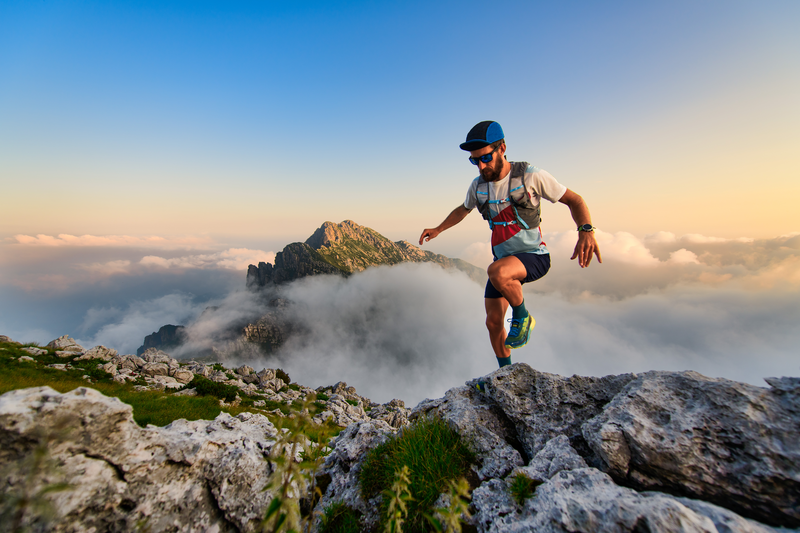
94% of researchers rate our articles as excellent or good
Learn more about the work of our research integrity team to safeguard the quality of each article we publish.
Find out more
OPINION article
Front. Public Health , 20 October 2022
Sec. Infectious Diseases – Surveillance, Prevention and Treatment
Volume 10 - 2022 | https://doi.org/10.3389/fpubh.2022.1001666
This article is part of the Research Topic Advances in the Diagnosis and Genomic Research of Surveillance-Response Activities in Emerging, Re-emerging, and Unidentified Infectious Diseases View all 12 articles
The world has been struggling with a major public health problem since December 2019: an infectious disease caused by a novel coronavirus called severe acute respiratory syndrome coronavirus 2 (SARS-CoV-2). Despite vaccination, people are still infected and die because of COVID-19 since the virus mutates very quickly (1, 2). While the world is struggling with the COVID-19 pandemic, a new virus called Monkeypox (MPXV) alerts scientists about whether a new pandemic will arise. Monkeypox is a zoonotic, neglected, and emerging disease caused by the MPXV belonging to the Orthopoxvirus genus of the Poxviridae family. MPXV was first identified in Macaca irus wild monkeys in 1958 in Denmark; it was the first time specified in humans in 1970 in the Democratic Republic of the Congo in a 9-month-old boy (3, 4). However, several rodent species were also reported as reservoirs of this virus (5). Monkeypox disease has been reported as an emerging outbreak affecting 43 countries with 2103 confirmed cases (6).
The transmission ways of MPXV are direct contact with an infected animal or infected person via body fluids, using contaminated objects, and inhaling virus-containing respiratory droplets. The incubation time of the disease takes 5–21 days, where the symptoms of MPXV infection are reported as headache, fever, muscle pain, back pain, swollen lymph nodes, chills, adenopathy, maculopapular rash, especially on the palms, and exhaustion. Lesions such as macules, papules, vesicles, pustules, and scabs have been reported mainly in the palms of the hands and the soles of the feet. There is no treatment for MPXV infection; however, smallpox vaccination is considered a treatment option (7, 8). The MPXV infection begins like other viral infections with the entry of the virus into the cells and replication, leading to the immune response in the host cells, such as blocking the antiviral T-cell activation and inflammatory cytokine production. However, cellular mechanisms of MPXV infection, host cell interactions, immune responses, and destruction are not fully understood in humans despite animals (9).
