetracycline in complex aquatic bacterial biofilms. Sci Total Environ. (2016) 553:587–95. doi: 10.1016/j.scitotenv.2016.02.103
160. Manaia CM, Rocha J, Scaccia N, Marano R, Radu E, Biancullo F, et al. Antibiotic resistance in wastewater treatment plants: tackling the black box. Environ Int. (2018) 115:312–24. doi: 10.1016/j.envint.2018.03.044
161. Sousa JM, Macedo G, Pedrosa M, Becerra-Castro C, Castro-Silva S, Pereira MFR, et al. Ozonation and Uv254nm radiation for the removal of microorganisms and antibiotic resistance genes from urban wastewater. J Hazard Mater. (2017) 323:434–41. doi: 10.1016/j.jhazmat.2016.03.096
162. Reis PJM, Homem V, Alves A, Vilar VJP, Manaia CM, Nunes OC. Insights on sulfamethoxazole bio-transformation by environmental proteobacteria isolates. J Hazard Mater. (2018) 358:310–8. doi: 10.1016/j.jhazmat.2018.07.012
163. McKinney CW, Pruden A. Ultraviolet disinfection of antibiotic resistant bacteria and their antibiotic resistance genes in water and wastewater. Environ Sci Technol. (2012) 46:13393–400. doi: 10.1021/es303652q
164. Garner E, Inyang M, Garvey E, Parks J, Glover C, Grimaldi A, et al. Impact of blending for direct potable reuse on premise plumbing microbial ecology and regrowth of opportunistic pathogens and antibiotic resistant bacteria. Water Res. (2019) 151:75–86. doi: 10.1016/j.watres.2018.12.003
165. Marti R, Scott A, Tien YC, Murray R, Sabourin L, Zhang Y, et al. Impact of manure fertilization on the abundance of antibiotic-resistant bacteria and frequency of detection of antibiotic resistance genes in soil and on vegetables at harvest. Appl Environ Microbiol. (2013) 79:5701–9. doi: 10.1128/AEM.01682-13
166. Subirats J, Murray R, Yin X, Zhang T, Topp E. Impact of chicken litter pre-application treatment on the abundance, field persistence, and transfer of antibiotic resistant bacteria and antibiotic resistance genes to vegetables. Sci Total Environ. (2021) 801:149718. doi: 10.1016/j.scitotenv.2021.149718
167. Topp E, Larsson DGJ, Miller DN, Van den Eede C, Virta MPJ. Antimicrobial resistance and the environment: assessment of advances, gaps and recommendations for agriculture, aquaculture and pharmaceutical manufacturing. FEMS Microbiol Ecol. (2018) 94:fix185. doi: 10.1093/femsec/fix185
168. Liu L, Bhatia R, Webster TJ. Atomic layer deposition of nano-Tio2 thin films with enhanced biocompatibility and antimicrobial activity for orthopedic implants. Int J Nanomedicine. (2017) 12:8711–23. doi: 10.2147/IJN.S148065
169. Shi D, Mi G, Wang M, Webster TJ. In vitro and ex vivo systems at the forefront of infection modeling and drug discovery. Biomaterials. (2019) 198:228–49. doi: 10.1016/j.biomaterials.2018.10.030
170. Durmus NG, Taylor EN, Inci F, Kummer KM, Tarquinio KM, Webster TJ. Fructose-enhanced reduction of bacterial growth on nanorough surfaces. Int J Nanomedicine. (2012) 7:537–45. doi: 10.2147/IJN.S27957
171. Alexander J, Knopp G, Dotsch A, Wieland A, Schwartz T. Ozone treatment of conditioned wastewater selects antibiotic resistance genes, opportunistic bacteria, and induce strong population shifts. Sci Total Environ. (2016) 559:103–12. doi: 10.1016/j.scitotenv.2016.03.154
172. Jager T, Hembach N, Elpers C, Wieland A, Alexander J, Hiller C, et al. Reduction of antibiotic resistant bacteria during conventional and advanced wastewater treatment, and the disseminated loads released to the environment. Front Microbiol. (2018) 9:2599. doi: 10.3389/fmicb.2018.02599
173. Boopathy R. Presence of methicillin resistant Staphylococcus aureus (Mrsa) in sewage treatment plant. Bioresour Technol. (2017) 240:144–8. doi: 10.1016/j.biortech.2017.02.093
174. Garcia J, Garcia-Galan MJ, Day JW, Boopathy R, White JR, Wallace S, et al. A review of emerging organic contaminants (Eocs), antibiotic resistant bacteria (Arb), and antibiotic resistance genes (Args) in the environment: increasing removal with wetlands and reducing environmental impacts. Bioresour Technol. (2020) 307:123228. doi: 10.1016/j.biortech.2020.123228
175. Grabert R, Boopathy R, Nathaniel R, LaFleur G. Effect of tetracycline on ammonia and carbon removal by the facultative bacteria in the anaerobic digester of a sewage treatment plant. Bioresour Technol. (2018) 267:265–70. doi: 10.1016/j.biortech.2018.07.061
176. Harnisz M, Korzeniewska E, Golas I. The impact of a freshwater fish farm on the community of tetracycline-resistant bacteria and the structure of tetracycline resistance genes in river water. Chemosphere. (2015) 128:134–41. doi: 10.1016/j.chemosphere.2015.01.035
