- 1Division of Infectious Disease, Department of Pediatrics, School of Medicine, Stanford University, Stanford, CA, United States
- 2School of Public Health, University of California, Berkeley, Berkeley, CA, United States
- 3College of Public Health, Kent State University, Kent, OH, United States
- 4School of Earth Sciences, Stanford University, Stanford, CA, United States
- 5Environment and Health Sciences Department, Technical University of Mombasa, Mombasa, Kenya
Background: Infectious disease epidemiology and planetary health literature often cite solid waste and plastic pollution as risk factors for vector-borne diseases and urban zoonoses; however, no rigorous reviews of the risks to human health have been published since 1994. This paper aims to identify research gaps and outline potential solutions to interrupt the vicious cycle of solid wastes; disease vectors and reservoirs; infection and disease; and poverty.
Methods: We searched peer-reviewed publications from PubMed, Google Scholar, and Stanford Searchworks, and references from relevant articles using the search terms (“disease” OR “epidemiology”) AND (“plastic pollution,” “garbage,” and “trash,” “rubbish,” “refuse,” OR “solid waste”). Abstracts and reports from meetings were included only when they related directly to previously published work. Only articles published in English, Spanish, or Portuguese through 2018 were included, with a focus on post-1994, after the last comprehensive review was published. Cancer, diabetes, and food chain-specific articles were outside the scope and excluded. After completing the literature review, we further limited the literature to “urban zoonotic and biological vector-borne diseases” or to “zoonotic and biological vector-borne diseases of the urban environment.”
Results: Urban biological vector-borne diseases, especially Aedes-borne diseases, are associated with solid waste accumulation but vector preferences vary over season and region. Urban zoonosis, especially rodent and canine disease reservoirs, are associated with solid waste in urban settings, especially when garbage accumulates over time, creating burrowing sites and food for reservoirs. Although evidence suggests the link between plastic pollution/solid waste and human disease, measurements are not standardized, confounders are not rigorously controlled, and the quality of evidence varies. Here we propose a framework for solutions-based research in three areas: innovation, education, and policy.
Conclusions: Disease epidemics are increasing in scope and scale with urban populations growing, climate change providing newly suitable vector climates, and immunologically naïve populations becoming newly exposed. Sustainable solid waste management is crucial to prevention, specifically in urban environments that favor urban vectors such as Aedes species. We propose that next steps should include more robust epidemiological measurements and propose a framework for solutions-based research.
Introduction
Rationale
The world is in a solid waste and plastic predicament (1–15)—single use-plastic packaging is increasing in an urbanized (11, 16–20) and globalized economy in which production of food happens farther from the consumer and packaging enables consumption far from the source; yet, plastics lack a circular economy (21–23) that would incentivize responsible management (3, 24–28), resulting in large accumulations of solid waste, specifically plastics which do not biodegrade (29).
The most common approach to eliminating accumulated trash in low- and middle- income countries is open burning. For example, in sub-Saharan Africa, more than 75% of waste is openly burned and worldwide an estimated 600 million tons are openly burned annually (30). Open burning of trash is dangerous for human health (6, 31–33) and the planet, as burning releases toxins into the air that pollute the environment and increase greenhouse gases which contribute to climate change (30).
Policies are slowly catching up to reduce single-use plastic supply, but these policies are only one part of a complete solution (34–37) to single-use plastic production, demand, and disposal (29) and these policies often face poor enforcement, especially in LMICs (29).
At the same time, the risk of zoonosis has increased with urbanization (38) and immunologically naïve populations are newly at risk for vector-borne disease transmission due to changing geographies of suitable vector climates (39–41). Vector-borne diseases such as dengue—transmitted by container breeding Aedes spp.—threaten about half a billion people in densely populated areas (42). One very important mosquito vector, Aedes aegypti, which spreads dengue, Zika, chikungunya, and yellow fever, prefers to breed in man-made containers (43, 44), such as recyclable plastic containers, tires, and trash. The 2,050 projections of over 6 billion people living in urban areas (45) suggest an impending increase in the risk of infectious disease transmission.
Objectives
Trash accumulation has been cited as a risk factor for infectious disease (46–50). Recent viewpoints discuss the subject (51–53), but analytical reviews are outdated (54–57). Other reviews exclude key references on trash and disease risk (19, 58–60), while others focus on urbanization or poverty (18, 19, 57). Some reviews take a narrow scope and are pathogen-specific [for example, we identified reviews on trash and dengue virus (61–63), protozoans (64), and leishmania (65)] or vector-specific [arthropods (51)], limited to landfills and incineration (66), microplastic-specific (67), or waste-specific (68). However, the potential risk of direct transmission of infectious diseases by any kind of solid waste depends on a multitude of inter-related factors including, but not limited to, the presence of an infectious agent, its viability in solid waste, and a susceptible host.
A holistic approach is needed to define the link between vector-borne diseases, urban zoonosis, and solid waste. Here, our objective is to identify research gaps through a review of current evidence on solid waste accumulation, in association with urban zoonosis and biological vector-borne disease risk, and to propose solutions that can interrupt the vicious cycle of solid waste accumulation and human health risks due to infectious diseases.
Research Question
We hypothesize that plastic pollution, including unused plastic bottles, containers, and tires, is a major environmental health risk and promotes vector-borne diseases (VBD) such as dengue, chikungunya, Zika, malaria, and other vectors of disease (triatomine, houseflies) and zoonotic reservoirs (rodents and canines).
Methods
Search Strategy
We conducted a hypothesis-driven review from January to March 2019. Literature was identified by searches of PubMed, Google Scholar, and Stanford Searchworks, and references from relevant articles using the search terms (“disease” OR “epidemiology”) AND (“plastic pollution,” “garbage,” and “trash,” “rubbish,” “refuse,” OR “solid waste”). Abstracts and reports from meetings were included only when they related directly to previously published work. Only articles published in English, Spanish, or Portuguese (translated using https://www.deepl.com/translator) through 2018 were included, with a focus on post-1994 (the publication year of the last rigorous review on the topic). Cancer, diabetes, mechanical vectors, and food chain-specific articles were outside the scope of this review and excluded. The search was not constrained by geography. After completing the literature review, we further limited the literature to only biological vector-borne and zoonotic diseases (see general concepts defined in the Supplementary Material).
Synthesized findings: published literature on vector-borne diseases, urban zoonosis, and solid waste
One hundred and fifty three references were identified in the literature review, 73 of which discussed vector-borne and zoonotic diseases. We discuss vector-borne diseases and urban zoonosis in the context of solid waste and highlight major vectors, reservoirs, and diseases.
We identified 45 references related to vector-borne disease risk and solid waste. We categorized the results according to vector [Aedes species, Phlebotomus spp. (sand flies), triatominae, Anopheles species], pathogen (dengue, chikungunya, and Zika viruses, Leishmania, Trypanosoma cruzi, and Plasmodium) and type of evidence (case study, observational, intervention, policy, or review). We summarize the evidence in Table 1 and details are available in Supplementary Material 1.
We identified 16 references related to urban zoonosis and solid waste. We categorized the results according to vector (rodent and canine), pathogen (Orientia tsutsugamushi, Leptospira, Yersinia pestis, Toxoplasma gondii, and rabies virus) and type of evidence (case study, observational, intervention, policy, or review). We summarize the evidence in Table 2 and details are available in Supplementary Material 2.
Vector-Borne Diseases and Solid Waste
Vector-borne diseases, especially Aedes-borne diseases, are associated with solid waste accumulation in the urban environment, even small cups, and wrappers, but vector preferences vary over season and region. Other vectors are associated with trash as a burrow, source of food, and breeding site.
Aedes Species
Aedes species mosquitoes prefer to breed in man-made plastic containers (43, 44) and transmit dengue (DENV), Zika (ZIKV), and chikungunya (CHIKV) viruses. Aedes albopictus is reported to preferentially breed in solid waste (91), and tires (92), open coconut shells (92, 93) and small plastic containers (92, 93). Aedes aegypti prefers to breed in discarded tires (95, 98) and artificial water containers (95); plastic containers (96), solid waste (96, 98), buckets (97), drums (97), tires (97), pots (97), and garbage dumps (98). Both Aedes albopictus and Aedes aegypti breed in plastic teacups (100, 101), plastic containers (79–82, 102, 103, 128), tires (79, 82, 101), trash (96, 101), bottles (103), and cans (103). However, these associations change seasonally and regionally. During transmission season, Aedes prefers solid waste (96) in Delhi, India. During the rainy season in Brazil, Aedes prefers tires (92), open coconut shells and small plastic containers (92). In India, breeding preference ratio was highest for tires and container breeding during pre-monsoon (79). Human DENV transmission was strongly associated with irregular garbage collection during low transmission periods/interepidemic intervals (44).
At the household-level, the evidence shows an increase of dengue risk with the presence of cans, plastic containers, tires (70), a lack of consistent garbage collection (44, 71, 73, 74, 77), and with garbage accumulation (75).
CHIKV and ZIKV have also been associated with garbage accumulation in ecological models (88, 89). However, ecological models can be subject to biases and residual confounding (76). In a case study, Krystosik, Curtis (129) used spatial video and Google Street View in Cali, Colombia to create sub-neighborhood risk surfaces compared with routinely reported clinical cases of dengue, chikungunya, and Zika. Ministry of Health officials and Community Health Workers perceived proximity to unplanned urbanizations without solid waste management as a risk factor for dengue, chikungunya and Zika hotspots. Lack of sanitation can be systematic, for example, 80–90% of housing on Reunion Island was built by squatters resulting in the absence of adequate drainage systems for sewage and rainwater and the lack of properly organized garbage disposal and providing breeding grounds for vector-borne diseases, especially CHIKV (90).
Conversely, removing trash and stagnant water from around the residence is protective (78, 84–87, 94, 99), especially when the government acts with intention and the community is consistently mobilized (85, 86). However, results depend on the local ecology of vector breeding (83, 87).
Other Vectors
Other vectors use trash as a burrow, source of food, and breeding site. To prevent tick-borne diseases, The US Centers for Disease Control recommends removing old furniture, mattresses, or trash that may give ticks a place to hide (49); however, no other evidence of an association between trash and tick-borne disease was found. Abbasi et al. (111) identified 33 species of arthropods from a Municipal Solid Waste landfill in Urmia, Iran, including medically important species: Periplaneta americana Linnaeus (Blattodea: Blattidae) and Shelfordella lateralis Walker (Blattodea: Ectobiidae). Ahmad et al. (112) report that malaria was associated with low rates of solid waste collection system use. However, this association was based on geospatial analysis that did not control for potential confounders. Others report that Anopheles stephensi also breeds in manufactured containers (130, 131).