Viral infection and replication are tightly associated with the dysregulated immune system and inflammatory response. Since humans have complicated defense mechanisms against pathogens, viruses can quickly adapt to changing conditions such as the host's immune system and drug treatments. For instance, viruses deregulate cellular signaling pathways, including oxidative stress metabolism and cell death mechanisms, to escape the host's immune system (10, 11). The crucial step for virus replication is escaping from the cellular defense mechanism of the host cell (12, 13). Viruses are disparate from all living things; they don't inherently have their metabolism. Major cytosolic and mitochondrial metabolic pathways are altered in virus-infected cells (14, 15). Specific anabolic pathways such as glycolysis, glycogenolysis, pentose phosphate pathway (PPP), lipogenesis, cholesterol synthesis, one-carbon metabolism, and various transporters such as glucose and glutamine transporters are upregulated in virally infected cells (16, 17). It has also been investigated that the Warburg effect, which can be seen in cancer cells using glucose and producing lactate under normoxia conditions, can also be in the virus metabolism (18).
Various intrinsic and extrinsic factors regulate oxidative stress metabolism by balancing reactive oxygen species (ROS) and antioxidant capacity. Antioxidant metabolism is one of the major defense systems in many pathological conditions, including viral infections. PPP plays a vital role in antioxidant defense by regulating different enzymes. Glucose 6-phosphate dehydrogenase (G6PD) is the rate-limiting enzyme in the PPP involved in glutathione metabolism, antioxidant response, and bioenergetic and biosynthetic pathways (19–22).
The cytosolic hexokinase enzyme rapidly converts glucose to glucose-6-phosphate (G6P) to trap the glucose inside the cell by using an ATP molecule. This enzymatic reaction is not just specific to glucose; the hexokinase enzyme phosphorylates all the six-carbon sugars. After the phosphorylation of these sugar phosphates, many cellular conditions, such as hormones, energy status, infections, and all cellular signals, determine the fate of the phosphorylated molecule. It would enter breakdown or synthesis pathways according to the metabolic signals (23–30). G6PD enzyme is found in all cells and regulates the NADP+/NADPH ratio involved in fatty acid, cholesterol, and neurotransmitter biosynthesis. Additionally, NADPH is the essential coenzyme in detoxification reactions via regulation of the balance between the oxidized glutathione (GSSG)/reduced glutathione (GSH) by involving in the glutathione reductase (GR)-catalyzed enzymatic and non-enzymatic reactions (31–34).
Furthermore, the reduced form of NADPH is also vital in cytochrome p450 superfamily-catalyzed reactions, such as cytochrome p450 monooxygenases and NADPH-cytochrome P450 reductase responsible for the xenobiotic detoxification, antioxidant-defense system, and cellular redox homeostasis. Since GSH/GSSG ratio is the major biomarker for oxidative stress, preserving the GSH pool is vital to maintaining antioxidant defense in the cell (35). Virus-infected cells also affect the mitochondrial pathways due to the high demand for biosynthetic processes such as the proliferation of virions. Mitochondria is the major source of ROS and enhanced ROS induces mitochondrial dysfunction leading to impaired electron transport chain (ETC) and energy metabolism (36). However, NADPH also protects mitochondria stress via a mitochondrial membrane from the effects of ROS via NADPH-dependent antioxidant enzymes (37). Human viral diseases, including COVID-19, increase the production of ROS and impair antioxidant mechanisms leading to the impairment of the immune system (38). On the other hand, virus-induced immune response contributes to oxidative stress as well, where oxidative stress increases inflammation, leading to enhanced oxidative stress as a vicious cycle (39). Danger signals trigger the immune system through pattern recognition receptors (PRRs) belonging to the Toll-like (TLRs) and the NOD-like (NLRs) families, where oxidative stress involves in these processes at several levels, including the release of danger molecules, activation by PRRs, and their downstream pathways (40). All viral infections cause redox imbalance in the host; for instance, prototypic poxvirus vaccinia virus (VACV) enhances ROS production at the side of the infection to promote viral replication. Additionally, high levels of ROS are required for VACV infection (41).
