- 1Department of Microbiology, All India Institute of Medical Sciences, New Delhi, India
- 2Geriatric Medicine, All India Institute of Medical Sciences, New Delhi, India
- 3Pediatrics, All India Institute of Medical Sciences, New Delhi, India
- 4Biostatistics, All India Institute of Medical Sciences, New Delhi, India
- 5Pulmonary, Critical Care, and Sleep Medicine, All India Institute of Medical Sciences, New Delhi, India
Background: Legionnaires' disease (LD) is a potentially fatal pneumonia predominantly caused by infection due to Legionella pneumophila although more than 50 other Legionella species are described. Water systems contaminated with Legionella spp. are the implicated sources of Legionnaires' disease. In this study, we aimed to assess Legionella contamination in the water sources of a tertiary care hospital and to determine the virulence properties and molecular characteristics of L. pneumophila environmental isolates.
Methods: During May 2015 through August 2018, a total of 201 hospital water samples were tested for L. pneumophila by standardized culture procedures; environmental isolates were examined for the presence of two virulence genes: Legionella vir homolog (lvh) and repeats in structural toxin (rtxA) by PCR. The genotyping of isolates was performed by sequence-based typing (SBT) according to the protocol of the European Study Group for Legionella Infections (ESGLI).
Results: L. pneumophila was isolated from 38/201 (18.9%) water samples; among the 46 isolates, the lvh locus was present in 45 (97.8%), the rtxA locus was found in 45 (97.8%), and both loci were found in 44 (95.7%) isolates. A total of 23 sequence types (STs) were identified among the 44 isolates (index of discrimination [IOD] of 0.929), and 11/23 (47.8%) STs were new to the ESGLI database.
Conclusions: The study results showed genetic diversity in L. pneumophila isolates from the hospital environment along with a high percentage of pathogenicity loci. Besides, certain STs may have an increased ability to cause legionellosis, thus requires specific infection control and prevention strategies whenever identified.
Introduction
Legionella pneumophila, the etiological agent of atypical pneumonia known as Legionnaires' disease (LD), is the inhabitants of both natural and human-made aquatic environments (1). LD is the most common waterborne disease, and the reported cases of legionellosis have shown rising trends both in the United States and Europe (2, 3). Presently, the genus Legionella includes more than 70 distinct serogroups from >60 known species, and of these, at least 30 species have been associated with opportunistic infections in humans. L. pneumophila accounts for ~90% of LD cases, and the majority of clinical cases are attributed to serogroup 1 of L. pneumophila (Lp1) (1, 4).
Legionella spp. can survive for prolonged periods in aquatic systems, can evade and multiply in free-living protozoa and replicate in the presence of biocides, including chlorine (5). Legionella contamination has been increasingly reported in cooling towers (CT), hot springs, foot spas, drinking and non-drinking water systems of hotels, nursing homes, and health care facilities (6, 7). The periodic monitoring of Legionella in the hospital water systems allows for risk prediction and the elimination of this pathogen from possible infection sources (7). In India, LD has been sporadically reported from specific locations, but the disease clusters and outbreaks are so far not identified (8, 9). The water safety regulations for Legionella monitoring and decontamination are absent in this country, and no active surveillance program exists for monitoring L. pneumophila in the hospital environment.
Studies indicate that difference exists between L. pneumophila strains, particularly in their ability to withstand in external environments and to produce infections in humans. These differences are mainly attributed to the presence or absence of specific genes encoding virulence among bacterial isolates (10). Several virulence genes in Legionella spp. including the type IV secretion system genes, intracellular multiplication/defective in organelle trafficking (icm/dot), tra1, Legionella vir homolog (lvh), type IV pilus genes pilDE, macrophage infectivity potentiator (mip), repeats in structural toxin (rtxA), and enhC have been characterized and are extensively studied (10–12). The lvh locus derives protein for a second type IV secretion system that contributes to conjugation and virulence (13). The rtxA gene is a pore-forming toxin that is regulated by the dot/icm complex that contributes to cellular entry and subsequent adherence to the host cell (14). Previous studies have reported that the lvh and rtxA regions are found more often in strains associated with human disease (10–12). Therefore, in the present study, we aimed to assess Legionella contamination in the water systems of a hospital, to identify the Legionella species and serogroups involved, and to survey the presence of two pathogenicity loci (the lvh and rtxA) in environmental isolates to determine their infection potential.
Molecular typing of L. pneumophila isolates is foremost important for epidemiological investigation of LD cases, clusters, and outbreaks. An outbreak source can be determined by linking strains from the environment to clinical strains by using different molecular typing methods (15). L. pneumophila isolates can be genotyped by sequence-based typing (SBT) using seven loci including five virulence genes (flaA, pilE, mip, mompS, and proA) and two housekeeping genes (asd and neuA) as proposed by the European Study Group for Legionella Infections (ESGLI) (16–18). The SBT is a rapid, reproducible, and highly discriminatory typing technique and, therefore, widely accepted as a gold standard for LD outbreak investigations and rapid identification of isolates that are closely related (4, 16, 17). Previous typing studies on L. pneumophila isolates indicate the dominance of certain sequence types (STs) in sporadic cases and outbreaks (4). However, from India, so far, no studies were reported to determine the DNA SBT of L. pneumophila isolates. Therefore, we further characterized L. pneumophila environmental isolates by SBT analysis and compared our data with the global database available at the ESGLI.
Materials and Methods
Environmental Surveillance
The study was conducted in a major tertiary care hospital in New Delhi, India, that has organ transplantation and cancer treatment facilities. During May 2015 through August 2018, 21 sites inside the hospital campus that spread over ~115 acres, including hospital, residential, and general areas, were monitored for the presence of L. pneumophila. Samplings were performed in different buildings hosting patient rooms, intensive care units, clinics, laboratories, and nursing stations. Both potable (PW; drinking water for patients, hospital staff, and public) and non-potable water samples (NPW; bathwater and water for handwashing) were collected, and Legionella testing was carried out four times per year. The samples were collected from distal outlets of hot and cold water taps and AC cooling towers (basin beneath the tower). The water temperature was measured at the time of sample collection by using a precision thermometer (Zeal, England). Legionella isolation and identification from water samples was done following the guidelines issued by the US Centers for Disease Control and Prevention (19). The detailed methodology regarding L. pneumophila environmental surveillance has been published elsewhere (20, 21).
Legionella Speciation and Identification of L. pneumophila Serogroup 1
All Legionella isolates collected during the surveillance period were subjected to a real-time PCR assay targeting the ssrA gene for the confirmation of the Legionella genus (22). Further identification of L. pneumophila was done by using a real-time PCR targeting the mip gene, and finally, the detection of Lp1 was done by using another real-time PCR targeting wzm gene (22). DNA isolated from L. pneumophila serogroup 1 ATCC 33152 (Strain Philadelphia) was used as a positive control for the standardization of real-time PCR.
