- 1State Key Laboratory of Infectious Disease Prevention and Control, Chinese Center for Disease Control and Prevention, National Institute for Communicable Disease Control and Prevention, Beijing, China
- 2Sierra Leone-China Friendship Biosafety Laboratory, Freetown, Sierra Leone
- 3Chinese Center for Disease Control and Prevention, National Institute of Parasitic Diseases, Shanghai, China
- 4Ministry of Health and Sanitation of Sierra Leone, Freetown, Sierra Leone
Background: Malaria is endemic in Sierra Leone, with stable and perennial transmission in all parts of the country. At present, the main prevention and control measures for mosquito vectors here involve insecticide treated nets (ITN) and indoor residual spraying (IRS). The most recent entomological surveillance was conducted prior to the civil war, between 1990 and 1994. Therefore, a new entomological surveillance required to support targeted malaria control strategies.
Methods: Anopheles mosquitoes were collected between June and December 2019 using the light trap method. On these, we conducted species identification, analyzed seasonal fluctuation and Plasmodium infection rate, and monitored insecticide resistance.
Results: Surveillance of seasonal fluctuation showed that there were two peak of Anopheles density in July (mean 13.67 mosquitoes/trap/night) and October (mean 13.00 mosquitoes/trap/night). Meanwhile, the lowest Anopheles density was seen in early September. Ninety-one representatives of Anopheles gambiae s.l. were selected and identified as An. coluzzii (n = 35) and An. gambiae s.s. (n = 56) using PCR. An. coluzzii and An. gambiae s.s. were found to be heterozygous resistant to the knockdown resistance (kdr) L1014F mutation (100%). Meanwhile, the East African mutation (kdr L1014S) was absent in the tested mosquitoes. Three mosquitoes that tested positive for the parasite, had an individual Plasmodium falciparum infection rate of 12.50, 16.67, and 14.29%. The sampling dates of positive mosquitoes were distributed in the two periods of peak Anopheles mosquito density.
Conclusion: This study identified the dominant Anopheles species in Freetown as An. gambiae while the predominant species within the An. gambiae complex was An. gambiae sensu stricto. Surveillance of seasonal fluctuations and high P. falciparum infection rates in Anopheles indicate that the alternation of drought and rainy seasons from June to July, and from October to November, are the key periods for malaria control and prevention in Freetown, Sierra Leone. The high frequency of kdr allele mutations in An. gambiae calls for close monitoring of vector susceptibility to insecticides and tracing of resistance mechanisms in order to develop more effective vector control measures and strategies.
Introduction
Malaria remains a global public health crisis (1). According to the world malaria report 2019, an estimated 228 million cases of malaria occurred worldwide in 2018. Moreover, the African Region of the World Health Organization (WHO), which still bears the largest burden of malaria morbidity, reported 213 million cases (93%) in 2018. Globally, 272 000 (67%) malaria deaths were estimated to occur in children aged <5 years. Almost 85% of all deaths in 2018 occurred in 20 countries in the WHO African Region and India, including Sierra Leone (2). Malaria, an endemic disease in Sierra Leone, has spread steadily throughout the country. Studies have reported high parasite prevalence (3) and high mortality (4) in children <5 years of age in Sierra Leone. Although, pregnant women and children <5 are the most affected, the entire population is at risk and under the burden of malaria. Indeed, malaria accounts for 40.3% of outpatient morbidity at all ages in this country (5).
Malaria is a mosquito-borne infectious disease caused by parasitic protozoa of the genus Plasmodium, transmitted by female Anopheles mosquitoes (6). Despite the heavy burden of malaria, the control of vector-borne diseases in Sierra Leone focuses mainly on case management, while limited effort is made to reduce and interrupt transmission. Mosquito vector control is one of the most effective methods for reducing malaria transmission (7, 8). At present, the main prevention and control measures of mosquito vectors in Sierra Leone are insecticide treated nets (ITN) and indoor residual spraying (IRS). The effective implementation of these measures must be based on entomological surveillance. However, the most recent entomological surveillance (9) was conducted before the civil war of 1990–1994. Freetown, the capital of Sierra Leone, is the largest city and an economic, commercial, educational, and cultural center of this country. Accurate reporting of Anopheles seasonal fluctuation and parasitic infection rate in vectors, species identification, and the monitoring of insecticide resistance in Freetown need to be undertaken to support local targeted malaria control strategies.
Anopheles gambiae s.l. is the predominant malaria vector in Sierra Leone. Among the An. gambiae complex, only An. colluzzi (formerly M-form An. gambiae), An. gambiae s.s. (formerly S-form An. gambiae), and An. melas were recorded in Sierra Leone (10). The main insecticides used in long-lasting insecticidal nets (LLINs) are pyrethroids, which are the only WHO-recommended type of insecticides. However, resistance of malaria vectors to pyrethroids is widespread in Africa (11) and resistance to other insecticides has been recorded in many countries (12–16). Pyrethroid insecticides work by targeting voltage-gated sodium channels. Different point mutations were found in the S6 transmembrane segment of the sodium channel gene domain II. In a wide range of insects, mutations can lead to a phenotype called knockdown resistance (kdr) (17–19). In An. gambiae, the kdr L1014F mutation is widely found in West and Central Africa, while the kdr L1014S mutation is more limited to East Africa (20). The transmission of these mutations among An. gambiae individuals will seriously affect malaria vector control strategies that are based on chemical insecticides (21). Plasmodium falciparum is the main parasite in all serious malaria cases and is further involved in more than 90% of uncomplicated malaria cases in Sierra Leone (5). There have been limited new studies (22–24) on the Plasmodium infection rate of the Anopheles mosquito vector, and especially the comparative data on the infection rate of An. coluzzii and An. gambiae s.s. in Freetown.