Community members in rural India report that visceral leishmaniasis-transmitting sand flies breed in trash (105). In two studies, the risk of visceral leishmaniasis increased in the absence of regular trash collection (104, 106).
In Yucatan Peninsula, Mexico, residents report triatomines, the vectors of Trypanosoma cruzi, burrow in accumulated trash, cardboard, and rocks (110). Strong entomological (109, 110) and clinical (107, 108) evidence supports this local perception. Dumonteil et al. (109) conducted entomological surveillance for one year in 38 randomly selected houses and created crude and adjusted models in which they observed a strong association between the practice of cleaning of trash from the peridomicile and house infestation by non-domiciliated Triatoma dimidiate (109). Fortunately, similar to Aedes interventions, environmental cleanup is associated with decreased risk of triatomine infestation (110). Clinical evidence also supports these findings. Trypanosoma cruzi infection seroprevalence in Venezuela was associated with the increase of accumulated garbage (108) and household disarray (measured as old and/or damaged artifacts accumulated, materials from construction, inadequate cleaning and free rubbish in the home) (107). Bonfante-Cabarcas et al. (107) speculate that accumulated garbage favors breeding of T. cruzi reservoirs (rats, mice, and opossum) and provides long-term refuge with immediate food sources for insects to reproduce and colonize the house for a long time, increasing the probability of intra-domiciliary vector transmission of T. cruzi.
Urban Zoonosis Associated With Solid Waste
Urban zoonoses, specifically those transmitted by rodent and canine reservoirs, are associated with solid waste, especially when garbage accumulates over time creating burrowing sites and food for reservoirs.
In a review of neglected tropical diseases and their impact on global health and development (50), Hotez states of zoonoses: “Of relevance to the NTDs, the poorest favelas do not benefit from regular garbage collection or sewage treatment, thereby creating excellent niches for rats and stray dogs.” Rodents and canines directly transmit disease of importance to urban zoonosis (123, 125, 132). Solid waste accumulation is an important factor for urban rodent and canine feeding and sheltering strategies (126) and can be used as a proxy in the absence of reliable data on rodent distribution in the city (117, 126). Presence of rubbish increased risk of scrub typhus (120); Toxoplasma infection in owners and their domiciled dogs was associated with dirty yards (126); and the bubonic plague has historically been associated with solid waste (121, 122).
For example, Kassir et al. (127) conducted an observational study to investigate the risk of rabies and the neighboring Syrian war and the local garbage crisis, finding both were concomitant with a notable increase in the number of dog bites and thus possible rabies exposure. The evidence lies in a time-series of data from the Lebanese Ministry of Public Health (LMOPH) Epidemiological Surveillance Unit public database from 2005 to 2016. A sharp increase in reported animal bites was reported post-2013 (1,004 ± 272 vs. 355 ± 145 bites per year). The authors explain:
“The accumulation of wastes in dumpsites led to the declaration of a severe problem in July 2015, and these open garbage dump sites have been previously shown to contribute to the rise in the number of stray dogs which amplifies the number of possible vectors. Garbage dumps are breeding areas of stray dogs, and if they are no longer around, dogs will migrate to other places. This is reflected by the peak in the stray to domestic dog ratio in October 2015, after heaps of garbage had been covering the Lebanese streets for several months. October, in fact, witnesses the beginning of the rain season in Lebanon, and the rainfall in the presence of open garbage dumps leads to the formation of leachate, a polluting by-product of organic matter. This poses both social and environmental problems such as nuisance, diseases and the spread of stray dogs and other harmful animals. This rise in stray dogs increases the possibility both of new vectors as well as new bites. It is noteworthy that this predominance of stray dog bites was only observed in October 2015, while it was not present in either 2013 or 2014. This further strengthens the correlation between the garbage crisis, a special circumstance of October 2015, and the increase in stray dog bites” (127).
Leptospirosis is associated with dogs (123, 125), accumulated refuse (114), garbage (113, 123) and open containers and debris in the peri-domestic area (123, 125). For example, leptospirosis emergence in Marseille, France is linked to garbage collection strikes that contribute to the expansion of the rat population (113). Among slum residents from Salvador, Brazil, residence <20 m from accumulated refuse was associated with increased odds of previous Leptospira infection (114). Residents of another urban slum in Salvador identified improving trash collection as necessary to control leptospirosis in their community and reported current payment for private trash collection service to avoid trash accumulation in their community or a willingness to pay for this service. Residents reported removing trash on a daily basis but identified that trash cans are >50 m from their homes (118). Leptospira interrogans and L. icterohaemorrhagiae are pathogens of severe diseases that may cluster in urban areas where trash accumulates (123) but are also found in rural households in peri-domestic open containers (debris found around the household areas including buckets, pails, jars, barrels, and old tires) (123). Evidence shows leptospirosis infection clusters at the household level (48). During a leptospirosis outbreak in Western Rio de Janeiro, Brazil, cases were associated with lower access to solid waste collection, measured as a percentage of households served by municipal solid waste collection (116), and waste accumulation was used as an indicator of probable rat presence (117).
Conversely, in Federal District Brazil, leptospirosis infection was negatively associated with population access to public services: sewage network, treated water network, and public garbage collection services (115); and in Salvador, Brazil, there was no association between leptospirosis infection and peri-domiciliary trash accumulation (119).
Framework for Solutions-Based Research
Here we propose a framework for solutions-based-research in three areas: innovation, education, and policy.
Lessons Learned From Previously Proposed Frameworks
Efforts to promote circular economies in plastics are gaining international attention (29, 133, 134). The United Nations Environment Programme published ‘Single-Use Plastics: A Roadmap for Sustainability, 2018 (29). However, it noted policies and regulations have recently been established and lack monitoring and accountability and suffer from poor implementation. Hawken discusses the short and long term costs and benefits to multiple solutions to Reverse Global Warming (133). However, the solutions require significant investment from business and government to change without a focus on upstream education and innovation. Precious Plastics (134) focuses on the community engagement aspects of reusing plastics but fails to integrate with upstream policy. Examples of successful recycling exist in the metals industry (135–137)—aluminum (135, 136), and steel (137) are recycled and traded as commodities globally.
Perhaps the most common framework is “re-use, reduce, and recycle.” Reusing and recycling receive ample attention given the technology involved, yet trends in the recycling industry are changing: China is no longer accepting foreign trash for recycling (138). Reusing is also challenging as few types of plastics are highly coveted and reusable. The poorer quality plastics are simply trash—unable to be reused or recycled. Therefore, while reusing/recycling/introducing plastic alternatives all have their place, reducing the consumption and sale of single-use plastics is key. Therefore, we are adapting the previously touted framework, emphasizing reduction, and encouraging a circular economy for re-use and recycle.
Building on previous frameworks (29, 133–137), we propose a framework (Figure 1) to reduce vector-borne disease risk and urban zoonoses from exposure to solid waste. Given the importance of intervening at the interface of solid-waste and disease-vectors-and-reservoirs, the framework creates a knowledge-to-action plan using policy and innovative plastic alternatives to decrease the upstream plastic supply, education and art to decrease the downstream global demand for plastic, and innovation to generate profitable uses for currently produced and consumed single-use plastics. The desired result is an action plan to create a circular economy of trash and reduce the supply and demand of single-use plastics and to cultivate empowered, educated, and healthy communities that resist trash accumulation to improve health via reduced vector-borne diseases and improved air quality. The expected impact relates to the critical need to understand how the complex system that generates and discards so much trash might be tweaked, so that less trash is produced or trash is put back into either the economic or ecological cycle. As current options are insufficient, we propose solution-oriented research to either better adapt these options or to create whole new options for plastics disposal, recycling, and reuse and discover possibilities for a future without disposable plastics through policy, education, and innovation. The evidence is summarized in Figure 1 and details are available in Supplementary Material 3.
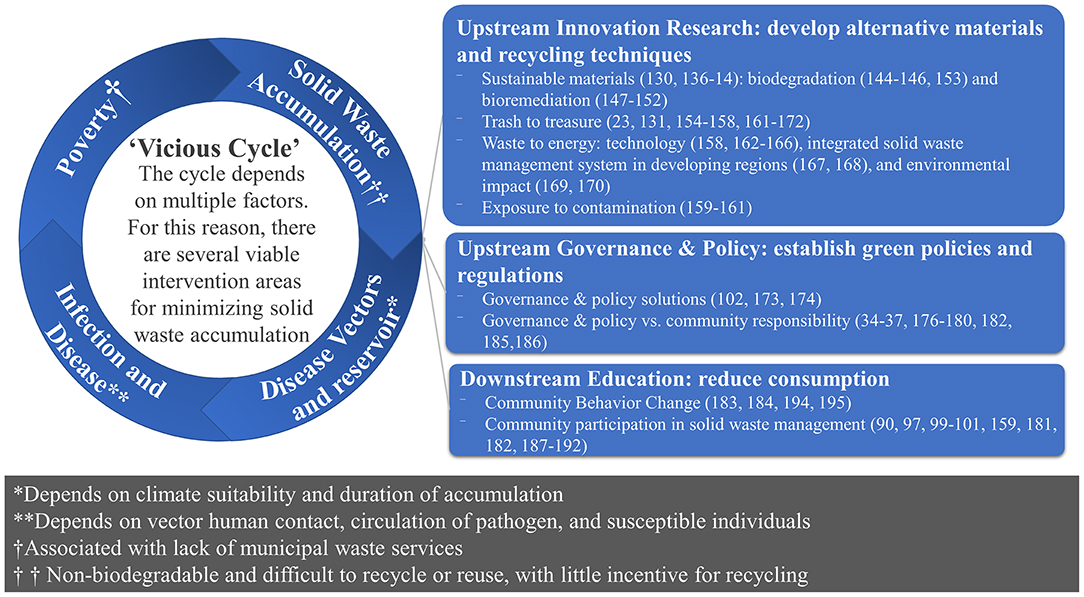
Figure 1. Framework for solutions-based research: upstream innovation research, upstream governance and policy, and downstream education. Recognizing that the current practices and incentive structures that drive these practices are inadequate to address the volume of trash generated globally, different approaches are needed to manage the growing waste problem. Notes for the symbols in the circle. Disease vectors and reservoirs *Depends on climate suitability and duration of accumulation; Infection and Disease **Depends on vector human contact, circulation of pathogen, and susceptible individuals; Poverty is †Associated with lack of municipal waste services; Solid waste accumulation refers to †† Non-biodegradable materials which are not incentivized for recycling.