Identification of Virulence Genes by Using PCR
All L. pneumophila isolates recovered from hospital water samples were surveyed for the presence of two virulence gene loci using previously published primers and PCR conditions (10, 11). Briefly, six-primer pairs were used in this study including lvh1/prpA-lvh2/prpA, lvh3/lvhB3-lvh4/lvhB4, lvh5/lvhB8-lvh6/lvhB9, and lvr1/lvrE-lvr2/lvrE for the amplification of the lvh region and rtx1/rtxA-rtx2/rtxA and rtx3/rtxA-rtx4/rtxA for the identification of the rtxA region. DNA extraction was performed by emulsifying 2–3 colonies in sterile water and boiling at 100°C for 10 min. PCR amplification involved 35 cycles of 1 min at 95°C for denaturation, 1 min at 55°C for annealing, and 1 min at 72°C for the extension. DNA isolated from L. pneumophila serogroup 1 ATCC 33152 (Strain Philadelphia) was used as a positive control for PCR.
L. pneumophila Genotyping by Sequence-Based Typing (SBT)
L. pneumophila SBT was performed by using the seven-gene (flaA, pilE, asd, mip, mompS, proA, neuA) protocol SBT scheme according to the guidelines issued by the ESGLI (version 5.0) (16–18). PCR amplification involved 35 cycles of 30 s at 94°C for denaturation, 30 s at 55°C for annealing, and 45 s at 72°C for the extension. After purification and sequencing (Barcode Biosciences Pvt. Ltd., Bangalore, India; Dr. KPC Life Sciences Pvt. Ltd., Kolkata, India), the forward and reverse sequence trace files were uploaded to the online Legionella Sequence Quality Tool (www.hpa-bioinformatics.org.uk/cgi-bin/legionella/sbt/seq_assemble_legionella1.cgi). Sequence alignment and trimming was performed by the tool and individual alleles, allelic profile, and a sequence type (ST) were identified. For each isolate, the profile of seven alleles at each of the loci was defined in the following order: flaA, pilE, asd, mip, mompS, proA, and neuA (e.g., 1, 4, 3, 1, 1, 1, 1). Finally, the ST was indicated by a number (e.g., ST1). For L. pneumophila non-Lp1 strains, if neuA is not amplified with standard neuA primers, amplification was done by using primers targeting neuAh (N-Acylneuraminate Cytidyltransferase homolog) according to an alternative ESGLI protocol (version 1.0) (23). The homolog (neuAh) has been described by Farhat et al. and is reported to be found in certain non-serogroup 1 (non-Lp1) strains when the neuA gene is not amplified with the standard neuA primers in the SBT protocol (23). Therefore, whenever the neuA gene is not amplified, in place of neuA result, the neuAh allele result was used according to the predetermined SBT order (flaA, pilE, asd, mip, mompS, proA, and neuAh) as recommended by the ESGLI (version 1.0). Finally, the combination of alleles is defined as 7-digit allelic profile (e.g., 8, 6, 34, 9, 2, 8, 209) and a ST was represented by a number (e.g., ST1417). Newly identified alleles and STs were submitted to the ESGLI SBT database (http://www.hpa-bioinformatics.org.uk/legionella/legionella_sbt/php/sbt_homepage.php).
Phylogenetic Analysis
We assessed the relationships between STs and within clonal complexes by using the goeBURST implemented in the PHYLOViZ program (http://www.phyloviz.net/goeburst/#Software). The default setting in the eBURST (the stringent group definition) was used; by this definition, a clonal complex contains STs that share six of the seven evaluated SBT alleles with at least one other member of the group and are all believed to be descended from the same founding genotype (primary founder) (24). Comparative goeBURST analysis was used to relate L. pneumophila STs identified in this study with those reported from Japan, China, and South Korea. The SBT data of 164 isolates from China, 135 isolates from Japan, and 104 isolates from South Korea were obtained from previous studies (6, 25, 26).
Statistical Analysis
Categorical variables were expressed in terms of numbers and percentages. The association between characteristics of water samples and L. pneumophila positivity was evaluated through odds ratio (OR) with 95% CI (confidence interval). A p-value (two-tailed) below 0.05 was considered to be statistically significant. The analysis was performed by using function odds. ratio in R-version 3.6.1. The index of diversity (IOD) was determined using Hunter and Gaston's modification of Simpson's index of diversity according to a previously described method (27).
Results
Environmental Surveillance
Among the 201 water samples collected during the study period, 38 (18.9%; 19 potable and 19 non-potable) were positive for L. pneumophila by culture. Of these, 10 (26.3%) each sample was collected from patient areas, residential areas, and general hospital areas, and 8 (21%) were from AC cooling towers. Regarding the 38 samples tested positive for L. pneumophila, the presence of Lp1 was detected in 25 (65.8%) samples, which included 11 potable and 14 non-potable water samples. The remaining 13 (34.2%) samples were contaminated due to Lp 2-14 serogroups. The temperature of L. pneumophila positive water samples ranged from 12 to 57°C (median temperature of 25°C), and the pathogen was most frequently isolated from a temperature range of 20°C-40°C (during 29 instances).
Among the 21 sampling sites, 15 were positive for L. pneumophila during at least one sampling event, two sites were positive for Legionella during two instances, three sites were positive during three instances, and four sites were positive during >4 instances. These four sites (two drinking water units and two AC cooling towers) that repeatedly tested positive for L. pneumophila were identified as high-risk sites. L. pneumophila positivity for the sampling sites and buildings located within these sites are shown in Table 1.
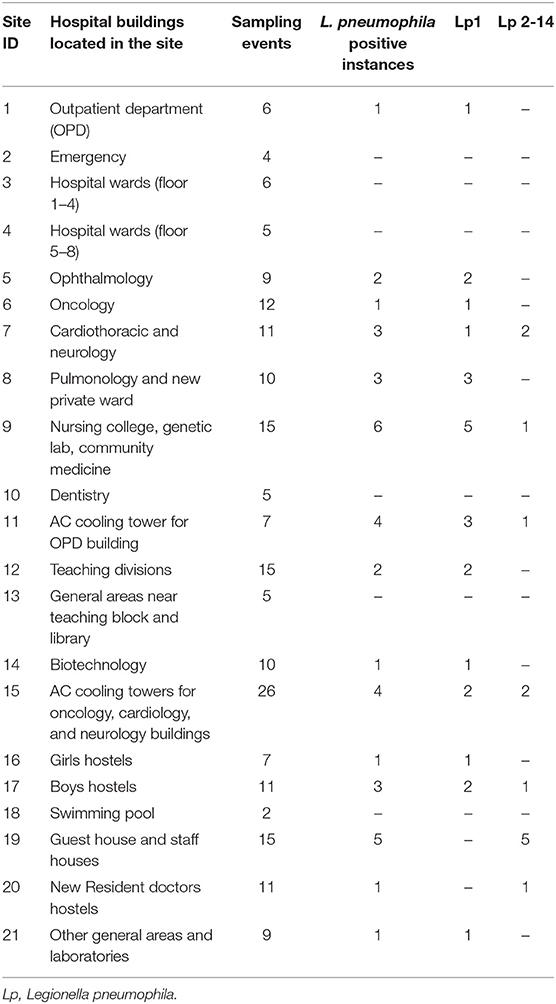
Table 1. Legionella pneumophila positivity according to water sampling sites and buildings in a Tertiary care hospital, India, 2015–2018.
We compared water samples with and without L. pneumophila and assessed characteristics including type, source, and temperature range of water samples, and age of water tanks or storage systems. None of these factors were found to have a significant association with L. pneumophila positivity (Table 2). During the study period, seasonal variations in Legionella positivity were not observed; the contamination was found to be consistent throughout the year.
