- 1Department of Agricultural Sciences, University of Bologna, Bologna, Italy
- 2Cesare Maltoni Cancer Research Center (CMCRC), Ramazzini Institute (RI), Bologna, Italy
- 3Radboud Institute for Health Sciences, Radboud University Medical Center (UMC), Nijmegen, Netherlands
- 4Bloomberg School of Public Health, Johns Hopkins University, Baltimore, MD, United States
Introduction: Glyphosate, an amino acid analog of glycine, is the most widely applied organophosphate pesticide worldwide and it is an active ingredient of all glyphosate-based herbicides (GBHs), including the formulation “Roundup. ” While glycine is an essential amino acid generally recognized safe, both epidemiological and toxicological in vivo and in vitro studies available in literature report conflicting findings on the toxicity of GBHs. In our earlier in vivo studies in Sprague–Dawley rats we observed that exposure to GBHs at doses of glyphosate of 1.75 mg/kg bw/day, induced different toxic effects relating to sexual development, endocrine system, and the alteration of the intestinal microbiome. In the present work, we aimed to comparatively test in in vitro models the cytotoxicity of glycine and GBHs.
Methods: We tested the cytotoxic effects of glycine, glyphosate, and its formulation Roundup Bioflow at different doses using MTT and Trypan Blue assays in human Caco2 and murine L929 cell lines.
Results: Statistically significant dose-related cytotoxic effects were observed in MTT and Trypan Blue assays in murine (L929) and human (Caco2) cells treated with glyphosate or Roundup Bioflow. No cytotoxic effects were observed for glycine. In L929, Roundup Bioflow treatment showed a mean IC50 value that was significantly lower than glyphosate in both MTT and Trypan Blue assays. In Caco2, Roundup Bioflow treatment showed a mean IC50 value that was significantly lower than glyphosate in the MTT assays, while a comparable IC50 was observed for glyphosate and Roundup Bioflow in Trypan Blue assays. IC50 for glycine could not be estimated because of the lack of cytotoxic effects of the substance.
Conclusion: Glyphosate and its formulation Roundup Bioflow, but not glycine, caused dose-related cytotoxic effects in in vitro human and murine models (Caco2 and L929). Our results showed that glycine and its analog glyphosate presented different cytotoxicity profiles. Glyphosate and Roundup Bioflow demonstrate cytotoxicity similar to other organophosphate pesticides (malathion, diazinon, and chlorpyriphos).
Introduction
Glyphosate [IUPAC chemical name N-(phosphonomethyl)-glycine], an amino acid analog of glycine, is the most widely applied organophosphate pesticide worldwide and it is an active ingredient of all glyphosate-based herbicides (GBHs), including in the formulation “Roundup” (1, 2). It is mainly marketed as a broad-spectrum systemic herbicide and crop desiccant (3). Glyphosate was in fact synthesized in 1950 by a Swiss chemist, Henri Martin, as an analog of the non-essential amino acid glycine, but its herbicidal properties were not discovered for another 20 years (4). The massive and increasing use of GBHs leads to a global burden of occupational exposures in manufacturing workers and GBH applicators (farmers), as well as increasing exposures in the general population, as demonstrated by environmental contamination from glyphosate residues found in air (5), groundwater (6, 7), drinking-water (8), crops (9, 10), food (11, 12), and animal feed (13). In humans, the main exposure routes to glyphosate are inhalation and dermal exposure in the occupational setting and for the general population consumption of contaminated drinking water and residues in food items (14).
The results of oral studies with [14C] glyphosate in rats, rabbits and goats indicate that absorption from the gastrointestinal tract is incomplete and amounts to up to 30% of the dose (15–17). The most relevant routes of excretion following oral administration of glyphosate [14C] are feces (70–80%) and urine (20–30%) (18). Therefore, most of the glyphosate assumed orally is not absorbed in the gastro-intestinal tract and is then excreted with the feces. On the other hand, glycine is very rapidly absorbed along the gastrointestinal tract via special carrier systems and then transported via the portal vein into the liver but also distributed within the whole body since it is involved in the body's production of haem, DNA, phospholipids, and collagen (19).
In March 2015, the World Health Organization's International Agency for Research on Cancer (IARC) classified three organophosphates (glyphosate, malathion, and diazinon) as “probably carcinogenic for humans” (Category 2A) (20). In contrast, in November 2015 the European Food Safety Agency determined glyphosate was “unlikely to pose a cancer risk for man” (EFSA 2015). In 2018 the European Chemicals Agency (21) Risk Assessment Committee concluded that “the scientific evidence so far available does not satisfy the criteria for classifying glyphosate as carcinogenic, mutagenic or toxic for reproduction” (21). In 2019 a US federal health agency, the Agency for Toxic Substances and Disease Registry (ATSDR) (22), part of the Centers for Disease Control and Prevention (23), determined that both cancer and non-cancer hazards derive from exposure to glyphosate and GBHs. The ATSDR 2019 report clearly lays out the vast array of scientific evidence linking both pure glyphosate (rodent studies) as well as formulations (in human epidemiologic studies) to cancer. In fact glyphosate, as the pure active substance, and its formulations may not have the same toxicity. Glyphosate formulations contain a number of so-called “inert” ingredients or adjuvants to facilitate the uptake by plants, most of which are patented and not publicly known (in many countries the law does not require a full disclosure of pesticide ingredients). GBHs that contain surfactants and adjuvants might act differently than glyphosate alone (24–26). In fact, adjuvants might be toxic in their own right and potentiate the toxic effects of glyphosate, as in the case of polyethoxylated tallow amine (POEA), that have been banned in the EU since 2016 (27–31).
