- 1EPIUnit - Instituto de Saúde Pública da Universidade do Porto, Porto, Portugal
- 2Departamento de Ciências da Saúde Pública e Forenses e Educação Médica, Faculdade de Medicina, Universidade do Porto, Porto, Portugal
- 3Faculty of Medicine Purpan, LEASP UMR 1027, Inserm-Université Toulouse III Paul Sabatier, Toulouse, France
- 4Department of Epidemiology and Health Systems, Center for Primary Care and Public Health (Unisanté), University of Lausanne, Lausanne, Switzerland
- 5Unit of Population Epidemiology, Division of Primary Care Medicine, Department of Community Medicine, Primary Care and Emergency Medicine, Geneva University Hospitals, Geneva, Switzerland
Background: This systematic review aimed to summarize evidence reporting epigenetic and/or neuro-immuno-endocrine embedding of adverse childhood events (ACEs) in children, with a particular focus on the short-term biological effect of those experiences.
Methods: A search was conducted in PsycINFO®, PubMed®, Isi Web of Knowledge and Scopus, until July 2019, to identify papers reporting the short-term biological effects of exposure to ACEs.
Results: The search identified 58 studies, that were included in the review. Regarding exposure, the type of ACE more frequently reported was sexual abuse (n = 26), followed by life stressors (n = 20) and physical abuse (n = 19). The majority (n = 17) of studies showed a positive association between ACEs and biomarkers of the immune system. Regarding DNA methylation 18 studies showed more methylation in participants exposed to ACEs. Two studies presented the effect of ACEs on telomere length and showed that exposure was associated with shorter telomere length.
Conclusion: Overall the associations observed across studies followed the hypothesis that ACEs are associated with biological risk already at early ages. This is supporting evidence that ACEs appear to get “under the skin” and induce physiological changes and these alterations might be strongly associated with later development of disease.
Introduction
Adverse childhood experiences (ACEs) are stressful and traumatic events that occur in childhood and adolescence, until the age of 18 years and encompass various aspects of family dysfunction such as experiences of sexual abuse, physical or emotional abuse, and physical neglect (1). These experiences cause suffering to children (2) and undermine their sense of safety, stability, and bonding (3), and consequently impact their normal growth and development (4).
ACEs have been compellingly associated with a life-long increased risk for psychopathology and stress-related chronic health problems (5–10). Evidence shows that exposure to ACEs is strongly associated with a higher likelihood of developing ischemic heart disease, cancer, stroke, chronic bronchitis, emphysema or diabetes later in life and even with pre-mature death (1, 2, 11, 12). However, the potential mechanisms involved in the biological embodiment of social adversity in early ages that would be translated into an increased risk of disease later in life are still not fully understood (13–15).
Two main biological pathways are proposed to explain how the ACEs “get under the skin” and be associated with later negative health outcomes. Indirectly, it can be explained by the adoption of unhealthy behaviors (e.g., poor diet, sedentary behavior, smoking), that are socially patterned and thus more likely to be acquired by individuals from contexts of greater social adversity, and also associated with increased risk of disease later in life; or via a direct physiological disruption of regulatory pathways responsive to stress caused by adverse experiences. These alterations might be precursors of disease onset later in life, may start to operate early in life and be tracked over the life course. Exposure to adverse experiences may result in a variety of physiological changes during childhood (2, 16), including epigenetic mechanisms (13, 15), alteration of neural function and structure (13–15), increased activation of neurobiological systems, such as the hypothalamic-pituitary-adrenal (HPA) axis or the sympathetic nervous system (16, 17). Therefore, increased activation of these systems leads to a cascade of physiological processes (16–18), which in adults, was linked with the development of central fat, dysregulated carbohydrate metabolism and the accumulation of blood lipids in the arterial lining, all of which accelerate chronic disease development (19).
Evidence allows us to hypothesize that exposure to adversity during the first years of life might already be biologically embedded well before adult life, independently of the effects of behaviors in this association. Exposure to stressful circumstances between conception into adolescence causes a cascade of physiological responses that may modify an individual's biology in the long term in a way that makes them vulnerable to develop disease later in life (7, 9, 18, 20).
As a biomarker or a biological marker is a measurable indicator of some biological state or condition and is often measured and evaluated to examine normal biological processes, pathogenic processes, or pharmacologic responses to therapeutic intervention, in this work we aimed to identify biomarkers that are part of biological/physiological systems and therefore can suffer alterations as a result of exposure to adversity. We know that ACEs impact a child's life, and those “scars” can be identified and are perceptible, such as internalizing (e.g., anxiety, depression) and externalizing (e.g., aggression) problems and learning difficulties (21). This review aims to investigate the “hidden” effects of such exposures on children's biology that can be measured and quantified and may have a major impact already in childhood but can also have the potential to be programming children's health and translating into negative health outcomes later in life.
Thus, identify the physiological systems that may be immediately affected by the exposure to adversity already at early ages would allow understanding the pathways by which ACEs may impact later development of disease, to estimate the impact of ACEs would have later in life, and consequently define interventions to protect children in a trajectory of increased risk of poor health or to mitigate the effects already in place to avoid the development of disease in the adult life. Therefore, this review aims to systematically summarize evidence reporting epigenetic and/or neuro-immuno-endocrine embedding of adverse experiences in childhood. Specifically, it aims to describe which ACEs have been associated within a short time span until quantification of biological markers, to identify which physiological systems have been more investigated to explain the association between ACEs and later development of disease, and finally, to describe the impact and consequences of ACEs on the normal functioning of physiological systems. In addition, it is intended to discuss potential methodological issues that might explain inconsistencies among studies, which should be addressed and enhanced in future research.
Methods
Search Strategy
PsycINFO®, PubMed®, Isi Web of Knowledge and Scopus were searched until July 2019, to identify published papers reporting biological effects of exposure to ACEs before the age of 18 years. The keywords were chosen based on the literature and previously published theoretical reviews (22) and systematic reviews (23, 24), according to the usually used markers to measure biological alterations, adapted to each database and included the following terms: child maltreatment, child trauma, child adversity, early life stress, child abuse, child neglect, emotional stress, violence, bullying, and C-reactive Protein, CRP, Tumor Necrosis Factor, TNF-α, cytokine, interleukin, IL-6, inflammatory, inflammation, fibrinogen, white blood cell, methylation, DNA, DNA methylation, nervous system, amygdala, amygdala volume, hippocampus, hippocampal volume, prefrontal cortex volume, endocrine system, HPA axis, cortisol.
Selection of Studies
The list of references retrieved was screened independently by two reviewers (SSo and VR), following pre-defined criteria, to determine the eligibility of each article (Figure 1). Inclusion criteria are as following: case-control and cohort studies; original research; studies evaluating adverse childhood experiences; studies reporting biomarker measures in adulthood (≤18 years old); studies reporting an association between ACEs and biomarkers. The criteria for exclusion of studies were the following: (1) research not involving humans (e.g., in vitro or animal research); (2) non-eligible publication types (reviews, editorials, comments, guidelines, conference abstracts); (3) studies in disease setting samples; (4) studies reporting biomarker measures in adulthood (>18 years-old); (5) studies not reporting an association between ACEs and biomarkers; (6) other (studies evaluating allostatic load, adverse experiences during pregnancy, post-traumatic stress disorder, laboratory procedures to induce stress).
ACEs were defined considering Felitti exposure categories (1), namely psychological, physical and sexual abuse, and household dysfunction. Also, we included in the review any adverse experiences involving close relationships (caregivers, family and peers). Then, adverse experiences were categorized into: sexual abuse (includes any type of sexual abuse reported), life stressors (that includes a more thorough and comprehensive summary of adversities related with relationships such as the death of a family member, trouble with a teacher, exposure to community violence), physical abuse (includes abuse perpetrated by parents, caregivers or other relatives and by teachers) and physical neglect (includes physical neglect by parents or other caregivers). Biomarkers were defined according to the definition from the International Program on Chemical Safety, led by the World Health Organization (WHO) and in coordination with the United Nations and the International Labor Organization, as “any substance, structure, or process that can be measured in the body or its products and influence or predict the incidence of outcome or disease” (25). Biological markers were then divided by the biological mechanism with which they fitted better (Table 1).
The decisions taken independently by the authors in each step were compared, and discrepancies were solved by consensus or after discussion with a third researcher (SF). PRISMA flow diagram of the literature search is depicted in Figure 1.
Data Extraction
Two investigators (SSo and VR) independently extracted data from 58 studies regarding the year of publication, country, and region where the study was conducted, sample characteristics (sample, sample size, participant's age, female proportion, type of ACEs, the instrument used to measure adverse experiences, age at event exposure and biological marker assessed).
Data Synthesis and Analysis
Two summary tables of results were created, compiling the extracted information (Tables 2, 3). Studies were divided according to the different development phases of growth using the age at which ACEs occurred, as following: toddlerhood (0–2 years); childhood (3–12 years and further classification into play from 3 to 5 years and middle childhood from 6 to 12 years); and adolescence (13–18 years and divided in mid-adolescence from 13 to 15 years and late adolescence from 15 to 18 years). Due to heterogeneity of ACEs measures, analytic methods and in the biomarkers, a qualitative description of the association and the strength of the reported association were assigned based on the magnitude of the reported effect measures (85), defined according to the author's results description, as strong or weak, and statistical significance of the provided results. Results were then summarized in a table presenting positive and inverse associations (associations were classified as positive when authors reported that participants exposed to adverse experiences presented higher levels of biological markers, and as inverse when a decrease in the biological markers when adversity was reported), and the strength of association (Table 4).