177. Harnisz M, Korzeniewska E. The prevalence of multidrug-resistant Aeromonas spp. in the municipal wastewater system and their dissemination in the environment. Sci Total Environ. (2018) 626:377–83. doi: 10.1016/j.scitotenv.2018.01.100
178. Zielinski W, Korzeniewska E, Harnisz M, Hubeny J, Buta M, Rolbiecki D. The prevalence of drug-resistant and virulent Staphylococcus spp. in a municipal wastewater treatment plant and their spread in the environment. Environ Int. (2020) 143:105914. doi: 10.1016/j.envint.2020.105914
179. Al-Jassim N, Ansari MI, Harb M, Hong P-Y. Removal of bacterial contaminants and antibiotic resistance genes by conventional wastewater treatment processes in Saudi Arabia: is the treated wastewater safe to reuse for agricultural irrigation? Water Res. (2015) 73:277–90. doi: 10.1016/j.watres.2015.01.036
180. Cheng H, Hong PY. Removal of antibiotic-resistant bacteria and antibiotic resistance genes affected by varying degrees of fouling on anaerobic microfiltration membranes. Environ Sci Technol. (2017) 51:12200–9. doi: 10.1021/acs.est.7b03798
181. Al-Jassim N, Mantilla-Calderon D, Wang T, Hong PY. Inactivation and gene expression of a virulent wastewater Escherichia coli strain and the nonvirulent commensal Escherichia coli Dsm1103 strain upon solar irradiation. Environ Sci Technol. (2017) 51:3649–59. doi: 10.1021/acs.est.6b05377
182. Osinska A, Korzeniewska E, Harnisz M, Felis E, Bajkacz S, Jachimowicz P, et al. Small-scale wastewater treatment plants as a source of the dissemination of antibiotic resistance genes in the aquatic environment. J Hazard Mater. (2020) 381:121221. doi: 10.1016/j.jhazmat.2019.121221
183. Korzeniewska E, Korzeniewska A, Harnisz M. Antibiotic resistant Escherichia coli in hospital and municipal sewage and their emission to the environment. Ecotoxicol Environ Saf. (2013) 91:96–102. doi: 10.1016/j.ecoenv.2013.01.014
184. Kinnebrew MA, Buffie CG, Diehl GE, Zenewicz LA, Leiner I, Hohl TM, et al. Interleukin 23 production by intestinal Cd103(+)Cd11b(+) dendritic cells in response to bacterial flagellin enhances mucosal innate immune defense. Immunity. (2012) 36:276–87. doi: 10.1016/j.immuni.2011.12.011
185. Ubeda C, Taur Y, Jenq RR, Equinda MJ, Son T, Samstein M, et al. Vancomycin-resistant enterococcus domination of intestinal microbiota is enabled by antibiotic treatment in mice and precedes bloodstream invasion in humans. J Clin Invest. (2010) 120:4332–41. doi: 10.1172/JCI43918
186. Keith JW, Pamer EG. Enlisting commensal microbes to resist antibiotic-resistant pathogens. J Exp Med. (2019) 216:10–9. doi: 10.1084/jem.20180399
187. Hoa PT, Managaki S, Nakada N, Takada H, Shimizu A, Anh DH, et al. Antibiotic contamination and occurrence of antibiotic-resistant bacteria in aquatic environments of Northern Vietnam. Sci Total Environ. (2011) 409:2894–901. doi: 10.1016/j.scitotenv.2011.04.030
188. Suzuki S, Ogo M, Miller TW, Shimizu A, Takada H, Siringan MAT. Who possesses drug resistance genes in the aquatic environment? Sulfamethoxazole (Smx) resistance genes among the bacterial community in water environment of Metro-Manila, Philippines. Front Microbiol. (2013) 4:102. doi: 10.3389/fmicb.2013.00102
189. Suzuki S, Nakanishi S, Tamminen M, Yokokawa T, Sato-Takabe Y, Ohta K, et al. Occurrence of Sul and Tet(M) genes in bacterial community in Japanese marine aquaculture environment throughout the year: profile comparison with Taiwanese and Finnish aquaculture waters. Sci Total Environ. (2019) 669:649–56. doi: 10.1016/j.scitotenv.2019.03.111
190. Uddin MJ, Dawan J, Jeon G, Yu T, He X, Ahn J. The role of bacterial membrane vesicles in the dissemination of antibiotic resistance and as promising carriers for therapeutic agent delivery. Microorganisms. (2020) 8:670. doi: 10.3390/microorganisms8050670
191. Jo A, Ding T, Ahn J. Synergistic antimicrobial activity of bacteriophages and antibiotics against Staphylococcus aureus. Food Sci Biotechnol. (2016) 25:935–40. doi: 10.1007/s10068-016-0153-0
192. Jung LS, Ding T, Ahn J. Evaluation of lytic bacteriophages for control of multidrug-resistant salmonella typhimurium. Ann Clin Microbiol Antimicrob. (2017) 16:66. doi: 10.1186/s12941-017-0237-6
193. Liu J, Zhao Z, Orfe L, Subbiah M, Call DR. Soil-borne reservoirs of antibiotic-resistant bacteria are established following therapeutic treatment of dairy calves. Environ Microbiol. (2016) 18:557–64. doi: 10.1111/1462-2920.13097
194. Subbiah M, Mitchell SM, Ullman JL, Call DR. Beta-lactams and florfenicol antibiotics remain bioactive in soils while ciprofloxacin, neomycin, and tetracycline are neutralized. Appl Environ Microbiol. (2011) 77:7255–60. doi: 10.1128/AEM.05352-11