While glycine is an essential amino acid generally recognized safe, both epidemiological and toxicological in vivo and in vitro studies available in literature report conflicting findings on the toxicity of GBHs. In our previous in vivo studies on Sprague–Dawley rats we observed that exposure to GBHs (pure glyphosate and Roundup Bioflow) at doses of glyphosate considered to be “safe,” the US ADI of 1.75 mg/kg bw/day, defined as the chronic Reference Dose (cRfD) determined by the US EPA, induced different toxic effects relating to sexual development, endocrine system, and the alteration of the intestinal microbiome (32–34). Furthermore, mechanistic data are increasingly important for hazard characterization, as exemplified by the 10 key characteristics of carcinogens considered by IARC in their evaluations, which include “Alters cell proliferation, cell death or nutrient supply” (35, 36). In particular, the MTT and Trypan Blue assays are routine and convenient methods for determination of cytotoxicity (37–39). MTT assay is a colorimetric assay of viable cells, while Trypan Blue assay is a dye exclusion staining assay. The combined and comparative use of MTT and Trypan Blue assays allows to overcome the limitations the single assays and increases the precision of the IC50 estimates derived from these studies (40–42). In the present work, we aimed to test the cytotoxic effects of glycine, glyphosate and its formulation Roundup Bioflow at different doses using MTT and Trypan Blue assays in human Caco2 and murine L929 cell lines.
Materials and Methods
Cell Cultures
L929 mouse fibroblasts (ATCC-CCL1) were cultured with Dulbecco's Modified Eagle Medium (DMEM, Gibco) added with 10%, fetal bovine serum (FBS, Gibco), 1 mM L-glutamine (Gibco), and 1% penicillin-streptomycin (Gibco). Caco2 human epithelial cell line (ATCC HTB-37) obtained from colorectal adenocarcinoma were cultured with DMEM with 10%, of FBS and 1% penicillin-streptomycin. Cells were grown in a monolayer condition at 37°C in an atmosphere of 5% CO2 and for the cell treatments, cells were cultured with DMEM alone.
Test Substance
Glyphosate (PestanalTM analytical standard, CAS number 1071-83-6, purity > 99.5%) was obtained from Sigma-Aldrich (Milan, Italy). The commercial formulation Roundup Bioflow (containing 360 g/L of glyphosate acid in the form of 480 g/l isopropylamine salts of glyphosate (41.5%), water (42.5%), and surfactant (16%, ingredient not disclosed by the producer) was supplied from a local agricultural consortium (Consorzio Agrario dell'Emilia, Bologna, Italy). Glycine was supplied from Biosolve (Valkenswaard, Netherlands).
Cell Treatments
Glycine (Biosolve), Glyphosate (Sigma-Aldrich), and Roundup®Bioflow were added to cells for 24 h. Glycine and Glyphosate were diluted in water and the treatment doses were prepared at different concentrations according the following formula:
The final concentration of the treatments was of 6.125 g/L [36.676 mmol/L]; 0.6125 g/L [3.667 mmol/L]; 0.06125 g/L [0.3667 mmol/L], and 0.006125 g/L [0.03667 mmol/L] (equivalent respectively to 175, 17.5, 1.75, and 0.175 mg/kg bw). Each dilution was prepared in DMEM and the pH of each final solution was corrected to 7.0 by adding NaHCO3 (Gibco).
Measurement of Cell Proliferation for Adherent Cells (MTT)
Cell cytotoxicity was measured using the3-(4,5-dimetiltiazol-2-il)-2,5-difeniltetrazolio (MTT) assay. L929 cells (5 × 104 cells/well) and Caco2 cells (105 cells/well) were plated in 96-well tissue culture plate in complete medium (100 μL/well). The multiwell plates were incubated at 37°C, 5% CO2 for 24 h. After 24 h, the culture medium was removed and equal volumes (100 μL) of the treatments were added to each well. In control wells, 100 μL DMEM were added. Control wells consisted of untreated cell cultures. Twenty-four hours later, proliferative cells were detected by MTT assay, according to the ISO 10993-5 International Standard procedure (43). The main purpose of the ISO 10993-5 procedure is to define a scheme for testing in vitro cytotoxicity of different extracts according to a multi-step approach. Briefly, cells were incubated with MTT solution (1 mg/mL, Life Technologies) at 37°C for 2 h. Then, MTT solution was removed and cells were solubilized with 100 μl of isopropanol. The formazan dye formation was evaluated by scanning multiwell spectrophotometer at 540 nm. The results were expressed as percentage of viable cells compared to controls.
Measurement of Cell Viability (Trypan Blue) and Average Cell Size
Cell viability was measured using the Trypan Blue assay. L929 and Caco2 cells were plated in 24-well tissue culture plate (50 × 105 cells/well) in complete medium. The day after, treatments diluted in DMEM were added to cells for 24 h. To detect viability and cells size, cells were trypsinized with a solution of trypsin 0.05% and EDTA 0.02%. Cell were then carefully diluted in a 0.4% Trypan Blue (Gibco) solution by preparing a 1:1 dilution with the cell suspension. Viable cells were counted and average cell size analyzed by using Countess®II FL (Thermo Fisher Scientific). The results were expressed as percentage of viable cells compared to controls.
Statistical Analysis
The MTT cell tests were carried with six replicates for each treatment and data were expressed as mean values of three different experiments. Statistical analysis was performed with R software (44). Normal and homoscedastic data were analyzed with ANOVA followed by pairwise comparison (Dunnett test) and Tukey post-hoc tests with Bonferroni correction. Non-normal homoscedastic data were analyzed with the non-parametric Kruskall–Wallis test and Dunn's post-hoc test with Bonferroni correction. Differences were considered to be significant at a p < 0.05. IC50 values were calculated by Probit regression.
