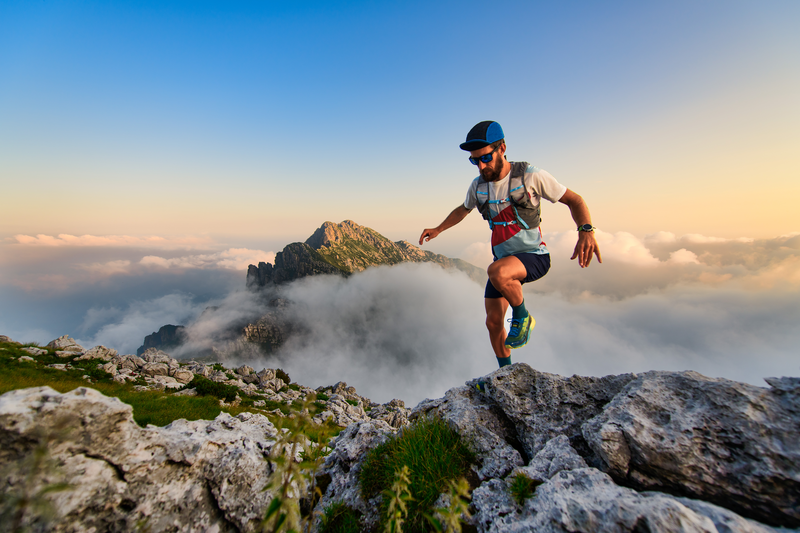
94% of researchers rate our articles as excellent or good
Learn more about the work of our research integrity team to safeguard the quality of each article we publish.
Find out more
ORIGINAL RESEARCH article
Front. Public Health , 27 November 2018
Sec. Environmental Health and Exposome
Volume 6 - 2018 | https://doi.org/10.3389/fpubh.2018.00327
This article is part of the Research Topic Exposure to Endocrine-Disrupting Chemicals and Cardiometabolic Disease: A Developmental Origins Approach View all 5 articles
Few studies have investigated longitudinal associations between early life phthalate exposure and subsequent obesity and cardiovascular risks in children with inconsistent results. We aimed to evaluate the associations between phthalate exposure during gestation and childhood with offspring obesity and cardiometabolic risk factors in 500 mother-child pairs from the Rhea pregnancy cohort in Crete, Greece. Seven phthalate metabolites [monoethyl phthalate (MEP), mono-n-butyl phthalate (MnBP), mono-isobutyl phthalate (MiBP), monobenzyl phthalate (MBzP), mono(2-ethylhexyl) phthalate (MEHP), mono(2-ethyl-5-hydroxyhexyl) phthalate (MEHHP), and mono(2-ethyl-5-oxohexyl) phthalate (MEOHP)] were quantified in spot urine samples collected from mothers (1st trimester) and their children at 4 years of age. We calculated the molar sum of DEHP metabolites (MEHP, MEHHP, MEOHP). We measured child weight, height, waist circumference, skinfold thicknesses, blood pressure (BP), and lipids at 4 and 6 years and leptin, adiponectin, and C-reactive protein at 4 years. We used generalized estimating equations to examine associations at each age and tested for interaction by sex. Child exposure to phthalate metabolites was associated with lower BMI z-scores in boys and higher BMI z-scores in girls. Each 10-fold increase in ΣDEHP was associated with a change in waist circumference of −2.6 cm (95% CI: −4.72, −0.48) in boys vs. 2.14 cm (95% CI: −0.14, 4.43) in girls (p-sex interaction = 0.003) and a change in waist-to-height ratio of −0.01 (95% CI: −0.03, 0.01) in boys vs. 0.02 (95% CI: 0.01, 0.04) in girls (p-sex interaction = 0.006). Phthalate metabolite concentrations at age 4 were negatively associated with systolic and diastolic blood pressure. MEP was associated with lower systolic BP z-scores (adj. β = −0.22; 95% CI: −0.36, −0.08) at 4 years. MnBP and MBzP were associated with lower diastolic BP z-scores (adj. β = −0.13; 95%CI: −0.23, −0.04, and adj. β = −0.11; 95% CI: −0.21, −0.01, respectively). A 10-fold increase in MiBP was associated with 4.4% higher total cholesterol levels (95% CI: 0.2, 8.7). Prenatal phthalate exposure was not consistently associated with child adiposity and cardiometabolic measures. Our findings suggest that early life phthalate exposure may affect child growth and adiposity in a sex-specific manner and depends on the timing of exposure.
Childhood obesity is a risk factor for obesity-related health outcomes such as cardiovascular and other chronic diseases later in life and one of the most common public health problems and challenges globally. The global prevalence of childhood overweight and obesity has increased over the last three decades to 124 million in 2016 (1) and if current trends continue, the World Health Organization (WHO) predicts that 70 million young children will be overweight or obese by 2025 (2). In Greece, the leading country in the childhood obesity epidemic worldwide, recent figures show that more than 40% of children at the age of 5–17 years are overweight or obese (3). Obesity and associated risk factors, such as high blood pressure and dyslipidemia, in childhood can induce changes in metabolism and contribute to the development of atherosclerosis in adulthood (4). Diet and physical inactivity are recognized causes, but exposure to environmental chemicals may also contribute to the pathogenesis of obesity and related cardiometabolic disorders (5, 6). Obesogens-chemicals that inappropriately regulate and promote lipid accumulation and adipogenesis-may contribute to obesity development, especially when exposure occurs during pregnancy and early life (7–9).