Upstream Innovation Research
Profitable upstream innovation research can decrease supply and improve the processing of solid wastes in an increasingly urbanized and market-based world. Immediate barriers are cost and scalability.
In his 2017 best-seller, Drawdown (133), Hawken discusses the possibility of converting up to 90% of current fossil-fuel based plastic production to bio-based production. However, he warns that the solution must include proper separation and processing to fulfill the goal of sustainable material. Innovation in this field is currently working to drop the price below that of current fossil-fuel-based production. According to a special report commission by the European Polysaccharide Network of Excellence and European Bioplastics, 90% of current plastics could be derived from plants (139). Zhang et al. (140) analyze sustainable materials, defined as a class of materials that are derived from renewable feedstocks and exhibit closed-loop life cycles including aliphatic polyesters and polycarbonates. They also discuss recent advancements that lower the technological barriers for developing more sustainable replacements for petroleum-based plastics including biopolymers (141–143) and agro polymers (144–146).
Two aspects of sustainable materials to consider are biodegradation (147–149) and bioremediation (150–155). Narancic and O'Connor (150–152, 156). We found bioremediation—whereby animals and bacteria can break down plastics into biodegradable products—to be particularly interesting. Narancic and O'Connor (156) review the advances and possibilities in the biotransformation and biodegradation of oil-based plastics, including bio-based and biodegradable polymers, end-of-life management of biodegradables, and a circular economy to reduce plastic waste pollution. New fungi species are biodegrading polyester polyurethane: Pestalotiopsis species (150) and Aspergillus tubingensis (151). Ideonella sakaiensis bacteria break PET (Polyethylene terephthalate) into terephthalic acid and ethylene glycol in 2 weeks (152). Mealworm larvae can digest Styrofoam in <24 h with no cost to survival over 1 month, converting 47.7% of the ingested Styrofoam into CO2 and biodegradable residue (153, 154). Wax moth Galleria mellonella caterpillars can biodegrade polyethylene bags (155). These methods are especially attractive as they require no behavior change and are sustainable and, in some cases, beneficial to the species performing the biodegradation. Yet, these pilot studies need to be studied at scale and adapted to local context to understand feasibility.
Repurposing trash for profit seems like a viable market-based solution (134, 157–161) but does carry some risk of exposure to contamination (162–164) for entrepreneurs and end-users depending on the type of materials and the processes used and this risk should be taken into consideration early in the process. One popular use case is waste-to-energy analyzes waste-to-energy strategies and concludes that for a net implementation cost of $36 billion, a net operational savings of $19.82 billion and 1.1 gigatons of CO2 reduction could be gained. For example, Sweden currently converts 50% of household wastes to energy (161). Yet, Haken warns that this is only a transitional strategy, citing emissions of heavy metals and toxic compounds, even in state-of-the-art facilities. Several reviews discuss waste-to-energy regarding technological options and challenges (165–169), integrated solid waste management in developing countries (170, 171), and the environmental impact (172, 173).
These innovations must come equipped with a knowledge-to-action plan and pilots of these small-scale or theoretical solutions and engagement of external stakeholders such as existing companies, policymakers, and community groups.
Upstream Policy
Policymakers are uniquely positioned to make political and normative changes relatively quickly but struggle with enforcement, sustainability subject to elected officials, and community buy-in.
Policymakers are uniquely positioned to prevent and solve public health crises, in collaboration with public health officials and communities (84, 174–176). For example, Chen et al. (84) reported that discarded containers account for 25.4% of Aedes vector breeding sites in endemic regions of Taiwan pre-intervention. In 1988, the Waste Disposal Act was amended to make manufacturers, importers, and distributors responsible for the proper recovery, treatment, and recycling of packaging and containers which become an environmental menace. Non-compliance resulted in business suspension. A waste recycling system was established, and a breeding site reduction campaign was promoted for waste management. The authors reported a 98% decrease in dengue incidence reported to the Department of Health from 1988 to 1993. Several countries in Africa continue to implement bans to curb single-use plastic bags which clog drains, sewage systems, or hold rainwater, create breeding grounds for vectors (34).
Experts call for more policy solutions (177–179) and there is evidence that policy agendas can be influenced by popular norms (34, 180). Others argue that informal associations such as waste-picker cooperatives (35, 36, 181, 182) should be strengthened to improve solid waste systems. However, enforcement of such policies may be difficult, especially for nations with challenging processes or non-existent systems (37), and others call for a more community-based approach to increase participation in sustainable waste management (183–185). Businesses that use disposable packaging can also be engaged through social pressure and responsibility to adopt sustainable corporate practices (186, 187) and recoup disposable packaging for recycling.
Downstream Education to Decrease Demand
Community-based education and communication have the potential to change norms and create sustainable change but require greater initial investments to tailor and iterate community-based approaches.
Eagle et al. (188) argue that social marketing principles (183, 189, 190) paired with education (75, 85–87, 182, 183, 189–191) and policy (section upstream policy) can intervene to change behavior to positively impact plastic pollution using a transdisciplinary approach to identify barriers to and enablers of sustained behavior change.
Creating awareness about the crisis and health and environmental risks surrounding plastic pollution will not immediately decrease supply, but information may increase social pressure and responsibility to adopt sustainable practices at household (75, 85, 183, 192), community (75, 86, 87, 99, 183, 191, 193–196), and corporate levels (186, 187) that may decrease demand in the future (see details in Supplementary Material 3). For example, Sommerfeld et al. (87) summarize a 5-year research and capacity-building initiative conducted in South Asia and South-East Asia. The initiative developed community-based interventions aimed at reducing dengue vector breeding and viral transmission. Where small discarded containers presented the main problem, groups experimented with solid waste management, composting and recycling schemes. Many intervention tools were locally produced, and all tools were implemented through community partnership strategies. All sites developed socially- and culturally-appropriate health education materials. The study also mobilized and empowered women, students, and community groups and at several sites organized new volunteer groups for environmental health.
Tana et al. (86) built an innovative community-centered dengue-ecosystem management intervention in Yogyakarta city, Indonesia and assessed the process and results. The intervention results included: better community knowledge, attitude, and practices in dengue prevention; increased household and community participation; improved partnership including a variety of stakeholders with prospects for sustainability; vector control efforts refocused on environmental and health issues; increased community ownership of dengue vector management including broader community development activities such as solid waste management and recycling. Tana et al. (86) note, the community-centered approach needs a lot of effort at the beginning but has better prospects for sustainability than the vertical “top-down” approach.
Discussion
Summary of Main Findings
Although evidence suggests the link between plastic pollution/solid waste and human disease, measurements are not standardized, confounders are not rigorously controlled, and the quality of evidence varies.
Here we have reviewed the available evidence for solid waste accumulation impact on biological vector-borne diseases. We hypothesized that plastic pollution, including unused plastic bottles, containers, plastic bags, and tires, is a major environmental health risk and promotes vector-borne diseases (VBD) such as dengue, chikungunya, Zika, malaria, and other VBD transmission. We conclude that solid waste accumulation is a risk factor for zoonotic and vector-borne disease transmission. However, measurements are not standardized, (107, 123, 197) and confounders are not rigorously controlled (106, 112, 123, 197, 198).
In the context of vicious cycles of solid waste accumulation, poor health, and poverty, policymakers use estimates of disease transmission, burden, and risk to inform the allocation of limited public health resources; thus, it is imperative epidemiological estimates control for known confounders and employ standardized measurement constructs (Table 3). Additionally, if surveillance data are used, hybrid surveillance (199, 200) should be employed to correct for known surveillance biases. A framework for solutions-based research is also critical to guide research priorities.
Of note, the landscape of single-use plastics innovations and policy is developing rapidly. For example, Christensen et al. described in April 2019 a next-generation plastic to incentivize recycling in closed-loop life cycles (201, 202). This new plastic can be disassembled and reassembled without loss of performance or quality, even in mixed waste streams (201). And the political trend is gaining momentum—in May 2019, 187 countries agreed to add plastics to the Basel Convention, a treaty that regulates the movement of hazardous materials from one country to another (202).
Limitations
We only included published literature and abstracts in English, Spanish, and Portuguese. We did not have access to primary data and relied on the interpretation of the publishing authors.
Multiple studies included relied on surveillance data which did not correct for selection bias. Multiple studies included did not control for variables possibly associated with both exposure (trash) and outcome (disease), for example, socio-economic status (SES), access to health care, or climate. The data needed to understand the context-specific risk factors are not yet available; particularly, the authors noted a paucity of data from sub-Saharan Africa, where policies and regulations have recently been established (29). Interestingly, although geography was not constrained in the review, most studies identified were from low- and middle-income tropical countries.
After completing our search, we constrained the scope to only urban zoonosis associated with wild mammals and domesticated animals of non-agricultural interest such as dogs and cats. This may exclude some important research related to geographical areas where cows or other domestics animals can serve as crucial reservoirs of important etiological agents.
Conclusions
Despite gaps in the research base—lack of standardized measures and residual confounding—it is clear solid wastes breed vector-borne diseases and urban zoonoses.
Future populations are at increased risk—disease epidemics are increasing in scope and scale (42) with urban populations growing (38, 45), climate change providing newly suitable vector climates (39–41), and naïve populations becoming newly at risk, sustainable solid waste management is crucial to prevention, specifically in urban environments that favor urban vectors such as Aedes species and in poor urban and rural populations which lack access to municipal solid waste services.
We propose a framework for solutions-based research which includes upstream innovation research, upstream policy, and downstream education to decrease demand for single-use plastics.
Author Contributions
JF, AK, FM, and AL conceived of the initial idea and secured funding. AK drafted the initial manuscript. AK and GN conducted the literature search. LO and AL provided editing on intellectual content. All authors contributed to manuscript revision, read, and approved the submitted version.