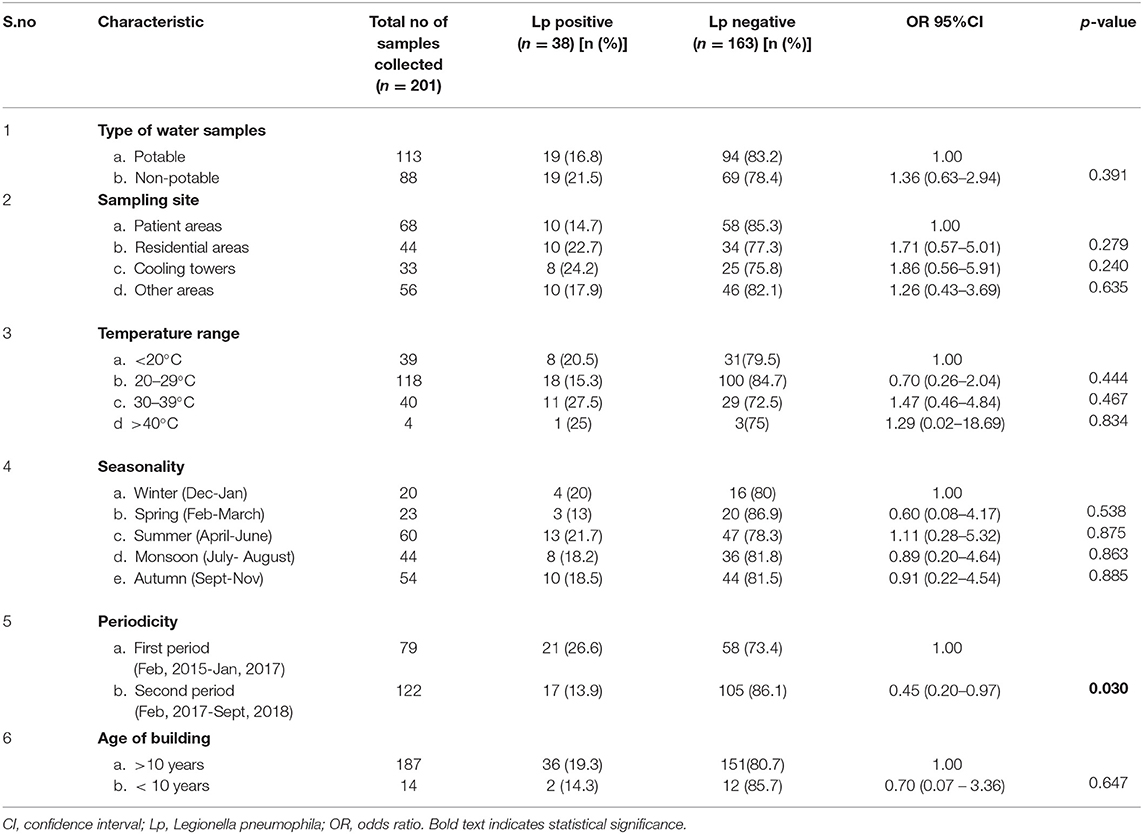
Table 2. Legionella pneumophila isolated from 201 water samples collected at a tertiary healthcare center, by type of water, sampling site, temperature, year and period, India, May 2015– August 2018.
Legionella Speciation and Identification of L. pneumophila Serogroup 1
A total of 47 L. pneumophila isolates were obtained from all positive samples during the study period. The number of Legionella isolates is not equal to the number of positive samples because, during some instances, we isolated different L. pneumophila serogroups (both Lp1 and Lp 2-14 [non-Lp1]) in the same sample. All isolates (n = 47) were identified as L. pneumophila by real-time PCR targeting the mip gene. Twenty-nine out of 47 (61.7%) isolates were identified as Lp1 by wzm gene real-time PCR, and the remaining isolates (n = 18 [38.3%]) were referred to as Lp 2-14. Serogrouping for the non-Lp1 isolates was not performed.
Identification of Virulence Genes by Using PCR
Of 47 L. pneumophila isolates, 46 were subjected to the identification of virulence genes. For one isolate, DNA was found to be degraded; therefore, excluded from the analysis. Among the tested isolates, at least one virulence gene loci (lvh or rtxA) was detected in all (100%) isolates. Specifically, the lvh locus was present in 45 (97.8%) isolates, the rtxA locus was found in 45 (97.8%), and both loci were found in 44 (95.7%) isolates. The simultaneous absence of the two loci was not observed in any of the tested isolates. Among the two groups (Lp1 and non-Lp1), both the gene loci were present in all Lp1 (n = 29, 100%) isolates tested. Of the non-Lp1 (n = 17) isolates, 15 (88.3%) tested positive for both genes, and the remaining two isolates showed the following pattern: lvh-positive, rtxA-negative and lvh-negative, rtxA-positive. Figure 1 shows PCR positive products in an environmental isolate tested for all primer pairs. Test results of the detection of virulence genes are shown in Table 3.
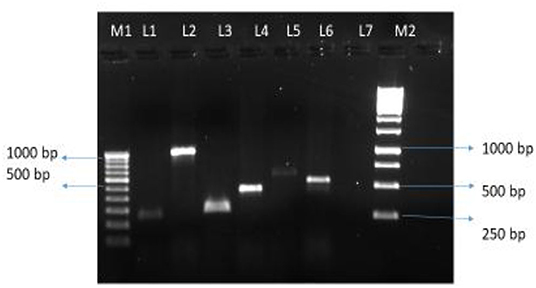
Figure 1. Legionella pneumophila isolate from water tested positive for six pairs of primers. Lane M1: 100 bp DNA ladder (100–1,000 bp) with DNA sizes indicated. Lane 1 to 6, PCR products for primer pairs for the lvh region: lvh1/prpA-lvh2/prpA (L1, 259 bp PCR product), lvh3/lvhB3-lvh4/lvhB4 (L2, 1007 bp PCR product), lvh5/lvhB8- lvh6/lvhB9 (L3, 294 bp PCR product), and lvr1/lvrE-lvr2/lvrE (L4, 423 bp PCR product), and for the rtxA region: rtx1/rtxA-rtx2/ rtxA (L5, 603 bp PCR product), and rtx3/rtxA-rtx4/rtxA (L6, 543 bp PCR product), Lane 7, blank, Lane M2: 1 Kb DNA ladder with representative sizes of DNA are indicated.
L. pneumophila Genotyping by Sequence-Based Typing (SBT)
SBT analysis assigned 44 environmental isolates into 23 distinct STs (IOD, 0.929). DNA of three isolates was found to be degraded; hence genotyping was not applied. For one Lp1 isolate, ST was not able to determine (Table 4). Six STs were associated with more than one isolate, and 17 STs were identified with one single isolate. The most predominant ST in this study was ST1, with 22.7% (10/44) of the isolates belonging to this sequence type. The other STs obtained more than once among the tested isolates were ST763 (n = 4; 9.1%), ST2848 (n = 4; 9.1%), ST2854 (n = 3; 6.8%), ST2868 (n = 3; 6.8%), and ST114 (n = 2; 4.5%). We identified the presence of the neuAh allele in 8 non-Lp1 isolates, which were not amplified using the standard neuA primers. They contained five different neuAh alleles, including neuAh 201, 207, 208, 209, and 228 (Table 4).