Phthalates, or diesters of phthalic acid, are a class of man-made chemicals used in a variety of common consumer and industrial products. Low-molecular weight phthalates (LMWP) are used as solvents and are typically found in medications and personal care items such as shampoos, deodorants, and lotions, while high-molecular weight phthalates (HMWP) are used in the manufacturing of flexible plastics for purposes such as vinyl flooring, adhesives, medical devices, and food packaging (10–12). Exposure to phthalates is almost ubiquitous (13) and may occur through dermal absorption, inhalation, or ingestion (14). Moreover, detectable levels of phthalate metabolites have been found in amniotic fluids and cord blood, indicating fetal exposure (12). In the Rhea mother-child cohort in Greece, Myridakis et al. found that daily intake of phthalate esters, calculated for 4 year old children, was lower than the corresponding daily intake for 2.5 year old children and that phthalate esters generally were assigned to combined exposure from plastic and diethyl phthalate from personal hygiene products/cosmetics (15, 16).
Phthalates are endocrine-disrupting chemicals with anti-androgenic and weakly estrogenic properties (17) and evidence from experimental studies suggests that phthalates may influence obesity through several mechanisms, including antithyroid hormone activities, and/or activation of peroxisome proliferator-activated receptors (PPARs), and epigenetic modulation (14, 18).
Results from cross-sectional studies in adults and children show that higher urinary phthalates concentrations are positively associated with adiposity and cardiometabolic markers (19–23). However, only a few longitudinal studies have examined the associations of early-life exposure to phthalate metabolites with childhood growth and obesity (24–32) and these studies have shown inconsistent results and sex-specific associations. In utero exposures were found to be associated with decreased BMI z-scores only in girls aged 4–7 years (25), or only in boys aged 4–7 years old (28, 29). A study in France that examined only boys found that prenatal phthalate exposure was associated with increased BMI at age 5 (26). Additionally, a US study that examined only girls found that exposure to phthalates at ages 6–8 were associated with a predicted decrease in BMI from the ages of 7–13 (31). Few studies have examined the effects of early life phthalate exposure on offspring cardiovascular traits other than adiposity. Valvi et al. (28) reported that prenatal exposure to phthalates was associated with lower systolic blood pressure z-scores at 4–7 years of age in girls but not in boys and Perng et al. (33) reported that concurrent exposure to phthalates was associated with lower cholesterol levels at 8–14 years of age.
In the present study, using data from the Rhea birth cohort, we assessed whether urinary phthalate metabolite concentrations in pregnant women and their children in early childhood were associated with measures of adiposity and a full range of cardiometabolic traits at ages 4–6 years and evaluated differences by child's sex.
The present study is part of the Rhea Study, a prospective mother-child cohort examining a population sample of pregnant women and their children at the prefecture of Heraklion, Crete, Greece. Methods are described in detail elsewhere (34). Briefly, women (Greek and immigrants) who became pregnant during a period of 1 year starting in February 2007 were asked to participate in the study. The first contact was made at the time of the first comprehensive ultrasound examination (mean ± SD 11.96 ± 1.49 weeks) and several contacts followed (6th month of pregnancy, at birth, 9 months, 1st year, 4 and 6 years after birth). To be eligible for inclusion in the study, women had to have a good understanding of the Greek language and be older than 16 years of age. The study was approved by the ethics committee of the University Hospital in Heraklion, Crete, Greece, and all participants provided written informed consent after complete description of the study.
Of 1363 singleton live births in the Rhea study, phthalate concentrations were measured in spot urine samples collected in the first trimester of pregnancy from 260 mothers and 500 children at 4 years of age; 500 children had at least one measure of BMI between 4 and 6 years of age.
All spot urine samples were collected in sterile, polypropylene urine cups, aliquoted in 4 ml cryotube vials (Thermo Fisher Scientific, USA) and stored at −80°C. Analyses were performed at the Environmental Chemical Processes Laboratory (ECPL) in the Department of Chemistry of the University of Crete. An aliquot of each urine sample (1 mL) was analyzed for the determination of the following 7 phthalate metabolites, using a previously described analytical protocol (35): monoethyl phthalate (MEP), mono-n-butyl phthalate (MnBP), mono-isobutyl phthalate (MiBP), monobenzyl phthalate (MBzP), mono(2-ethylhexyl) phthalate (MEHP), mono(2-ethyl-5-hydroxyhexyl) phthalate (MEHHP), and mono(2-ethyl-5-oxohexyl) phthalate (MEOHP). Samples exceeding the upper limit of linearity were reanalyzed, diluted with nanopure water. Two quality control samples (spiked pooled urine) and two blank samples (synthetic urine) were analyzed with every forty six (46) urine samples. The amount of each sample was quantified by the standard curve performed in each assay. All samples were measured in duplicates. We calculated the molar sums of ΣDEHP metabolites (MEHP, MEHHP, and MEOHP) by dividing metabolite concentrations by their molecular weight (MW) and summing across. Method limit of detection (LOD) ranged from 0.01 to 2.5 ng/mL and samples below LOD were assigned the value of LOD/√2. To account for urine dilution, a second aliquot of 0.5 mL urine was analyzed for creatinine concentration using the OLYMPUS 2700 immunoassay system (Beckman Coulter, USA), and phthalate metabolites concentrations were divided by urinary creatinine levels (i.e., creatinine-adjusted concentrations, hereafter). All creatinine-adjusted concentrations were log10 transformed to obtain normal distributions, as the original distributions were right skewed.
At 4 and 6 years, child anthropometry measures, including weight and length/height, waist circumference and skinfold thickness at four sites of the body (subscapular, triceps, suprailiac, and quadriceps) were obtained by specially trained research assistants following standard operating procedures. We calculated BMI (weight/height2) and converted raw values into sex- and age-specific standard deviation (SD) scores (z scores) by using internally generated growth reference curves (36). We analyzed child BMI z-score as a continuous outcome and in categories of overweight/obesity at 4 and 6 years according to the BMI cutoff points for sex and age proposed by the International Obesity Task Force (IOTF) definitions (37). The sum of the four aforementioned skinfolds was calculated as an indicator of subcutaneous fat. We also divided child waist circumference by height to calculate the waist-to-height ratio.