195. Call DR, Matthews L, Subbiah M, Liu J. Do antibiotic residues in soils play a role in amplification and transmission of antibiotic resistant bacteria in cattle populations? Front Microbiol. (2013) 4:193. doi: 10.3389/fmicb.2013.00193
196. Guo MT, Yuan QB, Yang J. Ultraviolet reduction of erythromycin and tetracycline resistant heterotrophic bacteria and their resistance genes in municipal wastewater. Chemosphere. (2013) 93:2864–8. doi: 10.1016/j.chemosphere.2013.08.068
197. Guo MT, Tian XB. Impacts on antibiotic-resistant bacteria and their horizontal gene transfer by graphene-based Tio2&Ag composite photocatalysts under solar irradiation. J Hazard Mater. (2019) 380:120877. doi: 10.1016/j.jhazmat.2019.120877
198. Yuan QB, Guo MT, Wei WJ, Yang J. Reductions of bacterial antibiotic resistance through five biological treatment processes treated municipal wastewater. Environ Sci Pollut Res Int. (2016) 23:19495–503. doi: 10.1007/s11356-016-7048-8
199. Manohar P, Tamhankar AJ, Lundborg CS, Nachimuthu R. Therapeutic characterization and efficacy of bacteriophage cocktails infecting Escherichia coli, Klebsiella pneumoniae, and Enterobacter Species. Front Microbiol. (2019) 10:574. doi: 10.3389/fmicb.2019.00574
200. Lien LT, Hoa NQ, Chuc NT, Thoa NT, Phuc HD, Diwan V, et al. Antibiotics in wastewater of a rural and an urban hospital before and after wastewater treatment, and the relationship with antibiotic use-a one year study from Vietnam. Int J Environ Res Public Health. (2016) 13:588. doi: 10.3390/ijerph13060588
201. Sharma M, Eriksson B, Marrone G, Dhaneria S, Lundborg CS. Antibiotic prescribing in two private sector hospitals; one teaching and one non-teaching: a cross-sectional study in Ujjain, India. BMC Infect Dis. (2012) 12:1–9. doi: 10.1186/1471-2334-12-155
202. Li B, Zhang X, Guo F, Wu W, Zhang T. Characterization of tetracycline resistant bacterial community in saline activated sludge using batch stress incubation with high-throughput sequencing analysis. Water Res. (2013) 47:4207–16. doi: 10.1016/j.watres.2013.04.021
203. Li AD, Ma L, Jiang XT, Zhang T. Cultivation-dependent and high-throughput sequencing approaches studying the co-occurrence of antibiotic resistance genes in municipal sewage system. Appl Microbiol Biotechnol. (2017) 101:8197–207. doi: 10.1007/s00253-017-8573-1
204. Che Y, Xia Y, Liu L, Li AD, Yang Y, Zhang T. Mobile antibiotic resistome in wastewater treatment plants revealed by nanopore metagenomic sequencing. Microbiome. (2019) 7:44. doi: 10.1186/s40168-019-0663-0
205. Fang H, Jing Y, Chen J, Wu Y, Wan Y. Recent trends in sedentary time: a systematic literature review. Heathcare. (2021) 9:969. doi: 10.3390/healthcare9080969
206. Wu Y, Chen J, Fang H, Wan Y. Intimate partner violence: a bibliometric review of literature. Int J Environ Res Public Health. (2020) 17:5607. doi: 10.3390/ijerph17155607
207. Bao G, Fang H, Chen L, Wan Y, Xu F, Yang Q, et al. Soft robotics: academic insights and perspectives through bibliometric analysis. Soft Robot. (2018) 5:229–41. doi: 10.1089/soro.2017.0135
208. Chen G, Ju B, Fang H, Chen Y, Yu N, Wan Y. Air bearing: academic insights and trend analysis. Int J Adv Manuf Technol. (2019) 106:1191–202. doi: 10.1007/s00170-019-04663-5
209. Ding Y, Chen D, Ding X, Wang G, Wan Y, Shen Q, et al. Bibliometric analysis of income and cardiovascular disease: status, hotspots, trends and outlook. Medicine. (2020) 99:e21828. doi: 10.1097/MD.0000000000021828
210. Bornmann L. How are excellent (highly cited) papers defined in bibliometrics? A quantitative analysis of the literature. Res Evaluat. (2014) 23:166–73. doi: 10.1093/reseval/rvu002
211. Adams J, Griliches Z. Measuring science: an exploration. Proc Natl Acad Sci U S A. (1996) 93:12664–70. doi: 10.1073/pnas.93.23.12664
212. Berendonk TU, Manaia CM, Merlin C, Fatta-Kassinos D, Cytryn E, Walsh F, et al. Tackling antibiotic resistance: the environmental framework. Nat Rev Microbiol. (2015) 13:310–7. doi: 10.1038/nrmicro3439
213. Munir M, Wong K, Xagoraraki I. Release of antibiotic resistant bacteria and genes in the effluent and biosolids of five wastewater utilities in Michigan. Water Res. (2011) 45:681–93. doi: 10.1016/j.watres.2010.08.033
214. Su JQ, Wei B, Ou-Yang WY, Huang FY, Zhao Y, Xu HJ, et al. Antibiotic resistome and its association with bacterial communities during sewage sludge composting. Environ Sci Technol. (2015) 49:7356–63. doi: 10.1021/acs.est.5b01012