Results
MTT: Effects of Glycine, Glyphosate, and Roundup Bioflow on L929 and Caco2 Cells Proliferation
Glycine did not modulate either L929 or Caco2 cell proliferation at any of the concentrations used in the MTT assays (Figure 1). Statistically significant decreases of proliferating cells were observed at all doses of glyphosate compared to controls in both L929 models and Caco2 models, except at the lowest dose in Caco2 (Figure 1). Glyphosate showed also positive correlation between the percentage of proliferating cells as a function of the concentration for both L929 cells (R = 0.957, Figure 2A) and Caco2 cells (R = 0.986, Figure 2B). Statistically significant decreases of proliferating cells were observed at all doses of Roundup Bioflow compared to controls in both L929 models and Caco2 models, except at the lowest dose in L929 (Figure 1). Roundup Bioflow showed also positive correlation between the percentage of proliferating cells as a function of the pesticide concentration for both L929 and Caco2 cells, with R = 0.956 and 0.978, respectively (Figures 2A,B).
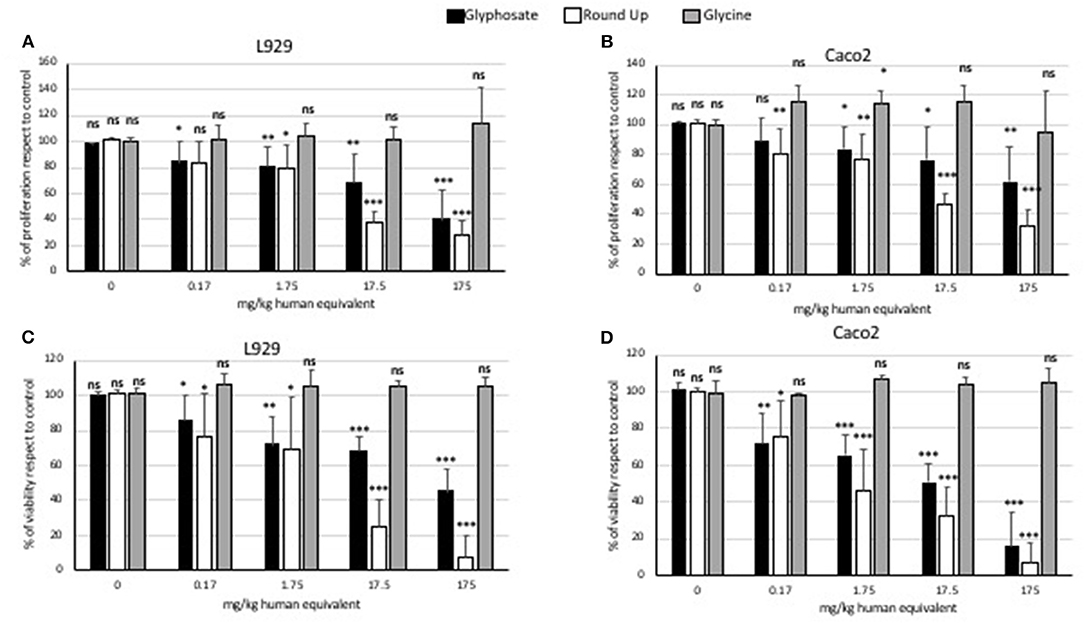
Figure 1. MTT (proliferation) assay and Trypan Blue (viability) assay: effects of pure glyphosate, Roundup Bioflow, and glycine at the dose range 0–175 mg kg−1 (human equivalent) on cell proliferation of L929 fibroblasts (A), Caco2 cells (B), and on cell viability of L929 fibroblasts (C) and Caco2 cells (D). Data are expressed as mean value (± st. dev.) (% compared to control). Pairwise comparison based on Anova (Dunnett test). ns, not significant: *P < 0.05; **P < 0.01; ***P < 0.001.
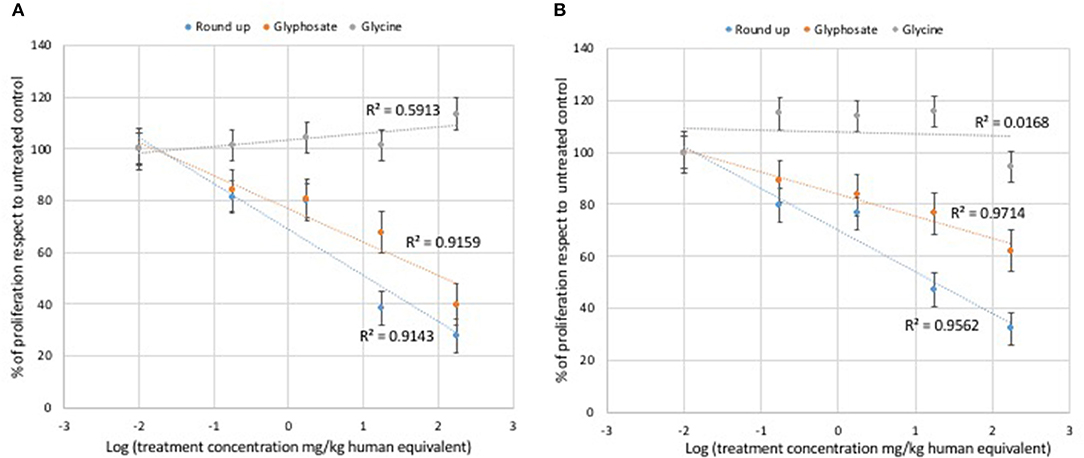
Figure 2. Relationship between pure glyphosate, Roundup Bioflow, and glycine at the dose range 0–175 mg kg−1 (human equivalent) (Log scale) and cell proliferation of L929 fibroblasts (A) and Caco2 cells (B). Data are expressed as mean value (± st. dev.) (% compared to control). R2, coefficient of determination.