Funding
This work was funded in part by NIH R01 (AI102918, PI: AL), BOVA network (PIs: FM and AL), Bechtel Faculty Scholar Award in Pediatric Translational Medicine (PI: AL), and Stanford Maternal and Child Health Research Institute Postdoctoral Scholar support (PI: AK).
Conflict of Interest
The authors declare that the research was conducted in the absence of any commercial or financial relationships that could be construed as a potential conflict of interest.
Acknowledgments
We gratefully acknowledge the assistance of the Stanford School of Medicine Lane Librarian in constructing initial literature search terms.
Supplementary Material
The Supplementary Material for this article can be found online at: https://www.frontiersin.org/articles/10.3389/fpubh.2019.00405/full#supplementary-material
References
1. Hoornweg D, Bhada-Tata P. What a Waste—A Global Review of Solid Waste Management. Washington, DC: The World Bank (2012).
2. Kaza S, Yao LC, Bhada-Tata P, Van Woerden F. What a Waste 2.0 : A Global Snapshot of Solid Waste Management to 2050. Washington, DC: World Bank (2018). doi: 10.1596/978-1-4648-1329-0
3. Heacock M, Kelly CB, Suk WA. E-waste: the growing global problem and next steps. Rev Environ Health. (2016) 31:131–5. doi: 10.1515/reveh-2015-0045
4. Rhodes CJ. Plastic pollution and potential solutions. Sci Progr. (2018) 101:207–60. doi: 10.3184/003685018X15294876706211
5. Schmidt C, Krauth T, Wagner S. Export of plastic debris by rivers into the sea. Environ Sci Technol. (2017) 51:12246–53. doi: 10.1021/acs.est.7b02368
6. Ziraba AK, Haregu TN, Mberu B. A review and framework for understanding the potential impact of poor solid waste management on health in developing countries. Arch Public Health. (2016) 74:55. doi: 10.1186/s13690-016-0166-4
7. Taylor D. Talking trash: the economic and environmental issues of landfills. Environ Health Perspect. (1999) 107:A404–9. doi: 10.1289/ehp.99107a404
8. Dangi MB, Pretz CR, Urynowicz MA, Gerow KG, Reddy JM. Municipal solid waste generation in Kathmandu, Nepal. J Environ Manage. (2011) 92:240–9. doi: 10.1016/j.jenvman.2010.09.005
9. Suthar S, Singh P. Household solid waste generation and composition in different family size and socio-economic groups: a case study. Sustain Cities Soc. (2015) 14:56–63. doi: 10.1016/j.scs.2014.07.004
10. Ogwueleka TC. Survey of household waste composition and quantities in Abuja, Nigeria. Resour Conserv Recy. (2013) 77:52–60. doi: 10.1016/j.resconrec.2013.05.011
11. Boadi KO, Kuitunen M. Environment, wealth, inequality and the burden of disease in the Accra metropolitan area, Ghana. Int J Environ Health Res. (2005) 15:193–206. doi: 10.1080/09603120500105935
12. Qu XY, Li ZS, Xie XY, Sui YM, Yang L, Chen Y. Survey of composition and generation rate of household wastes in Beijing, China. Waste Manag. (2009) 29:2618–24. doi: 10.1016/j.wasman.2009.05.014
13. Boer E, Jedrczak A, Kowalski Z, Kulczycka J, Szpadt R. A review of municipal solid waste composition and quantities in Poland. Waste Manag. (2010) 30:369–77. doi: 10.1016/j.wasman.2009.09.018
14. Adeniran AE, Nubi AT, Adelopo AO. Solid waste generation and characterization in the University of Lagos for a sustainable waste management. Waste Manag. (2017) 67:3–10. doi: 10.1016/j.wasman.2017.05.002
15. Ostle C, Thompson RC, Broughton D, Gregory L, Wootton M, Johns DG. The rise in ocean plastics evidenced from a 60-year time series. Nat Commun. (2019) 10:1622. doi: 10.1038/s41467-019-09506-1
16. Tauil PL. Urbanization and dengue ecology. Cad Saude Publica. (2001) 17 (Suppl.):99–102. doi: 10.1590/S0102-311X2001000700018
17. LaDeau SL, Allan BF, Leisnham PT, Levy MZ. The ecological foundations of transmission potential and vector-borne disease in urban landscapes. Funct Ecol. (2015) 29:889–901. doi: 10.1111/1365-2435.12487
18. Moore M, Gould P, Keary BS. Global urbanization and impact on health. Int J Hyg Environ Health. (2003) 206:269–78. doi: 10.1078/1438-4639-00223
19. Alirol E, Getaz L, Stoll B, Chappuis F, Loutan L. Urbanisation and infectious diseases in a globalised world. Lancet Infect Dis. (2011) 11:131–41. doi: 10.1016/S1473-3099(10)70223-1
20. Puri A, Kumar M, Johal E. Solid-waste management in Jalandhar city and its impact on community health. Indian J Occup Environ Med. (2008) 12:76–81. doi: 10.4103/0019-5278.43265
21. Indrianti N. Community-based solid waste bank model for sustainable education. Proc Soc Behav Sci. (2016) 224:158–66. doi: 10.1016/j.sbspro.2016.05.431
22. Lohri CR, Camenzind EJ, Zurbrugg C. Financial sustainability in municipal solid waste management–costs and revenues in Bahir Dar, Ethiopia. Waste Manag. (2014) 34:542–52. doi: 10.1016/j.wasman.2013.10.014
23. Public Radio International. The Plastic Bank Turns Plastic Waste into Money for the Poor. Waste360. New York, NY: Informa (2018).
24. Kinnaman TC. The economics of municipal solid waste management. Waste Manag. (2009) 29:2615–7. doi: 10.1016/j.wasman.2009.06.031
25. Magalini F. Global challenges for e-waste management: the societal implications. Rev Environ Health. (2016) 31:137–40. doi: 10.1515/reveh-2015-0035
26. Oguntoyinbo OO. Informal waste management system in Nigeria and barriers to an inclusive modern waste management system: a review. Public Health. (2012) 126:441–7. doi: 10.1016/j.puhe.2012.01.030
27. Ezeah C, Roberts CL. Analysis of barriers and success factors affecting the adoption of sustainable management of municipal solid waste in Nigeria. J Environ Manage. (2012) 103:9–14. doi: 10.1016/j.jenvman.2012.02.027
28. Zhou Z, Tang Y, Dong J, Chi Y, Ni M, Li N, et al. Environmental performance evolution of municipal solid waste management by life cycle assessment in Hangzhou, China. J Environ Manage. (2018) 227:23–33. doi: 10.1016/j.jenvman.2018.08.083
29. United Nations Environment Programme. Single-Use Plastics: A Roadmap for Sustainability. Geneva: United Nations Environment Programme (2018).
30. Cogut A. Open Burning of Waste: A Global Health Disaster. R20 Regions of Climate Action. Geneva: R20 (2016).
31. Verma R, Vinoda KS, Papireddy M, Gowda ANS. Toxic pollutants from plastic waste—a review. Proc Environ Sci. (2016) 35:701–8. doi: 10.1016/j.proenv.2016.07.069
32. Akpinar-Elci M, Coomansingh K, Blando J, Mark L. Household bush burning practice and related respiratory symptoms in Grenada, the Caribbean. J Air Waste Manage Assoc. (2015) 65:1148–52. doi: 10.1080/10962247.2015.1070773
33. Franchini M, Rial M, Buiatti E, Bianchi F. Health effects of exposure to waste incinerator emissions:a review of epidemiological studies. Ann Ist Super Sanita. (2004) 40:101–15. Available online at: http://old.iss.it/publ/anna/2004/1/doi.pdf
34. Clapp J, Swanston L. Doing away with plastic shopping bags: international patterns of norm emergence and policy implementation. Environ Polit. (2009) 18:315–32. doi: 10.1080/09644010902823717
35. Tirado-Soto MM, Zamberlan FL. Networks of recyclable material waste-picker's cooperatives: an alternative for the solid waste management in the city of Rio de Janeiro. Waste Manag. (2013) 33:1004–12. doi: 10.1016/j.wasman.2012.09.025
36. Ferri GL, Chaves Gde L, Ribeiro GM. Reverse logistics network for municipal solid waste management: the inclusion of waste pickers as a Brazilian legal requirement. Waste Manag. (2015) 40:173–91. doi: 10.1016/j.wasman.2015.02.036
37. Ezeah C, Roberts CL. Waste governance agenda in Nigerian cities: a comparative analysis. Habitat Int. (2014) 41:121–8. doi: 10.1016/j.habitatint.2013.07.007
38. Hassell JM, Begon M, Ward MJ, Fèvre EM. Urbanization and disease emergence: dynamics at the wildlife-livestock-human interface. Trends Ecol Evol. (2017) 32:55–67. doi: 10.1016/j.tree.2016.09.012
39. Mordecai EA, Cohen JM, Evans V, Gudapati P, Johnson LR, Lippi CA, et al. Detecting the impact of temperature on transmission of Zika, Dengue, and Chikungunya using mechanistic models. PLoS Negl Trop Dis. (2017) 11:e0005568. doi: 10.1371/journal.pntd.0005568
40. Huber JH, Childs ML, Caldwell JM, Mordecai EA. Seasonal temperature variation influences climate suitability for dengue, chikungunya, and Zika transmission. PLoS Negl Trop Dis. (2018) 12:e0006451. doi: 10.1371/journal.pntd.0006451
41. Ali S, Gugliemini O, Harber S, Harrison A, Houle L, Ivory J, et al. Environmental and social change drive the explosive emergence of Zika virus in the Americas. PLoS Negl Trop Dis. (2017) 11:e0005135. doi: 10.1371/journal.pntd.0005135
42. Eder M, Cortes F, Teixeira de Siqueira Filha N, Vinícius Araújo de França G, Degroote S, Braga C, Ridde V, et al. Scoping review on vector-borne diseases in urban areas: transmission dynamics, vectorial capacity and co-infection. Infect Dis Povert. (2018) 7:90. doi: 10.1186/s40249-018-0475-7
43. Ramos M, Mohammed H, Zielinski-Gutierrez E, Hayden MH, Lopez JL, Fournier M, et al. Epidemic dengue and dengue hemorrhagic fever at the Texas-Mexico border: results of a household-based Seroepidemiologic survey, December 2005. Am J Trop Med Hyg. (2008) 78:364–9. doi: 10.4269/ajtmh.2008.78.364
44. MacCormack-Gelles B, Lima Neto AS, Sousa GS, Nascimento OJ, Machado MMT, Wilson ME, et al. Epidemiological characteristics and determinants of dengue transmission during epidemic and non-epidemic years in Fortaleza, Brazil: 2011–2015. PLoS Negl Trop Dis. (2018) 12:e0006990. doi: 10.1371/journal.pntd.0006990
45. UN. 68% of the world population projected to live in urban areas by 2050, says UN | UN DESA | United Nations Department of Economic and Social Affairs. 2018-05-16. Geneva: United Nations Department of Economic and Social Affairs (2018)
46. Smiley SL, Curtis A, Kiwango JP. Using spatial video to analyze and map the water-fetching path in challenging environments: a case study of Dar es Salaam, Tanzania. Trop Med Infect Dis. (2017) 2:8. doi: 10.3390/tropicalmed2020008
47. Curtis A, Blackburn JK, Widmer JM, Morris JG Jr. A ubiquitous method for street scale spatial data collection and analysis in challenging urban environments: mapping health risks using spatial video in Haiti. Int J Health Geograph. (2013) 12:21. doi: 10.1186/1476-072X-12-21
48. Maciel EA, de Carvalho AL, Nascimento SF, de Matos RB, Gouveia EL, Reis MG, et al. Household transmission of leptospira infection in urban slum communities. PLoS Negl Trop Dis. (2008) 2:e154. doi: 10.1371/journal.pntd.0000154
49. CDC. Preventing ticks in the yard. (2019). Available online at: https://www.cdc.gov/ticks/avoid/in_the_yard.html (accessed February 14, 2019).