215. Gao P, Munir M, Xagoraraki I. Correlation of tetracycline and sulfonamide antibiotics with corresponding resistance genes and resistant bacteria in a conventional municipal wastewater treatment plant. Sci Total Environ. (2012) 421:173–83. doi: 10.1016/j.scitotenv.2012.01.061
216. Liu X, Steele JC, Meng XZ. Usage, residue, and human health risk of antibiotics in chinese aquaculture: a review. Environ Pollut. (2017) 223:161–9. doi: 10.1016/j.envpol.2017.01.003
217. Bouki C, Venieri D, Diamadopoulos E. Detection and fate of antibiotic resistant bacteria in wastewater treatment plants: a review. Ecotoxicol Environ Saf. (2013) 91:1–9. doi: 10.1016/j.ecoenv.2013.01.016
218. Rossi E, La Rosa R, Bartell JA, Marvig RL, Haagensen JAJ, Sommer LM, et al. Pseudomonas aeruginosa adaptation and evolution in patients with cystic fibrosis. Nat Rev Microbiol. (2021) 19:331–42. doi: 10.1038/s41579-020-00477-5
219. Turner NA, Sharma-Kuinkel BK, Maskarinec SA, Eichenberger EM, Shah PP, Carugati M, et al. Methicillin-resistant Staphylococcus aureus: an overview of basic and clinical research. Nat Rev Microbiol. (2019) 17:203–18. doi: 10.1038/s41579-018-0147-4
220. Wang J, Chu L, Wojnarovits L, Takacs E. Occurrence and fate of antibiotics, antibiotic resistant genes (ARGS) and antibiotic resistant bacteria (ARB) in municipal wastewater treatment plant: an overview. Sci Total Environ. (2020) 744:140997. doi: 10.1016/j.scitotenv.2020.140997
221. Homaeigohar S, Boccaccini AR. Antibacterial biohybrid nanofibers for wound dressings. Acta Biomater. (2020) 107:25–49. doi: 10.1016/j.actbio.2020.02.022
222. Munir MU, Ahmad MM. Nanomaterials aiming to tackle antibiotic-resistant bacteria. Pharmaceutics. (2022) 14:582. doi: 10.3390/pharmaceutics14030582
223. Tse Sum Bui B, Auroy T, Haupt K. Fighting antibiotic-resistant bacteria: promising strategies orchestrated by molecularly imprinted polymers. Angew Chem. (2022) 134:e202106493. doi: 10.1002/ange.202106493
224. Dijksteel GS, Ulrich MM, Middelkoop E, Boekema BK. Lessons learned from clinical trials using antimicrobial peptides (Amps). Front Microbiol. (2021) 12:616979. doi: 10.3389/fmicb.2021.616979
225. Abd El-Hack ME, El-Saadony MT, Shafi ME, Alshahrani OA, Saghir SA, Al-Wajeeh AS, et al. Prebiotics can restrict salmonella populations in poultry: a review. Anim Biotechnol. (2021) 1–10. doi: 10.1080/10495398.2021.1883637
226. Álvarez-Martínez F, Barrajón-Catalán E, Herranz-López M, Micol V. Antibacterial plant compounds, extracts and essential oils: an updated review on their effects and putative mechanisms of action. Phytomedicine. (2021) 90:153626. doi: 10.1016/j.phymed.2021.153626
227. Zhuang M, Achmon Y, Cao Y, Liang X, Chen L, Wang H, et al. Distribution of antibiotic resistance genes in the environment. Environ Pollut. (2021) 285:117402. doi: 10.1016/j.envpol.2021.117402
228. Anh HQ, Le TPQ, Da Le N, Lu XX, Duong TT, Garnier J, et al. Antibiotics in surface water of East and Southeast Asian countries: a focused review on contamination status, pollution sources, potential risks, and future perspectives. Sci Total Environ. (2021) 764:142865. doi: 10.1016/j.scitotenv.2020.142865
229. Majumder A, Gupta AK, Ghosal PS, Varma M. A review on hospital wastewater treatment: a special emphasis on occurrence and removal of pharmaceutically active compounds, resistant microorganisms, and SARS-CoV-2. J Environ Chem Eng. (2021) 9:104812. doi: 10.1016/j.jece.2020.104812
230. Baaloudj O, Assadi I, Nasrallah N, El Jery A, Khezami L, Assadi AA. Simultaneous removal of antibiotics and inactivation of antibiotic-resistant bacteria by photocatalysis: a review. J Water Process Eng. (2021) 42:102089. doi: 10.1016/j.jwpe.2021.102089
231. Foroughi M, Khiadani M, Kakhki S, Kholghi V, Naderi K, Yektay S. Effect of ozonation-based disinfection methods on the removal of antibiotic resistant bacteria and resistance genes (Arb/Args) in water and wastewater treatment: a systematic review. Sci Total Environ. (2021) 811:151404. doi: 10.1016/j.scitotenv.2021.151404
232. Hena S, Gutierrez L, Croué J-P. Removal of pharmaceutical and personal care products (PPCPS) from wastewater using microalgae: a review. J Hazard Mater. (2021) 403:124041. doi: 10.1016/j.jhazmat.2020.124041
233. Wu F, Zhao S, Yu B, Chen YM, Wang W, Song ZG, et al. A new coronavirus associated with human respiratory disease in China. Nature. (2020) 579:265–9. doi: 10.1038/s41586-020-2008-3
234. Zhou P, Yang XL, Wang XG, Hu B, Zhang L, Zhang W, et al. A pneumonia outbreak associated with a new coronavirus of probable bat origin. Nature. (2020) 579:270–3.