Querying the ESGLI database (available at http://www.ewgli.org), it was found that 11/23 (47.8%) STs were identified for the first time in this study. They are ST2848, ST2849, ST2850, ST2854, ST2855, ST2865, ST2866, ST2867, ST2868, ST2869, and ST2874. We also identified one new allele of the mip gene (mip 93) (Table 4).
Strains with indigenous STs were isolated from different water sources. Nine cooling tower (CT) isolates were divided into 6 STs (IOD, 0.888), 24 PW isolates were divided into 14 STs (IOD, 0.920), and 11 NPW isolates were divided into 8 STs (IOD, 0.933; except one isolate of which ST was not determined). ST1 was found in all three sources (PW; n = 6, NPW; n = 3, and CT; n = 1). Other common STs found across different water environments included ST2854 (CT; n = 2, NPW; n = 1), ST2848 (CT; n = 3, PW; n = 1), and ST763 (PW; n = 3, CT; n = 1). The only ST common across the patient, residential, and general areas of this hospital was ST1. Apart from ST1, 5 STs including ST114, ST322, ST1095, ST2849 (a new ST), and ST2869 (a new ST) were found to be present in the patient areas. Twenty-seven isolates of Lp1 were assigned into 11 STs (IOD, 0.824; except one isolate of which ST was not determined), and 17 non-LP1 isolates were assigned into 14 STs (IOD, 0.955). The common ST appeared in both groups (Lp1 and non-Lp1) was only ST114.
goeBURST Analysis
We applied goeBURST analysis to examine the relationship between STs obtained in this study with a single-locus variant selected (SLV). Five STs from this study were predicted to form two clonal complexes, whereas the remaining 18 STs did not relate to each other, therefore, identified as singletons. Clonal complex 1 (CC-1) consisted of 3 STs (ST1464, ST2850, ST2867), and 3/44 (6.8%) isolates belonged to this complex. The CC-2 consisted of two STs (ST1 and ST134), and 11/44 (25%) isolates belonged to this complex. The phylogenetic relationship between L. pneumophila STs identified by goeBURST analysis is shown in Figure 2.
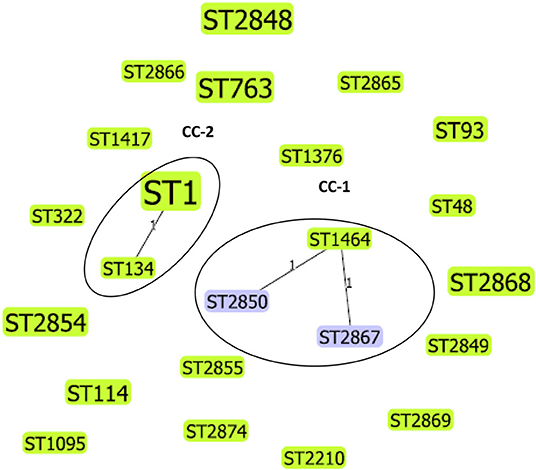
Figure 2. Phylogenetic relationship between L. pneumophila STs identified by goeBURST analysis, only the single-locus variants (SLV), is shown as a population snapshot. goeBURST analysis of 23 STs showed that 5 of these STs were predicted to form 2 clonal complexes, and 18 STs did not relate to each other and existed as singletons. Clonal complex 1 (CC-1) consisted of three STs and 3/44 (6.82%) isolates. Clonal complex 2 (CC-2) consisted of 2 STs and 11/44 (25%) isolates. The size of the ST node in the figure reflects the abundance of that ST in the input data. The determined group founder is shown in light green, and common nodes are shown in blue.
Comparative goeBURST Analysis of L. pneumophila Environmental Isolates From India, China, Japan, and South Korea
Forty-four L. pneumophila isolates detected in the present study were compared with 403 Lp1 environmental isolates from Japan (n = 135), China (n = 164), and South Korea (n = 104). The SBT data of Chinese, Japanese, and South Korean isolates were obtained from previously published studies (6, 25, 26). Altogether, these 447 isolates were divided into 127 STs. Most of these STs exclusively belonged to one country, and the only ST that found to be present in all the four countries was ST1. Apart from ST1, ST1464 detected in this study was also reported from China; similarly, ST48 was also reported from Japan. The comparative goeBURST analysis grouped 67 STs into 16 clonal complexes (CCs), and 60 STs were identified as singletons (Figure 3). Among the 16 CCs, four clonal complexes (CC-A, CC-C, CC-F, and CC-I) also contained STs identified in this present study.
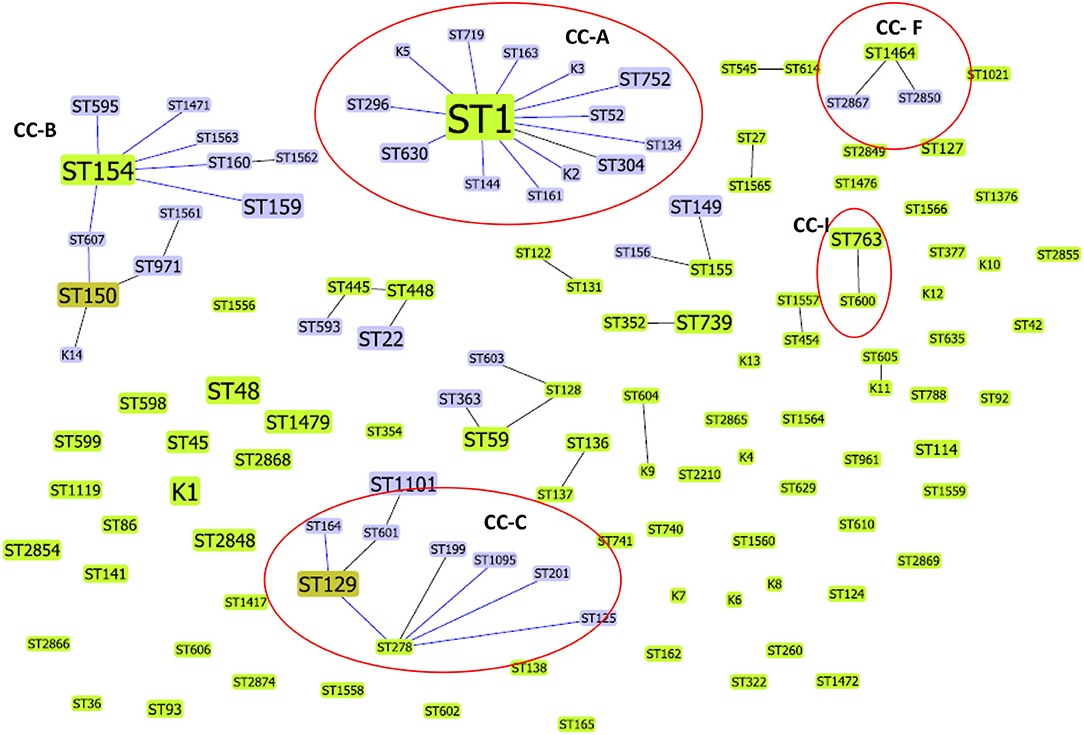
Figure 3. Comparative goeBURST analysis of L. pneumophila STs detected in this study with environmental L. pneumophila serogroup1 STs reported from China, Japan, and South Korea. Only single-locus variant (SLV) links are shown. The population snapshot contained 16 clonal complexes and 60 singletons. The group founders are shown in light green ST nodes, and dark green ST nodes represent the sub-group founders. Blue ST nodes are the common nodes. The clonal complexes CC-A, CC-C, CC-F, and CC-I (shown in circles) contained STs (ST1, ST134, ST763, ST1095, ST1464, ST2850, ST2867) identified in this study.
