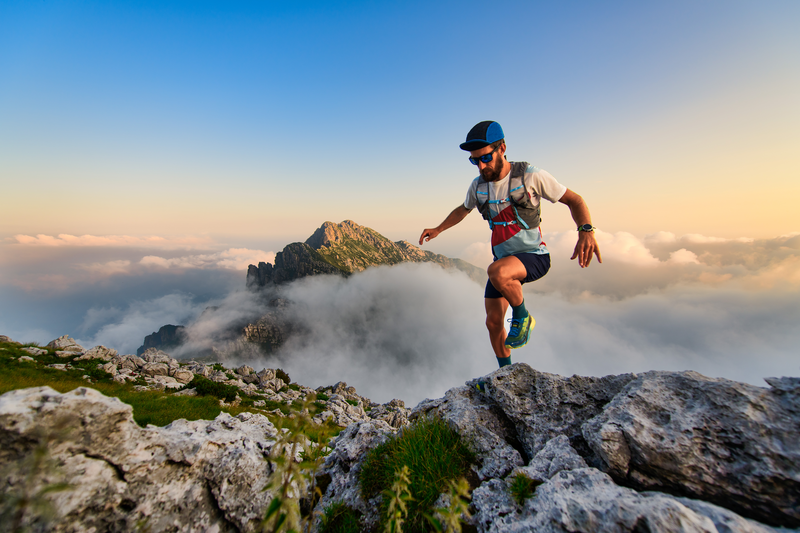
94% of researchers rate our articles as excellent or good
Learn more about the work of our research integrity team to safeguard the quality of each article we publish.
Find out more
REVIEW article
Front. Public Health , 06 December 2018
Sec. Environmental Health and Exposome
Volume 6 - 2018 | https://doi.org/10.3389/fpubh.2018.00337
The use of partially treated and untreated wastewater for irrigation is beneficial in agriculture but may be associated with human health risks. Reports from different locations globally have linked microbial outbreaks with agricultural reuse of wastewater. This article reviews the epidemiological evidence and health risks associated with this practice, aiming toward evidence-based conclusions. Exposure pathways that were addressed in this review included those relevant to agricultural workers and their families, consumers of crops, and residents close to areas irrigated with wastewater (partially treated or untreated). A meta-analysis gave an overall odds ratio of 1.65 (95% CI: 1.31, 2.06) for diarrheal disease and 5.49 (95% CI: 2.49, 12.10) for helminth infections for exposed agricultural workers and family members. The risks were higher among children and immunocompromised individuals than in immunocompetent adults. Predominantly skin and intestinal infections were prevalent among individuals infected mainly via occupational exposure and ingestion. Food-borne outbreaks as a result of crops (fruits and vegetables) irrigated with partially or untreated wastewater have been widely reported. Contamination of crops with enteric viruses, fecal coliforms, and bacterial pathogens, parasites including soil-transmitted helminthes (STHs), as well as occurrence of antibiotic residues and antibiotic resistance genes (ARGs) have also been evidenced. The antibiotic residues and ARGs may get internalized in crops along with pathogens and may select for antibiotic resistance, exert ecotoxicity, and lead to bioaccumulation in aquatic organisms with high risk quotient (RQ). Appropriate mitigation lies in adhering to existing guidelines such as the World Health Organization wastewater reuse guidelines and to Sanitation Safety Plans (SSPs). Additionally, improvement in hygiene practices will also provide measures against adverse health impacts.
The reuse of partially treated or untreated wastewater for irrigation has been linked to diarrheal and parasitic infections (1–4) as well as skin disorder and other systemic infections (5–8). An effective managing strategy of these health risks is challenging and depend on water quality (e.g., the type of wastewater: untreated, partially treated, or treated) as well as societal and behavioral factors which will vary between different settings (9–11). Health risks are not only dependent on exposure to microbial hazards (i.e., infectious pathogens) but also to chemicals or pharmaceuticals (12–14).
Populations exposed to wastewater are most often categorized into four main groups;
a) agricultural workers and their families;
b) crop merchants, handlers, and technical/operational staff;
c) consumers of farm produce, vegetables, meat, or milk products;
d) residents of areas irrigated with wastewater, including children, the elderly, and immunocompromised individuals, who are at the highest risks in particular (15).
The first two groups (a and b) would mainly be exposed occupationally, due to direct contact with the wastewater during application and handling. Consumers (group c) are directly exposed dependent on their diet. Residents (group d) of areas close to sites irrigated with wastewater can also be exposed through farm run-off, groundwater contamination and aerosols.
Wastewater reuse has environmental sustainability benefits when practiced safely (16, 17). Adverse health outcomes associated with its reuse have been documented, both directly through epidemiological studies (2, 5, 18–23), and indirectly using quantitative risk assessments (10, 24–31). Despite these reported health outcomes, the use of wastewater for irrigation is a widespread practice, partly due to its year-round availability and nutrient content (32). It will thereby contribute to the urban food basket and the overall improvement of the urban environment (33).
To protect public health and ensure that the full benefits associated with the reuse are achieved, regional, national, and international authorities have set guidelines for safe wastewater use in agriculture [e.g., 34]. However, it may be questioned if wastewater use in agriculture still causes adverse health effects, despite the existence of these guidelines for safe use. The purpose of this review is to summarize the evidence associated with the health impacts resulting from exposure to pathogens in partially or untreated wastewater used for agricultural purposes. Specifically, we report on the relevant exposure pathways and summarize epidemiological evidence of diarrheal, intestinal parasitic, and skin infections associated with direct exposure as well as indirect through produce from agriculture. The later refer to foodborne outbreaks from irrigated produce. We review the estimated health risks from partially treated and untreated wastewater irrigation using quantitative microbial risk assessment, and the risks associated with contaminants such as antibiotics and antibiotic resistance genes. Finally, we conclude by presenting approaches to mitigating the risks associated with the use in agriculture.
This review was based on literature searches in Science, Science Direct, PubMed, and Google Scholars till January, 2018. The keywords and word strings used in reference to microbial quality of wastewater intended for reuse were “wastewater OR treated wastewater OR partially treated wastewater OR untreated wastewater AND wastewater reuse AND microbial quality.” The health outcomes due to the reuse of wastewater was determined using the search string; “wastewater reuse AND epidemiological evidence OR diarrheal infections OR intestinal parasite infections OR infections OR microbial risk assessment OR quantitative microbial risk assessment (QMRA).” The last search string used in this review was; “wastewater reuse AND antibiotic residues OR antibiotic resistant genes OR antibiotic resistant bacteria.” The search results were reviewed by four reviewers.
Selections were made without restriction on the study location or year of publication, but only articles written in English language were accounted for. Articles that address direct epidemiological evidence, health risks, and those that estimated the health risks indirectly through QMRA were collated. Others are those that considered crop contamination associated with partially treated and untreated wastewater use and resulting in human infection or ecological impact. A number of studies quantifying antibiotics and antibiotic resistance genes predicated by the wastewater reuse were also included due their associated risks.
We specifically extracted and where appropriate tabulated information within the following areas:
a. Epidemiological evidence
b. Human health risks
c. Exposure pathways
d. Risk awareness
e. Ecotoxicity
f. Risk quotients
g. Risk mitigation
Tables and illustrative figures were developed, in which the relevant information were presented with geographical location (in some cases) and relevant statistical information reported where possible. The search yielded 1,223 articles, out of which a total of 167 publications were relevant to this review based on the inclusion criteria described in section Inclusions and Exclusion Criteria. This number comprises 156 articles, 2 book chapters, 5 relevant guidelines and four documents. Out of these publications, 50 were focused on human health risks from partially treated and untreated wastewater reuse, 19 linked reuse to specific infection and approximately 100 publications indirectly assessed the health risks from wastewater reuse. With wastewater reuse as written in the rest of the paper we include partially treated as well as untreated wastewater, if anything else is not stated. In the relevant sections the type of wastewater is stated to give clarity and aid in comparison.
Health risks associated with partially treated and untreated wastewater reuse are dependent of exposure combined with the presence and concentrations of hazards (e.g., pathogens). The excreted concentrations of pathogens (i.e., the hazards) vary based on the pathogen type and strain, the affected individual, and the phase of the infection cycle, and are thus dependent on the health of the population and the resilience of the pathogens to environmental stressors (35, 36). Figure 1 gives a general background graphical representation of the concentration of selected microbial indicators and pathogens reported in the literature. Detailed data from publications is presented in Table 1.
Figure 1. Visual representation of minimum and maximum concentration (Log10/L) of selected microorganisms in partially treated and untreated wastewater intended for reuse. The numbers on the bars represents the individual references with these reported concentrations. These articles are referenced in Table 1, where further information is provided. This is a brief introduction of the different concentrations reported in literature for the different categories of microorganisms. Table 1 gives detailed information on the literature added in this figure.
Table 1. Concentration of fecal indicator organisms and pathogens reported in selected literature from different types of wastewater used for irrigation.
Farmers and farm workers may be directly exposed to pathogens, and consumers of the farm produce will be indirectly affected. Additionally, the surrounding community can be further affected through the contamination of groundwater and run-off to surface water (59). Aerosols may also be formed in farms were sprinkler irrigation is practiced, affecting nearby communities as well (60, 61).
Different exposure pathways have been implicated in the spread of diseases associated with reuse of wastewater, especially partially treated and untreated, with examples shown in Table 2. The main route through direct contact could be termed as an occupational exposure pathway. This has been implicated in the spread of diarrhea (2, 4, 62, 64), parasitic infections (63, 67, 68) and skin infections (5, 6, 22)]. The amount of direct contact with wastewater during its application is largely dependent on the type of irrigation practiced and individual behavior. For example, the use of watering cans for the collection of irrigation water from ponds, as is the case in many developing countries where the practice of wastewater irrigation is common, results in the greatest exposure (76). This exposure leads to greater risk due to the non-use of personal protective equipment like boots and nose masks. Furrow or flood irrigation also increases the possibility of direct contact with the wastewater, increasing risks of infections (18, 77).
Table 2. Exposure used in the determination of diseases associated with wastewater irrigation from selected literature.
The use of sprinkler irrigation may also lead to greater exposure not only for farmers and other farm workers but for the general community because of exposure to aerosols (78–80). Communities living close to wastewater irrigation sites in Israel where sprinkler irrigation was practiced had an IRR of 1.08 for diarrhea, although this risk of infection was lower than the risk from direct exposure to partially treated and untreated wastewater (IRR = 1.23). That study particularly showed the impact of sprinkler irrigation on the health of a larger population (60). Aerosols may be inhaled or may settle on food (81). Aerosols from wastewater are also reported to contain several different types of pathogens. Li et al. (82) showed that the suspension of Actinomycetes (aetiological agent for actinomycosis) and other pathogenic microbes in aerosols from partially treated wastewater constitute health hazard in Xi'an, China.
Consumption of produce, especially vegetables, from wastewater irrigated farms poses an additional pathway for transmission of pathogens from wastewater to the general public. Agunwamba (83) demonstrated that consumers of vegetables from wastewater irrigated fields leads to an IRR of 1.75 for diarrhea. Section Food-Borne Outbreaks (Human) Associated With the Consumption of Fresh Produce Irrigated With Wastewater discusses this exposure pathway in detail.
Bacterial concentrations in wastewater and air quality around the farmyard have been shown to closely relate. A study by Teltsch and Katzenelson (84) detected aerosolized coliforms with concentration ≥103 CFU/mL. The pathogens carried may depend on their size and the force of the spray. Small sized pathogens are more commonly transported during irrigation (85, 86). Enteric viruses may occur in significant concentration in aerosolized form, when compared with the varieties of microorganisms found in wastewater (85). Their retention in wastewater for irrigation is usually due to the inability of some WWTPs to effectively achieve virus removal. It also depends on high resistance to environmental stress by some of these viruses. For aerosolized bacteria, relative humidity and solar irradiation may affect their viability and subsequently their concentration in the air. The aerosolized bacterial pathogens may also be affected by UV, as there was reports of 10 times more aerosolized bacteria measured during night irrigation than with day irrigation (84).
The concentrations of pathogens that reach farmyard houses and lead to exposure in consuming food and inhalation may depend on the wind velocity (87). The probability of initiating infection also depends on the infective dosage. For example, low quantities of viruses (102 pfu), such as for noroviruses, are enough to initiate infection through the respiratory pathway (85). Depending on the irrigation methods (i.e., sprays or sprinklers), farm workers and community members in the surrounding area may be exposed to the aerosolized pathogens from the wastewater (88, 89).
Aerosol spray may also facilitate deposition of contaminated wastewater on the edible parts of the crop. Surface contamination of these has been reported as a major means of transmission of diseases through consumption (90–93). Additionally, internalization of pathogens in different vegetables has been documented (94–98), which could be another route of transmission of these pathogens to the consumers. The re-growth of these pathogens on vegetables has also been reported (99) and is partly influenced by factors such as, their adhesion ability to the plant surfaces (100, 101) and the persistence of the particular pathogen (102, 103).