235. Guan W-j, Ni Z-y, Hu Y, Liang W-h, Ou C-q, He J-x, et al. Clinical characteristics of coronavirus disease 2019 in China. N Engl J Med. (2020) 382:1708–20. doi: 10.1056/NEJMoa2002032
236. Zhou F, Yu T, Du R, Fan G, Liu Y, Liu Z, et al. Clinical course and risk factors for mortality of adult inpatients with COVID-19 in Wuhan, China: a retrospective cohort study. Lancet. (2020) 395:1054–62. doi: 10.1016/S0140-6736(20)30566-3
237. Bhatraju PK, Ghassemieh BJ, Nichols M, Kim R, Jerome KR, Nalla AK, et al. COVID-19 in critically ill patients in the seattle region - case series. N Engl J Med. (2020) 382:2012–22. doi: 10.1056/NEJMoa2004500
238. Arons MM, Hatfield KM, Reddy SC, Kimball A, James A, Jacobs JR, et al. Presymptomatic SARS-CoV-2 infections and transmission in a skilled nursing facility. N Engl J Med. (2020) 382:2081–90. doi: 10.1056/NEJMoa2008457
239. Remuzzi A, Remuzzi G. COVID-19 and Italy: what next? Lancet. (2020) 395:1225–8. doi: 10.1016/S0140-6736(20)30627-9
240. Tiri B, Sensi E, Marsiliani V, Cantarini M, Priante G, Vernelli C, et al. Antimicrobial stewardship program, COVID-19, and infection control: spread of carbapenem-resistant Klebsiella pneumoniae colonization in ICU COVID-19 patients. What did not work? J Clin Med. (2020) 9:2744. doi: 10.3390/jcm9092744
241. Domingues CPF, Rebelo JS, Dionisio F, Botelho A, Nogueira T. The social distancing imposed to contain COVID-19 can affect our microbiome: a double-edged sword in human health. mSphere. (2020) 5:e00716–20. doi: 10.1128/mSphere.00716-20
242. Kurpe SR, Grishin SY, Surin AK, Panfilov AV, Slizen MV, Chowdhury SD, et al. Antimicrobial and amyloidogenic activity of peptides. Can antimicrobial peptides be used against SARS-CoV-2? Int J Mol Sci. (2020) 21:9552. doi: 10.3390/ijms21249552
243. Mancuso E, Tonda-Turo C, Ceresa C, Pensabene V, Connell SD, Fracchia L, et al. Potential of manuka honey as a natural polyelectrolyte to develop biomimetic nanostructured meshes with antimicrobial properties. Front Bioeng Biotechnol. (2019) 7:344. doi: 10.3389/fbioe.2019.00344
244. Wood RL, Jensen T, Wadsworth C, Clement M, Nagpal P, Pitt WG. Analysis of identification method for bacterial species and antibiotic resistance genes using optical data from DNA oligomers. Front Microbiol. (2020) 11:257. doi: 10.3389/fmicb.2020.00257
245. Alafeef M, Moitra P, Pan D. Nano-enabled sensing approaches for pathogenic bacterial detection. Biosens Bioelectron. (2020) 165:112276. doi: 10.1016/j.bios.2020.112276
246. Ebrahimi M, Mohammadi-Dehcheshmeh M, Ebrahimie E, Petrovski KR. Comprehensive analysis of machine learning models for prediction of sub-clinical mastitis: deep learning and gradient-boosted trees outperform other models. Comput Biol Med. (2019) 114:103456. doi: 10.1016/j.compbiomed.2019.103456
247. Amaya E, Reyes D, Paniagua M, Calderón S, Rashid MU, Colque P, et al. Antibiotic resistance patterns of Escherichia coli isolates from different aquatic environmental sources in Leon, Nicaragua. Clin Microbiol Infect. (2012) 18:E347–E54. doi: 10.1111/j.1469-0691.2012.03930.x
248. Rice LB. Federal funding for the study of antimicrobial resistance in nosocomial pathogens: no eskape. J Infect Dis. (2008) 197:1079–81. doi: 10.1086/533452
249. Boucher HW, Talbot GH, Bradley JS, Edwards JE, Gilbert D, Rice LB, et al. Bad Bugs, No Drugs: No Eskape! An update from the infectious diseases Society of America. Clin Infect Dis. (2009) 48:1–12. doi: 10.1086/595011
250. Kmietowicz Z. One in five GP prescriptions for antibiotics is inappropriate. BMJ. (2018) 360:k936. doi: 10.1136/bmj.k936
251. Xue H, Shi Y, Huang L, Yi H, Zhou H, Zhou C, et al. Drivers of inappropriate antibiotic prescriptions: a quasi-experimental study of antibiotic prescription by primary care providers in rural China. Lancet. (2018) 392:S40. doi: 10.1016/S0140-6736(18)32669-2
252. Chen C, Li J, Chen P, Ding R, Zhang P, Li X. Occurrence of antibiotics and antibiotic resistances in soils from wastewater irrigation areas in Beijing and Tianjin, China. Environ Pollut. (2014) 193:94–101. doi: 10.1016/j.envpol.2014.06.005