In this study, the entomological and molecular surveillance of Anopheles mosquitoes were conducted in 2019 from June to December in Freetown, Sierra Leone. Molecular identification and insecticide resistance monitoring were performed, while the seasonal fluctuation and malaria infection rate of Anopheles mosquitoes were determined.
Methods and Materials
Study Areas
The current study was carried out in Freetown, the capital of Sierra Leone. Sierra Leone, located on the west coast of Africa, has a typical tropical climate with a temperature range of 21–32°C and an average temperature of 25°C. There are two main seasons in a year: the rainy season (May to October) and the dry season (November to April). Notably, there is a large amount of rainfall from July to August. The average annual rainfall is 320 cm and the relative humidity ranges from 60 to 90%. Sierra Leone has a wide range of landforms, from coastal swamps, inland swamps, and tropical rainforests, to one of the highest mountains in West Africa (Bintumani Mountain). Secondary palms are the main forms of vegetation, and they are interspersed with many marshes for rice planting. Sierra Leone has a population of about 7.8 million. The capital city, Freetown, with a population of about 1 million, is located on the Atlantic coast of the Freetown peninsula (5). The study was conducted from June to December 2019 at nine surveillance sites in Freetown. According to the geographical characteristics and whether there are local volunteers to support, nine surveillance sites were selected for monitoring, including residential areas, organization (governmental or commercial sites), hospitals, and livestock sheds. The surveillance sites were Hill Station, Sorie Lane, Aberdeen, Congo Cross, New England, Waterloo, Lakka, Lumley, and Locust sites (Table 1); of these, Lakka, Waterloo, and Sorie Lane can be found in the western area rural of Freetown, while the other six sites are in the western area urban of Freetown. The distribution of the surveillance sites is shown in Figure 1.
Mosquito Collection
Mosquitoes were collected using the light-trap method once a week. Approximately 2–4 mosquito traps (MYFS-HJY-1, Dongguan Houji Electronic Technology Co., Ltd.) were set at each surveillance site. These traps were set up 1 h before sunset and the collection nets were collected 1 h after sunrise the following day. Subsequently, the mosquitoes were brought back to the laboratory for morphological identification (25). The mosquitoes were monitored weekly and stored in the laboratory at −40°C for further analysis.
DNA Extraction
Genomic DNA was extracted from individual mosquitoes using a DNA extraction kit (Bioteke, AU19014) and used for PCR analysis to identify sub-species of the An. gambiae complex.
Molecular Form Detection
The molecular identification of An. coustani and An. gambiae mosquitoes, involved polymerase chain reaction (PCR) amplification and sequencing of the cytochrome oxidase subunit 1 (COI) region (26). An. gambiae individuals were identified to species using PCR (27). In performing this analysis, 25 μL of PCR mix containing UN, GA, AR, QD, and ME primers (Table 2); Premix Taq (TAKARA, RR901); water; and DNA extracted from a single mosquito was prepared. The PCR was carried out with an initial step performed at 5 min with a temperature of 94°C to activate the DNA polymerase. This was followed by 35 cycles, each comprising a 30 s denaturation at 94°C, 30 s annealing at 50°C, and 30 s extension at 72°C. The final cycle products were extended for 5 min at 72°C. Only females of An. gambiae (s.l.) were selected for further analysis.
The molecular forms of An. gambiae sensu stricto were identified further using PCR (28). This method allows for the simultaneous identification of An. colluzzi and An. gambiae s.s. The 25 μL PCR mix contained R5, R3, Mopint, and B/Sint primers (Table 2); Premix Taq (TAKARA, RR901); water; and DNA extracted from a single mosquito. The annealing temperature for PCR amplification was 63°C.
kdr Mutation Detection
Real-time polymerase chain reaction (qPCR) was used to detect kdr L1014F or kdr L1014S mutations by TaqMan analysis. (29). The primers kdr-Forward, kdr-Reverse, and the WT probe were all contained in the same reaction system. Meanwhile, the probe kdr W was used to detect kdr L1014F, and the probe kdr E was used to detect kdr L1014S (Table 2). The PCR reaction system (25 μL) contained 2 μL genomic DNA, 12.5 μL qPCR Master Mix (H&R, SJ-2101B), 900 nM of each primer, and 200 nM of each probe. The PCR was carried out using the following temperature cycling conditions: 10 min at 95°C, followed by 40 cycles at 95°C for 10 s and 60°C for 45 s.