Trypan Blue: Effects of Glycine, Glyphosate, and Roundup Bioflow on L929 and Caco2 Cells Viability
Glycine did not modulate either L929 or Caco2 cell viability at any of the concentrations used for cell treatments (Figure 1). Statistically significant decreases of vital cells were observed at all doses of glyphosate compared to controls in both L929 models and Caco2 models (Figure 1). Glyphosate showed also a positive correlation between the percentage of vital cells compared to control and the dose treatments both for L929 fibroblasts (R = 0.978, Figure 3A) and intestinal cell Caco2 (R = 0.972, Figure 3B). Statistically significant decreases of vital cells were observed at all doses of Roundup Bioflow compared to controls in both L929 models and Caco2 models (Figure 1). Roundup Bioflow showed also a positive correlation as a function of the pesticide concentration for both L929 and Caco2 cells with R = 0.975 (Figure 3A) and R = 0.996 (Figure 3B), respectively.
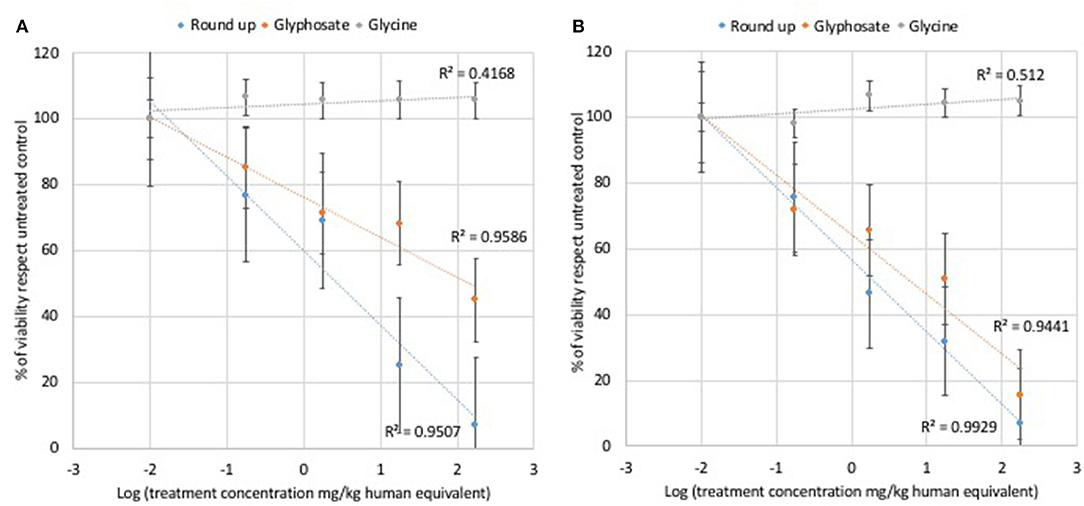
Figure 3. Relationship between pure glyphosate, Roundup Bioflow, and glycine at the dose range 0–175 mg kg−1 (human equivalent) (Log scale) and cell viability of L929 fibroblasts (A) and Caco2 cells (B). Data are expressed as mean value (± st. dev.) (% compared to control). R2, coefficient of determination.
Effects of Glyphosate and Roundup Bioflow on L929 and Caco2 Average Cell Size
Statistically significant decreases of average cell size were observed at all doses of glyphosate compared to controls in both L929 models and Caco2 models (Figure 4). Statistically significant decreases of average cell size were observed at all doses of Roundup Bioflow compared to controls in both L929 models and Caco2 models (Figure 4).
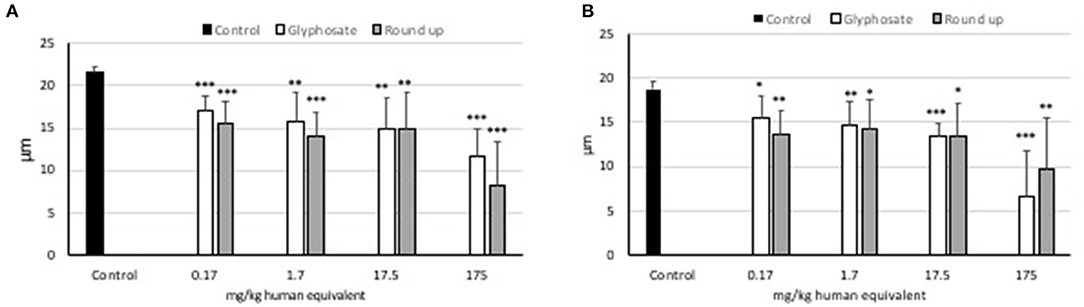
Figure 4. Effects of pure glyphosate and Roundup Bioflow at the dose range 0–175 mg kg−1 (human equivalent) on cell size of L929 fibroblasts (A) and Caco2 cells (B). Data are expressed as mean value (± st. dev.). Pairwise comparison based on Anova (Dunnett test). ns, not significant: *P < 0.05; **P < 0.01; ***P < 0.001.
Correlation Between MTT and Trypan Blue Assays for L929 and Caco2 Cells Treated With Glyphosate and Roundup Bioflow
A correlation was performed between results obtained from the MTT assay and the viability analysis obtained by the treatment of L929 (Figure 5) and Caco2 cells (Figure 6) with Glyphosate and Roundup Bioflow. Regarding L929 fibroblasts, both Glyphosate (Figure 5A) and Roundup Bioflow (Figure 5B) treatments showed a positive correlation as a function of the concentrations, even if it resulted more evident when L929 fibroblasts were treated with Roundup Bioflow. Regarding Caco2 cell treatments, both Glyphosate (Figure 6A) and Roundup Bioflow (Figure 6B) treatments showed a positive correlation as a function of the concentrations even if it resulted more evident when L929 fibroblasts were treated with Roundup Bioflow. Analysis revealed that both of the correlations were statistically significant at P < 0.05.