253. Currie J, Lin W, Meng J. Addressing antibiotic abuse in China: an experimental audit study. J Dev Econ. (2014) 110:39–51. doi: 10.1016/j.jdeveco.2014.05.006
254. Currie J, Lin W, Zhang W. Patient knowledge and antibiotic abuse: evidence from an audit study in China. J Health Econ. (2011) 30:933–49. doi: 10.1016/j.jhealeco.2011.05.009
255. English BK, Gaur AH. The use and abuse of antibiotics and the development of antibiotic resistance. Adv Exp Med Biol. (2010) 659:73–82. doi: 10.1007/978-1-4419-0981-7_6
256. Goldman E. Antibiotic abuse in animal agriculture: exacerbating drug resistance in human pathogens. Hum Ecol Risk Assess. (2004) 10:121–34. doi: 10.1080/10807030490281016
257. Ramakrishnan N, Sriram K. Antibiotic overuse and clostridium difficile infections: the Indian paradox and the possible role of dietary practices. Nutrition. (2015) 31:1052–3. doi: 10.1016/j.nut.2015.02.002
258. De Luca M, Dona D, Montagnani C, Lo Vecchio A, Romanengo M, Tagliabue C, et al. Antibiotic prescriptions and prophylaxis in Italian children. Is it time to change? Data from the Arpec project. PLoS ONE. (2016) 11:e0154662. doi: 10.1371/journal.pone.0154662
259. Larsen J, Raisen CL, Ba X, Sadgrove NJ, Padilla-Gonzalez GF, Simmonds MSJ, et al. Emergence of methicillin resistance predates the clinical use of antibiotics. Nature. (2022) 602:135–41.
260. Zahn LM. The many roads to resistance. Science. (2021) 371:793–5. doi: 10.1126/science.371.6531.793-n
261. Hanna CC, Hermant YO, Harris PWR, Brimble MA. Discovery, synthesis, and optimization of peptide-based antibiotics. Acc Chem Res. (2021) 54:1878–90. doi: 10.1021/acs.accounts.0c00841
262. Torres MDT, Melo MCR, Crescenzi O, Notomista E, de la Fuente-Nunez C. Mining for encrypted peptide antibiotics in the human proteome. Nat Biomed Eng. (2022) 6:67–75. doi: 10.1038/s41551-021-00801-1
263. de la Fuente-Nunez C. Antibiotic discovery with machine learning. Nat Biotechnol. (2022) 40:833–4. doi: 10.1038/s41587-022-01327-w
264. Shkoporov AN, Turkington CJ, Hill C. Mutualistic interplay between bacteriophages and bacteria in the human gut. Nat Rev Microbiol. (2022) 1–13. doi: 10.1038/s41579-022-00755-4
265. Toporek A, Lechtzin N. Viruses to the rescue—use of bacteriophage to treat resistant pulmonary infections. Cell. (2022) 185:1807–8. doi: 10.1016/j.cell.2022.04.037
266. Thänert R, Sawhney SS, Schwartz DJ, Dantas G. The resistance within: antibiotic disruption of the gut microbiome and resistome dynamics in infancy. Cell Host Microbe. (2022) 30:675–83. doi: 10.1016/j.chom.2022.03.013
267. Zimmermann M, Patil KR, Typas A, Maier L. Towards a mechanistic understanding of reciprocal drug–microbiome interactions. Mol Syst Biol. (2021) 17:e10116. doi: 10.15252/msb.202010116
268. Micoli F, Bagnoli F, Rappuoli R, Serruto D. The role of vaccines in combatting antimicrobial resistance. Nat Rev Microbiol. (2021) 19:287–302. doi: 10.1038/s41579-020-00506-3
269. Shatalin K, Nuthanakanti A, Kaushik A, Shishov D, Peselis A, Shamovsky I, et al. Inhibitors of bacterial H2s biogenesis targeting antibiotic resistance and tolerance. Science. (2021) 372:1169–75. doi: 10.1126/science.abd8377
270. Xin Q, Shah H, Nawaz A, Xie W, Akram MZ, Batool A, et al. Antibacterial carbon-based nanomaterials. Adv Mater. (2019) 31:1804838. doi: 10.1002/adma.201804838
271. Lai H-Z, Chen W-Y, Wu C-Y, Chen Y-C. Potent antibacterial nanoparticles for pathogenic bacteria. ACS Appl Mater Interfaces. (2015) 7:2046–54. doi: 10.1021/am507919m
272. Murray CJL, Ikuta KS, Sharara F, Swetschinski L, Robles Aguilar G, Gray A, et al. Global burden of bacterial antimicrobial resistance in 2019: a systematic analysis. Lancet. (2022) 399:629–55. doi: 10.1016/S0140-6736(21)02724-0
273. Beukes LS, King TLB, Schmidt S. Assessment of pit latrines in a peri-urban community in Kwazulu-Natal (South Africa) as a source of antibiotic resistant E. coli. strains. Int J Hyg Environ Health. (2017) 220:1279–84. doi: 10.1016/j.ijheh.2017.08.002