Another PCR method was used to detect kdr mutation in some samples (18). Approximately 10 ± 50 ng of genomic DNA prepared as above were combined in a 25 μl total volume with the four primers Agd1, Agd2, Agd3, and Agd4 (Table 2). The PCR reaction conditions were as follows: 1 min at 94°C, 2 min at 48°C, and 2 min at 72°C for 40 cycles with a final extension step at 72°C for 10 min. Amplified fragments were analyzed using electrophoresis on a 1.5% agarose gel and were visualized through ethidium bromide staining under UV light.
Malaria Pathogen Detection
rPLU5 and rPLU6 primers were used for the first round of amplification, and the amplified products were used as templates for the second amplification with four Plasmodium-specific primers. The second PCR with the rFAL1 and rFAL2 primers generated products of 205 bp for P. falciparum, rVIV1 and rVIV2 primers generated a 120 bp product for Plasmodium vivax; rMAL1 and rMAL2 primers generated a 144 bp product for Plasmodium malariae; and rOVA1 and rOVA2 primers generated an 800 bp product for Plasmodium ovale (Table 2) (30).
Statistical Analysis
ArcGIS 10.7 was used to map the Anopheles mosquito surveillance sites in Freetown, Sierra Leone. Meanwhile, the Microsoft Excel 2019 software was used to analyze the monitoring data of Anopheles mosquitoes from the surveillance sites. This included a sum of the total number of Anopheles mosquitoes, and calculation of the distribution ratio and the seasonal variation of mosquito density, as well as a count of the malaria infection rate of Anopheles mosquitoes. SPSS v21.0 software was used for statistical analysis.
Results
Distribution Analysis of Collected Mosquitoes
During the period from June 26 to December 31, 2019, mosquito vector density monitoring was carried out a total of 26 times at 9 surveillance sites, and 3 012 mosquitoes were collected. Among these, 2 556 Culex mosquitoes accounted for 84.86%, 410 Anopheles mosquitoes of malaria vectors accounted for 13.61%, 43 Aedes mosquitoes accounted for 1.43%, and 3 other mosquito species accounted for 0.10% of the total number of mosquitoes captured. The average mosquito density in Freetown was 4.35 mosquitoes/trap/night.
The average density of Anopheles mosquitoes in Freetown was 0.61 mosquitoes/trap/night. The number of Anopheles collected at Lakka surveillance site was 330, accounting for 80.49% of the total number, and the average density of Anopheles was 4.78 mosquitoes/trap/night. In addition, 30 Anopheles were captured at the Waterloo and Locust, accounting for 7.32% of the total number, and the average density of Anopheles were 0.25 and 0.63 mosquitoes/trap/night. In other surveillance sites, the number of Anopheles trapped was lower (details are shown in Table 3). Statistics by region showed that 362 Anopheles were trapped in the western area rural, accounting for 88.29% of the total. The average density was 1.31 mosquitoes/trap/night. A total of 48 Anopheles were trapped in the western area urban, accounting for 11.71% of the total. The average density was 0.12 mosquitoes/trap/night.
Density of Anopheles Mosquitoes in Different Habitats
The results showed that the density of Anopheles was the highest in livestock shed (2.45 mosquitoes/trap/night). The density of Anopheles in residential areas was 0.60 mosquitoes/trap/night, while that in organization and hospitals was 0.11 and 0.03 mosquitoes/trap/night, respectively. The density of Anopheles trapped in different livestock sheds was further compared. The densities of Anopheles in the livestock sheds of Lakka, Waterloo, Lumley, and Sorie Lane were 8.55, 0.69, 0.12, and 0.07 mosquitoes/trap/night, respectively.
Surveillance of Seasonal Fluctuation of Anopheles Density in the Lakka Community
Most of field-collected Anopheles mosquitoes (80.49%) were from Lakka; therefore, this study focused on the Lakka surveillance site as a representative region to conduct further research and analysis. Lakka is in the western area rural of Freetown, Sierra Leone and its environs are rice fields.
The seasonal fluctuation trend of Anopheles density in the Lakka community is shown in Figure 2. During the monitoring period, there were two periods of peak Anopheles density, the first from July 3 to August 7, and the second from October 9 to November 20. The mosquito density was 13.67 mosquitoes/trap/night on July 31, while in August, the density of Anopheles gradually decreased reaching its lowest level in early September. The density of Anopheles mosquitoes then began to rise, reaching a second peak at the end of October, at 13.00 mosquitoes/trap/night. Subsequently, the Anopheles density again began to decrease in December.