At the 4 and 6 year examination, after 5 min rest in the seated position, trained research assistants measured systolic (SBP), and diastolic (DBP) blood pressure levels on the child's right arm using an automatic oscillometric device (Dinamap, Pro Care 400) with a cuff of appropriate size for arm circumference. Five measurements were made with 1 min intervals and the average of these measurements was used for analysis (38). We calculated age, sex, and height specific blood pressure SD scores. Non-fasting blood samples were collected from the children at the end of the visit in 10 mL BD gel separator vacutainers with the use of standard procedures, with samples immediately spun, separated, and frozen at −80°C. Analysis of lipids (total cholesterol and high-density lipoprotein cholesterol [HDL-C]) was performed by standard enzymatic methods (Medicon, Greece). Leptin, adiponectin, and CRP levels were measured at 4 years of age. All inter- and intra-assay coefficients of variation were <5.5%.
Descriptive analyses of the study population characteristics, exposures, and outcomes were conducted. Generalized additive models (GAMs) were applied to explore the shape of the relationships between prenatal or early-childhood phthalate metabolite concentrations and outcomes under study. Linearity was assumed if the p-gain defined as the difference in normalized deviance between the GAM model and the linear model for the same exposure and outcome was >0.10. We used generalized estimating equations (GEEs) with a Gaussian or Poisson family specification and with exchangeable correlation structure to examine the associations between phthalate metabolite concentrations and repeated continuous and binary outcomes, respectively. We included interaction terms between the exposure variable and child age at examination to explore the possibility of age-specific associations. We used linear regression analyses to test whether phthalate metabolite concentrations were associated with serum leptin, adiponectin and CRP levels at 4 years. Due to non-normal distributions, we natural log-(ln)-transformed serum leptin, adiponectin, and CRP levels at 4 years, and total and HDL cholesterol at 4 and 6 years and present results as a % difference in each of these outcomes per 10-fold increase in exposure.
For every outcome variable, we first studied the association of interest in the crude model. To control for confounding, we considered maternal and child covariates that were of a priori interest as independent predictors of child adiposity and growth or that may be related to phthalate levels: maternal pre-pregnancy BMI (kg/m2), maternal age at birth (years), parity (primiparous, multiparous), maternal educational level [low level: ≤ 9 years of mandatory schooling, medium level: >9 years of schooling up to attending post-secondary school education and high level: attending university or having a university/technical college degree], smoking during pregnancy (never, ever), gestational weight gain [GWG (kg)], ethnic origin (Greek, non-Greek), residence (urban, rural), delivery type (vaginal delivery, cesarean section), delivery hospital (private, public), marital status (married, not married), and working during pregnancy (yes, no), breastfeeding (yes, no), gestational length, sex, age at outcome assessment, time watching television at 4 and 6 years (< 30 min, 1–2 h, ≥3 h per day), and child's BMI at 4 and 6 years (for models that used cardiometabolic risk factors as outcomes). We included covariates in the final models if they were associated with both exposure and any of the outcomes at p-value < 0.2 or if they modified the coefficient for the phthalate exposure variable by >10%. To assess the potential modifying effects of child sex (male, female), we included interaction terms between the exposure variable and sex in the models and stratified the sample, on the basis of prior studies (25, 28, 29). We also performed sensitivity analysis in order to explore remaining confounding. In particular, we repeated the analyses excluding children who had been born preterm (< 37 gestational weeks) or at low birth weight (< 2,500 g). Statistical significance was defined by an alpha level of 0.10 for interaction terms and of 0.05 for all other effect estimates. Analyses were conducted using STATA software, version 13.0 (Statacorp, College Station, TX).
Maternal and child characteristics of the study population are presented in Table 1. Participating mothers were predominantly of Greek origin, had a mean (±SD) age of 29.5 (± 5.1) years at delivery, about half of them had medium educational level (52%) and were multiparous (56%). Before pregnancy, 20% of mothers were overweight, the majority of mothers (86%) initiated breastfeeding and the mean (±SD) length of breastfeeding was 4.1 (± 4.3) months. Fifty-six percent of the children included in the analysis were boys, their mean (±SD) birth weight was 3,203 (± 462.8) g and the average (±SD) gestation was 38.2 (± 1.5) weeks. Twenty four percent of the children were overweight at 4 years and 22% at 6 years.
The distribution of urinary phthalate metabolite concentrations in the pregnant women and their children is shown in Table 2. The majority of samples show detectable concentrations of creatinine-corrected metabolites, with the lowest detection in MEOHP in the mothers. The phthalate metabolite with the highest concentrations in maternal and child urine was MEP. Spearman correlations of creatinine-corrected individual phthalate metabolites were weakly to highly correlated (range 0.11–0.95) within each time period while concentrations between prenatal and child measures were not consistently correlated with each other (data not shown).
Table 2. Maternal and children urinary concentrations of phthalate metabolites (μg/g creatinine), Mother-child cohort “Rhea” in Crete, Greece.
GAMs examining the shape of the relationships of phthalate metabolite concentrations at age 4 with adiposity indicators and cardiometabolic risk factors at all ages showed no significant departures from linearity (see Figure S1 for blood pressure at age 4).
Prenatal MnBP was associated with a change in waist-to-height ratio at ages 4 to 6 years of 0.19 (95% CI: 0.11, 0.27) in boys vs. −0.01 (95% CI: −0.03, 0.01) in girls (p-sex interaction < 0.001; see Table S1). MiBP was associated with lower diastolic BP z-score overall (adj. β = −0.2; 95% CI: −0.37, −0.03) and in boys (adj. β = −0.26; 95% CI: −0.48, −0.04; Table 3). There was a marginally significant association between ΣDEHP and systolic (adj. β = −0.16; 95% CI: −0.35, 0.03) and diastolic (adj. β = −0.12; 95% CI: −0.25, 0.01) BP z-score. There was a significant interaction of prenatal MEP and age (P-interaction < 0.05); the association between MEP and HDL-C was negative at 4 years (adj. β = −5.8; 95% CI: −11.3, 0.0) and positive but not statistically significant at 6 years of age (adj. β = 2.8; 95% CI: −3.6, 9.7).