Globally there have been different reported outbreaks, for example 11 lettuce-related outbreaks of gastroenteritis with a total of 260 reported cases were reported in Denmark (104). Researchers emphasize that adhesion of pathogens to surfaces and internalization of pathogens placed high demand of responsibilities on consumers to avoid gastroenteritis (105). Most outbreaks have been attributed to infection with different strains of Salmonella (106–109), Enterohemorrhagic E. coli (110–113), and viruses such as norovirus (114). Table 3 presents examples of food-borne human outbreaks (microbial) associated with the consumption of fresh produce irrigated with wastewater. The type of irrigation practice plays a critical role in the contamination of farm produce. A study by Makkaew et al. (121) assessed the contamination of E. coli in lettuce grown under four different methods of wastewater irrigation; open spray, spray under plastic sheet cover, open drip, and drip under plastic sheet cover. E. coli contaminations were detected in all the lettuce samples with all types of spray beds. Submersed lettuce irrigated with wastewater contained 1,300 E. coli MPN/100 mL and had equal levels of contamination as with the spray irrigation. The scenario was similar in both laboratory and experimental investigations (121). The study also showed that crops are seriously imparted by wastewater irrigation, independent of the irrigation schemes used (121). In a study on the use of wastewater in agriculture, Antwi-Agyei (122) identified irrigation with partially treated and untreated wastewater as a key risk factor for the observed contamination of 80% of produce samples. The study reported the median concentration ranging from 0.64 to 3.84 log10 E. coli/g produce, and fresh salad having as high as 4.23 log10 E. coli/g. In a similar study, Bouwknegt et al. (123) estimated the following risks per serving of lettuce based on the QMRA models: 3 × 10−4 (6 × 10−6 −5 × 10−3) for NoV infection and 3 × 10−8 (7 × 10−10 −3 × 10−6) for hepatitis A jaundice. In that study however, the risk of wastewater irrigation was less than the effect of hand's contact (123).
Table 3. Examples of food-borne outbreaks (human) associated with the consumption of fresh produce irrigated with wastewater from selected literature (Only the reports with year of outbreak, food implicated and number of cases were considered).
Risk awareness might also be a factor that includes handling of the crops and vegetables irrigated with wastewater. Antwi-Agyei et al. (105) reported that knowledge of the irrigation water source and associated quality were associated with higher awareness of health risks (OR = 4.6, p = 0.06). However, the outcome was not impacted by demographic factors like age, education, or gender (OR = 4.7, p = 0.12). A higher awareness of health risks may change on-farm practices and also reduce the exposure of farmers to the pathogens in the wastewater, as well as, reduce the concentration of pathogens on the farm produce leading to lesser adverse health impact on the both farmers and consumers. Reuse of wastewater in aquaculture has also been linked with adverse health effects (124). For instance, fish farmers without understanding of health risk and protective clothing were found to have a high exposure to the pathogens in the wastewater, leading to skin infections (21).
The different types of infections reported for people exposed to partially treated and untreated wastewater used in agriculture are discussed in the following sections. Section Wastewater Reuse, Diarrhea and intestinal infection summarizes the epidemiological evidence of diarrheal and other intestinal infections. Section Wastewater Reuse and Intestinal Parasitic Infections summarizes the evidence of intestinal parasitic infections and section Skin Infections the evidence of skin infections.
Partially treated and untreated wastewater reuse has been directly linked with diarrheal diseases (Figure 2). Our meta-analysis gave an overall odds ratio of 1.65 (95% CI: 1.31, 2.06) for diarrheal diseases among agricultural workers and family members exposed to wastewater for irrigation. Direct exposure by farm workers to wastewater has been shown as the main transmission route. However, farm workers may also be exposed to pathogens based on their contact with soil that has been contaminated by wastewater, especially partially treated and untreated. In addition to the normal microflora, wastewater irrigated soils have elevated pathogen concentrations. For example, Klutse (125) reported higher concentrations of Enterobacteriaceae, Bacillus spp., Staphylococcus spp., Pseudomonas spp., and Clostridium spp. in irrigated soils. Antwi-Adyei et al. (126) identified soil as the main risk pathway with factors such as working barefooted (93% of farmers), hand contact with contaminated soil (86% of the farmers), and contaminated hands to mouth (53% of the farmers) as the main contributors. Additionally, the regrowth of bacteria in the environment may contribute to the increased incidence of diarrhea, as the high nutrient content of wastewater can enhance bacterial regrowth (127, 128). Modeled risks based only on pathogens contained in the liquid effluent from wastewater treatment plants, without accounting for regrowth, may lead to the underestimation of risks.
Figure 2. Odds ratio for exposure to partially treated and untreated wastewater and diarrheal disease incidence from selected literature.
The majority of the epidemiological studies on reuse and diarrheal diseases have focused on the direct exposure to the wastewater especially by the farmers and farm workers. However, Shuval et al. (60) demonstrated that aerosols could lead to a diarrheal incidence rate ratio of 1.08 for people living close to irrigation fields in Israel. Living in a household with someone engaged in untreated wastewater reuse could also result in higher risk of diarrhea (OR = 2.69) (21). This relate to the possibility of additional human to human transmission within the home. This is important because domestic hygiene has been implicated in the increase in diarrheal diseases within the home setting for children (129, 130). Within the domestic domain several other sources of contamination, like from food, contaminated drinking water in the home (131) or direct exposure to feces from humans and animals will superimpose on the effects that is due to the irrigation with wastewater with linked exposure.
Children and immunocompromised individuals have been shown to have higher risks of diarrheal infections than immunocompetent adults (132–135). Blumenthal et al. (77) reported an increase in the risk of diarrhea (OR = 1.75) among children aged <5 years. This diarrhea may be from the ingestion of pathogenic microorganisms, such as Salmonella species and Diarrheagenic Escherichia coli (2, 70, 77, 136) in either the soil or wastewater.
Intestinal parasitic infections, especially soil-transmitted helminths, are reported as the major health concern associated with wastewater reuse (34). This is partly due to their high persistence in the environment. In line with this concern, epidemiological studies have shown an increase in parasitic infections from the use of wastewater (Figure 3). The main exposure route accounted for in these studies has been direct contact for farmers and other farm workers, where increased risks with ORs 0.58–3 have been reported (3, 19, 137, 138). In a study conducted in urban and peri-urban transition zones in Hanoi, Vietnam, Fuhrimann et al. (139) reported that peri-urban farmers had the highest adjusted odds of acquiring intestinal parasitic infection among various groups considered (OR 5.3, 95% CI: 2.1–13.7). However, Trang et al. (72) established that wastewater reuse did not pose significant risks for helminth infections among rice farmers using wastewater in Vietnam, although untreated wastewater is used. Our meta-analysis gave an overall odds ratio of 5.49 (95% CI: 2.49, 12.10) for helminth infections in agricultural workers and family members exposed to wastewater for irrigation (Figure 3).
Figure 3. Odds ratio for exposure to partially treated/untreated wastewater and helminth infections from selected literature.
The infection risks may differ between the types of parasitic pathogens found in wastewater due to variations in concentration and the mode of exposure to the irrigation water. There is however similarities in the consistency of found values. For instance, in Ghana risks of infection with Ascaris and hookworm was found among the farmers and family member exposed to irrigation water (surface water contaminated with untreated wastewater) to be similar with ORs of 3.9 (95% CI, 1.15–13.86) and 3.07 (95% CI, 0.87–10.82), respectively (3). Pham-Duc (19) reported Ascaris infection OR of 2.1 (95% CI 1.4–3.2) vs. an OR of 1.5 (95% CI 1.0–2.3) for Trichuris trichiura. A cross-sectional survey of children exposed to wastewater irrigation in Morocco showed a 20.5% higher prevalence of Ascaris infection compared to 3.8% for unexposed children (18). From the same study in Morocco the difference in Trichuris infection among the exposed (0.4%) and unexposed (0.3%) children was reported to be non-significant. Furthermore, approximately 45% of farmers using wastewater effluents for irrigation in Asmara villages, Eritrea were infected with giardiasis, with consumers of their vegetables 7% more likely to be infected with giardiasis than a control group (140).
These differences and similarities in infection prevalence from the exposure could be attributed to (1) the difference in the concentration of the infectious eggs in the wastewater, which is dependent on the type of wastewater treatment, (2) differences in their persistence in soil or on crops, or (3) the efficiency with which they are transferred from irrigation water to soil/crops. For example, Verbyla et al. (10, 141) reported higher ratios of helminth egg and parasitic (oo)cyst (Giardia and Cryptosporidium) concentrations on irrigated soil and crops relative to their concentrations in wastewater used for irrigation, with lower corresponding ratios for coliphages and for the viral fecal indicator pepper mild mottle virus. The parasite eggs and (oo)cysts may be more efficiently transferred from irrigation water to crops/soil than some viruses, and/or it supports previous findings that the parasites persist longer on crops or in soil than viruses (142). Additionally, pathogen concentrations in wastewater are also a reflection of the infection levels in the populations. Hookworms in particular remain a major cause of morbidity in developing countries, which are accompanied by gastrointestinal symptoms and chronic anemia (143). This epidemiological evidence therefore shows a linkage or interconnectedness of infection and pathogen concentration in wastewater.
The risk of parasitic infections from either direct or indirect exposure to wastewater also will vary depending on factors, such as age, gender, frequency of exposure etc. For instance, Blumenthal et al. (77) reported a close association between direct exposure to untreated wastewater and an excess risk of A. lumbricoides infection in children aged < 5 years (OR = 18.0). Also, a cross-sectional survey of people aged ≥ 18 years at risk to wastewater exposure showed a 30% point-prevalence of intestinal parasitic infections (139).
Exposure to wastewater could result in different types of skin infections, such as, dermatitis, urticarial, fungal infections of toe- or fingernails (23). Trang et al. (5, 6) suggested that the skin diseases may be deep systemic dermatitis and other fungal infections. Studies conducted in Musi River, India; Phnom Penh, Cambodia, and Hanoi, Vietnam showed skin infection and irritation as the major effect of the exposure (7, 22). However, only a few studies have focused on an epidemiological link. For example, a cross-sectional survey in Vietnam involving over 235 farmers using wastewater for irrigation or aquaculture reported an OR for dermatitis of 3.0 (95% CI: 1.1–7.7) (22). A similar study from the same country reported a lower OR of 2.7 (95% CI: 1.3–5.8) for direct contact with wastewater (6). In addition to direct contact, living in a household with someone involved in wastewater reuse increased the risks of skin infections (5). Frequency of exposure, immunological status, occupation and the lack of protective coverings were identified as the determining factor predisposing individuals to this effect of wastewater (5, 144).
Quantitative Microbial Risk Assessment (QMRA) is a modeling process that estimates the potential human health risks from exposure to different pathogens (e.g., human pathogenic viruses, protozoa, and bacteria) (145) especially through food and/or water (146). This is a structured approach that integrates information and data with mathematical models to examine exposure and spread of microbial pathogens and in the process characterize the human health risks (147, 148). QMRA has been used extensively in the risk estimation of infections for different exposed groups related to wastewater reuse (25, 29, 31, 149, 150). The risk estimates from these studies vary, mainly due to difference in the concentration, for example by viable STH eggs ingested by exposed populations. QMRA has been used by the WHO (34, 151) and the Australian government (152, 153) to determine pathogen reductions needed to achieve a health target of 10−6 DALYs per person per year (ppy) (154).
The use of QMRA allows for the quantification of infection risks for different populations that has ingested or been exposed to different concentrations of pathogen. The exposure could be either from intentional or accidental ingestion of wastewater, consumption of farm produce or ingestion of groundwater from irrigation fields etc. Assuming ingestion of wastewater and soil, Seidu et al. (31) showed that two farmers out a hundred using wastewater for irrigation were likely to be infected with Ascaris due to accidental ingestion of the irrigation water. However, accidental ingestion of the farm soil may result in infection of all exposed farmers, possibly due to accumulation of the parasitic eggs in the soil. Considering risks from inhalation of aerosols, Courault et al. (40) estimated norovirus infection risks of >10−4. Carlander et al. (88) reported high risks of rotavirus infections in Culmore, Northern Ireland [P(inf) 8 × 10(−1)] and Kvidinge, Sweden [P(inf) 7 × 10(−1)] when wastewater is used for irrigation. Estimation of infection risks from aerosols using QMRA, generally takes into account the wind speed and distance of the exposed population.
QMRA estimation of infection risks could also account for different crops. This is important especially in relation to the amount of irrigation water that could possibly remain on the crops by the time of harvest and subsequent consumption. While considering different wastewater-irrigated vegetables, Mok et al. (155) observed that consumption of lettuce posed the greatest health risks as compared to cucumber and broccoli. The researchers reported that the median annual norovirus disease burden across the different vegetables ranged from 7.95 × 10−5 to 2.34 × 10−3 DALY/person/year (155).
Risk estimates produced by QMRA are dependent on the input dose or concentration of pathogen used among other factors such the dose response model used and frequency of exposure. The concentration of the pathogen could be either determined in the laboratory or assumed. Some researchers use fecal indicator microorganisms to estimate pathogen concentrations in wastewater and thereafter determine the infection risks. This is mainly due to the ease of detection of these microorganisms, however the survival and even removal/inactivation of these in the environment differs greatly from many pathogenic microorganisms. It was observed by Owusu-Ansah et al. (156) that the use of fecal indicator conversion ratio model to estimate health risks results in the underestimation of the risks involved. Using improved methods of detection, Cutolo et al. (25) reported average densities of 1 cysts L−1 and 6 eggs L−1 for Entamoeba coli and Ascaris spp., respectively. The reported annual risks of Ascaris infection resulting from accidental ingestion of wastewater irrigation were therefore reported to be 7.5 × 10−2 in 208 days and 8.7 × 10−2 in 240 days (25).