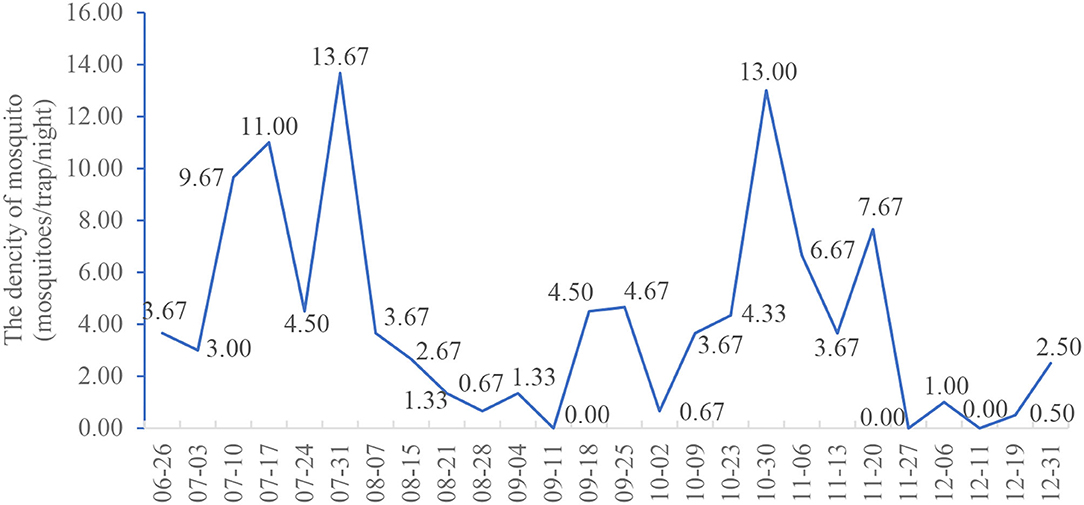
Figure 2. Seasonal variation of the mean density of Anopheles in Lakka, Freetown from June to December 2019.
Molecular Identification of Anopheles Mosquitoes
The mosquitoes were identified morphologically and their identities were then confirmed using the COI sequence. From July 17, 2019 to November 6, 2019, 180 An. gambiae complex (85.31%) and 31 An. coustani (14.69%) mosquitoes were collected. The principal malaria vectors in An. gambiae complex are An. gambiae sensu stricto, An. arabiensis, An. quadriannulatus species A and An. quadriannulatus species B, as well as An. melas, An. merus, and An. bwambae (31). By amplifying the IGS gene, 91 representatives of An. gambiae complex were selected and identified as An. gambiae sensu stricto using PCR. Anopheles gambiae sensu stricto was recently reclassified as two species, An. coluzzii and An. gambiae s.s. Subsequently, both An. coluzzii and An. gambiae s.s. were identified (Table 4). There were 35 An. coluzzii mosquitoes accounting for 38.46% (35/91) and 56 An. gambiae s.s. mosquitoes, accounting for 61.54% (56/91) of the An. gambiae complex identified by molecular method.
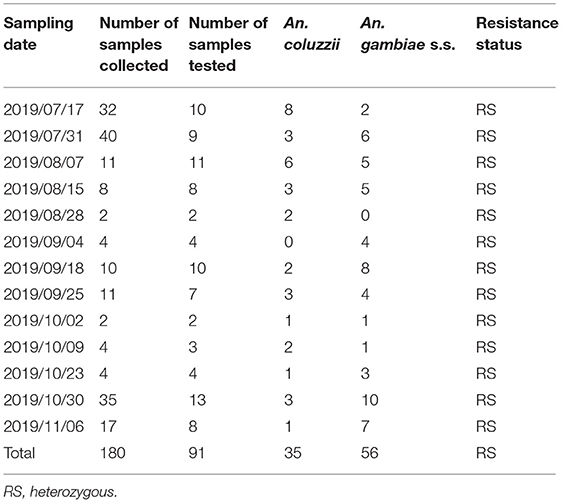
Table 4. Molecular identification of members of the An. gambiae complex collected in the Lakka surveillance site.
Detection of Resistance Genes
All three kdr alleles (kdr 1014L, kdr 1014F, and kdr 1014S) were detected in both An. coluzzii and An. gambiae s.s. In each kdr 1014F mutant assay, an intermediate increase in both VIC fluorescence and FAM fluorescence indicated a heterozygote. In each kdr 1014S mutant assay, a substantial increase in VIC fluorescence, with no increase in FAM fluorescence indicated that there was no kdr 1014S mutant. According to the above results, 35 An. coluzzii and 56 An. gambiae s.s. mosquitoes were all heterozygous resistant to the kdr L1014F mutation (100%) (Table 4). Some samples were confirmed by another test method. Furthermore, the East African mutation (kdr L1014S) was absent in the tested mosquitoes.
Malaria Parasite Infection in Field-Collected Mosquitoes
A total of 91 An. gambiae complex and 31 An. coustani were further tested for the presence of malarial parasites. While P. falciparum parasites were detected in three mosquitoes, Plasmodium vivax, Plasmodium malariae, and Plasmodium ovale parasites were not found. Overall, the An. gambiae complex had an individual P. falciparum infection rate of 3.30%, while no P. falciparum infection was detected in the An. coustani individuals (Table 5). Individually, the An. coluzzii and An. gambiae s.s. mosquitoes had P. falciparum infection rates of 5.71 and 1.79%, respectively. An. coluzzii was found to have a higher individual P. falciparum infection rate than An. gambiae s.s., but the difference was not statistically significant (P > 0.05). Meanwhile, the three P. falciparum parasite-positive mosquitoes had an individual P. falciparum infection rate of 12.50% (July 17, 2019), 16.67% (August 7, 2019), and 14.29% (November 6, 2019), respectively. The sampling dates of positive mosquitoes were distributed in the two periods of peak Anopheles density (from July 3 to August 7 and from October 9 to November 20).