50. Hotez PJ. Forgotten People, Forgotten Diseases : the Neglected Tropical Diseases and Their Impact on Global Health and Development. Washington, DC: ASM Press (2013). doi: 10.1128/9781555818753
51. Lizzi KM, Qualls WA, Brown SC, Beier JC. Expanding integrated vector management to promote healthy environments. Trends Parasitol. (2014) 30:394–400. doi: 10.1016/j.pt.2014.06.001
52. Vethaak AD, Leslie HA. Plastic debris is a human health issue. Environ Sci Technol. (2016) 50:6825–6. doi: 10.1021/acs.est.6b02569
53. Hupert N. Who's invading whom? Zika and intergenerational public health. Soc Res. (2017) 84:83–105.
54. Knudsen AB, Slooff R. Vector-borne disease problems in rapid urbanization: new approaches to vector control. Bull World Health Organ. (1992) 70:1–6.
55. Ault SK. Environmental management: a re-emerging vector control strategy. Am J Trop Med Hyg. (1994) 50(Suppl. 6):35–49. doi: 10.4269/ajtmh.1994.50.35
56. Lee YS. Urban planning and vector control in Southeast Asian cities. Gaoxiong Yi Xue Ke Xue Za Zhi. (1994) 10 (Suppl.):S39–51.
57. Lines J, Harpham T, Leake C, Schofield C. Trends, priorities and policy directions in the control of vector-borne diseases in urban environments. Health Policy Plan. (1994) 9:113–29. doi: 10.1093/heapol/9.2.113
58. Stettler A. Changes in prevention of infection: a historic retrospect. Ther Umsch. (1991) 48:205–9.
59. Alam P, Ahmade K. Impact of solid waste on health and the environment. Int J Sustain Dev Green Econ. (2013) 2:165–8. Available online at: https://pdfs.semanticscholar.org/2ae9/675a58adb025fb799703750cd477ca838bab.pdf
60. World Health Organization. Inheriting a Sustainable World? Atlas on Children's Health and the Environment. Geneva, Switzerland: World Health Organization (2017).
61. Gubler DJ. Dengue, urbanization and globalization: the unholy trinity of the 21(st) century. Trop Med Health. (2011) 39 (Suppl. 4):3–11. doi: 10.2149/tmh.2011-S05
62. Kay BH. Intersectoral approaches to dengue vector control. Gaoxiong Yi Xue Ke Xue Za Zhi. (1994) 10 (Suppl.):S56–61.
63. Chang AY, Fuller DO, Carrasquillo O, Beier JC. Social justice, climate change, and dengue. Health Hum Rights. (2014) 16:93–104. Available online at: www.jstor.org/stable/healhumarigh.16.1.93
64. Graczyk TK, Knight R, Tamang L. Mechanical transmission of human protozoan parasites by insects. Clin Microbiol Rev. (2005) 18:128–32. doi: 10.1128/CMR.18.1.128-132.2005
65. Alvar J, Yactayo S, Bern C. Leishmaniasis and poverty. Trends Parasitol. (2006) 22:552–7. doi: 10.1016/j.pt.2006.09.004
66. Mattiello A, Chiodini P, Bianco E, Forgione N, Flammia I, Gallo C, et al. Health effects associated with the disposal of solid waste in landfills and incinerators in populations living in surrounding areas: a systematic review. Int J Public Health. (2013) 58:725–35. doi: 10.1007/s00038-013-0496-8
67. Karbalaei S, Hanachi P, Walker TR, Cole M. Occurrence, sources, human health impacts and mitigation of microplastic pollution. Environ Sci Poll Res Int. (2018) 25:36046–63. doi: 10.1007/s11356-018-3508-7
68. Hossain MS, Santhanam A, Norulaini NAN, Omar AKM. Clinical solid waste management practices and its impact on human health and environment–a review. Waste Manag. (2011) 31:754–66. doi: 10.1016/j.wasman.2010.11.008
70. Hayes JM, Garcia-Rivera E, Flores-Reyna R, Suárez-Rangel G, Rodríguez-Mata T, Coto-Portillo R, et al. Risk factors for infection during a severe dengue outbreak in El Salvador in 2000. Am J Trop Med Hyg. (2003) 69:629–33. doi: 10.4269/ajtmh.2003.69.629
71. Brunkard JM, Robles López JL, Ramirez J, Cifuentes E, Rothenberg SJ, Hunsperger EA, et al. Dengue fever seroprevalence and risk factors, Texas-Mexico border, 2004. Emerg Infect Dis. (2007) 13:1477–83. doi: 10.3201/eid1310.061586
72. Perez-Guerra CL, Zielinski-Gutierrez E, Vargas-Torres D, Clark GG. Community beliefs and practices about dengue in Puerto Rico. Revis Panam Salud Publica. (2009) 25:218–26. doi: 10.1590/S1020-49892009000300005
73. Heukelbach J, de Oliveira FA, Kerr-Pontes LR, Feldmeier H. Risk factors associated with an outbreak of dengue fever in a favela in Fortaleza, north-east Brazil. Trop Med Int Health. (2001) 6:635–42. doi: 10.1046/j.1365-3156.2001.00762.x
74. Kenneson A, Beltran-Ayala E, Borbor-Cordova MJ, Polhemus ME, Ryan SJ, Endy TP, et al. Social-ecological factors and preventive actions decrease the risk of dengue infection at the household-level: results from a prospective dengue surveillance study in Machala, Ecuador. PLoS Negl Trop Dis. (2017) 11:e0006150. doi: 10.1371/journal.pntd.0006150
75. Suwannapong N, Tipayamongkholgul M, Bhumiratana A, Boonshuyar C, Howteerakul N, Poolthin S. Effect of community participation on household environment to mitigate dengue transmission in Thailand. Trop Biomed. (2014) 31:149–58.
76. Lippi CA, Stewart-Ibarra AM, Munoz AG, Borbor-Cordova MJ, Mejía R, Rivero K, et al. The social and spatial ecology of dengue presence and burden during an outbreak in Guayaquil, Ecuador, 2012. Int J Environ Res Public Health. (2018) 15:E827. doi: 10.3390/ijerph15040827
77. Cordeiro R, Donalisio MR, Andrade VR, Mafra ACN, Nucci LB, Brown JC, et al. Spatial distribution of the risk of dengue fever in southeast Brazil, 2006–2007. BMC Public Health. (2011) 11:355. doi: 10.1186/1471-2458-11-355
78. Chen B, Yang J, Luo L, Yang Z, Liu Q. Who is vulnerable to dengue fever? a community survey of the 2014 outbreak in Guangzhou, China. Int J Environ Res Public Health. (2016) 13:712. doi: 10.3390/ijerph13070712
79. Vijayakumar K, Sudheesh Kumar TK, Nujum ZT, Umarul F, Kuriakose A. A study on container breeding mosquitoes with special reference to Aedes (Stegomyia) aegypti and Aedes albopictus in Thiruvananthapuram district, India. J Vector Borne Dis. (2014) 51:27–32.
80. Rohani A, Aidil Azahary AR, Malinda M, Zurainee MN, Rozilawati H, Wan Najdah WM, et al. Eco-virological survey of Aedes mosquito larvae in selected dengue outbreak areas in Malaysia. J Vector Borne Dis. (2014) 51:327–32.
81. Boornema AR, Senthil Murugan TK. Breeding habitats of Aedes aegypti mosquitoes and awareness about prevention of dengue in urban Chidambaram: a cross sectional study. Int J Commun Med Public Health. (2018) 5:10. doi: 10.18203/2394-6040.ijcmph20184014
82. Kusumawathie PHD, Fernando WP. Breeding habitats of Aedes aegypti Linnaeus and Ae. albopictus Skuse in a dengue transmission area in Kandy, Sri Lanka. Ceylon J Med Sci. (2003) 46:51–9. doi: 10.4038/cjms.v46i2.4829
83. Focks DA, Brenner RJ, Chadee DD, Trosper JH. The use of spatial analysis in the control and risk assessment of vector-borne diseases. Am Entomol. (1999) 45: 173–83. doi: 10.1093/ae/45.3.173
84. Chen YR, Hwang JS, Guo YJ. Ecology and control of dengue vector mosquitoes in Taiwan. Gaoxiong Yi Xue Ke Xue Za Zhi. (1994) 10 (Suppl.):S78–87.