Antibiotic residues and antibiotic resistant genes ARGs have been reported in wastewater released from wastewater treatment plants (157–162). The presence of these in wastewater has been identified as a possible source of antibiotic resistance microorganisms (13, 163). This resistance could be developed through induction, selection or horizontal gene transfer of ARGs (162, 164). Varying antibiotic removal efficiencies from wastewater for reuse has been reported (165–167).
These studies show that most treatment plants have antibiotics in their effluents which when reused may impact and accumulate in soil and be taken up by the crops. Reported concentration of antibiotics and antibiotic resistance genes identified in treated wastewater are exemplified in Table 4. Negreanu (185) assessed the presence of antibiotic-resistant bacteria and antibiotic resistant genes in agricultural soils irrigated with wastewater and Broszat et al. (186) concluded that wastewater irrigation increases abundance of potentially harmful Gamma-proteobacteria with antibiotic resistance genes in soils from Mezquital Valley, Mexico. These studies suggest the emergence of antibiotic resistance as future potential concern for reuse of wastewater.
Table 4. Reported concentration (from selected literature) of antibiotic residues and antibiotic resistance genes in partially treated and untreated wastewater for reuse and the irrigated soil.
The concentration of antibiotics will be reduced during treatment and the efficiency of removal is dependent on the treatment technology (187, 188) and the physical state of the antibiotic compound (189). Varying removal efficiencies of different antibiotics have been reported in the USA and China (165, 172, 190–192). All studies reported that residual antibiotics persist in treated wastewater and sludge in most cases and can be conveyed along the reuse pathways.
Some antibiotics remain intact in wastewater after excretion, while others may form complexes or change the parental configuration. This may however be reconverted into their parent compounds by deconjugation during biological treatment (193). Due to their hydrophobicity some of the antibiotics may also be sorbed to particles. Based on the removal of particles during wastewater treatment, the aqueous concentration of antibiotics will change but they will be transferred to sludge. Some may also be desorbed with intact configuration and appear in the final effluents together with hydrophilic ones (194). Norfloxacin and tetracycline exemplifies sorption to particulate matters, which can be highly variable.
When the wastewater is used for irrigation on farmland the residual antibiotics and ARGs may impact the normal microflora of the soil (157–162, 164). Their persistence in soil depends on factors that includes sorption to soil particles that also influences leaching potential to groundwater and their biotic and abiotic degradation. The most essential abiotic reactions are photochemical and chemical mediated transformations, which may include hydrolysis, oxidation and reduction, which would occur in upper soil layers (195). One research report by Pan and Chu (196) postulate that tetracycline possesses a higher sorption affinity in agricultural soil when compared with quinolones, macrolides, chloramphenicol, and sulphonamides and sulphonamides were reported to have high leaching potential to the groundwater (197).
The antibiotics in wastewater for irrigation will impact the soil directly and may result in eco-toxicological effects (198). The effects of wastewater irrigation on the occurrence of ARGs were reported by Jechalke et al. (199) over a period of 100 years of irrigation. Because the nutrients in the wastewater support regrowth of some bacterial pathogens the presence of sub-inhibitory concentration of the antibiotics can bring about resistance by induction and/or expression of ARGs taken up.
Christou et al. (178) reported an uptake of sulfamethoxazole and trimethoprim by tomato crop plants with fruit bio-accumulating 5.26 μg kg−1 of sulfamethoxazole and 3.40 μg kg−1 of trimethoprim. The antibiotics, ARGs and the antibiotic resistant pathogens (ARP) may be taken up (like nutrients). The ARP may pass through the natural root apertures into the xylem or phloem of the plants, be translocated and internalized in edible part of the crop (200–203). By this they may impact the consumers (204) especially when the crops involved are to be eaten raw (200, 204). For these categories of uncooked crops, certain bacterial species like Stenotrophomonas maltophilia and Acinetobacter species usually found in wastewater and root rhizosphere can be a threat if allowed to contaminate the crop (14, 205). This is because they are also known for being reservoirs of wide range of ARGs, extreme drug resistance and as etiological agent of life threatening infections (14, 206, 207).
These ARP might be difficult to treat due to their antibiotic resistance status. Carey et al. (208) detected total enterococci and vancomycin resistant Enterococci in 71% (34/48) and 4% (2/48) of reclaimed water samples, respectively with Enterococcus faecalis as the most prominent species. These Enterococci in the reclaimed water exhibited high antibiotic resistance at the point of water use as measured using their minimum inhibitory concentrations (MICs). Contaminated water used for irrigation may initiate common source epidemics as in the outbreak of food borne pathogen in some countries in Europe as reviewed by Okoh et al. (209).
Apart from ARP, antibiotics in the wastewater cause phytotoxicity. A screening for the level of phytotoxicity by antibiotics internalized into seedlings and plants was done using seed germination experiment by Hillis et al. (210) and Pan and Chu (196) as well as plant growth tests (211). The results showed varying toxic effects of antibiotics on the cultivars. Pan and Chu (196) showed that the antibiotics were taken up and it inhibited root elongation significantly (p < 0.05). Tetracycline was noted to have the highest level of toxicity among the antibiotics while lettuce crop was found to be the most affected by larger number of the veterinary antibiotics (212).
Residual antibiotics and ARGs remain two major concerns in integrated human health risk assessment associated with the use of wastewater (213–215). The risk associated with reuse of wastewater containing antibiotics or any pharmaceutical is generally expressed as risk quotient (RQ) or hazard quotient (HQ). The risk quotient (RQ) for antibiotic in reused wastewater reflects the extent of associated ecological risks (216). RQ, which is a calculated index, constitutes a vital analytical tool for assessing ecotoxicological risks (217, 218). It varies proportionally with the detected concentration of the antibiotics in the wastewater. It is the ratio of measured concentration (MEC) in wastewater to the predicted no effect concentration where no effect concentration (PNEC) as follows:
In Equation (1), RQ < 0.1 means low risk, < RQ < 1 means medium risk and, RQ > 1 means high ecological risk (219, 220).
Also in this equation, PNEC equals the LC50/1000 where LC50 is the chemical concentration that results in death to 50% of the studied population.
Instead of the three levels of risks of antibiotics (as well as other pharmaceuticals) shown above, the following four levels of risks (ecotoxicity) have been proposed by FASS (221): RQ ≤ 0.1 for insignificant risks, 0.1 ≤ RQ ≤ 1 for low risks, 1 ≤ RQ ≤ 10 for moderate risks and, RQ > 10 for high risk.
Many research studies report high risk quotients of partially treated and untreated wastewater reuse associated with the presence of erythromycin (222–224), clarithromycin (222), and ciprofloxacin (225). The risk quotients in these instances were mostly >1, suggesting high ecological risk (219, 220). This also shows the potential environmental impact of antibiotic residues that may enter surface water from reclaimed water used for irrigation. Chlortetracycline and oxytetracycline, were reportedly the most prevalent antibiotics found in livestock wastewater treatment plants (226) with enhanced hazard to ecosystems in Korea. Following a study on long-term wastewater irrigation, Christou et al. (178) concluded that the estimated threshold of toxicity concern (TTC) and high hazard quotients (HQ) values of pharmaceuticals observed, revealed that wastewater irrigation has an impact on human health, because the pharmaceutical gets internalized and bioaccumulated in tissues causing toxicity.
There are several approaches to consider mitigating the risks associated with the reuse of wastewater. These approaches have been captured in the World Health Organization (WHO) wastewater reuse guidelines (34, 151) and in sanitation safety plans (227). One approach that has proven to show positive results is the cessation of irrigation before harvesting (36, 228). The main risk reduction factor associated with cessation of irrigation is the decay of pathogens with time. Keraita et al. (228) studied the reduction of microbial contamination on wastewater-irrigated lettuce due to cessation of irrigation before harvesting and obtained a risk reduction of 0.65 log10 units for thermotolerant coliforms and 0.4 log10 helminth eggs per 100 g of lettuce. In addition, Sjölander (36) reported a decay rate of 0.4 day−1 for Ascaris suum on lettuce following cessation of irrigation before harvesting. The need to employ an optimum model to determine the appropriate time of die-off is thus imperative, particularly for interventions associated with health risk reduction (229). When accuracy in the actual prediction of the potential risk is involved, Oron et al. (230) recommended that research should incorporate definite acceptable risk criteria, more accurate dose-response modeling information including survival time of pathogen in treated wastewater. Information on uptake of pathogens by irrigated plants as well as the eating habits of the populations would provide a more pertinent impact (230). In relevance to these criteria such as plant uptake or eating habits of exposed population, and analytical tools' harmonization, Beaudequin et al. (231) proposed the use of a Bayesian network as a useful tool in situations where empirical data necessary for QMRA calculation requirements are insufficiently available. This may be integrated into other models to predict effectiveness of appropriate cessation period before harvest. The cessation may be used with other treatment technologies for wastewater before reuse (232).
In handling some viruses, Kobayashi et al. (232) have optimized a down-flow hanging sponge (DHS) reactor treating municipal wastewater. The optimization depicts that in DHS effluent for agricultural irrigation for 95% human population, a log10 reduction of 2.6 and 3.7 for norovirus GII in the DHS is attainable. This would, respectively, retain ≤ 10−4 and 10−6 DALYs loss per person per year (232). The impacts of using wastewater for irrigation on the one hand and post-harvest handling and storage contamination on the other, needs to be further addressed. The specific situation with the potential impact of internalization and uptake of pathogens as compared to deposition on outer surfaces need much more attention and documentation, before long-term handling and management practices can be issued and related to modes of application.
In addition, the specific situation with regards to chemical contaminants (e.g., uptake of antibiotics and other organic compounds) as well as the impact of use of these in livestock and among humans and the further fate in agricultural fields need to be addressed. Chen and Zhang (233) noted that constructed wetlands are effective for removing antibiotics and ARGs and compares favorably with mechanical wastewater treatment processes (p > 0.05). Modern processes involving ozonation, advanced oxidation, activated carbon, nano-filtration and reverse osmosis have also been recommended as treatment technologies (234).
Onsite filtration of the wastewater for irrigation during application may reduce the extent of farmland contamination. This may be considered in relation to riverbank filtration (RBF) proposed by Verbyla et al. (10). RBF may be accompanied with UV device for additional treatment before use (235). Toscano et al. (235) reported a significant (p < 0.05) average reduction of pathogen concentration of reclaimed water from 0.35 to 1.23 log units by using the UV system.
The guidelines by WHO on the reuse of wastewater in Agriculture (236) has provided a path to safeguard human health. If adequate care is taken, it is possible to achieve a better crop yield with wastewater effluents without constituting hazards (237, 238). Multiple barrier approach recommended in the guidelines is to protect the farmer and farm household with contacts to the wastewater being reused. It is also meant for protecting the food chain at critical control or entry points, especially in arid and semi-arid countries where reuse of treated or untreated wastewater is rampant (236). Sometimes, there can be the need for necessary steps and considerations of social marketing, incentive systems, awareness creation or education and local regulations (239). This would encompass a framework to protect both the farmers and the consumers.
The reuse of partially treated or untreated wastewater for agricultural irrigation is widespread due to its availability and high nutrient content. The agricultural reuse of wastewater contributes to poverty alleviation especially in resource-constraint regions. Despite the many benefits associated with the practice serious health concerns still exist as presented in this review. The health impact is dependent on the concentration of the contaminants which varies, as shown in Table 1. The concentration of the contaminants is dependent on the type of wastewater, either treated, partially treated or untreated. The WHO wastewater reuse guidelines as well as the Sanitation Safety plans has been shown to protect public health, however the evidence presented in this review indicates a shortcoming in the implementation. This calls for a more concerted effort in adapting these guidelines/plans into local frameworks. The planning and implementation of these local frameworks should involve all stakeholders, such as policy makers, farmers, health professionals and the general public.
All authors listed have made a substantial, direct and intellectual contribution to the work, and approved it for publication.
This work was supported by the Bill and Melinda Gates Foundation (Grant Number: OPP1122681). The support of the South African Research Chairs Initiative of the Department of Science and Technology and National Research Foundation of South Africa is also duly acknowledged. We are also grateful to our respective host institutions for their support.
The authors declare that the research was conducted in the absence of any commercial or financial relationships that could be construed as a potential conflict of interest.
We are grateful to the South African Research Chairs Initiative (SARChI) of the Department of Science and Technology and National Research Foundation (NRF) of South Africa and the Institute for Water and Wastewater Technology (IWWT), Durban University of Technology for the support.