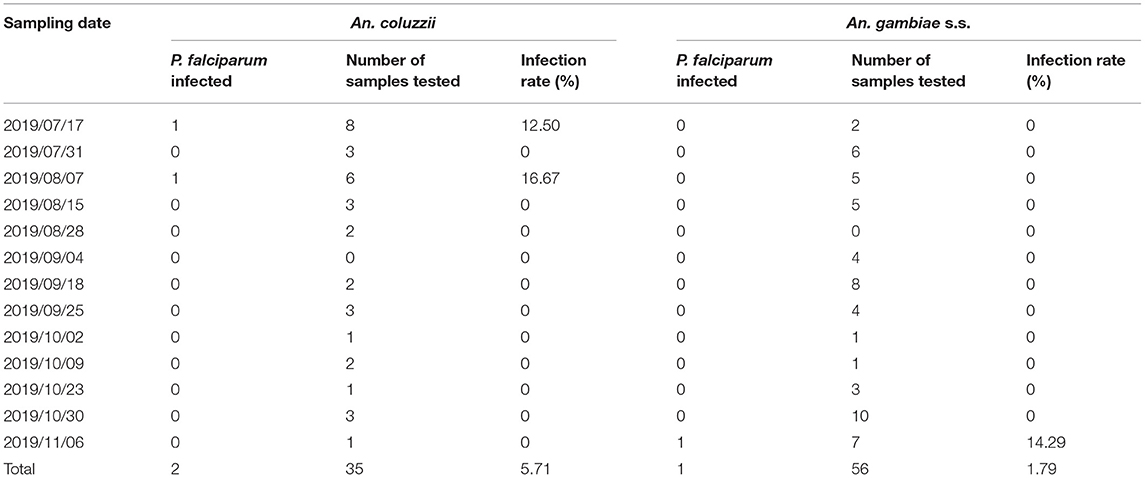
Table 5. Plasmodium falciparum infection of An. gambiae complex individuals collected in Lakka from June to December 2019.
Discussion
The monitoring results showed that Culex accounted for the highest proportion of the total number of mosquitoes, which was the dominant species in Freetown, followed by Anopheles, and Aedes, which had the lowest density. However, Aedes was mainly active in the daytime, and the mosquito traps were used at night, so the data of Aedes could not represent the real situation of the region. In this study, 6 surveillance sites were in the western area urban, while only 3 surveillance sites were in the western area rural. However, the number of Anopheles trapped in western areas rural accounted for 88.29%, and the average density of Anopheles trapped was much higher than that in western urban areas. It may be that there are many paddy fields and swamps in the western area rural, which are breeding areas of Anopheles. These results suggest that the risk of malaria transmitting in western areas rural is higher than that in western areas urban in Freetown.
Two periods of peak Anopheles density appeared during our study, one in July and the other in October. In Sierra Leone, rainfall typically increases after May, which is the main reason for the increase in mosquito density. Consequently, the Anopheles density was high at the end of June and the beginning of July in the alternate period of the drought and rainy seasons. Heavy rains occurred in July and August, and the mosquito density gradually decreased from the end of July, reaching its lowest point in late August. Previous studies have shown that it takes at least seven days for mosquitoes to develop from eggs to adults in stable water and suitable temperature conditions (32). The lower adult mosquito density may be attributed to rainfall washing away mosquito eggs and larvae from breeding sites during periods of heavy rain. In other studies, it has been reported that large amounts of rain can affect mosquito reproduction (33). In addition, it was easy to form a stable water body for mosquito breeding in September, when the rainfall decreased. The increase of breeding places will lead to the increase of mosquito density (34, 35). Therefore, mosquito density began to rebound and reached its peak in October. Similarly, the observed decrease in the density of Anopheles may be due to a decrease in breeding sites in the dry season. Meanwhile, three P. falciparum parasite-positive mosquitoes were collected on July 17, August 7, and November 6, 2019, respectively, which was consistent with the peak period density of Anopheles. These results explain the two peaks of malaria transmission in Sierra Leone; one that begins during the rainy season in May and the second that starts toward the end of the season in October/November (36). These results suggest that the alternation of drought and rainy seasons from June to July and from October to November are the key periods for malaria control and prevention in Sierra Leone.
At the peak of the malaria epidemic, the P. falciparum infection rate of An. gambiae reached as high as 16.67%, which is similar to the infection rate in other malaria endemic areas (37–39). Three Plasmodium-infected Anopheles mosquitoes were collected during the two periods of peak Anopheles density and also in the malaria epidemic season. These results suggest that the high infection rate of Anopheles is closely related to the prevalence of malaria. It is very important to reduce the plasmodium infection rate of Anopheles for malaria control, and, therefore, case management should be strengthened. First, access to effective and timely diagnosis and treatment of malaria is a key intervention in malaria control efforts (5). However, it is very important to ensure that the patient is not bitten by mosquitoes during the illness. As a preventative measure, patients should wear long clothes and long sleeves and remove mosquito breeding places in their living environment. Finally, doctors need to strengthen publicity and education efforts for patients to enhance their public health awareness.