ST1 was the determined primary founder of the largest clonal complex CC-A; it had 13 SLVs, including ST134, which is detected in the present study. The CC-C with determined primary founder ST278 and subgroup founder ST129 contained ST1095, a ST identified in our study that shares 6/7 alleles with ST278 (ST reported from Japan). The CC-F, which is observed in the present study, contained ST1464 as a predicted primary founder and ST2867 and ST2850 as obtained SLVs. Another ST reported in this study, ST763 belonged to CC-I and is closely related to ST600 (a ST reported from Japan) (Figure 3).
Discussion
Environmental Surveillance
Drinking water colonization by Legionella spp. is directly linked to the occurrence of HALD, and several national public health agencies have mandated routine environmental surveillance as a preventive measure. In our study, L. pneumophila was isolated from 18% of hospital water samples, and these findings are in line with a previous study from India that reported a positivity of 15% (28). In studies reported from Spain and Italy, the occurrence of Legionella in the hospital environment has been found to be higher (60 and 74.1%, respectively) (29, 30). Surveys of Legionella colonization in hospitals have been performed in the USA, UK, Canada, and Spain, with Legionella positivity rates varied from 12 to 85% (31, 32). In a large-scale hospital survey conducted in Taiwan (belonging to East Asia), L. pneumophila contamination was found in the water systems of 10 out of 16 hospitals (33).
In the present study, during 19 instances, potable water tested positive for L. pneumophila, and it is reported that in hospital settings, potable water rather than cooling towers has been implicated as a potential source of legionellosis (7). One possible solution to prevent the spread of Legionella from the water was the application of filters to the taps, thus allowing safe water free of the pathogen (34). Therefore, as an initial infection control measure, point-of-use filters were applied to the drinking water taps from where Legionella was isolated. Repeat sampling from these sites did not show Legionella re-colonization after 6 and 12 months. However, to evaluate the efficacy of any Legionella-disinfection method, monitoring over a prolonged period is required. The cooling towers were cleaned and disinfected at least once every 12 months, and water was sampled and tested at least once every 3 months for Legionella spp. These sites were found to be positive for Legionella after 4 months, and the bacteria were isolated from cooling towers until they closed for the next cycle of annual maintenance. Legionella isolation rates reduced significantly in water systems from an average of 26.6% during Feb 2015- January 2017 to an average of 13.9% during February 2017-September 2018 (OR 0.45; 95% CI 0.20–0.97; p = 0.03). This reduction in Legionella isolation rates could be due to Legionella-specific interventions and control measures.
As a part of Legionella risk management, the physicians were informed regarding Legionella colonization in the hospital water systems, and intensive clinical surveillance for this pathogen was initiated. Furthermore, in the future, if a high-level Legionella colonization is observed in this facility, it is pertinent to install a systemic disinfection system with long term-commitment with a specific aim of preventing legionellosis in the exposed population.
Detection of Virulence Genes
The lvh and rtxA loci are seen frequently in L. pneumophila isolates associated with human infections. Therefore, these loci can be used as markers for determining the infection potential of isolates (10–12). Our results showed that these genetic loci are found at a very high percentage in L. pneumophila strains from hospital water systems. This finding is in agreement with studies from Greece, Australia, and China that reported a high percentage of the pathogenicity loci in L. pneumophila environmental isolates (11, 35, 36). Despite the high prevalence L. pneumophila containing virulence genes in our hospital environment, HALD clusters were not identified during the clinical surveillance. Similarly, even though Legionella colonization was observed in 24% of tap waters in Singapore, HALD cases were not identified during 1998–2002 (33, 37). However, in many health care facilities, HALD cases have been discovered after the implementation of Legionella environmental monitoring and clinical surveillance (7, 32). Our results indicate the presence of disease-causing L. pneumophila in the hospital environment; therefore, warrant the necessity of investigating Legionella among all patients having nosocomial pneumonia in this facility.
L. pneumophila Sequence-Based Typing
Over the last decade, SBT analysis has been accepted as a gold standard for the genotyping of L. pneumophila isolates. Besides, SBT can be applied to study the genetic diversity and clonal expansion of L. pneumophila populations. The method has the advantage of better classification potential, good reproducibility, and is more economical (4, 16–18). Our study represents SBT analysis of environmental L. pneumophila isolated from the water systems of a tertiary health care center in India, and the results depict the genetic diversity of this pathogen even though all isolates were derived from the human-made environment. The IOD of the 44 environmental isolates was found to be 0.929 that is higher compared to studies reported from Canada (IOD, 0.888), Japan (IOD, 0.886), and the USA (IOD, 0.751), but lower than those reported from Singapore (IOD, 0.970) (4, 25, 38, 39). We also found that non-Lp1 isolates (IOD, 0.955) are more variable than Lp1 isolates (IOD, 0.824), which could be due to the high prevalence of ST1 among the Lp1 isolates. Additionally, NPW isolates were found to have high IOD (IOD, 0.933) followed by PW (IOD, 0.920) and CT isolates (IOD, 0.888).
ST1, the most common ST distributed throughout the world, was the dominant profile in this study (4, 6, 25, 26, 39). Multiple outbreaks due to ST1 strains have been reported from the USA, Canada, and Europe (4, 39). ST1 isolates are well-adapted to survive in human-made water environments such as a cooling tower, and the ability of this ST to adapt to natural water environments, including hot springs and soil, is found to be low (25). From studies conducted in Japan and South Korea, it was observed that most of the Lp1 environmental isolates, especially those from CT, belonged to ST1 (25, 26). In contrast to these findings, in the present study, ST1 was the most dominant ST in Lp1 isolates from PW (42.8%), followed by those from NPW (33.3%) and CT (16.6%). In a Chinese study, ST1 accounted for 92.3 and 53.1% of the isolates from PW and CT, respectively (6).
Additionally, in a US study, it was reported that ST1 was the dominant ST in both PW and CT isolates (4). These differences could be possibly due to the predominance of specific STs or unique strains in various water systems types in these countries. Besides, the genes coding for proteins (e.g., flagellum, pilin, outer membrane protein, macrophage infectivity potentiator, Zinc metalloproteinase) may interact with external environments; therefore, an isolate to get adapt to an environmental source may have a particular ST suitable for each environment (40).
L. pneumophila serogroup 1 STs previously reported from Japan, China, and South Korea (countries belong to East Asia) were compared with STs obtained in this study. The results indicated that some STs (e.g., ST1) are distributed world-wide across different countries, but some clones and STs could be unique circulating only in specific regions. The possibility of recombination events between L. pneumophila STs has been previously reported (6, 25). ST161 (11,4,3,1,1,1,1) in CC-A was reported to be a recombinant between ST1 (1,4,3,1,1,1,1) and ST154 (11,14,16,16,15,13,2) the determined primary founders of CC-A and CC-B, respectively (Figure 3). Similarly ST2850 (2,10,3,28,19,4,3) which is identified in our study was a recombinant of ST1464 (2,10,15,28,19,4,3) and ST1 (1,4,3,1,1,1,1) the predicted primary founders of CC-1 and CC-2, respectively (Figure 2).