274. Katakweba AA, Moller KS, Muumba J, Muhairwa AP, Damborg P, Rosenkrantz JT, et al. Antimicrobial resistance in faecal samples from buffalo, wildebeest and zebra grazing together with and without cattle in Tanzania. J Appl Microbiol. (2015) 118:966–75. doi: 10.1111/jam.12738
275. Hlashwayo DF, Sigauque B, Noormahomed EV, Afonso SMS, Mandomando IM, Bila CG, et al. Systematic review and meta-analysis reveal that Campylobacter spp. and antibiotic resistance are widespread in humans in Sub-Saharan Africa. PLoS ONE. (2021) 16:e0245951. doi: 10.1371/journal.pone.0245951
276. Algammal AM, Enany ME, El-Tarabili RM, Ghobashy MOI, Helmy YA. Prevalence, antimicrobial resistance profiles, virulence and enterotoxins-determinant genes of Mrsa isolated from subclinical bovine mastitis in Egypt. Pathogens. (2020) 9:362. doi: 10.3390/pathogens9050362
277. Fashae K, Engelmann I, Monecke S, Braun SD, Ehricht R. Molecular characterisation of extended-spectrum SS-lactamase producing Escherichia coli in wild birds and cattle, Ibadan, Nigeria. BMC Vet Res. (2021) 17:33. doi: 10.1186/s12917-020-02734-4
278. Audu BJ, Norval S, Bruno L, Meenakshi R, Marion M, Forbes KJ. Genomic diversity and antimicrobial resistance of Campylobacter spp. from humans and livestock in Nigeria. J Biomed Sci. (2022) 29:7. doi: 10.1186/s12929-022-00786-2
279. Najem S, Eick D, Boettcher J, Aigner A, Aboutara M, Fenner I, et al. High prevalence of multidrug-resistant gram-negative bacteria carriage in children screened prospectively for multidrug resistant organisms at admission to a paediatric hospital, Hamburg, Germany, September 2018 to May 2019. Euro Surveill. (2022) 27:2001567. doi: 10.2807/1560-7917.ES.2022.27.15.2001567
280. Baggs J, Rose AN, McCarthy NL, Wolford H, Srinivasan A, Jernigan JA, et al. Antibiotic resistant infections among COVID-19 inpatients in U.S. hospitals. Clin Infect Dis. (2022) 75:S294–7. doi: 10.1093/cid/ciac517
281. Taylor L. COVID-19: antimicrobial misuse in americas sees drug resistant infections surge, says who. BMJ. (2021) 375:n2845. doi: 10.1136/bmj.n2845
282. Iacobucci G. GP efforts to cut antibiotic use failed to curb spread of drug resistant E. coli, evaluation finds. Br Med J. (2021) 374:n1953. doi: 10.1136/bmj.n1953
283. Pacios E. Antibiotic stewardship in the real world. Lancet Infect Dis. (2022) 22:448–9. doi: 10.1016/S1473-3099(22)00147-5
284. Stracy M, Snitser O, Yelin I, Amer Y, Parizade M, Katz R, et al. Minimizing treatment-induced emergence of antibiotic resistance in bacterial infections. Science. (2022) 375:889–94. doi: 10.1126/science.abg9868
285. Scaccia N, Vaz-Moreira I, Manaia CM. The risk of transmitting antibiotic resistance through endophytic bacteria. Trends Plant Sci. (2021) 26:1213–26. doi: 10.1016/j.tplants.2021.09.001
286. Zumstein MT, Werner JJ, Helbling DE. Exploring the specificity of extracellular wastewater peptidases to improve the design of sustainable peptide-based antibiotics. Environ Sci Technol. (2020) 54:11201–9. doi: 10.1021/acs.est.0c02564
287. Charon J, Manteca A, Innis CA. Using the bacterial ribosome as a discovery platform for peptide-based antibiotics. Biochemistry. (2018) 58:75–84. doi: 10.1021/acs.biochem.8b00927
288. Yadav V, Misra R. A review emphasizing on utility of heptad repeat sequence as a tool to design pharmacologically safe peptide-based antibiotics. Biochimie. (2021) 191:126–39. doi: 10.1016/j.biochi.2021.09.001
289. Kumar P, Kizhakkedathu JN, Straus SK. Antimicrobial peptides: diversity, mechanism of action and strategies to improve the activity and biocompatibility in vivo. Biomolecules. (2018) 8:4. doi: 10.3390/biom8010004
290. Wang G, Hanke ML, Mishra B, Lushnikova T, Heim CE, Chittezham Thomas V, et al. Transformation of human cathelicidin Ll-37 into selective, stable, and potent antimicrobial compounds. ACS Chem Biol. (2014) 9:1997–2002. doi: 10.1021/cb500475y
291. Pasupuleti M, Schmidtchen A, Malmsten M. Antimicrobial peptides: key components of the innate immune system. Crit Rev Biotechnol. (2012) 32:143–71. doi: 10.3109/07388551.2011.594423