Historically, pyrethrum spraying began in Western Freetown to control adult mosquitoes in 1940. In 1946, the IRS was introduced in Freetown and Port Loko. The use of ITN began in 2002, mainly for pregnant women and children under 5 years old. In 2006, the nationwide free large-scale distribution of LLIN for children under 1-year-old and a measles vaccine campaign were carried out. In 2010, 2014, and 2017, the large-scale distribution of LLIN continued (5). A study in 2013 showed that high proportions of kdr mutations (96.2%) were detected in the An. gambiae populations in Freetown and the majority mosquitoes were RR homozygotes for kdr 1014F mutation, with very few heterozygotes (40). However, in this study, An. coluzzii and An. gambiae s.s. were all heterozygous resistant to the kdr L1014F mutation (100%). This phenomenon cannot be well-explained at present. It may be related to the pressure of Lakka environmental selection, or because of the limited sample size. It is worth further study in the future. This rapid decrease in susceptibility across sentinel sites may be due to the scale-up of LLINs in the country (41). Although, it has been reported that the extensive use of pyrethroids in agriculture contributes to the emergence of resistance in some parts of Africa, the large-scale increase in LLIN and IRS to control malaria is the main reason for the growing problem of resistance (42). Therefore, it is recommended that non-pyrethroid insecticides be used for IRS and LLIN to prevent and control malaria transmission, which is also suggested by WHO (43, 44). The high frequency of kdr allele mutation in An. gambiae calls for close monitoring of vector susceptibility to insecticides and tracing of resistance mechanisms in order to develop more effective vector control strategies and measures.
Data Availability Statement
The raw data supporting the conclusions of this article will be made available by the authors, without undue reservation.
Ethics Statement
All procedures were performed in accordance with established International Guiding Principles. The study was approved by the National Malaria Control Programme, Ministry of Health and Sanitation. Permission to collect mosquito from the fields was obtained from the field owners. Our study did not pose any danger to the communities involved or the staff that participated.
Author Contributions
NZ, IS, HT, and FY made substantial contribution to the design of the research. NZ, IS, and HT conducted field work for mosquito collection. NZ, FY, LL, and YG had analyzed the data of mosquito surveillance. NZ, LL, XS, JW, XL, YY, and HW tested mosquitoes collected from surveillance sites. NZ, IS, LL, and FY contributed to the drafting of the manuscript and the important intellectual content herewith. QL supervised the work as the project administrator. All authors have read and agreed to the published version of the manuscript.
Funding
This work was financially supported by the pilot project on building capacity of malaria molecular lab epidemiology network in Sierra Leone, Grant No. INV-003421, and by the visual identification of important invasive vectors and pathogens and their invasion risk, Grant No. 2020YFC1200101.
Conflict of Interest
The authors declare that the research was conducted in the absence of any commercial or financial relationships that could be construed as a potential conflict of interest.
Acknowledgments
The authors wish to thank the residents living around the nine surveillance sites for supporting their work and providing assistance with field mosquitoes collection. The authors also wish to thank the National Malaria Control Program of Ministry of Health and Sanitation for the cooperation and support.
References
1. Cibulskis RE, Alonso P, Aponte J, Aregawi M, Barrette A, Bergeron L, et al. Malaria: global progress 2000 - 2015 and future challenges. Infect Dis Poverty. (2016) 5:61. doi: 10.1186/s40249-016-0151-8
3. Sierra Leone Malaria Indicator Survey 2013. National Malaria Control Programme (NMCP) [Sierra Leone] Statistics Sierra Leone University of Sierra Leone Catholic Relief Services and ICF International. Freetown: NMCP, SSL, CRS, and ICF International (2013).
4. Sierra Leone demographic and Health Survey 2013. Statistics Sierra Leone (SSL) and ICF International. Freetown: SSL and ICF International (2014).
5. Sierra Leone Malaria Control Strategic Plan 2016-2020. Freetown: Sierra Leone Ministry of Health and Sanitation. (2015). p. 23–41.
6. Wiebe A, Longbottom J, Gleave K, Shearer FM, Sinka ME, Massey NC, et al. Geographical distributions of African malaria vector sibling species and evidence for insecticide resistance. Malar J. (2017) 16:85. doi: 10.1186/s12936-017-1734-y