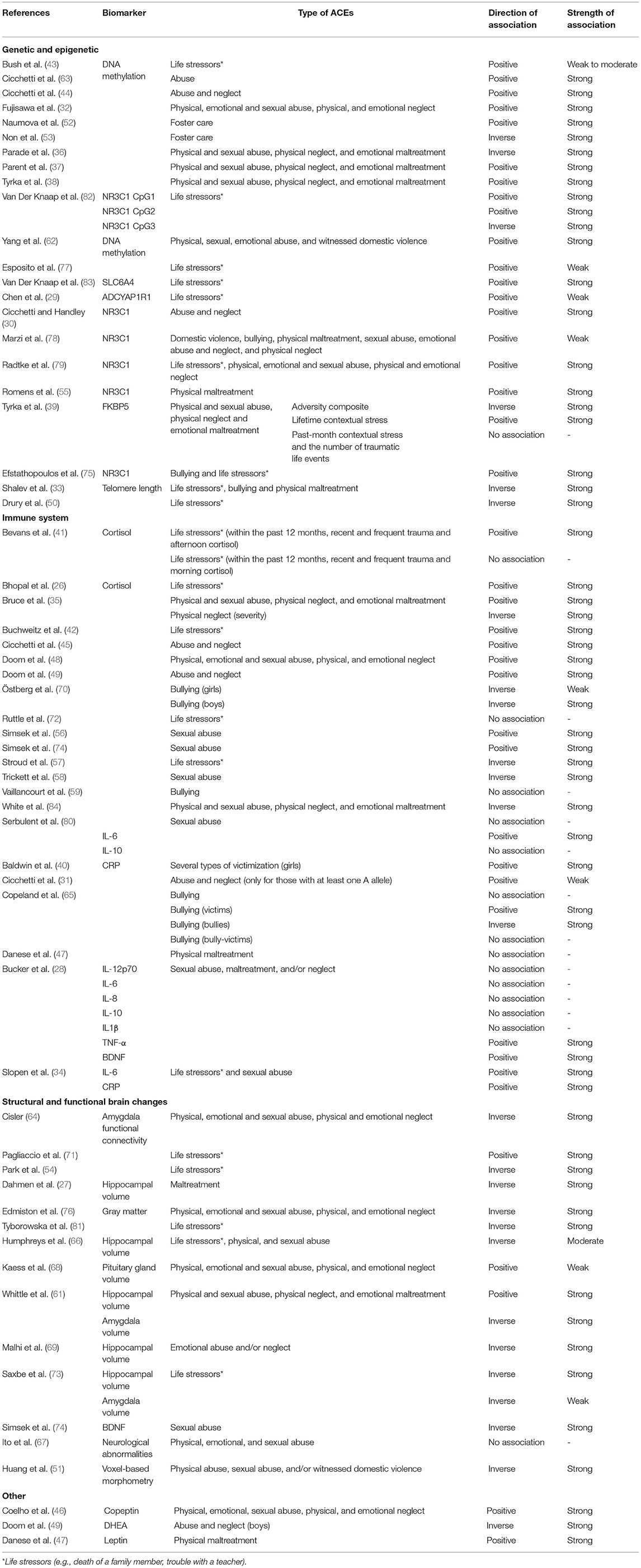
Table 4. Direction and strength of association between exposure to ACEs and biomarker by biological mechanism (positive associations indicate that biomarker increases with ACEs exposure and/or frequency; inverse associations indicate that biomarker decreases with ACEs exposure and/or frequency).
The Methodological Quality of Studies
The quality of reporting of the included studies was assessed using the Strengthening Reporting of Observational Studies in Epidemiology (STROBE) Statement: Guidelines for Reporting Observational Studies (86). All studies scoring higher than the median in the STROBE checklist for cohort, case-control, and cross-sectional studies (combined) and thus revealing a satisfactory to good quality were included (Table 2).
Results
The characteristics of the 58 included publications are described in Tables 2, 3.
Twelve studies were conducted in Europe (5 countries), 36 in the Americas (4 countries), six in Asia (4 countries), three in Australia and one in Africa (Tanzania). Most studies were conducted in the United States of America (USA) (31 studies), and the sample size varied from 21 to 5,802 participants. Studies were divided according to the time at which ACEs occurred. The distribution of papers is as follows: two papers during toddlerhood, 34 studies during childhood (seven from 3 to 12 years, five from play - 3 to 5 years- and 21 from middle childhood − 6–12 years), 15 studies during adolescence (12 studies in adolescence−13–18 years, one in mid-adolescence−13–15 years and two in late adolescence−15–18 years) and seven studies that present ACEs measured from an overall period—comprising experiences occurred before 18 years (Tables 2, 3). In childhood, most publications (15 studies) are in the “immune system” and “genetic and epigenetic” categories, while “structural and functional brain changes” has three publications. During adolescence there are six publications with biomarkers from the “immune system,” nine studies from the “structural and functional brain changes,” and seven studies from the “genetic and epigenetic” category.
Publication of studies increased over time, with most of the studies being published after 2012. The first study using DNA methylation as a biomarker of exposure to adversity was published in 2012, and after that, the number of papers studying the association with genetic and epigenetic biomarkers has been consistently increasing (Figure 2).
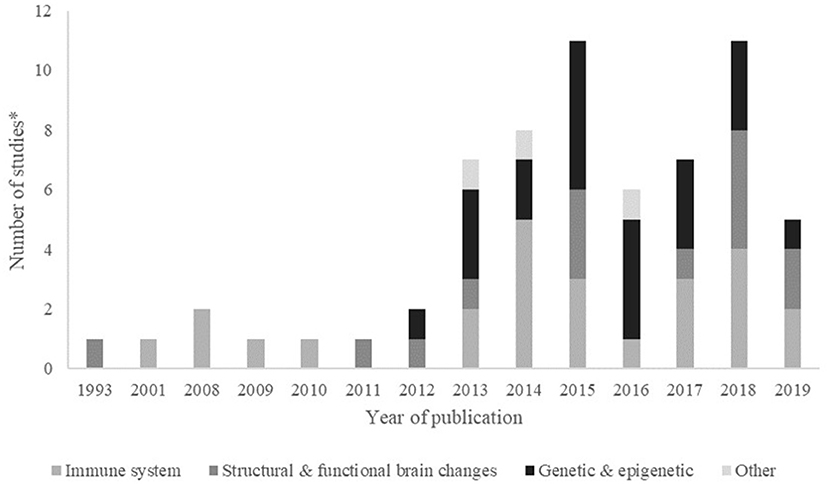
Figure 2. Frequency (number of studies*) by biological mechanism, published per year. *Some papers evaluated more than one biomarker, within or not the same biological mechanism. Year 2019 includes papers published online until July 2019.
We observed that ACEs were mostly assessed by standardized instruments, although some authors used non-validated questions (17 studies). The most frequently used instrument was the Childhood Trauma Questionnaire (nine studies), followed by the Maltreatment Classification System (eight studies). High heterogeneity was found among studies both in the exposure measurement and in the outcome summary measures. Regarding exposure, the most frequent adverse event measured in these studies was sexual abuse (26 studies, 16 studies in childhood and 10 studies in adolescence), followed by the life stressors category, that includes the death of a family member, trouble with a teacher, exposure to community violence, among others (20 studies, 10 in childhood, and 10 in adolescence), by physical abuse (18 studies, 11 studies in childhood and seven in adolescence) and physical neglect (15 studies, nine studies in childhood and six in adolescence). The minimum time from exposure to ACEs and measurement of biomarkers was 72 h and a maximum of 18 years. In toddlerhood the average time between exposure to ACEs and measurement of biomarkers was 2 years, in childhood was 7.2 years and during adolescence was 5.5 years (Table 2).
We categorized papers according to the outcome measured, i.e., referring to the biological marker used to assess the effect of ACEs on biological mechanisms. Biological markers were then divided by the biological mechanism with which they fitted better: “immune system” (including CRP, IL-6, TNF-α, IL-1b, IL-10, IL-12p70, IL-8, and cortisol), “structural and functional brain changes” (BDNF, hippocampal volume, amygdala volume, amygdala functional connectivity, gray matter, neurologic abnormalities, pituitary gland volume, voxel-based morphometry), “genetic and epigenetic” (including methylation and telomere length) and others [including copeptin, leptin, and dehydroepiandrosterone (DHEA)].
In almost all studies, exposure to ACEs was associated with biomarker alterations already during childhood, while six found no evidence of effect modification (Table 4).
Mainly due to the nature and type of biomarkers, associations observed can be expressed through an increase or a decrease in respective biomarkers. An increase is mainly reflected if higher biomarker levels are observed after exposure to ACEs than it would be expected if no exposure to ACEs occurred. A decrease will be defined if the observed biomarker levels are lower than after exposure to ACEs than they would be if no exposure to ACEs were in place. Thirty-nine studies presented a positive association, meaning that participants exposed to adverse experiences presented higher levels of biological markers, and 29 studies showed inverse associations, corresponding to a decrease in the biological markers when adversity was reported. We observed that most authors study the association of ACEs with biomarkers of the immune system followed by genetic and epigenetic biomarkers and then structural and functional brain changes.