292. Nandi A, Megiddo I, Ashok A, Verma A, Laxminarayan R. Reduced burden of childhood diarrheal diseases through increased access to water and sanitation in india: a modeling analysis. Soc Sci Med. (2017) 180:181–92. doi: 10.1016/j.socscimed.2016.08.049
293. Chow LKM, Ghaly TM, Gillings MR. A survey of sub-inhibitory concentrations of antibiotics in the environment. J Environ Sci. (2021) 99:21–7. doi: 10.1016/j.jes.2020.05.030
294. Lee GC, Reveles KR, Attridge RT, Lawson KA, Mansi IA, Lewis JS, et al. Outpatient antibiotic prescribing in the United States: 2000 to 2010. BMC Med. (2014) 12:1–8. doi: 10.1186/1741-7015-12-96
295. Alcaine SD, Molla L, Nugen SR, Kruse H. Results of a pilot antibiotic resistance survey of albanian poultry farms. J Glob Antimicrob Resist. (2016) 4:60–4. doi: 10.1016/j.jgar.2015.11.003
296. Pruden A. Balancing water sustainability and public health goals in the face of growing concerns about antibiotic resistance. Environ Sci Technol. (2014) 48:5–14. doi: 10.1021/es403883p
297. Baur D, Gladstone BP, Burkert F, Carrara E, Foschi F, Döbele S, et al. Effect of antibiotic stewardship on the incidence of infection and colonisation with antibiotic-resistant bacteria and clostridium difficile infection: a systematic review and meta-analysis. Lancet Infect Dis. (2017) 17:990–1001. doi: 10.1016/S1473-3099(17)30325-0
298. Ikhimiukor OO, Odih EE, Donado-Godoy P, Okeke IN. A bottom-up view of antimicrobial resistance transmission in developing countries. Nat Microbiol. (2022) 7:757–65. doi: 10.1038/s41564-022-01124-w
299. Theuretzbacher U, Savic M, Outterson K. Market watch: innovation in the preclinical antibiotic pipeline. Nat Rev Drug Discov. (2017) 16:744–5. doi: 10.1038/nrd.2017.195
300. Wang Z, Koirala B, Hernandez Y, Zimmerman M, Park S, Perlin DS, et al. A naturally inspired antibiotic to target multidrug-resistant pathogens. Nature. (2022) 601:606–11. doi: 10.1038/s41586-021-04264-x
301. Myers AG, Clark RB. Discovery of macrolide antibiotics effective against multi-drug resistant gram-negative pathogens. Acc Chem Res. (2021) 54:1635–45. doi: 10.1021/acs.accounts.1c00020
302. Brown ED, Wright GD. Antibacterial drug discovery in the resistance era. Nature. (2016) 529:336–43. doi: 10.1038/nature17042
303. Cox G, Sieron A, King AM, De Pascale G, Pawlowski AC, Koteva K, et al. A common platform for antibiotic dereplication and adjuvant discovery. Cell Chem Biol. (2017) 24:98–109. doi: 10.1016/j.chembiol.2016.11.011
304. Hampton T. Novel programs and discoveries aim to combat antibiotic resistance. JAMA. (2015) 313:2411–3. doi: 10.1001/jama.2015.4738
305. Price LB, Rogers L, Lo K. Policy reforms for antibiotic use claims in livestock. Science. (2022) 376:130–2. doi: 10.1126/science.abj1823
Keywords: antibiotic resistant bacteria, antibiotic resistance, antibiotics, bibliometrics, keyword analysis
Citation: Sun G, Zhang Q, Dong Z, Dong D, Fang H, Wang C, Dong Y, Wu J, Tan X, Zhu P and Wan Y (2022) Antibiotic resistant bacteria: A bibliometric review of literature. Front. Public Health 10:1002015. doi: 10.3389/fpubh.2022.1002015
Received: 24 July 2022; Accepted: 20 October 2022;
Published: 17 November 2022.
Edited by:
Mohiuddin Md. Taimur Khan, Washington State University Tri-Cities, United StatesReviewed by:
Dejian Yu, Nanjing Audit University, ChinaSadaf Shabbir, Nanjing University of Information Science and Technology, China
Copyright © 2022 Sun, Zhang, Dong, Dong, Fang, Wang, Dong, Wu, Tan, Zhu and Wan. This is an open-access article distributed under the terms of the Creative Commons Attribution License (CC BY). The use, distribution or reproduction in other forums is permitted, provided the original author(s) and the copyright owner(s) are credited and that the original publication in this journal is cited, in accordance with accepted academic practice. No use, distribution or reproduction is permitted which does not comply with these terms.
*Correspondence: Yuehua Wan, d2FueXVlaHVhJiN4MDAwNDA7emp1dC5lZHUuY24=
†These authors have contributed equally to this work and share first authorship