Antioxidant administration has been reported to ameliorate virus-induced side effects or to reduce viral replication yield, according to various studies. For instance, N-acetyl-L-cysteine (NAC) inhibits pro-inflammatory mediators in the alveolar cells infected with influenza virus A and B and with the respiratory syncytial virus (RSV) (42). The antioxidant molecule butylated hydroxyanisole (BHA) treatment ameliorates RSV-induced lung inflammation (43). Terameprocol (TMP) is a methylated derivative of nordihydroguaiaretic acid, which is a phenolic antioxidant derived from creosote bush. TMP showed antiviral and anti-inflammatory effects via potently inhibiting the growth of both cowpox virus and vaccinia virus in vitro, where TMP treatment effectively reduced the infectious virus yield (44). On the other hand, resveratrol altered genome replication and post-replicative gene expression of MXPV (45). Resveratrol (RV) is a natural polyphenol non-flavonoid compound found in grapes, berries, and several other plants. RV is accepted as one of the powerful polyphenols with many positive effects on metabolism and health and significantly reduces the replication of MPXV (46, 47). No studies reveal the antioxidant's impact on the MPXV infection in humans since monkeypox is an emerging disease worldwide. Thus, the possible effect of the antioxidants on the MPXV infection in humans can be investigated to develop antioxidant-based therapeutic approaches to ease the severe symptoms.
All viruses depend entirely on the host's cell cellular metabolism, and every virus family has different molecular machinery to enter, using the host cells' energy and metabolic pathways multiplication and all steps in viral infection. However, we need novel studies to increase our knowledge on virus and virus-infected host cell metabolism, especially during the pandemic and the Monkeypox outbreak. Since antioxidants can reduce MPXV replication in vitro, according to the studies, antioxidant molecules can be investigated to develop therapeutic approaches or to ease the symptoms of MPXV infection.
DA and NU are responsible for the conceptualization and writing the manuscript. All authors contributed to the article and approved the submitted version.
The authors gratefully acknowledge the use of the services and facilities of the Koc University Research Center for Translational Medicine (KUTTAM), funded by the Presidency of Turkey, the Presidency of Strategy and Budget.
The authors declare that the research was conducted in the absence of any commercial or financial relationships that could be construed as a potential conflict of interest.
All claims expressed in this article are solely those of the authors and do not necessarily represent those of their affiliated organizations, or those of the publisher, the editors and the reviewers. Any product that may be evaluated in this article, or claim that may be made by its manufacturer, is not guaranteed or endorsed by the publisher.
The content is solely the responsibility of the authors and does not necessarily represent the official views of the Presidency of Strategy and Budget.
1. Aydemir D, Ulusu NN. Correspondence: angiotensin-converting enzyme 2 coated nanoparticles containing respiratory masks, chewing gums and nasal filters may be used for protection against COVID-19 infection. Travel Med Infect Dis. (2020) 37:101697. doi: 10.1016/j.tmaid.2020.101697
2. Harapan H, Itoh N, Yufika A, Winardi W, Keam S, Te H, et al. Coronavirus disease 2019 (COVID-19): a literature review. J Infect Public Health. (2020) 13:667–73. doi: 10.1016/j.jiph.2020.03.019