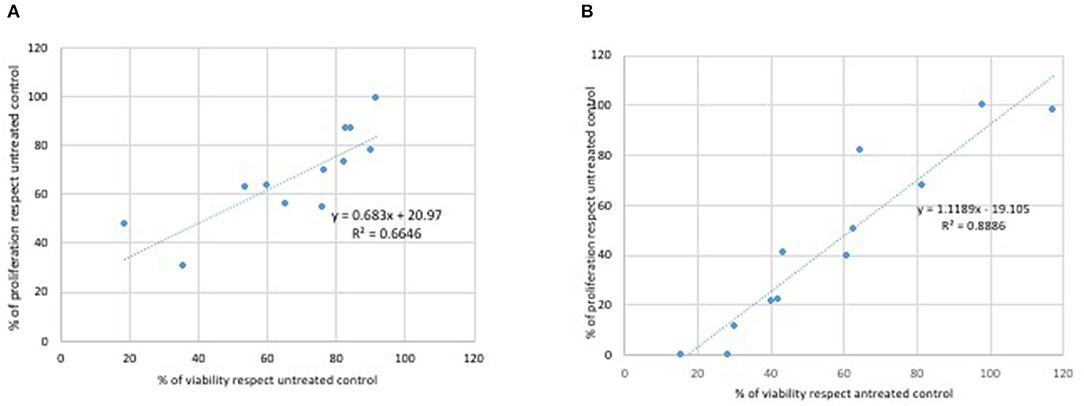
Figure 5. Correlation between MTT and Trypan Blue test for L929 cells treated with different doses of Glyphosate (A) and Roundup (B). Both correlations were statistically significant at P < 0.05.
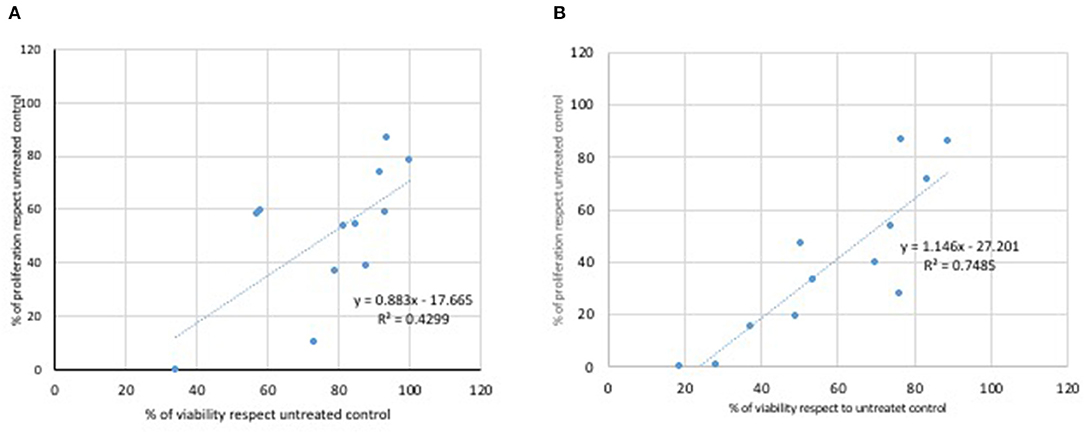
Figure 6. Correlation between MTT and Trypan Blue test for Caco2 cells treated with different doses of Glyphosate (A) and Roundup (B). Both correlations were statistically significant at P < 0.05.
IC50 (ug/L) Mean Values Calculated for MTT and Trypan Blue on L929 and Caco2 Cell Lines
IC50 mean values for MTT and viability and size regarding the treatments of Glycine, Glyphosate, and Roundup Bioflow was calculated related L929 fibroblasts and Caco2 cells. For Glycine, it was not possible to calculate IC50 for either L929 fibroblasts or Caco2 cells. In L929 treatments (Table 1) Roundup Bioflow showed IC50 mean value significantly lower compared to the IC50 mean value induced by Glyphosate treatments for both the MTT and Trypan Blue assays. In Caco2 treatments (Table 2), Roundup Bioflow showed IC50 which was significantly lower than the one induced by Glyphosate for MTT, while statistical significance was not reached for differences relating viability assay.