Biomarkers
Immune System
Of the studies that addressed the biological consequences of ACEs on the immune system, 16 focused on cortisol, five on CRP, three on IL-6, two on IL-10, one on TNF-α, IL-1b, IL-12p70 and IL-8. Of these, the majority (17 studies) showed a positive association between ACEs exposure and biomarkers of the immune system, meaning that those exposed to adverse experiences presented higher levels of biomarkers of the immune system. Other studies showed an inverse association (five studies), with exposure to ACEs being associated with lower levels of biomarkers, or no association (five studies). The majority of studies presented strong associations, while four publications reported weak associations between exposure to ACEs and biomarkers. Regarding the type of ACEs more associated with biomarkers of the immune system, we saw that the categories sexual abuse, life stressors and physical abuse, neglect, maltreatment were the more prevalent (Figure 3). Changes in cortisol levels can be observed as early as between 3 and 6 years. Also, analyzing the distribution of publications by age, 11 studies on the immune system were conducted between the ages of 6 and 12 years. Four studies were conducted between 13 and 18 years and three between 3 and 12 years.
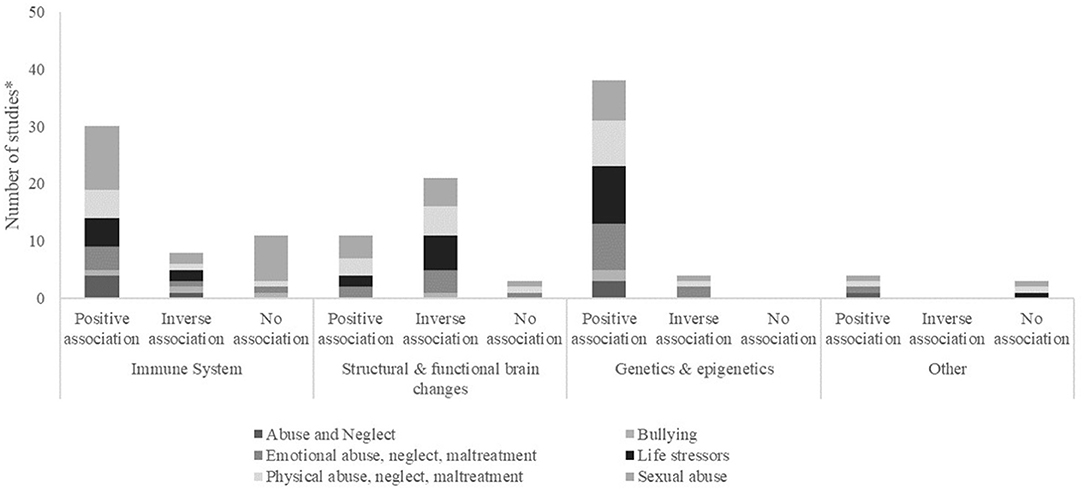
Figure 3. Frequency (number of studies*) categorized by presence of association (positive, negative) or absence of association (no association) found between exposure to ACEs and biomarker, by biological mechanism and type of ACE. *Some papers evaluated more than one biomarker, within or not the same biological mechanism.
Structural and Functional Brain Changes
The authors measured the impact of ACEs in the structural and functional brain changes, using several types of outcomes. Hippocampal volume was measured in five studies, and amygdala functional connectivity in three, BDNF, amygdala volume and gray matter in two, neurologic abnormalities, pituitary gland volume and voxel-based morphometry in one study each. Of these, three studies showed a moderate or weak association between exposure to ACEs and the outcomes measured, while all the others presented a strong association. Most studies showed an inverse association of ACEs, namely when reporting the association between sexual abuse, life stressors and physical abuse, neglect, maltreatment with structural and functional brain changes (Figure 3). Amygdala functional connectivity was the biomarker of the group “Structural and functional brain changes,” that presented changes measured earlier (mean = 6.1 years). Examining the distribution of publications by age, the majority of studies in this category (seven studies) were conducted between the ages of 13 and 18 years, and three studies were conducted between 6 and 12 years.
Genetic and Epigenetic
DNA methylation was assessed in 20 studies, with 18 showing more methylation in participants exposed to ACEs, four showing less methylation and one reporting no association. Methylation is observed in a multiplicity of genes or focused on specific genes, such as NR3C1, SLC6A4, and FKBP5. The effect of ACEs on telomere length was presented in two studies and showed that exposure was associated with shorter telomere length. The majority of associations observed was regarding the association with sexual abuse, life stressors and physical abuse (Table 4). DNA methylation is altered as early as between 3 and 5 years, and changes in telomere length can be observed at 10.2 years. Also, analyzing the distribution of publications by age, eight studies of the “genetic and epigenetic” category were conducted between 3 and 12 years and seven studies were conducted between the ages of 6 and 12 years.
Others
One study evaluated copeptin, and other DHEA, and both showed a positive association with ACEs exposure and were conducted in middle childhood, i.e., between 6 and 12 years old. A study on leptin showed no association with ACEs.
Exposures
Types of Abuse
Among the 11 studies evaluating abuse (28, 30, 38, 39, 44, 45, 48, 49, 62, 82, 83), only three present the associations with biomarkers stratified by types of abuse (45, 82, 83). In one study (45), it was observed that average morning cortisol in the group of sexually and physically abused participants (M = 0.99, SD = 0.83) was higher than in the non-maltreated (M = 0.32, SD = 0.67), emotionally maltreated (M = 0.35, SD = 0.58), neglected (M = 0.28, SD = 0.65), and physically abused (M = 0.14, SD = 0.62) participants. Regarding methylation results, results of linear regression models (82) showed that exposure to stressful life events between birth and 15 years and exposure to traumatic youth experiences significantly predicted higher methylation rates in amplicon 1. In amplicon 2, an only single exposure to sexual abuse predicted higher methylation rates (B = 0.44, P = 0.001). For amplicon 3, repeated exposure to other traumatic youth experiences was associated with lower methylation rates (B = −0.26, P = 0.01). The other study (83) reports that exposure to perinatal adversity or traumatic young experiences was not related to methylation, while exposure to stressful life events in the first 15 years of life significantly predicted higher methylation levels. In the model including both stressful life events during childhood and adolescence, exposure to stressful life events in adolescence was related to higher methylation levels.
Bullying Involvement
Six studies were identified evaluating bullying as an experience of adversity. One studied the impact of bullying on CRP, and found that CRP levels were higher in victims of bullying and lower in aggressors (65); two studies reported that DNA methylation was higher among victims of bullying (75, 78), and other that telomere length was shorter (33). Two other studies evaluated cortisol and one described lower levels of cortisol among victims of bullying (70) while another study found no association (59).
Discussion
This review shows that exposure to ACEs might impact the immune system, structural and functional brain changes and genetic and epigenetic changes, and these changes can be observed as early as childhood. However, a high heterogeneity is observed between included studies in ACEs measures, analytic methods and heterogeneity in the biomarkers.
Assessment of ACEs Among Children
In these studies, ACEs were assessed through different methods of inquiry and instruments. The development and testing of measures of retrospective adult recall of ACEs have been a fruitful area of research for the past few decades with several measures being developed and field-tested. Thus, most studies used retrospective measures to identify exposure to ACEs. The major issue raised is that several critical aspects of the measurement systems are inconsistent across studies, making it difficult to synthesize knowledge generated to date (87). In this review, by focusing on studies that assess exposure and outcome measured in the first 18 years of life, we see that biological alterations caused by exposure to traumatic events can be observed in the first years of life. The majority of included publications studies the effect of adversity in toddlerhood and childhood, i.e., before the age of 12 years (36 studies) while 15 studies evaluated adverse experiences between 13 and 18 years of age.
The heterogeneity on measurement instruments used gives rise to another assessment inconsistency, in particular, the fact that not all types of victimization are alike. The majority of studies presents results by adversity composite or number of adversities, by chronicity or timing of abuse, and few have analyzed by type of trauma. Some involve physical injury (sexual or physical abuse), whereas others involve psychological insult (emotional abuse or neglect). Also, some papers refer only to one type of adversity while others report several exposures to ACEs. In this review, we observed that sexual abuse was, among the categories of ACEs studied, the type of adversity that most studies presented in association with different biomarkers. This might be explained by the fact that the biological embedding of social experiences occurs sooner when the experience is very traumatic or repeated over time (88).
Potential Biological Mechanisms to the Embodiment of ACEs
The impact of ACEs in the immune system, structural and functional brain, as well as the genetic and epigenetic changes, was explored in the reviewed studies including samples of children. Overall, the associations observed followed the hypothesis that ACEs are associated with biological risk, which can be expressed through increases or decreases in respective biomarker levels above or under the expected levels if no exposure to ACEs was in place, depending on the nature and type of biomarker.
Immune and non-immune cells produce cytokines, messenger proteins such as TNF-α, IL-1β, IL-8, IL-6, IL-10, and IL-12p70, whose role is to regulate immune responses and interplay between pro and anti-inflammatory mediators (89, 90). CRP is an acute-phase protein synthesized by the liver in response to systemic effects of inflammation (91) and may intervene in the biological chain that embeds exposure to ACEs. Cortisol is the product of the HPA axis and has been widely used as a stress biomarker. All of these biomarkers play a role in the regulation of the immune responses and interplay between pro and anti-inflammatory mediators (89, 90) indicating an interrelated activation of the entire inflammatory cascade (92). More recently, evidence has reviewed the effect of early exposure to adversity on the chronic inflammatory state (23, 93) and concluded that early adversity is likely to increase inflammation (18, 23, 24, 93) and risk for poor health outcomes in adulthood (8, 93), independent of clinical comorbidities (23, 24). Our results show that these biomarkers seem to present alterations in the first 18 years of life, and thus the effect of exposure to childhood adversity in the immune system, in particular in the inflammatory biomarkers, where alterations were reported as early as between 3 and 6 years.