1. Okoh AI, Sibanda T, Gusha SS. Inadequately treated wastewater as a source of human enteric viruses in the environment. Int J Environ Res Public Health (2010) 7:2620–37. doi: 10.3390/ijerph7062620
2. Ferrer A, Nguyen-Viet H, Zinsstag J. Quantification of diarrhea risk related to wastewater contact in Thailand. Ecohealth (2012) 9:49–59. doi: 10.1007/s10393-012-0746-x
3. Amoah ID, Abubakari A, Stenström TA, Abaidoo RC, Seidu R. Contribution of wastewater irrigation to soil transmitted helminths infection among vegetable farmers in Kumasi, Ghana. PLoS Negl Trop Dis. (2016) 10:e0005161. doi: 10.1371/journal.pntd.0005161
4. Contreras JD, Meza R, Siebe C, Rodríguez-Dozal S, López-Vidal YA, Castillo-Rojas G, et al. Health risks from exposure to untreated wastewater used for irrigation in the Mezquital Valley, Mexico: a 25-year update. Water Res. (2017) 23:834–50. doi: 10.1016/j.watres.2017.06.058
5. Trang DT, Molbak K, Cam PD, Dalsgaard A. Incidence of and risk factors for skin ailments among farmers working with wastewater-fed agriculture in Hanoi, Vietnam. Trans R Soc Trop Med Hyg. (2007b) 101:502–10. doi: 10.1016/j.trstmh.2006.10.005
6. Trang DT, van der Hoek W, Tuan ND, Cam PD, Viet VH, Luu DD, et al. Skin disease among farmers using wastewater in rice cultivation in Nam Dinh, Vietnam. Trop Med Int Health (2007a) 12:51–8. doi: 10.1111/j.1365-3156.2007.01941.x
7. Anh VT, van der Hoek W, Ersbøll AK, Vicheth C, Cam PD, Dalsgaard A. Peri-urban aquatic plant culture and skin disease in Phnom Penh, Cambodia. J Water Health (2009) 7:302–11. doi: 10.2166/wh.2009.128
8. Lam S, Nguyen-Viet H, Tuyet-Hanh TT, Nguyen-Mai H, Harper S. Evidence for public health risks of wastewater and excreta management practices in Southeast Asia: a scoping review. Int J Environ Res Public Health (2015) 12:12863–85. doi: 10.3390/ijerph121012863
9. Verbyla ME, Cairns MR, Gonzalez PA, Whiteford LM, Mihelcic Jr. Emerging challenges and synergies for pathogen control and resource recovery in natural wastewater treatment systems. WIREs Water (2015) 2:701–14. doi: 10.1002/wat2.1101
10. Verbyla ME, Symonds EM, Kafle RC, Cairns MR, Iriarte M, Mercado GA, et al. Managing microbial risks from indirect wastewater reuse for irrigation in urbanizing watersheds. Environ Sci Technol. (2016) 50:6803–13. doi: 10.1021/acs.est.5b05398
11. Mihelcic Jr, Naughton CC, Verbyla ME, Zhang Q, Schweitzer RW, Oakley SM, et al. The grandest challenge of all: the role of environmental engineering to achieve sustainability in the world's developing regions. Environ. Eng. Sci. (2017) 34:16–41. doi: 10.1089/ees.2015.0334
12. Marcussen H, Holm PE, Ha LT, Dalsgaard A. Food safety aspects of toxic element accumulation in fish from wastewater-feed ponds in Hanoi, Vietnam. Trop Med Int Heal. (2007) 12:34–9. doi: 10.1111/j.1365-3156.2007.01939.x
13. Adegoke AA, Faleye AC, Singh G, Stenström TA. Antibiotic resistant superbugs: assessment of the interrelationship of occurrence in clinical settings and environmental niches. Molecules (2016) 22:E29. doi: 10.3390/molecules22010029
14. Adegoke AA, Stenström TA, Okoh AI. Stenotrophomonas maltophilia as an emerging ubiquitous pathogen: looking beyond contemporary antibiotic therapy. Front. Microbiol. (2017) 8:2276. doi: 10.3389/fmicb.2017.0227
15. Embrey M. Subpopulations susceptible to waterborne diseases are surprisingly diverse. J Am Water Works Assoc. (2003) 95:34. doi: 10.1002/j.1551-8833.2003.tb10307.x
16. Cornejo PK, Zhang Q, Mihelcic JR. How does scale of implementation impact the environmental sustainability of wastewater treatment integrated with resource recovery? Environ Sci Technol. (2016) 50:6680–9. doi: 10.1021/acs.est.5b05055
17. Verbyla ME, Oakley SM, Mihelcic JR. Wastewater infrastructure for small cities in an urbanizing world: integrating protection of human health and the environment with resource recovery and food security. Environ Sci Technol. (2013) 7:3598–605. doi: 10.1021/es3050955
18. Habbari K, Tifnouti A, Bitton G, Mandil A. Geohelminth infections associated with raw wastewater reuse for agricultural purposes in Beni-Mallal, Morocco. Parasitol Int. (2000) 48:249–54. doi: 10.1016/S1383-5769(99)00026-4
19. Pham-Duc P, Nguyen-Viet H, Hattendorf J, Cam PD, Zurbrügg C, Zinsstag J, et al. Diarrhoeal diseases among adult population in an agricultural community Hanam province, Vietnam, with high wastewater and excreta re-use. BMC Public Health (2014) 14:978. doi: 10.1186/1471-2458-14-978
20. Chary SN, Kamala CT, Raj DSS. Assessing risk of heavy metals from consuming food grown on sewage irrigated soils and food chain transfer. Ecotoxicol Environ Saf. (2008) 69:513–24. doi: 10.1016/j.ecoenv.2007.04.013
21. Trang DT, Hien BTT, Mølbak K, Cam PD, Dalsgaard A. Epidemiology and aetiology of diarrhoeal diseases in adults engaged in wastewater-fed agriculture and aquaculture in Hanoi, Vietnam. Trop Med Int Health (2007c) 12:23–33. doi: 10.1111/j.1365-3156.2007.01938.x
22. Anh VT, van der Hoek W, Ersbøll AK, Thuong NV, Tuan ND, Cam PD, et al. Dermatitis among farmers engaged in peri-urban aquatic food production in Hanoi, Vietnam. Trop Med Int Heal. (2007) 12:59–65. doi: 10.1111/j.1365-3156.2007.01942.x
23. Anh VT, van der Hoek W, Cam PD, Dalsgaard A. Perceived health problems among users of wastewater for aquaculture in Southeast Asian cities. Urban Water J. (2007) 4:269–74. doi: 10.1080/15730620701563405
24. Dickin SK, Schuster-Wallace CJ, Qadir M, Pizzacalla K. A review of health risks and pathways for exposure to wastewater use in agriculture. Environ Health Perspect. (2016) 124:900–9. doi: 10.1289/ehp.1509995
25. Cutolo SA, Piveli RP, Santos JG, Montes CR, Sundefeld G, Campos F, et al. Parasitological risk assessment from wastewater reuse for disposal in soil in developing countries. Water Sci Technol. (2012) 65:1357–67. doi: 10.2166/wst.2012.012
26. Navarro I, Jimenez B, Cifuentes E, Lucario S. Application of Helminth ova infection dose curve to estimate the risks associated with biosolid application on soil. J Water and Health (2009) 7:31–44.
27. Navarro I, Jiménez B, Cifuentes E, Lucario S. A quantitative microbial risk assessment of helminth ova in reusing sludge for agricultural production in developing countries. Risk Anal. (2008) 6:65–74. doi: 10.2166/wh.2009.113
28. O'Connor NA, Surapaneni A, Smith D, Stevens D. Occurrence and fate of Ascaris lumbricoides ova in biosolids in Victoria, Australia: a human health risk assessment of biosolids storage periods. Wat Sci Technol. (2017) 76:1332–46. doi: 10.2166/wst.2017.222
29. Kundu A, Poma HR, Jenkins MW, Rajal VB, Wuertz S. QMRA of intestinal nematode infection via multimedia exposure pathways. In: Ames DP, Quinn NWT, Rizzoli AE, editors. International Environmental Modelling and Software Society (iEMSs) 7th Intl. Congress on Env. Modelling and Software. San Diego, CA (2014).
30. Schönning C, Westrell T, Stenström TA, Arnbjerg-Nielsen K, Hasling AB, Høibye L, et al. Microbial risk assessment of local handling and use of human faeces. J Water Health. (2007) 5:117–28. doi: 10.2166/wh.2006.049
31. Seidu R, Heistad A, Amoah P, Drechsel P, Jenssen PD, Stenström TA. Quantification of the health risk associated with wastewater reuse in Accra, Ghana: a contribution toward local guidelines. J Water Health (2008) 6:461–71. doi: 10.2166/wh.2008.118
32. Jaramillo MF, Restrepo I. wastewater reuse in agriculture: a review about its limitations and benefits. Sustainability (2017) 9:1734–53. doi: 10.3390/su9101734
33. Van der Hoek W, De NV, Konradsen F, Cam PD, Hoa NTV, Toan ND, et al. Current status of soil-transmitted helminths in Vietnam. Southeast Asian, J. Trop. Med. Public Health (2003) 34(Suppl. 1):1–11.
34. World Health Organization. Guidelines for the Safe Use of Wastewater, Excreta and Greywater. Volume I–IV. Geneva: World Health Organization (2006a).
35. Cabral JPS. Water microbiology. Bacterial pathogens and water. Int J Environ Res Public Health (2010) 7:3657–703. doi: 10.3390/ijerph7103657
36. Sjölander I. Modeling the Decay of E. coli and Ascaris Suum in Wastewater Irrigated Vegetable: Implication for Microbial Health Risk Reduction. Master's thesis, Department of Mathematical Sciences and Technology, Norwegian University of Life Sciences (2012). Available online at: https://brage.bibsys.no/xmlui/bitstream/handle/11250/188919/masterthesisIngridS.pdf?sequence=1
37. Gatta G, Libutti A, Beneduce L, Gagliardi A, Disciglio G, Lonigro A, et al. Reuse of treated municipal wastewater for globe artichoke irrigation: assessment of effects on morpho-quantitative parameters and microbial safety of yield. Sci Hortic. (2016) 213:55–65. doi: 10.1016/j.scienta.2016.10.011
38. Truchado P, Hernandez N, Gil MI, Ivanek R, Allende A. Correlation between E. coli levels and the presence of foodborne pathogens in surface irrigation water: establishment of a sampling program. Water Res. (2018) 128:226–33. doi: 10.1016/j.watres.2017.10.041
39. De Sanctis M, Del Moro G, Chimienti S, Ritelli P, Levantesi C, Di Iaconi C. Removal of pollutants and pathogens by a simplified treatment scheme for municipal wastewater reuse in agriculture. Sci Total Environ. (2017) 580:17–25. doi: 10.1016/j.scitotenv.2016.12.002
40. Courault D, Albert I, Perelle S, Fraisse A, Renault P, Salemkour A, et al. Assessment and risk modeling of airborne enteric viruses emitted from wastewater reused for irrigation. Sci Total Environ. (2017) 592:512–26. doi: 10.1016/j.scitotenv.2017.03.105
41. Sidhu JPS, Sena K, Hodgers L, Palmer A, Toze S. Comparative enteric viruses and coliphage removal during wastewater treatment processes in a sub-tropical environment. Sci Tot Environ. (2018) 616–617:669–77. doi: 10.1016/j.scitotenv.2017.10.265
42. Cui J, Chen X, Nie M, Fang S, Tang B, Quan Z, et al. Effects of Spartina alterniflora invasion on the abundance, diversity, and community structure of sulfate reducing bacteria along a successional gradient of coastal salt marshes in China. Wetlands (2017) 37:221–32. doi: 10.1007/s13157-016-0860-6
43. Palese AM, Pasquale V, Celano G, Figliuolo G, Masi S, Xiloyannis C. Irrigation of olive groves in Southern Italy with treated municipal wastewater: effects on microbiological quality of soil and fruits. Agric Ecosyst Environ. (2009) 129:43–51. doi: 10.1016/j.agee.2008.07.003
44. Orlofsky E, Bernstein N, Sacks M, Vonshak A, Benami M, Kundu A, et al. Comparable levels of microbial contamination in soil and on tomato crops after drip irrigation with treated wastewater or potable water. Agric Ecosyst Environ. (2016) 215:140–50. doi: 10.1016/j.agee.2015.08.008
45. Xiao S, Hu S, Zhang Y, Zhao X, Pan W. Influence of sewage treatment plant effluent discharge into multipurpose river on its water quality: a quantitative health risk assessment of Cryptosporidium and Giardia. Environ Pollut. (2018) 233:797–805. doi: 10.1016/j.envpol.2017.11.010
46. Al-Lahham O, El Assi NM, Fayyad M. Impact of treated wastewater irrigation on quality attributes and contamination of tomato fruit. Agric Water Manag. (2003) 6151–62. doi: 10.1016/S0378-3774(02)00173-7
47. Libutti A, Gatta G, Gagliardi A, Vergine P, Pollice A, Beneduce L, et al. Agro-industrial wastewater reuse for irrigation of a vegetable crop succession under Mediterranean conditions. Agric Water Manag. (2018) 196:1–14. doi: 10.1016/j.agwat.2017.10.015
48. Decol LT, Casarin LS, Hessel CT, Batista ACF, Allende A, Tondo EC. Microbial quality of irrigation water used in leafy green production in Southern Brazil and its relationship with produce safety. Food Microbiol. (2017) 65:105–13. doi: 10.1016/j.fm.2017.02.003
49. Gupta N, Khan DK, Santra SC. Prevalence of intestinal helminth eggs on vegetables grown in wastewater-irrigated areas of Titagarh, West Bengal, India. Food Control (2009) 20:942–5. doi: 10.1016/j.foodcont.2009.02.003
50. Domenech E, Amorós I, Moreno Y, Alonso JL. Cryptosporidium and Giardia safety margin increase in leafy green vegetables irrigated with treated wastewater. Int J Hyg Environ Health (2018) 221:112–9. doi: 10.1016/j.ijheh.2017.10.009
51. Thurston-Enriquez JA, Watt P, Dowd SE, Enriquez R, Pepper IL, Gerba CP. Detection of protozoan parasites and microsporidia in irrigation waters used for crop production. J Food Prot. (2002) 65:378–82. doi: 10.4315/0362-028X-65.2.378
52. Silverman AI, Akrong MO, Amoah P, Drechsel P, Nelson KL. Quantification of human norovirus GII, human adenovirus, and fecal indicator organisms in wastewater used for irrigation in Accra, Ghana. J Water Health (2013) 11:473–88. doi: 10.2166/wh.2013.025
53. Chaidez C, Soto M, Gortares P, Mena K. Occurrence of Cryptosporidium and Giardia in irrigation water and its impact on the fresh produce industry. Int J Environ Health Res. (2005) 15:339–45. doi: 10.1080/09603120500289010
54. Bailey ES, Casanova LM, Simmons OD, Sobsey MD. Tertiary treatment and dual disinfection to improve microbial quality of reclaimed water for potable and non-potable reuse: a case study of facilities in North Carolina. Sci Total Environ. (2018) 630:379–88. doi: 10.1016/j.scitotenv.2018.02.239
55. Moazeni M, Nikaeen M, Hadi M, Moghim S, Mouhebat L, Hatamzadeh M, et al. Estimation of health risks caused by exposure to enteroviruses from agricultural application of wastewater effluents. Water Res. (2017) 125:104–13. doi: 10.1016/j.watres.2017.08.028
56. Skosana GG, du Preez HH. Assessment of the viability of the reuse of Sedibeng District municipal secondary effluent in Southern Gauteng, South Africa. J Water Resource Prot. (2017) 9:1043–61. doi: 10.4236/jwarp.2017.98069
57. Corzo B, de la Torre T, Sans C, Escorihuela R, Navea S, Malfeito JJ. Long-term evaluation of a forward osmosis-nanofiltration demonstration plant for wastewater reuse in agriculture. Chem Eng J. (2018) 338:383–91. doi: 10.1016/j.cej.2018.01.042
58. Elfanssi S, Ouazzani N, Mandi L. Soil properties and agro-physiological responses of alfalfa (Medicago sativa L.) irrigated by treated domestic wastewater. Agric Water Manage. (2018) 202:231–40. doi: 10.1016/j.agwat,.2018.02.003
59. Lugoli F, Leopizzi MI, Bagordo F, Grassi T, Guido M, De Donno A. Widespread microbiological groundwater contamination in the South-eastern Salento (Puglia-Italy). J Environ Monit. (2011) 13:192–200. doi: 10.1039/C0EM00193G
60. Shuval HI, Adin A, Fattal B, Rawitz E, Yekutiel P. Wastewater Irrigation in Developing Countries: Health Effects and Technical Solutions. Washington, DC: World Bank (1986).