3. Parker S, Buller RM. A review of experimental and natural infections of animals with monkeypox virus between 1958 and 2012. Fut Virol. (2013) 8:129–57. doi: 10.2217/fvl.12.130
4. Alakunle E, Moens U, Nchinda G, Okeke MI. Monkeypox virus in nigeria: infection biology, epidemiology, and evolution. Viruses. (2020) 12:1257. doi: 10.3390/v12111257
5. Silva NIO, de Oliveira JS, Kroon EG, Trindade GS, Drumond BP. Here, there, and everywhere: the wide host range and geographic distribution of zoonotic orthopoxviruses. Viruses. (2020) 13:43. doi: 10.3390/v13010043
6. WHO. Multi-Country Monkeypox Outbreak: Situation Update. WHO. Available online at: https://www.who.int/emergencies/disease-outbreak-news/item/2022-DON393
7. Cann JA, Jahrling PB, Hensley LE, Wahl-Jensen V. Comparative pathology of smallpox and monkeypox in man and macaques. J Comp Pathol. (2013) 148:6–21. doi: 10.1016/j.jcpa.2012.06.007
8. Peter OJ, Kumar S, Kumari N, Oguntolu FA, Oshinubi K, Musa R. Transmission dynamics of monkeypox virus: a mathematical modelling approach. Model Earth Syst Environ. (2021) 8:3423–34. doi: 10.1007/s40808-021-01313-2
9. Tortorella D, Gewurz BE, Furman MH, Schust DJ, Ploegh HL. Viral subversion of the immune system. Annu Rev Immunol. (2000) 18:861–926. doi: 10.1146/annurev.immunol.18.1.861
10. Hanada S, Pirzadeh M, Carver KY, Deng JC. Respiratory viral infection-induced microbiome alterations and secondary bacterial pneumonia. Front Immunol. (2018) 9:2640. doi: 10.3389/fimmu.2018.02640
11. Pratheek BM, Saha S, Maiti PK, Chattopadhyay S, Chattopadhyay S. Immune regulation and evasion of mammalian host cell immunity during viral infection. Indian J Virol. (2013) 24:1–15. doi: 10.1007/s13337-013-0130-7
12. de Beeck AO, Caillet-Fauquet P. Viruses and the cell cycle. In: Progress in Cell Cycle Research. Boston, MA: Springer US (1997). p. 1–19. doi: 10.1007/978-1-4615-5371-7_1
13. Meylan E, Tschopp J. Toll-Like receptors and RNA helicases: two parallel ways to trigger antiviral responses. Mol Cell. (2006) 22:561–9. doi: 10.1016/j.molcel.2006.05.012
14. Morrison AJ. Cancer cell metabolism connects epigenetic modifications to transcriptional regulation. FEBS J. (2022) 289:1302–14. doi: 10.1111/febs.16032
15. Gusev E, Zhuravleva Y. Inflammation: a new look at an old problem. Int J Mol Sci. (2022) 23:4596. doi: 10.3390/ijms23094596
16. Perła-Kaján J, Jakubowski H. COVID-19 and one-carbon metabolism. Int J Mol Sci. (2022) 23:4181. doi: 10.3390/ijms23084181
17. Mayer KA, Stöckl J, Zlabinger GJ, Gualdoni GA. Hijacking the supplies: metabolism as a novel facet of virus-host interaction. Front Immunol. (2019) 10:1533. doi: 10.3389/fimmu.2019.01533
18. Icard P, Lincet H, Wu Z, Coquerel A, Forgez P, Alifano M, et al. The key role of Warburg effect in SARS-CoV-2 replication and associated inflammatory response. Biochimie. (2021) 180:169–77. doi: 10.1016/j.biochi.2020.11.010
19. Aydemir D, Ulusu NN. Is glucose-6-phosphate dehydrogenase enzyme deficiency a factor in Coronavirus-19 (COVID-19) infections and deaths? Pathog Glob Health. (2020) 114:109–10. doi: 10.1080/20477724.2020.1751388
20. Aydemir D, Daglioglu G, Candevir A, Kurtaran B, Bozdogan ST, Inal TC, et al. COVID-19 may enhance risk of thrombosis and hemolysis in the G6PD deficient patients. Nucleosides Nucleotides Nucleic Acids. (2021) 40:505–17. doi: 10.1080/15257770.2021.1897457