Table 3. Sex-stratified associations between prenatal urinary individual and summed ΣDEHP phthalate metabolites (log10 Transformed, in μg/g Creatinine) with lipids and blood pressure levels in children aged 4–6 years.
Child sex modified the relationships between MnBP and ΣDEHP and CRP at 4 years (Table 5). A 10-fold increase in prenatal MnBP was associated with −49.1% (95% CI: −76.7, 11.2) lower and 144.3% (95% CI: 26.0, 373.8; p-sex interaction = 0.001) higher CRP levels in boys and girls, respectively (p-sex interaction = 0.001; Table 5). Associations of prenatal ΣDEHP with CRP were negative in boys (adj. β = −22.8; 95% CI: −58.8, 44.7) and positive in girls (adj. β = 116; 95% CI: −18.9, 475.1; p-sex interaction = 0.053) but not statistically significant. There were no other associations with adiposity indicators and cardiometabolic risk factors detected from the prenatal exposure period and we did not observe any modification of associations by sex.
Overall, creatinine-adjusted phthalate metabolite concentrations at age 4 were not significantly associated with BMI z-scores at 4 to 6 years of age (Figure 1 and Table S2). However, we detected sex-specific associations with respect to postnatal exposure. Child urinary phthalate metabolite concentrations were negatively associated with BMI z-scores in boys and positively in girls at ages 4 to 6 (Figure 1). MEP and MnBP were associated with lower BMI z-scores in boys (adj. β = −0.22; 95% CI: −0.44, −0.01 and adj. β = −0.1; 95% CI: −0.35, −0.15, respectively) and with higher BMI z-scores in girls (adj. β = 0.17; 95% CI: −0.12, 0.45 and adj. β = 0.39; 95% CI: 0.11, 0.66, respectively; p-sex interaction = 0.051 and 0.010, respectively; Table S2). Each 10-fold increase in MiBP was associated with a change in BMI z-score of −0.31 (95% CI: −0.6, −0.02) in boys vs. 0.74 (95% CI: 0.37, 1.1) in girls (p-sex interaction < 0.001). Similar associations were observed for ΣDEHP (Figure 1 and Table S2). When we analyzed dichotomous outcomes, results for overweight were consistent with those for BMI z-scores (data not shown).
Figure 1. Sex-stratified associations between child urinary individual and summed ΣDEHP phthalate metabolites (log10 Transformed, in μg/g Creatinine) with child BMI z scores at 4 to 6 years of age. All models are adjusted for child sex, exact age at examination, and maternal characteristics (age at delivery, parity, education, pre-pregnancy BMI, and smoking in pregnancy).
Similarly, child urinary phthalate metabolite concentrations were negatively associated with waist circumference, sum of skinfolds and waist-to-height ratio in boys and positively in girls at age 4 and 6 (Table S2). For example, each 10-fold increase of ΣDEHP was associated with a change in waist circumference of −2.6 (95% CI: −4.72, −0.48) in boys vs. 2.14 (95% CI: −0.14, 4.43) in girls (p-sex interaction = 0.003) and a change in waist-to-height ratio of −0.01 (95% CI: −0.03, 0.01) in boys vs. 0.02 (95% CI: 0.01, 0.04) in girls (p-sex interaction = 0.006).
We observed consistent negative associations between phthalate metabolite concentrations at age 4 and systolic and diastolic blood pressure at all ages, overall and in girls and boys separately (Table 4). For example, MEP was associated with lower systolic BP z-score (adj. β = −0.22; 95% CI: −0.36, −0.08). MnBP and MBzP were associated with lower diastolic BP z-score (adj. β = −0.13; 95%CI: −0.23, −0.04 and adj. β = −0.11; 95% CI: −0.21, −0.01, respectively). A 10-fold increase in MiBP was associated with 4.4% higher total cholesterol levels (95% CI: 0.2, 8.7).
Table 4. Sex-stratified associations between child urinary individual and summed ΣDEHP phthalate metabolites (log10 Transformed, in μg/g Creatinine) with lipids and blood pressure levels in children aged 4–6 years.
Sex modified the associations between child MBzP and leptin (p-sex interaction = 0.059), and child MnBP, MBzP, and ΣDEHP and adiponectin (p-sex interaction = 0.047, 0.082, and 0.016, respectively) with negative associations in boys vs. positive in girls at 4 years, but none of the associations reached the level of statistical significance (Table 5). In contrast, among boys we observed higher CRP with increases in ΣDEHP whereas in girls the association was positive (p-sex interaction = 0.021).
Table 5. Sex-stratified associations between maternal and child urinary individual and summed ΣDEHP phthalate metabolites (log10 Transformed, in μg/g Creatinine) with leptin, adiponectin and CRP levels in children aged 4 years.
Effect estimates of the crude models for the associations between phthalate metabolite concentrations and all the obesity-related outcomes under study did not differ substantially from the final models adjusted for child and maternal characteristics (data not shown). The exclusion of preterm newborns and infants with low birth weight did not appreciably change our results (data not shown).
In this prospective cohort study we found that childhood exposure to phthalate metabolites was associated with markers of adiposity and metabolic function and many of these associations were dependent on sex. Additionally, we observed consistent negative associations between phthalate metabolite concentrations at age 4 and systolic and diastolic blood pressure at all ages, overall and in girls or boys separately. To our knowledge this study is the first to examine the relationship between exposure to phthalate metabolites measured prenatally and during childhood with repeated measures of adiposity and a full range of cardiometabolic traits in children.