61. Rojas-Valencia MN, Mier MV. Analysis of allergenic bioaerosols distributed through sprinkling irrigation systems and mitigating strategies. In: Méndez-Vilas A, editor. Microbial Pathogens and Strategies for Combating Them: Science, Technology and Education. Badajoz: Formatex (2013). p. 1439–47.
62. Dalahmeh SS, Lalander C, Pell M, Vinnerås B, Jönsson H. Quality of greywater treated in biochar filter and risk assessment of gastroenteritis due to household exposure during maintenance and irrigation. J Appl Microbiol. (2016) 121:1427–43. doi: 10.1111/jam.13273
63. Pham-Duc P, Nguyen-Viet H, Hattendorf J, Zinsstag J, Phung-Dac C, Zurbrügg C, et al. Ascaris lumbricoides and Trichuris trichiura infections associated with wastewater and human excreta use in agriculture in Vietnam. Parasitol Int. (2013) 62:172–80. doi: 10.1016/j.parint.2012.12.007
64. Pavione DM, Bastos RK, Bevilacqua PD. Quantitative microbial risk assessment applied to irrigation of salad crops with waste stabilization pond effluents. Water Sci Technol. (2013) 67:1208–15. doi: 10.2166/wst.2013.674
65. Tserendorj A, Anceno AJ, Houpt ER, Icenhour CR, Sethabutr O, Mason CS, et al. Molecular techniques in ecohealth research toolkit: facilitating estimation of aggregate gastroenteritis burden in an irrigated periurban landscape. Ecohealth (2011) 8:349–64. doi: 10.1007/s10393-011-0724-8
66. Daley K, Jamieson R, Rainham D, Truelstrup HL. Wastewater treatment and public health in Nunavut: a microbial risk assessment framework for the Canadian Arctic. Environ Sci Pollut Res Int. (2017) 25:32860–72. doi: 10.1007/s11356-017-8566-8
67. Gumbo JR, Malaka EM, Odiyo JO, Nare L. The health implications of wastewater reuse in vegetable irrigation: a case study from Malamulele, South Africa. Int J Environ Health Res (2010) 20:201–11. doi: 10.1080/09603120903511093
68. Ensink JH, Blumenthal UJ, Brooker S. Wastewater quality and the risk of intestinal nematode infection in sewage farming families in Hyderabad, India. Am J Trop Med Hyg. (2008) 79:561–7. doi: 10.4269/ajtmh.2008.79.561
69. Yajima A, Kurokura H. Microbial risk assessment of livestock-integrated aquaculture and fish handling in Vietnam. Fish Sci. (2008) 74:1062–8. doi: 10.1111/j.1444-2906.2008.01625.x
70. Hien BTT, Do TT, Scheutz F, Cam PD, Mølbak K, Dalsgaard A. Diarrhoeagenic Escherichia coli and other causes of childhood diarrhoea: a case-control study in children living in a wastewater-use area in Hanoi, Vietnam. J Med Microbiol. (2007) 56:1086–96. doi: 10.1099/jmm.0.47093-0
71. Ensink JH, van der Hoek W, Mukhtar, M, Tahir, Z, Amerasinghe FP. High risk of hookworm infection among wastewater farmers in Pakistan. Trans R Soc Trop Med Hyg. (2005) 99:809–18. doi: 10.1016/j.trstmh.2005.01.005
72. Trang DT, van der Hoek W, Cam PD, Vinh KT, Hoa VN, Dalsgaard A. Low risk for helminth infection in wastewater-fed rice cultivation in Vietnam. J Water Health (2006) 4:321–31. doi: 10.2166/wh.2006.013
73. Olsen A, Thuan LK, Murrell KD, Dalsgaard A, Johansen MV, De NV. Cross-sectional parasitological survey for helminth infections among fish farmers in Nghe An Province, Vietnam. Acta Trop. (2006) 100:199–204. doi: 10.1016/j.actatropica.2006.10.010
74. Amahmid O, Bouhoum K. Assessment of the health hazards associated with wastewater reuse: transmission of geohelminthic infections (Marrakech, Morocco). Int J Environ Health Res. (2005) 15:127–33. doi: 10.1080/09603120500062037
75. Verle P, Kongs A, De NV, Thieu NQ, Depraetere K, Kim HT, et al. Prevalence of intestinal parasitic infections in northern Vietnam. Trop Med Int Health (2003) 8:961–4. doi: 10.1046/j.1365-3156.2003.01123.x
76. Keraita B, Amoah P. Fecal Exposure Pathways in Accra: A Literature Review with Specific Focus on IWMI's Work on Wastewater Irrigated Agriculture (IWMI). Literature Review Report. Submitted to the Centre for Global Safe Water, Emory University (2011).
77. Blumenthal UJ, Cifuentes E, Bennett S, Quigley M, Ruiz-Palacios G. The risk of enteric infections associated with wastewater reuse: the effect of season and degree of storage of wastewater. Trans R Soc Trop Med Hyg. (2001) 95:131–7. doi: 10.1016/S0035-9203(01)90136-1
78. Fattal B, Bercovier H, Derai-Cochin M, Shuval HI. Wastewater reuse and exposure to Legionella organisms. Water Resour. (1985) 19:693–6.
79. Fattal B, Margalith M, Shuval HI, Wax Y, Morag A. Viral antibodies in agricultural populations exposed to aerosols from wastewater irrigation during a viral disease outbreak. Am J Epidemiol. (1987) 125:899–906. doi: 10.1093/oxfordjournals.aje.a114607
80. Margalith M, Morag A, Fattal B. Antibodies to polioviruses in an Israeli population and overseas volunteers. J Med Virol. (1990) 30:68–72. doi: 10.1002/jmv.1890300115
81. Rodríguez-Lázaro D, Cook N, Ruggeri FM, Sellwood J, Nasser A, Nascimento MSJ, et al. Virus hazards from food, water and other contaminated environments. FEMS Microbiol Rev. (2012) 36:786–814. doi: 10.1111/j.1574-6976.2011.00306.x
82. Li YP, Yang LW, Meng QL, Qiu XH, Feng YJ. Emission characteristics of microbial aerosols in a municipal sewage treatment plant in Xi'an, China. Aerosol Air Qual Res. (2013) 13:343–9. doi: 10.4209/aaqr.2012.05.0123
83. Agunwamba JC. Analysis of socioeconomic and environmental impacts of waste stabilization pond and unrestricted wastewater irrigation: interface with maintenance. Environ Manage. (2001) 27:463–76. doi: 10.1007/s002670010162
84. Teltsch B, Katzenelson E. Airborne enteric bacteria and viruses from spray irrigation with wastewater. Appl Environ Microbiol. (1978) 35:290–6.
85. Courault D, Girardin G, Capowiez L, Albert I, Krawczyk C, Ball C, et al. Bioaerosol Dispersion in Relation With Wastewater Reuse for Crop Irrigation. Fall Meeting, Abstract id. H23R-04, Am Geophys Union (2014).
86. Girardin G, Renault P, Bon F, Capowiez L, Chadoeuf J, Krawczyk C, et al. Viruses carried to soil by irrigation can be aerosolized later during windy spells Agron. Sustain Dev. (2016) 36:59. doi: 10.1007/s13593-016-0393-7
87. Paez-Rubio T, Viau E, Romero-Hernandez S, Peccia J. Source bioaresol and rRNA gene-based identification of microoganisms aerosolized at a flood irrigation wastewater reuse site. Appl Environ Microbiol. (2005) 71:804–10. doi: 10.1128/AEM.71.2.804-810.2005
88. Carlander A, Schönning C, Stenström TA. Energy forest irrigated with wastewater: a comparative microbial risk assessment. J Water Health (2009) 7:413–33. doi: 10.2166/wh.2009.020
89. Ayuso-Gabella N, Page D, Masciopinto C, Aharoni A, Salgot M, Wintgens T. Quantifying the effect of Managed Aquifer Recharge on the microbiological human health risks of irrigating crops with recycled water. Agric Water Manag. (2011) 99:93–102. doi: 10.1016/j.agwat.2011.07.014
90. Oliveira M, Viñas I, Usall J, Anguera M, Abadias M. Presence and survival of Escherichia coli O157:H7 on lettuce leaves and in soil treated with contaminated compost and irrigation water. Int J Food Microbiol. (2012) 156:133–40. doi: 10.1016/j.ijfoodmicro.2012.03.014
91. Oliveira M, Viñas I, Usall J, Anguera M, Gatius F, Abadias M. Microbiological quality of fresh lettuce from organic and conventional production. Food Microbiol. (2010) 27:679–84. doi: 10.1016/j.fm.2010.03.008
92. Kroupitski Y, Pinto R, Belausov E, Sela S. Distribution of Salmonella typhimurium in romaine lettuce leaves. Food Microbiol. (2011) 28:990–7. doi: 10.1016/j.fm.2011.01.007
93. Viswanathan P, Kaur R. Prevalence and growth of pathogens on salad vegetables, fruits and sprouts. J Food Prot. (2001) 203:205–13. doi: 10.1078/S1438-4639(04)70030-9
94. Ziuzina D, Han L, Cullen PJ, Bourke P. Cold plasma inactivation of internalised bacteria and biofilms for Salmonella enterica serovar Typhimurium, Listeria monocytogenes and Escherichia coli. Int J Food Microbiol. (2015) 210:53–61. doi: 10.1016/j.ijfoodmicro.2015.05.019
95. Wang J, Kim KH, Kim SK, Kim YS, Li QX, Jun S. Simple quantitative analysis of Escherichia coli K-12 internalized in baby spinach using Fourier Transform Infrared spectroscopy. Int J Food Microbiol. (2010) 144:147–51. doi: 10.1016/j.ijfoodmicro.2010.09.013
96. Hirneisen KA, Kniel KE. Inactivation of internalized and surface contaminated enteric viruses in green onions. Int J Food Microbiol. (2013) 166:201–6. doi: 10.1016/j.ijfoodmicro.2013.07.013
97. Hou Z, Fink RC, Radtke C, Sadowsky MJ, Diez-Gonzalez F. Incidence of naturally internalized bacteria in lettuce leaves. Int J Food Microbiol. (2013) 162:260–5. doi: 10.1016/j.ijfoodmicro.2013.01.027
98. Bin Z, Luo Y, Bauchan G, Feng H, Stommel J. Visualizing pathogen internalization pathways in fresh tomatoes using MicroCT and Confocal laser scanning microscopy. Food Control (2017) 85:276–82. doi: 10.1016/j.foodcont.2017.09.027
99. Beuchat LR. Ecological factors influencing survival and growth of human pathogens on raw fruits and vegetables. Microb Infect. (2002) 4:413–23. doi: 10.1016/S1286-4579(02)01555-1
100. Barak JD, Gorski L, Naraghi-Arani P, Charkowski AO. Salmonella enterica virulence genes are required for bacterial attachment to plant tissue. Appl Environ Microbiol. (2005) 71:5685–91. doi: 10.1128/AEM.71.10.5685-5691.2005
101. Berger CN, Shaw RK, Brown DJ, Mather H, Clare S, Dougan G, et al. Interaction of Salmonella enterica with basil and other salad leaves. ISME J. (2009) 3:261–5. doi: 10.1038/ismej.2008.95
102. Gibson DL, White AP, Snyder SD, Martin S, Heiss C, Azadi P, et al. Salmonella produces an O-antigen capsule regulated by AgfD and important for environmental persistence. J Bacteriol. (2006) 188:7722–30. doi: 10.1128/JB.00809-06
103. Jonas K, Tomenius H, Kader A, Normark S, Romling U, Belova LM, et al. Roles of curli, cellulose and BapA in Salmonella biofilm morphology studied by atomic force microscopy. BMC Microbiol. (2007) 7:70. doi: 10.1186/1471-2180-7-70
104. Ethelberg S, Lisby M, Böttiger B, Schultz AC, Villif A, Jensen T, et al. Outbreaks of gastroenteritis linked to lettuce, Denmark, January 2010. Euro Surveill. (2010) 15:19484.