Querying the ESGLI SBT database (available at http://www.esgli.org), it was found that of the 23 STs we obtained in this study, 11 were new to the database, and among the remaining STs, 3 (ST1095, ST1464, ST2210) were unique to Asia. Notably, all three STs were reported to be isolated from environmental sources, and no clinical infections due to these STs have been documented so far (Table 5). It will be interesting to see whether these STs will be associated with any LD cases or outbreaks in the future. The goeBURST analysis has shown that of the 11 STs that are newly identified in this study, two STs have single-locus variants (SLVs), 5 STs have double-locus variants (DLVs), and 2 had triple-locus variants (TLV) within our STs. Besides, querying the ESGLI database, it was found that 7/11 STs are having SLVs abroad (Table 5). Further studies are needed to determine if these STs will persist in this geographical region or expand to other continents.
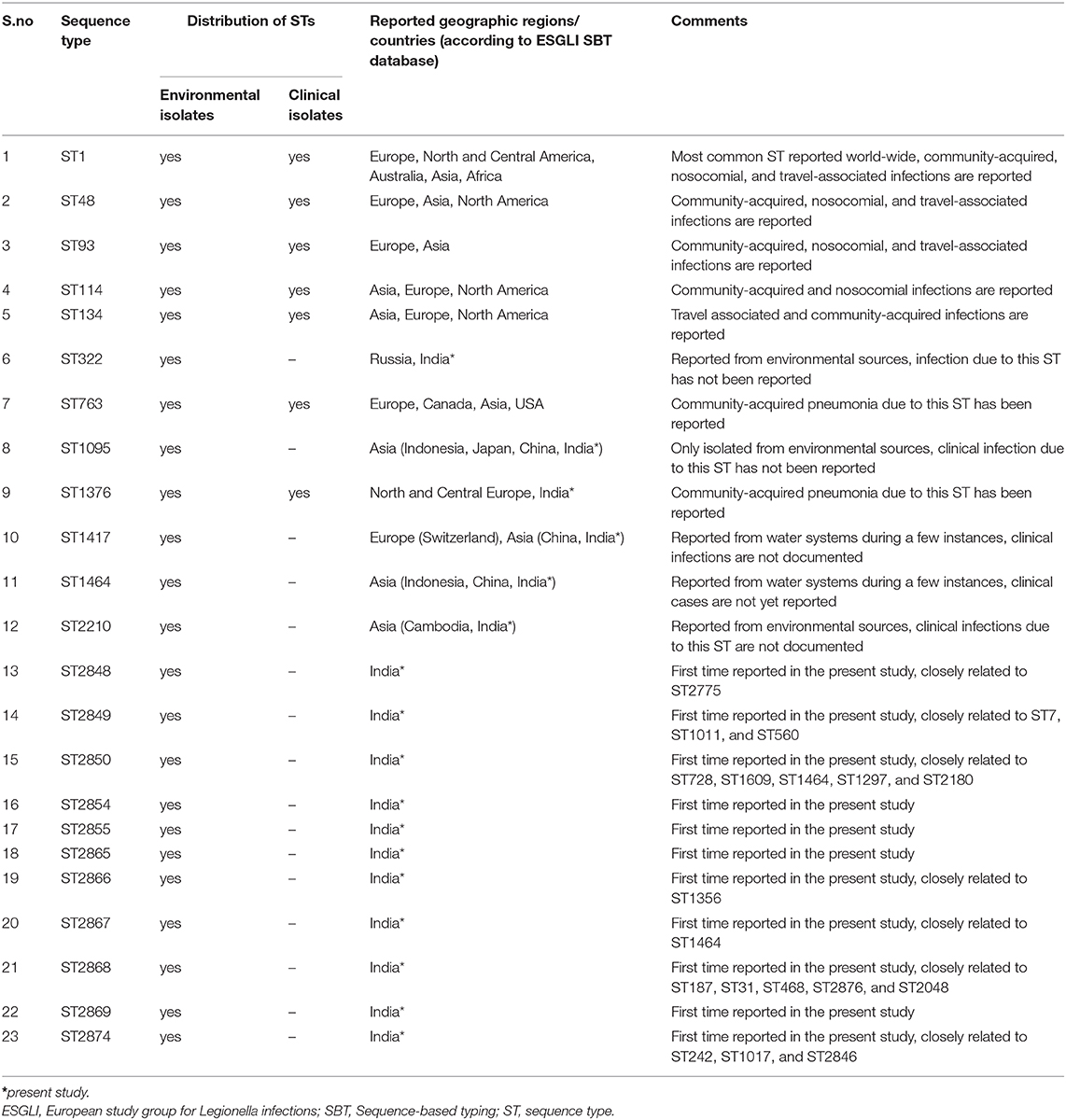
Table 5. The ESGLI database information regarding the L. pneumophila STs identified in this study (as of 11, January, 2020).
According to the ESGLI SBT database, few STs described in this study are found to be associated with LD cases and outbreaks. Clinical infections due to STs, including ST1, ST48, ST93, ST114, ST134, ST763, and ST1376, are reported in the ESGLI SBT database (Table 5). These STs that are common to clinical isolates may have an increased ability to cause LD; therefore, their water system sources represent a potential source of legionellosis. ST1 and ST134 (representing CC-2 in this study, Figure 2) were associated with multiple sporadic cases and outbreaks in many parts of the world (4, 39). Furthermore, it was hypothesized that similar to certain Legionella spp. and serogroups, few STs also have an enhanced ability to cause infections in humans (41). Hence Legionella control strategies can specifically target these STs that cause the majority of human infections.
During the study period, we could not obtain clinical isolates from patients for genotyping, but SBT was performed directly on the respiratory sample (BAL fluid) of an LD case-patient who was diagnosed by PCR and urine antigen testing (BinaxNOW, Alere, USA). Briefly, we used a nested PCR derived SBT directly to the DNA isolated from respiratory fluid and assigned allele, and finally, a sequence type (ST) using the online ESGLI SBT database (42). We identified that the infection was due to ST1, but was not able to perform epidemiological investigations to determine the possible source of the infection as the patient has acquired infection from another facility. Further studies are needed to analyze the correlation between L. pneumophila environmental and clinical isolates from this region.
Even though we described the distribution and classification of environmental L. pneumophila isolates in a healthcare facility, in future, large-scale studies using whole-genome sequencing (WGS) are needed to classify L. pneumophila environmental and clinical isolates and to identify factors that give fitness to this bacteria to survive in the aquatic environments and to infect humans.
Data Availability Statement
The original contributions presented in the study are publicly available. This data can be found here: GenBank MT890971.
Ethics Statement
The studies involving human participants were reviewed and approved by The ethics committee of AIIMS, New Delhi. The patients/participants provided their written informed consent to participate in this study.
Author Contributions
RC: conceptualization, project administration, resources, validation, writing-review, and editing. KS and RC: data curation. KS, RC, and BT: formal analysis. KS and RC: investigation and methodology. KS and EV: software. RC, SK, AD, RG: supervision. KS: writing-original draft. All authors contributed to the article and approved the submitted version.