Several papers included in this review assessed methylation in a multiplicity of genes or focused on specific genes, such as NR3C1, SLC6A4, and FKBP5. These three genes seem to play an active role in the biological embodiment of exposure to ACEs and we hypothesized that the effect of adversities would be observed on alterations already at early ages. On one hand, NR3C1 is a gene known to encode glucocorticoid receptor, involved in inflammatory responses (94), and the higher level of methylation has been associated with childhood violence (95); and the SLC6A4 gene that encodes an integral membrane protein and seems to play a role in depression-susceptibility in people experiencing emotional trauma (96). FKBP5 encodes to a protein member of the immunophilin protein family, which play a role in immunoregulation and basic cellular processes. Genetic studies have identified a role of this gene in post-traumatic stress disorder, depression and anxiety (97) and have been found to interact with childhood trauma to predict the severity of adult post-traumatic stress disorder (98).
Although multiple types of epigenetic modifications have already been identified (99), all involve chemical modifications that regulate chromatin structure and/or DNA accessibility. Methylation, corresponding to the covalent modification of DNA whereby methyl groups are coupled to cytosine residues at CpG sites, is perhaps the best studied of these epigenetic mechanisms, due in part to its tractability (100). In this review, we identified several studies evaluating DNA methylation after exposure to ACEs. As dynamic molecular markers that have been shown to change with age (101) and experience (102), epigenetic signatures are attractive candidates for elucidating the underlying mechanisms of complex diseases (103).
Emerging evidence shows that environmental signals give rise to epigenetic changes, affecting phenotypic trajectories by altering the expression of genes (104). Thus, changes in epigenetic regulation of gene expression seem to be responsible for an increased immune activation via modifications of the HPA axis. Neuroplasticity-related methylation patterns (13, 105) may be a possible mechanism through which the association between early adverse experiences and long-term alterations in human stress response and immune systems are mediated.
Also, although not very conclusive, some structural and functional brain changes after exposure to adverse experiences have been identified by the studies explored in the review. Six studies concluded that hippocampal and amygdala volume and gray matter decreased after participants experienced adverse experiences. However, more evidence is needed to have a comprehensive view of the effect of ACEs in these systems.
Impact of ACEs on the Physiological Systems
Most of the included studies showed a significant impact of ACEs on the different physiological systems. Nevertheless, some studies showed increases in biomarker levels, while others presented decreases in those levels, depending on the nature and type of biomarker. Regarding telomere length, amygdala and hippocampal volume, the direction of the observed associations was consistent with our hypotheses. Telomere length decline is a normal consequence of cellular division, aging, differentiation, and senescence. Accelerated telomere shortening in adults has been associated with a history of childhood maltreatment and early adversity (106, 107). DNA methylation also can occur via hypermethylation, i.e., increased methylation, that was found in the promoter region of SLC6A4 in adult men after early and recent life stress (108), or hypomethylation, i.e., decreasing methylation, observed at intron 7 of FKBP5 in adults exposed to childhood trauma (109). Thus, the direction of methylation may depend on the gene, promoter and/or region studied. However, we did not expect to find different directions of association for biomarkers such as cortisol. But, there is some evidence of the attenuation hypothesis (110), suggesting that exposure to early and severe stress leads to an initial heightened stress response, that may be suppressed over time. This suppression may be suggestive of an adaptive response. Cortisol levels increase immediately after exposure to ACEs, and attenuate after a certain time, but continue to reflect the effects of severe trauma. Evidence from primates showed that early life stressors, when not tremendously severe, were associated with the subsequent development of biological and social resilience suggesting that ACEs represent a challenge that, when overcome, bring about functional adaptations (111). Regarding amygdala functional connectivity, some inconsistencies might be explained by within-subject variability and fluctuations in large-scale network patterns, including connectivity between a limbic and default mode network, results that seem to suggest that bi-nodal functional connectivity, may generally reflect larger-scale network patterns.
Additionally, our review shows that age at exposure is very different across publications, varying from <6 months to under 18 years old. The wide range of ages included is due to the inclusion of all experiences occurred before adult life, and thus during the major period of growth and development of a human being. Although there is great variability across studies, it has been defended that given the vast array of developmental processes occurring between conception and adolescence, every developmental window is in fact characterized by a different susceptibility depending on various environmental factors (112).
With this review, we cannot assess if the experiences reported are single episodes or if they are related to several experiences throughout childhood and adolescence resulting in cumulative exposures during these maturation periods. The exception is one study that specifically states that adversity must last for at least 6 months (51). There is evidence showing that cumulative exposures seem to have stronger associations with later health outcomes (1). This means that we could be looking at an interplay between biological functions and the environment across the life course which we cannot disentangle from the mechanism of accumulation. For example, an individual most at risk of developing cancer or ischemic heart disease after childhood exposure to violence or adversity is also more likely to have accumulated further negative experiences over time and to adopt risky health behaviors as a stress-reducing escape. However, by restricting the search to studies with participants 18-year-old or younger, the time for accumulation of risk-taking behaviors is sufficiently limited to avoid an impact on the studied association. Moreover, when compared to adult life, neurodevelopment during childhood and adolescence is more plastic and susceptible to programming influences from stressful environmental and social contexts (113). Also, even though there is evidence that different biomarkers show alterations upon exposure to ACEs, we cannot disregard that these alterations do not necessarily mean an increased risk of disease onset. The development and progression of disease may occur due to the interplay of a group of correlated molecules or a network, rather than from the malfunction of the individual gene, protein, or biomarker.
Biological Consequences of Bullying Involvement
It is not consensual to include bullying as an adverse experience in childhood. However, the awareness of this problem has widely increased, and it was shown to compromise the child's health. Literature settles on the conviction that social and psychological effects of bullying involvement may be independent of other childhood experiences (114), but the biological mechanisms of the embodiment of these experiences are still not fully elucidated. Although some authors agree that one potential mechanism is related to the chronic systemic low-grade inflammation (115), once inflammation is activated similarly by a diverse range of health risky behaviors (poor diet, sedentary life) and environmental challenges (low socioeconomic status, psychosocial stress) (116), others support the hypothesis of embodiment throughout HPA axis activation or autonomic nervous system (ANS) activation. Bullying has also some specificity as the type of involvement, as the victim or as the aggressor or both simultaneously might have a different biological impact. Evidence has shown that although being bullied predicted higher increases in CRP levels, bullying others predicted lower increases in CRP compared with those uninvolved in bullying, even when controlling for potential confounders (65). This review identified six studies evaluating bullying as an experience of adversity. Thus, further investigation is needed to explore the impact of children's type of involvement in bullying on different biological markers.
Nowadays, another important and prevalent form of bullying is by using technologies and social media, named cyberbullying. Due to the potential of widespread accessibility of victims and an infinite audience by using communication technologies (117), cyberbullying is another important source of stress and consequently to biological alterations that can later lead to disease. This is another important issue that deserves attention in future studies.
Strengths and Limitations
We believe that this review is comprehensive and robust enough to show the studied association. Even though there is always the possibility of residual confounding when exploring the association between childhood exposure and biological markers, we believe that studying these biomarkers already during childhood is an important step to eliminate the effect of health-risk behaviors that may confound this association. We must acknowledge that different biological, psychological and social aspects may contribute to the changes in the biomarkers studied, which are difficult to control for. However, our results are in line with previously reported associations (23, 104, 118, 119), and allow us to retrieve important conclusions on the effect of early exposure to ACEs and alterations in human stress response and biological systems, already during childhood. The reported biomarkers were also chosen based on previously published literature, and others emerged from the search, showing that several systems may be affected by adverse experiences in childhood. Even though we cannot exclude the hypothesis that more biomarkers might be affected by these experiences, we believe that our comprehensive search allowed us to catch most studies. Nevertheless, excluding allostatic load (AL) from our search might be considered a limitation. AL is posited to represent a sub-clinical measure of physiological wear and tear resulting from chronic exposure to life course stressors providing a measure of cumulative physiological dysregulation across multiple biological systems. We did not include the AL in our search because, despite its apparent utility, AL is affected by many methodological and conceptual choices that have hampered its potential clinical utility. Among those, we may highlight the difficulty to agree on a core set of biomarkers that define the construct, and the different AL scoring algorithms, limiting our ability to compare results across studies. Moreover, the cumulative nature of the allostatic load, identifying which biological system would suffer the most the impact of exposure to adversity would be more difficult. Also, to our knowledge, only one publication assessed the effect of maltreatment on allostatic load in children (120). In this study (120), participants were aged 8–10 years, included maltreated or non-maltreated low-income children that attended a summer research day camp. The authors observed that maltreatment did not independently predict differences in allostatic load levels.
Additionally, due to the diversity of ACEs measures, analytic methods and heterogeneity in the biomarkers we were not able to calculate a summary measure of association between ACEs and biological markers, and thus we were unable to conduct a meta-analysis. Instead, a qualitative description of the strength of association was assigned based on the magnitude of effect measures.
None of the exclusion criteria chosen to conduct this review is related to any aspect of human differences such as socioeconomic status, race, ethnicity, language, nationality, sex, gender identity, sexual orientation, religion, geography, ability, age, or culture. Thus, this review holds diversity as a core value and all papers were included based on the criteria defined and no other.