7. Brochero H, Quinones ML. Challenges of the medical entomology for the surveillance in public health in Colombia: reflections on the state of malaria. Biomedica. (2008) 28:18–24. doi: 10.7705/biomedica.v28i1.105
8. Ulrich JN, Naranjo DP, Alimi TO, Muller GC, Beier JC. How much vector control is needed to achieve malaria elimination? Trends Parasitol. (2013) 29:104–9. doi: 10.1016/j.pt.2013.01.002
9. Bockarie MJ, Gbakima AA, Barnish G. It all began with Ronald Ross: 100 years of malaria research and control in Sierra Leone (1899-1999). Ann Trop Med Parasitol. (1999) 93:213–24. doi: 10.1080/00034983.1999.11813416
10. Tredre RF. The role of Anopheles gambiae var. melas in the transmission of malaria in the vicinity of Freetown Estuary, Sierra Leone, 1943. Ann Trop Med Parasitol. (1946) 40:380–420. doi: 10.1080/00034983.1946.11685297
11. Ranson H, N'Guessan R, Lines J, Moiroux N, Nkuni Z, Corbel V. Pyrethroid resistance in African anopheline mosquitoes: what are the implications for malaria control? Trends Parasitol. (2011) 27:91–8. doi: 10.1016/j.pt.2010.08.004
12. Koffi AA, Ahoua Alou LP, Adja MA, Chandre F, Pennetier C. Insecticide resistance status of Anopheles gambiae s.s population from M'Be: a WHOPES-labelled experimental hut station, 10 years after the political crisis in Cote d'Ivoire. Malar J. (2013) 12:151. doi: 10.1186/1475-2875-12-151
13. Dabire KR, Diabate A, Djogbenou L, Ouari A, N'Guessan R, Ouedraogo JB, et al. Dynamics of multiple insecticide resistance in the malaria vector Anopheles gambiae in a rice growing area in South-Western Burkina Faso. Malar J. (2008) 7:188. doi: 10.1186/1475-2875-7-188
14. Hargreaves K, Hunt RH, Brooke BD, Mthembu J, Weeto MM, Awolola TS, et al. Anopheles arabiensis and An. quadriannulatus resistance to DDT in South Africa. Med Vet Entomol. (2003) 17:417–22. doi: 10.1111/j.1365-2915.2003.00460.x
15. Djogbenou L, Dabire R, Diabate A, Kengne P, Akogbeto M, Hougard JM, et al. Identification and geographic distribution of the ACE-1R mutation in the malaria vector Anopheles gambiae in south-western Burkina Faso, West Africa. Am J Trop Med Hyg. (2008) 78:298–302. doi: 10.4269/ajtmh.2008.78.298
16. Knox TB, Juma EO, Ochomo EO, Pates Jamet H, Ndungo L, Chege P, et al. An online tool for mapping insecticide resistance in major Anopheles vectors of human malaria parasites and review of resistance status for the Afrotropical region. Parasit Vectors. (2014) 7:76. doi: 10.1186/1756-3305-7-76
17. Williamson MS, Martinez-Torres D, Hick CA, Devonshire AL. Identification of mutations in the housefly para-type sodium channel gene associated with knockdown resistance (kdr) to pyrethroid insecticides. Mol Gen Genet. (1996) 252:51–60. doi: 10.1007/s004389670006
18. Martinez-Torres D, Chandre F, Williamson MS, Darriet F, Berge JB, Devonshire AL, et al. Molecular characterization of pyrethroid knockdown resistance (kdr) in the major malaria vector Anopheles gambiae s.s. Insect Mol Biol. (1998) 7:179–84. doi: 10.1046/j.1365-2583.1998.72062.x
19. Ranson H, Jensen B, Vulule JM, Wang X, Hemingway J, Collins FH. Identification of a point mutation in the voltage-gated sodium channel gene of Kenyan Anopheles gambiae associated with resistance to DDT and pyrethroids. Insect Mol Biol. (2000) 9:491–7. doi: 10.1046/j.1365-2583.2000.00209.x
20. Santolamazza F, Calzetta M, Etang J, Barrese E, Dia I, Caccone A, et al. Distribution of knock-down resistance mutations in Anopheles gambiae molecular forms in west and west-central Africa. Malar J. (2008) 7:74. doi: 10.1186/1475-2875-7-74
21. Kelly-Hope L, Ranson H, Hemingway J. Lessons from the past: managing insecticide resistance in malaria control and eradication programmes. Lancet Infect Dis. (2008) 8:387–9. doi: 10.1016/S1473-3099(08)70045-8
22. Bockarie MJ, Service MW, Barnish G, Maude GH, Greenwood BM. Malaria in a rural area of Sierra Leone. III. Vector ecology and disease transmission. Ann Trop Med Parasitol. (1994) 88:251–62. doi: 10.1080/00034983.1994.11812865
23. Bockarie MJ, Service MW, Barnish G, Toure YT. Vectorial capacity and entomological inoculation rates of Anopheles gambiae in a high rainfall forested area of southern Sierra Leone. Trop Med Parasitol. (1995) 46:164–71.
24. Bockarie MJ, Alexander N, Bockarie F, Ibam E, Barnish G, Alpers M. The late biting habit of parous Anopheles mosquitoes and pre-bedtime exposure of humans to infective female mosquitoes. Trans R Soc Trop Med Hyg. (1996) 90:23–5. doi: 10.1016/S0035-9203(96)90465-4
25. Leeson HS. Keys to the known larvae and adults of West African Anopheline mosquitoes. Bull Entomol Res. (1939) 30:131–41. doi: 10.1017/S0007485300004442
26. Folmer O, Black M, Hoeh W, Lutz R, Vrijenhoek R. DNA primers for amplification of mitochondrial cytochrome c oxidase subunit I from diverse metazoan invertebrates. Mol Mar Biol Biotechnol. (1994) 3:294–9.