We observed that child phthalate concentrations were negatively associated with BMI z-score, waist circumference, sum of skinfolds and waist-to-height ratio in boys and positively in girls at age 4 to 6. These findings are consistent with the observations by Yang et al. (27) that, in cross-sectional analyses, found a negative association of child's urine phthalate concentrations with waist circumference and sum of skinfold thicknesses in boys. However, our findings are not consistent with a US study that examined only girls and found that exposure to phthalates at ages 6–8 were associated with a predicted decrease in BMI from the ages of 7–13 (31). We did not observe statistically significant associations between prenatal phthalate metabolites and any of the examined adiposity measures in childhood, consistent with observations from US cohort, where no associations with fat mass in children aged 4–9 years were observed (24), a Mexican study that did not observe any relationships between prenatal phthalate exposure and child BMI after excluding children who had initiated puberty (27) and the HOME study from Ohio that reported no association between prenatal phthalate exposure and child adiposity at age 8. However, depending on age, sex, timing of exposure, and phthalate metabolites, other studies report discrepant findings (25, 26, 28–32). Prenatal exposure to MEP was associated with increased obesity outcomes at ages 5–12 years (30) and increased weight growth velocity at ages 2 and 4 years and increased BMI at 5 years in boys from the French EDEN cohort (26). Furthermore, in utero exposure to phthalate metabolites was associated with increased BMI and risk for overweight/obesity between 5 and 12 years of age in the US CHAMACOS study (30). In contrast, prenatal exposure to different phthalate metabolites was associated with decreased BMI z-scores only in girls aged 4–7 years (25), or only in boys aged 4 or 7 years old (28), and 5–7 years old (29).
Our results indicate that that exposure to phthalates may influence adiposity differently in boys and girls. Although still not clear, there are several potential biologic mechanisms underlying associations between phthalates and body size that may be linked to some of the previously observed sex-specific effects. The most plausible mechanism for associations, specifically for body weight and adiposity measures, is that phthalates interfere with peroxisome proliferator-activated receptors (PPARs), which are involved in the metabolism of fat, carbohydrates, and protein (18) and are key regulators of adipogenesis and energy storage, in both rodent and human cell lines (39). Phthalates can activate PPAR-γ resulting in changes in adipocyte differentiation and release of leptin and adiponectin from adipocytes (40). We found some evidence suggesting that sex also modifies the association between phthalates and adipokines. Because early childhood adiposity has been positively associated with leptin levels (41) these sexually dimorphic associations might explain the positive associations that we observed with adiposity markers in girls, however, effect heterogeneity by child sex merit further exploration in larger populations. Moreover, differences in PPAR activity by sex could potentially explain sex-specific associations (42–44). Some phthalates also have anti-androgenic and weakly estrogenic properties (17) and they have been associated with altered sexual differentiation in male rodents (45), lower testosterone levels in an vitro study using human testis (46), and decreased steroid hormone levels in adult men (47) which may explain some of the sex differences reported previously. Another potential mechanism linking phthalates to obesity is through thyroid disruption (14) and negative associations of phthalates with thyroid hormones, insulin-like growth factor I (IGF-I), and growth have been reported in children (48).
Investigations of early life phthalate exposure and cardiometabolic outcomes in humans are scant. Our results suggest that prenatal MEP exposure is associated with lower HDL cholesterol, at age 4 and postnatal MiBP exposure is associated with higher total cholesterol at 4–6 years. The only other study that examined associations of in utero and concurrent phthalate exposure with lipid profile reported that prenatal phthalate exposure was not associated with lipid profile at 8–14 years but concurrent exposure was associated with lower total and LDL cholesterol in boys (33).
Moreover, we found that exposure to DEHP metabolites during pregnancy was associated with lower systolic and diastolic BP z-scores and we observed consistent negative associations between phthalate metabolite concentrations at age 4 and systolic and diastolic blood pressure at all ages, overall and in girls or boys separately. These findings are consistent with the only other prospective study that examined prenatal phthalate exposure and blood pressure in childhood and found that ΣHMWPm and ΣLMWPm were associated with lower systolic blood pressure z-scores but only in girls at 4–7 years of age in Spanish INMA cohort (28). However, a recent cross-sectional study of children who participated in the National Health and Nutrition Examination Survey (NHANES), reported positive associations between phthalate exposure and systolic blood pressure in children 6–19 years (23).
There are some biologically plausible mechanisms linking phthalates to increased cardiovascular risk, independent of body mass effects. Laboratory studies have found that phthalate metabolites increase cytokine production (49), while biomarkers of phthalate exposure have been associated with increases in serum markers of inflammation and oxidative stress in adults (50). Recent findings suggest that phthalates may produce increases in low-grade albuminuria in children, a marker of vascular dysfunction associated with chronic kidney and CVD risks (51). Thus, further prospective follow-up of the observed associations will be required to explore whether the associations shown between phthalate exposure and blood pressure persist or reverse at later ages.
The current study has several strengths including the population-based prospective design, our ability to examine the potential effects of phthalate exposure in two critical developmental time periods (pregnancy and childhood), and our comprehensive assessment of adiposity and cardiometabolic outcomes. Limitations of this study include the possibility of exposure misclassification due to the use of a single spot-urine during pregnancy and childhood, and the potential for residual confounding, in particular with respect to unmeasured factors such as parental income or social class. Additionally, as this analysis was exploratory, we have chosen not to control for multiple comparisons, thus we cannot rule out the possibility of some false significant findings.