Conflict of Interest
The authors declare that the research was conducted in the absence of any commercial or financial relationships that could be construed as a potential conflict of interest.
Acknowledgments
We thank Dr. Baharak Afshar, Public Health England, London for providing access to the ESGLI SBT database and for assigning the newly identified allele and sequence types. We acknowledge Dr. Arti Kapil, Dr. Bijay Ranjan Mirdha, Dr. Benu Dhawan, and Dr. Urvashi B Singh for their valuable input and suggestions. We also thank Dr. Arvind Valavane for helpful discussions and Dr. Achuthan C Raghavamenon for critical reading of the manuscript. KS acknowledges the Indian Council for Medical Research (ICMR) for providing Senior Research Fellowship (SRF) during the study period.
References
1. Fields BS, Benson RF, Besser RE. Legionella and legionnaires' disease: 25 years of investigation. Clin Microbiol Rev. (2002) 15:506–26. doi: 10.1128/CMR.15.3.506-526.2002
2. Centers for Disease Control and Prevention. Legionnaires' Disease Surveillance Summary Repor, 2014–2015 (2008).
3. European Centre for Disease Prevention and Control. Legionnaires' Disease in Europe, 2015. Stockholm: ECDC (2017).
4. Kozak-Muiznieks NA, Lucas CE, Brown E, Pondo T, Taylor TH, Frace M, et al. Prevalence of sequence types among clinical and environmental isolates of Legionella pneumophila serogroup 1 in the United States from 1982 to 2012. J Clin Microbiol. (2014) 52:201–11. doi: 10.1128/JCM.01973-13
5. Harb OS, Gao LY, Kwaik YA. From protozoa to mammalian cells: a new paradigm in the life cycle of intracellular bacterial pathogens. Environ Microbiol. (2000) 2:251–65. doi: 10.1046/j.1462-2920.2000.00112.x
6. Qin T, Zhou H, Ren H, Guan H, Li M, Zhu B, et al. Distribution of sequence-based types of Legionella pneumophila serogroup 1 strains isolated from cooling towers, hot springs, and potable water systems in China. Appl Environ Microbiol. (2014) 80:2150–7. doi: 10.1128/AEM.03844-13
7. Sabria M, Victor LY. Hospital-acquired legionellosis: solutions for a preventable infection. Lancet Infect Dis. (2002) 2:368–73. doi: 10.1016/S1473-3099(02)00291-8
8. Chaudhry R, Valavane A, Sreenath K, Choudhary M, Sagar T, Shende T, et al. Detection of Mycoplasma pneumoniae and Legionella pneumophila in patients having community-acquired pneumonia: a multicentric study from New Delhi, India. Am J Trop Med Hyg. (2017) 97:1710–6. doi: 10.4269/ajtmh.17-0249
9. Chaudhry R, Sreenath K, Agrawal SK, Valavane A. Legionella and legionnaires' disease: time to explore in India. Indian J Med Microbiol. (2018) 36:324. doi: 10.4103/ijmm.IJMM_18_298
10. Samrakandi MM, Cirillo SL, Ridenour DA, Bermudez LE, Cirillo JD. Genetic and phenotypic differences between Legionella pneumophila strains. J Clin Microbiol. (2002) 40:1352–62. doi: 10.1128/JCM.40.4.1352-1362.2002
11. Huang B, Heron BA, Gray BR, Eglezos S, Bates JR, Savill J. A predominant and virulent Legionella pneumophila serogroup 1 strain detected in isolates from patients and water in Queensland, Australia, by an amplified fragment length polymorphism protocol and virulence gene-based PCR assays. J Clin Microbiol. (2004) 42:4164–8. doi: 10.1128/JCM.42.9.4164-4168.2004
12. Huang B, Yuan Z, Heron BA, Gray BR, Eglezos S, Bates JR, et al. Distribution of 19 major virulence genes in Legionella pneumophila serogroup 1 isolates from patients and water in Queensland, Australia. J Med Microbiol. (2006) 55:993–7. doi: 10.1099/jmm.0.46310-0
13. Segal G, Russo JJ, Shuman HA. Relationships between a new type IV secretion system and the icm/dot virulence system of Legionella pneumophila. Mol Microbiol. (1999) 34:799–809. doi: 10.1046/j.1365-2958.1999.01642.x
14. D'Auria G, Jiménez N, Peris-Bondia F, Pelaz C, Latorre A, Moya A. Virulence factor RTX in Legionella pneumophila, evidence suggesting it is a modular multifunctional protein. BMC Genomics. (2008) 9:14. doi: 10.1186/1471-2164-9-14
15. Fry NK, Alexiou-Daniel S, Bangsborg JM, Bernander S, Pastoris MC, Etienne J, et al. A multicenter evaluation of genotypic methods for the epidemiologic typing of Legionella pneumophila serogroup 1: results of a pan-European study. Clin Microbiol Infect. (1999) 5:462–77. doi: 10.1111/j.1469-0691.1999.tb00176.x
16. Gaia V, Fry NK, Afshar B, Lück PC, Meugnier H, Etienne J, et al. Consensus sequence-based scheme for epidemiological typing of clinical and environmental isolates of Legionella pneumophila. J Clin Microbiol. (2005) 43:2047–52. doi: 10.1128/JCM.43.5.2047-2052.2005
17. Ratzow S, Gaia V, Helbig JH, Fry NK, Lück PC. Addition of neuA, the gene encoding N-acylneuraminate cytidylyl transferase, increases the discriminatory ability of the consensus sequence-based scheme for typing Legionella pneumophila serogroup 1 strains. J Clin Microbiol. (2007) 45:1965–8. doi: 10.1128/JCM.00261-07
18. Mentasti M, Fry NK. Sequence-Based Typing Protocol for Epidemiological Typing of Legionella pneumophila. ESCMID Study Group for Legionella Infections, European Society of Clinical Microbiology and Infectious Diseases, Basel, Switzerland (2012).
19. Centers for Disease Control and Prevention. Procedures for the Recovery of Legionella From the Environment. Atlanta: US Department of Health and Human Services, Public Health Service (2005). p. 1–13.
20. Chaudhry R, Sreenath K, Arvind V, Vinayaraj EV, Tanu S. Legionella pneumophila serogroup 1 in the water facilities of a tertiary healthcare center, India. Emerg Infect Dis. (2017) 23:1924–5. doi: 10.3201/eid2311.171071
21. Sreenath K, Chaudhry R, Vinayaraj EV, Thakur B. Antibiotic susceptibility of environmental Legionella pneumophila isolated in India. Future Microbiol. (2019) 14:661–9. doi: 10.2217/fmb-2019-0049
22. Benitez AJ, Winchell JM. Clinical application of a multiplex real-time PCR assay for simultaneous detection of legionella species, Legionella pneumophila, and Legionella pneumophila serogroup 1. J Clin Microbiol. (2013) 51:348–51. doi: 10.1128/JCM.02510-12
23. Mentasti M, Fry NK. Analysis of the N-acylneuraminate cytidyltransferase homologue (neuAh) found in some non-serogroup 1 strains of Legionella pneumophila, version 1.0. ESCMID Study Group for Legionella Infections, European Society of Clinical Microbiology and Infectious Diseases, Basel, Switzerland. (2012).