27. Scott JA, Brogdon WG, Collins FH. Identification of single specimens of the Anopheles gambiae complex by the polymerase chain reaction. Am J Trop Med Hyg. (1993) 49:520–9. doi: 10.4269/ajtmh.1993.49.520
28. Favia G, Lanfrancotti A, Spanos L, Siden-Kiamos I, Louis C. Molecular characterization of ribosomal DNA polymorphisms discriminating among chromosomal forms of Anopheles gambiae s.s. Insect Mol Biol. (2001) 10:19–23. doi: 10.1046/j.1365-2583.2001.00236.x
29. Bass C, Nikou D, Donnelly MJ, Williamson MS, Ranson H, Ball A, et al. Detection of knockdown resistance (kdr) mutations in Anopheles gambiae: a comparison of two new high-throughput assays with existing methods. Malar J. (2007) 6:111. doi: 10.1186/1475-2875-6-111
30. Snounou G, Viriyakosol S, Zhu XP, Jarra W, Pinheiro L, do Rosario VE, et al. High sensitivity of detection of human malaria parasites by the use of nested polymerase chain reaction. Mol Biochem Parasitol. (1993) 61:315–20. doi: 10.1016/0166-6851(93)90077-B
31. Bass C, Williamson MS, Wilding CS, Donnelly MJ, Field LM. Identification of the main malaria vectors in the Anopheles gambiae species complex using a TaqMan real-time PCR assay. Malar J. (2007) 6:155. doi: 10.1186/1475-2875-6-155
33. Loaiza JR, Miller MJ. Seasonal pattern of avian Plasmodium-infected mosquitoes and implications for parasite transmission in central Panama. Parasitol Res. (2013) 112:3743–51. doi: 10.1007/s00436-013-3562-5
34. Matthys B, Vounatsou P, Raso G, Tschannen AB, Becket EG, Gosoniu L, et al. Urban farming and malaria risk factors in a medium-sized town in Cote d'Ivoire. Am J Trop Med Hyg. (2006) 75:1223–31. doi: 10.4269/ajtmh.2006.75.1223
35. Premasiri DA, Wickremasinghe AR, Premasiri DS, Karunaweera N. Malarial vectors in an irrigated rice cultivation area in southern Sri Lanka. Trans R Soc Trop Med Hyg. (2005) 99:106–14. doi: 10.1016/j.trstmh.2004.02.009
36. Malaria operational plan FY 2018 and FY 2019 Sierra Leone. Freetown: President's Malaria Initiative. (2017).
37. Ogola E, Villinger J, Mabuka D, Omondi D, Orindi B, Mutunga J, et al. Composition of Anopheles mosquitoes, their blood-meal hosts, and Plasmodium falciparum infection rates in three islands with disparate bed net coverage in Lake Victoria, Kenya. Malar J. (2017) 16:360. doi: 10.1186/s12936-017-2030-6
38. Sanford MR, Cornel AJ, Nieman CC, Dinis J, Marsden CD, Weakley AM, et al. Plasmodium falciparum infection rates for some Anopheles spp. from Guinea-Bissau, West Africa. F1000Res. (2014) 3:243. doi: 10.12688/f1000research.5485.2
39. Boudin C, Robert V, Verhave JP, Carnevale P, Ambroise-Thomas P. Plasmodium falciparum and P. malariae epidemiology in a West African village. Bull World Health Organ. (1991) 69:199–205.
40. de Souza DK, Koudou BG, Bolay FK, Boakye DA, Bockarie MJ. Filling the gap 115 years after Ronald Ross: the distribution of the Anopheles coluzzii and Anopheles gambiae s.s from Freetown and Monrovia, West Africa. PLoS ONE. (2013) 8:e64939. doi: 10.1371/journal.pone.0064939
41. N'Guessan R, Corbel V, Akogbeto M, Rowland M. Reduced efficacy of insecticide-treated nets and indoor residual spraying for malaria control in pyrethroid resistance area, Benin. Emerg Infect Dis. (2007) 13:199–206. doi: 10.3201/eid1302.060631
42. Hemingway J. The role of vector control in stopping the transmission of malaria: threats and opportunities. Philos Trans R Soc Lond B Biol Sci. (2014) 369:20130431. doi: 10.1098/rstb.2013.0431
43. World Health Organization. Global Plan for Insecticide Resistance Management in Malaria Vectors. Geneva: WHO Press (2012). p. 44–5.
Keywords: Anopheles gambiae, seasonal fluctuation, plasmodium infection rate, kdr, Sierra Leone
Citation: Zhao N, Sesay I, Tu H, Yamba F, Lu L, Guo Y, Song X, Wang J, Liu X, Yue Y, Wu H and Liu Q (2021) Entomological and Molecular Surveillance of Anopheles Mosquitoes in Freetown, Sierra Leone, 2019. Front. Public Health 9:649672. doi: 10.3389/fpubh.2021.649672
Received: 05 January 2021; Accepted: 26 May 2021;
Published: 17 June 2021.
Edited by:
Leonard E. G. Mboera, Sokoine University of Agriculture, TanzaniaReviewed by:
Luc Salako Djogbénou, Université d'Abomey-Calavi, BeninFlorence Fournet, Institut de Recherche pour le Développement, Côte d'Ivoire
Copyright © 2021 Zhao, Sesay, Tu, Yamba, Lu, Guo, Song, Wang, Liu, Yue, Wu and Liu. This is an open-access article distributed under the terms of the Creative Commons Attribution License (CC BY). The use, distribution or reproduction in other forums is permitted, provided the original author(s) and the copyright owner(s) are credited and that the original publication in this journal is cited, in accordance with accepted academic practice. No use, distribution or reproduction is permitted which does not comply with these terms.
*Correspondence: Qiyong Liu, bGl1cWl5b25nQGljZGMuY24=