Understanding the biological mechanisms between ACEs and negative health outcomes is important as it offers avenues for treatments that could target these intermediary pathways to prevent or reduce the risk and burden of diseases such as cancer and cardiovascular disease. Nevertheless, we must be aware that some of the associations may be mediated by depression (121) or even life course socioeconomic and health behavioral factors (18), as these factors have been suggested as impacting inflammatory processes.
Conclusion
Despite the considerable inconsistency in ACEs assessment, most articles reviewed found an association between exposure to ACEs and biological markers, where the increase or decrease in the biomarker is associated with a heightened risk to subsequent health. Experiences of violence in childhood appear to “get under the skin” and induce physiological changes, such as increases in immune, structural, and functional brain changes, and genetic and epigenetic markers, from childhood. Thus, supporting evidence of a more immediate biological impact of these exposures and alterations might be strongly associated with the later development of disease. These results allow us to argue that the population's burden of disease could be reduced if all violence toward children was successfully prevented (122) and when it does occur, appropriately treated to mitigate the consequences (123). Exposure to adverse childhood experiences should be prevented as a question of human rights, and children should be protected against all types of abuse by law enforcement and providing nurturing childhood environments. Moreover, as adverse experiences seem to impact children's biology and children may be growing in a trajectory of worse health throughout life, beginning at early ages, when exposure to adversity cannot be prevented, clinicians may have an important role in helping identify any biological alterations related with adversity victimization and intervene to mitigate their impact on health.
Data Availability Statement
The original contributions presented in the study are included in the article/supplementary material, further inquiries can be directed to the corresponding author.
Author Contributions
SSo and SF designed the study and wrote the protocol. SSo managed the literature searches, extraction of data and analyses, and wrote the first draft of the manuscript. VR collaborated in the extraction of data. SF helped solve differences in the data extraction. SF, MK-I, and SSt reviewed and discussed the manuscript. All authors contributed to and have approved the final manuscript.
Funding
This work was supported by the European Regional Development Fund (ERDF) through the Operational Program Competitiveness and Internationalization and national funding from the Foundation for Science and Technology (FCT), Portuguese Ministry of Science, Technology and Higher Education under the projects BioAdversity: How childhood social adversity shapes health: The biology of social adversity (POCI-01- 0145-FEDER-016838; Reference PTDC/DTP-EPI/1687/2014), HIneC: When do health inequalities start? Understanding the impact of childhood social adversity on health trajectories from birth to early adolescence (POCI-01-0145-FEDER-029567; Reference PTDC/SAU-PUB/29567/2017). It is also supported by the Unidade de Investigação em Epidemiologia - Instituto de Saúde Pública da Universidade do Porto (EPIUnit) (POCI-01-0145-FEDER-006862; Reference UID/DTP/04750/2013), PhD Grants SFRH/BD/108742/2015 (to SSo) and SFRH/BD/103726/2014 (to VR) co-funded by FCT and the Human Capital Operational Programme (POCH/FSE Program); FCT Investigator contract CEECIND/01516/2017 (to SF). We also thank the support of the LIFEPATH project funded by the European Commission (Horizon 2020 Grant No. 633666).
Conflict of Interest
The authors declare that the research was conducted in the absence of any commercial or financial relationships that could be construed as a potential conflict of interest.
Publisher's Note
All claims expressed in this article are solely those of the authors and do not necessarily represent those of their affiliated organizations, or those of the publisher, the editors and the reviewers. Any product that may be evaluated in this article, or claim that may be made by its manufacturer, is not guaranteed or endorsed by the publisher.
References
1. Felitti VJ, Anda RF, Nordenberg D, Williamson DF, Spitz AM, Edwards V, et al. Relationship of childhood abuse and household dysfunction to many of the leading causes of death in adults. The adverse childhood experiences (ACE). Study Am J Prev Med. (1998) 14:245–58. doi: 10.1016/S0749-3797(98)00017-8
2. Nelson CA, Bhutta ZA, Burke Harris N, Danese A, Samara M. Adversity in childhood is linked to mental and physical health throughout life. BMJ. (2020) 371:m3048. doi: 10.1136/bmj.m3048
3. National Center for Injury Prevention and Control DOVP. Preventing Adverse Childhood Experiences. Atlanta, GA: National Center for Injury Prevention and Control DOVP (2021).
4. Frederiksen L. The Developing Brain and Adverse Childhood Experiences (ACEs). (2018). Available online at: https://www.pacesconnection.com/blog/the-developing-brain-and-adverse-childhood-experiences-aces (accessed February, 2020).
5. Glodich A. Traumatic exposure to violence: a comprehensive review of the child and adolescent literature. Smith Coll Stud Soc Work. (1998) 68:321–45. doi: 10.1080/00377319809517534
6. Moffitt TE, The Klaus-Grawe Think T. Childhood exposure to violence and lifelong health: clinical intervention science and stress biology research join forces. Dev Psychopathol. (2013) 25:801. doi: 10.1017/S0954579413000801
7. Kalmakis KA, Chandler GE. Health consequences of adverse childhood experiences: a systematic review. J Am Assoc Nurse Pract. (2015) 27:457–65. doi: 10.1002/2327-6924.12215
8. Hughes K, Bellis MA, Hardcastle KA, Sethi D, Butchart A, Mikton C, et al. The effect of multiple adverse childhood experiences on health: a systematic review and meta-analysis. Lancet Public Health. (2017) 2:e356–66. doi: 10.1016/S2468-2667(17)30118-4
9. Chang X, Jiang X, Mkandarwire T, Shen M. Associations between adverse childhood experiences and health outcomes in adults aged 18-59 years. PLoS ONE. (2019) 14:e0211850. doi: 10.1371/journal.pone.0211850
10. Sheffler JL, Stanley I, Sachs-Ericsson N. Chapter 4 - ACEs and mental health outcomes. In Asmundson GJG, Afifi TO, editors. Adverse Childhood Experiences. Cambridge, MA: Academic Press (2020). p. 47–69.
11. Kelly-Irving M, Lepage B, Dedieu D, Bartley M, Blane D, Grosclaude P, et al. Adverse childhood experiences and premature all-cause mortality. Eur J Epidemiol. (2013) 28:721–34. doi: 10.1007/s10654-013-9832-9
12. Kelly-Irving M, Mabile L, Grosclaude P, Lang T, Delpierre C. The embodiment of adverse childhood experiences and cancer development: potential biological mechanisms and pathways across the life course. Int J Public Health. (2013) 58:3–11. doi: 10.1007/s00038-012-0370-0
13. Liu D, Diorio J, Tannenbaum B, Caldji C, Francis D, Freedman A, et al. Maternal care, hippocampal glucocorticoid receptors, and hypothalamic-pituitary-adrenal responses to stress. Science. (1997) 277:1659–62. doi: 10.1126/science.277.5332.1659
14. Meaney MJ. Maternal care, gene expression, and the transmission of individual differences in stress reactivity across generations. Annu Rev Neurosci. (2001) 24:1161–92. doi: 10.1146/annurev.neuro.24.1.1161
15. Rutter M. Achievements and challenges in the biology of environmental effects. Proc Natl Acad Sci USA. (2012) 2:17149–53. doi: 10.1073/pnas.1121258109
16. Danese A, Mcewen BS. Adverse childhood experiences, allostasis, allostatic load, and age-related disease. Physiol Behav. (2012) 106:29–39. doi: 10.1016/j.physbeh.2011.08.019
17. Miller GE, Chen E, Parker KJ. Psychological stress in childhood and susceptibility to the chronic diseases of aging: moving toward a model of behavioral and biological mechanisms. Psychol Bull. (2011) 137:959–97. doi: 10.1037/a0024768
18. Chen M, Lacey RE. Adverse childhood experiences and adult inflammation: findings from the 1958 British birth cohort. Brain Behav Immun. (2018) 69:582–90. doi: 10.1016/j.bbi.2018.02.007
19. Mcewen BS, Gianaros PJ. Central role of the brain in stress and adaptation: links to socioeconomic status, health, and disease. Ann N Y Acad Sci. (2010) 1186:190–222. doi: 10.1111/j.1749-6632.2009.05331.x
20. Kelly-Irving M, Delpierre C. A critique of the adverse childhood experiences framework in epidemiology and public health: uses and misuses. Soc Policy Soc. (2019) 18:445–56. doi: 10.1017/S1474746419000101
21. Burke NJ, Hellman JL, Scott BG, Weems CF, Carrion VG. The impact of adverse childhood experiences on an urban pediatric population. Child Abuse and Neglect. (2011) 35:408–13. doi: 10.1016/j.chiabu.2011.02.006
22. Bick J, Nelson CA. Early adverse experiences and the developing brain. Neuropsychopharmacology. (2016) 41:177–96. doi: 10.1038/npp.2015.252
23. Coelho R, Viola TW, Walss-Bass C, Brietzke E, Grassi-Oliveira R. Childhood maltreatment and inflammatory markers: a systematic review. Acta Psychiatr Scand. (2014) 129:180–92. doi: 10.1111/acps.12217
24. Baumeister D, Akhtar R, Ciufolini S, Pariante CM, Mondelli V. Childhood trauma and adulthood inflammation: a meta-analysis of peripheral C-reactive protein, interleukin-6 and tumour necrosis factor-alpha. Mol Psychiatry. (2016) 21:642–9. doi: 10.1038/mp.2015.67
25. World Health Organization, International Programme on Chemical Safety. Biomarkers in Risk Assessment: Validity and Validation. Geneva: WHO (2001).