21. Lee SR, Roh JY, Ryu J, Shin HJ, Hong EJ. Activation of TCA cycle restrains virus-metabolic hijacking and viral replication in mouse hepatitis virus-infected cells. Cell Biosci. (2022) 12:7. doi: 10.1186/s13578-021-00740-z
22. Moreno-Altamirano MMB, Kolstoe SE, Sánchez-García FJ. Virus control of cell metabolism for replication and evasion of host immune responses. Front Cell Infect Microbiol. (2019) 9:95. doi: 10.3389/fcimb.2019.00095
23. Aydemir D, Öztaşci B, Barlas N, Ulusu NN. Effects of butylparaben on antioxidant enzyme activities and histopathological changes in rat tissues. Arch Indust Hyg Toxicol. (2019) 70:315–24. doi: 10.2478/aiht-2019-70-3342
24. Gök M, Ulusu NN, Tarhan N, Tufan C, Ozansoy G, Ari N, et al. Flaxseed protects against diabetes-induced glucotoxicity by modulating pentose phosphate pathway and glutathione-dependent enzyme activities in rats. J Diet Suppl. (2016) 13:339–51. doi: 10.3109/19390211.2015.1036188
25. Nóbrega-Pereira S, Fernandez-Marcos PJ, Brioche T, Gomez-Cabrera MC, Salvador-Pascual A, Flores JM, et al. G6PD protects from oxidative damage and improves healthspan in mice. Nat Commun. (2016) 7:10894. doi: 10.1038/ncomms10894
26. Bermúdez-Muñoz JM, Celaya AM, Hijazo-Pechero S, Wang J, Serrano M, Varela-Nieto I. G6PD overexpression protects from oxidative stress and age-related hearing loss. Aging Cell. (2020) 19:e13275. doi: 10.1111/acel.13275
27. Dashty M. A quick look at biochemistry: carbohydrate metabolism. Clin Biochem. (2013) 46:1339–52. doi: 10.1016/j.clinbiochem.2013.04.027
29. Tandogan B, Kuruüzüm-Uz A, Sengezer C, Güvenalp Z, Demirezer LÖ, Nuray Ulusu N. In vitro effects of rosmarinic acid on glutathione reductase and glucose 6-phosphate dehydrogenase. Pharm Biol. (2011) 49:587–94. doi: 10.3109/13880209.2010.533187
30. Ulusu NN. Glucose-6-phosphate dehydrogenase deficiency and Alzheimer's disease: partners in crime? The hypothesis. Med Hypotheses. (2015) 85:219–23. doi: 10.1016/j.mehy.2015.05.006
31. Aydemir D, Hashemkhani M, Durmusoglu EG, Acar HY, Ulusu NN. A new substrate for glutathione reductase: glutathione coated Ag2S quantum dots. Talanta. (2019) 194:501–6. doi: 10.1016/j.talanta.2018.10.049
32. Ulusu NN, Acan NL, Turan B, Tezcan EF. Inhibition of glutathione reductase by cadmium ion in some rabbit tissues and the protective role of dietary selenium. Biol Trace Elem Res. (2003) 91:151–6. doi: 10.1385/BTER:91:2:151
33. Ulusu NN, Tandogan B. Purification and kinetic properties of glutathione reductase from bovine liver. Mol Cell Biochem. (2007) 303:45–51. doi: 10.1007/s11010-007-9454-1
34. Aydemir D, Ulusu NN. Comment on the: molecular mechanism of CAT and SOD activity change under MPA-CdTe quantum dots induced oxidative stress in the mouse primary hepatocytes (Spectrochim Acta A Mol Biomol Spectrosc. 2019 Sep 5; 220:117104). Spectrochim Acta A Mol Biomol Spectrosc. (2020) 229:117792. doi: 10.1016/j.saa.2019.117792
35. Manikandan P, Nagini S. Cytochrome P450 structure, function and clinical significance: a review. Curr Drug Targets. (2018) 19:38–54. doi: 10.2174/1389450118666170125144557
36. Combs JA, Norton EB, Saifudeen ZR, Bentrup KHZ, Katakam P v., Morris CA, et al. Human cytomegalovirus alters host cell mitochondrial function during acute infection. J Virol. (2020) 94:e01183–19. doi: 10.1128/JVI.01183-19
37. Tarafdar A, Pula G. The role of NADPH oxidases and oxidative stress in neurodegenerative disorders. Int J Mol Sci. (2018) 19:3824. doi: 10.3390/ijms19123824
38. Hejrati A, Nurzadeh M, Roham M. Association of coronavirus pathogencity with the level of antioxidants and immune system. J Fam Med Prim Care. (2021) 10:609. doi: 10.4103/jfmpc.jfmpc_1007_20