Discussion
The MTT and viability results confirm a different mechanism of action of glycine and its analog glyphosate (and its formulation Roundup Bioflow). In fact glycine did not show any sign of cytotoxicity, confirming the extremely safe profile of this substance, in line with the reported EU NOAEL (2,000 mg/Kg bw). On the other hand, glyphosate and its formulation Roundup showed a clear dose-related cytotoxic effects in MTT and viability assays in both Caco2 human intestinal cell line and L929 murine fibroblast cell line. The cytotoxic effects were also observed at doses that are lower than the current EU NOAEL (50 mg/Kg bw). Therefore, our results confirm previous evidence of cytotoxicity of glyphosate in in vitro models on Raji human hematological cell lines (45). In addition, our findings support the hypothesis of a higher toxic potency of the formulation, compared to pure glyphosate, in line with the results observed in other in vitro cytotoxicity studies by other authors (28, 46) and the results of our recent in vivo studies on Sprague–Dawley rats exposed to the same formulation (Roundup Bioflow) (34). Furthermore, different organophosphates other than glyphosate proved had similar effects in different in vitro models: in particular malathion exposure induced dose-dependent cytotoxic effects in MTT assays performed on HepG2 human liver cell line (47), diazinon exposure induced dose-dependent cytotoxic effects in MTT assays performed on HCT116 human intestinal cell line (48), chlorpyrifos induced dose-and time-dependent cytotoxic effects in MTT assays performed on SH-SY5Y human neural cell line (49). However, our experiment presents certain limitations: (1) direct quantitative extrapolations of IC50 values from cell lines to the human in vivo situation might under or overestimate the effects, therefore further studies are recommended to establish a dose-response relationship in vivo (50) (2) the equivalent dose was calculated based on the assumption that all of the substance administered would lead to exposure of the intestinal system (in the case of the intestinal model Caco2) or in a distant organ (the case of the fibroblast model L929), however the first is a probably more realistic exposure scenario for glyphosate in light of the relatively limited systemic absorption of glyphosate (and the high level of gastrointestinal exposure) (17), while the second might be a more realistic exposure for glycine that presents higher systemic absorption rates; (3) routes of exposures other than oral that are particularly relevant in the occupational setting, thus inhalation and/or skin absorption, were not taken into account in this model, although glyphosate can reach through this routes other organs and systems before being metabolized to AMPA; (4) we could not test separately the cytotoxicity of the adjuvants present in the formulation Roundup Bioflow as these are trade secrets.
Conclusion
Glyphosate and its formulation Roundup Bioflow, but not glycine, caused dose-related cytotoxic effects in in vitro MTT and viability assays in human intestinal and murine fibroblast models (Caco2 and L929). Our results showed that glycine and its analog glyphosate presented different cytotoxicity profiles. Glyphosate and Roundup Bioflow demonstrate cytotoxicity similar to other organophosphates, in particular malathion, diazinon, and chlorpyriphos. Notably, glyphosate, diazinon, and malathion have been recently classified as probable carcinogen by IARC (Group 2A) (51). The formulation Roundup Bioflow seem to be more cytotoxic than pure glyphosate. Cytotoxic effects of GBHs were observed at doses that are lower than the current EU NOAEL (50 mg/Kg bw).
Data Availability Statement
The original contributions presented in the study are included in the article, further inquiries can be directed to the corresponding author.
Author Contributions
DM and FT prepared the first draft of manuscript. DM, FT, and GD designed the experiments. FT conducted the experiments. GD and FB supervised the work. GD performed statistical analyses and reviewed the manuscript. PS, ES, and FG critically revised the manuscript and originally contributed to the methods and the elaboration of the results. All authors contributed to the article and approved the submitted version.
Funding
This work was funded by Institutional funds of Ramazzini Institute, Bologna, Italy; Fondazione Carisbo; Coopfond Fondo Mutualistico Legacoop; EU-COST Action DiMoPEx (under Grant no. CA 15129).
Conflict of Interest
The authors declare that the research was conducted in the absence of any commercial or financial relationships that could be construed as a potential conflict of interest.
Acknowledgments
We thank the over 30,000 associates and volunteers of the Ramazzini Institute and Coopfond Fondo Mutualistico Legacoop for supporting our research activity. The authors also thank all the EU-COST Action DiMoPEx colleagues for support, in particular its late Chair Prof. Lygia Therese Budnik that recently passed away and will be deeply missed.
References
1. Myers JP, Antoniou MN, Blumberg B, Carroll L, Colborn T, Everett LG, et al. Concerns over use of glyphosate-based herbicides and risks associated with exposures: a consensus statement. Environ Health. (2016) 15:19. doi: 10.1186/s12940-016-0117-0
2. Benbrook CM. Trends in glyphosate herbicide use in the United States and globally. Environ Sci Europe. (2016) 28:3. doi: 10.1186/s12302-016-0070-0
3. Smith EA, Oehme FW. The biological activity of glyphosate to plants and animals: a literature review. Vet Hum Toxicol. (1992) 34:531–43.
4. Nandula VK. Glyphosate Resistance in Crops and Weeds: History, Development, and Management. Hoboken, NJ: John Wiley & Sons (2010).
5. Majewski MS, Coupe RH, Foreman WT, Capel PD. Pesticides in Mississippi air and rain: a comparison between 1995 and 2007. Environ Toxicol Chem. (2014) 33:1283–93. doi: 10.1002/etc.2550
6. ISPRA. National Report of Pesticides in Water (2013–2014) [Rapporto Nazionale Pesticidi Nelle Acque Dati 2013–2014 (in italian)] Rapporti 244/2016 (2016).
7. Battaglin WA, Meyer MT, Kuivila KM, Dietze JE. Glyphosate and its degradation product AMPA occur frequently and widely in U.S. soils, surface water, groundwater, and precipitation. J Am Water Resour Assoc. (2014) 50:214–90. doi: 10.1111/jawr.12159
8. Rendón-von Osten J, Dzul-Caamal R. Glyphosate residues in groundwater, drinking water and urine of subsistence farmers from intensive agriculture localities: a survey in Hopelchén, Campeche, Mexico. Int J Environ Res Public Health. (2017) 14:595. doi: 10.3390/ijerph14060595
9. Cuhra M. Review of GMO safety assessment studies: glyphosate residues in Roundup Ready crops is an ignored issue. Environ Sci Eur. (2015) 27:20. doi: 10.1186/s12302-015-0052-7
10. USDA. Agricultural Marketing Service. Pesticide data program annual summary, program year 2011. In: Appendix C Distribution of Residues in Soybean by Pesticide. Washington, DC: U.S. Department of Agriculture (2013).