105. Antwi-Agyei P, Peasey A, Biran A, Bruce J, Ensink J. Risk perceptions of wastewater use for urban agriculture in Accra, Ghana. PLoS ONE (2016) 11:e0150603. doi: 10.1371/journal.pone.0150603
106. Jung Y, Jang H, Matthews KR. Effect of the food production chain from farm practices to vegetable processing on outbreak incidence. Microb Biotechnol. (2014) 7:517–27. doi: 10.1111/1751-7915.12178
107. Sivapalasingam S, Friedman CR, Cohen L, Tauxe RV. Fresh produce: a growing cause of outbreaks of foodborne illness in the United States, 1973 through 1997. J Food Prot. (2004) 67:2342–53. doi: 10.4315/0362-028X-67.10.2342
108. Gupta SK, Nalluswami K, Snider C, Perch M, Balasegaram M, Burmeister D, et al. Outbreak of Salmonella Braenderup infections associated with Roma tomatoes, northeastern United States, 2004: a useful method for subtyping exposures in field investigations. Epidemiol Infect. (2007) 135:1165–73. doi: 10.1017/S0950268807007911
109. Greene SK, Daly ER, Talbot EA, Demma LJ, Holzbauer S, Patel NJ, et al. Recurrent multistate outbreak of Salmonella Newport associated with tomatoes from contaminated fields, 2005. Epidemiol Infect. (2008) 136:157–65. doi: 10.1017/S095026880700859X
110. Friesema I, Sigmundsdottir G, van der Zwaluw K, Heuvelink A, Schimmer B, de Jager C, et al. An international outbreak of Shiga toxin-producing Escherichia coli O157 infection due to lettuce, September–October 2007. Euro Surveill. (2008) 13:19065. doi: 10.2807/ese.13.50.19065-en
111. Grant J, Wendelboe AM, Wendel A, Jepson B, Torres P, Smelser C, et al. Spinach-associated Escherichia coli O157:H7 outbreak, Utah and New Mexico, 2006. Emerg Infect Dis. (2008) 14:1633–6. doi: 10.3201/eid1410.071341
112. Söderström A, Österberg P, Lindqvist A, Jonsson B, Lindberg A, Blide Ulander S, et al. A large Escherichia coli O157 outbreak in Sweden associated with locally produced lettuce. Foodborne Pathog Dis. (2008) 5:339–49. doi: 10.1089/fpd.2007.0065
113. Wendel AM, Johnson DH, Sharapov U, Grant J, Archer JR, Monson T, et al. Multistate outbreak of Escherichia coli O157:H7 infection associated with consumption of packaged spinach, August–September 2006: the wisconsin investigation. Clin Infect Dis. (2009) 48:1079–86. doi: 10.1086/597399
114. Falkenhorst G, Krusell L, Lisby M, Madsen SB, Bottiger B, and Mølbak K Imported frozen raspberries cause a series of norovirus outbreaks in Denmark. Eurosurveillance (2005) 10:10.
115. Wang SK, Wang ZG, Zhang XH, Yang B, Wu Q, Kan B, et al. An outbreak of paratyphoid fever in a county of Yunnan province, 2010–2011. Zhonghua Liu Xing Bing Xue Za Zhi (2017) 38:200–4. doi: 10.3760/cma.j.issn.0254-6450.2017.02.013
116. Edelstein M, Sundborger C, Hergens MP, Ivarsson S, Dryselius R, Insulander M, et al. Barriers to trace-back in a salad-associated EHEC outbreak, Sweden, June 2013. PLoS Curr. (2014) 6. doi: 10.1371/currents.outbreaks.80bbab3af3232be0372ea0e904dcd1fe
117. Nygard K, Lassen J, Vold L, Andersson Y, Fisher I, Lofdahl S, et al. Outbreak of Salmonella thompson infections linked to imported rucola lettuce. Foodborne Pathog Dis. (2008) 5:165–73. doi: 10.1089/fpd,.2007.0053
118. Hoang LM, Fyfe M, Ong C, Harb J, Champagne S, Dixon B, et al. Outbreak of cyclosporiasis in British Columbia associated with imported Thai basil. Epidemiol Infect. (2005) 133:23–7. doi: 10.1017/S0950268804003176
119. Doller PC, Dietrich K, Filipp N, Brockmann S, Dreweck C, Vonthein R, et al. Cyclosporiasis outbreak in Germany associated with the consumption of salad. Emerg Infect Dis. (2000) 8:992–4. doi: 10.3201/eid0809.010517
120. Barrimah E, Salem KA, Gabal MS. An outbreak of hepatitis A associated with treated waste water used for irrigation. J Egypt Public Health Assoc. (1999) 74:227–39.
121. Makkaew P, Miller M, Fallowfield HJ, Cromar NJ. Microbial risk in wastewater irrigated lettuce: comparing Escherichia coli contamination from an experimental site with a laboratory approach. Water Sci Technol. (2016) 74:749–55. doi: 10.2166/wst.2016.237
122. Antwi-Agyei P, Cairncross S, Peasey A, Price V, Bruce J, Baker K, et al. A farm to fork risk assessment for the use of wastewater in agriculture in Accra, Ghana. PLoS ONE (2015) 10:e0142346. doi: 10.1371/journal.pone.0142346
123. Bouwknegt M, Verhaelen K, Rzezutka A, Kozyra I, Maunula L, von Bonsdorff C-H, et al. Quantitative farm-to-fork risk assessment model for norovirus and hepatitis A virus in European leafy green vegetable and berry fruit supply chains. Int J Food Microbiol. (2015) 198:50–8. doi: 10.1016/j.ijfoodmicro.2014.12.013
124. Zaibel I, Zilberg D, Groisman L, Arnon S. Impact of treated wastewater reuse and floods on water quality and fish health within a water reservoir in an arid climate. Sci Total Environ. (2016) 559:268–81. doi: 10.1016/j.scitotenv.2016.03.099
125. Klutse CK. Microbial Analysis of Soil Samples in a Wastewater Irrigated Vegetable Production Site: Case Study at Atonsu, Kumasi. M. Sc thesis, Department of Theoretical and Applied Biology, KNUST, Kumasi (2009).
126. Antwi-Agyei P, Biran A, Peasey A, Bruce J, Ensink J. A faecal exposure assessment of farm workers in Accra, Ghana: a cross sectional study. BMC Public Health (2016) 16:587. doi: 10.1186/s12889-016-3266-8
127. Matheyarasu R, Bolan NS, Naidu R. Abattoir Wastewater Irrigation Increases the Availability of Nutrients and Influences on Plant Growth and Development. Water Air Soil Pollut. (2016) 227:253–69. doi: 10.1007/s11270-016-2947-3
128. Fuhrimann S, Pham-Duc P, Cissé G, Tram NT, Thu Ha H, Dung do T, et al. (Microbial contamination along the main open wastewater and storm water channel of Hanoi, Vietnam, and potential health risks for urban farmers. Sci Total Environ. (2016) 566–567:1014–22. doi: 10.1016/j.scitotenv.2016.05.080
129. Cairncross S. Health impacts in developing countries: new evidence and new prospects. J Inst Water Environ Manage. (1990) 4:571–7. doi: 10.1111/j.1747-6593.1990.tb01471.x
130. Cairncross S, Blumenthal U, Kolsky P, Moraes L, Tayeh A. The public and domestic domains in the transmission of disease. Trop Med Int Health. (1996) 1:27–34. doi: 10.1046/j.1365-3156.1996.d01-9.x
131. Jensen PK, Ensink JHJ, Jayasinghe G, Van Der Hoek W, Cairncross S, Dalsgaard A. Domestic transmission routes of pathogens: the problem of in-house contamination of drinking water during storage in developing countries. Trop Med Int Health (2002) 7:604–9. doi: 10.1046/j.1365-3156.2002.00901.x
132. Blumenthal UJ, Mara DD, Peasey A, Ruiz-Palacios G, Stott R. Guidelines for the microbiological quality of treated wastewater used in agriculture: recommendations for revising WHO guidelines. Bull World Health Organ. (2000) 78:1104–16.
133. Yang X, Shang C, Huang J-C. DBP formation in breakpoint chlorination of wastewater. Water Res. (2005) 39:4755–67. doi: 10.1016/j.watres.2005.08.033
134. Mara DD, Sleigh PA, Blumenthal UJ, Carr RM. Health risks in wastewater irrigation: comparing estimates from quantitative microbial risk analyses and epidemiological studies. J Water Health (2007) 5:39–50. doi: 10.2166/wh.2006.055
135. Poole CJM, Wong M. Allergic bronchopulmonary aspergillosis in garden waste (compost) collectors—occupational implications. Occup Med. (2013) 63:517–9. doi: 10.1093/occmed/kqt097
136. Melloul AA, Hassani L. Salmonella infection in children from the wastewater-spreading zone of Marrakesh city (Morocco). J Appl Microbiol. (1999) 87:536–9. doi: 10.1046/j.1365-2672.1999.00847.x
137. Nguyen PH, Nguyen KC, Nguyen TD, Le MB, Bern C, Flores R, et al. Intestinal helminth infections among reproductive age women in Vietnam: prevalence, co-infection and risk factors. Southeast Asian J Trop Med Public Health (2006) 37:865–74.
138. Trang DT, Mølbak K, Cam PD, Dalsgaard A. Helminth infections among people using wastewater and human excreta in peri-urban agriculture and aquaculture in Hanoi, Vietnam. Trop Med Int Health (2007d) 12:82–90. doi: 10.1111/j.1365-3156.2007.01945.x
139. Fuhrimann S, Winkler MS, Kabatereine NB, Tukahebwa EM, Halage AA, Rutebemberwa E, et al. Risk of intestinal parasitic infections in people with different exposures to wastewater and fecal sludge in Kampala, Uganda: a cross-sectional study. PLoS Negl Trop Dis. (2016) 10:e0004469. doi: 10.1371/journal.pntd.0004469
140. Srikanth R, Naik D. Health effects of wastewater reuse for agriculture in the suburbs of Asmara City, Eritrea. Int J Occup Environ Health (2004) 10:284–8. doi: 10.1179/oeh.2004.10.3.284
141. Verbyla ME, Iriarte MM, Mercado Guzmán A, Coronado O, Almanza M, Mihelcic Jr. Pathogens and fecal indicators in waste stabilization pond systems with direct reuse for irrigation: fate and transport in water, soil and crops. Sci Total Environ. (2016) 551–552:429–437. doi: 10.1016/j.scitotenv.2016.01.159
142. Mara DD, Feachem RGA. Water- and excreta-related diseases: unitary environ- mental classification. J Environ Eng. (1999) 125:334–9. doi: 10.1061/(ASCE)0733-9372(1999)125:4(334)
143. Tariq M, Muzammil SM, Shaikh FA, Pal KM. Hookworm infestation as a cause of melena and severe anaemia in farmer. J Pak Med Assoc. (2017) 67:327–9.
144. Carducci A, Donzelli G, Cioni L, Verani M. Quantitative microbial risk assessment in occupational settings applied to the airborne human. Int. J. Environ. Res. Public Health (2016) 13:733. doi: 10.3390/ijerph13070733
145. Haas CN, Rose JB, Gerba CP. Quantitative Microbial Risk Assessment. 2nd Edn. New York, NY: John Wiley and Sons. (2014). doi: 10.1002/9781118910030
146. Beaudequin D, Harden F, Roiko A, Stratton H, Lemckert C, Mengersen K. Beyond QMRA: modelling microbial health risks as a complex system using Bayesian networks. Environ Int. (2015) 80:8–18. doi: 10.1016/j.envint.2015.03.013
147. CAMRA-Center for Advancing Microbial Risk Assessment. Michigan State University, East Lansing, Michigan. (2013). Available online at: http://qmrawiki.canr.msu.edu/index.php/Quantitative_Microbial_Risk_Assessment_(QMRA)_Wiki (Accessed July 12, 2017).