Our findings suggest that the effect of early life exposure to phthalate metabolite may influence postnatal growth and adiposity in a sex specific manner and depends on the timing of exposure. Additional longitudinal studies with multiple repeated phthalate measurements throughout childhood and adolescence and diverse study populations are necessary to confirm these findings.
LC, the principal investigator of the study, and MV conceived and designed the study. MV, TR and KM analyzed data and performed statistical analysis. MV interpreted the results and wrote the manuscript. AM participated in writing the manuscript. All authors made significant contributions to the interpretation of data and participated in drafting and revising the manuscript. All authors have approved the final version.
The Rhea project was financially supported by European projects (EU FP6-2003-Food-3-NewGeneris, EU FP6. STREP Hiwate, EU FP7 ENV.2007.1.2.2.2. Project No 211250 Escape, EU FP7-2008-ENV-1.2.1.4 Envirogenomarkers, EU FP7-HEALTH- 2009- single stage CHICOS, EU FP7 ENV.2008.1.2.1.6. Proposal No 226285 ENRIECO, EUFP7- HEALTH-2012 Proposal No 308333 HELIX, FP7 European Union project, No. 264357 MeDALL), and the Greek Ministry of Health (Program of Prevention of obesity and neurodevelopmental disorders in preschool children, in Heraklion district, Crete, Greece: 2011–2014; Rhea Plus: Primary Prevention Program of Environmental Risk Factors for Reproductive Health, and Child Health: 2012–15).
MV has no competing financial interests but she discloses that she receives research funding from Roche, Biogen, and NIH, outside of current study.
The remaining authors declare that the research was conducted in the absence of any commercial or financial relationships that could be construed as a potential conflict of interest.
The authors would like to thank all study participants and the doctors, nurses, midwives, and laboratory technicians who assisted with its conduct, for their generous collaboration.
The Supplementary Material for this article can be found online at: https://www.frontiersin.org/articles/10.3389/fpubh.2018.00327/full#supplementary-material
1. NCD Risk Factor Collaboration (NCD-RisC). Worldwide trends in body-mass index, underweight, overweight, and obesity from 1975 to 2016: a pooled analysis of 2416 population-based measurement studies in 128.9 million children, adolescents, and adults. Lancet (2017) 390:2627–42. doi: 10.1016/S0140-6736(17)32129-3
2. Commission on Ending Childhood Obesity. Report of the Commission on Ending Childhood Obesity. (2016) Available online at: http://www.who.int/end-childhood-obesity/en/. (Accessed Feb 21 2017).
3. IOTF 2. 2013 22/01/2015. (2015). Available online at: http://www.oecd.org/health/obesity-update.htm.
4. Raitakari OT, Juonala M, Kahonen M, Taittonen L, Laitinen T, Maki-Torkko N, et al. Cardiovascular risk factors in childhood and carotid artery intima-media thickness in adulthood: the Cardiovascular Risk in Young Finns Study. JAMA (2003) 290:2277–83. doi: 10.1001/jama.290.17.2277
5. Heindel JJ, Blumberg B, Cave M, Machtinger R, Mantovani A, Mendez MA, et al. Metabolism disrupting chemicals and metabolic disorders. Reprod Toxicol. (2017) 68:3–33. doi: 10.1016/j.reprotox.2016.10.001
6. Heindel JJ, Newbold R, Schug TT. Endocrine disruptors and obesity. Nat Rev Endocrinol. (2015) 11:653–61. doi: 10.1038/nrendo.2015.163
7. Grun F, Blumberg B. Endocrine disrupters as obesogens. Mol Cell Endocrinol. (2009) 304:19–29. doi: 10.1016/j.mce.2009.02.018
8. Tang-Peronard JL, Andersen HR, Jensen TK, Heitmann BL. Endocrine-disrupting chemicals and obesity development in humans: a review. Obes Rev. (2011) 12:622–36. doi: 10.1111/j.1467-789X.2011.00871.x
9. Romano ME, Savitz DA, Braun JM. Challenges and future directions to evaluating the association between prenatal exposure to endocrine disrupting chemicals and childhood obesity. Curr Epidemiol Rep. (2014) 1:57–66. doi: 10.1007/s40471-014-0007-3
10. Schettler T. Human exposure to phthalates via consumer products. Int J Androl. (2006) 29:134–9; discussion 181–5. doi: 10.1111/j.1365-2605.2005.00567.x
11. Meeker JD, Sathyanarayana S, Swan SH. Phthalates and other additives in plastics: human exposure and associated health outcomes. Philos Trans R Soc Lond B Biol Sci. (2009) 364:2097–113. doi: 10.1098/rstb.2008.0268
12. Braun JM, Sathyanarayana S, Hauser R. Phthalate exposure and children's health. Curr Opin Pediatr. (2013) 25:247–54. doi: 10.1097/MOP.0b013e32835e1eb6
13. Zota AR, Calafat AM, Woodruff TJ. Temporal trends in phthalate exposures: findings from the National Health and Nutrition Examination Survey, 2001-(2010). Environ Health Perspect. (2014) 122:235–41. doi: 10.1289/ehp.1306681
14. Kim SH, Park MJ. Phthalate exposure and childhood obesity. Ann Pediatr Endocrinol Metab. (2014) 19:69–75. doi: 10.6065/apem.2014.19.2.69
15. Myridakis A, Fthenou E, Balaska E, Vakinti M, Kogevinas M, Stephanou EG. Phthalate esters, parabens and bisphenol-A exposure among mothers and their children in Greece (Rhea cohort). Environ Int. (2015) 83:1–10. doi: 10.1016/j.envint.2015.05.014