11. EFSA. The 2014 European Union Report on pesticide residues in food. EFSA J. (2016) 14:e04611. doi: 10.2903/j.efsa.2016.4611
12. PRIF. Expert Committee on Pesticide Residues in Food (PRiF). Annual Report for 2016 (2016). Available online at: https://wwwgovuk/government/publications/expert-committee-on-pesticide-residues-in-food-prif-annual-report
13. Mesnage R, Defarge N, Rocque LM, Spiroux de Vendomois J, Seralini GE. Laboratory rodent diets contain toxic levels of environmental contaminants: implications for regulatory tests. PLoS One. (2015) 10:e0128429. doi: 10.1371/journal.pone.0128429
14. WHO. Glyphosate and AMPA in Drinking-Water. Background document for development of WHO Guidelines for Drinking-water Quality. World Health Organization (2005).
15. Powles P. 14C-Glyphosate: Absorption, Distribution, Metabolism and Excretion Following Repeated Oraladministration to the Dairy Goat. Unpublished report No. 676/9-1011, dated 7 November 1994 from Hazle-ton Europe, Harrogate, England. Submitted to WHO by Cheminova A/S, Lemvig, Denmark (1994).
16. Colvin L, Miller J. CP 67573 Residue and Metabolism. Part 9: the Metabolism of N-Phosphonmethylglycine-14C (CP 67573-14C) in the Rabbit. Unpublished report (1973). 298 p.
17. Brewster DW, Warren J, Hopkins WE. Metabolism of glyphosate in Sprague-Dawley rats: tissue distribution, identification, and quantitation of glyphosate-derived materials following a single oral dose. Fundam Appl Toxicol. (1991) 17:43–51. doi: 10.1093/toxsci/17.1.43
18. NTP. NTP Technical Report on the Toxicity Studies of Glyphosate (CAS No. 1071-83-6) Administered In Dosed Feed To F344/N Rats and B6C3F1 Mice. Toxicity Report Series 16:1-D3 (1992).
19. ECHA. Glycine. Registered Dossier. EC number: 200-272-2 | CAS number: 56-40-6 (2020) (accessed December 16, 2020)
20. IARC. Outdoor Air Pollution. IARC Monographs on the Evaluation of Carcinogenic Risks to Humans Volume 109 (2015).
21. ECHA. REACH Regulation. (2015). Available online at: http://echa.europa.eu/regulations/reach (accessed June 3, 2015).
22. ATSDR. Toxicological Profile for Silica. Atlanta, GA: U.S. Department of Health and Human Services, Public Health Service (2019).
24. Mullin CA, Fine JD, Reynolds RD, Frazier MT. Toxicological risks of agrochemical spray adjuvants: organosilicone surfactants may not be safe. Front Public Health. (2016) 4:92. doi: 10.3389/fpubh.2016.00092
25. Landrigan PJ, Benbrook C. GMOs, herbicides, and public health. N Engl J Med. (2015) 373:693–5. doi: 10.1056/NEJMp1505660
26. Nagy K, Duca RC, Lovas S, Creta M, Scheepers PTJ, Godderis L, et al. Systematic review of comparative studies assessing the toxicity of pesticide active ingredients and their product formulations. Environ Res. (2020) 181:108926. doi: 10.1016/j.envres.2019.108926
27. Coalova I, Rios de Molina Mdel C, Chaufan G. Influence of the spray adjuvant on the toxicity effects of a glyphosate formulation. Toxicol In Vitro. (2014) 28:1306–11. doi: 10.1016/j.tiv.2014.06.014
28. Mesnage R, Bernay B, Seralini GE. Ethoxylated adjuvants of glyphosate-based herbicides are active principles of human cell toxicity. Toxicology. (2013) 313:122–8. doi: 10.1016/j.tox.2012.09.006
29. Defarge N, Takacs E, Lozano VL, Mesnage R, Spiroux de Vendomois J, Seralini GE, et al. Co-formulants in glyphosate-based herbicides disrupt aromatase activity in human cells below toxic levels. Int J Environ Res Public Health. (2016) 13:264. doi: 10.3390/ijerph13030264
30. Williams GM, Kroes R, Munro IC. Safety evaluation and risk assessment of the herbicide Roundup and its active ingredient, glyphosate, for humans. Regul Toxicol Pharmacol. (2000) 31(2 Pt 1):117–65. doi: 10.1006/rtph.1999.1371
31. Peillex C, Pelletier M. The impact and toxicity of glyphosate and glyphosate-based herbicides on health and immunity. J Immunotoxicol. (2020) 17:163–74. doi: 10.1080/1547691X.2020.1804492
32. Mao Q, Manservisi F, Panzacchi S, Mandrioli D, Menghetti I, Vornoli A, et al. The Ramazzini Institute 13-week pilot study on glyphosate and Roundup administered at human-equivalent dose to Sprague Dawley rats: effects on the microbiome. Environ Health. (2018) 17:50. doi: 10.1186/s12940-018-0394-x
33. Panzacchi S, Mandrioli D, Manservisi F, Bua L, Falcioni L, Spinaci M, et al. The Ramazzini Institute 13-week study on glyphosate-based herbicides at human-equivalent dose in Sprague Dawley rats: study design and first in-life endpoints evaluation. Environ Health. (2018) 17:52. doi: 10.1186/s12940-018-0393-y
34. Manservisi F, Lesseur C, Panzacchi S, Mandrioli D, Falcioni L, Bua L, et al. The Ramazzini Institute 13-week pilot study glyphosate-based herbicides administered at human-equivalent dose to Sprague Dawley rats: effects on development and endocrine system. Environ Health. (2019) 18:15. doi: 10.1186/s12940-019-0453-y
35. Guyton KZ, Rusyn I, Chiu WA, Corpet DE, van den Berg M, Ross MK, et al. Application of the key characteristics of carcinogens in cancer hazard identification. Carcinogenesis. (2018) 39:614–22. doi: 10.1093/carcin/bgy031
36. Samet JM, Chiu WA, Cogliano V, Jinot J, Kriebel D, Lunn RM, et al. The IARC monographs: updated procedures for modern and transparent evidence synthesis in cancer hazard identification. J Natl Cancer Inst. (2019) 112:30–7. doi: 10.1093/jnci/djz169
37. Strober W. Trypan blue exclusion test of cell viability. Curr Protoc Immunol. (2015) 111:A3.B.1–A3.B.3. doi: 10.1002/0471142735.ima03bs111
38. Riss TL, Moravec RA, Niles AL, Duellman S, Benink HA, Worzella TJ, et al. Cell viability assays. In: Markossian S, Sittampalam GS, Grossman A. editors. Assay Guidance Manual. Bethesda, MD: Eli Lilly & Company and the National Center for Advancing Translational Sciences (2016).