148. Havelaar AH. QMRA — a framework for assessing microbiological public health risks. In: The European College of Veterinary Public Health Annual Scientific Conference. Maastricht: Dutch National Institute for Public Health and the Environment (RIVM) and Utrecht University (2012).
149. Amha YM, Kumaraswamy R, Ahmad F. A probabilistic QMRA of Salmonella in direct agricultural reuse of treated municipal wastewater. Water Sci Technol. (2015) 71:1203–11. doi: 10.2166/wst.2015.093
150. Navarro I, Jiménez B. Evaluation of the WHO helminth eggs criteria using a QMRA approachfor the safe reuse of wastewater and sludge in developing countries. Water Sci. Technol. (2011) 63:1499–505 doi: 10.2166/wst.2011.394
151. World Health Organization. Guidelines for the Safe Use of Excreta Wastewater and Greywater: Wastewater and Excreta Use in Aquaculture. Volume 3. Geneva: World Health Organisation (2006b).
152. NRMMC. Guidelines for Sewage Systems, Biosolids Management. Canberra, ACT: Natural Resource Management Ministerial Council (NRMMC) (2004).
153. NRMMC EPHC AHMC. Australian guidelines for water recycling: managing health and environmental risks (Phase 1). In: Environment Protection and Heritage Council Australian Health Ministers' Conference. Canberra, ACT: Natural Resource Management Ministerial Council (2006).
154. Schoen ME, Garland J. Review of pathogen treatment reductions for onsite non-potable reuse of alternative source waters. Microbial Risk Analysis (2017) 5:25–31. doi: 10.1016/j.mran.2015.10.001
155. Mok HF, Barker SF, Hamilton AJ. A probabilistic quantitative microbial risk assessment model of norovirus disease burden from wastewater irrigation of vegetables in Shepparton, Australia. Water Res. (2014) 54:347–62. doi: 10.1016/j.watres.2014.01.060
156. Owusu-Ansah EDJ, Sampson A, Amponsah SK, Abaidoo RC, Dalsgaard A, Hald T. Probabilistic quantitative microbial risk assessment model of norovirus from wastewater irrigated vegetables in Ghana using genome copies and fecal indicator ratio conversion for estimating exposure dose. Sci Total Environ. (2017) 601–602:1712–9. doi: 10.1016/j.scitotenv.2017.05.168
157. Szekeres E, Baricz A, Chiriac CM, Farkas A, Opris O, Soran ML, et al. Abundance of antibiotics, antibiotic resistance genes and bacterial community composition in wastewater effluents from different Romanian hospitals. Environ Pollut. (2017) 225:304–15. doi: 10.1016/j.envpol.2017.01.054
158. Chen J, Ying GG, Wei XD, Liu YS, Liu SS, Hu LX, et al. Removal of antibiotics and antibiotic resistance genes from domestic sewage by constructed wetlands: effect of flow configuration and plant species. Sci Total Environ. (2016) 571:974–82. doi: 10.1016/j.scitotenv.2016.07.085
159. Novo A, Manaia CM. Factors influencing antibiotic resistance burden in municipal wastewater treatment plants. Appl Microbiol Biotechnol. (2010) 87:1157–66. doi: 10.1007/s00253-010-2583-6
160. Łuczkiewicz A, Jankowska K, Fudala-Ksiazek S, Olanczuk-Neyman K. Antimicrobial resistance of fecal indicators in municipal wastewater treatment plant. Water Res. (2010) 44:5089–97. doi: 10.1016/j.watres.2010.08.007
161. Kümmerer K. Antibiotics in the aquatic environment —A review—Part II. Chemosphere (2009) 75:435–41. doi: 10.1016/j.chemosphere.2008.12.006
162. Szczepanowski R, Linke B, Krahn I, Gartemann KH, Gützkow T, Eichler W, et al. Detection of 140 clinically relevant antibiotic-resistance genes in the plasmid metagenome of wastewater treatment plant bacteria showing reduced susceptibility to selected antibiotics. Microbiology (2009) 155(Pt 7):2306–19. doi: 10.1099/mic.0.028233-0
163. Li X, Zheng W, Machesky ML, Yates SR, Katterhenry M. Degradation kinetics and mechanism of antibiotic ceftiofur in recycled water derived from a beef farm. J Agric Food Chem. (2011) 59:10176–81. doi: 10.1021/jf202325c
164. Zhang T, Zhang XX, Ye L. Plasmid metagenome reveals high levels of antibiotic resistance genes and mobile genetic elements in activated sludge. PLoS ONE (2011) 6:e26041. doi: 10.1371/journal.pone.0026041
165. Gao T, Chen R, Wang X, Ngo HH, Li YY, Zhou J, et al. Application of disease burden to quantitative assessment of health hazards for a decentralized water reuse system. Sci Total Environ. (2016) 551–552:83–91. doi: 10.1016/j.scitotenv.2016.01.210
166. Verlicchi P, Aukidy M, Al Zambello E. Occurrence of pharmaceutical compounds in urban wastewater: removal, mass load and environmental risk after a secondary treatment—a review. Sci. Total Environ. (2012) 429:123–55. doi: 10.1016/j.scitotenv.2012.04.028
167. Michael I, Rizzo L, McArdell CS, Manaia CM, Merlin C, Schwartz T, et al. Urban waste water treatment plants as hotspots for the release of antibiotics in the environment: a review. Water Res. (2013) 47:957–93. doi: 10.1016/j.watres.2012.11.027
168. Zhang H, Li X, Yang Q, Sun L, Yang X, Zhou M, et al. Plant growth, antibiotic uptake, and prevalence of antibiotic resistance in an endophytic system of pakchoi under antibiotic exposure. Int J Environ Res Public Health (2017) 14:E1336. doi: 10.3390/ijerph14111336
169. Ziembinska-Buczynska A, Felis E, Folkert J, Meresta A, Stawicka D, Gnida A, et al. (2015). Detection of antibiotic resistance genes in wastewater treatment plant—Molecular and classical approach. Arch Environ Prot. 41, 23–32. doi: 10.1515/aep-2015-0035
170. Novo A, André S, Viana P, Nunes OC, Manaia CM. Antibiotic resistance, antimicrobial residues and bacterial community composition in urban wastewater. Water Res. (2013) 47:1875–87. doi: 10.1016/j.watres.2013.01.010
171. Minh TB, Leung HW, Loi IH, Chan WH, So MK, Mao JQ, et al. Antibiotics in the Hong Kong metropolitan area: ubiquitous distribution and fate in Victoria Harbour. Mar Pollut Bull. (2009) 58:1052–62. doi: 10.1016/j.marpolbul.2009.02.004
172. Gulkowska A, Leung HW, So MK, Taniyasu S, Yamashita N, Yeung LW, et al. Removal of antibiotics from wastewater by sewage treatment facilities in Hong Kong and Shenzhen, China. Water Res. (2008) 42:395–403. doi: 10.1016/j.watres.2007.07.031
173. Watkinson AJ, Murby EJ, Kolpin DW, Costanzo SD. The occurrence of antibiotics in an urban watershed: from wastewater to drinking water. Sci Total Environ. (2009) 407:2711–23. doi: 10.1016/j.scitotenv.2008.11.059
174. Li D, Yang M, Hu J, Zhang J, Liu R, Gu X, et al. Antibiotic-resistance profile in environmental bacteria isolated from penicillin production wastewater treatment plant and the receiving river. Environ Microbiol. (2009) 11:1506–17. doi: 10.1111/j.1462-2920.2009.01878.x
175. Zuccato E, Castiglioni S, Fanelli R. Identification of the pharmaceuticals for human use contaminating the Italian aquatic environment. J Hazard Mater. (2005) 122:205–9. doi: 10.1016/j.jhazmat.2005.03.001
176. Ternes TA, Hirsch R. Occurrence and behavior of X-ray contrast media in sewage facilities and the aquatic environment. Environ Sci Technol. (2000) 34:2741–8. doi: 10.1021/es991118m
177. Berto J, Rochenbach GC, Barreiros MAB, Correa AXR, Peluso-Silva S, Radetski CM. Physicochemical microbiological, and ecotoxicological evaluation of a septic tank/Fenton reaction combination for the treatment of hospital wastewaters. Ecotoxicol Environ Saf. (2009) 72:1076–81. doi: 10.1016/j.ecoenv.2008.12.002
178. Aguera A, Bayona JM, Cytryn E, Fotopoulos V, Lambropoulou D, Fatta-Kassinos D. The potential implications of reclaimed wastewater reuse for irrigation on the agricultural environment: the knowns and unknowns of the fate of antibiotics and antibiotic resistant bacteria and resistance genes - A review. Water Res. (2017) 123:448–467. doi: 10.1016/j.watres.2017.07.004
179. Pan M, Wong CKC, Chu LM. Distribution of antibiotics in wastewater-irrigated soils and their accumulation in vegetable crops in the pearl river delta Southern China. J Agri Food Chem. (2014) 62:11062–9. doi: 10.1021/jf503850v
180. Franklin AM. Analysis of Four Human Antibiotics and Antibiotic Resistant Bacteria in Water and Soils Impacted by Wastewater Treatment Plant Effluent. College of Agricultural Sciences, The Graduate School, The Pennsylvania State University (2015).
181. Panditi VR. Assessment of the Occurrence and Potential Risks of Antibiotics and their Metabolites in South Florida Waters Using Liquid Chromatography Tandem Mass Spectrometry. FIU Electronic Theses and Dissertations (2013). Available online at: http://digitalcommons.fiu.edu/etd/916
182. Singh G, Vajpayee P, Rani N, Amoah ID, Stenström TA, Shanker R. Exploring the potential reservoirs of non-specific TEM beta lactamase (bla(TEM)) gene in the Indo-Gangetic region: a risk assessment approach to predict health hazards. J Hazard Mater. (2016) 314:121–8. doi: 10.1016/j.jhazmat.2016.04.036
183. Al-Jassim N, Ansari MI, Harb M, Hong PY. Removal of bacterial contaminants and antibiotic resistance genes by conventional wastewater treatment processes in Saudi Arabia: is the treated wastewater safe to reuse for agricultural irrigation? Water Res. (2015) 73:277–90. doi: 10.1016/j.watres.2015.01.036
184. Verlicchi P, Galletti A, Petrovic M, Barcelo D. Hospital effluents as a source of emerging pollutants: an overview of micropollutants and sustainable treatment options. J Hydrol. (2010) 389:416–28. doi: 10.1016/j.jhydrol.2010.06.005
185. Negreanu Y. Effect of Treated Wastewater Irrigation on the Proliferation of Antibiotic Resistance in Agricultureal Soils. a Master's Degree Thesis submitted to Robert, H. Smith Faculty of Agricultural, Food and Environment of the Hebrew University of Jerusalem (2011). Available online at: http://www.agri.gov.il/download/files/NegreanuYael2011MSc_1.pdf
186. Broszat M, Nacke H, Blasi R, Siebe C, Huebner J, Daniel R, et al. Wastewater irrigation increases the abundance of potentially harmful gammaproteobacteria in soils in Mezquital Valley, Mexico. Appl Environ Microbiol. (2014) 80:5282–91. doi: 10.1128/AEM.01295-14
187. Andreozzi R, Canterino M, Marotta R, Paxeus N. Antibiotic removal from wastewaters: the ozonation of amoxicillin. J Hazardous Mater. (2005) 122, 243–250. doi: 10.1016/j.jhazmat.2005.03.004
188. Kim S, Eichhorn P, Jensen JN, Weber AS, Aga DS. Removal of antibiotics in wastewater: effect of hydraulic and solid retention times on the fate of tetracycline in the activated sludge process. Environ Sci Technol. (2005) 39:5816–23. doi: 10.1021/es050006u
189. Zorita S, Mårtensson L, Mathiasson L. Occurrence and removal of pharmaceuticals in a municipal sewage treatment system in the south of Sweden. Sci Total Environ. (2009) 407:2760–70. doi: 10.1016/j.scitotenv.2008.12.030
190. Karthikeyan KG, Meyer MT. Occurrence of antibiotics in wastewater treatment facilities in Wisconsin, USA. Sci Total Environ. (2006) 361:196–207. doi: 10.1016/j.scitotenv.2005.06.030
191. Xiao Y, Chang H, Jia A, Hu JY. Trace analysis of quinolone and fluoroquinolone antibiotics from wastewaters by liquid chromatography-electrospray tandem mass spectrometry. J Chromatogr A (2008) 1214:100–8. doi: 10.1016/j.chroma.2008.10.090
192. Zhou H, Liu W, Xu L, Deng L, Deng Q, Zhuo J, et al. Spread of Neisseria meningitidis serogroup W clone, China. Emerging infectious diseases (2013) 19:1496–9. doi: 10.3201/eid1909.130160
193. Gobel A, McArdell CS, Joss A, Siegrist H, Giger W. Fate of sulfonamides, macrolides, and trimethoprim in different wastewater treatment technologies. Sci Total Environ. (2007) 372:361–71. doi: 10.1016/j.scitotenv.2006.07.039
194. Xu W, Zhang G, Li X, Zou S, Li P, Hu Z, et al. Occurrence and elimination of antibiotics at four sewage treatment plants in the Pearl River Delta (PRD), South China. Water Res. (2007) 41:4526–34. doi: 10.1016/j.watres.2007.06.023
195. Thiele-Bruhn S, Peters D. Photodegradation of pharmaceutical antibiotics on slurry and soil surfaces. Landbauforschung Völkenrode (2007) 57:13–23.