16. Myridakis A, Chalkiadaki G, Fotou M, Kogevinas M, Chatzi L, Stephanou EG. Exposure of Preschool-Age Greek Children (RHEA Cohort) to Bisphenol A, Parabens, Phthalates, and Organophosphates. Environ Sci Technol. (2016) 50:932–41. doi: 10.1021/acs.est.5b03736
17. Takeuchi S, Iida M, Kobayashi S, Jin K, Matsuda T, Kojima H. Differential effects of phthalate esters on transcriptional activities via human estrogen receptors alpha and beta, and androgen receptor. Toxicology (2005) 210:223–33. doi: 10.1016/j.tox.2005.02.002
18. Desvergne B, Feige JN, Casals-Casas C. PPAR-mediated activity of phthalates: a link to the obesity epidemic? Mol Cell Endocrinol. (2009) 304:43–8. doi: 10.1016/j.mce.2009.02.017
19. Hatch EE, Nelson JW, Qureshi MM, Weinberg J, Moore LL, Singer M, et al. Association of urinary phthalate metabolite concentrations with body mass index and waist circumference: a cross-sectional study of NHANES data, 1999-(2002). Environ Health (2008) 7:27. doi: 10.1186/1476-069X-7-27
20. Trasande L, Attina TM, Sathyanarayana S, Spanier AJ, Blustein J. Race/ethnicity-specific associations of urinary phthalates with childhood body mass in a nationally representative sample. Environ Health Perspect. (2013) 121:501–6. doi: 10.1289/ehp.1205526
21. Yaghjyan L, Sites S, Ruan Y, Chang SH. Associations of urinary phthalates with body mass index, waist circumference and serum lipids among females: National Health and Nutrition Examination Survey 1999-(2004). Int J. (2015) 39:994–1000. doi: 10.1038/ijo.2015.8
22. Zhang Y, Meng X, Chen L, Li D, Zhao L, Zhao Y, et al. Age and sex-specific relationships between phthalate exposures and obesity in Chinese children at puberty. PLoS ONE (2014) 9:e104852. doi: 10.1371/journal.pone.0104852
23. Trasande L, Sathyanarayana S, Spanier AJ, Trachtman H, Attina TM, Urbina EM. Urinary phthalates are associated with higher blood pressure in childhood. J Pediatr. (2013) 163:747–53 e1. doi: 10.1016/j.jpeds.2013.03.072
24. Buckley JP, Engel SM, Mendez MA, Richardson DB, Daniels JL, Calafat AM, et al. Prenatal phthalate exposures and childhood fat mass in a New York city cohort. Environ Health Perspect. (2016) 124:507–13. doi: 10.1289/ehp.1509788
25. Buckley JP, Engel SM, Braun JM, Whyatt RM, Daniels JL, Mendez MA, et al. Prenatal phthalate exposures and body mass index among 4- to 7-year-old children: a pooled analysis. Epidemiology (2016) 27:449–58. doi: 10.1097/EDE.0000000000000436
26. Botton J, Philippat C, Calafat AM, Carles S, Charles MA, Slama R, et al. Phthalate pregnancy exposure and male offspring growth from the intra-uterine period to five years of age. Environ Res. (2016) 151:601–9. doi: 10.1016/j.envres.2016.08.033
27. Yang TC, Peterson KE, Meeker JD, Sanchez BN, Zhang Z, Cantoral A, et al. Bisphenol A and phthalates in utero and in childhood: association with child BMI z-score and adiposity. Environ Res. (2017) 156:326–33. doi: 10.1016/j.envres.2017.03.038
28. Valvi D, Casas M, Romaguera D, Monfort N, Ventura R, Martinez D, et al. Prenatal phthalate exposure and childhood growth and blood pressure: evidence from the Spanish INMA-sabadell birth cohort study. Environ Health Perspect. (2015) 123:1022–9. doi: 10.1289/ehp.1408887
29. Maresca MM, Hoepner LA, Hassoun A, Oberfield SE, Mooney SJ, Calafat AM, et al. Prenatal exposure to phthalates and childhood body size in an Urban cohort. Environ Health Perspect. (2016) 124:514–20. doi: 10.1289/ehp.1408750
30. Harley KG, Berger K, Rauch S, Kogut K, Henn BC, Calafat AM, et al. Association of prenatal urinary phthalate metabolite concentrations and childhood BMI and obesity. Pediatr Res. (2017) 82:405–15. doi: 10.1038/pr.2017.112
31. Deierlein AL, Wolff MS, Pajak A, Pinney SM, Windham GC, Galvez MP, et al. Longitudinal associations of phthalate exposures during childhood and body size measurements in young girls. Epidemiology (2016) 27:492–9. doi: 10.1097/EDE.0000000000000489
32. Shoaff J, Papandonatos GD, Calafat AM, Ye X, Chen A, Lanphear BP, et al. Early-life phthalate exposure and adiposity at 8 years of age. Environ Health Perspect. (2017) 125:097008. doi: 10.1289/EHP1022
33. Perng W, Watkins DJ, Cantoral A, Mercado-Garcia A, Meeker JD, Tellez-Rojo MM, et al. Exposure to phthalates is associated with lipid profile in peripubertal Mexican youth. Environ Res. (2017) 154:311–7. doi: 10.1016/j.envres.2017.01.033
34. Chatzi L, Leventakou V, Vafeiadi M, Koutra K, Roumeliotaki T, Chalkiadaki G, et al. Cohort-profile: the mother-child cohort in crete, Greece (Rhea Study). Int J Epidemiol. (2017) 46:1392–3k. doi: 10.1093/ije/dyx084
35. Myridakis A, Balaska E, Gkaitatzi C, Kouvarakis A, Stephanou EG. Determination and separation of bisphenol A, phthalate metabolites and structural isomers of parabens in human urine with conventional high-pressure liquid chromatography combined with electrospray ionisation tandem mass spectrometry. Anal Bioanal Chem. (2015) 407:2509–18. doi: 10.1007/s00216-015-8497-5
36. Royston P, Wright EM. A method for estimating age-specific reference intervals (‘normal ranges') based on fractional polynomials and exponential transformation. J R Stat Soc Ser A (1998) 161:79–101.