39. Aydemir D, Ulusu NN. People with blood disorders can be more vulnerable during COVID-19 pandemic: a hypothesis paper. Transfus Apher Sci. (2021) 60:103080. doi: 10.1016/j.transci.2021.103080
40. Lugrin J, Rosenblatt-Velin N, Parapanov R, Liaudet L. The role of oxidative stress during inflammatory processes. Biol Chem. (2014) 395:203–30. doi: 10.1515/hsz-2013-0241
41. Bidgood SR, Samolej J, Novy K, Collopy A, Albrecht D, Krause M, et al. Poxviruses package viral redox proteins in lateral bodies and modulate the host oxidative response. PLoS Pathog. (2022) 18:e1010614. doi: 10.1371/journal.ppat.1010614
42. Mata M, Morcillo E, Gimeno C, Cortijo J. N-acetyl-l-cysteine (NAC) inhibit mucin synthesis and pro-inflammatory mediators in alveolar type II epithelial cells infected with influenza virus A and B and with respiratory syncytial virus (RSV). Biochem Pharmacol. (2011) 82:548–55. doi: 10.1016/j.bcp.2011.05.014
43. Castro SM, Guerrero-Plata A, Suarez-Real G, Adegboyega PA, Colasurdo GN, Khan AM, et al. Antioxidant treatment ameliorates respiratory syncytial virus–induced disease and lung inflammation. Am J Respir Crit Care Med. (2006) 174:1361–9. doi: 10.1164/rccm.200603-319OC
44. Pollara JJ, Laster SM, Petty ITD. Inhibition of poxvirus growth by terameprocol, a methylated derivative of nordihydroguaiaretic acid. Antiviral Res. (2010) 88:287–95. doi: 10.1016/j.antiviral.2010.09.017
45. Abdelkhalek A, Salem MZM, Kordy AM, Salem AZM, Behiry SI. Antiviral, antifungal, and insecticidal activities of eucalyptus bark extract: HPLC analysis of polyphenolic compounds. Microb Pathog. (2020) 147:104383. doi: 10.1016/j.micpath.2020.104383
46. Salehi B, Mishra A, Nigam M, Sener B, Kilic M, Sharifi-Rad M, et al. Resveratrol: a double-edged sword in health benefits. Biomedicines. (2018) 6:91. doi: 10.3390/biomedicines6030091
Keywords: antioxidant molecules, monkeypox, MPXV infection, oxidative stress, immune response, antioxidant enzymes
Citation: Aydemir D and Ulusu NN (2022) The possible importance of the antioxidants and oxidative stress metabolism in the emerging monkeypox disease: An opinion paper. Front. Public Health 10:1001666. doi: 10.3389/fpubh.2022.1001666
Received: 23 July 2022; Accepted: 30 September 2022;
Published: 20 October 2022.
Edited by:
Hui Chen, Brigham and Women's Hospital and Harvard Medical School, United StatesReviewed by:
Binsen Li, UCLA Health System, United StatesCopyright © 2022 Aydemir and Ulusu. This is an open-access article distributed under the terms of the Creative Commons Attribution License (CC BY). The use, distribution or reproduction in other forums is permitted, provided the original author(s) and the copyright owner(s) are credited and that the original publication in this journal is cited, in accordance with accepted academic practice. No use, distribution or reproduction is permitted which does not comply with these terms.
*Correspondence: Nuriye Nuray Ulusu, bnVsdXN1QGt1LmVkdS50cg==; Duygu Aydemir, ZGF5ZGVtaXIxNkBrdS5lZHUudHI=
Disclaimer: All claims expressed in this article are solely those of the authors and do not necessarily represent those of their affiliated organizations, or those of the publisher, the editors and the reviewers. Any product that may be evaluated in this article or claim that may be made by its manufacturer is not guaranteed or endorsed by the publisher.
Research integrity at Frontiers
Learn more about the work of our research integrity team to safeguard the quality of each article we publish.