26. Bhopal S, Verma D, Roy R, Soremekun S, Kumar D, Bristow M, et al. The contribution of childhood adversity to cortisol measures of early life stress amongst infants in rural India: findings from the early life stress sub-study of the SPRING cluster randomised controlled trial (SPRING-ELS). Psychoneuroendocrinology. (2019) 107:241–50. doi: 10.1016/j.psyneuen.2019.05.012
27. Dahmen B, Puetz VB, Scharke W, Von Polier GG, Herpertz-Dahlmann B, Konrad K. Effects of early-life adversity on hippocampal structures and associated HPA axis functions. Dev Neurosci. (2018) 40:13–22. doi: 10.1159/000484238
28. Bucker J, Fries GR, Kapczinski F, Post RM, Yatham LN, Vianna P, et al. Brain-derived neurotrophic factor and inflammatory markers in school-aged children with early trauma. Acta Psychiatr Scand. (2015) 131:360–8. doi: 10.1111/acps.12358
29. Chen W, Boutaoui N, Brehm JM, Han YY, Schmitz C, Cressley A, et al. ADCYAP1R1 and asthma in Puerto Rican children. Am J Respir Crit Care Med. (2013) 187:584–8. doi: 10.1164/rccm.201210-1789OC
30. Cicchetti D, Handley ED. Methylation of the glucocorticoid receptor gene, nuclear receptor subfamily 3, group C, member 1 (.NR3C1), in maltreated and nonmaltreated children: associations with behavioral undercontrol, emotional lability/negativity, and externalizing and internalizing symptoms. Dev Psychopathol. (2017) 29:1795–806. doi: 10.1017/S0954579417001407
31. Cicchetti D, Handley ED, Rogosch FA. Child maltreatment, inflammation, and internalizing symptoms: investigating the roles of C-reactive protein, gene variation, and neuroendocrine regulation. Dev Psychopathol. (2015) 27:553–66. doi: 10.1017/S0954579415000152
32. Fujisawa TX, Nishitani S, Takiguchi S, Shimada K, Smith AK, Tomoda A. Oxytocin receptor DNA methylation and alterations of brain volumes in maltreated children. Neuropsychopharmacology. (2019) 44:2045–53. doi: 10.1038/s41386-019-0414-8
33. Shalev I, Moffitt TE, Sugden K, Williams B, Houts RM, Danese A, et al. Exposure to violence during childhood is associated with telomere erosion from 5 to 10 years of age: a longitudinal study. Mol Psychiatry. (2013) 18:576–81. doi: 10.1038/mp.2012.32
34. Slopen N, Kubzansky LD, Mclaughlin KA, Koenen KC. Childhood adversity and inflammatory processes in youth: a prospective study. Psychoneuroendocrinology. (2013) 38:188–200. doi: 10.1016/j.psyneuen.2012.05.013
35. Bruce J, Fisher PA, Pears KC, Levine S. Morning cortisol levels in preschool-aged foster children: differential effects of maltreatment type. Dev Psychobiol. (2009) 51:14–23. doi: 10.1002/dev.20333
36. Parade SH, Parent J, Rabemananjara K, Seifer R, Marsit CJ, Yang BZ, et al. Change in FK506 binding protein 5 (FKBP5) methylation over time among preschoolers with adversity. Dev Psychopathol. (2017) 29:1627–34. doi: 10.1017/S0954579417001286
37. Parent J, Parade SH, Laumann LE, Ridout KK, Yang BZ, Marsit CJ, et al. Dynamic stress-related epigenetic regulation of the glucocorticoid receptor gene promoter during early development: the role of child maltreatment. Dev Psychopathol. (2017) 29:1635–48. doi: 10.1017/S0954579417001298
38. Tyrka AR, Parade SH, Eslinger NM, Marsit CJ, Lesseur C, Armstrong DA, et al. Methylation of exons 1(D), 1(F), and 1(H) of the glucocorticoid receptor gene promoter and exposure to adversity in preschool-aged children. Dev Psychopathol. (2015) 27:577–85. doi: 10.1017/S0954579415000176
39. Tyrka AR, Ridout KK, Parade SH, Paquette A, Marsit CJ, Seifer R. Childhood maltreatment and methylation of FK506 binding protein 5 gene (FKBP5). Dev Psychopathol. (2015) 27:1637–45. doi: 10.1017/S.0954579415000991
40. Baldwin JR, Arseneault L, Caspi A, Fisher HL, Moffitt TE, Odgers CL, et al. Childhood victimization and inflammation in young adulthood: a genetically sensitive cohort study. Brain Behav Immun. (2018) 67:211–7. doi: 10.1016/j.bbi.2017.08.025
41. Bevans K, Cerbone A, Overstreet S. Relations between recurrent trauma exposure and recent life stress and salivary cortisol among children. Dev Psychopathol. (2008) 20:257–72. doi: 10.1017/S0954579408000126
42. Buchweitz A, De Azeredo LA, Sanvicente-Vieira B, Metsavaht Cará V, Bianchini Esper N, Soder RB, et al. Violence and Latin-American preadolescents: a study of social brain function and cortisol levels. Dev Sci. (2019) 22:e12799. doi: 10.1111/desc.12799
43. Bush NR, Edgar RD, Park M, Macisaac JL, Mcewen LM, Adler NE, et al. The biological embedding of early-life socioeconomic status and family adversity in children's genome-wide DNA methylation. Epigenomics. (2018) 10:1445–61. doi: 10.2217/epi-2018-0042
44. Cicchetti D, Hetzel S, Rogosch FA, Handley ED, Toth SL. An investigation of child maltreatment and epigenetic mechanisms of mental and physical health risk. Dev Psychopathol. (2016) 28:1305–17. doi: 10.1017/S0954579416000869
45. Cicchetti D, Rogosch FA, Cox Kearns S. Diverse patterns of neuroendocrine activity in maltreated children. Dev Psychopathol. (2001) 13:677–93. doi: 10.1017/S0954579401003145
46. Coelho R, Levandowski ML, Mansur RB, Da Cunha GR, Asevedo E, Zugman A, et al. Serum copeptin in children exposed to maltreatment. Psychiatry Clin Neurosci. (2016) 70:434–41. doi: 10.1111/pcn.12412
47. Danese A, Dove R, Belsky DW, Henchy J, Williams B, Ambler A, et al. Leptin deficiency in maltreated children. Transl Psychiatry. (2014) 4:e446. doi: 10.1038/tp.2014.79
48. Doom JR, Cicchetti D, Rogosch FA. Longitudinal patterns of cortisol regulation differ in maltreated and nonmaltreated children. J Am Acad Child Adolesc Psychiatry. (2014) 53:1206–15. doi: 10.1016/j.jaac.2014.08.006
49. Doom JR, Cicchetti D, Rogosch FA, Dackis MN. Child maltreatment and gender interactions as predictors of differential neuroendocrine profiles. Psychoneuroendocrinology. (2013) 38:1442–54. doi: 10.1016/j.psyneuen.2012.12.019
50. Drury SS, Mabile E, Brett ZH, Esteves K, Jones E, Shirtcliff EA, et al. The association of telomere length with family violence and disruption. Pediatrics. (2014) 134:e128–37. doi: 10.1542/peds.2013-3415
51. Huang H, Gundapuneedi T, Rao U. White matter disruptions in adolescents exposed to childhood maltreatment and vulnerability to psychopathology. Neuropsychopharmacology. (2012) 37:2693–701. doi: 10.1038/npp.2012.133
52. Naumova OY, Lee M, Koposov R, Szyf M, Dozier M, Grigorenko EL. Differential patterns of whole-genome DNA methylation in institutionalized children and children raised by their biological parents. Dev Psychopathol. (2012) 24:143–55. doi: 10.1017/S0954579411000605
53. Non AL, Hollister BM, Humphreys KL, Childebayeva A, Esteves K, Zeanah CH, et al. DNA methylation at stress-related genes is associated with exposure to early life institutionalization. Am J Phys Anthropol. (2016) 161:84–93. doi: 10.1002/ajpa.23010
54. Park AT, Leonard JA, Saxler PK, Cyr AB, Gabrieli JDE, Mackey AP. Amygdala-medial prefrontal cortex connectivity relates to stress and mental health in early childhood. Soc Cogn Affect Neurosci. (2018) 13:430–9. doi: 10.1093/scan/nsy017
55. Romens SE, Mcdonald J, Svaren J, Pollak SD. Associations between early life stress and gene methylation in children. Child Dev. (2015) 86:303–9. doi: 10.1111/cdev.12270
56. Simsek S, Kaplan I, Uysal C, Yuksel T, Alaca R. The levels of cortisol, oxidative stress, and DNA damage in the victims of childhood sexual abuse: a preliminary study. J Child Sex Abus. (2016) 25:175–84. doi: 10.1080/10538712.2016.1123790
57. Stroud CB, Chen FR, Doane LD, Granger DA. Early adversity and internalizing symptoms in adolescence: mediation by individual differences in latent trait cortisol. Dev Psychopathol. (2019) 31:509–24. doi: 10.1017/S0954579418000044
58. Trickett PK, Noll JG, Susman EJ, Shenk CE, Putnam FW. Attenuation of cortisol across development for victims of sexual abuse. Dev Psychopathol. (2010) 22:165–75. doi: 10.1017/S0954579409990332
59. Vaillancourt T, Duku E, Decatanzaro D, Macmillan H, Muir C, Schmidt LA. Variation in hypothalamic-pituitary-adrenal axis activity among bullied and non-bullied children. Aggress Behav. (2008) 34:294–305. doi: 10.1002/ab.20240