37. Cole TJ, Lobstein T. Extended international (IOTF) body mass index cut-offs for thinness, overweight and obesity. Pediatr Obes. (2012) 7:284–94. doi: 10.1111/j.2047-6310.2012.00064.x
38. Gillman MW, Cook NR. Blood pressure measurement in childhood epidemiological studies. Circulation (1995) 92:1049–57. doi: 10.1161/01.CIR.92.4.1049
39. Hurst CH, Waxman DJ. Activation of PPARalpha and PPARgamma by environmental phthalate monoesters. Toxicol Sci. (2003) 74:297–308. doi: 10.1093/toxsci/kfg145
40. Taxvig C, Dreisig K, Boberg J, Nellemann C, Schelde AB, Pedersen D, et al. Differential effects of environmental chemicals and food contaminants on adipogenesis, biomarker release and PPARgamma activation. Mol Cell Endocrinol. (2012) 361:106–15. doi: 10.1016/j.mce.2012.03.021
41. Mantzoros CS, Rifas-Shiman SL, Williams CJ, Fargnoli JL, Kelesidis T, Gillman MW. Cord blood leptin and adiponectin as predictors of adiposity in children at 3 years of age: a prospective cohort study. Pediatrics (2009) 123:682–9. doi: 10.1542/peds.2008-0343
42. Jalouli M, Carlsson L, Ameen C, Linden D, Ljungberg A, Michalik L, et al. Sex difference in hepatic peroxisome proliferator-activated receptor alpha expression: influence of pituitary and gonadal hormones. Endocrinology (2003) 144:101–9. doi: 10.1210/en.2002-220630
43. Huang Q, Chen Q. Mediating roles of PPARs in the effects of environmental chemicals on sex steroids. PPAR Res. (2017) 2017:3203161. doi: 10.1155/2017/3203161
44. Fornes D, White V, Higa R, Heinecke F, Capobianco E, Jawerbaum A. Sex-dependent changes in lipid metabolism, PPAR pathways and microRNAs that target PPARs in the fetal liver of rats with gestational diabetes. Mol Cell Endocrinol. (2018) 461:12–21. doi: 10.1016/j.mce.2017.08.004
45. Gray LE Jr, Ostby J, Furr J, Price M, Veeramachaneni DN, Parks L. Perinatal exposure to the phthalates DEHP, BBP, and DINP, but not DEP, DMP, or DOTP, alters sexual differentiation of the male rat. Toxicol Sci. (2000) 58:350–65.
46. Desdoits-Lethimonier C, Albert O, Le Bizec B, Perdu E, Zalko D, Courant F, et al. Human testis steroidogenesis is inhibited by phthalates. Hum Reprod. (2012) 27:1451–9. doi: 10.1093/humrep/des069
47. Meeker JD, Calafat AM, Hauser R. Urinary metabolites of di(2-ethylhexyl) phthalate are associated with decreased steroid hormone levels in adult men. J Androl. (2009) 30:287–97. doi: 10.2164/jandrol.108.006403
48. Boas M, Frederiksen H, Feldt-Rasmussen U, Skakkebaek NE, Hegedus L, Hilsted L, et al. Childhood exposure to phthalates: associations with thyroid function, insulin-like growth factor I, and growth. Environ Health Perspect. (2010) 118:1458–64. doi: 10.1289/ehp.0901331
49. Jepsen KF, Abildtrup A, Larsen ST. Monophthalates promote IL-6 and IL-8 production in the human epithelial cell line A549. Toxicol In Vitro (2004) 18:265–9. doi: 10.1016/j.tiv.2003.09.008
50. Ferguson KK, Loch-Caruso R, Meeker JD. Urinary phthalate metabolites in relation to biomarkers of inflammation and oxidative stress: NHANES 1999-(2006). Environ Res. (2011) 111:718–26. doi: 10.1016/j.envres.2011.02.002
Keywords: phthalates, pregnancy, children, obesity, cardiometabolic risk
Citation: Vafeiadi M, Myridakis A, Roumeliotaki T, Margetaki K, Chalkiadaki G, Dermitzaki E, Venihaki M, Sarri K, Vassilaki M, Leventakou V, Stephanou EG, Kogevinas M and Chatzi L (2018) Association of Early Life Exposure to Phthalates With Obesity and Cardiometabolic Traits in Childhood: Sex Specific Associations. Front. Public Health 6:327. doi: 10.3389/fpubh.2018.00327
Received: 05 February 2018; Accepted: 25 October 2018;
Published: 27 November 2018.
Edited by:
Wei Perng, University of Colorado, United StatesReviewed by:
Kelly Ferguson, National Institute of Environmental Health Sciences (NIEHS), United StatesCopyright © 2018 Vafeiadi, Myridakis, Roumeliotaki, Margetaki, Chalkiadaki, Dermitzaki, Venihaki, Sarri, Vassilaki, Leventakou, Stephanou, Kogevinas and Chatzi. This is an open-access article distributed under the terms of the Creative Commons Attribution License (CC BY). The use, distribution or reproduction in other forums is permitted, provided the original author(s) and the copyright owner(s) are credited and that the original publication in this journal is cited, in accordance with accepted academic practice. No use, distribution or reproduction is permitted which does not comply with these terms.
*Correspondence: Marina Vafeiadi, YmFmb21AdW9jLmdy
Disclaimer: All claims expressed in this article are solely those of the authors and do not necessarily represent those of their affiliated organizations, or those of the publisher, the editors and the reviewers. Any product that may be evaluated in this article or claim that may be made by its manufacturer is not guaranteed or endorsed by the publisher.
Research integrity at Frontiers
Learn more about the work of our research integrity team to safeguard the quality of each article we publish.