85. Abeyewickreme W, Wickremasinghe AR, Karunatilake K, Sommerfeld J, Axel K. Community mobilization and household level waste management for dengue vector control in Gampaha district of Sri Lanka; an intervention study. Pathog Glob Health. (2012) 106:479–87. doi: 10.1179/2047773212Y.0000000060
86. Tana S, Umniyati S, Petzold M, Kroeger A, Sommerfeld J. Building and analyzing an innovative community-centered dengue-ecosystem management intervention in Yogyakarta, Indonesia. Pathog Glob Health. (2012) 106:469–78. doi: 10.1179/2047773212Y.0000000062
87. Sommerfeld J, Kroeger A. Eco-bio-social research on dengue in Asia: a multicountry study on ecosystem and community-based approaches for the control of dengue vectors in urban and peri-urban Asia. Pathog Glob Health. (2012) 106:428–35. doi: 10.1179/2047773212Y.0000000055
88. Campos MC, Dombrowski JG, Phelan J, Marinho CRF, Hibberd M, Clark TG, et al. Zika might not be acting alone: using an ecological study approach to investigate potential co-acting risk factors for an unusual pattern of microcephaly in Brazil. PLoS ONE. (2018) 13:e0201452. doi: 10.1371/journal.pone.0201452
89. Aguiar BS, Lorenz C, Virginio F, Suesdek L, Chiaravalloti-Neto F. Potential risks of Zika and chikungunya outbreaks in Brazil: a modeling study. Int J Infect Dis. (2018) 70:20–9. doi: 10.1016/j.ijid.2018.02.007
90. Aoustin T. Chikungunya and urban sprawl on Reunion Island. Med Trop. (2012) 72:51–59. Available online at: https://www.jle.com/fr/MedSanteTrop/2012/72.1/051-059%20Chikungunya%20et%20habitat%20informel%20(Aoustin).pdf
91. Abramides GC, Roiz D, Guitart R, Quintana S, Gimenez N. Control of the Asian tiger mosquito (Aedes albopictus) in a firmly established area in Spain: risk factors and people's involvement. Trans Royal Soc Trop Med Hygiene. (2013) 107:706–14. doi: 10.1093/trstmh/trt093
92. Alencar CHM. Infestation by Aedes albopictus (skuse) in natural and artificial breeding sites found in green areas in the city of Fortaleza, Ceará. J Venom Anim Toxin Includ Trop Dis. (2009) 15:582. doi: 10.1590/S1678-91992009000300018
93. Rao BB, George B. Breeding patterns of Aedes stegomyia albopictus in periurban areas of Calicut, Kerala, India. Southeast Asian J Trop Med Public Health. (2010) 41:536–40.
94. Fonseca DM, Unlu I, Crepeau T, Farajollahi A, Healy SP, Bartlett-Healy K, et al. Area-wide management of Aedes albopictus. Part 2: gauging the efficacy of traditional integrated pest control measures against urban container mosquitoes. Pest Manag Sci. (2013) 69:1351–61. doi: 10.1002/ps.3511
95. Getachew D, Tekie H, Gebre-Michael T, Balkew M, Mesfin A. Breeding sites of Aedes aegypti: potential dengue vectors in Dire Dawa, East Ethiopia. Interdiscip Perspect Infect Dis. (2015) 2015:706276. doi: 10.1155/2015/706276
96. Vikram K, Nagpal BN, Pande V, Srivastava A, Gupta SK, Anushrita, et al. Comparison of Ae. aegypti breeding in localities of different socio-economic groups of Delhi, India. Int J Mosquito Res. (2015) 2:83–8.
97. Ngugi HN, Mutuku FM, Ndenga BA, Musunzaji PS, Mbakaya JO, Aswani P, et al. Characterization and productivity profiles of Aedes aegypti (L.) breeding habitats across rural and urban landscapes in western and coastal Kenya. Parasit Vectors. (2017) 10:331. doi: 10.1186/s13071-017-2271-9
98. Espinosa M, Weinberg D, Rotela CH, Polop F, Abril M, Scavuzzo CM. Temporal dynamics and spatial patterns of Aedes aegypti breeding sites, in the context of a dengue control program in Tartagal (Salta Province, Argentina). PLoS Negl Trop Dis. (2016) 10:e0004621. doi: 10.1371/journal.pntd.0004621
99. Raju AK. Community mobilization in Aedes aegypti. control programme by source reduction in peri-urban district of Lautoka, Viti Levu, Fiji Islands. Dengue Bull. (2003) 27:149–155. Available online at: https://apps.who.int/iris/bitstream/handle/10665/163791/dbv27p149.pdf;sequence=1
100. Hiriyan J, Tewari SC, Tyagi BK. Aedes albopictus (Skuse) breeding in plastic cups around tea-vendor spots in Ernakulam City, Kerala State, India. Dengue Bull. (2003) 27:195–6.
101. Jain J, Kushwah RBS, Singh SS, Sharma A, Adak T, Singh OP, et al. Evidence for natural vertical transmission of chikungunya viruses in field populations of Aedes aegypti in Delhi and Haryana states in India-a preliminary report. Acta Trop. (2016) 162:46–55. doi: 10.1016/j.actatropica.2016.06.004
102. Banerjee S, Aditya G, Saha GK. Household disposables as breeding habitats of dengue vectors: linking wastes and public health. Waste Manag. (2013) 33:233–9. doi: 10.1016/j.wasman.2012.09.013
103. Chena CD, Leeb HL, Stella-Wonga SP, Laua KW, Sofian-Aziruna M. Container survey of mosquito breeding sites in a university campus in Kuala Lumpur, Malaysia. Dengue Bull. (2009) 33:187–93.
104. Werneck GL, Rodrigues L, Santos MV, Araújo IB, Moura LS, Moreira S, et al. Household structure and urban services: neglected targets in the control of visceral leishmaniasis. Ann Trop Med Parasitol. (2005) 99:229–36. doi: 10.1179/136485905X28018
105. Singh SP, Reddy DC, Mishra RN, Sundar S. Knowledge, attitude, and practices related to Kala-azar in a rural area of Bihar state, India. Am J Trop Med Hyg. (2006) 75:505–8. doi: 10.4269/ajtmh.2006.75.505
106. Lima ID, Lima ALM, Mendes-Aguiar CO, Coutinho JFV, Wilson ME, Pearson RD, et al. Changing demographics of visceral leishmaniasis in northeast Brazil: lessons for the future. PLoS Negl Trop Dis. (2018) 12:e0006164. doi: 10.1371/journal.pntd.0006164
107. Bonfante-Cabarcas R, Rodríguez-Bonfante C, Oviol Vielma B, García D, Saldivia AM, Aldana E, et al. Seroprevalence for Trypanosoma cruzi infection and associated factors in an endemic area of Venezuela. Cad Saude Publica. (2011) 27:1917–29. doi: 10.1590/s0102-311x2011001000005
108. Garcia-Jordan N, Berrizbeitia M, Rodriguez J, Concepcion JL, Caceres A, Quinones W. Seroprevalence of Trypanosoma cruzi infection in the rural population of Sucre State, Venezuela. Cad Saude Publica. (2017) 33:e00050216. doi: 10.1590/0102-311x00050216
109. Dumonteil E, Nouvellet P, Rosecrans K, Ramirez-Sierra MJ, Gamboa-León R, Cruz-Chan V, et al. Eco-bio-social determinants for house infestation by non-domiciliated Triatoma dimidiata in the Yucatan Peninsula, Mexico. PLoS Negl Trop Dis. (2013) 7:e2466. doi: 10.1371/journal.pntd.0002466
110. Rosecrans K, Cruz-Martin G, King A, Dumonteil E. Opportunities for improved chagas disease vector control based on knowledge, attitudes and practices of communities in the yucatan peninsula, Mexico. PLoS Negl Trop Dis. (2014) 8:e2763. doi: 10.1371/journal.pntd.0002763
111. Abbasi E, Rafinejad J, Hosseinpoor S, Gholami-Borujeni F, Gholizadeh S. Diversity of arthropods in municipal solid waste landfill of Urmia, Iran. J Med Entomol. (2019) 56:268–70. doi: 10.1093/jme/tjy187
112. Ahmad SS, Aziz N, Butt A, Shabbir R, Erum S. Spatio-temporal surveillance of water based infectious disease (malaria) in Rawalpindi, Pakistan using geostatistical modeling techniques. Environ Monitor Assess. (2015) 187:555. doi: 10.1007/s10661-015-4779-9
113. Socolovschi C, Angelakis E, Renvoise A, Fournier PE, Marié JL, Davoust B, et al. Strikes, flooding, rats, and leptospirosis in Marseille, France. Int J Infect Dis. (2011) 15:e710–5. doi: 10.1016/j.ijid.2011.05.017
114. Reis RB, Ribeiro GS, Felzemburgh RD, Santana FS, Mohr S, Melendez AXTO, et al. Impact of environment and social gradient on Leptospira infection in urban slums. PLoS Negl Trop Dis. (2008) 2:e228. doi: 10.1371/journal.pntd.0000228
115. Santos IOC, Landi MFA, Cruz LM, Bofill MIR, Santos DED, Lima EMM, et al. Human leptospirosis in the Federal District, Brazil, 2011–2015: eco-epidemiological characterization. Rev Soc Bras Med Trop. (2017) 50:777–82. doi: 10.1590/0037-8682-0234-2017
116. Barcellos C, Sabroza PC. Socio-environmental determinants of the leptospirosis outbreak of 1996 in western Rio de Janeiro: a geographical approach. Int J Environ Health Res. (2000) 10:301–13. doi: 10.1080/0960312002001500
117. Barcellos C, Sabroza PC. The place behind the case: leptospirosis risks and associated environmental conditions in a flood-related outbreak in Rio de Janeiro. Cad Saude Publica. (2001) 17 (Suppl.):59–67. doi: 10.1590/S0102-311X2001000700014
118. Navegantes de Araujo W, Finkmoore B, Ribeiro GS, Reis RB, Felzemburgh RD, Hagan JE, et al. Knowledge, attitudes, and practices related to Leptospirosis among urban slum residents in Brazil. Am J Trop Med Hyg. (2013) 88:359–63. doi: 10.4269/ajtmh.2012.12-0245
119. Sarkar U, Nascimento SF, Barbosa R, Martins R, Nuevo H, Kalofonos I, et al. Population-based case-control investigation of risk factors for leptospirosis during an urban epidemic. Am J Trop Med Hyg. (2002) 66:605–10. doi: 10.4269/ajtmh.2002.66.605
120. Vallee J, Thaojaikong T, Moore CE, Phetsouvanh R, Richards AL, Souris M, et al. Contrasting spatial distribution and risk factors for past infection with scrub typhus and murine typhus in Vientiane City, Lao PDR. PLoS Negl Trop Dis. (2010) 4:e909. doi: 10.1371/journal.pntd.0000909
121. Milke M. Plague in Sydney and its solid waste lessons. Waste Manag. (2004) 24:321–3. doi: 10.1016/j.wasman.2004.02.006
122. Boisier P, Rahalison L, Rasolomaharo M, Ratsitorahina M, Mahafaly M, Razafimahefa M, et al. Epidemiologic features of four successive annual outbreaks of bubonic plague in Mahajanga, Madagascar. Emerg Infect Dis. (2002) 8:311–6. doi: 10.3201/eid0803.010250
123. Munoz-Zanzi C, Mason MR, Encina C, Astroza A, Romero A. Leptospira contamination in household and environmental water in rural communities in southern Chile. Int J Environ Res Public Health. (2014) 11:6666–80. doi: 10.3390/ijerph110706666
124. Ganoza CA, Matthias MA, Collins-Richards D, Brouwer KC, Cunningham CB, Segura ER, et al. Determining risk for severe leptospirosis by molecular analysis of environmental surface waters for pathogenic Leptospira. PLoS Med. (2006) 3:e308. doi: 10.1371/journal.pmed.0030308
125. Caldas EM, Sampaio MB. Leptospirosis in the city of Salvador, Bahia, Brazil: a case-control seroepidemiologic study. Int J Zoonoses. (1979) 6:85–96.