61. Whittle S, Dennison M, Vijayakumar N, Simmons JG, Yücel M, Lubman DI, et al. Childhood maltreatment and psychopathology affect brain development during adolescence. J Am Acad Child Adolesc Psychiatry. (2013) 52:940–52.e941. doi: 10.1016/j.jaac.2013.06.007
62. Yang BZ, Zhang H, Ge W, Weder N, Douglas-Palumberi H, Perepletchikova F, et al. Child abuse and epigenetic mechanisms of disease risk. Am J Prev Med. (2013) 44:101–7. doi: 10.1016/j.amepre.2012.10.012
63. Cicchetti D, Hecker T, Radtke KM, Hermenau K, Papassotiropoulos A, Elbert T. Associations among child abuse, mental health, and epigenetic modifications in the proopiomelanocortin gene (POMC): a study with children in Tanzania. Dev Psychopathol. (2016) 28:1401–12. doi: 10.1017/S0954579415001248
64. Cisler JM. Childhood trauma and functional connectivity between amygdala and medial prefrontal cortex: a dynamic functional connectivity and large-scale network perspective. Front Syst Neurosci. (2017) 11:e00029. doi: 10.3389/fnsys.2017.00029
65. Copeland WE, Wolke D, Lereya ST, Shanahan L, Worthman C, Costello EJ. Childhood bullying involvement predicts low-grade systemic inflammation into adulthood. Proc Natl Acad Sci USA. (2014) 111:7570–5. doi: 10.1073/pnas.1323641111
66. Humphreys KL, King LS, Sacchet MD, Camacho MC, Colich NL, Ordaz SJ, et al. Evidence for a sensitive period in the effects of early life stress on hippocampal volume. Dev Sci. (2019) 22:12775. doi: 10.1111/desc.12775
67. Ito Y, Teicher MH, Glod CA, Harper D, Magnus E, Gelbard HA. Increased prevalence of electrophysiological abnormalities in children with psychological, physical, and sexual abuse. J Neuropsychiatry Clin Neurosci. (1993) 5:401–8. doi: 10.1176/jnp.5.4.401
68. Kaess M, Whittle S, O'brien-Simpson L, Allen NB, Simmons JG. Childhood maltreatment, pituitary volume and adolescent hypothalamic-pituitary-adrenal axis -Evidence for a maltreatment-related attenuation. Psychoneuroendocrinology. (2018) 98:39–45. doi: 10.1016/j.psyneuen.2018.08.004
69. Malhi GS, Das P, Outhred T, Irwin L, Gessler D, Bwabi Z, et al. The effects of childhood trauma on adolescent hippocampal subfields. Aust N Z J Psychiatry. (2019) 53:447–57. doi: 10.1177/0004867418824021
70. Östberg V, Låftman SB, Modin B, Lindfors P. Bullying as a stressor in mid-adolescent girls and boys-associations with perceived stress, recurrent pain, and salivary cortisol. Int J Environ Res Public Health. (2018) 15:364. doi: 10.3390/ijerph15020364
71. Pagliaccio D, Luby JL, Bogdan R, Agrawal A, Gaffrey MS, Belden AC, et al. Amygdala functional connectivity, HPA axis genetic variation, and life stress in children and relations to anxiety and emotion regulation. J Abnorm Psychol. (2015) 124:817–33. doi: 10.1037/abn0000094
72. Ruttle PL, Armstrong JM, Klein MH, Essex MJ. Adolescent internalizing symptoms and negative life events: the sensitizing effects of earlier life stress and cortisol. Dev Psychopathol. (2014) 26:1411–22. doi: 10.1017/S0954579414001114
73. Saxbe D, Khoddam H, Stoycos SA, Margolin G, Kaplan JT, Piero LD, et al. Community violence exposure in early adolescence: longitudinal associations with hippocampal and amygdala volume and resting state connectivity. Dev Sci. (2018) 21:12686. doi: 10.1111/desc.12686
74. Simsek S, Yuksel T, Kaplan I, Uysal C, Alaca R. Examining the levels of BDNF and cortisol in children and adolescent victims of sexual abuse–a preliminary study. Compr Psychiatry. (2015) 61:23–7. doi: 10.1016/j.comppsych.2015.04.013
75. Efstathopoulos P, Andersson F, Melas PA, Yang LL, Villaescusa JC, Ruegg J, et al. NR3C1 hypermethylation in depressed and bullied adolescents. Transl Psychiatry. (2018) 8. doi: 10.1038/s41398-018-0169-8
76. Edmiston EE, Wang F, Mazure CM, Guiney J, Sinha R, Mayes LC, et al. Corticostriatal-limbic gray matter morphology in adolescents with self-reported exposure to childhood maltreatment. Arch Pediatr Adolesc Med. (2011) 165:1069–77. doi: 10.1001/archpediatrics.2011.565
77. Esposito EA, Jones MJ, Doom JR, Macisaac JL, Gunnar MR, Kobor MS. Differential DNA methylation in peripheral blood mononuclear cells in adolescents exposed to significant early but not later childhood adversity. Dev Psychopathol. (2016) 28:1385–99. doi: 10.1017/S0954579416000055
78. Marzi SJ, Sugden K, Arseneault L, Belsky DW, Burrage J, Corcoran DL, et al. Analysis of DNA methylation in young people: limited evidence for an association between victimization stress and epigenetic variation in blood. Am J Psychiatry. (2018) 175:517–29. doi: 10.1176/appi.ajp.2017.17060693
79. Radtke KM, Schauer M, Gunter HM, Ruf-Leuschner M, Sill J, Meyer A, et al. Epigenetic modifications of the glucocorticoid receptor gene are associated with the vulnerability to psychopathology in childhood maltreatment. Transl Psychiatry. (2015) 5:e571. doi: 10.1038/tp.2015.63
80. Serbulent K, Ozlem K, Murat T. Inflammatory parameters in sexually abused children. Saudi Med J. (2017) 38:1213–8. doi: 10.15537/smj.2017.12.21463
81. Tyborowska A, Volman I, Niermann HCM, Pouwels JL, Smeekens S, Cillessen AHN, et al. Early-life and pubertal stress differentially modulate grey matter development in human adolescents. Sci Rep. (2018) 8. doi: 10.1038/s41598-018-27439-5
82. Van Der Knaap LJ, Riese H, Hudziak JJ, Verbiest MM, Verhulst FC, Oldehinkel AJ, et al. Glucocorticoid receptor gene (NR3C1) methylation following stressful events between birth and adolescence. TRAILS study Transl Psychiatry. (2014) 4:e381. doi: 10.1038/tp.2014.22
83. Van Der Knaap LJ, Riese H, Hudziak JJ, Verbiest MM, Verhulst FC, Oldehinkel AJ, et al. Adverse life events and allele-specific methylation of the serotonin transporter gene (SLC6A4) in adolescents: the TRAILS study. Psychosom Med. (2015) 77:246–55. doi: 10.1097/PSY.0000000000000159
84. White LO, Ising M, Von Klitzing K, Sierau S, Michel A, Klein AM, et al. Reduced hair cortisol after maltreatment mediates externalizing symptoms in middle childhood and adolescence. J Child Psychol Psychiatry Allied Discip. (2017) 58:998–1007. doi: 10.1111/jcpp.12700
85. Johnson SC, Cavallaro FL, Leon DA. A systematic review of allostatic load in relation to socioeconomic position: poor fidelity and major inconsistencies in biomarkers employed. Soc Sci Med. (2017) 192:66–73. doi: 10.1016/j.socscimed.2017.09.025
86. Elm EV, Altman D, Egger M. The strengthening the reporting of observational studies in epidemiology (STROBE) statement: guidelines for reporting observational studies. Ann Intern Med. (2007) 147:573–7. doi: 10.7326/0003-4819-147-8-200710160-00010
87. Baker AJL. Adult recall of childhood psychological maltreatment: definitional strategies and challenges. Child Youth Serv Rev. (2009) 31:703–14. doi: 10.1016/j.childyouth.2009.03.001
88. Hertzman C. Putting the concept of biological embedding in historical perspective. Proc Natl Acad Sci USA. (2012) 109(Suppl. 2):17160–7. doi: 10.1073/pnas.1202203109
89. Esposito E, Cuzzocrea S. TNF-alpha as a therapeutic target in inflammatory diseases, ischemia-reperfusion injury and trauma. Curr Med Chem. (2009) 16:3152–67. doi: 10.2174/092986709788803024
90. Hirano T. Revisiting the 1986 molecular cloning of interleukin 6. Front Immunol. (2014) 5:e00456. doi: 10.3389/fimmu.2014.00456
91. Pepys MB, Baltz ML. Acute phase proteins with special reference to C-reactive protein and related proteins (pentaxins) and serum amyloid A protein. Adv Immunol. (1983) 34:141–212. doi: 10.1016/S0065-2776(08)60379-X
92. Bruunsgaard H, Andersen-Ranberg K, Jeune B, Pedersen AN, Skinhoj P, Pedersen BK. A high plasma concentration of TNF-alpha is associated with dementia in centenarians. J Gerontol A Biol Sci Med Sci. (1999) 54:M357–364. doi: 10.1093/gerona/54.7.M357
93. Chiang JJ, Taylor SE, Bower JE. Early adversity, neural development, and inflammation. Dev Psychobiol. (2015) 57:887–907. doi: 10.1002/dev.21329
94. NCBI. NR3C1 Nuclear Receptor Subfamily 3 GROUP C MEMBER 1 [Homo sapiens (human)]. NCBI (2017). Available online at: https://www.ncbi.nlm.nih.gov/gene?Db=gene&Cmd=DetailsSearch&Term=2908 (accessed February 2020).