39. Gardner RM, Nyland JF, Silbergeld EK. Differential immunotoxic effects of inorganic and organic mercury species in vitro. Toxicol Lett. (2010) 198:182–90. doi: 10.1016/j.toxlet.2010.06.015
40. Kamiloglu S, Sari G, Ozdal T, Capanoglu E. Guidelines for cell viability assays. Food Front. (2020) 1:332–49. doi: 10.1002/fft2.44
41. van Tonder A, Joubert AM, Cromarty AD. Limitations of the 3-(4,5-dimethylthiazol-2-yl)-2,5-diphenyl-2H-tetrazolium bromide (MTT) assay when compared to three commonly used cell enumeration assays. BMC Res Notes. (2015) 8:47. doi: 10.1186/s13104-015-1000-8
42. Avelar-Freitas BA, Almeida VG, Pinto MC, Mourão FA, Massensini AR, Martins-Filho OA, et al. Trypan blue exclusion assay by flow cytometry. Braz J Med Biol Res. (2014) 47:307–15. doi: 10.1590/1414-431X20143437
43. ISO. 10993-5, INTERNATIONAL STANDARD. Biological Evaluation of Medical Devices -Part 5:Tests for In vitro Cytotoxicity, Third Edition 2009. International Organization for Standardization (2009).
44. R Core-Team. A Language and Environment for Statistical Computing. Vienna: R Foundation for Statistical Computing (2016). Available online at: http://www.R-project.org (accessed April 18, 2020).
45. Townsend M, Peck C, Meng W, Heaton M, Robison R, O'Neill K. Evaluation of various glyphosate concentrations on DNA damage in human Raji cells and its impact on cytotoxicity. Regul Toxicol Pharmacol. (2017) 85:79–85. doi: 10.1016/j.yrtph.2017.02.002
46. Wozniak E, Sicinska P, Michalowicz J, Wozniak K, Reszka E, Huras B, et al. The mechanism of DNA damage induced by Roundup 360 PLUS, glyphosate and AMPA in human peripheral blood mononuclear cells - genotoxic risk assessement. Food Chem Toxicol. (2018) 120:510–22. doi: 10.1016/j.fct.2018.07.035
47. Moore PD, Yedjou CG, Tchounwou PB. Malathion-induced oxidative stress, cytotoxicity, and genotoxicity in human liver carcinoma (HepG2) cells. Environ Toxicol. (2010) 25:221–6. doi: 10.1002/tox.20492
48. Boussabbeh M, Ben Salem I, Hamdi M, Ben Fradj S, Abid-Essefi S, Bacha H. Diazinon, an organophosphate pesticide, induces oxidative stress and genotoxicity in cells deriving from large intestine. Environ Sci Pollut Res. (2016) 23:2882–9. doi: 10.1007/s11356-015-5519-y
49. Raszewski G, Lemieszek MK, Łukawski K, Juszczak M, Rzeski W. Chlorpyrifos and cypermethrin induce apoptosis in human neuroblastoma cell line SH-SY5Y. Basic Clin Pharmacol Toxicol. (2015) 116:158–67. doi: 10.1111/bcpt.12285
Keywords: mechanism, glyphosate, cancer, toxicity, biomarker
Citation: Truzzi F, Mandrioli D, Gnudi F, Scheepers PTJ, Silbergeld EK, Belpoggi F and Dinelli G (2021) Comparative Evaluation of the Cytotoxicity of Glyphosate-Based Herbicides and Glycine in L929 and Caco2 Cells. Front. Public Health 9:643898. doi: 10.3389/fpubh.2021.643898
Received: 19 December 2020; Accepted: 08 April 2021;
Published: 07 May 2021.
Edited by:
Mohiuddin Md. Taimur Khan, Washington State University Tri-Cities, United StatesReviewed by:
M. Jahangir Alam, University of Houston, United StatesPaola Ingaramo, National University of Littoral, Argentina
Copyright © 2021 Truzzi, Mandrioli, Gnudi, Scheepers, Silbergeld, Belpoggi and Dinelli. This is an open-access article distributed under the terms of the Creative Commons Attribution License (CC BY). The use, distribution or reproduction in other forums is permitted, provided the original author(s) and the copyright owner(s) are credited and that the original publication in this journal is cited, in accordance with accepted academic practice. No use, distribution or reproduction is permitted which does not comply with these terms.
*Correspondence: Daniele Mandrioli, bWFuZHJpb2xpZCYjeDAwMDQwO3JhbWF6emluaS5pdA==; Giovanni Dinelli, Z2lvdmFubmkuZGluZWxsaSYjeDAwMDQwO3VuaWJvLml0
†These authors share first authorship