196. Pan M, Chu LM. Phytotoxicity of veterinary antibiotics to seed germination and root elongation of crops. Ecotoxicol Environ Saf. (2016) 126:228–37. doi: 10.1016/j.ecoenv.2015.12.027
197. Kay PA, Blackwell PA, Boxall BA. Fate of veterinary antibiotics in a macroporous tile drained clay soil. Environ Toxicol Chem. (2004) 23:1136–44.
198. Gatica J, Cytryn E. Impact of treated wastewater irrigation on antibiotic resistance in the soil microbiome. Environ Sci Pollut Res. (2013) 20:3529–38. doi: 10.1007/s11356-013-1505-4
199. Jechalke S, Broszat M, Lang F, Siebe C, Smalla K, Grohmann E. Effects of 100 years wastewater irrigation on resistance genes, class 1 integrons and IncP-1 plasmids in Mexican soil. Front Microbiol. (2015) 6:163. doi: 10.3389/fmicb.2015.00163
200. Hara-Kudo Y, Konuma H, Iwaki M, Kasuga F, Sugita-Konishi Y, Ito Y, et al. Potential hazard of radish sprouts as a vehicle of E. coli O157:H7. J Food Prot. (1997) 60:1125–7. doi: 10.4315/0362-028X-60.9.1125
201. Itoh Y, Sugita-Konishi Y, Kasuga F, Iwaki M, Hara-Kudo Y, Saito N, et al. Enterohemorrhagic Escherichia coli 0157:H7 present in radish sprouts. Appl Environ Microbiol. (1998) 64:1532–5.
202. Gandhi M, Golding S, Yaron S, Matthews KR. Use of green fluorescent protein expressing Salmonella Stanley to investigate survival, spatial location, and control on alfalfa sprouts. J Food Prot. (2001) 64:1891–8. doi: 10.4315/0362-028X-64.12.1891
203. Warriner K, Spaniolas S, Dickinson M, Wright C, Waites WM. Internalization of bioluminescent Escherichia coli and Salmonella montevideo in growing bean sprouts. J Appl Microbiol. (2003) 95:719–27. doi: 10.1046/j.1365-2672.2003.02037.x
204. Delaquis P, Bach S, Dinu LD. Behavior of E. coli O157:H7 in leafy vegetables. J Food Prot. (2007) 70:1966–74. doi: 10.4315/0362-028X-70.8.1966
205. Adegoke AA, Okoh AI. Antibiogram of Stenotrophomonas maltophilia isolated from nkonkobe municipality, Eastern Cape Province, South Africa. Jundishapur J Microbiol. (2015) 8:e13975. doi: 10.5812/jjm.13975
206. Stenström TA, Okoh AI, Adegoke AA. Antibiogram of Environmental Isolates of Acinetobacter calcoaceticus from Nkonkobe Municipality, South Africa. Fresenius Environ Bull. (2016) 25:3059–65.
207. Adegoke AA, Mvuyo T, Okoh AI. Ubiquitous Acinetobacter species as beneficial commensals but gradually being emboldened with antibiotic resistance genes. J Basic Microbiol. (2012) 52:620–7. doi: 10.1002/jobm.201100323
208. Carey SA, Goldstein RE, Gibbs SG, Claye E, He X, Sapkota AR. Occurrence of vancomycin-resistant and -susceptible Enterococcus spp. in reclaimed water used for spray irrigation. Environ Res. (2016) 147:350–5. doi: 10.1016/j.envres.2016.02.030
209. Okoh AI, Adegoke AA, Adesemoye OO, Babalola OO, Igbinosa INH, Aghdasi F. Escherichia coli, a beneficial Bug, but a dynamic Threat to Public Health: Call to Caution. J Pure Appl Microbiol. (2012) 6:1069–85.
210. Hillis DG, Fletcher J, Solomon KR, Sibley PK. Effects of ten antibiotics on seed germination and root elongation in three plant species. Arch Environ Contam Toxicol. (2011) 60:220–32. doi: 10.1007/s00244-010-9624-0
211. Wei X, Wu SC, Nie XP, Yediler A, Wong MH. The effects of residual tetracycline on soil enzymatic activities and plant growth. J Environ Sci Health Part B. (2009) 44:461–71. doi: 10.1080/03601230902935139
212. Yao JH, Niu DK, Li ZJ, Liang YC, Zhang SQ. Effects of antibiotics oxytetracycline on soil enzyme activities and microbial biomass in wheat rhizosphere. Sci Agric Sin. (2010) 43:721–8.
213. Finley RL, Collignon P, Larsson DGJ, McEwen SA, Li XZ, Gaze WH, et al. The scourge of antibiotic resistance: the important role of the environment. Clin Infect Dis. (2013) 57:704–10. doi: 10.1093/cid/cit355
214. Zhang S, Gu J, Wang C, Jiao S, He Z, Han B. Characterization of antibiotics and antibiotic resistance genes on an ecological farm system. J Chem. (2015). 8:526143. doi: 10.1155/2015/526143
215. Adegoke AA, Awolusi OO, Stenström TA. Organic fertilizers: public health intricacies. In: Larramendy M, editor. Organic Fertilizers—From Basic Concepts to Applied Outcomes. Vienna: INTECH (2016).
216. Bouissou-Schurtz C, Houeto P, Guerbet M, Bachelot M, Casellas C, Mauclaire AC, et al. Ecological risk assessment of the presence of pharmaceutical residues in a French national water survey. Regul Toxicol Pharm. (2014) 69:296–303. doi: 10.1016/j.yrtph.2014.04.006
217. Nannou CI, Kosma CI, Albanis TA. Occurrence of pharmaceuticals in surface waters: analytical method development and environmental risk assessment. Inter J Environ An Chem. (2015) 95:1242–62. doi: 10.1080/03067319.2015.1085520
218. Liu X, Steele JC, Meng XZ. Usage, residue, and human health risk of antibiotics in Chinese aquaculture: a review. Environ Pollut. (2017) 223:161–9. doi: 10.1016/j.envpol.2017.01.003
219. Peng X, Ou W, Wang C, Wang Z, Huang Q, Jin J, et al. Occurrence and ecological potential of pharmaceuticals and personal care products in groundwater and reservoirs in the vicinity of municipal landfills in China. Sci Total Environ. (2014) 490:889–98. doi: 10.1016/j.scitotenv.2014.05.068
220. EMA. European Medicine Agency Guideline on the Environmental Risk Assessment of Medicinal Products for Human Use 2006 (EMEA/CHMP/SWP/4447/00) (2006). Available online at: http://www.ema.europa.eu/ema/pages/includes/document/open_document.jsp?WebContentId=WC500003978
221. FASS. Environment Classification of Pharmaceuticals. (2012). Avaialble online at: http://www.fass.se: guidance for pharmaceutical companies. (Accessed June 20, 2017).
222. Isidori M, Lavorgna M, Nardelli A, Pascarella L, Parrella A. Toxic and genotoxic evaluation of six antibiotics on non-target organisms. Sci Total Environ. (2005) 346:87–98. doi: 10.1016/j.scitotenv.2004.11.017
223. Hernando MD, Mezcua M, Fernandez-Alba AR, Barcelo D. Environmental risk assessment of pharmaceutical residues in wastewater effluents, surface waters and sediments. Talanta (2006) 69:334–42. doi: 10.1016/j.talanta.2005.09.037
224. Gonzalez-Pleiter M, Gonzalo S, Rodea-Palomares I, Leganes F, Rosal R, Boltes K, et al. Toxicity of five antibiotics and their mixtures towards photosynthetic aquatic organisms: Implications for environmental risk assessment. Water Res. (2013) 47:2050–64. doi: 10.1016/j.watres.2013.01.020
225. Halling-Sorensen B, Lutzhoft HCH, Andersen HR, Ingerslev F. Environmental risk assessment of antibiotics: comparison of mecillinam, trimethoprim and ciprofloxacin. J Antimicrob Chemother. (2000) 46(Suppl. 1):53–8. doi: 10.1093/jac/46.suppl_1.53
226. Lim SJ, Jang E, Lee SH, Yoo BH, Kim SK, Kim TH. Antibiotic resistance in bacteria isolated from freshwater aquacultures and prediction of the persistence and toxicity of antimicrobials in the aquatic environment. J Environ Sci Health B (2013) 48:495–504. doi: 10.1080/03601234.2013.761911
227. World Health Organization (WHO). Sanitation Safety Planning: Manual for Safe Use and Disposal of Wastewater, Greywater and Excreta. Geneva: World Health Organization (2015).
228. Keraita B, Konradsen F, Drechsel P, Abaidoo RC. Reducing microbial contamination on wastewater-irrigated lettuce by cessation of irrigation before harvesting, Trop. Med Inter Health (2007) 12:8–14. doi: 10.1111/j.1365-3156.2007.01936.x
229. Seidu R, Sjølander I, Abubakari A, Amoah D, Larbi JA, Stenström TA. Modeling the die-off of E. coli and Ascaris in wastewater-irrigated vegetables: implications for microbial health risk reduction associated with irrigation cessation. Water Sci Technol. (2013) 68:1013–21. doi: 10.2166/wst.2013.335
230. Oron G, Gillerman L, Lael A, Manor Y, Braude E, Bick A. Minimizing health risks during secondary effluent application via subsurface drip irrigation. Water Sci Technol. (2010) 62:2330–7. doi: 10.2166/wst.2010.502
231. Beaudequin D, Harden F, Roiko A, Mengersen K. Utility of Bayesian networks in QMRA-based evaluation of risk reduction options for recycled water. Sci Total Environ. (2016) 541:1393–409. doi: 10.1016/j.scitotenv.2015.10.030
232. Kobayashi N, Oshiki M, Ito T, Segawa T, Hatamoto M, Kato T, et al. Removal of human pathogenic viruses in a down-flow hanging sponge (DHS) reactor treating municipal wastewater and health risks associated with utilization of the effluent for agricultural irrigation. Water Res. (2017) 110:389–98. doi: 10.1016/j.watres.2016.10.054
233. Chen H, Zhang M. Effects of advanced treatment systems on the removal of antibiotic resistance genes in wastewater treatment plants from Hangzhou, China. Environ Sci Technol. (2013) 47:8157–63. doi: 10.1021/es401091y
234. Derakhshan Z, Mokhtari M, Babaei F, Ahmadi RM, Ehranpoush MH, Faramarzian M. Removal methods of antibiotic compounds from aqueous environments–a review. J Environ Health Sustain Dev. (2016) 1:43–62.
235. Toscano A, Hellio C, Marzo A, Milani M, Lebret K, Cirelli GL, et al. Removal efficiency of a constructed wetland combined with ultrasound and UV devices for wastewater reuse in agriculture. Environ Technol. (2013) 34:2327–36. doi: 10.1080/09593330.2013.767284
236. World Health Organization (WHO). Guidelines for the Safe Use of Wastewater, Excreta and Greywater: Wastewater Use in Agriculture – Volume 2. Geneva: World Health Organization (2006c).
237. Aiello R, Cirelli GL, Consoli S, Licciardello F, Toscano A. Risk assessment of treated municipal wastewater reuse in Sicily. Water Sci Technol. (2013) 67:89–98. doi: 10.2166/wst.2012.535
238. Castorina A, Consoli S, Barbagallo S, Branca F, Farag A, Licciardello F, et al. Assessing environmental impacts of constructed wetland effluents for vegetable crop irrigation. Int J Phytoremediat. (2016) 18:626–33. doi: 10.1080/15226514.2015.1086298
Keywords: epidemiological evidence, health risk, epidemics, exposure, antibiotics, pathogens, wastewater reuse
Citation: Adegoke AA, Amoah ID, Stenström TA, Verbyla ME and Mihelcic JR (2018) Epidemiological Evidence and Health Risks Associated With Agricultural Reuse of Partially Treated and Untreated Wastewater: A Review. Front. Public Health 6:337. doi: 10.3389/fpubh.2018.00337
Received: 29 March 2018; Accepted: 01 November 2018;
Published: 06 December 2018.
Edited by:
Erin Katherine Lipp, University of Georgia, United StatesReviewed by:
Amy Rebecca Sapkota, University of Maryland, Baltimore, United StatesCopyright © 2018 Adegoke, Amoah, Stenström, Verbyla and Mihelcic. This is an open-access article distributed under the terms of the Creative Commons Attribution License (CC BY). The use, distribution or reproduction in other forums is permitted, provided the original author(s) and the copyright owner(s) are credited and that the original publication in this journal is cited, in accordance with accepted academic practice. No use, distribution or reproduction is permitted which does not comply with these terms.
*Correspondence: Isaac D. Amoah, YW1vYWhraWRAZ21haWwuY29t
Disclaimer: All claims expressed in this article are solely those of the authors and do not necessarily represent those of their affiliated organizations, or those of the publisher, the editors and the reviewers. Any product that may be evaluated in this article or claim that may be made by its manufacturer is not guaranteed or endorsed by the publisher.
Research integrity at Frontiers
Learn more about the work of our research integrity team to safeguard the quality of each article we publish.