24. Feil EJ, Li BC, Aanensen DM, Hanage WP, Spratt BG. eBURST: inferring patterns of evolutionary descent among clusters of related bacterial genotypes from multilocus sequence typing data. J Bacteriol. (2004) 186:1518–30. doi: 10.1128/JB.186.5.1518-1530.2004
25. Amemura-Maekawa J, Kikukawa K, Helbig JH, Kaneko S, Suzuki-Hashimoto A, Furuhata K, et al. Distribution of monoclonal antibody subgroups and sequence-based types among Legionella pneumophila serogroup 1 isolates derived from cooling tower water, bathwater, and soil in Japan. Appl Environ Microbiol. (2012) 78:4263–70. doi: 10.1128/AEM.06869-11
26. Lee HK, Shim JI, Kim HE, Yu JY, Kang YH. Distribution of Legionella species from environmental water sources of public facilities and genetic diversity of L. pneumophila serogroup 1 in South Korea. Appl Environ Microbiol. (2010) 76:6547–54. doi: 10.1128/AEM.00422-10
27. Hunter PR, Gaston MA. Numerical index of the discriminatory ability of typing systems: an application of Simpson's index of diversity. J Clin Microbiol. (1988) 26:2465–6. doi: 10.1128/JCM.26.11.2465-2466.1988
28. Valavane A, Chaudhry R, Malhotra P. Multiplex polymerase chain reaction of genetic markers for detection of potentially pathogenic environmental Legionella pneumophila isolates. Indian J Med Res. (2017) 146:392. doi: 10.4103/ijmr.IJMR_623_16
29. Graells T, Hernández-García M, Pérez-Jové J, Guy L, Padilla E. Legionella pneumophila recurrently isolated in a Spanish hospital: two years of antimicrobial resistance surveillance. Environ Res. (2018) 166:638–46. doi: 10.1016/j.envres.2018.06.045
30. Laganà P, Facciolà A, Palermo R, Delia. S. Environmental surveillance of legionellosis within an Italian university hospital—results of 15 years of analysis. Int J Environ Res Public. Health. (2019) 16:1103. doi: 10.3390/ijerph16071103
31. Liu WK, Healing DE, Yeomans JT, Elliott TS. Monitoring of hospital water supplies for Legionella. J Hosp Infect. (1993) 24:1–9. doi: 10.1016/0195-6701(93)90084-D
32. Sabria M, Modol JM, Garcia-Nunez M, Reynaga E, Pedro-Botet ML, Sopena N, et al. Environmental cultures and hospital-acquired Legionnaires' disease: a 5-year prospective study in 20 hospitals in Catalonia, Spain. Infect Control Hosp Epidemiol. (2004) 25:1072–6. doi: 10.1017/S019594170007750X
33. Yu PY, Lin YE, Lin WR, Shih HY, Chuang YC, Ben RJ, et al. The high prevalence of Legionella pneumophila contamination in hospital potable water systems in Taiwan: implications for hospital infection control in Asia. Int. J Infect Dis. (2008) 12:416–20. doi: 10.1016/j.ijid.2007.11.008
34. Lin YE, Stout JE, Victor LY. Controlling Legionella in hospital drinking water: an evidence-based review of disinfection methods. Infect Control Hosp Epidemiol. (2011) 32:166–73. doi: 10.1086/657934
35. Katsiaflaka A, Pournaras S, Kristo I, Mouchtouri VA, Kyritsi M, Velonakis E, et al. Epidemiological investigation of Legionella pneumophila serogroup 2 to 14 isolates from water samples by amplified fragment length polymorphism and sequence-based typing and detection of virulence traits. Appl Environ Microbiol. (2016) 82:6102–8. doi: 10.1128/AEM.01672-16
36. Zeng LZ, Liao HY, Luo LZ, He SS, Tian QI, Zhou HJ, et al. An investigation on the molecular characteristics and intracellular growth ability among environmental and clinical isolates of Legionella pneumophila in Sichuan Province, China. Biomed Environ Sci. (2019) 32:520–30. doi: 10.3967/bes2019.069
37. Goh KT, Ng DL, Yap J, Ma S, Ooi EE. Surveillance, prevention, and control of legionellosis in a tropical city-state. Am J Infect Control. (2005) 33:286–91. doi: 10.1016/j.ajic.2004.12.001
38. Reimer AR, Au S, Schindle S, Bernard KA. Legionella pneumophila monoclonal antibody subgroups and DNA sequence types isolated in Canada between 1981 and 2009: laboratory component of national surveillance. Eur J Clin Microbiol Infect Dis. (2010) 29:191–205. doi: 10.1007/s10096-009-0840-3
39. Kozak NA, Benson RF, Brown E, Alexander NT, Taylor TH, Shelton BG, et al. Distribution of lag-1 alleles and sequence-based types among Legionella pneumophila serogroup 1 clinical and environmental isolates in the United States. J Clin Microbiol. (2009) 47:2525–35. doi: 10.1128/JCM.02410-08
40. Amemura-Maekawa J, Kura F, Chida K, Ohya H, Kanatani JI, Isobe J, et al. egionella pneumophila and other Legionella species isolated from legionellosis patients in Japan between 2008 and 2016. Appl Environ Microbiol. (2018) 84:e00721–18. doi: 10.1128/AEM.00721-18
41. Harrison TG, Fry NK, Afshar B, Bellamy W, Doshi N, Underwood AP. Typing of Legionella pneumophila and its role in elucidating the epidemiology of legionnaire's disease. In Cianciotto NP, Kwaik YA, Edelstein PH, Fields BS, Geary DF, Harrison TG, et al. editors. Legionella: State of the Art 30 Years After Its Recognition. Washington, DC: ASM Press. (2005). p. 94–99. doi: 10.1128/9781555815660.ch25
Keywords: Legionella, legionellosis, environmental surveillance, virulence, genotyping, sequence-based typing, molecular epidemiology
Citation: Sreenath K, Chaudhry R, Vinayaraj EV, Dey AB, Kabra SK, Thakur B and Guleria R (2020) Distribution of Virulence Genes and Sequence-Based Types Among Legionella pneumophila Isolated From the Water Systems of a Tertiary Care Hospital in India. Front. Public Health 8:596463. doi: 10.3389/fpubh.2020.596463
Received: 19 August 2020; Accepted: 12 October 2020;
Published: 23 November 2020.
Edited by:
Thandavarayan Ramamurthy, National Institute of Cholera and Enteric Diseases (ICMR), IndiaReviewed by:
Durg Vijai Singh, Institute of Life Sciences (ILS), IndiaM. Jahangir Alam, University of Houston, United States
Copyright © 2020 Sreenath, Chaudhry, Vinayaraj, Dey, Kabra, Thakur and Guleria. This is an open-access article distributed under the terms of the Creative Commons Attribution License (CC BY). The use, distribution or reproduction in other forums is permitted, provided the original author(s) and the copyright owner(s) are credited and that the original publication in this journal is cited, in accordance with accepted academic practice. No use, distribution or reproduction is permitted which does not comply with these terms.
*Correspondence: Rama Chaudhry, ZHJyYW1hY2gmI3gwMDA0MDtnbWFpbC5jb20=
†Present address: Bhaskar Thakur, Division of Biostatistics and Epidemiology, Texas Tech Health Science Center, EI Paso, TX, United States