126. Benitez ADN, Martins FDC, Mareze M, Santos NJR, Ferreira FP, Martins CM, et al. Spatial and simultaneous representative seroprevalence of anti-Toxoplasma gondii antibodies in owners and their domiciled dogs in a major city of southern Brazil. PLoS ONE. (2017) 12:e0180906. doi: 10.1371/journal.pone.0180906
127. Kassir MF, El Zarif T, Kassir G, Berry A, Musharrafieh U, Bizri AR. Human rabies control in Lebanon: a call for action. Epidemiol Infect. (2018):1–8. doi: 10.1017/S095026881800300X
128. Banerjee S, Aditya G, Saha GK. Household wastes as larval habitats of dengue vectors: comparison between urban and rural areas of Kolkata, India. PLoS ONE. (2015) 10:e0138082. doi: 10.1371/journal.pone.0138082
129. Krystosik AR, Curtis A, Buritica P, Ajayakumar J, Squires R, Dávalos D, et al. Community context and sub-neighborhood scale detail to explain dengue, chikungunya and Zika patterns in Cali, Colombia. PLoS ONE. (2017) 12:e0181208. doi: 10.1371/journal.pone.0181208
130. Gayan Dharmasiri AG, Perera AY, Harishchandra J, Herath H, Aravindan K, Jayasooriya HTR, et al. First record of Anopheles stephensi in Sri Lanka: a potential challenge for prevention of malaria reintroduction. Malaria J. (2017) 16:326. doi: 10.1186/s12936-017-1977-7
131. Thomas S, Ravishankaran S, Justin JA, Asokan A, Mathai MT, Valecha N, et al. Overhead tank is the potential breeding habitat of Anopheles stephensi in an urban transmission setting of Chennai, India. Malar J. (2016) 15:274. doi: 10.1186/s12936-016-1321-7
132. CDC. Diseases Directly Transmitted by Rodents. (2017). Available online at: https://www.cdc.gov/rodents/diseases/ (accessed February 28, 2019).
133. Hawken P. Drawdown—The Most Comprehensive Plan Ever Proposed to Reverse Global Warming. New York, NY: Penguin Books (2017).
134. Hakkens D. Precious Plastics. (2019). Available online at: https://preciousplastic.com/ (accessed February 1, 2019).
136. Das SK, Yin W. The worldwide aluminum economy: the current state of the industry. JOM. (2007) 59:57–63. doi: 10.1007/s11837-007-0142-0
137. Pauliuk S, Wang T, Müller DB. Moving toward the circular economy: the role of stocks in the Chinese steel cycle. Environ Sci Technol. (2012) 46:148–54. doi: 10.1021/es201904c
139. Shen L, Haufe J, Patel MK. Product overview and market Projection of emerging bio-based plastics copernicus institute for sustainable development and innovation, Utrecht University, The Netherlands: European polysaccharide network of excellence. Eur Bioplast. (2009) 1–243.
140. Zhang X, Fevre M, Jones GO, Waymouth RM. Catalysis as an enabling science for sustainable polymers. Chem Rev. (2018) 118:839–85. doi: 10.1021/acs.chemrev.7b00329
141. Pathak S, Sneha CLR, Mathew BB. Bioplastics: its timeline based scenario and challenges. J Polym Biopolym Phys Chem. (2014) 2:84–90.
142. Erich M, Hannes G, Maximilian L. PHB – bio based and biodegradable replacement for PP: a review. Nov Tech Nutri Food Sci. 2:1–4. doi: 10.31031/NTNF.2018.02.000546
143. Pena-Francesch A, Demirel MC. Squid-inspired tandem repeat proteins: functional fibers and films. Front Chem. (2019) 7:69. doi: 10.3389/fchem.2019.00069
144. Niranjana Prabhu T, Prashantha K. A review on present status and future challenges of starch based polymer films and their composites in food packaging applications. Polym Compos. (2018) 39:2499–522. doi: 10.1002/pc.24236
145. Banerjee A, Dick GR, Yoshino T, Kanan MW. Carbon dioxide utilization via carbonate-promoted C–H carboxylation. Nature. (2016) 531:215. doi: 10.1038/nature17185
146. Ottesen V, Kumar V, Toivakka M, Carrasco GC, Syverud K, Gregersen Ø. Viability and properties of roll-to-roll coating of cellulose nanofibrils on recycled paperboard. Nordic Pulp Paper Res J. 32:179–88. doi: 10.3183/npprj-2017-32-02-p179-188
147. Banerjee A, Chatterjee K, Madras G. Enzymatic degradation of polymers: a brief review. Mater Sci Technol. (2014) 30:567–73. doi: 10.1179/1743284713Y.0000000503
148. Zheng Y, Yanful EK, Bassi AS. A review of plastic waste biodegradation. Crit Rev Biotechnol. (2005) 25:243–50. doi: 10.1080/07388550500346359
149. Narancic T, Verstichel S, Reddy Chaganti S, Morales-Gamez L, Kenny ST, De Wilde, Bet al. Biodegradable plastic blends create new possibilities for end-of-life management of plastics but they are not a panacea for plastic pollution. Environ Sci Technol. (2018) 52:10441–52. doi: 10.1021/acs.est.8b02963
150. Russell JR, Huang J, Anand P, Kucera K, Sandoval AG, Dantzler KW, et al. Biodegradation of polyester polyurethane by endophytic fungi. Appl Environ Microbiol. (2011) 77:6076–84. doi: 10.1128/AEM.00521-11
151. Khan S, Nadir S, Shah ZU, Shah AA, Karunarathna SC, Xu J, et al. Biodegradation of polyester polyurethane by Aspergillus tubingensis. Environ Pollut. (2017) 225:469–80. doi: 10.1016/j.envpol.2017.03.012
152. Yoshida S, Hiraga K, Takehana T, Taniguchi I, Yamaji H, Maeda Y, et al. A bacterium that degrades and assimilates poly(ethylene terephthalate). Science. (2016) 351:1196. doi: 10.1126/science.aad6359
153. Yang Y, Yang J, Wu W-M, Zhao J, Song Y, Gao L, et al. Biodegradation and mineralization of polystyrene by plastic-eating mealworms: part 2. Role of gut microorganisms. Environ Sci Technol. (2015) 49:12087–93. doi: 10.1021/acs.est.5b02663
154. Yang Y, Yang J, Wu W-M, Zhao J, Song Y, Gao L, et al. Biodegradation and mineralization of polystyrene by plastic-eating mealworms: part 1. Chemical and physical characterization and isotopic tests. Environ Sci Technol. (2015) 49:12080–6. doi: 10.1021/acs.est.5b02661
155. Bombelli P, Howe CJ, Bertocchini F. Polyethylene bio-degradation by caterpillars of the wax moth Galleria mellonella. Curr Biol. (2017) 27:R292–3. doi: 10.1016/j.cub.2017.02.060
156. Narancic T, O'Connor KE. Plastic waste as a global challenge: are biodegradable plastics the answer to the plastic waste problem? Microbiology. (2018). 165:129–37. doi: 10.1099/mic.0.000749
157. Temple EK, Rose E. Sustainable construction in rural Guatemala. Archiv Dis Childhood. (2011) 96:1048–51. doi: 10.1136/adc.2010.192641
158. Katz D. Plastic Bank. (2019). Available online at: https://www.plasticbank.com (accessed April 17, 2019).
159. PSF. Plastic Soup Foundation. (2019). Available online at: https://www.plasticsoupfoundation.org/2019 (accessed April 17, 2019).
161. Hinde D. The Swedish Recycling Revolution. (2019). Available online at: https://sweden.se/nature/the-swedish-recycling-revolution/ (accessed April 29, 2019).
162. Spee T, Huizer D. Comparing REACH chemical safety assessment information with practice-a case-study of polymethylmethacrylate (PMMA) in floor coating in The Netherlands. Int J Hyg Environ Health. (2017) 220:1190–4. doi: 10.1016/j.ijheh.2017.05.012
163. He Z, Li G, Chen J, Huang Y, An T, Zhang C. Pollution characteristics and health risk assessment of volatile organic compounds emitted from different plastic solid waste recycling workshops. Environ Int. (2015) 77:85–94. doi: 10.1016/j.envint.2015.01.004
164. National Institute for Occupational Safety and Health. Health and Safety Guide for Plastic Fabricators. Cincinnati, OH: U.S. Dept. of Health, Education, and Welfare, Public Health Service, Center for Disease Control, National Institute for Occupational Safety and Health, Division of Technical Services (1975).