95. Mcgowan PO, Sasaki A, D'alessio AC, Dymov S, Labonte B, Szyf M, et al. Epigenetic regulation of the glucocorticoid receptor in human brain associates with childhood abuse. Nat Neurosci. (2009) 12:342–8. doi: 10.1038/nn.2270
96. NCBI. SLC6A4 Solute Carrier Family 6 Member 4 [Homo sapiens (human)]. NCBI (2017). Available online at: https://www.ncbi.nlm.nih.gov/gene?Db=gene&Cmd=DetailsSearch&Term=6532 (accessed February 2020).
97. Binder EB, Bradley RG, Liu W, Epstein MP, Deveau TC, Mercer KB, et al. Association of FKBP5 polymorphisms and childhood abuse with risk of posttraumatic stress disorder symptoms in adults. JAMA. (2008) 299:1291–305. doi: 10.1001/jama.299.11.1291
98. Yehuda R, Cai G, Golier JA, Sarapas C, Galea S, Ising M, et al. Gene expression patterns associated with posttraumatic stress disorder following exposure to the World Trade Center attacks. Biol Psychiatry. (2009) 66:708–11. doi: 10.1016/j.biopsych.2009.02.034
99. Kim JK, Samaranayake M, Pradhan S. Epigenetic mechanisms in mammals. Cell Mol Life Sci. (2009) 66:596–612. doi: 10.1007/s00018-008-8432-4
100. Uddin M, Koenen KC, Aiello AE, Wildman DE, De Los Santos R, Galea S. Epigenetic and inflammatory marker profiles associated with depression in a community-based epidemiologic sample. Psychol Med. (2011) 41:997–1007. doi: 10.1017/S0033291710001674
101. Bjornsson HT, Sigurdsson MI, Fallin MD, Irizarry RA, Aspelund T, Cui H, et al. Intra-individual change over time in DNA methylation with familial clustering. JAMA. (2008) 299:2877–83. doi: 10.1001/jama.299.24.2877
102. Kang HJ, Adams DH, Simen A, Simen BB, Rajkowska G, Stockmeier CA, et al. Gene expression profiling in postmortem prefrontal cortex of major depressive disorder. J Neurosci. (2007) 27:13329–40. doi: 10.1523/JNEUROSCI.4083-07.2007
103. Petronis A. Epigenetics as a unifying principle in the aetiology of complex traits and diseases. Nature. (2010) 465:721–7. doi: 10.1038/nature09230
104. Bick J, Naumova O, Hunter S, Barbot B, Lee M, Luthar SS, et al. Childhood adversity and DNA methylation of genes involved in the hypothalamus-pituitary-adrenal axis and immune system: whole-genome and candidate-gene associations. Dev Psychopathol. (2012) 24:1417–25. doi: 10.1017/S0954579412000806
105. Mehta D, Klengel T, Conneely KN, Smith AK, Altmann A, Pace TW, et al. Childhood maltreatment is associated with distinct genomic and epigenetic profiles in posttraumatic stress disorder. Proc Natl Acad Sci USA. (2013) 110:8302–7. doi: 10.1073/pnas.1217750110
106. Kananen L, Surakka I, Pirkola S, Suvisaari J, Lönnqvist J, Peltonen L, et al. Childhood adversities are associated with shorter telomere length at adult age both in individuals with an anxiety disorder and controls. PLoS ONE. (2010) 5:e10826. doi: 10.1371/journal.pone.0010826
107. Kiecolt-Glaser JK, Gouin JP, Weng NP, Malarkey WB, Beversdorf DQ, Glaser R. Childhood adversity heightens the impact of later-life caregiving stress on telomere length and inflammation. Psychosom Med. (2011) 73:16–22. doi: 10.1097/PSY.0b013e31820573b6
108. Duman EA, Canli T. Influence of life stress, 5-HTTLPR genotype, and SLC6A4 methylation on gene expression and stress response in healthy Caucasian males. Biol Mood Anxiety Disord. (2015) 5:2. doi: 10.1186/s13587-015-0017-x
109. Klengel T, Mehta D, Anacker C, Rex-Haffner M, Pruessner JC, Pariante CM, et al. Allele-specific FKBP5 DNA demethylation mediates gene-childhood trauma interactions. Nat Neurosci. (2013) 16:33–41. doi: 10.1038/nn.3275
110. Susman EJ. Psychobiology of persistent antisocial behavior: stress, early vulnerabilities and the attenuation hypothesis. Neurosci Biobehav Rev. (2006) 30:376–89. doi: 10.1016/j.neubiorev.2005.08.002
111. Lyons DM, Parker KJ. Stress inoculation-induced indications of resilience in monkeys. J Trauma Stress. (2007) 20:423–33. doi: 10.1002/jts.20265
112. Anderson V, Spencer-Smith M, Leventer R, Coleman L, Anderson P, Williams J, et al. Childhood brain insult: can age at insult help us predict outcome? Brain. (2009) 132:45–56. doi: 10.1093/brain/awn293
113. Gee DG, Casey BJ. The impact of developmental timing for stress and recovery. Neurobiol Stress. (2015) 1:184–94. doi: 10.1016/j.ynstr.2015.02.001
114. Copeland WE, Wolke D, Angold A, Costello EJ. Adult psychiatric outcomes of bullying and being bullied by peers in childhood and adolescence. JAMA Psychiatry. (2013) 70:419–26. doi: 10.1001/jamapsychiatry.2013.504
115. Danese A, Caspi A, Williams B, Ambler A, Sugden K, Mika J, et al. Biological embedding of stress through inflammation processes in childhood. Mol Psychiatry. (2011) 16:244–6. doi: 10.1038/mp.2010.5
116. O'connor MF, Bower JE, Cho HJ, Creswell JD, Dimitrov S, Hamby ME, et al. To assess, to control, to exclude: effects of biobehavioral factors on circulating inflammatory markers. Brain Behav Immun. (2009) 23:887–97. doi: 10.1016/j.bbi.2009.04.005
117. Shariff S, Hoff DL. Cyber bullying: clarifying legalboundaries for school supervision in cyberspace. Int J Cyber Criminol. (2007) 76–118. doi: 10.5281/zenodo.18279
118. Baumeister D, Russell A, Pariante CM, Mondelli V. Inflammatory biomarker profiles of mental disorders and their relation to clinical, social and lifestyle factors. Soc Psychiatry Psychiatr Epidemiol. (2014) 49:841–9. doi: 10.1007/s00127-014-0887-z
119. Aas M, Dieset I, Hope S, Hoseth E, Mørch R, Reponen E, et al. Childhood maltreatment severity is associated with elevated C-reactive protein and body mass index in adults with schizophrenia and bipolar diagnoses. Brain Behav Immun. (2017) 65:342–9. doi: 10.1016/j.bbi.2017.06.005
120. Rogosch FA, Dackis MN, Cicchetti D. Child maltreatment and allostatic load: consequences for physical and mental health in children from low-income families. Dev Psychopathol. (2011) 23:1107–24. doi: 10.1017/S0954579411000587
121. Russell AE, Heron J, Gunnell D, Ford T, Hemani G, Joinson C, et al. Pathways between early-life adversity and adolescent self-harm: the mediating role of inflammation in the avon longitudinal study of parents and children. J Child Psychol Psychiatry. (2019) 60:1094–103. doi: 10.1111/jcpp.13100
122. Bellis MA, Hughes K, Ford K, Ramos Rodriguez G, Sethi D, Passmore J. Life course health consequences and associated annual costs of adverse childhood experiences across Europe and North America: a systematic review and meta-analysis. Lancet Public Health. (2019) 4:e517–28. doi: 10.1016/S2468-2667(19)30145-8
Keywords: biomarkers, biology of social adversity, ACES, review—systematic, adverse childhood events
Citation: Soares S, Rocha V, Kelly-Irving M, Stringhini S and Fraga S (2021) Adverse Childhood Events and Health Biomarkers: A Systematic Review. Front. Public Health 9:649825. doi: 10.3389/fpubh.2021.649825
Received: 05 January 2021; Accepted: 28 July 2021;
Published: 19 August 2021.
Edited by:
Morenike Oluwatoyin Folayan, Obafemi Awolowo University, NigeriaReviewed by:
Susan Elizabeth Esposito, Life University, United StatesNishant Goyal, Central Institute of Psychiatry, India
Copyright © 2021 Soares, Rocha, Kelly-Irving, Stringhini and Fraga. This is an open-access article distributed under the terms of the Creative Commons Attribution License (CC BY). The use, distribution or reproduction in other forums is permitted, provided the original author(s) and the copyright owner(s) are credited and that the original publication in this journal is cited, in accordance with accepted academic practice. No use, distribution or reproduction is permitted which does not comply with these terms.
*Correspondence: Sílvia Fraga, c2lsdmlhLmZyYWdhJiN4MDAwNDA7aXNwdXAudXAucHQ=