165. Kumar A, Samadder SR. A review on technological options of waste to energy for effective management of municipal solid waste. Waste Manag. (2017) 69:407–22. doi: 10.1016/j.wasman.2017.08.046
166. Moya D, Aldas C, Lopez G, Kaparaju P. Municipal solid waste as a valuable renewable energy resource: a worldwide opportunity of energy recovery by using Waste-To-Energy Technologies. Enrgy Proced. (2017) 134:286–95. doi: 10.1016/j.egypro.2017.09.618
167. Brunner PH, Rechberger H. Waste to energy–key element for sustainable waste management. Waste Manag. (2015) 37:3–12. doi: 10.1016/j.wasman.2014.02.003
168. Bosmans A, Vanderreydt I, Geysen D, Helsen L. The crucial role of Waste-to-Energy technologies in enhanced landfill mining: a technology review. J Clean Prod. (2013) 55:10–23. doi: 10.1016/j.jclepro.2012.05.032
169. Stehlik P. Contribution to advances in waste-to-energy technologies. J Clean Prod. (2009) 17:919–31. doi: 10.1016/j.jclepro.2009.02.011
170. Marshall RE, Farahbakhsh K. Systems approaches to integrated solid waste management in developing countries. Waste Manag. (2013) 33:988–1003. doi: 10.1016/j.wasman.2012.12.023
171. Chattopadhyay S, Dutta A, Ray S. Municipal solid waste management in Kolkata, India—a review. Waste Manag. (2009) 29:1449–58. doi: 10.1016/j.wasman.2008.08.030
172. Tabasova A, Kropac J, Kermes V, Nemet A, Stehlik P. Waste-to-energy technologies: impact on environment. Energy. (2012) 44:146–55. doi: 10.1016/j.energy.2012.01.014
173. Kothari R, Tyagi VV, Pathak A. Waste-to-energy: a way from renewable energy sources to sustainable development. Renew Sust Energ Rev. (2010) 14:3164–70. doi: 10.1016/j.rser.2010.05.005
174. Novak RJ. A North American model to contain the spread of Aedes albopictus through tire legislation. Parassitologia. (1995) 37:129–39.
175. Forum WE. India Will Abolish All Single-Use Plastic by 2022, vows Narendra Modi. (2019). Available online at: https://www.weforum.org/agenda/2018/06/india-will-abolish-all-single-use-plastic-by-2022-vows-narendra-modi/ (accessed May 7, 2019).
176. Haregu TN, Ziraba AK, Aboderin I, Amugsi D, Muindi K, Mberu B. An assessment of the evolution of Kenya's solid waste management policies and their implementation in Nairobi and Mombasa: analysis of policies and practices. Environ Urban. (2017) 29:515–32. doi: 10.1177/0956247817700294
177. Fernandes JN, Moise IK, Maranto GL, Beier JC. Revamping mosquito-borne disease control to tackle future threats. Trends Parasitol. (2018) 34:359–68. doi: 10.1016/j.pt.2018.01.005
178. Gyawali N, Bradbury RS, Aaskov JG, Taylor-Robinson AW. Neglected Australian arboviruses and undifferentiated febrile illness: addressing public health challenges arising from the 'developing Northern Australia' government policy. Front Microbiol. (2017) 8:2150. doi: 10.3389/fmicb.2017.02150
179. Hotez PJ, Murray KO. Dengue, West Nile virus, chikungunya, Zika-and now Mayaro? PLoS Negl Trop Dis. (2017) 11:e0005462. doi: 10.1371/journal.pntd.0005462
180. Schnurr REJ, Alboiu V, Chaudhary M, Corbett RA, Quanz ME, Sankar K, et al. Reducing marine pollution from single-use plastics (SUPs): a review. Mar Pollut Bull. (2018) 137:157–71. doi: 10.1016/j.marpolbul.2018.10.001
181. Gutberlet J. Cooperative urban mining in Brazil: collective practices in selective household waste collection and recycling. Waste Manag. (2015) 45:22–31. doi: 10.1016/j.wasman.2015.06.023
182. I Got Garbage. I Got Garbage. (2019). Available online at: https://www.igotgarbage.com/what-we-do/2019 (accessed April 17, 2019).
183. Xiao LS, Zhang GQ, Zhu Y, Lin T. Promoting public participation in household waste management: a survey based method and case study in Xiamen city, China. J Clean Prod. (2017) 144:313–22. doi: 10.1016/j.jclepro.2017.01.022
184. Xanthos D, Walker TR. International policies to reduce plastic marine pollution from single-use plastics (plastic bags and microbeads): a review. Mar Pollut Bull. (2017) 118:17–26. doi: 10.1016/j.marpolbul.2017.02.048
185. Capolongo S, Rebecchi A, Dettori M, Appolloni L, Azara A, Buffoli M, et al. Healthy design and urban planning strategies, actions, and policy to achieve salutogenic cities. Int J Environ Res Public Health. (2018) 15:E2698. doi: 10.3390/ijerph15122698
186. Babiak K, Trendafilova S. CSR and environmental responsibility: motives and pressures to adopt green management practices. Corp Soc Responsib Environ Manag. (2011) 18:11–24. doi: 10.1002/csr.229
187. Adebanjo D, Pei-Lee T, Ahmed PK. The impact of external pressure and sustainable management practices on manufacturing performance and environmental outcomes. Int J Operat Prod Manag. (2016) 36:995–1013. doi: 10.1108/IJOPM-11-2014-0543
188. Eagle L, Hamann M, Low DR. The role of social marketing, marine turtles and sustainable tourism in reducing plastic pollution. Mar Pollut Bull. (2016) 107:324–32. doi: 10.1016/j.marpolbul.2016.03.040
189. Belontz SL, Corcoran PL, Davis H, Hill KA, Jazvac K, Robertson K, et al. Embracing an interdisciplinary approach to plastics pollution awareness and action. Ambio. (2018) 48:855–66. doi: 10.1007/s13280-018-1126-8
190. Hammami MBA, Mohammed EQ, Hashem AM, Al-Khafaji MA, Alqahtani F, Alzaabi S, et al. Survey on awareness and attitudes of secondary school students regarding plastic pollution: implications for environmental education and public health in Sharjah city, UAE. Environ Sci Pollut Res Int. (2017) 24:20626–33. doi: 10.1007/s11356-017-9625-x
191. Walther BA, Kunz A, Hu CS. Type and quantity of coastal debris pollution in Taiwan: a 12-year nationwide assessment using citizen science data. Mar Pollut Bull. (2018) 135:862–72. doi: 10.1016/j.marpolbul.2018.08.025
192. Dhokhikah Y, Trihadiningrum Y, Sunaryo S. Community participation in household solid waste reduction in Surabaya, Indonesia. Resour Conserv Recy. (2015) 102:153–62. doi: 10.1016/j.resconrec.2015.06.013
193. Ruckstuhl NA. Voluntary beach cleanups at famara beach, lanzarote—fighting marine litter invasion and accumulation locally. In: Baztan J, Jorgensen B, Pahl S, Thompson RC, Vanderlinden J-P, editors. Fate and Impact of Microplastics in Marine Ecosystems. Cambridge, MA: Elsevier (2017). p. 24. doi: 10.1016/B978-0-12-812271-6.00212-X
194. Konecny C, Fladmark V, De la Puente S. Towards cleaner shores: Assessing the Great Canadian Shoreline Cleanup's most recent data on volunteer engagement and litter removal along the coast of British Columbia, Canada. Mar Pollut Bull. (2018) 135:411–7. doi: 10.1016/j.marpolbul.2018.07.036
195. Sekito T, Prayogo TB, Dote Y, Yoshitake T, Bagus I. Influence of a community-based waste management system on people's behavior and waste reduction. Resour Conserv Recy. (2013) 72:84–90. doi: 10.1016/j.resconrec.2013.01.001
196. Tauil MC, de Azevedo AC. Community participation in health activities in an Amazon community of Brazil. Bull Pan Am Health Organ. (1978) 12:95–103.
197. Dung-Gwom JY, Yakubu Magaji J. The environmental health problems associated with solid waste management in Gwagwalada-Abuja. Abuja J Geogr Dev. (2007) 1:110–126.
198. Catapreta CA, Heller L. Association between household solid waste disposal and health, Belo Horizonte (MG), Brasil. Rev Panam Salud Publica. (1999) 5:88–96. doi: 10.1590/S1020-49891999000200003
199. Andrews JR, Barkume C, Yu AT, Saha SK, Qamar FN, Garrett D, et al. Integrating facility-based surveillance with healthcare utilization surveys to estimate enteric fever incidence: methods and challenges. J Infect Dis. (2018). doi: 10.1093/infdis/jiy494
200. Luby SP, Saha S, Andrews JR. Towards sustainable public health surveillance for enteric fever. Vaccine. (2015) 33 (Suppl. 3):C3–7. doi: 10.1016/j.vaccine.2015.02.054
201. Christensen PR, Scheuermann AM, Loeffler KE, Helms BA. Closed-loop recycling of plastics enabled by dynamic covalent diketoenamine bonds. Nat Chem. (2019) 11:442–8. doi: 10.1038/s41557-019-0249-2
202. Picheta R, Dean S. Over 180 Countries—Not Including the US—Agree to Restrict Global Plastic Waste Trade. CNN, 9:39 AM ET, Sat May 11, 2019. (2019). Available online at: https://www.cnn.com/2019/05/11/world/basel-convention-plastic-waste-trade-intl/index.html?no-st=1558034809 (accessed May 16, 2019).
Keywords: planetary health, infectious disease epidemiology, plastic pollution, vector-borne diseases, urban zoonoses, solid waste
Citation: Krystosik A, Njoroge G, Odhiambo L, Forsyth JE, Mutuku F and LaBeaud AD (2020) Solid Wastes Provide Breeding Sites, Burrows, and Food for Biological Disease Vectors, and Urban Zoonotic Reservoirs: A Call to Action for Solutions-Based Research. Front. Public Health 7:405. doi: 10.3389/fpubh.2019.00405
Received: 17 May 2019; Accepted: 19 December 2019;
Published: 17 January 2020.
Edited by:
Alfonso J. Rodriguez-Morales, Technological University of Pereira, ColombiaReviewed by:
Celio Geraldo Freire-de-Lima, Federal University of Rio de Janeiro, BrazilOscar David Kirstein, Emory University, United States
Copyright © 2020 Krystosik, Njoroge, Odhiambo, Forsyth, Mutuku and LaBeaud. This is an open-access article distributed under the terms of the Creative Commons Attribution License (CC BY). The use, distribution or reproduction in other forums is permitted, provided the original author(s) and the copyright owner(s) are credited and that the original publication in this journal is cited, in accordance with accepted academic practice. No use, distribution or reproduction is permitted which does not comply with these terms.
*Correspondence: Amy Krystosik, YW15a3J5c3Rvc2lrJiN4MDAwNDA7Z21haWwuY29t